- 1College of Environmental Science and Engineering, Tongji University, Shanghai, China
- 2Shanghai Municipal Engineering Design Institute (Group) Co. Ltd, Shanghai, China
Dam construction interfered with the original environment of the river system and greatly affected the geochemical behaviors of trace metals. Thus, a set of toxic metals of Cr, Ni, Cu, Zn, As, Cd, Pb and Hg in soil/sediment of the Three Gorges Reservoir (TGR) during the period of 2008–2020 were analyzed and summarized. The results showed that levels of trace metals (except Cr) were apparently higher than the soil background in the TGR and China, in which Cu, Zn, As, Cd, Pb and Hg corresponded to the moderately to highly contaminated grade. As expected, most trace metals (except Ni and As) were observed an evident increase after the full impoundment stage of 2008–2014, suggesting the dam construction of the TGR that promoting the sediment adsorption effects for trace metals. For spatial patterns, metal levels largely depended on the sampling sites, that intensive anthropogenic activities might well be the primary contributors. Main stream with higher concentrations of trace metals in comparison with tributaries reflected the larger loads of metal pollution. In the water-level-fluctuating zone, hydrological regime induced by damming played a critical role on the redistribution of trace metals through eroding soil/sediment particles or bedrocks and altering the physiochemical characteristics and vegetation coverage of soil/sediment. Finally, submerged sediment seemed as a major sink of trace metals that had greater concentration than that in the water-level-fluctuating zone.
1 Introduction
Dam construction can evidently alter the original environment of riverine systems, such as flow velocity, hydraulic retention time, sediment discharge, primary productivity, nutrient retention and reoxygenation capacity, and ultimately regulate the accumulation, distribution, and transportation of toxic metals (Maavara et al., 2020; Bing et al., 2022). In the aquatic environment, sediment is not only a large pool for heavy metals, but also a habitat and food source for benthic fauna (Miranda et al., 2021). Toxic metals would be bioaccumulated and bioamplified through the food chain and end up in the diet of humans (Gurung et al., 2018; Hajri et al., 2022). Thus, it is of great significance to pay attention to heavy metal pollution in reservoirs.
The Three Gorges Reservoir (TGR) is the world’s largest hydroelectric project. Since 2008, the water level has varied from the base level elevation of 145 m in the period of water drawdown to the high level elevation of 175 m in the period of storage (Chen et al., 2013). A water-level-fluctuating zone (WLFZ) 30 m in altitude (accounting for 55% of the total flooded area) is annually subject to shifting wetness and red-ox conditions that plays a vital role on the behaviors of heavy metals (Wang and Zhang, 2013; Eckley et al., 2015). The desiccation of the sediments in summer tends to form Fe/Mn oxides and/or hydroxides to adsorb metals (e.g., Cd, Cu, Pb and Zn), whereas the rewetting of the sediment reduces Fe/Mn oxides and/or hydroxides, thereby releasing the heavy metals adsorbed on them (Zhu et al., 2019). For another, secondary geological disasters and soil erosion generated by periodic flooding, as well as strong human disturbances (e.g., agricultural activities) probably leaded to the migration of accumulated sediments in the WLFZ to increase metals in the overlying water (Bao et al., 2015; Gao et al., 2016; Wang et al., 2017). Thus, heavy metal pollution in the TGR after impoundment has been concerned (Guo et al., 2021; Bing et al., 2022; Dong et al., 2022). From the perspective of previous researches, trace metals such as Cd, Pb, and Zn in the sediments reached a moderate or even high contaminated degree after impoundment of the TGR (Wang et al., 2017; Gao et al., 2019; Zhu et al., 2019; Bing et al., 2022). However, the majority of these studies mainly considered a limited spatial scale (or study unit) or short time scale, so that large-scale and long-term spatial variations are still unclear. To address these problems, concentrations of trace metals in soil/sediment in previous studies during the period from 2008 to 2020 were summarized and analyzed. The primary objectives of this article were to, 1) assess the contamination state of heavy metals in soil/sediment from the TGR; 2) investigate the spatial and temporal distribution for heavy metals in soil/sediment from the TGR; 3) better understand the impact of the hydrological regime on the redistribution of heavy metals.
2 Materials and methods
2.1 Study area
The region of the TGR (28◦28′-31◦44′N, 105◦49′-110◦12′E) covers a number of counties and cities in Chongqing and Hubei Province (Figure 1), with a total water surface area of 1,080 km2 and a storage capacity of 39.3 billion m3 (Bing et al., 2022). This area experiences a humid subtropical monsoon climate, with rainfall focused on April to October. During the period of 2003–2020, the annual average air temperature and rainfall are 18°C and 1,127 mm, respectively. The information about pollutant discharges (e.g., industrial effluents, urban sewage, fertilizer usage, ship sewage) were shown in Supplementary Figure S1.
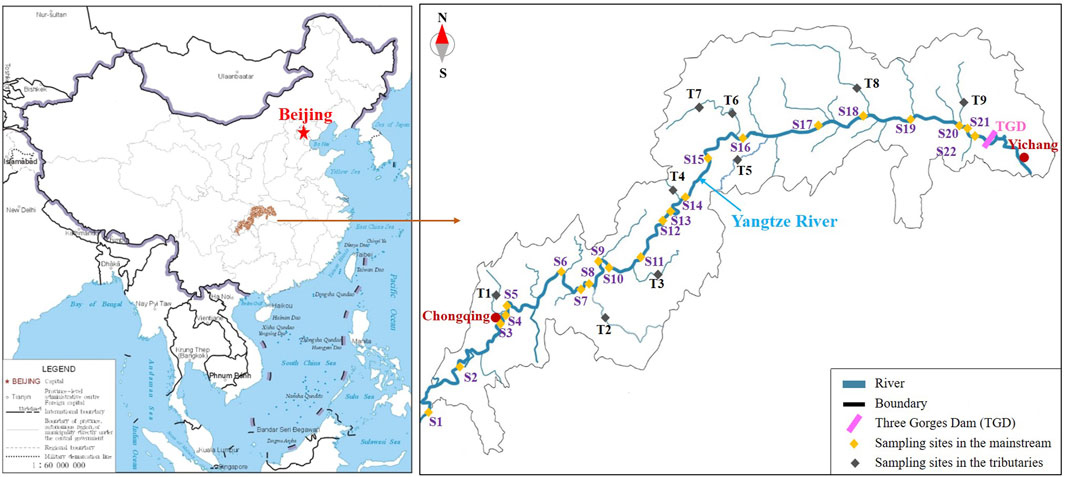
FIGURE 1. Location of the TGR (S1-S22 and T1-T9 represented the sampling sites that had statistical concentrations of trace metals in this study).
2.2 Data sources
Data used in this study were all obtained from previous studies Supplementary Table S1 and Supplementary Table S2.
2.3 Statistical analysis
The calculation method for the geo-accumulation index (Igeo) was provided in Supplementary Material. Statistical analysis was performed using SPSS software (version 25) for Windows. Mann-Whitney U test, t-test and Kruskal–Wallis test were employed to compare significant difference between paired or unpaired samples. Pearson correlation coefficient were computed for different heavy metals. Significant differences were all declared at p < 0.05.
3 Results and discussion
3.1 Levels of trace metals
The average concentrations of trace metals in the TGR from 2008 to 2020 were 74.8 mg/kg (Cr), 42.2 mg/kg (Ni), 51.2 mg/kg (Cu), 126 mg/kg (Zn), 12.1 mg/kg (As), 0.77 mg/kg (Cd), 44.1 mg/kg (Pb) and 0.13 mg/kg (Hg), respectively (Supplementary Table S3). The levels of most heavy metals (apart from Cd and Hg) exceeded the threshold effect concentration (TEC) indicating risks of adverse effects, whereas all trace metals were below the probable effect concentration (PEC) (Macdonald et al., 2000). The mean value of Cr (74.8 mg/kg) was basically consistent with the soil background in the TGR, which mainly came from the crust and rocks (Chen et al., 2011). Levels of Ni, Cu, Zn, As, Pb and Hg were 2-3 times higher than the soil background values in the TGR and China, and Cd was 6-7 times higher (Wei et al., 1991; Tang et al., 2008). Based on the average Igeo values (Supplementary Figure S2), the increasing order was Cr (−0.75) < Ni (−0.09) < Pb (0.11) < Zn (0.19) < Cu (0.28) < As (0.31) < Hg (0.63) < Cd (1.63). It implied that Cr and Ni had no contamination, while Pb, Zn, Cu, As, Hg and Cd contaminated soil/sediment mildly to moderately. Parts of Igeo values for Cd ranged 3 to 5, reflecting highly to extremely polluted levels. The labile percentage of Cd with high bioavailability was reported to exceed 50%, followed by Zn (10%–20%), Cu (5%–15%), Pb (around 5%), Ni (<5%) and Cr (<1%) (Bing et al., 2022). Thus, Cd was the most polluted metal in the TGR, which is the key factor of ecological risk in the TGR (Gao et al., 2019; Bing et al., 2022).
3.2 Temporal and spatial variations of heavy metals
Most trace metals (except Ni and As) in soil/sediment were observed an evident increase after the full impoundment stage of 2008–2014, and then showed dynamic fluctuation change (Figure 2). Despite the high levels of Cr and Hg were observed in 1985, this might be attributed to the difference of sampling sites (Xu et al., 1999). In comparison with the monitoring data before impoundment, the concentrations of all trace metals have increased to some extent, especially As and Cd were about doubled (Figure 2). This might be attributed to the dam construction of the TGR that promoting the sediment adsorption effects for trace metals (Wei et al., 2016; Bing et al., 2022). After impoundment, notable decreased water flow velocity and increased water retention time accelerated the sinking process of suspended particulate matter and attached heavy metals (Wang et al., 2017; Bing et al., 2022). Moreover, the effects of damming on river ecosystems are long-lasting and likely to persist for few decades (Porvari, 1998; Bodaly et al., 2007). The correlation analysis for Cu, Zn, Cd and Pb clearly identified a consistent positive correlation with each other (Supplementary Table S4, p < 0.05), and studies have shown that they were primarily from industrial effluents or intensive fertilizer use (Chen et al., 2011; Zhu et al., 2019; Shui et al., 2020). Meanwhile, Pb was confirmed to come from ore mining and smelting, coal combustion, aerosols and traffic exhaust by isotope ratios (Bing et al., 2016; Zhu et al., 2019). Ni generally had a close correlation with all other trace metals (p < 0.05), indicating diverse sources.
For spatial patterns, metal contents largely depended on the sampling sites (Lin et al., 2020). There were no obvious changing trends for trace metals from upstream to the Three Gorges Dam (TGD) (Figure 3). In previous studies, the concentrations for some trace metals in the soil/sediments generally appeared a rising trend towards the dam, suggesting the “self-purification” of the reservoir (Gao et al., 2019; Zhu et al., 2019; Lin et al., 2020; Bing et al., 2022), whereas the opposite result was simultaneously discovered (e.g., Cd) (Zhu et al., 2019). In the upper section, most metals (Cu, As, Cd, Pb and Hg) had relatively low levels in S1 and S2 (Figure 3). But in and around the main urban area of Chongqing City with intensive human activities, Cr, Cu, Zn, Pb and Hg exhibited comparatively high values (Figure 3). Traffic exhaust and industrial effluents were recognized as the main sources, reflecting anthropogenic effects on trace metal contamination in sediments (Chen et al., 2011). After that, agricultural activities and animal husbandry industries probably contributed to the metal accumulation in the soil/sediment of the middle section (Bing et al., 2022). Near the dam, high values were observed for some trace metals (e.g., Cr, Cd, Zn and Pb) that might be connected with the high geological background levels in the host rock (limestone) (Bing et al., 2022). Furthermore, the average concentration of trace metals in the soil/sediment of the main stream was commonly higher than that of the tributaries (Supplementary Figure S3), indicating the higher metal pollution inputs in main stream (Gao et al., 2015; Gao et al., 2019). The concentrations of Ni, Cu and Zn were found significant differences between main stream and tributaries (Supplementary Figure S3, Mann-Whitney U test, p < 0.05).
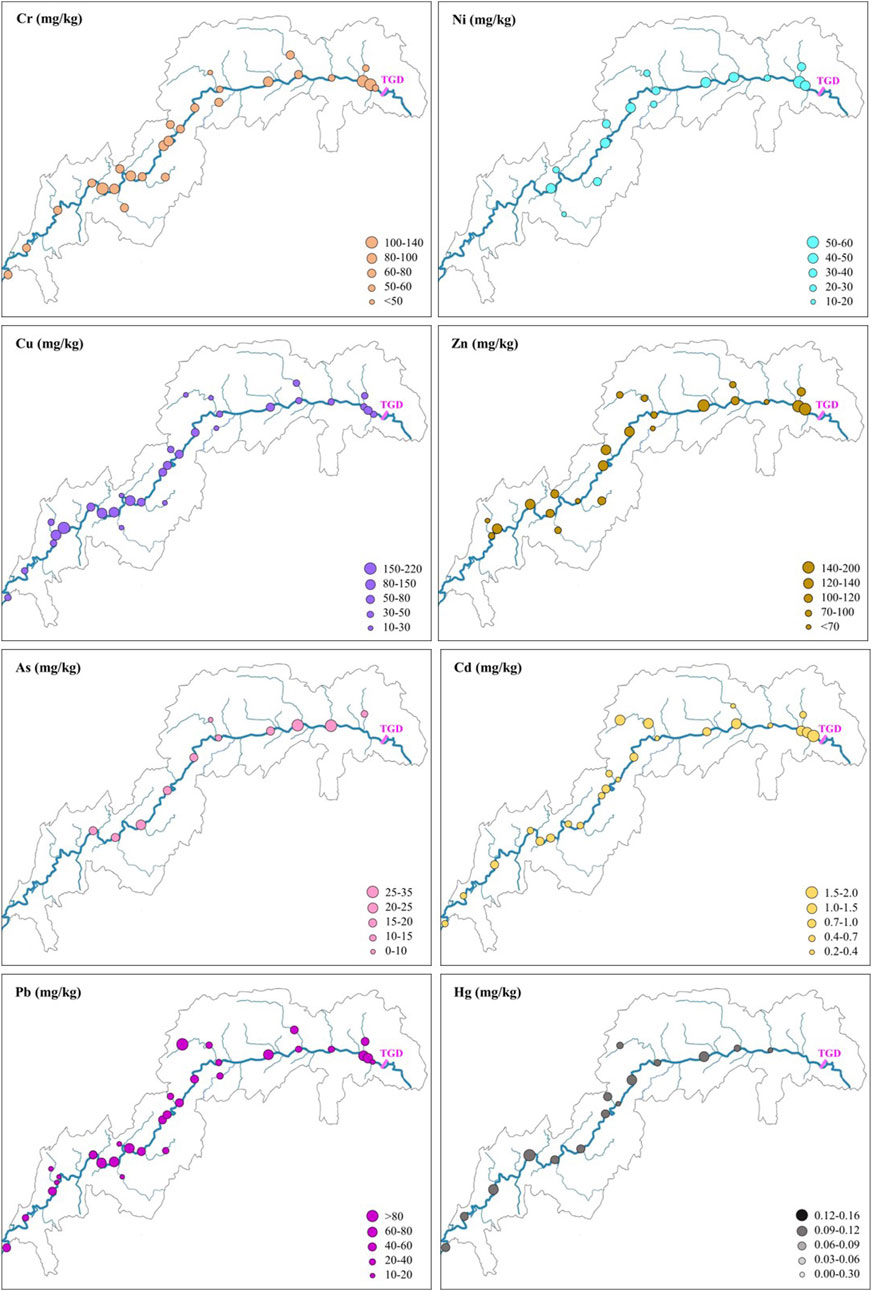
FIGURE 3. The Spatial distribution patterns of heavy metals in soil/sediment of the TGR from 2008 to 2020.
3.3 Impact of the hydrological regime on heavy metal distribution
The water level scheduling mode of “impoundment in winter and effusion in summer” in the TGR created a WLFZ with a total area of 349 km2 (Bao et al., 2015). The WLFZ demonstrated spatially diverse patterns that responded differently to hydrological regimes. There were three common types (Supplementary Table S5), of which type 1 and type 2 represented the most dynamic part of the TGR disturbance zone. Type 1 (the gradient less than 15°) comprised of purple and red rocks and a generally thick soil layer with sparsely distributed vegetation, and type 2 consisted of the lower section that similar to type1 and the upper section that had shallow soil layer with the gradient greater than 25° (Bao et al., 2015). During the warm season, rills and gullies generated by overland flow during storm events were scattered throughout these areas so that erosional particulates attached to heavy metals flowed into the water and deposited in sediments (Bao et al., 2015; Zhu et al., 2019). Type 3 (the gradient greater than 45°) was relatively stable that dominated by bedrocks with a thin layer of bare soils (Zhang et al., 2009; Bao et al., 2015). The cycling rising and falling of water level could bring about rockfalls and bedding landslides that rock-forming elements were significantly correlated with heavy metals in submerged sediments of the TGR (Chen et al., 2011; Bing et al., 2016; Zhu et al., 2019). Hence, different WLFZ patterns affected the spatial distribution of trace metals in the TGR.
In the WLFZ, the distribution patterns of heavy metals with water level elevation were ambiguous (Supplementary Figure S4). No significant differences were observed among three kinds of water level elevations (145–155 m, 155–165 m and 165–175 m) for all trace metals (Kruskal–Wallis test, p > 0.05). As a whole, concentrations of trace metals in soil/sediment in the submerged zone (SUB) were all higher than those in the WFLZ (Figure 4). Levels of Cr, Cu, Zn and Pb showed statistically significant difference between the SUB and the WFLZ (t-test, p < 0.05). During the low water-level period, soil/sediment in the WLFZ suffered from the intensive anthropogenic activities (e.g., improper agricultural activities, industrial and residential wastes) encouraged the enrichment of heavy metals, but contaminants were likely transferred through the complex geochemical process (e.g., soil erosion or flushing effects of wet deposition), and ultimately accumulated in the sediments (Gao et al., 2016; Wang et al., 2017). Thus, submerged sediment seemed to act as a major sink of heavy metals.
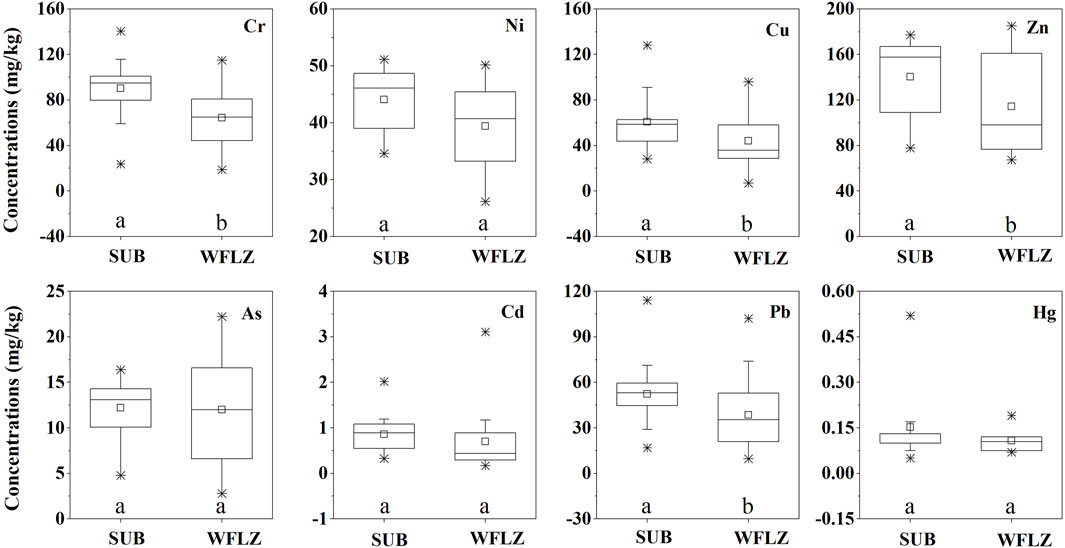
FIGURE 4. Concentrations of heavy metals in soil/sediment in submerged zone (SUB) and water-level-fluctuating zone (WFLZ) of the Three Gorges Reservoir from 2008 to 2020 (“ ”, the minimum and maximum value; “ ”, mean value; “——“, mid-value; a and b show significant levels after t-test; the data sets were log-transferred when they were not normal.).
Hydrological regime brought great changes to the WLFZ and affected the redistribution of various pollutants (e.g., heavy metals). Firstly, the drought-rewetting cycles controlled the physiochemical characteristics of soil/sediment, such as pH, oxidation-reduction potential (OPR), organic matter, particle size, element contents, the functional groups and crystal structure (Lin et al., 2018; Pei et al., 2018; Fu et al., 2020). The contents of NO3−-N, pH and element (except C element) in sediment were discovered to increase with the elevation of water level, whereas the OPR, TC, TN, C/N, NH4+-N, clay, silt and some trace metals (e.g., Cr, Cd, Cu and Pb) simultaneously decreased (Lin et al., 2018; Fu et al., 2020). Moreover, the Fourier transform infrared spectroscopy (FTIR) spectra of sediments were significantly different at various lateral altitudes, suggesting diverse active functional groups (like hydroxyl), some of which were susceptive to heavy metal ion binding (Fu et al., 2020). Due to rainfall runoff and riverbank erosion, sediment in lower altitude frequently tended to be coarser, more porous, and had a higher ratio of silt to clay componence (Tang et al., 2016; Wang et al., 2016; Lin et al., 2018). Particularly, there are more crystalline minerals in fine and silty sand that allows heavy metal contaminants to be easily adsorbed and fixed on mineral surfaces (Maity and Maiti, 2016; Fu et al., 2020). Additionally, plant diversity and species richness decreased markedly under the long-term periodic hydrodynamic disturbance (Ye et al., 2020). The flooding-tolerant plants survived to become the dominant species, and differed significantly at different elevations in some belt transects (e.g., Zhong County, Kaizhou and Xiangxi river) (Yin et al., 2020; Zhu et al., 2020). The vegetated area presented an upward trend with increasing water level elevation (Zhu et al., 2020). Here plants have been recognized as an important contributor to heavy metals in soil/sediment, and their well-developed root systems were primarily responsible for the uptake and accumulation of pollutants (Hall and St. Louis, 2004; Wang et al., 2022). During the high water-level period, flooded plants decomposed to aggravate anaerobic states and release nutrients that altered the morphology and bioavailability of heavy metals (Hall and St. Louis, 2004; Yin et al., 2020). However, microbial community properties of soil/sediment seemed to be less affected by the hydrological regimes, suggesting that microbes were more resilient than plants (Ye et al., 2020).
4 Conclusion
Levels of trace metals were apparently higher than the soil background values in the TGR (except for Cr) and China, in which Cd was the most polluted metal in the TGR. The dam construction of the TGR promoted the sediment adsorption effects resulting in an evident increase for trace metals (except Ni and As) after the full impoundment. Intensive anthropogenic activities largely contributed to the trace metals of soil/sediment from upstream to dam, and higher metal pollution inputs were found in the main stream. In the WLFZ, hydrological regime altered the physiochemical characteristics and vegetation coverage of soil/sediment, disrupting the distribution of heavy metals. Ultimately, trace metals were accumulated in submerged sediment resulting greater concentration than that in the WLFZ.
Data availability statement
The original contributions presented in the study are included in the article/Supplementary Material, further inquiries can be directed to the corresponding author.
Author contributions
QX: Conceptualization, Data curation, Methodology, Writing–original draft. KZ: Supervision, Validation, Writing–review and editing. BW: Funding acquisition, Writing–review and editing.
Funding
The author(s) declare financial support was received for the research, authorship, and/or publication of this article. This study was funded by Science and Technology Innovation Plan of Shanghai Science and Technology Commission (Grant number 21YF1449100, 21230714500).
Conflict of interest
KZ was employed by Shanghai Municipal Engineering Design Institute (Group) Co. Ltd.
The remaining authors declare that the research was conducted in the absence of any commercial or financial relationships that could be construed as a potential conflict of interest.
Publisher’s note
All claims expressed in this article are solely those of the authors and do not necessarily represent those of their affiliated organizations, or those of the publisher, the editors and the reviewers. Any product that may be evaluated in this article, or claim that may be made by its manufacturer, is not guaranteed or endorsed by the publisher.
Supplementary material
The Supplementary Material for this article can be found online at: https://www.frontiersin.org/articles/10.3389/fenvs.2023.1269138/full#supplementary-material
References
Bao, Y., Gao, P., and He, X. (2015). The water-level fluctuation zone of three gorges reservoir — a unique geomorphological unit. EARTH-SCI Rev. 150, 14–24. doi:10.1016/j.earscirev.2015.07.005
Bing, H., Liu, Y., Huang, J., Tian, X., Zhu, H., and Wu, Y. (2022). Dam construction attenuates trace metal contamination in water through increased sedimentation in the Three Gorges Reservoir. WATER Res. 217, 118419. doi:10.1016/j.watres.2022.118419
Bing, H., Zhou, J., Wang, X., Sun, H., and Li, R. (2016). Current state, sources, and potential risk of heavy metals in sediments of Three Gorges Reservoir, China. Environ. Pollut. 214, 485–496. doi:10.1016/j.envpol.2016.04.062
Bodaly, R. A., Jansen, W. A., Majewski, A. R., Fudge, R. J. P., Strange, N. E., Derksen, A. J., et al. (2007). Postimpoundment time course of increased mercury concentrations in fish in hydroelectric reservoirs of northern Manitoba, Canada. Arch. Environ. CON TOX 53 (3), 379–389. doi:10.1007/s00244-006-0113-4
Chen, Y., Li, S., Zhang, Y., Tong, X., and Zhang, Q. (2013). Assessing heavy metal pollution in the water level fluctuation zone of China's Three Gorges Reservoir using geochemical and soil microbial approaches. Environ. Monit. Assess. 185 (1), 231–240. doi:10.1007/s10661-012-2547-7
Chen, Y., Li, S., Zhang, Y., and Zhang, Q. (2011). Assessing soil heavy metal pollution in the water-level-fluctuation zone of the Three Gorges Reservoir, China. J. HAZARD MATER 191 (1-3), 366–372. doi:10.1016/j.jhazmat.2011.04.090
Dong, C., Yang, Z., Zhu, Q., Tang, H., Li, S., and Chen, X. (2022). Fraction characteristics and ecological risk of heavy metals in surface sediments of Three Gorges Reservoir and the upper reaches. J. HYDROECOL 43 (1), 16–23.
Eckley, C. S., Luxton, T. P., McKernan, J. L., Goetz, J., and Goulet, J. (2015). Influence of reservoir water level fluctuations on sediment methylmercury concentrations downstream of the historical Black Butte mercury mine, OR. Or. Appl. Geochem. 61, 284–293. doi:10.1016/j.apgeochem.2015.06.011
Fu, C., Lan, Q., Wu, Y., Yan, B., Ping, W., Huang, L., et al. (2020). Influence of sediment characteristics on heavy metal fraction distribution in the water-level fluctuation zone of the three gorges reservoir area, China. WATER AIR SOIL Poll. 231, 175. doi:10.1007/s11270-020-04525-x
Gao, B., Zhou, H., Yang, Y., and Wang, Y. (2015). Occurrence, distribution, and risk assessment of the metals in sediments and fish from the largest reservoir in China. RSC Adv. 5, 60322–60329. doi:10.1039/c5ra09220e
Gao, L., Gao, B., Xu, D., Peng, W., and Lu, J. (2019). Multiple assessments of trace metals in sediments and their response to the water level fluctuation in the Three Gorges Reservoir, China. Sci. TOTAL Environ. 648, 197–205. doi:10.1016/j.scitotenv.2018.08.112
Gao, Q., Li, Y., Cheng, Q., Yu, M., Hu, B., Wang, Z., et al. (2016). Analysis and assessment of the nutrients, biochemical indexes and heavy metals in the Three Gorges Reservoir, China, from 2008 to 2013. WATER Res. 92, 262–274. doi:10.1016/j.watres.2015.12.055
Guo, Y., Ding, W., Zhu, X., and Cui, W. (2021). Spatial distribution and pollution characteristics of heavy metal contents in Three Goreges Reservoir area. Bull. SOIL WATER Conserv. 41 (6), 105–112.
Gurung, B., Race, M., Fabbricino, M., Kominkova, D., Libralato, G., Siciliano, A., et al. (2018). Assessment of metal pollution in the lambro creek (Italy). ECOTOX Environ. SAFE 148 (FEB), 754–762. doi:10.1016/j.ecoenv.2017.11.041
Hajri, D., Smajlovi, M., Antunovi, B., Smajlovi, A., Alagi, D., Tahirovi, D., et al. (2022). Risk assessment of heavy metal exposure via consumption of fish and fish products from the retail market in Bosnia and Herzegovina. FOOD control. 133, 108631. doi:10.1016/j.foodcont.2021.108631
Hall, B. D., and St. Louis, V. L. (2004). Methylmercury and total mercury in plant litter decomposing in upland forests and flooded landscapes. Environ. Sci. Technol. 38 (19), 5010–5021. doi:10.1021/es049800q
Lin, J., Shuai, Z., Dan, L., Yu, Z., Zhang, L., Jian, C., et al. (2018). Mobility and potential risk of sediment-associated heavy metal fractions under continuous drought-rewetting cycles. Sci. Total Environ. 625, 79–86. doi:10.1016/j.scitotenv.2017.12.167
Lin, L., Li, C., Yang, W., Zhao, L., Liu, M., Li, Q., et al. (2020). Spatial variations and periodic changes in heavy metals in surface water and sediments of the Three Gorges Reservoir, China. Chemosphere 240, 124837. doi:10.1016/j.chemosphere.2019.124837
Maavara, T., Chen, Q., Meter, K. V., Lee, E. B., Zhang, J., Ni, J., et al. (2020). River dam impacts on biogeochemical cycling. Nat. Rev. EARTH Envit. 1, 103–116. doi:10.1038/s43017-019-0019-0
Macdonald, D. D., Ingersoll, C. G., and Berger, T. A. (2000). Development and evaluation of consensus-based sediment quality guidelines for freshwater ecosystems. Arch. Environ. CON TOX 39 (1), 20–31. doi:10.1007/s002440010075
Maity, S. K., and Maiti, R. (2016). Understanding the sediment sources from mineral composition at the lower reach of Rupnarayan River, West Bengal, India – XRD-based analysis. GEORESJ 9-12, 91–103. doi:10.1016/j.grj.2016.09.004
Miranda, L. S., Ayoko, G. A., Egodawatta, P., and Goonetilleke, A. (2021). Adsorption-desorption behavior of heavy metals in aquatic environments: Influence of sediment, water and metal ionic properties. J. Hazard Mater 421 (5), 126743. doi:10.1016/j.jhazmat.2021.126743
Pei, S., Jian, Z., Guo, Q., Ma, F., Qin, A., Zhao, Y., et al. (2018). Temporal and spatial variation and risk assessment of soil heavy metal concentrations for water-level-fluctuating zones of the Three Gorges Reservoir. J. Soil Sediment. 18 (9), 2924–2934. doi:10.1007/s11368-018-1966-7
Porvari, P. (1998). Development of fish mercury concentrations in Finnish reservoirs from 1979 to 1994. Sci. TOTAL Environ. 213 (1-3), 279–290. doi:10.1016/s0048-9697(98)00101-6
Shui, L., Pan, X., Chen, X., Chang, F., Wan, D., Liu, D., et al. (2020). Pollution characteristics and ecological risk assessment of heavy metals in sediments of the three gorges reservoir. Water 12, 1798. doi:10.3390/w12061798
Tang, J., Zhong, Y., and Wang, L. (2008). Background value of soil heavy metal in the three gorges reservoir district. Chin. J. ECO-AGRIC 16 (4), 848–852. doi:10.3724/sp.j.1011.2008.00848
Tang, Q., Bao, Y., He, X., Fu, B., Collins, A. L., and Zhang, X. (2016). Flow regulation manipulates contemporary seasonal sedimentary dynamics in the reservoir fluctuation zone of the Three Gorges Reservoir, China. Sci. TOTAL Environ. 548-549, 410–420. doi:10.1016/j.scitotenv.2015.12.158
Wang, B., Yan, D., Wen, A., and Chen, J. (2016). Influencing factors of sediment deposition and their spatial variability in riparian zone of the Three Gorges Reservoir, China. J. Mt. Sci-Engl. 13 (8), 1387–1396. doi:10.1007/s11629-015-3806-1
Wang, F. Y., and Zhang, J. Z. (2013). Mercury contamination in aquatic ecosystems under a changing environment:Implications for the Three Gorges Reservoir. Chin. Sci. Bull. 58 (2), 141–149. doi:10.1007/s11434-012-5490-7
Wang, T., Pan, J., and Liu, X. (2017). Characterization of heavy metal contamination in the soil and sediment of the Three Gorges Reservoir, China. J. Environ. Sci. HEALTH PART A TOXIC/HAZARD Subst. Environ. Eng. 52 (3-4), 201–209. doi:10.1080/10934529.2016.1246931
Wang, X., Cai, D., Ji, M., Chen, Z., Yao, L., and Han, H. (2022). Isolation of heavy metal-immobilizing and plant growth-promoting bacteria and their potential in reducing Cd and Pb uptake in water spinach. Sci. TOTAL Environ. 819, 153242. doi:10.1016/j.scitotenv.2022.153242
Wei, F., Chen, J., Wu, Y., and Zheng, C. (1991). Study on the background contents on 61 elements of soil in China. J. Environ. SCI-CHINA 12 (4), 11–20.
Wei, X., Han, L., Gao, B., Zhou, H., Lu, J., and Wan, X. (2016). Distribution, bioavailability, and potential risk assessment of the metals in tributary sediments of Three Gorges Reservoir: The impact of water impoundment. Ecol. Indic. 61, 667–675. doi:10.1016/j.ecolind.2015.10.018
Xu, X. Q., Deng, G. Q., Hui, J. Y., Zhang, X. H., and Qiu, C. Q. (1999). The pollution characteristics of heavy metal in sediments in Three Gorges Reservoir. ACTA HYDROBIO SIN. 1, 1–10.
Ye, C., Butler, O. M., Chen, C., Liu, W., Du, M., and Zhang, Q. (2020). Shifts in characteristics of the plant-soil system associated with flooding and revegetation in the riparian zone of Three Gorges Reservoir, China. GEODERMA 361, 114015. doi:10.1016/j.geoderma.2019.114015
Yin, D., Wang, Y., Xiang, Y., Xu, Q., Xie, Q., Zhang, C., et al. (2020). Production and migration of methylmercury in water-level-fluctuating zone of the Three Gorges Reservoir, China: Dual roles of flooding-tolerant perennial herb. J. Hazard Mater 381, 120962. doi:10.1016/j.jhazmat.2019.120962
Zhang, B., Han, J., Shi, X. H., Guo, W. S., Xia, S. L., Shi, Q., et al. (2009). Thinking about geomorphologic evolution of slopes in hydrofluctuation belt of three gorges reservoir. Bull. SOIL WATER Conserv. 3, 1–5.
Zhu, H., Bing, H., Wu, Y., Zhou, J., Sun, H., Wang, X., et al. (2019). The spatial and vertical distribution of heavy metal contamination in sediments of the Three Gorges Reservoir determined by anti-seasonal flow regulation. Sci. TOTAL Environ. 664, 79–88. doi:10.1016/j.scitotenv.2019.02.016
Keywords: dam construction, heavy metals, soil, sediment, three gorges reservoir
Citation: Xu Q, Zhou K and Wu B (2023) Dam construction reshapes heavy metal pollution in soil/sediment in the three gorges reservoir, China, from 2008 to 2020. Front. Environ. Sci. 11:1269138. doi: 10.3389/fenvs.2023.1269138
Received: 29 July 2023; Accepted: 25 August 2023;
Published: 08 September 2023.
Edited by:
Jifu Ma, Yan’an University, ChinaReviewed by:
Deliang Yin, Guizhou University, ChinaXun Wang, Chinese Academy of Sciences (CAS), China
Copyright © 2023 Xu, Zhou and Wu. This is an open-access article distributed under the terms of the Creative Commons Attribution License (CC BY). The use, distribution or reproduction in other forums is permitted, provided the original author(s) and the copyright owner(s) are credited and that the original publication in this journal is cited, in accordance with accepted academic practice. No use, distribution or reproduction is permitted which does not comply with these terms.
*Correspondence: Boran Wu, Ym9yYW53dUB0b25namkuZWR1LmNu