- 1Sichuan Academy of Forestry, Chengdu, China
- 2Key Laboratory of Southwest China Wildlife Conservation (China West Normal University), Ministry of Education, Nanchong, Sichuan, China
- 3Nanchong Vocational College of Culture and Tourism, Langzhong, Sichuan, China
- 4Biocontrol and Molecular Ecology, Manaaki Whenua Landcare Research, Lincoln, New Zealand
Natural and artificial approaches are the mainly management strategy used in degraded lands restoration, while few studies examine the effect of the two strategies on soil nutrient properties in an earthquake-triggered degraded ecosystem. We compared soil chemical traits and major nutrient stoichiometry from areas following landslides that had undergone natural restoration (D. NR.) and artificial restoration (D. AR.), as well as neighboring undisturbed areas (Und.), following the 2017 magnitude 7.0 earthquake in Jiuzhaigou, eastern Qinghai-Tibet Plateau. The results showed that soil organic carbon (C), total nitrogen (N), available nitrogen (AN), available phosphorus (AP), exchangeable calcium (eCa), exchangeable magnesium (eMg), C/P, C/K, N/P, N/K, P/K, cation exchange capacity, and vegetation cover in landslides of D. NR. and D. AR. were lower than those in Und. land, while their pH and total potassium (K) concentration were higher. Compared to D. NR., most of these traits were higher in D. AR., except for the C/N, which was reduced in D. AR. Soil C was positively related to AN, C/K, N/P, N/K, P/K in each land type, while in D. NR., it was not related to N, AP, AK, eCa, eMg, C/N, although it was negatively related to P and K concentration. The findings demonstrated that vegetation restoration strategies could affect not only soil nutrient content but also the macronutrient stoichiometry (N, P, K). Furthermore, artificial restoration projects can enhance soil nutrient concentration and facilitate vegetation recovery more quickly than natural restoration, which is primarily driven by soil N rather than P or K.
Introduction
Soil nutrients play a crucial role in plant growth and development, and are essential components of the terrestrial ecosystem. Soil organic carbon, for instance, is an indicator of soil quality, influencing the soil biochemical cycle (Duval et al., 2013; Nie et al., 2023). Soil nitrogen, phosphorus, and potassium directly impact leaf photosynthesis and are critical determinants of forest productivity (Wright et al., 2011; Santiago et al., 2012; Shen et al., 2020). In addition to soil nutrient content, the stoichiometry of major elements has gained recognition as a crucial ecological driver of plant growth, distribution, and ecosystem structure and function (Sterner and Elser, 2002). For example, ratios of carbon to nitrogen and carbon to phosphorus are used as indicators of nutrient cycling and soil quality, while nitrogen to phosphorus ratio has emerged as an indicator of plant growth limitation (Fan et al., 2015; Scharler et al., 2015; Di-Palo and Fornara, 2017; Liu et al., 2020). Additionally, exchangeable cations (e.g., Ca2+, Mg2+, Na+) can impact soil carbon accumulation and vegetation dynamics (Berthrong, et al., 2009; Hu and Lan, 2020). Studies investigating soil nutrients and their stoichiometry across different vegetation types can provide insights into controlling vegetation dynamics, which can inform the reconstruction of degraded terrestrial ecosystems (Wardle et al., 2004; Di-Palo and Fornara, 2017; Guo et al., 2019; Su et al., 2019; Liu et al., 2020).
Powerful earthquakes that occur in mountainous regions can trigger mountain collapses and landslides, leading to vegetation destruction and changes in soil conditions (Cui et al., 2012; Lin et al., 2017; Lebrato et al., 2019). These disturbances often result in the loss of nutrient-rich soil (Guo et al., 2013; Lin et al., 2017), which may degrade ecosystem productivity and stability (Allen et al., 2020; Thomsen et al., 2020). For instance, studies have shown that the magnitude 7.0 Wenchuan earthquake in 2008 resulted in soil organic carbon loss and ecological degradation (Cheng et al., 2012; Cui et al., 2012; Wu et al., 2012; Zhang et al., 2014). The co-degradation of the vegetation and soil system induced by the earthquake increased the difficulty of ecosystem function restoration (Cui et al., 2012). Although decreased nutrient contents in soils following earthquake-induced landslides have been observed, fewer studies have concurrently addressed soil nutrient and stoichiometry changes in the post-earthquake period (Lin et al., 2017).
In recent decades, both approaches by natural restoration (also known as natural regeneration or passive restoration) and artificial restoration (also known as planted restoration or active restoration)have been used to restore severely degraded lands (Palmer et al., 1997; Stokes et al., 2010; Wang et al., 2021). However, studies have shown that outcomes of artificial restoration on soil quality and nutrients can differ from those of natural restoration (Liu et al., 2019; Zhang et al., 2019), as well as soil nutrient stoichiometry (Deng et al., 2016). Although artificial restoration can confer advantages by quickly improving soil quality and vegetation recovery compared to natural restoration (Li et al., 2014; Zhang et al., 2014; Deng et al., 2016; Jiang et al., 2021), it may result in less soil biodiversity than natural restoration (Wang et al., 2019). However, these discrepancies may be related to different site soils and disturbance types. Therefore, understanding how changes in soil nutrient properties respond to natural and artificial restorations following earthquake-induced landslides remains an important area of research (Lin et al., 2017).
Jiuzhaigou, located in the eastern Qinghai-Tibet plateau, is a UNESCO World Heritage Site, a World Biosphere Reserve, and a national park, recognized for its global biodiversity conservation hotspot, including the endangered giant panda and golden snub-nosed monkey, approximately 140 bird species, and valuable ecotourism industry (Li et al., 2005; Wang et al., 2018). However, the local landscape is a typical karst ecosystem with a rocky and thin soil layer, and significant soil erosion has resulted in fragile karst landscapes (Xu et al., 2016; Xia et al., 2020). On 8 August 2017, a magnitude 7.0 earthquake in Jiuzhaigou County caused significant landscape degradation and loss of life (Hu et al., 2019). The earthquake triggered numerous landslides, destroying the original vegetation and resulting in changes to soil physical and chemical properties that caused a series of ecological and environmental problems (Wang et al., 2018; Zhao et al., 2018). Therefore, understanding how to restore vegetation following landsides is essential for local vegetation, biodiversity conservation, and ecosystem functioning. Zhang et al. (2019) found that vegetation restoration practices could greatly influence soil quality in karst landscapes. The Chinese government has conducted some landslide restoration projects since 2018, but the efficacy of such projects on recovery processes is rarely evaluated.
In this study, we aimed to compare the differences in soil nutrient contents and C, N, P, K stoichiometry across different land types (areas post-landslide with natural restoration and artificial restoration, as well as nearby areas unaffected by the landslide) to answer the following questions: 1) How do soil nutrient content and major nutrient element stoichiometry in the two types of post-landslide vegetation soil differ from undestroyed vegetation soil? 2) Does artificial restoration benefit soil accumulation more than natural restoration in Jiuzhaigou? 3) How do correlations between soil nutrient contentsor stoichiometry differ across land types in Jiuzhaigou? Our findings can provide a guide for efficient decision-making and policy planning for post-earthquake ecosystem management and recovery.
Material and methods
Study sites
The study was conducted in Jiuzhaigou National Nature Reserve in August 2019 (Figure 1; Table 1). The sites located in the lower middle of valley are the core of the natural reserves. The annual average temperature is 7.1°C, and the annual average precipitation ranges from 696.6 to 957.5 mm. The natural vegetation is a secondary forest, which was from a restoration effort in the 1960s. The natural vegetation is temperate broadleaf and mixed forest, and the dominant tree species are Picea asperata, Abies ernestii, and Betula albosinensis (Xu et al., 2016). The main surface soil of these sites is dark brown soil. The magnitude 7.0 earthquake triggered many landsides that removed much of the local surface vegetation. In March 2018, several areas affected by these landslides were included in artificial restoration programs that used slope cutting, masonry retaining walls, bamboo fencing, terraced sandbag engineering to reconstruct the landscape and then planted native grass species (mainly Avena sativa), while other affected areas were not restored (Figure 1).
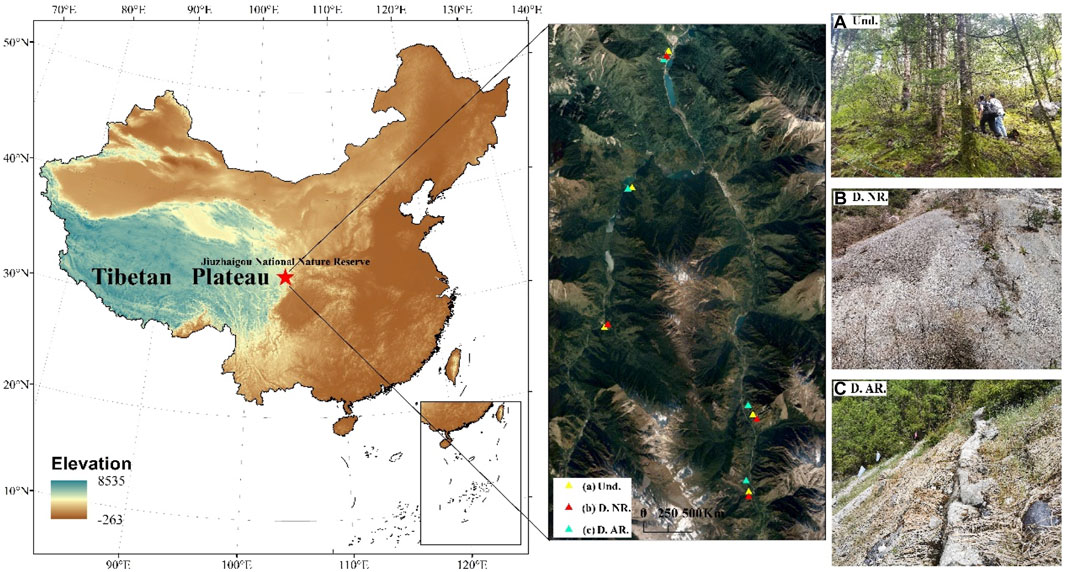
FIGURE 1. Sample sites in Jiuzhaigou. Und.: undestroyed land; D. NR: destroyed land with natural restoration; D. AR: destroyed land with artificial restoration.
Sampling design
In September 2019, two growing seasons following the artificial restoration, we selected four areas that were affected by the landslides (D. AR. that received artificial restoration and D. NR. that were left untouched and therefore underwent natural restoration), as well as five neighboring areas that were unaffected by the landslides in 2017 (Figure 1; Table 1). These unaffected sites served as controls. The average slope of all sites was about 40° (the difference of the landside slope was less than 5°) and the aspect of land in each site was less than 10° to minimize potential slope and aspect effects on soil nutrients (Jiang et al., 2021). At each site, we conducted a 10 m by 10 m sample at the middle of slope and estimated the coverage of vegetation by digital images according to Zhou et al. (Zhou et al., 1998).
Five soil samples were collected from each 10 m by 10 m sample plot. Each soil sample from surface soil (0–10 cm) collected at least four soil subsamples across the whole plot and mixed well. Leaf litter was excluded before each soil collection.
Soil analyses
The collected mineral soil samples were air-dried, ground, and passed through a 2-mm sieve. Soil pH value was measured at a 1:2.5 soil: solution ratio (in deionized water) by using a pH electrode. Soil organic carbon (C) was analyzed following wet digestion with H2SO4–K2Cr2O7 solution; total nitrogen (N), total phosphorus (P), available nitrogen (AN), and available phosphorus (AP) were determined using by Auto Discrete Analyzers (Cleverchem200, Dechem-Tech Germany). Total potassium (K) and available potassium (AK) were determined in flame atomic absorption spectrometry (F-AAS, Shimadzu AA-7000, Japan). We determined the potential cation exchange capacity (CEC) and exchangeable calcium (eCa) and exchangeable magnesium (eMg) by using the leaching method with 1 M ammonium acetate at pH 7. We followed the method details of these nutrient measurements as de-scribed by Bao (2000). The soil stoichiometric ratios (C/N, C/P, C/K, N/P and N/K) were represented as mass ratios.
Statistical analysis
We used one-way analysis of variance (ANOVA) to test the effects of restoration type (natural, artificial, unaffected) on vegetation coverage and soil, and Tukey’s honestly significant difference (HSD) test was conducted if the differences in the effects were significant (p < 0.05). Principal component analysis (PCA) was also undertaken to examine the associations among soil chemical properties in three restoration type lands. The Pearson’s correlation analysis was used to correlate among soil physical properties in the three type lands or between soil organic carbon and the other nutrient properties in each type land. ANOVA and Pearson coefficients were analyzed with SPSS (Chicago, IL, United States) version 16.0, and PCA was performed with Canoco 5 (Microcomputer Power, NY, United States).
Results
We found a significant difference in vegetation coverage between D. NR. and D. AR. (p < 0.001) after two growing seasons following the 7.0 magnitude earthquake (Figure 1). There was still an decrease (−59.7%) in vegetation coverage in destroyed landsides with different restoration approaches compared to undestroyed lands, and vegetation coverage from artificial restoration lands showed a significant increase (about +130.2%) compared to vegetation coverage in natural restoration areas (Figure 2).
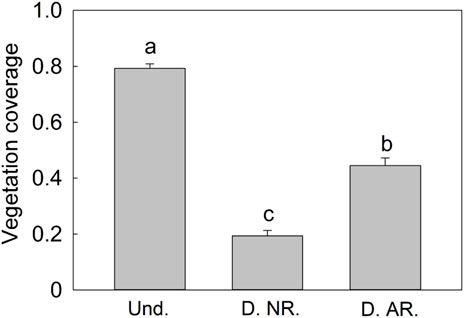
FIGURE 2. Difference in vegetation coverage under different type lands in Jiuzhaigou. Und.: undestroyed land; D. NR.: destroyed land with natural restoration; D. AR.: destroyed land with artificial restoration. Bars represent mean ± standard error (n = 4–5); different letters mean statistical differences (p < 0.05) among slope types according to Turkey’s HSD test.
Soil pH, CEC, contents of C, N, P, K, AN, AP, AK, eCa and eMg were significantly different among the three types of land (Figures 3A–K). Compared to the unaffected land areas, both soils from areas with natural restoration and with artificial restoration increased in pH and K content, but decreased their values of CEC, C, N, AN, AP, AK, eCa and eMg. Additionally, areas with artificial restoration exhibited a significant higher soil CEC (+178.8%), C (+58.0%), N (+32.2%), P (+62.9%), AN (+138.8%), AK (+14.5%) and eCa (+278.4%) compared to areas with natural restoration.
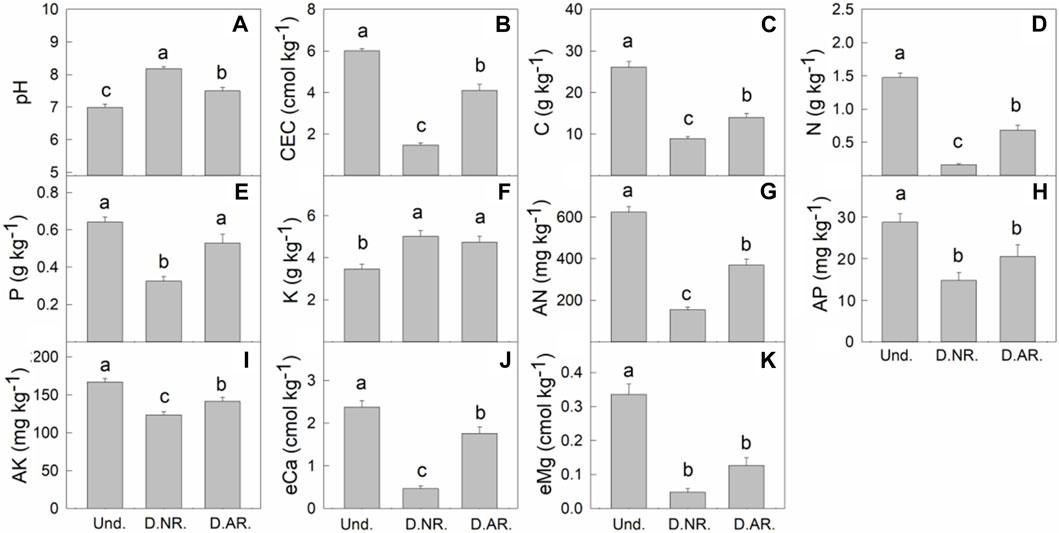
FIGURE 3. Differences in soil pH, cation exchange capacity (CEC) and nutrient content under different types of slope in Jiuzhaigou. C represents soil organic carbon; N represents total nitrogen; P represents total phosphorus; K represents total potassium; AN represents available nitrogen; AP represents available phosphorus; AK represents available potassium; eCa represents exchangeable calcium; eMg represents exchangeable magnesium. Und.: undestroyed land; D. NR.: destroyed land with natural restoration; D. AR.: destroyed land with artificial restoration. Bars represent mean ± standard error (n = 20–25); different letters mean statistical differences (p < 0.05) among slope types according to Turkey’s HSD test.
Soil C, N, P and K stoichiometry were also significantly affected by earthquake-induced land types (Figures 4A–F). Besides C/N, areas affected by landslides following the earthquake exhibited decreased C/P, C/K, N/P, N/K and P/K. The artificial restoration treatment did not significantly influence C/P or C/K, but increased N/P, N/K or P/K compared to those measurements from the natural restoration.
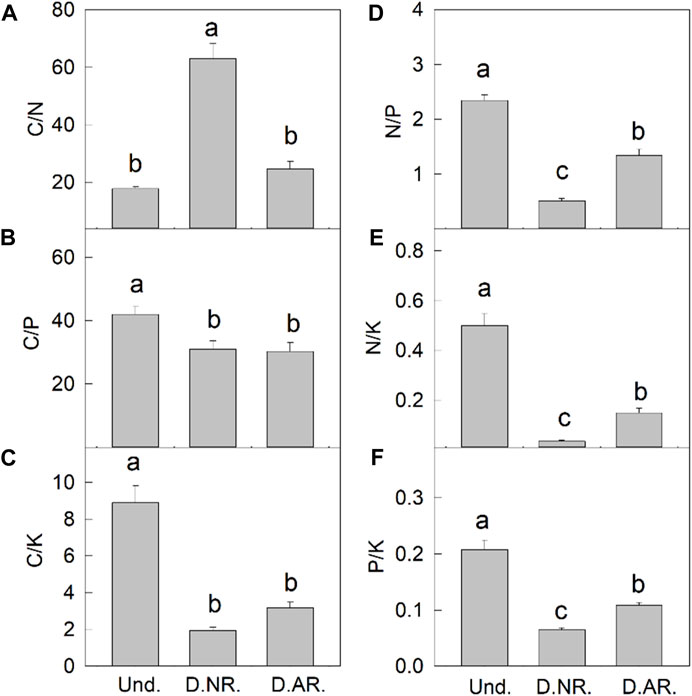
FIGURE 4. Differences in soil organic carbon, nitrogen, phosphorus, potassium stoichiometry under different type lands in Jiuzhaigou. Und.: undestroyed land; D. NR.: destroyed land with natural restoration; D. AR.: destroyed land with artificial restoration. Bars represent mean ± standard error (n = 20–25); different letters mean statistical differences (p < 0.05) among slope types according to Turkey’s HSD test.
The PCA showed clear delineation based on soil pH, CEC, nutrient content and C, N, P, K stoichiometry trait combinations in the different lands (Figure 5). These traits among natural restoration, artificial restoration and undestroyed lands were well separated from each other. The two-component PCA models explained 83.19% of the observed total variance. PC1 explained 67.78% was strongly influenced by most of the traits in pH, C, N/K, eMg, N/P, P/K, AN, N, CEC, eCa and AK, and PC2 explained 15.41% was mainly influenced by C/P.
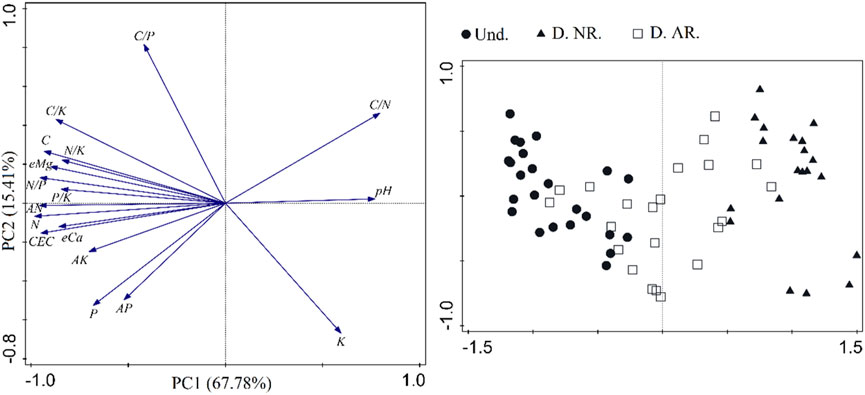
FIGURE 5. Results of a principal components analysis of soil chemical properties among the three type lands in Jiuzhaigou. Und.: undestroyed land; D. NR.: destroyed land with natural restoration; D. AR.: destroyed land with artificial restoration; Chemical property abbreviations are defined in Figure 3.
Across all samples, pH was positively related with K and C/N, while was negatively related with the other soil chemical content and C, N, P, K stoichiometry (Table 2). Soil organic carbon was positively correlated with most of the nutrient contents and their stoichiometry (except K and C/N). N was positively correlated with P, AN, AP, AK, eCa and eMg, but was negatively correlated with total K and C/N. Moreover, K was negatively correlated with AN, AK, eCa, eMg, C/P, C/K, N/P, N/K and P/K. In addition, C/N was negatively related with most of soil chemical properties, while C/P, C/K, N/P or P/K were negatively related with most of soil chemical properties.
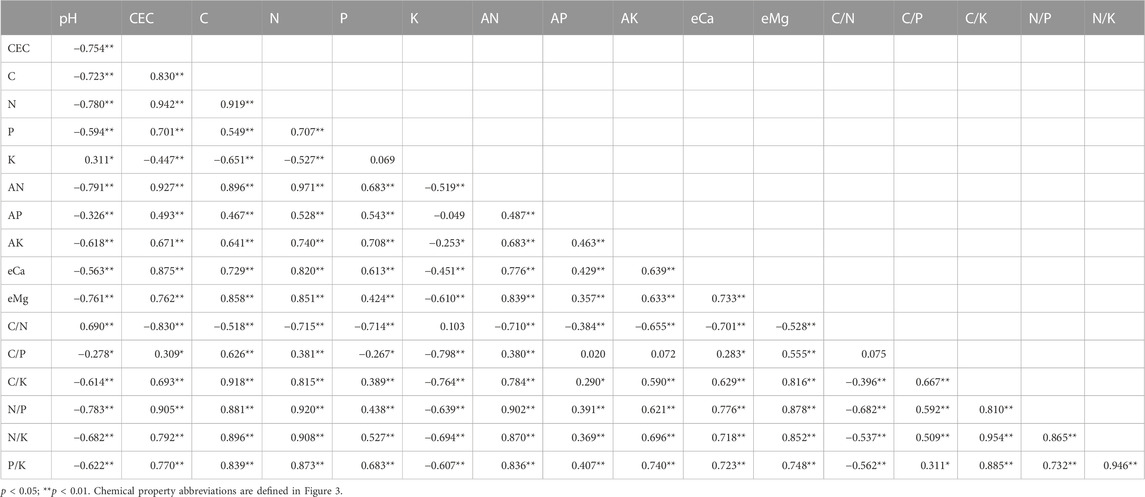
TABLE 2. Pearson’s correlation coefficients for soil chemical properties among the three type lands in Jiuzhaigou
Soil organic carbon was positively correlated with AN, C/K, N/P and N/K in each type of land, while the relationship between soil organic carbon and the other nutrient content or stoichiometry was related to land type (Table 3). In unaffected and artificial restoration slopes, C was positively related with N, eMg and P/K, but no significant relationship of these traits was found in the areas with natural restoration. Additionally, there was a negative relationship between C and P or K in the areas with natural restoration.

TABLE 3. Pearson’s correlation coefficients between soil organic carbon and the other nutrient properties in each type land in Jiuzhaigou
Discussion
Our results indicate that restoration approaches after earthquake-induced landslides in Jiuzhaigou not only affect soil nutrient contents but also the balance between nutrients, consistent with previous studies on forest recovery following earthquakes (Guo et al., 2013; Lin et al., 2017). Moreover, our findings suggest that most values of soil nutrient accumulation and vegetation coverage in areas affected by landslides with an artificial restoration project were higher than in areas that underwent natural restoration. This suggests that artificial restoration projects were more beneficial for the recovery of local vegetation in Jiuzhaigou than natural restoration.
Earthquake-induced landslides affect soil nutrient content and stoichiometry
We found that most nutrient content from areas that experience landslides were significantly lower than that on unaffected lands. These findings are similar to the results from previous studies addressing the outcomes of earthquake-induced landsides (Wu et al., 2012; Guo et al., 2013; Morgenroth et al., 2014). The lower nutrient contents are likely caused by the surface soil loss during the magnitude 7.0 earthquake in 2017, as soil macronutrients abundance in forests depend on vegetation (Guo et al., 2013; Qi et al., 2020). Landslides in Jiuzhaigou decimated the landscape, stripping away the vegetation and surface soil, which resulted in soil nutrient decrement. The results that most nutrient traits are positively related to soil organic carbon (Table 2; Figure 5) also support this conclusion.
Our study found that destroyed vegetation resulted a more decrease in nitrogen (including N and AN) than phosphorus (including P and AP), which may be related to different biogeochemical processes for nitrogen and phosphorus in karst ecosystems (Li et al., 2020; Shen et al., 2020). In areas affected by landslides, soil organic carbon was likely the major limiting factor for soil formation and biological processes, as indicated by the significant decrease in soil organic carbon content (Dialynas et al., 2016). Moreover, K, Ca and Mg are macronutrient elements that play crucial roles in plant nutrient uptake and ecosystem functions, such as maintaining organism osmosis balance, improving water use efficiency, and decreasing water loss (Sardans and Penuelas, 2015; Tang et al., 2019; Hu and Lan, 2020; Wu et al., 2022). Our results revealed that the lower contents of AK, eCa, and eMg in areas affected by landslides than in unaffected areas suggested that earthquake-induced landslides might have influenced water balance in the plant-soil system. Interestingly, we also found that total potassium concerntration increased in the post-landslide areas in Jiuzhaigou. Since K is mostly derived from the residues of soluble carbonate from nearby rock outcrops and from organic decomposition. Therefore, the high K in the post-landslide areas, where have low organic matter, imply that rock outcrops process much more important role biogeochemical cycle than expected, more studies are need to investigate the role of K in forest restoration in karst geology.
In this study, the soil C: N and C:P ratios were used to represent the supply capacity of nitrogen and phosphorus, respectively, as well as the decomposition ability of organic matter (Jiang et al., 2019; Qi et al., 2020). We found that the soil C:N ratio in the landslide-affected areas undergoing natural restoration (with an average value of 62.96) was higher than in the unaffected lands and higher than the average value of China’s soil (12.30) (Tian et al., 2010). This suggests that the nitrogen deficiency in the affected areas was greater than the decrease in soil organic carbon. A C:N ratio above 25 indicates nitrogen deficiency, which causes slow organic carbon conversion by microbes (Bui and Henderson, 2013). The average value of C:N in the unaffected land (17.76) was also higher than the average values in China soil, indicating nitrogen deficiency in the study region, and the earthquake disturbance worsened the nitrogen deficiency.
Additionally, we found that the average values of soil C:P in the post-landslide areas (30.93) were lower than in the unaffected areas (40.92) and lower than the average values of China soil (52.70) (Tian et al., 2010), indicating low effectiveness of phosphorus utilization in Jiuzhaigou. Furthermore, N:P ratios have been widely used to diagnose nutrient limitations of N or P (Gusewell, 2004; Cleveland and Liptzin, 2007; Qi et al., 2020). We found that the average values of soil N:P (0.51) in the post-landslide areas were lower than in the unaffected areas (2.35), and both were lower than the average values of China’s soil (3.90) (Tian et al., 2010). These results suggest that nitrogen is more deficient than phosphorus in Jiuzhaigou forest surface soil, especially in areas affected by landslides. Our findings are consistent with previous research that indicates that nitrogen is the most limiting nutrient in temperate forests (Vitousek and Howarth, 1991) and in the early stage of vegetation restoration in karst rocky desertification regions (Zhang et al., 2018; Liu et al., 2019; Lan et al., 2020). Therefore, these results suggest that nitrogen limitation is critical for post-landslide restoration.
The lower values in C:K, N:K, and P:K in the areas affected by landslides than the unaffected areas likely caused by the decrease in soil organic carbon, nitrogen, or phosphorus rather than K. As the total K concentration increased in the affected areas. However, despite the increase in total K concerntration in the post-landslide areas, the available K was still lower than that available in the unaffected areas. These findings suggest that local K is mainly non-available K, and the transformation process of K availability is limited by soil leaching, which is common in karst geology with a montane climate (Shen et al., 2020). Although K is the most abundant cation in plant cells and is beneficial for stress tolerance (Sardans and Penuelas, 2015), relatively less attention has been paid to K-related stoichiometry compared to N or P. The balance of K and N or P may be crucial for biogeochemical processes in montane climates (Shen et al., 2020). Our results show that total K was negatively related to N, AN, eCa, eMg, and CEC (Table 2), which suggests that rich soil K in Jiuzhaigou may inhibit soil other cation cycles, especially the nitrogen cycle. The rich soil K stocks may hamper vegetation recovery in Jiuzhaigou, as a recent study has confirmed that soil K stocks correlated negatively with biomass accumulation in the karst forest (Shen et al., 2020). Until recently, the role of K, especially on the stoichiometry of K and the other macronutrient element, in degraded ecosystems remained largely unexplored. Therefore, additional studies should be conducted on K-related stoichiometry during vegetation succession in the monsoon climate (Sardans and Penuelas, 2015; Qiu et al., 2018; Shen et al., 2020).
Difference of soil nutrient properties between artificial restoration and natural restoration areas
The elevated soil nutrient levels in artificial restoration areas are most likely due to the planted vegetation, which can effectively prevent soil erosion, stabilize soil aggregates, and enhance soil organic matter through root exudation and leaf litter (Li et al., 2020). Increased soil organic matter generally results in greater microbial diversity, which, in turn, benefits soil nutrient accumulation through various rhizosphere processes (Liu et al., 2019). Moreover, we observed a positive correlation between most soil nutrients and soil organic carbon across all three land types (Figure 5; Table 2), as well as an increase in vegetation cover in artificial restoration areas (Figure 2). Thus, soil organic matter and nutrients may be the primary limiting factors affecting vegetation recovery in Jiuzhaigou following landslides. Similar results have been observed in natural succession studies following landslides (Dialynas et al., 2016; Blonska et al., 2018).
Notably, we observed that in artificial restoration areas, soil organic carbon was positively correlated with total N, exchangeable Ca, and exchangeable Mg, while the opposite was true in natural restoration areas, where total P or total K was negatively related to soil organic carbon (Table 2). These findings suggest that in the natural restoration areas, local soil P and K may have been provided by other geological processes rather than vegetation import. Overall, our results suggest that natural restoration is an inefficient approach for vegetation recovery following earthquake-induced landslides when compared to artificial restoration. In artificial restoration land, the soil nutrient cycle was limited by organic matter, which was replenished by artificially planted plant species. Moreover, we found that N, rather than P or K, is the major limiting macronutrient in artificial restoration areas. The annual native Poaceae species in the artificial restoration areas can quickly provide organic matter through leaf litter, which can promote microorganism colonization and provide nutrient elements to some extent. Based on the positive relationship between total N and organic carbon, we recommend the consideration of nitrogen-fixing plant species in future artificial restoration strategies in Jiuzhaigou.
Implications of vegetation restoration approach on karst ecosystem management
The rocky karst terrain is highly vulnerable to land degradation, making natural recovery of vegetation challenging (Xie et al., 2015). In eastern Qinghai-Tibet, frequent earthquakes, steep slopes, and sensitive climate have led to significant soil erosion (Guo et al., 2013; Xia et al., 2020; Nie et al., 2022). Although our study has shown that artificial restoration has advantages in terms of soil nutrient and rapid vegetation recovery compared to natural restoration, its impact on other ecosystem services (such as fertility maintenance, soil erosion control, and biodiversity conservation) in the aftermath of natural forest lands devastation remains unexplored. The role of vegetation restoration in ecosystem services is essential for ecosystem management, especially in this important area for world biodiversity conservation (Liu et al., 2023; Jayachandran et al., 2017; Zhang et al., 2018).
However, previous studies comparing natural restoration with artificial restoration have revealed some limitations of the latter. For instance, artificial restoration programs have resulted in decreased soil nitrogen-cycling diversity in pine plantations, as well as a narrower range of ecosystem functions (Wang et al., 2019; Wang et al., 2020). Other studies have identified decreased spatial heterogeneity of soil conditions, decreased cost-effectiveness, and potential high-yield returns in ecosystem services (Chazdon, 2008). To enhance the effectiveness and benefits of artificial restoration, a comprehensive management approach that combines natural and artificial restoration may improve the ecological value of the forest and ensure long-term ecosystem function (Nilsson et al., 2006; Wang et al., 2021). For the fragile karst ecosystem in Jiuzhaigou, investigating the effects of different restoration approaches, including their long-term impacts on other ecosystem services, especially on biodiversity, is still necessary.
Conclusion
The Jiuzhaigou earthquake with a magnitude of 7.0 triggered numerous landslides, resulting in a significant reduction in vegetation coverage and soil nutrients, particularly soil organic carbon, nitrogen, exchangeable Ca and Mg, rather than P and K. Our study showed that artificial restoration, despite being implemented for only two growing seasons, led to improved soil nutrient and vegetation coverage compared to natural restoration. Furthermore, our findings suggest that the soil in Jiuzhaigou is mainly limited by soil nitrogen rather than P or K. These results indicate that incorporating native nitrogen-fixing plant species in restoration strategies is recommended to reduce nitrogen limitation and promote restoration success in the future. Our results could provide valuable insights for post-earthquake forest restoration in karst regions worldwide.
Data availability statement
The original contributions presented in the study are included in the article/Supplementary material, further inquiries can be directed to the corresponding authors.
Author contributions
XH: Writing–original draft, Investigation. TX: Investigation, Writing–original draft. TL: Investigation, Software, Writing–review and editing. LH: Writing–review and editing. MD: Writing–review and editing, Validation. DD: Conceptualization, Project administration, Supervision, Writing–review and editing. TD: Conceptualization, Supervision, Writing–original draft, Writing–review and editing.
Funding
The author(s) declare financial support was received for the research, authorship, and/or publication of this article. The work was supported by the Jiuzhaigou Post-Disaster Restoration and Reconstruction Program (5132252018000202) and Natural Science Foundation of Sichuan Province (2022NSFSC0094).
Acknowledgments
We thank the Administration of Jiuzhaigou National Nature Reserve.
Conflict of interest
The authors declare that the research was conducted in the absence of any commercial or financial relationships that could be construed as a potential conflict of interest.
Publisher’s note
All claims expressed in this article are solely those of the authors and do not necessarily represent those of their affiliated organizations, or those of the publisher, the editors and the reviewers. Any product that may be evaluated in this article, or claim that may be made by its manufacturer, is not guaranteed or endorsed by the publisher.
References
Allen, R. B., MacKenzie, D. I., Bellingham, P. J., Wiser, S. K., Arnst, E. A., Coomes, D. A., et al. (2020). Tree survival and growth responses in the aftermath of a strong earthquake. J. Ecol. 108, 107–121. doi:10.1111/1365-2745.13238
Bao, S. D. (2000). Agricultural and chemistry analysis of soil. Beijing, China: China Agriculture Press.
Berthrong, S. T., Jobbagy, E. G., and Jackson, R. B. (2009). A global meta-analysis of soil exchangeable cations, pH, carbon, and nitrogen with afforestation. Ecol. Appl. 19, 2228–2241. doi:10.1890/08-1730.1
Blonska, E., Lasota, J., Piaszczyk, W., Wiechec, M., and Klamerus-Iwan, A. (2018). The effect of landslide on soil organic carbon stock and biochemical properties of soil. J. Soils Sediment. 18, 2727–2737. doi:10.1007/s11368-017-1775-4
Bui, E. N., and Henderson, B. L. (2013). C:N:P stoichiometry in Australian soils with respect to vegetation and environmental factors. Plant Soil 373, 553–568. doi:10.1007/s11104-013-1823-9
Chazdon, R. L. (2008). Beyond deforestation: restoring forests and ecosystem services on degraded lands. Science 320, 1458–1460. doi:10.1126/science.1155365
Cheng, S., Yang, G., Yu, H., Li, J. Y., and Zhang, L. (2012). Impacts of Wenchuan Earthquake-induced landslides on soil physical properties and tree growth. Ecol. Indic. 15, 263–270. doi:10.1016/j.ecolind.2011.09.028
Cleveland, C. C., and Liptzin, D. (2007). C: N: P stoichiometry in soil: is there a "Redfield ratio" for the microbial biomass? Biogeochemistry 85, 235–252. doi:10.1007/s10533-007-9132-0
Cui, P., Lin, Y. M., and Chen, C. (2012). Destruction of vegetation due to geo-hazards and its environmental impacts in the Wenchuan earthquake areas. Ecol. Eng. 44, 61–69. doi:10.1016/j.ecoleng.2012.03.012
Deng, J., Sun, P. S., Zhao, F. X., Han, X. H., Yang, G. H., Feng, Y. Z., et al. (2016). Soil C, N, P and its stratification ratio affected by artificial vegetation in subsoil, loess plateau China. PLoS One 11, e0151446. doi:10.1371/journal.pone.0151446
Dialynas, Y. G., Bastola, S., Bras, R. L., Billings, S. A., Markewitz, D., and Richter, D. D. (2016). Topographic variability and the influence of soil erosion on the carbon cycle. Glob. Biogeochem. Cycle 30, 644–660. doi:10.1002/2015gb005302
Di-Palo, F., and Fornara, D. A. (2017). Plant and soil nutrient stoichiometry along primary ecological successions: is there any link? PLoS One 12, 18. doi:10.1371/journal.pone.0182569
Duval, M. E., Galantini, J. A., Iglesias, J. O., Canelo, S., Martinez, J. M., and Wall, L. (2013). Analysis of organic fractions as indicators of soil quality under natural and cultivated systems. Soil Tillage Res. 131, 11–19. doi:10.1016/j.still.2013.03.001
Fan, H. B., Wu, J. P., Liu, W. F., Yuan, Y. H., Hu, L., and Cai, Q. K. (2015). Linkages of plant and soil C:N:P stoichiometry and their relationships to forest growth in subtropical plantations. Plant Soil 392, 127–138. doi:10.1007/s11104-015-2444-2
Guo, H. X., Sun, G., Shi, F. S., Lu, T., Wang, Q., Wu, Y., et al. (2013). Water, soil and nutrient losses caused by Wenchuan Earthquake: a case study in Pengzhou. Water Sci. Technol. 68, 1055–1062. doi:10.2166/wst.2013.343
Guo, N., Degen, A. A., Deng, B., Shi, F. Y., Bai, Y. F., Zhang, T., et al. (2019). Changes in vegetation parameters and soil nutrients along degradation and recovery successions on alpine grasslands of the Tibetan plateau. Agric. Ecosyst. Environ. 284, 106593. doi:10.1016/j.agee.2019.106593
Gusewell, S. (2004). N: P ratios in terrestrial plants: variation and functional significance. New Phytol. 164, 243–266. doi:10.1111/j.1469-8137.2004.01192.x
Hu, N., and Lan, J. C. (2020). Impact of vegetation restoration on soil organic carbon stocks and aggregates in a karst rocky desertification area in Southwest China. J. Soil Sediment. 20, 1264–1275. doi:10.1007/s11368-019-02532-y
Hu, X. D., Hu, K. H., Tang, J. B., You, Y., and Wu, C. H. (2019). Assessment of debris-flow potential dangers in the Jiuzhaigou Valley following the August 8, 2017, Jiuzhaigou earthquake, western China. Eng. Geol. 256, 57–66. doi:10.1016/j.enggeo.2019.05.004
Jayachandran, S., De-Laat, J., Lambin, E. F., Stanton, C. Y., Audy, R., and Thomas, N. E. (2017). Cash for carbon: a randomized trial of payments for ecosystem services to reduce deforestation. Science 357, 267–273. doi:10.1126/science.aan0568
Jiang, L., He, Z. S., Liu, J. F., Xing, C., Gu, X. G., Wei, C. S., et al. (2019). Elevation gradient altered soil C, N, and P stoichiometry of Pinus taiwanensis forest on daiyun mountain. Forests 10, 1089. doi:10.3390/f10121089
Jiang, X., Xu, D. P., Rong, J. J., Ai, X. Y., Ai, S. H., Su, X. Q., et al. (2021). Landslide and aspect effects on artificial soil organic carbon fractions and the carbon pool management index on road-cut slopes in an alpine region. Catena 199, 105094. doi:10.1016/j.catena.2020.105094
Lan, J. C., Hu, N., and Fu, W. L. (2020). Soil carbon-nitrogen coupled accumulation following the natural vegetation restoration of abandoned farmlands in a karst rocky desertification region. Ecol. Eng. 158, 106033. doi:10.1016/j.ecoleng.2020.106033
Lebrato, M., Wang, Y. V., Tseng, L. C., Achterberg, E. P., Chen, X. G., Molinero, J. C., et al. (2019). Earthquake and typhoon trigger unprecedented transient shifts in shallow hydrothermal vents biogeochemistry. Sci. Rep. 9, 16926. doi:10.1038/s41598-019-53314-y
Li, J. Y., Yuan, X. L., Ge, L., Li, Q., Li, Z. G., Wang, L., et al. (2020). Rhizosphere effects promote soil aggregate stability and associated organic carbon sequestration in rocky areas of desertification. Agri. Ecosyst. Environ. 304, 107126. doi:10.1016/j.agee.2020.107126
Li, W. J., Ge, X. D., and Liu, C. Y. (2005). Hiking trails and tourism impact assessment in protected area: Jiuzhaigou Biosphere Reserve, China. Environ. Monit. Assess. 108, 279–293. doi:10.1007/s10661-005-4327-0
Li, Y. Y., Dong, S. K., Wen, L., Wang, X. X., and Wu, Y. (2014). Soil carbon and nitrogen pools and their relationship to plant and soil dynamics of degraded and artificially restored grasslands of the Qinghai-Tibetan Plateau. Geoderma 213, 178–184. doi:10.1016/j.geoderma.2013.08.022
Lin, Y. M., Deng, H. J., Du, K., Li, J., Lin, H., Chen, C., et al. (2017). Soil quality assessment in different climate zones of China's Wenchuan earthquake affected region. Soil Tillage Res. 165, 315–324. doi:10.1016/j.still.2016.09.009
Liu, J., Du, J., Zhang, C., Zhang, J., Yang, H., Donald, M. L., et al. (2023). Ecosystem service assessment under ecological restoration programs: a systematic review of studies from China. Front. Ecol. Evol. 11, 1152907. doi:10.3389/fevo.2023.1152907
Liu, W. C., Fu, S. Y., Yan, S. J., Ren, C. J., Wu, S. J., Deng, J., et al. (2020). Responses of plant community to the linkages in plant-soil C:N:P stoichiometry during secondary succession of abandoned farmlands, China. J. Arid. Land 12, 215–226. doi:10.1007/s40333-020-0009-6
Liu, X., Zhang, W., Wu, M., Ye, Y. Y., Wang, K. L., and Li, D. J. (2019). Changes in soil nitrogen stocks following vegetation restoration in a typical karst catchment. Land Degrad. Dev. 30, 60–72. doi:10.1002/ldr.3204
Liu, Y., Lei, S. G., and Gong, C. G. (2019). Comparison of plant and microbial communities between an artificial restoration and a natural restoration topsoil in coal mining subsidence area. Environ. Earth Sci. 78, 204. doi:10.1007/s12665-019-8195-2
Morgenroth, J., Almond, P., Scharenbroch, B. C., Wilson, T. M., and Sharp-Heward, S. (2014). Soil profile inversion in earthquake-induced liquefaction-affected soils and the potential effects on urban trees. Geoderma 213, 155–162. doi:10.1016/j.geoderma.2013.07.038
Nie, X., Wang, D., Chen, Y., Yang, L., and Zhou, G. (2022). Storage, distribution, and associated controlling factors of soil total phosphorus across the northeastern Tibetan plateau shrublands. J. Soil Sci. Plant Nutr. 22, 2933–2942. doi:10.1007/s42729-022-00857-1
Nie, X., Wang, D., Ren, L., Du, Y., and Zhou, G. (2023). Storage and controlling factors of soil organic carbon in alpine wetlands and meadow across the Tibetan Plateau. Eur J Soil Sci 74, e13383. doi:10.1111/ejss.13383
Nilsson, U., Orlander, G., and Karlsson, M. (2006). Establishing mixed forests in Sweden by combining planting and natural regeneration - effects of shelterwoods and scarification. For Ecol Manage 237, 301–311. doi:10.1016/j.foreco.2006.09.053
Palmer, M. A., Ambrose, R. F., and Poff, N. L. (1997). Ecological theory and community restoration Ecology. Restor. Ecol. 5, 291–300. doi:10.1046/j.1526-100x.1997.00543.x
Qi, K. B., Pang, X. Y., Yang, B., and Bao, W. K. (2020). Soil carbon, nitrogen and phosphorus ecological stoichiometry shifts with tree species in subalpine plantations. PeerJ 8, e9702. doi:10.7717/peerj.9702
Qiu, K. Y., Xie, Y. Z., Xu, D. M., and Pott, R. (2018). Ecosystem functions including soil organic carbon, total nitrogen and available potassium are crucial for vegetation recovery. Sci. Rep. 8, 7607. doi:10.1038/s41598-018-25875-x
Santiago, L. S., Wright, S. J., Harms, K. E., Yavitt, J. B., Korine, C., Garcia, M. N., et al. (2012). Tropical tree seedling growth responses to nitrogen, phosphorus and potassium addition. J. Ecol. 100, 309–316. doi:10.1111/j.1365-2745.2011.01904.x
Sardans, J., and Penuelas, J. (2015). Potassium: a neglected nutrient in global change. Glob. Ecol. Biogeogr. 24, 261–275. doi:10.1111/geb.12259
Scharler, U. M., Ulanowicz, R. E., Fogel, M. L., Wooller, M. J., Jacobson-Meyers, M. E., Lovelock, C. E., et al. (2015). Variable nutrient stoichiometry (carbon:nitrogen:phosphorus) across trophic levels determines community and ecosystem properties in an oligotrophic mangrove system. Oecologia 179, 863–876. doi:10.1007/s00442-015-3379-2
Shen, Y. X., Yu, Y., Lucas-Borja, M. E., Chen, F. J., Chen, Q. Q., and Tang, Y. Y. (2020). Change of soil K, N and P following forest restoration in rock outcrop rich karst area. Catena 186, 104395. doi:10.1016/j.catena.2019.104395
Sterner, R. W., and Elser, J. J. (2002). Ecological Stoichiometry: Biology of Elements from Molecules to the Biosphere. Princeton, NJ: Princeton University Press.
Stokes, A., Sotir, R., Chen, W., and Chestem, M. (2010). Soil bio- and eco-engineering in China: past experience and future priorities. Eco. Eng. 36, 247–257. doi:10.1016/j.ecoleng.2009.07.008
Su, L., Du, H., Zeng, F. P., Peng, W. X., Rizwan, M., Nunez-Delgado, A., et al. (2019). Soil and fine roots ecological stoichiometry in different vegetation restoration stages in a karst area, southwest China. J. Environ. Manage 252, 109694. doi:10.1016/j.jenvman.2019.109694
Tang, J., Tang, X. X., Qin, Y. M., He, Q. S., Yi, Y., and Ji, Z. L. (2019). Karst rocky desertification progress: soil calcium as a possible driving force. Sci. Total Environ. 649, 1250–1259. doi:10.1016/j.scitotenv.2018.08.242
Thomsen, M. S., Metcalfe, I., Siciliano, A., South, P. M., Gerrity, S., Alestra, T., et al. (2020). Earthquake-driven destruction of an intertidal habitat cascade. Aquat. Bot. 164, 103217. doi:10.1016/j.aquabot.2020.103217
Tian, H. Q., Chen, G. S., Zhang, C., Melillo, J. M., and Hall, C. A. S. (2010). Pattern and variation of C:N:P ratios in China's soils: a synthesis of observational data. Biogeochemistry 98, 139–151. doi:10.1007/s10533-009-9382-0
Vitousek, P. M., and Howarth, R. W. (1991). Nitrogen limitation on land and in the sea - how can it occur. Biogeochemistry 13, 87–115. doi:10.1007/bf00002772
Wang, J., Jin, W., Cui, Y. F., Zhang, W. F., Wu, C. H., and Pasuto, A. (2018). Earthquake-triggered landslides affecting a UNESCO natural site: the 2017 Jiuzhaigou earthquake in the world national park, China. J. Mt. Sci. 15, 1412–1428. doi:10.1007/s11629-018-4823-7
Wang, M., Liu, Q. H., and Pang, X. Y. (2021). Evaluating ecological effects of roadside slope restoration techniques: a global meta-analysis. J. Environ. Manage 281, 111867. doi:10.1016/j.jenvman.2020.111867
Wang, W., Liu, J., Kozak, R., Jin, M., and Innes, J. L. (2018). How do conservation and the tourism industry affect local livelihoods? A comparative study of two nature reserves in China. Sustainability 10 (6), 1925. doi:10.3390/su10061925
Wang, Y., Zheng, H., Chen, F. L., Yang, Y. F., Zeng, J., Van-Nostrand, J. D., et al. (2019). Artificial reforestation produces less diverse soil nitrogen-cycling genes than natural restoration. Ecosphere 10, 10. doi:10.1002/ecs2.2562
Wang, Y., Zheng, H., Chen, F. L., Zeng, J., Zhou, J. Z., and Ouyang, Z. Y. (2020). Stabilities of soil organic carbon and carbon cycling genes are higher in natural secondary forests than in artificial plantations in southern China. Land Degrad. Dev. 31, 2986–2995. doi:10.1002/ldr.3649
Wardle, D. A., Walker, L. R., and Bardgett, R. D. (2004). Ecosystem properties and forest decline in contrasting long-term chronosequences. Science 305, 509–513. doi:10.1126/science.1098778
Wright, S. J., Yavitt, J. B., Wurzburger, N., Turner, B. L., Tanner, E. V. J., Sayer, E. J., et al. (2011). Potassium, phosphorus, or nitrogen limit root allocation, tree growth, or litter production in a lowland tropical forest. Ecology 92, 1616–1625. doi:10.1890/10-1558.1
Wu, C., Wang, J., Lu, T., Wu, Y., and Wu, N. (2012). Effect of wenchuan earthquake on soil physical and chemical properties of the longmen mountain, southwestern China. Chin. J. Appl. Environ. Biol. 18, 911–916. doi:10.3724/sp.j.1145.2012.00911
Wu, F., Xu, Z., and Yang, W. (2022). Carbon and nutrient transfer via above- and below-ground litter in forests. Forests 13, 2176. doi:10.3390/f13122176
Xia, B., Fan, X., Guo, X., Yang, F., Zou, C., and Xiong, K. (2020). Spatial distribution characteristics and analysis of soil erosion under earthquake effect:A case study of Jiuzhaigou earthquake. Sci. Soil Water Conserv. 18, 79–89. doi:10.16843/j.sswc.2020.01.010
Xie, L. W., Zhong, J., Chen, F. F., Cao, F. X., Li, J. J., and Wu, L. C. (2015). Evaluation of soil fertility in the succession of karst rocky desertification using principal component analysis. Solid earth. 6, 515–524. doi:10.5194/se-6-515-2015
Xu, B., Zhu, Z., Li, J., Wu, Y., Deng, G., Wu, N., et al. (2016). Soil nutrient characteristics in different forest types at the Jiuzhaigou National Nature Reserve, China. Chin. J. Appl. Environ. Biol. 22, 767–772. doi:10.3724/SP.J.1145.2016.03022
Zhang, J. D., Hull, V., Huang, J. Y., Yang, W., Zhou, S. Q., Xu, W. H., et al. (2014). Natural recovery and restoration in giant panda habitat after the Wenchuan earthquake. For Ecol Manage 319, 1–9. doi:10.1016/j.foreco.2014.01.029
Zhang, J. Z., Luo, M. T., Yue, H., Chen, X. Y., and Feng, C. (2018). Critical thresholds in ecological restoration to achieve optimal ecosystem services: an analysis based on forest ecosystem restoration projects in China. Land Use Pol. 76, 675–678. doi:10.1016/j.landusepol.2018.02.050
Zhang, Y. H., Xu, X. L., Li, Z. W., Liu, M. X., Xu, C. H., Zhang, R. F., et al. (2019). Effects of vegetation restoration on soil quality in degraded karst landscapes of southwest China. Sci. Total Environ. 650, 2657–2665. doi:10.1016/j.scitotenv.2018.09.372
Zhao, B., Wang, Y. S., Luo, Y. H., Li, J., Mang, X., and Shen, T. (2018). Landslides and dam damage resulting from the Jiuzhaigou earthquake (8 August 2017), Sichuan, China. R. Soc. Open Sci. 5, 171418. doi:10.1098/rsos.171418
Keywords: landslide restoration, karst ecosystem, restoration strategy, soil nutrient, Jiuzhaigou
Citation: Huang X, Xian T, Long T, He L, Donald ML, Deng D and Dong T (2024) Impacts of vegetation restoration strategies on soil nutrients and stoichiometry of the earthquake-induced landslides in Jiuzhaigou, eastern Qinghai-Tibet Plateau. Front. Environ. Sci. 11:1296187. doi: 10.3389/fenvs.2023.1296187
Received: 15 October 2023; Accepted: 20 November 2023;
Published: 08 January 2024.
Edited by:
José Manuel Fernández-Guisuraga, University of León, SpainReviewed by:
Rudong Zhao, Chinese Academy of Sciences (CAS), ChinaXiuqing Nie, Chinese Academy of Forestry, China
Leilei Ding, Guizhou Academy of Agricultural Sciences, China
Mingzhi Lu, Northeast Normal University, China
Copyright © 2024 Huang, Xian, Long, He, Donald, Deng and Dong. This is an open-access article distributed under the terms of the Creative Commons Attribution License (CC BY). The use, distribution or reproduction in other forums is permitted, provided the original author(s) and the copyright owner(s) are credited and that the original publication in this journal is cited, in accordance with accepted academic practice. No use, distribution or reproduction is permitted which does not comply with these terms.
*Correspondence: Tingfa Dong, ZG9uZ2ZhckAxNjMuY29t; Dongzhou Deng, ZG9uZ3pob3VkQDE2My5jb20=
†These authors have contributed equally to this work