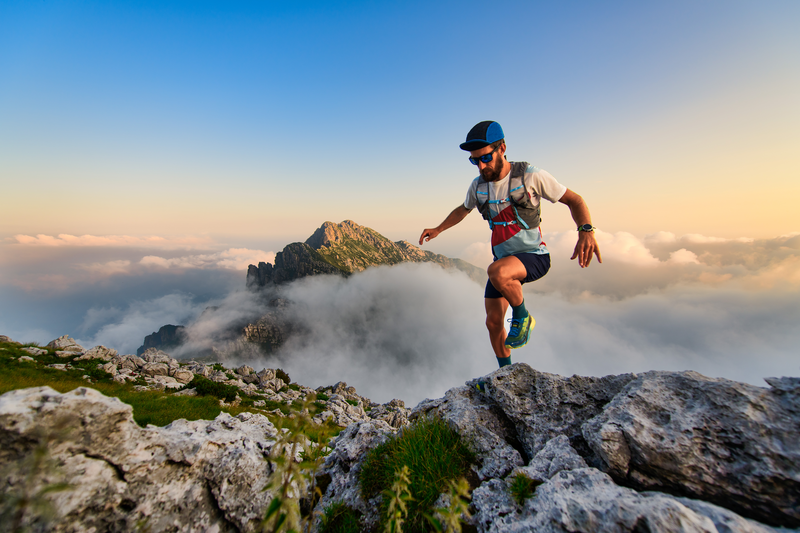
94% of researchers rate our articles as excellent or good
Learn more about the work of our research integrity team to safeguard the quality of each article we publish.
Find out more
ORIGINAL RESEARCH article
Front. Environ. Sci. , 06 October 2023
Sec. Toxicology, Pollution and the Environment
Volume 11 - 2023 | https://doi.org/10.3389/fenvs.2023.1273930
This article is part of the Research Topic Resource Conversion and Safe Disposal of Solid Wastes Containing Heavy Metals View all 4 articles
Introduction: Municipal solid waste (MSW) incineration fly ash is a harmful residue formed during the incineration process. It contains high concentrations of hazardous heavy metals, such as lead, zinc, aluminum, and iron.
Methodology: In this study, bioleaching integrated with an electrokinetic approach for heavy metal remediation from MSW incineration fly ash using Acidithiobacillus ferrooxidans and Acidithiobacillus thiooxidans bacteria was tested.
Results and discussion: The physicochemical properties of fly ash included a particle size of 26.1 μm, with the presence of heavy metals. A. ferrooxidans and A. thiooxidans produced sulphuric acid (0.0289 M and 0.0352 M) during the proliferation; this acid enhances the bioleaching of heavy metals from fly ash. The results of an integrated approach showed an 85%, 47%, 92%, 85%, 46%, 67% 11%, and 55% removal of the heavy metals K, Na, Ca, Mg, Al, Zn, Pb, and Mg, respectively, in the presence of A. ferrooxidans. Overall, these results evidenced that heavy metals were completely removed from the fly ash using an integrated approach. Therefore, this integrated approach can be used as an effective heavy metal removal method for treating fly ash in MSW.
Incineration is widely used in handling municipal solid waste (MSW) in places where land scarcity is a problem. Typically, the process results in a volume reduction of 95%–96% and a mass reduction of 80%–85%, depending on the degree of recovery and composition of materials, such as metals from the ashes for the recycling process (Ramboll, 2006; Tammaro et al., 2015). In this case, incineration does not completely replace landfilling, which reduces the necessary quantity for disposal significantly. The incineration fly ash and bottom ash are non-combustible elements that remain after the incineration process (Chiang and Pan, 2017). Fly ash refers to particles that are retained in the flue gas cleaning system, such as an electrostatic precipitator or baghouse filters (Jaworek et al., 2019). Since fly ash frequently includes significant quantities of heavy metals, such as lead, cadmium, copper, and zinc, as well as minor levels of dioxins and furans, it poses a greater health risk than bottom ash (Chan and Chau, 1997; Sushil and Batra, 2006). Owing to its toxicity, the majority of fly ash is either immobilized with cement and placed in regulated landfills or kept underground. Fly ash, on the other hand, may be regarded as an “artificial ore” as certain elements are present in quantities that allow for profitable metal recovery (Bosshard et al., 1996; Nagib and Inoue, 2000). Resource recycling and recovery from fly ash can reduce the potential for environmental pollution and minimize environmental impacts. In addition, revenue may be obtained from the recovered products (Liu et al., 2021).
Traditional hydrometallurgical processes, such as thermal and chemical leaching, allow the quick treatment and full annihilation of hazardous organic components in fly ash. It has been suggested that the treatment of fly ash could lead to detoxification and potential resource recovery. Conventionally, chloride evaporation, thermal treatment, and chemical leaching are used in the detoxification or decontamination of incineration fly ash (Abbas et al., 2020). Unfortunately, they frequently require a large amount of energy, resulting in the emission of dangerous substances into the environment. Because of the negative environmental effect and high expense of current approaches, researchers are looking at cleaner and more economical methods for extracting heavy metals from fly ash. An integrated approach of bioleaching and electrokinetics has recently received great attention and shows promise for resource recovery and the remediation of heavy metals. Bioleaching provides various benefits over traditional fly ash treatment, including low energy requirements, cheap capital costs, decreased landfill area, and potential resource recovery (Krebs et al., 1997). Electrokinetic approaches are advantageous technologies because of their low power consumption, control over the flow direction of water and dissolved pollutants, and containment of contaminants in the electrode chambers, which simplifies future treatment and can potentially be applied in situ and ex situ (Acar and Alshawabkeh, 1993; Virkutyte et al., 2002; Cameselle et al., 2013).
Bioleaching processes are based on the competence of microorganisms to transform solid compounds, with the production of organic/inorganic acids, which can recover the soluble and extractable elements from the fly ash. Numerous groups of microorganisms play significant roles in the bioleaching process, including heterotrophic bacteria, chemolithoautotrophic bacteria (Acidithiobacillus ferrooxidans and Acidithiobacillus thiooxidans), and fungi (Krebs et al., 1997). Patents for pilot or commercial-scale bioleaching systems have been issued, with the majority focusing on low-grade ore (Pradhan et al., 2008). Recently, there has been some interest in applying bioleaching from mining ores to industrial wastes, as huge volumes of toxic industrial waste have been created (Castro et al., 2021).
The integration of bioleaching and electrokinetics can potentially overcome numerous limitations of the individual methods, with the prospect that some of the integrated approaches may demonstrate synergy (Maini et al., 2000a). Metals as hydroxides or oxides may be solubilized by electrokinetic acidification, but those present as insoluble sulfides will not be extracted using this method (Maini et al., 2000a). However, the presence of bacteria can convert metal sulfides to sulfates in the bioleaching processes, empowering their solubilization and subsequent transport by electromigration (Schippers, 2007). However, the directional transport of metal ions by electrokinetics is a valuable aspect of bioleaching, as solubilized metals may be removed at the cathode for simple recovery. An additional advantage of this method is the potential to reduce more expensive and hazardous chemicals, such as sulphuric acid. The present study evaluates the electrochemically enhanced bioleaching of fly ash treatment. Efforts are directed at the physical, chemical, and biological processes to augment bacterial activity and growth for metal dissolution. Additionally, the study evaluates the efficiency of the integrated approach of bioleaching-enhanced electrokinetic remediation of heavy metals from municipal solid incinerated fly ash.
MSW incinerator fly ash was collected from the Waste-to-Energy Research Facility (WTERF) at Tuas South in Singapore. The dry ash was collected into sterile plastic containers, stored in a dry cabinet, and transferred to the lab. The fly ash was autoclaved at 121°C for 15 min for further studies.
Particle size and shape were analyzed using the particle size analyzer (Coulter LS 230), with particles in the range of 0.04 μm–2,000 μm, and scanning electron microscopy (SEM), respectively. The specific surface area was determined using a QuantaChrome Corporation Nova 3,000 Brunauer–Emmett–Teller analyzer based on the BET equation. The bulk density was calculated using the ASTM D1895-96 standard. A funnel positioned above a 10 mL measuring cylinder was filled with fly ash that was allowed to flow freely into the cylinder until it reached 10 mL. The bulk density was estimated after weighing the sample and the cylinder (Wu and Ting, 2006).
The elemental composition of the fly ash was analyzed using the US EPA SW 846 method 3050B. After acid digestion, the material was examined by inductively coupled plasma mass spectrometry (ICP-MS). Toxicity characteristic leaching procedure (TCLP) tests were performed in accordance with the US EPA SW 846 method 1,311 to determine its toxicity. During the leaching experiments, TCLP extraction fluid #2 (un-buffered acetic acid solution) was employed. The metal concentrations leached in TCLP were compared with the levels authorized by the US EPA and Singapore’s National Environment Agency (NEA) (Xu and Ting, 2004).
Acidithiobacillus was obtained from the DSMZ microorganism culture collection in Germany (A. thiooxidans, DSMZ 11478; A. ferrooxidans, DSMZ 3320). A. ferrooxidans was cultured in Silverman and Lundgren 9k nutrient medium, which contained ferrous sulphate as the energy source. The medium was prepared in two parts: 1) basal salt solution consisting of 0.1 g of KCl, 3.0 g of (NH4)2SO4, 0.5 g of MgSO4.7H2O, 0.5 g of K2HPO4, and 0.01 g of Ca(NO3)2 dissolved in 700 mL of de-ionized water; 2) iron sulphate solution (44.1 g of FeSO4.7H2O in 300 mL of water acidified by 1 mL of H2SO4 10N. The basal salt solution was sterilized and autoclaved at 121°C for 15 min. The iron sulphate solution was sterilized by filtering the solution through a 0.04 μm syringe membrane as it decomposed upon heating and thus could not be autoclaved. The cultures were incubated at 30°C with shaking at 120 rpm (Zhang et al., 2013).
A. thiooxidans was prepared using a medium that consisted of 2.0 g of (NH4)2SO4, 0.5 g of K2HPO4, and 0.25 g of MgSO47H2O with powdered sulphur (10 g/L) in 1 L of de-ionized water; the medium was then sterilized. Finally, the cultures were incubated at 30°C with shaking at 120 rpm (Zhang et al., 2013).
The growth cycle of the bacteria is obtained by using three different methods and correlating them, e.g., growth pattern, pH reading (Narenkumar et al., 2019), and titration. It is important to obtain the growth cycle because the bacterium produces the most metabolites and acid near the end of the exponential phase. The experiment was conducted using the spent medium with the highest amount of metabolites and acid, as it was more economical.
A STAT Titrino auto-titrator was used with 0.1028M of NaOH to determine the amount and concentration of the acid produced. Inoculate (2 mL) was obtained from the culture and diluted to 20 mL for titration. From the auto-titration, the volume of NaOH used was obtained as follows:
Moles of NaOH used = volume of NaOH × 0.1028 M;
Moles of H2SO4 in 2 mL = moles of NaOH used × ½; and Concentration of H2SO4 of inoculate = moles of H2SO4 in 2 mL × ½ × 103.
The electrokinetic cell was built using polycarbonate (three compartments) in the configuration, as shown in Figure 1. The volumes of the cathode and anode compartments were 200 mL each and the volume of the substrate compartment was 100 mL. The cells were covered to minimize loss through evaporation. A magnetic stirrer was placed in all substrate compartments to ensure a homogenous mixing of the contaminant and the leaching agents. Fly ash (20 g) was used for the electrokinetic experiments. Two separators with pores were used as support for the IONICS® ion exchange membranes from GE water.
The anolyte and catholyte reservoirs were filled with different sets of solutions, such as the spent medium, sulphuric acid, and sterile H2O as a working solution, and steel 316L served as the anode and cathode electrodes. The concentration of sulphuric acid was prepared to the same molarity as the spent medium to reduce the number of variables and observe any possible effects of the metabolites produced by the bacteria.
The experimental runs were conducted over a period of 14 days at a constant voltage of 20 V or 1 V/cm (Rodrigo et al., 2014). Samples (1 mL) was taken from the cathode and anode compartments every 2 days to monitor trends in the ionic movements. At the end of 14 days, the fly ashes were collected as sludge from the substrate compartment and dried using a drying oven to obtain the dry masses. The fly ashes were analyzed for their chemical composition and toxicity. The pH of the cathode site was controlled by adding 5% nitric acid and maintained at a constant low pH to prevent precipitation. When necessary, the loss of the liquid due to evaporation was compensated with the addition of water in the anode compartment. The residual fly ash absorption was analyzed during electrokinesis and was characterized through acid digestion, TCLP, and ICP-MS (Arul Prakash et al., 2021). ICP multi-element standard IV at a concentration of 1,000 mg/L was used to prepare the calibration standards, which were diluted to 2 ppm, 5 ppm, 8 ppm, 10 ppm, 15 ppm, and 20 ppm (1.99 mg/L, 4.99 mg/L, 7.99 mg/L, 9.99 mg/L, 14.99 mg/L, and 19.99 mg/L).
According to Xu and Ting (2004), the concentration of heavy metals in the fly ash (mg/kg) was calculated as follows:
Metal concentration (mg/kg fly ash) = concentration in solution (mg metal/liter solution) × dilution factor × liquid to solid ratio (liter solution/kg fly ash).
MSW incineration fly ash is defined as hazardous waste due to its high concentration of heavy metals, chloride salts, and dioxins (Dingwen and Anhui, 2020). The particle size of fly ash in this study was approximately 26.1 µm and within the size range commonly reported for these solids. The particle size is comparatively smaller then the range given in the literature. This might be because the fly ash sample originated from more effective incineration systems, which can consume huge char flakes and lower the size of fly ash particles. The bulk density was 0.7869 g/cm3 and the same order of magnitude as reported in the literature (Table 1). Likewise, the variance in density has been ascribed to the effectiveness of the cremation process and the efficiency of equipment collection (Ontiveros et al., 1989; Xu et al., 2014). Although they exist as fine particulates, the fly ash shows a low specific surface of 11.68 m2/g due to its non-porous nature. As shown in the SEM micrographs (Figure 2), clusters and non-porous structures were evident, with some showing cylindrical rod shapes.
The elemental composition of fly ash was analyzed using ICP-MS and SEM/EDX and the results are shown in Table 2. Significant constituents (>10,000 mg/kg) found in the MSW fly ash included mostly Al, K, Ca, Na, Cl, O, and C. The minor elements (1,000–10,000 mg/kg) in the fly ash were primarily heavy metals, such as Mg, Fe, Zn, and Pb. Some elements, such as Cu, Mn, and Sr, were found in trace amounts (<1,000 mg/kg). The MSW fly ash contained a high concentration of calcium (close to 13%). This is due to the lime spray tand flue gas scrubber treatments, which are critical for neutralizing acidic gases and reducing the degree of metal release in acidic circumstances. In addition, there was a high chloride concentration of approximately 9.5%, which might be due to the presence of polyvinyl chloride in the feedstock (Saikia, 2019). Oxygen was also detected by SEM/EDX, possibly due to the formation of metal oxides during the incineration process. Additionally, a higher amount of carbon was detected. This quantity of potentially unburned carbon is a significant source of organic pollutants. Owing to its huge adsorptive surface area, unburned carbon increases the amount of organic pollutants (Huang et al., 2003). The presence of Mn, Pb, and Zn in fly ash may be attributed to the presence of storage batteries and electrical devices in the feed source (Saikia, 2019). Toxic metals such as Ag were not detected in the fly ash.
A reasonably good agreement for fly ash elements was observed with ICP-MS and SEM/EDX. Nevertheless, there were slight differences due to the resolution limitation of the fine particles in SEM/EDX. Elements of low abundance (<0.5 %w) are less noticeable with SEM/EDX. In addition, the heterogeneity of the fly ash means there are differences between the bulk composition and surface components of the fly ash (Liu et al., 2009). The SEM/EDX technique, which is surface-sensitive, only evaluates the composition at the particle surface, whereas acid digestion provides bulk composition analyses. However, the digestion/ICP-MS approach is limited to the examination of metallic elements, whereas SEM/EDX can identify any elements with an atomic number greater than 4 (Xu et al., 2014).
The TCLP result revealed a higher Pb concentration (33 mg/L) than that authorized by the US EPA (5 mg/L) and Singapore’s National Environment Agency (NEA) (5 mg/L). As a result, fly ash might be deemed harmful. The significant leaching yield of Pb in the TCLP extract is attributable to the leachate’s high pH (above 11). Pb is more easily liberated in the acidic TCLP solution due to the alkaline ash’s strong acid neutralization capability. As a result of interaction with the extremely alkaline fly ash, the simulated acid rain TCLP fluid has become an alkaline leachate, and Pb, an amphoteric element, is leached when the pH exceeds 9.0 (Wu and Ting, 2006). This amphoteric metal forms complex ions and becomes soluble in alkaline conditions according to Equation 1:
As a result of this high leaching yield of Pb when the ash is in contact with highly alkaline leachate (Table 3), it is suggested that prior leaching of Pb in alkaline conditions should be considered (Xu et al., 2014).
The exponential phase of bacterial growth started on the sixth day of inoculation and ended on the seventh day of inoculation, after which it underwent the stationary phase until the 10th day, when the death phase started. In this experiment, it is more economically viable to obtain the spent medium at the end of the exponential phase when the bacteria would have produced the most amount of metabolites and sulphuric acid in the shortest possible number of days. This result was supported by pH reports too (Figure 3). In pH analysis, the pH of A. ferrooxidans and A. thiooxidans (pH 2 and 1.39 at day 14) was significantly reduced compared with the control (pH 2.59 and 2.2 at day 14) due to bacterial metabolites (Hallberg et al., 2010). It seems that cultures of this bacterium (A. ferrooxidans) often harbor heterotrophic contaminants, which are probably responsible for this anomaly (Schippers, 2007). Such contaminants live in symbiosis with A. ferrooxidans, and many others are members of the genus Acidophilium (Vera et al., 2013). It has been reported that most contaminants are recalcitrant to common purification techniques (Hallberg et al., 2010). Thus, it is apparent that much of the data accumulated on this bacterium should be interpreted with some caution.
FIGURE 3. pH analysis at different immersion periods. (A) Acidithiobacillus ferrooxidans. (B) Acidithiobacillus thiooxidans.
During the electrokinetic analysis, the pH of the sample played a crucial role in the electrokinetic method. The anodic position shows an acidic condition due to the formation of H+ ions in the anodic reaction, whereas the cathodic sites show an alkaline condition (Arul Prakash et al., 2021). This condition might be favorable for the leaching of metal ions.
The average heavy metal leaching efficiencies in different bacterial media (A. thiooxidans/A. ferrooxidans) are presented in Table 4. This result reveals that K, Na, Ca, and Mg (A. thiooxidans, 85%, 47%, 92%, and 85%; A. ferrooxidans, 85%, 25%, 75%, and 74%, respectively) show higher leaching efficiencies than other metals, such as lead (Pd) and zinc (Zn). These results correlate with a previous report by Maini et al. (2000b). In the experiments using A. thiooxidans, the ACID and SM setups are more efficient in the leaching of all metals except lead (Pd) and selenium (Se) compared with the DI setup; this is due to the fact that the metals solubilize more easily in the acidic conditions created by the biochemically and chemically enhanced fly ash. Hence, it can be concluded that there is no added effect from the metabolites of A. thiooxidans.
However, A. ferrooxidans remarkably improved the leaching efficiency of Al, Zn, Pb, and Mg (46%, 67% 11%, and 55%) respectively (Figure 4), which might due to the higher concentration of sulphuric acid (0.0289M) produced by A. ferrooxidans. It can be concluded that bio-enhanced electrokinetic remediation is more efficient than chemically enhanced electrokinetic remediation (Mouna et al., 2021). Thus, it cannot be concluded that the bacterial metabolites of the spent medium have the ability to increase the efficiency of electrokinetic remediation. This integrated method can be applied to remediate the fly ash contaminated soil in an ecofriendly manner. Further studies are required to determine the cost-effectiveness of using bacteria-produced sulphuric acid, taking into account the cost of the medium, batch culture time, and any waste processing cost.
FIGURE 4. Different heavy metal leaching efficiencies of fly ash for Acidithiobacillus thiooxidans and Acidithiobacillus ferrooxidans.
The TCLP results are shown in Table 5. In the presence of A. thiooxidans, the results showed that lead concentrations decreased for all three setups; the concentrations for the ACID and DI setups decreased below the US EPA and NEA requirements. However, the concentrations of Cr, Mn, Zn, Ni, and Cu increased due to the dissolution of the anode, which is made of steel, containing Cr, Mn, and Ni as its constituents. As for Zn and Cu, the dissolution of the copper wires and crocodile clips from the connections could have added to the anode compartment. These metals may have formed complexes and migrated toward the substrate compartment, passing through the anion exchange membrane. A more worrying fact is that Zn, Ni, and Cr far exceeded the US EPA and NEA requirements. In the presence of A. ferrooxidans, Pb toxicity decreased for the SM setups, possibly due to metal leaching (Mouna et al., 2021).
This study investigated the integrated approach of bioleaching-enhanced electrokinetic remediation of fly ash from MSW. Enhanced remediation of 85%, 47%, 92%, 85%, 46%, 67% 11%, and 55% for K, Na, Ca, Mg, Al, Zn, Pb, and Mg, respectively, was observed in the presence of A. ferrooxidans. The bacterial metabolites were found to play a crucial role in the remediation and bioleaching. The toxicity assay result reveals that lead (Pd) concentration was negative after the electrochemical remediation. Hence, this integrated bioleaching-enhanced electrokinetic approach can be used for heavy metal removal and is an efficient eco-friendly process for the remediation of fly ash with the intention of safe release into the environment.
The original contributions presented in the study are included in the article/supplementary material, further inquiries can be directed to the corresponding authors.
JN: Methodology, Writing–original draft. KS: Writing–review and editing. BD: Software, Writing–review and editing. AR: Supervision, Validation, Writing–review and editing. RRk: Validation, Writing–review and editing. RRm: Validation, Writing–review and editing. TM: Validation, Writing–review and editing.
The author(s) declare that no financial support was received for the research, authorship, and/or publication of this article.
The authors acknowledge Researchers Supporting Project (RSP 2023R465), King Saud University, Riyadh, Saudi Arabia, for funding this research. We also thank the Department of Science and Technology, Government of India, for the award of National Postdoctoral Fellow (PDF/2022/001253).
The authors declare that the research was conducted in the absence of any commercial or financial relationships that could be construed as a potential conflict of interest.
All claims expressed in this article are solely those of the authors and do not necessarily represent those of their affiliated organizations, or those of the publisher, the editors and the reviewers. Any product that may be evaluated in this article, or claim that may be made by its manufacturer, is not guaranteed or endorsed by the publisher.
Abbas, R., Khereby, M. A., Ghorab, H. Y., and Elkhoshkhany, N. (2020). Preparation of geopolymer concrete using Egyptian kaolin clay and the study of its environmental effects and economic cost. Clean Technol. Environ. Policy 22, 669–687. doi:10.1007/s10098-020-01811-4
Acar, Y. B., and Alshawabkeh, A. N. (1993). Principles of electrokinetic remediation. Environ. Sci. Technol. 27 (13), 2638–2647. doi:10.1021/es00049a002
Arul Prakash, A., Prabhu, N. S., Rajasekar, A., Parthipan, P., AlSalhi, M. S., Devanesan, S., et al. (2021). Bio-electrokinetic remediation of crude oil contaminated soil enhanced by bacterial biosurfactant. J. Hazard. Mater. 405, 124061. doi:10.1016/j.jhazmat.2020.124061
Bosshard, P. P., Bachofen, R., and Brandl, H. (1996). Metal leaching of fly ash from municipal waste incineration by Aspergillus Niger. Environ. Sci. Technol. 30 (10), 3066–3070. doi:10.1021/es960151v
Cameselle, C., Gouveia, S., Eddine, D., and &Belhadj, B. (2013). Advances in electrokinetic remediation for the removal of organic contaminants in soils. InTech. doi:10.5772/54334
Castro, L., Blázquez, M. L., González, F., and Muñoz, J. Á. (2021). Biohydrometallurgy for rare earth elements recovery from industrial wastes. Molecules 26 (20), 6200. doi:10.3390/molecules26206200
Chan, C. C., and Chau, K. T. (1997). An overview of power electronics in electric vehicles. IEEE Trans. Industrial Electron. 44 (1), 3–13. doi:10.1109/41.557493
Chiang, P. C., and Pan, S. Y. (2017). “Fly ash, bottom ash, and dust,” in Carbon dioxide mineralization and utilization (Singapore: Springer), 253–264.
Dingwen, Z., and Anhui, W. (2020). Review on the properties and engineering application of geopolymer cementitious materials. Chin. J. Build. Sci. Eng. 37 (05), 13–38.
Hallberg, K. B., González-Toril, E., and Johnson, D. B. (2010). Acidithiobacillusferrivorans, sp. nov.; facultatively anaerobic, psychrotolerant iron-and sulfur-oxidizing acidophiles isolated from metal mine-impacted environments. Extremophiles 14 (1), 9–19. doi:10.1007/s00792-009-0282-y
Hong, Y. Y., Morris, M. W., Chiu, C. Y., and Benet-Martinez, V. (2000). Multicultural minds: A dynamic constructivist approach to culture and cognition. Am. Psychol. 55 (7), 709–720. doi:10.1037/0003-066x.55.7.709
Huang, Y., Takaoka, M., and Takeda, N. (2003). Removal of unburned carbon from municipal solid waste fly ash by column flotation. Waste Manag. 23 (4), 307–313. doi:10.1016/s0956-053x(02)00069-7
Jaworek, A., Sobczyk, A. T., Krupa, A., Marchewicz, A., Czech, T., and Śliwiński, L. (2019). Hybrid electrostatic filtration systems for fly ash particles emission control.A review. Sep. Purif. Technol. 213, 283–302. doi:10.1016/j.seppur.2018.12.011
Krebs, W., Brombacher, C., Bosshard, P. P., Bachofen, R., and Brandl, H. (1997). Microbial recovery of metals from solids. FEMS Microbiol. Rev. 20 (3-4), 605–617. doi:10.1111/j.1574-6976.1997.tb00341.x
Liu, Y., Zheng, L., Li, X., and Xie, S. (2009). SEM/EDS and XRD characterization of raw and washed MSWI fly ash sintered at different temperatures. J. Hazard. Mater. 162 (1), 161–173. doi:10.1016/j.jhazmat.2008.05.029
Liu, F., Liu, H., Yang, N., and Wang, L. (2021). Comparative study of municipal solid waste incinerator fly ash reutilization in China: Environmental and economic performances. Resour. Conservation Recycl. 169, 105541. doi:10.1016/j.resconrec.2021.105541
Maini, G., Sharman, A. K., Sunderland, G., Knowles, C. J., and Jackman, S. A. (2000a). An integrated method incorporating sulfur-oxidizing bacteria and electrokinetics to enhance removal of copper from contaminated soil. Environ. Sci. Technol. 34 (6), 1081–1087. doi:10.1021/es990551t
Maini, G., Sharman, A. K., Knowles, C. J., Sunderland, G., and Jackman, S. A. (2000b). Electrokinetic remediation of metals and organics from historically contaminated soil. J. Chem. Technol. Biotechnol. Int. Res. Process, Environ. Clean Technol. 75 (8), 657–664. doi:10.1002/1097-4660(200008)75:8<657::aid-jctb263>3.0.co;2-5
Mouna, H. M., Baral, S. S., and Mohapatra, P. (2021). Leaching of metals from spent fluid catalytic cracking catalyst using Acidothiobacillusferrooxidans and comparing its leaching efficiency with organic and inorganic acids. J. Environ. Chem. Eng. 9 (5), 105522. doi:10.1016/j.jece.2021.105522
Nagib, S., and Inoue, K. (2000). Recovery of lead and zinc from fly ash generated from municipal incineration plants by means of acid and/or alkaline leaching. Hydrometallurgy 56 (3), 269–292. doi:10.1016/s0304-386x(00)00073-6
Narenkumar, J., Elumalai, P., Subashchandrabose, S., Megharaj, M., Balagurunathan, R., Murugan, K., et al. (2019). Role of 2-mercaptopyridine on control of microbial influenced corrosion of copper CW024A metal in cooling water system. Chemosphere 222, 611–618. doi:10.1016/j.chemosphere.2019.01.193
Ontiveros, J. L., Clapp, T. L., and Kosson, D. S. (1989). Physical properties and chemical species distributions within municipal waste combuster ashes. Environ. Prog. 8 (3), 200–206. doi:10.1002/ep.3300080319
Pradhan, N., Nathsarma, K. C., Rao, K. S., Sukla, L. B., and Mishra, B. K. (2008). Heap bioleaching of chalcopyrite: A review. Miner. Eng. 21 (5), 355–365. doi:10.1016/j.mineng.2007.10.018
Rodrigo, M. A., Oturan, N., and Oturan, M. A. (2014). Electrochemically assisted remediation of pesticides in soils and water: A review. Chem. Rev. 114 (17), 8720–8745. doi:10.1021/cr500077e
Saikia, N. (2019). “Recycling of industrial and municipal solid wastes in cement-based applications,” in Advances in waste management (Singapore: Springer), 17–30.
Schippers, A. (2007). “Microorganisms involved in bioleaching and nucleic acid-based molecular methods for their identification and quantification,” in Microbial processing of metal sulfides (Dordrecht: Springer), 3–33.
Sushil, S., and Batra, V. S. (2006). Analysis of fly ash heavy metal content and disposal in three thermal power plants in India. Fuel 85 (17-18), 2676–2679. doi:10.1016/j.fuel.2006.04.031
Tammaro, M., Rimauro, J., Fiandra, V., and Salluzzo, A. (2015). Thermal treatment of waste photovoltaic module for recovery and recycling: Experimental assessment of the presence of metals in the gas emissions and in the ashes. Renew. Energy 81, 103–112. doi:10.1016/j.renene.2015.03.014
Theis, T. L., and Gardner, K. H. (1990). Environmental assessment of ash disposal. Crit. Rev. Environ. Sci. Technol. 20 (1), 21–42. doi:10.1080/10643389009388388
Vera, M., Schippers, A., and Sand, W. (2013). Progress in bioleaching: Fundamentals and mechanisms of bacterial metal sulfide oxidation—part A. Appl. Microbiol. Biotechnol. 97, 7529–7541. doi:10.1007/s00253-013-4954-2
Virkutyte, J., Sillanpää, M., and Latostenmaa, P. (2002). Electrokinetic soil remediation—Critical overview. Sci. total Environ. 289 (1-3), 97–121. doi:10.1016/s0048-9697(01)01027-0
Wang, S. Y., and Vipulanandan, C. (1996). Leachability of lead from solidified cement-fly ash binders. Cem. Concr. Res. 26 (6), 895–905. doi:10.1016/0008-8846(96)00070-1
Wu, H. Y., and Ting, Y. P. (2006). Metal extraction from municipal solid waste (MSW) incinerator fly ash—chemical leaching and fungal bioleaching. Enzyme Microb. Technol. 38 (6), 839–847. doi:10.1016/j.enzmictec.2005.08.012
Xu, T. J., and Ting, Y. P. (2004). Optimisation on bioleaching of incinerator fly ash by Aspergillus Niger–use of central composite design. Enzyme Microb. Technol. 35 (5), 444–454. doi:10.1016/j.enzmictec.2004.07.003
Xu, T. J., Ramanathan, T., and Ting, Y. P. (2014). Bioleaching of incineration fly ash by Aspergillus Niger–precipitation of metallic salt crystals and morphological alteration of the fungus. Biotechnol. Rep. 3, 8–14. doi:10.1016/j.btre.2014.05.009
Keywords: fly ash, A.ferrooxidans, municipal solid waste, electro-kinetic, integrated approach
Citation: Narenkumar J, Sathishkumar K, Das B, Rajasekar A, Rajakrishnan R, Rajamohan R and Malik T (2023) An integrated approach of bioleaching-enhanced electrokinetic remediation of heavy metals from municipal waste incineration fly ash using Acidithiobacillus spp. Front. Environ. Sci. 11:1273930. doi: 10.3389/fenvs.2023.1273930
Received: 07 August 2023; Accepted: 05 September 2023;
Published: 06 October 2023.
Edited by:
Yujie Yan, University of Exeter, United KingdomReviewed by:
Manuel Cánovas, Catholic University of the North, ChileCopyright © 2023 Narenkumar, Sathishkumar, Das, Rajasekar, Rajakrishnan, Rajamohan and Malik. This is an open-access article distributed under the terms of the Creative Commons Attribution License (CC BY). The use, distribution or reproduction in other forums is permitted, provided the original author(s) and the copyright owner(s) are credited and that the original publication in this journal is cited, in accordance with accepted academic practice. No use, distribution or reproduction is permitted which does not comply with these terms.
*Correspondence: Tabarak Malik, bWFsaWtpdHJjQGdtYWlsLmNvbQ==; Jayaraman Narenkumar, bmFyZW5jaGVycnk3N0BnbWFpbC5jb20=; Rajaram Rajamohan, cmFqbW9oYW5hdUB5dS5hYy5rcg==
Disclaimer: All claims expressed in this article are solely those of the authors and do not necessarily represent those of their affiliated organizations, or those of the publisher, the editors and the reviewers. Any product that may be evaluated in this article or claim that may be made by its manufacturer is not guaranteed or endorsed by the publisher.
Research integrity at Frontiers
Learn more about the work of our research integrity team to safeguard the quality of each article we publish.