- 1Department of Soil Science, Patuakhali Science and Technology University, Patuakhali, Bangladesh
- 2Centre for River and Coastal Engineering (CRCE), Universiti Teknologi Malaysia (UTM), Johor Bahru, Malaysia
- 3Department of Water and Environmental Engineering, Faculty of Civil Engineering, Universiti Teknologi Malaysia (UTM), Johor Bahru, Malaysia
- 4Department of Disaster Management, Begum Rokeya University, Rangpur, Bangladesh
- 5Department of Development Studies, Daffodil Internaitonal University, Dhaka, Bangladesh
- 6Institute of Nuclear Science and Technology, Bangladesh Atomic Energy Commission, Dhaka, Bangladesh
- 7Department of Horticulture, Bangabandhu Sheikh Mujibur Rahman Agricultural University (BSMRAU), Gazipur, Bangladesh
- 8Department of Environmental Science and Resource Management, Mawlana Bhashani Science and Technology University, Tangail, Bangladesh
- 9Hydrobiology and Fisheries Research Unit, Department of Zoology, Nnamdi Azikiwe University, Awka, Nigeria
- 10Hydrobiology / Aquatic Sciences Research Unit, Department of Zoology and Environmental Biology, University of Nigeria, Nsukka, Nigeria
- 11Department of Biology, College of Science, King Khalid University, Abha, Saudi Arabia
- 12Center for Environment and Tourism Studies and Research, King Khalid University, Abha, Saudi Arabia
- 13Department of Chemistry, College of Science, King Khalid University, Abha, Saudi Arabia
This work assesses the contamination of trace elements (Cr, Cu, Ni, As, Zn Cd, Mn, Fe, and Pb) in soil and different tissues of the banana plant (Musa spp.), the ecological risks of trace elements using various indices, and the probable health risks using a chemometric approach. Soil and different banana plant tissues were collected from banana fields around the industrial area of the capital of old Pundranagar (the earliest urban archaeological location), Bangladesh. Samples were digested by acid digestion, and trace elements were measured by inductively coupled plasma spectrophotometer (ICP-MS). The concentrations of Cr, Ni, Cu, As, Cd, Pb, Fe, Mn, and Zn in soil ranged from 1.50–61.7, 2.42–87.4, 2.00–100.8, 0.25–31.2, 0.10–12.7, 0.60–91.8, 11330–23782, 8.69–105.9, and 7.50–125.9 mg/kg, respectively. The mean concentrations of trace elements in four tissues descend in order of roots > leaves > stems > fruits. The abundances of trace elements varied in both soil and plant samples, which apparently occurred due to the variations of soil parent materials and the excessive use of agrochemicals for long-term banana cultivation. The soil exhibited a moderate to high degree of contamination with trace elements, and Cr, Pb, Zn, Cd, and As mainly originated from anthropogenic sources. Both non-cancer and cancer risks were perceived due to Cr and As exposure from the fruit tissue of banana plants in the study area.
Introduction
The banana plant (Musa spp.) is the fourth most important crop in the world after rice, wheat, and maize, and one of the most important horticulture crops in Bangladesh (FAO, 2017). Around the world, bananas are regarded as nutrients-dense fruits. They are grown in many tropical areas where they can be utilized as nutritional complements. Different tissues of the banana plant, including leaves, roots, stems, fruits, and flowers, have been used as pest control for the management of insect bites in the agricultural fields and as a medicinal treatment for new injuries (Onyenekwe et al., 2013). The banana fruit is an important component of the human diet because it supplies nutrients such as potassium, calcium, phosphorus, iron, and vitamins C and E (Kennedy, 2009; Kumar et al., 2012). It also contains cancer chemoprevention activities that could potentially provide human health benefits (Falcomer et al., 2019; Oliveira et al., 2020). Several banana plant tissues, including leaves, stems, fruits, and roots, are used to make ropes, candy, feed for fish and poultry, vermicompost, and fabrics; they are also used in religious ceremonies (Mohiuddin et al., 2014). Bangladesh ranks as number 14 among the top 20 banana-producing nations in the world, and its annual production is approximately 1.0 million tons (Hossain, 2014). Banana plants are highly adaptable to intercropping and diversified agricultural practices and can thrive in various environmental and soil conditions (Israeli and Lahav, 2017).
However, pests and diseases infest banana plants due to the long-term cultivation of bananas on the same land, reducing soil fertility and productivity. To overcome these situations, farmers must apply different chemical fertilizers and pesticides (Lin et al., 2010). Because a banana tree takes many nutrients to develop and thrive, it is critical to give it the fertilizer it demands. A balanced fertilizer is one of the best fertilizers for banana plants because it provides all elements that the banana plant requires to grow strong and healthy. Nitrogen (N), phosphorus (P), and potassium (K) are the primary macronutrients, and the requirement of NPK fertilizer compounds is 150, 90, and 300 g/plant/year (Fonsah et al., 2017). The secondary macronutrients are calcium, magnesium, and sulfur. Micronutrients are elements required in small quantities. Despite being needed in small quantities, micronutrients are essential for the overall performance and health of the banana plant. They include iron, manganese, zinc, copper, molybdenum, and boron. In addition, the banana field’s soil may have been contaminated by trace elements (Mohamed et al., 2017; Sahodaran and Ray, 2018). Studies have shown that different banana plant tissues have the potential to accumulate trace elements such as Cu, Co, Fe, Ni, and Zn and toxic elements, such as Cd and Pb, from the soil around industrial areas (Flores et al., 2018). Romero-Estevez et al. (2019) specified that increased toxic element concentrations, especially Cd, As, Ni, and Pb, pose a significant risk to consumer health, especially children. Their presence in legumes, fruits, vegetables, rice, and other foods is also shaped by a variety of variables, including contamination, fertilizers, climate, and the type and physicochemical characteristics of the soil (Ghazali et al., 2012; Marles, 2017; Stambulska et al., 2018; Islam et al., 2021a). Previous studies (Miedico et al., 2017; Bawuro et al., 2018; Kasozi et al., 2018; Islam et al., 2021a; 2022a) demonstrated that a considerable number of toxic metals (Cd, Pb, and As) and essential metals, such as Cu and Zn, have the potential for toxicity to humans and are present in animals, aromatic plants (Dghaim et al., 2015), and other types of foodstuffs (Islam et al., 2022b; Mizan et al., 2023). Therefore, it is very important to assess the trace elements in foods, especially bananas, to maintain their quality for use as a food product (FAO, 2019).
Soil is a natural resource that is needed for all human activities, especially producing crops. In the industrialized urban environment of the earth, surface soil acts as the major receiver of various contaminants, especially trace metal(loid)s (Zabir et al., 2016; Kormoker et al., 2019). Excess accumulation of trace metal(loid)s in surface soil has a destructive impact on the terrestrial ecosystem and thereby poses threats to its inhabitants (Mallick et al., 2019). If a banana plant is cultivated on contaminated soil containing trace toxic elements, it may pose significant risks to human health due to the ingestion of fruits or other parts of the plant (Flores et al., 2018). Continuous banana cultivation on the same land is responsible for trace element accumulation in soil and nearby plants and could potentially cause deterioration of the soil ecology. Hence, research on the possible impact of trace element pollution in the surface soil of banana fields on the environment and public health is important for making policies to reduce pollution and improve soil function.
Bioconcentration factor (BCF) and translocation factor (TF) are index methods employed by researchers to establish the connection between metals in the topsoil used for long-term banana cultivation and their accumulation in various tissues of plants, (Yoon et al., 2006; Igwe et al., 2014; Islam et al., 2021b). There should be concern about the ecotoxicological status of soil used for banana cultivation and human health hazards due to trace element contamination in different banana plant tissues. Methods like the contamination factor (CF), the enrichment factor (EF), the geoaccumulation index (Igeo), the pollution load index (PLI), and the Nemerow pollution (PN) index have been established to assess the contamination level of trace elements in the surface soil of banana fields (Liu et al., 2014; Kormoker et al., 2019). In the current study, surface soil has been considered for trace element analysis because of quick and cost-effective methods of soil sampling that provide reliable information on a wide variety of habitats and storage of bulk contaminants of soil–plant ecosystems (Ravankhah et al., 2016; Proshad et al., 2017). Consequently, the analysis and source identification of trace elements in soil for long-time banana cultivation should be seriously considered to ascertain the degree of pollution, the ecological risk of trace elements in the soil of banana fields, and the formulation of remediation approaches. Numerous methods have been adopted to evaluate health risks. Target cancer risk (TCR) is utilized for carcinogenic risk estimation, while target hazard quotients (THQs) are often predicated on the levels of trace elements in the edible portions in relation to the required dose of the element acceptable by the body (USEPA, 2010).
Islam et al. (2021b) showed that vegetables grown near a waste facility in an urban area of Bangladesh are a major source of toxic substances that cause potential risks to human health. There should be concern about the long-term cultivation of bananas in the urban soil around the ancient city of Pundranagar, Bangladesh. Excessive metal accumulation in soil and plants can cause public health defects. No systematic studies have been conducted to assess the levels of trace elements in the soil used for long-term banana cultivation, nor assesshuman health risks due to the exposure of trace elements from different tissues of banana plants. The biological danger to topsoil from the introduction of trace metals and the risk accompanying the consumption of contaminated banana fruit are sources of concern. The extent of health hazard consequences, ecological risk, and exposure assessments were conducted to explain the effects of soil adulteration by trace components due to long-term banana cultivation. Therefore, the current study hypothesized that long-term cultivation of bananas in the same field has a potential effect on trace element buildup in topsoil as well as various tissues of plants. The current study is the first to evaluate the level of trace elements in surface soil and the ecological risk of trace elements posed by long-term banana cultivation, to observe the accumulation of trace elements using the BCF in various banana plant tissues, and to evaluate the potential health risks for the inhabitants living close to the industrial area of the ancient capital city of Pundranagar, Bangladesh.
Materials and methods
Study area and sampling
The industrial section of the ancient city of historical Pundranagar, Bangladesh, and the farmlands used for long-term banana farming were chosen as the study sites (Figure 1). We selected some virgin fields in the study region to compare the consequences of longstanding banana cultivation on the physicochemical properties of topsoil. The selected site of the current study is one of the earliest urban archaeological locations in Bangladesh, known as Paundravardhanapura or Pundranagara, in the region of Pundravardhana (Hossain, 2006). Pundranagara, a significant city of the Maurya Empire, was occupied until the eighth century. The economy of Bangladesh in the study area comprises various activities, such as agrarian activities, manufacturing events like convenience garments, poultry feed production, food packaging, and agrochemical industries (Zakir et al., 2013). During sampling, we observed that farmers have been using untreated wastes from various industries and chemical fertilizers/pesticides in the banana fields for 10 years or more. A number of surface soil samples (51) were taken at a 0–20 cm depth, and 56 different stem, root, leaf, and fruit tissue samples were collected during January–June 2019. Triplicate plant specimens of the banana (Musa spp.) species were randomly selected from the banana fields. The plant tissues and soil samples (approximately 500 g each) were placed in polyethylene bags, carefully labeled, and brought to the lab, where they were dried in the sun for a few days. To achieve the constant weight, samples were then ground, homogenized, and dried for 72 h at 65°C in the oven. Before the chemical analysis, the prepared soil and plant specimens were preserved hermetically in clean Ziploc bags in a refrigerator (4°C).
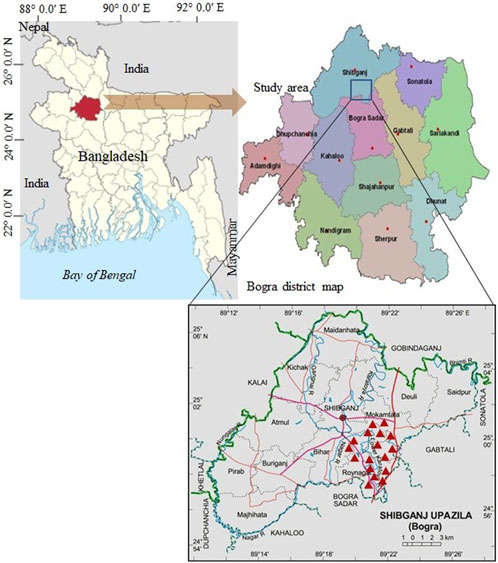
FIGURE 1. Study area map for soil samples from banana fields located around the industrial area of the capital of ancient Pundranagar, Bangladesh.
Sample analysis for trace elements
Trace elements in soil and plant specimens were analyzed in the Laboratory of Plant Nutrition and Fertilizer, using inductively coupled plasma mass spectrometry (ICP-MS, 7800, Agilent Technologies). Approximately 2–4 mg of plant tissues and 3.0 g of dry soil samples were digested by adding 4.0 mL of 65% HNO3, 1.0 mL concentrated HCl, and 2 mL of 30% H2O2 (Wako Chemical Co., Japan), using a microwave digesting apparatus with a Teflon tube (Kühnlenz et al., 2016). A standard solution XSTC-13 (Spex CertiPrep® USA) was employed (R2 > 0.999) to create the calibration plot. Indium, yttrium, beryllium, telium, cobalt, and titanium (1.0 mg/L) were used as internal standards. Ultrapure-grade 5% (v/v) HNO3 was used for sample preparation and dilution. A similar process was followed to verify the batch-specific accuracy throughout the experimental performance; triple measurement protocol blanks were run and verified using Japanese-certified reference material (NMIJ CRM 7303-a) and Polish-certified reference material (INCT-CF-3) of corn flour (Supplementary Table S1).
Data calculation
Evaluation of metal accumulation and bioavailability
The BCF is widely used to measure the extent to which a living organism (plants and animals) absorbs any element from its environment and transfers it to tissues in the organism. Generally, a BCF value of less than or equal to 1 (BCF ≤1.0) suggests that the organism can uptake trace elements in its environmental compartments (soil, air, or water), but those elements only accumulate in their tissues in a minor quantity. However, a BCF value above 1 (BCF >1.0) implies a considerable accumulation of trace elements in tissues (Liu et al., 2009). The BCF of trace elements in the different banana plant tissues was expressed following the method of Islam et al. (2018):
where Croot/stem/fruits/leaves stands for the trace element contents (mg/kg dw) in banana roots, stems, fruits, and leaves, while Csoil is the measured trace element value in the soil collected from the banana field (Islam et al., 2018).
Ecological risk assessment of trace elements
Contamination factor
To calculate the CF of studied trace elements, the concentration of each element in the soil of the target sites of the banana field was divided by the background value selected from the background samples at the research site:
where CF indicates a contamination factor, Celement is the abundance of trace elements in the soil sample, and Cbackground is the background abundance of trace elements in preindustrial soil samples. The background abundances of Cr, Ni, Cu, As, Cd, Pb, Fe, Mn, and Zn were 3.07, 3.28, 1.99, 1.19, 0.31, 2.09, 11570, 18.97, and 7.77 mg/kg, respectively. For the background sample, a soil sample was collected using a percussion hammer corer (Schottler and Engstrom, 2006). Lead-210 dating by alpha spectrometry was employed to understand the levels of trace elements in the background sample before the industrial or other anthropogenic effects based on the assumption of the “pre-industrialization” state (Islam M. S. et al., 2015). Håkanson (1980) classified four grades of CF for trace element monitoring in any contaminated area over a certain period (Supplementary Table S2).
Degree and modified degree of contamination
The degree of contamination (Cd) and modified degree of contamination (mCd) in soil were investigated using the following formulae developed by Håkanson (1980). The Cd and mCd were determined as follows:
where CF is the contamination factor and n is the total number of trace elements analyzed in soil samples. Håkanson (1980) proposed four grades, and Abrahim and Parker (2008) proposed seven grades of degree of contamination (Cd) to explain the modified degree of contamination (mCd) for trace elements in soil (Supplementary Table S2).
Pollution load index
The PLI, which is calculated as the following CF multiplication of the trace elements, is known as the nth root:
Based on the value of PLI, Sadhu et al. (2012) classified three grades of soil due to trace elements contamination in soil (Supplementary Table S2).
Geoaccumulation index
For contamination assessment, the geoaccumulation index (Igeo) has been calculated as follows:
where Cn is the determined abundance of trace element “n” in the soil sample and Bn is the baseline value of element “n” in the background soil sample. A factor of 1.5 is applied to reduce any fluctuations in the background values caused by lithogenic influences. Müller (1981) interpreted seven classes of Igeo for trace elements in soil (Supplementary Table S2).
Enrichment factor
The magnitude of trace elements in any contaminated environment can be assessed by the enrichment factor (EF) (Franco-Uria et al., 2009). For the present study, the Fe value from each field site was used for trace element normalization and calculated as follows:
The ratio of the trace elements in the examined sample (Cn) to the element in the background is standardized by normalizing the amount of the element in the soil and background to the amount of Fe (mg/kg) in the soil and background (Islam et al., 2022a). Birch and Olmos (2008) classified seven CF groups presented in Supplementary Table S2.
Nemerow pollution index
The Nemerow pollution index (PN) is utilized to evaluate the comprehensive status of soil due to trace element contamination (Islam et al., 2022a). Natural changes of trace elements can be seen in the soil environment at any particular location; PN differentiates from other pollution assessment indices in that it considers both the maximum and average abundances of trace elements under consideration. It is calculated as follows:
where CF indicates the mean contamination factor value of trace elements in the soil under investigation and CFmax is the highest CF for a specific element in a specific soil sample (Chen, 2010).
Mean effects range median quotient
The mean effects range median quotient (MERM-Q) of trace elements can be calculated by the following equation:
where Cn is the concentration of the metal in the soil sample, and the ERM values for Cr, Ni, Cu, As, Cd, Pb, Fe, Mn, and Zn are 370, 52, 270, 70, 10, 218, 15000, 550, and 410, respectively (Islam et al., 2022b).
Potential ecological risk
The potential ecological risk index (RI) was used to evaluate the degree of trace element contamination of the examined soil that can be used for long-term banana cultivation, and the calculation procedure is as follows:
where
Health risk assessment
Daily intake of trace elements
The estimated daily intakes (EDIs) of trace elements (mg/kg-bw/day) were computed based on elemental concentration in banana fruits, daily consumption, and the average body weight of the occupants. The calculation procedure is as follows:
where FIR indicates banana intake (g/person/day), C is the value of the analyzed trace elements [mg/kg, fresh weight (fw)], and BW is the average body weight (60 kg for adults and 16 kg for a child; FAO, 2006). The daily intake rates of banana fruit were given as 50.59 g and 25.33 g for adults and children, respectively (HIES, 2017).
Non-carcinogenic and carcinogenic risks
The THQ and target carcinogenic risk (TCR) were applied to assess the non-carcinogenic and carcinogenic health threats associated with banana fruit intake (USEPA, 1989). THQ can be measured as follows:
where EFr is the exposure frequency (365 days/year), ED is the exposure duration (assuming 70 years) (USEPA, 1991), RfD indicates oral reference dose (mg/kg/day), and AT is the averaging time for non-carcinogens (365 days/year × number of exposure years, assuming 70 years). The oral reference doses were 1.5, 0.02, 0.04, 0.0003, 0.003, 0.004, 0.7, 0.14, and 0.3 mg/kg/day for Cr, Ni, Cu, As, Cd, Pb, Fe, Mn, and Zn, respectively (USEPA, 2010). Following USEPA (1989), the TCR was estimated using Eq. 14:
where CSFo represents the oral carcinogenic slope factors 0.5 and 1.5 (mg/kg/day)−1 for Cr and As, respectively (USEPA, 2010).
Statistical analysis
The data were evaluated using the statistical package SPSS 20.0 (SPSS, USA). Nine trace elements in the soil of banana farms were chosen, and their means, standard deviations, variances, relative standard deviations, kurtosis, and skewness were computed. Principal component analysis (PCA) was used to identify potential pathways or origins of the trace elements by measuring the variable’s average value. The isolated component’s eigenvalue and loading value were greater than 1 and 0.5, respectively. Statistical analysis, such as analysis of variance (ANOVA), was carried out to check the variability of metal concentration in soil and banana tissues. We applied bivariate correlations analysis with a Tukey post hoc test to verify significant differences in metal concentrations in soil and different tissues of banana plants among sampling sites. Microsoft Excel 2010 was also used for other calculations.
Results and discussion
Descriptive statistics of trace elements in soil
Table 1 and Supplementary Figure S1 show the descriptive statistics of Cr, Cu, Ni, As, Pb, Cd, Fe, Mn, and Zn in the topsoil of a banana field used for long-term banana farming. There was a perceptible change in the amounts of the examined trace elements in the soil samples. Among the measured elements, the highest mean value was observed for Fe (15,390 mg/kg), followed by Zn (49.37 mg/kg). The average values of trace elements in soil samples followed the descending order of Fe > Zn > Mn > Cu > Ni > Pb > Cr > As > Cd. As highlighted in Table 1, an elevated standard deviation was observed for the evaluated trace elements in surface soil samples, which exhibited relatively substantial spatial variability. This variation might be due to the application of various metal-contaminated agrochemicals, such as diazinon, trichlorfon, carbofuran, chlorpyrifos, carbaryl, 1,3-dichloropropene, thiabendazole, atrazine, and glufosinate ammonium (Henriques et al., 1997; Dong et al., 2019; Rosa et al., 2020). There was a finding that trace element extents in banana fields were far higher than in the virgin field (Supplementary Figure S1), indicating that the application of various insecticides or pesticides and excavation in the banana fields could be the causes of the increased contaminant content in banana cultivated soil.
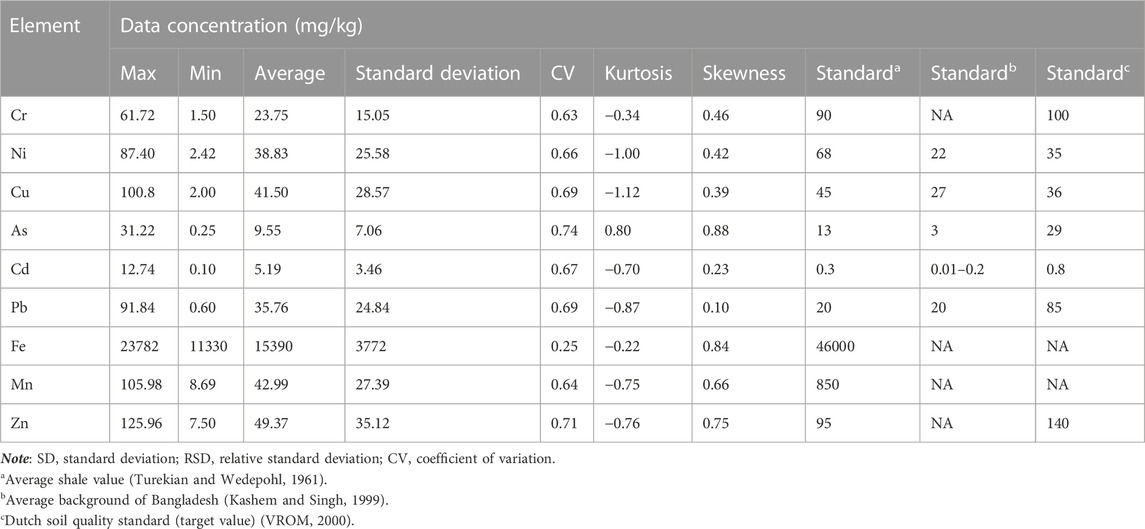
TABLE 1. Descriptive statistics for trace elements (mg/kg) in soil collected from the banana fields around the capital of ancient Pundranagar, Bangladesh, compared to the standard values.
The average extents of Cu, Cd, As, and Pb in the topsoil of banana fields in the present study were higher than the average shale values (ASV) (Turekian and Wedepohl, 1961), the BV (background value) in Bangladesh (Kashem and Singh, 1999), and the standard of Dutch soil quality (VROM, 2000), indicating their contamination in the soil and the possibility of potential ecological risk to the banana field soils. Islam et al. (2021b) conducted a study in the soils of Bangladesh, and they stated that toxic-element-contaminated waste from burning or metal processing sites of an industrial area and imbalanced use of pesticides in agricultural fields are responsible for the elevated levels of As, Cu, Pb, and Cd. This study highlighted similar reasons for elevated trace elements in the soil of banana fields around the industrial area in Bangladesh. In the current study, surprisingly an anomalous concentration of Cd was observed, which was 17.3 times higher than the average shale standard of Cd in soil (Table 1). On the earth’s surface, Cd is the most eco-poisonous component; its elevated level in farming soil is a notable concern of environmental scientists due to its adverse effect on the soil ecosystems, especially on soil flora and fauna (Ekere et al., 2020).
The coefficient of variation (CV) of As, Zn, Cu, Cd, and Pb is distinctly greater than that of other examined elements, which demonstrated that the studied trace elements, especially As, Zn, Cu, Cd, and Pb, are distributed unevenly in the surface soil of banana fields and are affected by the human activities of banana cultivation (Zhuang et al., 2021). The value of kurtosis indicates that As in the examined soil had a typical distribution and was likely due to a variety of banana cultivation activities and industries in the study area. Either a positive or negative attribute of skewness is possible where the high values of skewness for the examined trace elements confirm the present situation for banana cultivation in soil over a long period of time. The skewness values of As, Fe, Zn, and Mn were 0.88, 0.84, 0.75, and 0.66, respectively, indicating a positive skewness toward the higher concentrations in soil in the present study (Table 1). In this work, the skewness of the examined metalloids in surface soil was positive, which revealed their asymmetric distribution (Sadhu et al., 2012; Abbasi, 2019).
The concentration of trace elements in banana tissues
The quantities of Ni, Cr, Cu, Cd, As, Pb, Fe, Zn, and Mn (mg/kg dw) in the four main tissues (leaves, stems, fruits, and roots) of the banana plant are presented in Table 2. Varied abundances of trace elements were observed in the soil, which could be reflected in the considerable variations of trace elements in the examined four banana plant tissues. The average level of trace elements in the tissues of banana plants followed the descending order of roots > leaves > stems > fruits. Among the plant tissues, roots accumulate more trace elements than other parts, which may be due to their direct contact with soil. The second highest abundance of trace elements was found in banana leaves, which can be explained by the fact that the large surface area of leaves is regarded as the first recipient of contaminants from industrial emission (Liu et al., 2009; Rosa et al., 2020). An exploration of weed species plants was led by Zhao and Duo (2015), who stated that leaves accumulate higher levels of trace elements than other parts. Interestingly, Cu was detected in substantial amounts in plant leaves, possibly as a result of the chloroplasts’ photosynthetic activity, plant respiration, and assistance in the plant metabolism of carbohydrates and proteins. Because Cu regulates enzymatic activity for lignin formation, and chloroplasts are the typical component of leaves, substantial Cu buildup is anticipated in plant leaves (Islam et al., 2021b).
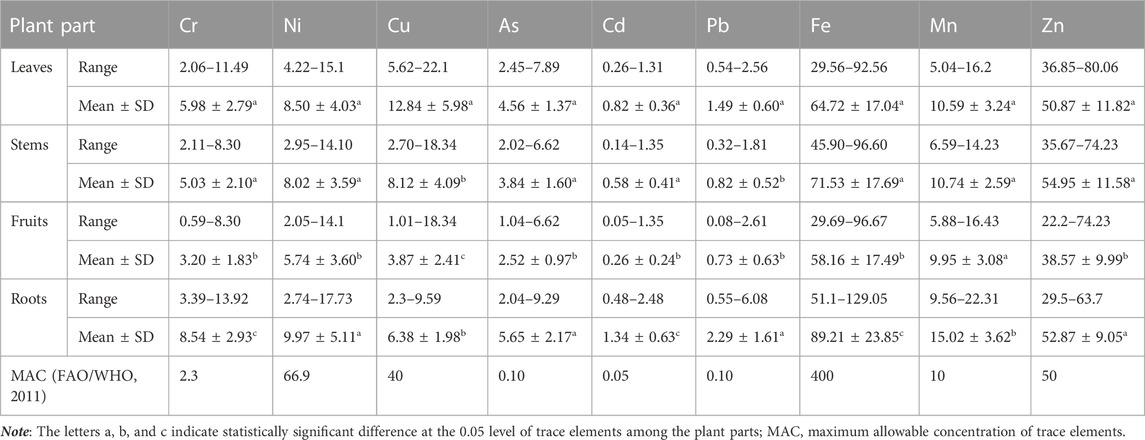
TABLE 2. Mean concentrations (mg/kg fw) of trace elements in different tissues of banana plants collected from the fields around the industrial area of the capital of ancient Pundranagar, Bangladesh (n = 3).
Mean abundances of Cr (mg/kg) were found to be 5.98 in leaves, 5.03 in stems, 2.30 in fruits, and 8.54 in roots (Table 2). Islam et al. (2015a) reported that Cr content in banana fruits in Bangladesh was 1.2 mg/kg (range 0.06–3.6 mg/kg), and Shaheen et al. (2016) also observed 0.32 mg/kg Cr in banana, which was in line with the present study. The mean concentrations of Ni, Cu, As, Cd, Pb, Fe, Mn, and Zn in leaves, stems, fruits, and roots are presented in Table 2. Significant differences were observed for trace elements in different tissues of plants, which indicated that plant tissues had different accumulation potentials for trace elements. In contrast to soil and water, food is the predominant supplier of possibly dangerous substances to humans, including Cr, Pb, Cd, and As (Ma et al., 2018; Kuerban et al., 2020). However, mean extents of Cr, As, Cd, Pb, Mn, and Zn in banana plants were higher than the permissible limits proposed by FAO/WHO (2011) (Table 2), suggesting the contamination of different banana plant tissues by those elements and potentially imposing risks to consumers. The enhanced levels of Zn, Mn, Cr, As, Pb, and Cd in banana tissues might be due to the effects of applying various wastes from the waste piles around the industrial area and imbalanced application of fertilizers, pesticides, and other agrochemicals to the banana fields (Lin et al., 2010; Ahmed et al., 2016; Rosa et al., 2020). The mean extents of trace elements in various tissues of the banana plant followed the descending order of Fe > Zn > Mn > Cu > Ni > Cr > As > Pb > Cd.
Bioconcentration of trace elements
Trace elements are absorbed from the soil by the banana plant roots and are dispersed to other parts of the plant. The calculated values of the BCF are shown in Figure 2. The BCF values of As, Cr, Cd, Fe, and Pb in roots were higher than those in stems, fruits, and leaves (Figures 2A, B), indicating that roots have more accumulation potential of these elements. This was in line with earlier research by Bonanno (2013), who reported that essential micronutrients can be easily ingested by the lower parts of plants like the roots. Although the BCF value for trace elements in fruits was relatively lower than the other three tissues, fruits could have a direct adverse health impact on the consumers who frequently consume bananas from the study sites. Considering all the examined tissues, the mean BCF values of trace elements followed in the descending order of Zn > As > Mn > Cr > Ni > Cu > Cd > Pb > Fe. Among the studied elements, the most concerning toxic elements are Cd and Pb, which showed a relatively lower BCF value, possibly due to their stable form in the soil of the cultivated field (Khairiah et al., 2009; Islam et al., 2021a) or the situation of their oxide form in the topsoil (Juen et al., 2014; Islam et al., 2022b). The BCF values of Zn in leaves, stems, fruits, and roots were 1.03, 1.16, 0.80, and 1.04, respectively, which were slightly higher than 1.0, suggesting that the bioavailability of Zn is high, and plants can easily accumulate this element from soil and disperse it to the aboveground tissues. Previous studies by Subramanian et al. (2022) and Islam M. D. et al. (2020) stated that variations in the plants’ element adsorption capacity, species of plant, the anchoring behavior of nutrients by root systems, and the prevailing ecological conditions of soil account for the variation of trace element accumulation by banana plants.
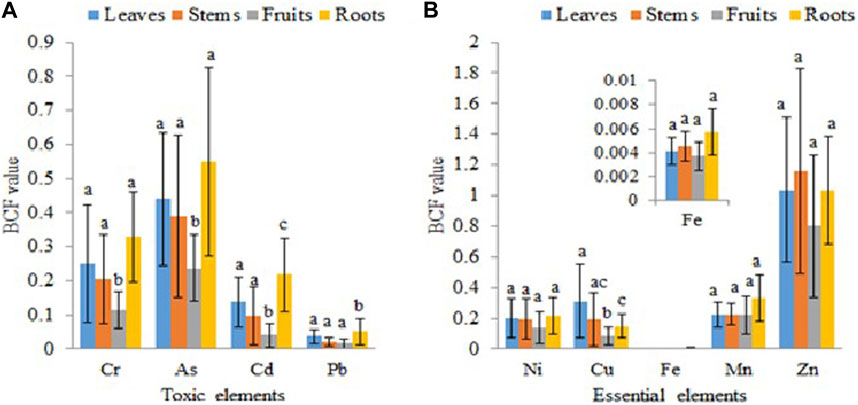
FIGURE 2. Bioconcentration factor (BCF) of toxic (A) and essential (B) elements in leaves, stems, fruits, and roots of banana plants cultivated in the capital of ancient Pundranagar, Bangladesh. Error bars represent the standard deviation (n = 3). Letters a, b, and c indicate a statistically significant difference at the 5% level.
Soil contamination assessment
The contamination of soil by trace elements was assessed by various chemometric approaches such as the geoaccumulation index (Igeo), the contamination factor (CF), the enrichment factor (EF), the pollution load index (PLI), the degree of contamination (Cd), the modified degree of contamination (mCd), the mean effects range median quotient (MERM-Q), the potential ecological risk index (PERI), and the Nemerow pollution index (PN). The data are presented in Figure 3 and Figure 4 and Supplementary Figures S2, S3. The Igeo values are presented in Figure 3. Considering the virgin and banana fields, the Igeo values are in the descending order of Cu > Cd > Pb > Ni > Cr > As > Zn > Mn > Fe (Figure 3). According to Müller (1981), Igeo values indicated that the studied soil was moderately to heavily contaminated with Cu, Cd, Pb, and Ni, whereas it was uncontaminated to moderately contaminated with Cr, As, and Zn (Figure 3). Based on Igeo values, the soil of virgin land was not contaminated by trace elements, unlike the soil of long-term banana cultivation, confirming that long-term banana cultivation practices are responsible for elevated trace elements in banana field soil.
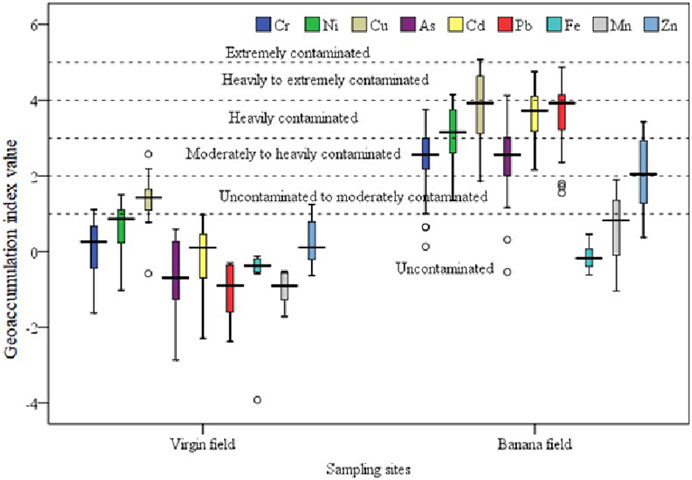
FIGURE 3. Geoaccumulation index (Igeo) of trace elements in banana field soil around the capital of ancient Pundranagar, Bangladesh. The horizontal dotted line indicates the class of the Igeo value.
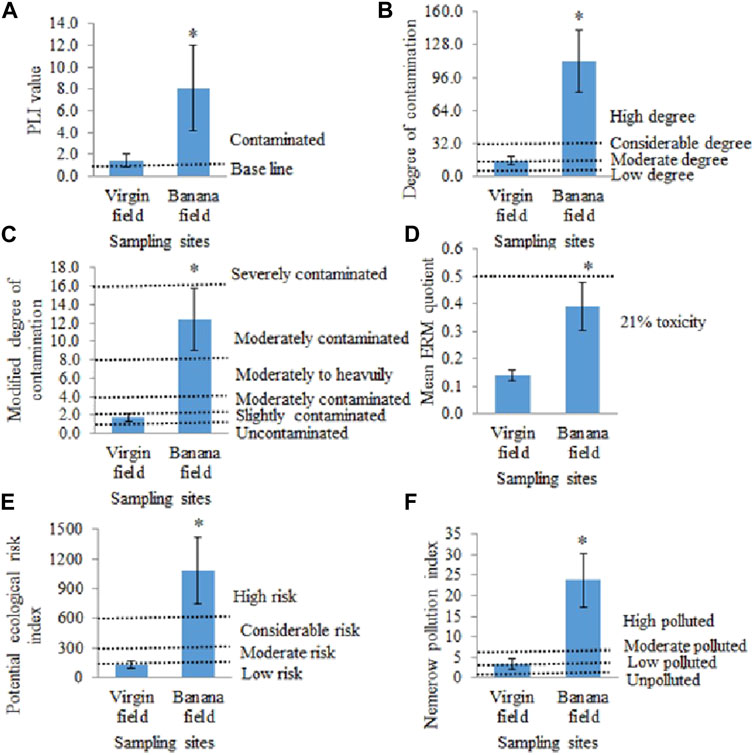
FIGURE 4. Distribution of the ecological risk index value. (A) Pollution load index (PLI), (B) degree of contamination (Cd), (C) modified degree of contamination (mCd), (D) mean effects range median quotient (MERM-Q), (E) potential ecological risk index (PERI), and (F) Nemerow pollution index (PN) due to trace elements in banana field soil around the capital of ancient Pundranagar, Bangladesh. Horizontal dotted lines indicate the level of contamination for different risk indices. “*” indicates a statistically significant difference at the 0.01 level.
EF is a tool that can categorize the natural or anthropogenic origin of trace elements (Proshad et al., 2019; Asomba et al., 2023). Trace elements were somewhat enriched in the soils of the virgin field, whereas severe to very severe modification was observed for trace elements except Fe and Mn in the banana field (Supplementary Figure S2). Altogether, the EF of the studied elements were in the descending order of Cu > Pb > Cd > Ni > As > Cr > Zn > Mn > Fe (Supplementary Figure S2). The EF value of studied trace elements in the banana field was larger than 1.5, indicating man-made activity. Industries are responsible for the high enrichment of trace elements in the surface soil of the examined positions (Islam M. S. et al., 2020; Ustaoğlu and Islam, 2020). Shirani et al. (2020) stated that anthropogenic activity accounted for the higher enrichment of Cd, Pb, Cr, and Ni in the aquatic setting of Iran, which is consistent with this study.
According to the classification system of Håkanson (1980), the examined trace elements in the soil of virgin fields demonstrated little significant pollution whereas, in banana fields, soil presented very high contamination for most of the trace elements, except for Fe and Mn, which showed moderate contamination (Supplementary Figure S3).
The PLI acts as an effective technique for pollution evaluation. A PLI of zero indicates the suitability of soil for crop production, a PLI value of 1 indicates background levels of trace elements, and PLI values greater than 1 designate the soil as contaminated by trace elements (Proshad et al., 2021). The mean values of PLI were observed as 1.49 and 8.12 for virgin and banana fields, respectively (Figure 4A), which indicated that the soils of the current study are polluted by trace elements (PLI >1). The PLI of the banana field was significantly higher than that of the virgin field, indicating the effect of long-term banana cultivation on trace element accumulation in soil. The PLI value for the virgin field was greater than one, confirming the operation of metal processing industries and open waste-burning activities in the studied area (Islam et al., 2015b).
Similar spatial patterns to PLI were observed for the degree of contamination (Cd) and modified degree of contamination (mCd), where their values in banana field samples were significantly higher than those of the virgin field samples (Figures 4B, C). The values of Cd and mCd for the virgin field samples demonstrated a low to moderate degree of trace element contamination. However, the banana field samples presented a high degree of contamination (Cd > 32 and mCd value as 8 ≤ mCd < 16) (Figures 4B, C), which could be due to the impact of agricultural operations and banana plantation cultivation methods (Lin et al., 2010; Kumar et al., 2021). The obtained values of MERM-Q for studied trace elements in surface soil showed 21% toxicity (Figure 4D). Håkanson (1980) described a potential ecological risk factor (Eri) for evaluating soil ecological hazards based on trace element contamination. The value of potential ecological risks (
Sources of trace elements
In this study, the sources of trace elements in surface soil and banana plants were identified using PCA (Figure 5). The PCA was utilized based on the profusions of trace elements in soil and plant trials with the varimax rotation technique. Three principal components accounted for the variability of trace elements in both soil and banana plants and cumulatively explained 58.58% and 60.30% in soil and plant samples, respectively (Figure 5). The first principal component (PC1) contributed 22.18% and 23.25% of the total variance in soil and plant samples, respectively, and loading with Cr, Zn, Pb, and As in soil and Zn, Cd, and Pb in plant samples (Figure 5). The sources of Cr, Zn, Pb, As, and Cd in the soil and plant samples from the study area may arise from paint flakes, leakage of oil and lubricants, waste from old scrap batteries, metal workshops, and industrial exhaust emissions (Ekere et al., 2017; Zglobicki et al., 2018; Roy et al., 2019). The second component explained 21.48% of the total variance in soil and presented positive loading for Ni, Mn, Cu, and Cd, whereas 23.021% of the total variance in plant showed positive loading with Cr, Cu, Ni, As, and Mn (Figure 5). These elements in soil and plant samples could arise from multiple origins, viz., waste burning, industrial emissions, excess use of fertilizers and pesticides in banana fields, or lithogenic sources (Pandey et al., 2012; Islam et al., 2021a). The PC3 accounted for 14.91% and 14.03% of the total variance in soil and plant samples, respectively, and presented positive loading for Fe (Figure 5). A study by Iqbal and Shah (2011) mentioned that Fe is a crucial component of the earth’s topsoil and crust and is mainly attributed to some general causes. From the PCA, we have observed diverse distributions of identical types of trace elements in soil and plant samples that may be caused by the element’s source emission characteristics as well as the variation of its accumulation in the soil and plants. The ANOVA results showed that there were significant differences in metal concentrations in soil and different banana plant tissues, whereas no significant difference was observed for Zn concentration in soil and different tissues of banana plants (Table 3). Multivariate analysis revealed that anthropogenic sources, rather than geogenic, are the leading factors which agrees with the previous demonstrations of Bhuiyan et al. (2021).
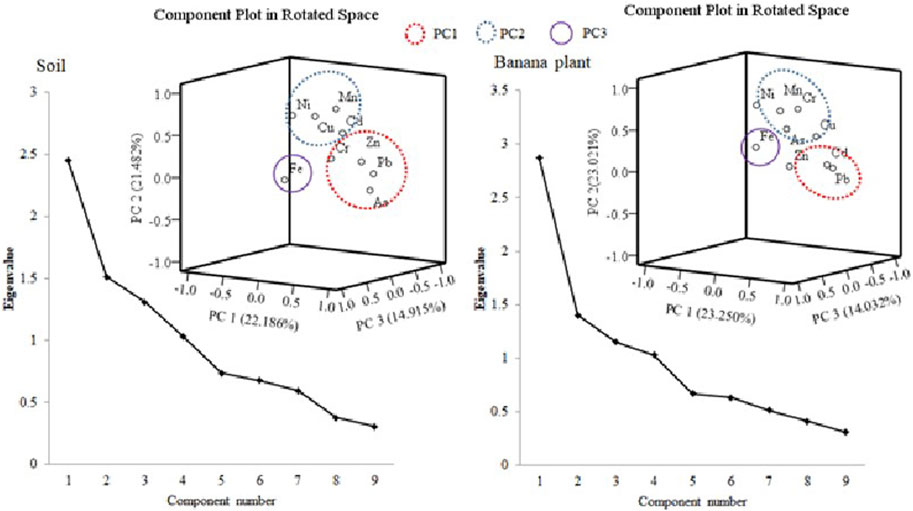
FIGURE 5. Principal component analysis (PCA) of trace elements in soil and banana plants around the capital of ancient Pundranagar, Bangladesh.
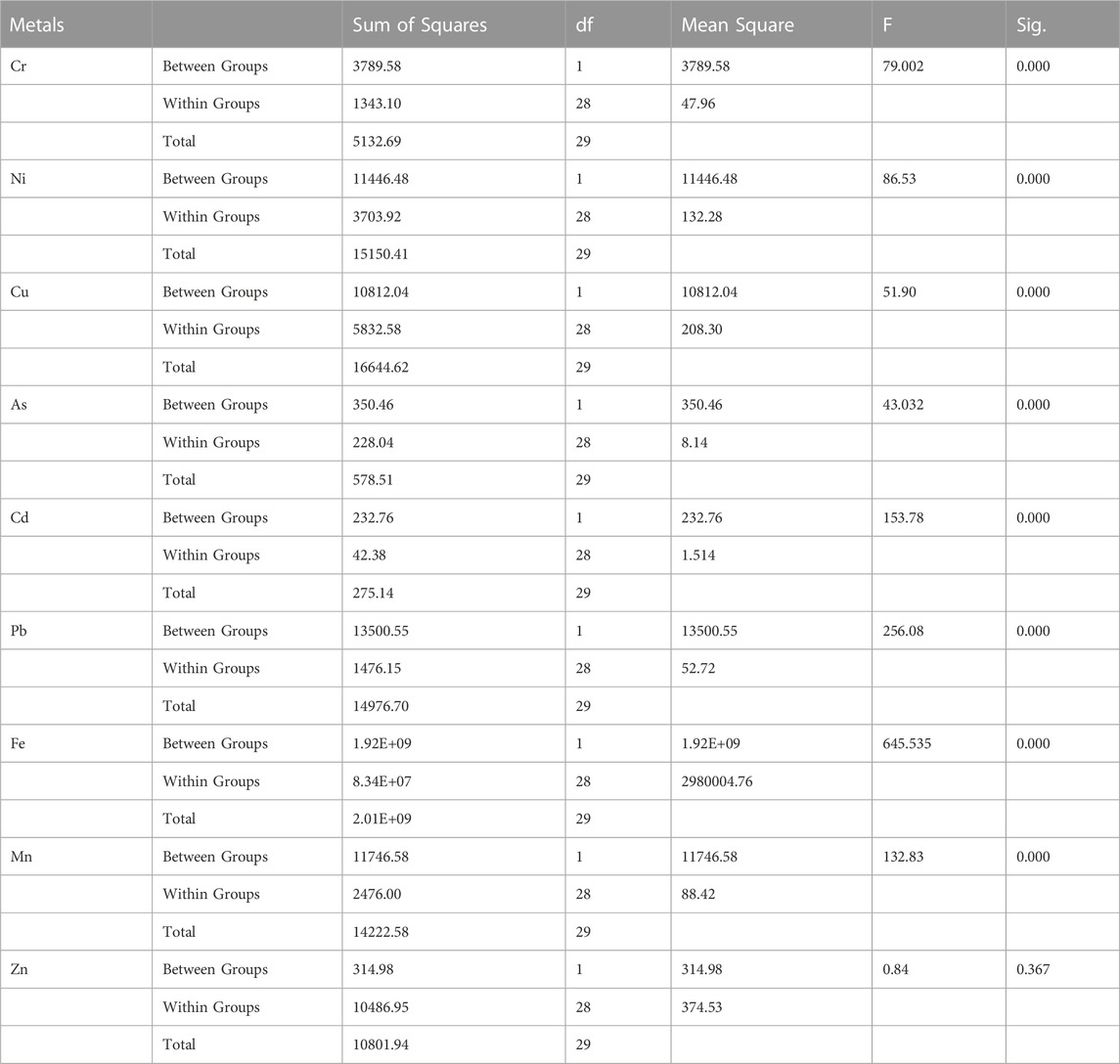
TABLE 3. Analysis of variance (ANOVA) for metal concentrations in soil and different tissues of banana plants.
Health risk
Data are shown in Table 4 for the exposure of adults and children to the trace elements in banana fruits. The mean values of EDI from banana fruits followed the descending order of Fe > Zn > Mn > Ni > Cu > Cr > As > Pb > Cd. Compared to the maximum acceptable daily ingestion of trace elements (JECFA 2011), EDI levels for the studied elements were found to be lower than the MTDI (Table 4), indicating that the studied elements had a minor effect on human health via banana consumption in the considered area. The non-carcinogenic risk (THQ) for eight elements (except Pb) and TR of Cr and As of adult and child consumption of banana fruit are shown in Table 4. Except for children, the THQs for the studied elements were lower than the threshold value (THQ <1), indicating no probable non-carcinogenic concerns associated with banana consumption. However, the total THQ of multiple elements from banana fruits was higher than the allowable levels for adults and children (THQ >1), indicating a potential risk to human health.
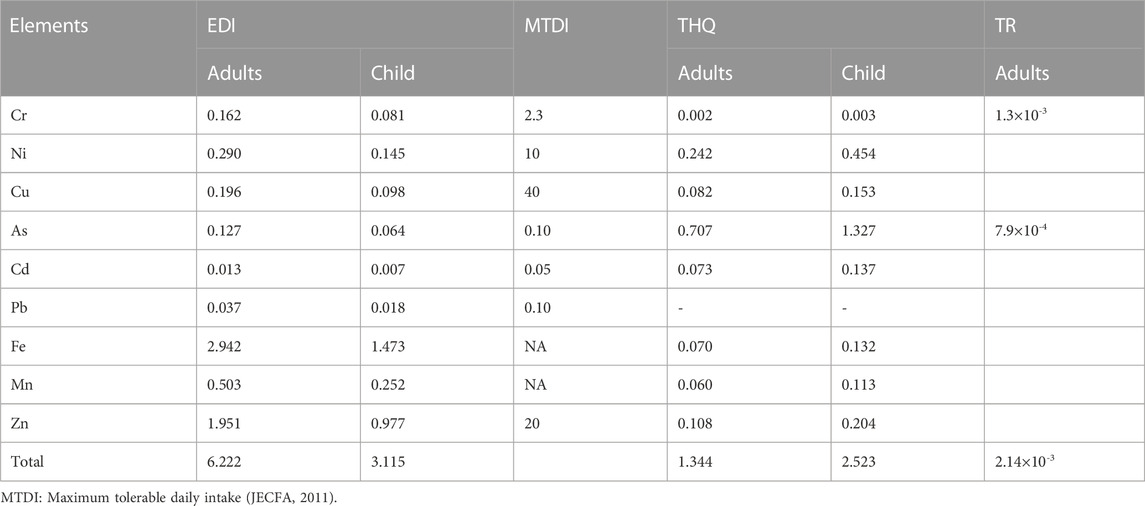
TABLE 4. Estimated daily intake (EDI), carcinogenic (TR) and non-carcinogenic (THQ) risks of trace elements from consumption of banana fruit in the study area, Bangladesh.
The target cancer risk for Cr and As was determined to be 1.3 × 10−3 and 7.9 × 10−4, respectively (Table 4). These values were somewhat larger than the permitted value (10–4), indicating a cancer risk to adult consumers (USEPA, 2010, USEPA 2015). The current study considered 50% of inorganic As in banana fruit for carcinogenic risk calculation (Saha and Zaman, 2013). In the current study, only the edible part of the banana was considered for health risk estimation. Other food items, such as cereals, vegetables, other fruits, and non-piscine protein source foodstuffs (meat, milk, and egg), from the research area should be considered in order to gain the real scenario of health benefits from dietary intake of bio-accessible forms of trace elements (Praveena and Omar 2017). This is a limitation of this study.
Findings from the present study also supported the idea that the manufacturing of deleterious substances, especially those that generate trace elements, should be curtailed by adopting tough measures such as the imposition of higher taxes on those products that cause food insecurity or have a negative impact on public health. Proper education on soil nutrients in banana plant systems could assist residents in comprehending some likely risks from trace elements. Therefore, result-oriented approaches should be adopted by the concerned authorities for better management of trace-element-contributing sources in the banana field soil in Bangladesh. Presently, no efficient management approach has been developed for addressing trace elements pollution in Bangladesh. However, the present research revealed different untapped research themes such as economic and ecological aspects of soil–banana plant systems and ecotoxicological profiles, trace elements in agro-food, their hazards, and potential remedies through intervention options and proper regulatory measures (Figure 6). The available literature on soil–plant management systems should be incorporated into these untapped research themes to address the present challenges of assessment and identification of trace elements in the banana field soils. Strict enforcement of any existing policy concerning the use of agrochemicals on agrarian soils will restrict trace element pollution in the soil–banana plant systems of Bangladesh. More so, the transfer of trace elements along the food chain and their toxicity in other agroecosystems should be studied. Additionally, eco-friendly and reliable technology should be incorporated with modern global approaches for the mitigation of potentially toxic elements. Thus, a careful evaluation of existing research gaps and global policy synchronization should be undertaken by authorized bodies to ensure proper management of trace element pollution in the soils of banana fields and other agroforestry systems in Bangladesh.
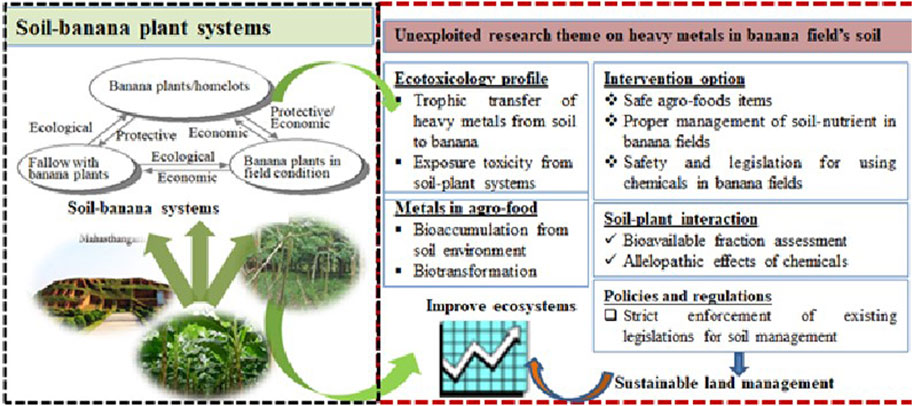
FIGURE 6. Regulatory acts for safety assessment of heavy metals in the banana field soil with the conceptual policy framework for sustainable soil management in Bangladesh.
Conclusion
In the current study, we found the average concentrations of Cu, Pb, As, and Cd in the soils of banana fields were higher than the world average shale standard; the background values of trace elements in soils of the ancient city of historical Pundranagar, Bangladesh; virgin fields; and the Dutch soil quality standard, indicating their contamination in the soil and the possibility that it may destroy the soil ecosystems of long-term cultivated banana fields. The concentrations of As, Cr, Pb, Cd, Zn, and Mn in four tissues of banana plants exceeded the maximum allowable concentration of trace elements, suggesting that their contamination represents a potential threat to the local inhabitants of the study area. The most elevated concentrations were observed in roots followed by leaves. Considering all the examined tissues, the mean BCF values of trace elements followed the descending order of Zn > As > Mn > Cr > Ni > Cu > Cd > Pb > Fe. The mean values of EF, Igeo, CF, PLI, Cd, mCd, MERM-Q, PERI, and PN indicated a moderate to high degree of contamination for trace elements in banana field soil. Three principal components accounted for the variability of trace elements in both soil and banana plants where Cr, Zn, Pb, As, and Cd were enriched due to anthropogenic activities. The total THQ of multiple elements from the consumption of banana fruits was higher than the allowable levels for adults and children (THQ >1), indicating a potential risk to human health. The TCRs of Cr and As crossed the threshold limit (1 × 10−6), showing a cancer risk for the adult inhabitants of the study area who consume and use these banana plant tissues. Therefore, it is critical that Cr and As levels are managed, as they have already entered the banana plants and are present in the soil. Continuous monitoring of trace elements in bananas and other foods in the study area is recommended to understand the reasons for the accumulation of trace elements in soil and banana plants around the intensively cultivated agricultural regions of the capital of earliest Pundranagar, Bangladesh.
Data availability statement
The original contributions presented in the study are included in the article/Supplementary Material; further inquiries can be directed to the corresponding authors.
Author contributions
MSI: conceptualization, methodology, validation, and writing–original draft. MTI: investigation, methodology, validation, and writing–review and editing. ZI: writing–review and editing, conceptualization, data curation, validation, and visualization. AI: conceptualization, investigation, project administration, and writing–review and editing. RK: data curation, formal analysis, investigation, and writing–review and editing. FH: investigation, methodology, project administration, and writing–review and editing. MK: conceptualization, formal analysis, methodology, and writing–review and editing. BE: conceptualization, formal analysis, and writing–review and editing. KI: conceptualization, funding acquisition, validation, and writing–review and editing. AI: conceptualization, funding acquisition, investigation, supervision, and writing–review and editing.
Funding
The authors extend their appreciation to the Deanship of Scientific Research at King Khalid University for funding this work through the Large Group Research Project under grant number (R.G.P.2/324/44).
Acknowledgments
The authors are thankful to the authority of Soil Resource Development Institute, Bogra, Bangladesh, for sample collection, the governing body of Patuakhali Science and Technology University for sample processing, and Bangabandhu Sheikh Mujibur Rahman Agricultural University, Bangladesh, and the University of Tokyo, Japan, for sample analysis.
Conflict of interest
The authors declare that the research was conducted in the absence of any commercial or financial relationships that could be construed as a potential conflict of interest.
Publisher’s note
All claims expressed in this article are solely those of the authors and do not necessarily represent those of their affiliated organizations, or those of the publisher, the editors, and the reviewers. Any product that may be evaluated in this article, or claim that may be made by its manufacturer, is not guaranteed or endorsed by the publisher.
Supplementary material
The Supplementary Material for this article can be found online at: https://www.frontiersin.org/articles/10.3389/fenvs.2023.1272840/full#supplementary-material
References
Abbasi, A. (2019). 137Cs distribution in the South Caspian region, transfer to biota and dose rate assessment. Int. J. Environ. Anal. Chem. 1−15. doi:10.1080/03067319.2019.1637431
Abrahim, G., and Parker, R. (2008). Assessment of heavy metal enrichment factors and the degree of contamination in marine sediments from Tamaki Estuary, Auckland, New Zealand. Environ. Monit. Assess. 136 (1–3), 227–238. doi:10.1007/s10661-007-9678-2
Ahmed, M. K., Shaheen, N., Islam, M. S., Islam, S., Islam, M. M., Kundu, G. K., et al. (2016). A comprehensive assessment of arsenic in commonly consumed foodstuffs to evaluate the potential health risk in Bangladesh. Sci. Total Environ. 544, 125–133. doi:10.1016/j.scitotenv.2015.11.133
Asomba, H. C., Ezewudo, B. I., Okeke, C. J., and Islam, M. S. (2023). Grain size analysis and ecological risk assessment of metals in the sediments of Knin River and Igboho dam reservoir, Oyo State, Nigeria, under agricultural disturbances. Environ. Monit. Assess. 195, 378. doi:10.1007/s10661-023-11009-y
Bawuro, A. A., Voegborlo, R. B., and Adimado, A. A. (2018). Bioaccumulation of heavy metals in some tissues of fish in Lake Geriyo, Adamawa state, Nigeria. J. Environ. Public Health 2018, 1–7. doi:10.1155/2018/1854892
Bhuiyan, M. A. H., Karmaker, S. C., Bodrud-Doza, M., Rakib, M. A., and Saha, B. B. (2021). Enrichment, sources and ecological risk mapping of heavy metals in agricultural soils of dhaka district employing SOM, PMF and GIS methods. Chemosphere 263, 128339. doi:10.1016/j.chemosphere.2020.128339
Birch, G. F., and Olmos, M. A. (2008). Sediment-bound heavy metals as indicators of human influence and biological risk in coastal water bodies. ICES J. Mar. Sci. 65, 1407–1413. doi:10.1093/icesjms/fsn139
Bonanno, G. (2013). Comparative performance of trace element bioaccumulation and biomonitoring in the plant species Typha domingensis, Phragmites australis and Arundo donax. Ecotoxicol. Environ. Saf. 97, 124–130. doi:10.1016/j.ecoenv.2013.07.017
Dghaim, R., Khatib, S. A., Rasool, H., and Khan, M. A. (2015). Determination of heavy metals concentration in traditional herbs commonly consumed in the United Arab Emirates. J. Environ. Public Health 2015, 973878. doi:10.1155/2015/973878
Dong, B., Zhang, R., Gan, Y., Cai, L., Freidenreich, A., Wang, K., et al. (2019). Multiple methods for the identification of heavy metal sources in cropland soils from a resource-based region. Sci. Total Environ. 651 (2), 3127–3138. doi:10.1016/j.scitotenv.2018.10.130
Ekere, N. R., Ugbor, M. C. J., Ihedioha, J. N., Ukwueze, N. N., and Abugu, H. O. (2020). Ecological and potential health risk assessment of heavy metals in soils and food crops grown in abandoned urban open waste dumpsite. J. Environ. Health Sci. Eng. 18, 711–721. doi:10.1007/s40201-020-00497-6
Ekere, N., Yakubu, N., and Ihedioha, J. (2017). Ecological risk assessment of heavy metals and polycyclic aromatic hydrocarbons in sediments of rivers Niger and Benue Confluence, Lokoja, Central Nigeria. Environ. Sci. Pollut. Res. 24, 18966–18978. doi:10.1007/s11356-017-9511-6
Falcomer, A. L., Riquette, R. F. R., de Lima, B. R., Ginani, V. C., and &and Zandonadi, R. P. (2019). Health benefits of green banana consumption: A systematic review. Nutrients 11 (6), 1222. doi:10.3390/nu11061222
FAO (Food and Agriculture Organization) (2017). Food and agriculture organization of the united nations: Banana and plantain processing technologies. Available at: http://faostat3.fao.org/browse/Q/QC/E.
FAO (Food and Agriculture Organization) (2006). Arsenic contamination of irrigation water, soil and crops in Bangladesh: Risk implications for sustainable agriculture and food safety in Asia. Bangkok: Food and Agriculture Organization of the United Nations Regional Office for Asia and the Pacific.
FAO (Food and Agriculture Organization) (2019). Good agricultural practices for bananas. Available at: http://www.fao.org/world-bananaforum/projects/good-practices/good-agricultural-practices/en/.
FAO/WHO (2011). Joint FAO/WHO Food Standards programme Codex committee on contaminants in foods, 1–89. CF/5 INF/1.
Flores, V., Victorio, C., Direito, I., and Cardoso, A. (2018). Heavy metals accumulation in banana (Musa spp.) leaves from industrial area in Rio de Janeiro. Orbital Electron. J. Chem. 10 (4), 364–366. doi:10.17807/orbital.v10i4.1054
Fonsah, E. G., Manower, T., Hussain, A., Chattapadhya, S., Islam, S., Islam, M. S., et al. (2017). Factors affecting banana agricultural value chain in Bangladesh. J. Food Distribution Res. 48 (1), 26.
Franco-Uria, A., López-Mateo, C., Mari, E. R., and Fernández-Marcos, L. (2009). Source identification of heavy metals in pasture land by multivariate analysis in NW Spain. J. Hazard. Mater. 165, 1008–1015. doi:10.1016/j.jhazmat.2008.10.118
Ghazali, A. R., Razak, N. E. A., Othman, M. S., Ishak, I., Lubis, S. H., Mohammad, N., et al. (2012). Study of heavy metal levels among farmers of muda agricultural development authority, Malaysia. J. Environ. Public Health 2012, 758349. doi:10.1155/2012/758349
Guo, W., Liu, X., Liu, Z., and Li, G. (2010). Pollution and potential ecological risk evaluation of heavy metals in the sediments around dongjiang harbor, tianjin. Tianjin. Procedia. Environ. Sci. 2, 729–736. doi:10.1016/j.proenv.2010.10.084
Håkanson, L. (1980). An ecological risk index for aquatic pollution control. A sedimentological approach. Water Res. 14, 975–1001. doi:10.1016/0043-1354(80)90143-8
Henriques, W., Jeffers, R. D., Lacher, T. J. R., and Kendall, R. J. (1997). Agrochemical use on banana plantations in Latin America: Perspectives on ecological risk. Environ. Toxicol. Chem. 16, 91–99. doi:10.1002/etc.5620160110
HIES (Household Income and Expenditure Survey) (2017). Preliminary report on household income and expenditure survey 2016. Bangladesh Bureau of Statistics, Ministry of Planning, Govt. of the People’s Republic of Bangladesh. Dhaka-1000, Bangladesh.
Hossain, M. F. (2014). A study of banana production in Bangladesh: Area, yield and major constraints. ARPN J. Agric. Biol. Sci. 9 (6), 206.
Igwe, O., Adepehin, E. J., Iwuanyanwu, C., and Una, C. O. (2014). Risks associated with the mining of Pb-Zn minerals in some parts of the Southern Benue trough, Nigeria. Environ. Monit. Assess. 186 (6), 3755–3765. doi:10.1007/s10661-014-3655-3
Iqbal, J., and Shah, M. H. (2011). Distribution, correlation and risk assessment of selected metals in urban soils from Islamabad, Pakistan. J. Hazard. Mater. 192, 887–898. doi:10.1016/j.jhazmat.2011.05.105
Islam, M. D., Hasan, M. M., Rahaman, A., Haque, P., Islam, M. S., and Rahman, M. M. (2020a). Translocation and bioaccumulation of trace metals from industrial effluent to locally grown vegetables and assessment of human health risk in Bangladesh. SN Appl. Sci. 2, 1315. doi:10.1007/s42452-020-3123-3
Islam, M. S., Ahmed, M. K., Al-Mamun, M. H., and Eaton, D. W. (2020b). Calcium signaling: From basic to bedside. Pedosphere 30, 1–6. doi:10.1007/978-3-030-12457-1_1
Islam, M. S., Ahmed, M. K., Al-Mamun, M. H., and Masunaga, S. (2015b). Potential ecological risk of hazardous elements in different land-use urban soils of Bangladesh. Sci. Total Environ. 512–513, 94–102. doi:10.1016/j.scitotenv.2014.12.100
Islam, M. S., Ahmed, M. K., Al-Mamun, M. H., and Raknuzzaman, M. (2015a). The concentration, source and potential human health risk of heavy metals in the commonly consumed foods in Bangladesh. Ecotoxicol. Environ. Saf. 122, 462–469. doi:10.1016/j.ecoenv.2015.09.022
Islam, M. S., Ahmed, M. K., Idris, A. M., Phoungthong, K., Habib, M. A., and Mustafa, R. A. (2021b). Geochemical speciation and bioaccumulation of trace elements in different tissues of pumpkin in the abandoned soils: Health hazard perspective in a developing country. Toxin. Rev. 41, 1124–1138. doi:10.1080/15569543.2021.1977325
Islam, M. S., Ahmed, M. K., Raknuzzaman, M., Habibullah-Al-Mamun, M., and Islam, M. K. (2015c). Heavy metal pollution in surface water and sediment: a preliminary assessment of an urban river in a developing country. Ecol. Indic. 48, 282–291. doi:10.1016/j.ecolind.2014.08.016
Islam, M. S., Islam, A. R. M. T., Phoungthong, K., Ustaoğlu, F., Tokatli, C., Ahmed, R., et al. (2021a). Potentially toxic elements in vegetable and rice species in Bangladesh and their exposure assessment. J. Food Compos. Analysis 106, 104350. doi:10.1016/j.jfca.2021.104350
Islam, M. S., Ismail, Z., Jamal, M. H., Ibrahim, Z., Jumain, M., Islam, A. R. M. T., et al. (2022a). Heavy metals from different land use soil in the capital of ancient pundranagar, Bangladesh: A preliminary study for ecological risk assessment. Chem. Ecol. 38, 720–743. doi:10.1080/02757540.2022.2100360
Islam, M. S., Khanam, M. S., and Sarker, N. I. (2018). Health risk assessment of metals transfer from soil to the edible part of some vegetables grown in Patuakhali province of Bangladesh. Arch. Agric. Environ. Sci. 3 (2), 187–197. doi:10.26832/24566632.2018.0302013
Islam, M. S., Mustafa, R. A., Phoungthong, K., Islam, A. R. M. T., Islam, T., Choudhury, T. R., et al. (2022b). Arsenic in the food stuffs: Potential health appraisals in a developing country, Bangladesh. Environ. Sci. Pollut. Res. 30, 26938–26951. doi:10.1007/s11356-022-24119-w
Israeli, Y., and Lahav, E. (2017). Encyclopedia of applied plant sciences. Banana in tropical agriculture. 2nd ed, 363.
Juen, L. Y., Aris, A. Z., Ying, L. W., and Haris, H. (2014). Bioconcentration and translocation efficiency of metals in paddy (Oryza sativa): A case study from alor setar, kedah, Malaysia. Sains Malays. 43, 521–528.
Kabir, M. H., Islam, M. S., Hoq, M. E., Tusher, T. R., and Islam, M. S. (2020). Appraisal of heavy metal contamination in sediments of the Shitalakhya River in Bangladesh using pollution indices, geo-spatial and multivariate statistical analysis. Arabian. J. Geosciences 13, 1135. doi:10.1007/s12517-020-06072-5
Kashem, M. A., and Singh, B. R. (1999). Heavy metal contamination of soil and vegetation in the vicinity of industries in Bangladesh. Water, Air, and Soil Pollut. 115, 347–361. doi:10.1023/a:1005193207319
Kasozi, K. I., Natabo, P. C., Namubiru, S., Tayebwa, D. S., Tamale, A., and &and Bamaiyi, P. H. (2018). Food safety analysis of milk and beef in southwestern Uganda. J. Environ. Public Health 2018, 1–7. doi:10.1155/2018/1627180
Kennedy, J. (2009). Bananas and people in the homeland of genus musa: Not just pretty fruit. Ethnobot. Res. Appl. 7, 179–197. doi:10.17348/era.7.0.179-197
Khairiah, J., Habibah, H. J., Anizan, I., Maimon, A., Aminah, A., and Ismail, B. S. (2009). Content of heavy metals in soil collected from selected paddy cultivation areas in kedah and perlis, Malaysia. J. Appl. Sci. Res. 5 (12), 2179–2188.
Kormoker, T., Proshad, R., Islam, M. S., Ahmed, S., Chandra, K., Uddin, M., et al. (2019). Toxic metals in agricultural soils near the industrial areas of Bangladesh: ecological and human health risk assessment. Toxin Rev. 40, 1135–1154. doi:10.1080/15569543.2019.1650777
Kuerban, M., Maihemuti, B., Waili, Y., and Tuerhong, T. (2020). Ecological risk assessment and source identification of heavy metal pollution in vegetable bases of Urumqi, China, using the positive matrix factorization (PMF) method. PLOS ONE 15 (4), e0230191. doi:10.1371/journal.pone.0230191
Kühnlenz, T., Hofmann, C., Uraguchi, S., Schmidt, H., Schempp, S., Weber, M., et al. (2016). Phytochelatin synthesis promotes leaf Zn accumulation of Arabidopsis thaliana plants grown in soil with adequate Zn supply and is essential for survival on Zn-contaminated soil. Plant and Cell Physiology 57 (11), 2342–2352. doi:10.1093/pcp/pcw148
Kumar, K. P. S., Bhowmik, D., Duraivel, S., and Umadevi, M. (2012). Traditional and medicinal uses of banana. J. Pharmacogn. Phytochemistry 1, 51–63.
Kumar, S., Islam, A. R. M. T., Hasanuzzaman, M., Salam, R., Khan, R., and Islam, M. S. (2021). Preliminary assessment of heavy metals in surface water and sediment in Nakuvadra-Rakiraki River, Fiji using indexical and chemometric approaches. J. Environ. Manag. 298, 113517. doi:10.1016/j.jenvman.2021.113517
Lin, D., Ouyang, Y., Huang, C. H., and Huang, D. Y. (2010). Characterization of heavy metals from banana farming soils. CLEAN—Soil, Air, Water 38 (5−6), 430–436. doi:10.1002/clen.201000020
Liu, G., Yua, Y., Hou, J., Xue, W., Liu, X., Liu, Y., et al. (2014). An ecological risk assessment of heavy metal pollution of the agricultural ecosystem near a lead-acid battery factory. Ecol. Indic. 47, 210–218. doi:10.1016/j.ecolind.2014.04.040
Liu, W. X., Liu, J. W., Wu, M. Z., Li, Y., Zhao, Y., and Li, S. R. (2009). Accumulation and translocation of toxic heavy metals in winter wheat (Triticum aestivum L.) growing in agricultural soil of Zhengzhou, China. Bull. Environ. Contam. Toxicol. 82 (3), 343–347. doi:10.1007/s00128-008-9575-6
Ma, Z., Li, L. Y., Ye, C., Lin, X. Y., and Wei, (2018). The influence of farmland pollution on the quality and safety of agricultural products. IOP Conf. Ser. Earth Environ. Sci. 113, 012114. doi:10.1088/1755-1315/113/1/012114
Mallick, S. R., Proshad, R., Islam, M. S., Sayeed, A., Uddin, M., Gao, J., et al. (2019). Heavy metals toxicity of surface soils near industrial vicinity: A study on soil contamination in Bangladesh. Archives Agric. Environ. Sci. 4 (4), 356–368. doi:10.26832/24566632.2019.040401
Marles, R. J. (2017). Mineral nutrient composition of vegetables, fruits and grains: the context of reports of apparent historical declines. J. Food Compos. Analysis 56, 93–103. doi:10.1016/j.jfca.2016.11.012
Martin, J. A. R., Miras, J. J. R., Boluda, R., and Carrasco, C. G. D. (2013). Spatial relations of heavy metals in arable and greenhouse soils of a Mediterranean environment region (Spain). Geoderma 200-201, 180–188. doi:10.1016/j.geoderma.2013.02.014
Miedico, O., Pompa, C., Tancredi, C., Cera, A., Pellegrino, E., Tarallo, M., et al. (2017). Characterisation and chemometric evaluation of 21 trace elements in three edible seaweed species imported from south-east Asia. J. Food Compos. Analysis 64, 188–197. doi:10.1016/j.jfca.2017.09.004
Mizan, A., Mamun, M. A. H., and Islam, M. S. (2023). Metal contamination in soil and vegetables around savar tannery area, dhaka, Bangladesh: A preliminary study for risk assessment. Heliyon 9, e13856. doi:10.1016/j.heliyon.2023.e13856
Mohamed, A. B. J., Ali, M. H., Khamis, S. A., and Ali, A. I. (2017). Level of nutritious and non-nutritious elements in banana grown in zanzibarZanzibar. Acad. J. Chem. 2, 21–27.
Mohiuddin, A., Saha, M. K., Hossian, M. S., and Ferdoush, i. A. (2014). Usefulness of banana (Musa paradisiaca) wastes in manufacturing of bio-products: a review. Agric. 12 (1), 148–158. doi:10.3329/agric.v12i1.19870
Müller, G. (1981). Die schwermetallbelastung der sedimente des Neckars und seiner Nebenflusse: Eine Bestandsaufnahme. Chem. Ztg. 105, 157–164.
Oliveira, B. G., Pimentel, E. F., Pereira, A. C. H., Tosato, F., Pinto, F. E., Ventura, J. A., et al. (2020). Phenolic and glycidic profiling of bananas Mmusa sp associated with maturation stage and cancer chemoprevention activities. Microchem. J. 153, 104391. doi:10.1016/j.microc.2019.104391
Onyenekwe, P. C., Okereke, O. E., and Owolewa, S. O. (2013). Phytochemical screening and effect of Musa paradisiaca stem extrude on rat haematological parameters. Curr. Res. J. Biol. Sci. 5, 26–29. doi:10.19026/crjbs.5.5468
Pandey, R., Shubhashish, K., and Pandey, J. (2012). Dietary intake of pollutant aerosols via vegetables influenced by atmospheric deposition and wastewater irrigation. Ecotoxicol. Environ. Saf. 76, 200–208. doi:10.1016/j.ecoenv.2011.10.004
Praveena, S. M., and Omar, N. A. (2017). Heavy metal exposure from cooked rice grain ingestion and its potential health risks to humans from total and bioavailable forms analysis. Food Chem. 235, 203–211. doi:10.1016/j.foodchem.2017.05.049
Proshad, R., Ahmed, S., Rahman, M., and Kumar, T. (2017). Apportionment of hazardous elements in agricultural soils around the vicinity of brick kiln in Bangladesh. J. Environ. Anal. Toxicol. 7 (2), 1–6. doi:10.4172/2161-0525.1000439
Proshad, R., Islam, M. S., Kormoker, T., Sayed, A., Khadka, S., and Idris, A. M. (2021). Potential toxic metals (PTMs) contamination in agricultural soils and foodstuffs with associated source identification and model uncertainty. Sci. Total Environ. 789, 147962. doi:10.1016/j.scitotenv.2021.147962
Proshad, R., Kormoker, T., Islam, M. S., and Chandra, K. (2019). Potential health risk of heavy metals via consumption of rice and vegetables grown in the industrial areas of Bangladesh. Hum. Ecol. Risk Assess. Int. J. 22 (4), 921–943. doi:10.1080/10807039.2018.1546114
Ravankhah, N., Mirzaei, R., and Masoum, S. (2016). Spatial eco-risk assessment of heavy metals in the surface soils of industrial city of Aran-o-Bidgol, Iran. Bull. Environ. Contam. Toxicol. 96 (4), 516–523. doi:10.1007/s00128-016-1761-3
Romero-Estevez, D., Yanez-Jacome, G. S., Simbaña-Farinango, K., and Navarrete, H. (2019). Distribution, contents, and health risk assessment of cadmium, lead, and nickel in bananas produced in Ecuador. Foods 8 (8), 330. doi:10.3390/foods8080330
Rosa, R. H., Fernandes, M. R., Melo, E. S. P., Arakaki, D. G., Lima, N. V., Leite, L. C. S., et al. (2020). Determination of macro- and microelements in the Inflorescences of banana tree using ICP- OES: Evaluation of the daily recommendations of intake for humans. Sci. World J. 2020, 1–9. doi:10.1155/2020/8383612
Roy, S., Gupta, S. K., Prakash, J., Habib, G., Baudh, K., and Nasr, M. (2019). Ecological and human health risk assessment of heavy metal contamination in road dust in the national capital territory (NCT) of Delhi, India. Environ. Sci. Pollut. Res. 26, 30413–30425. doi:10.1007/s11356-019-06216-5
Sadhu, K., Adhikari, K., and Gangopadhyay, A. (2012). Assessment of heavy metal contamination of soils in and around open cast mines of Raniganj area, India. Int. J. Environ. Eng. Res. 2, 77–85.
Saha, N., and Zaman, M. R. (2013). Evaluation of possible health risks of heavy metals by consumption of foodstuffs available in the central market of Rajshahi City, Bangladesh. Environ. Monit. Assess. 185, 3867–3878. doi:10.1007/s10661-012-2835-2
Sahodaran, N. K., and Ray, J. G. (2018). Heavy metal contamination in “chemicalized” green revolution banana fields in southern India. Environ. Sci. Pollut. Res. 25 (27), 26874–26886. doi:10.1007/s11356-018-2729-0
Schottler, S. P., and Engstrom, D. R. (2006). A chronological assessment of Lake Okeechobee (Florida) sediments using multiple dating markers. J. Paleolimnol. 36, 19–36. doi:10.1007/s10933-006-0007-5
Shaheen, N., Irfan, M. N., Khan, I. N., Islam, S., Islam, M. S., and Ahmed, M. K. (2016). Presence of heavy metals in fruits and vegetables: Health risk implications in Bangladesh. Chemosphere 152, 431–438. doi:10.1016/j.chemosphere.2016.02.060
Shirani, M., Afzali, K. N., Jahan, S., Strezov, V., and Sardo, M. S. (2020). Pollution and contamination assessment of heavy metals in the sediments of Jazmurian playa in southeast Iran. Sci. Rep. 10 (1), 4775–4811. doi:10.1038/s41598-020-61838-x
Stambulska, U. Y., Bayliak, M. M., and Lushchak, V. I. (2018). Chromium(VI) toxicity in legume plants: Modulation effects of rhizobial symbiosis. BioMed Res. Int. 2018, 1–13. doi:10.1155/2018/8031213
Subramanian, D., Subha, R., and Murugesan, A. K. (2022). Accumulation and translocation of trace elements and macronutrients in different plant species across five study sites. Ecol. Indic. 135, 108522. doi:10.1016/j.ecolind.2021.108522
Turekian, K. K., and Wedepohl, K. H. (1961). Distribution of the elements in some major units of the earth’s crust. Geol. Soc. Am. Bull. 72, 175–192. doi:10.1130/0016-7606(1961)72[175:doteis]2.0.co;2
USEPA (1991). OSWER directive 9285, 6–03.Human health evaluation manual, supplemental guidance: Standard default exposure factors
USEPA (2010). Risk based concentration table. Available at: http://www.epa.gov/reg3hwmd/risk/human/index.htm.
Ustaoğlu, F., and Islam, M. S. (2020). Potential toxic elements in sediment of some rivers at giresun, northeast Turkey: A preliminary assessment for ecotoxicological status and health risk. Ecol. Indic. 113, 106237–106251. doi:10.1016/j.ecolind.2020.106237
VROM (Volkshuisvesting, Ruimtelijke Ordening enMilieubeheer) (2000). Ircular on target values and intervention values for soil remediation. Netherlands: Spatial Planning and Environment. Ministry of Housing.C
Yoon, J., Cao, X., Zhou, Q., and Ma, L. Q. (2006). Accumulation of Pb, Cu, and Zn in native plants growing on a contaminated Florida site. Sci. Total Environ. 368, 456–464. doi:10.1016/j.scitotenv.2006.01.016
Zabir, A. A., Zzaman, M. W. U., Hossen, M. Z., Uddin, M. N., Islam, M. S., and Islam, M. S. (2016). Spatial dissemination of some heavy metals in soil adjacent to Bhaluka industrial area, Mymensingh, Bangladesh. Am. J. Appl. Sci. Res. 2 (6), 38–47.
Zakir, H. M., Hasan, M. N., Quadir, Q. F., Sharmin, S., and Ahmed, I. (2013). Cadmium and lead pollution in sediments of midstream of the river Karatoa in Bangladesh. Int. J. Eng. Sci. 2, 34–42.
Zglobicki, W., Telecka, M., Skupiński, S., Pasierbińska, A., and Koziel, M. (2018). Assessment of heavy metal contamination levels of street dust in the city of Lublin, E Poland. E Pol. Environ. Earth Sci. 77, 774. doi:10.1007/s12665-018-7969-2
Zhao, S., and Duo, L. (2015). Bioaccumulation of cadium, copper, zinc, and nickel by weed species from municipal solid waste compost. Polishl J. Environ. Stud. 24 (1), 413–417. doi:10.15244/pjoes/28960
Keywords: banana, trace elements, ecological risk, bioconcentration, target cancer risk, Bangladesh
Citation: Islam MS, Islam MT, Ismail Z, Islam ARMT, Khan R, Hasan F, Kabir MH, Ezewudo BI, Ibrahim KA and Idris AM (2023) Assessment of trace elements in the long-term banana cultivation field’s soil. Front. Environ. Sci. 11:1272840. doi: 10.3389/fenvs.2023.1272840
Received: 04 August 2023; Accepted: 20 September 2023;
Published: 07 November 2023.
Edited by:
Rui Zhang, University of Jinan, ChinaReviewed by:
Sazal Kumar, The University of Newcastle, AustraliaJohann Antoine, University of the West Indies, Jamaica
Emmanuel Temiotan Ogbomida, Copperbelt University, Zambia
Copyright © 2023 Islam, Islam, Ismail, Islam, Khan, Hasan, Kabir, Ezewudo, Ibrahim and Idris. This is an open-access article distributed under the terms of the Creative Commons Attribution License (CC BY). The use, distribution or reproduction in other forums is permitted, provided the original author(s) and the copyright owner(s) are credited and that the original publication in this journal is cited, in accordance with accepted academic practice. No use, distribution or reproduction is permitted which does not comply with these terms.
*Correspondence: Md Saiful Islam, bXNhaWZ1bHBzdHVAeWFob28uY29t; Zulhilmi Ismail, enVsaGlsbWlAdXRtLm15; Abubakr M. Idris, ZHJhbWlkcmlzQGdtYWlsLmNvbQ==
†ORCID: Abubakr M. Idris, orcid.org/0000-0003-4038-4769