- Environment Coast and Ocean Research Laboratory, Universidad Politécnica de Madrid, Campus Ciudad Universitaria, Madrid, Spain
Coastal zones in Latin America support a significant regional population and are vital for providing essential ecosystem services that underpin crucial socio economic activities. However, these zones face pressing challenges, with coastal erosion being a prominent concern. Coastal erosion not only jeopardizes coastal stability but also leads to occasional loss of natural habitats. This is particularly worrisome for sandy beaches due to the consistent shoreline retreat in specific regions. Short term adverse effects of coastal erosion are often attributed to human activities. Nonetheless, the influence of hydrodynamic processes, intensified by extreme events, is gaining prominence. This is due to the combined impact of tides, waves, currents, and other factors, resulting in accelerated shoreline retreat and significant losses of infrastructure and beach areas across Latin America. This article conducts a comprehensive analysis of case studies in Latin America related to coastal erosion due to hydrodynamic processes. Employing a systematic review approach, data is extracted from Scopus and Web of Science databases. From an initial dataset of 701 records, 62 records meet strict eligibility criteria. Case studies present evidence of how hydrodynamic processes interact with various factors, encompassing oceanic climatic conditions, atmospheric climatic conditions, anthropogenic influences, geomorphological and bathymetric characteristics, geological and tectonic factors, and climate change related aspects. These interactions have an immediate and significant impact on sandy beaches, emphasizing the necessity of comprehensively addressing these linkages when analyzing shoreline changes. This approach aids in identifying key erosion mechanisms and developing effective mitigation measures. Furthermore, the article provides a concise overview of data sources, tools, and methodologies identified in the case studies, spanning from field investigations to the utilization of geographic information systems and numerical models. Underscores the crucial role of international academic collaboration in coastal erosion research, facilitating the adoption of innovative methodologies from other regions, potentially offering a more comprehensive perspective in the formulation of mitigation and adaptation strategies. Finally, potential areas for future research in Latin America are explored, including the assessment of extreme event variability and its impacts on local and regional hydrodynamic processes. This aspect holds critical importance in steering future research towards scenarios that encompass economic and social considerations.
1 Introduction
Coastal areas play a vital role in supporting human populations around the world, providing essential ecosystem services that form an important part of the economic base of many countries. Therefore, the natural attractions and the benefits that these areas offer have promoted their rapid urbanization in different countries. As a result, approximately 40% of the global population resides within 100 km of the coast (NASA, 2023; NSIDC, 2023). Another notable observation is the concentration of illuminated settlements within 5 km of the coastline (Small and Nicholls, 2003). However, these human settlements face significant vulnerabilities due to the dynamic nature of coastal environments, the occurrence of extreme events, and the loss of natural defenses due to anthropogenic causes.
Climate change has further exacerbated the challenges faced by coastal areas. Rising sea levels, alterations in coastal hydrodynamics, and intensified impacts of extreme events have accelerated the problem of coastal erosion. This not only leads to the loss of valuable coastline but also causes the destruction of coastal ecosystems and human settlements, which are along the low-lying sandy shores such as beaches (Hinkel et al., 2013; Toimil et al., 2017).
Coastal erosion poses a significant threat to sandy beaches worldwide, with 70% experiencing retreat (Bird, 1985). A study by Luijendijk et al. (2018) reveals that between 1984 and 2016, 24% of beaches exhibited erosion rates exceeding 0.5 m/yr. Furthermore, it is projected that during the 21st century, between 6,000 and 17,000 km2 of land will be lost, leading to the displacement of 1.6–5.3 million people, contributing to an increase in the number of climate-displaced individuals. The economic losses resulting from coastal erosion and sea level rise, together with other drivers, are estimated to range from 300 to 1,000 billion dollars (Kim, 2010). Projection models by Vousdoukas et al., 2020 considering different climate change scenarios (RCP 4.5 and RCP 8.5), identify Australia, Canada, and Chile as the countries with the highest sandy beach coastlines at risk of erosion, with losses surpassing 5,000 and 6,000 km. Following closely are Mexico, China, United States of America, Russia, and Argentina, with erosion-induced losses exceeding 2,000 and 3,000 km. While developed cities generally possess more resources to protect their densely populated coastal areas through measures like breakwaters and beach nourishment, they also face more complex challenges in managing and mitigating risks compared to dispersed rural communities (Small and Nicholls, 2003).
Additionally, it is crucial to consider the effects of the El Niño-Southern Oscillation (ENSO) in coastal management plans. The ENSO cycle involves variations in the surface temperature of the tropical eastern Pacific Ocean, resulting in warming known as El Niño and cooling known as La Niña. This cycle is correlated with increased sea surface temperatures in the tropical Atlantic, primarily caused by reduced northeasterly trade winds at the surface (Reguero et al., 2013). These events often have short-term consequences that typically require mitigation actions, except in exceptional cases where beaches are experiencing accretion prior to El Niño events (Barnard et al., 2017). El Niño events are associated with a higher frequency of tropical cyclones and elevated water levels in the Eastern Tropical Pacific, significantly impacting low-lying coastlines (Komar and Enfield, 1987; Barnard et al., 2017; NASA, 2019). These events also lead to increased energy and directional changes in waves, which amplify erosive processes, particularly on sandy beaches. The impacts of the El Niño phenomenon will be further intensified by climate change, thereby increasing the risk in coastal areas of the Pacific Ocean (Barnard et al., 2015). Furthermore, in the coastal regions of Peru, the connection between the Niño3 climate index and Mean Sea Level (MSL) has been observed, as presented in the study by Losada et al. (2013). In this study, it was concluded that for every unit change in the standardized Niño3 index, there is an estimated alteration in MSL of approximately 30.47 mm. Subsequent research, such as the study conducted by Jigena-Antelo et al. (2023), which utilized data from local monitoring stations, revealed significant increases in sea level during exceptional El Niño episodes. This was particularly evident in January 1983 and December 1997, with deviations of 0.38, 0.34, and 0.31 m compared to monthly averages. This study underscores the importance of conducting analyses using data from local monitoring stations, as global measurements provided by the Intergovernmental Panel on Climate Change (IPCC) differ from local observations. Until 2003, the rates of sea level rise were notably lower than those reported by the IPCC, ranging from 0.70 to 1.2 mm/year in contrast to the IPCC’s figure of 1.80 mm/year. Similarly, between 2006 and 2015, measurements at Peruvian stations recorded significantly higher values, ranging from 8.3 to 13.3 mm/year, as opposed to the IPCC’s estimate of 3.60 mm/year.
Furthermore, although variations in wave climate have been observed, a systematic trend similar to sea-level rise has not yet been identified (Reguero et al., 2019). However, an analysis of wave climate in Latin America and the Caribbean reveals an increase in the average annual wave height (these data can be consulted at https://c3a.ihcantabria.com/). During extreme storms, cyclonic surges generate high tide levels along the coast (Allan and Komar, 2002). These storm events cause coastal erosion due to a combination of high wave energy, increased infragravity or incidentally oscillating energies, rising sea levels, and potential wind-driven cyclonic surges, along with intensified rip currents and nearshore currents (Komar and Holman, 1986; Guido-Aldana et al., 2009). To understand how climatic events can impact the behavior of waves at a regional level, reference is made to the findings of the study conducted by (Martell-Dubois et al., 2018). This study focuses on analyzing wave characteristics during hurricanes in the Caribbean, using records of directional wave spectra to assess the intensity of incident energy and its distribution in terms of direction and frequency. The results indicate that in hurricanes like Wilma, the coexistence of local and remote waves is evident, generated under particular conditions during the intensity of these climatic events. This leads to the emergence of bimodality in both wave frequency and direction. A specific example is highlighted during Hurricane Wilma (October 2005), where the distance between the hurricane’s center and the observation point allowed for the significant presence of both types of waves, characterized by high energy. However, other research indicates a significant decrease in extreme events in the tropical Pacific Ocean region overall. In the Eastern Tropical Pacific region, where the El Niño-Southern Oscillation (ENSO) phenomenon originates, is expected to experience a strong increase in average conditions and uncertainty in extreme wave heights (Lobeto et al., 2021; CHW Platform, 2023). An example is that of the Chilean coasts, where an erosive process has been observed on certain beaches, attributed to the increase in extreme storms during the warm phases of ENSO phenomenon. Interestingly, although no significant historical changes have been recorded in wave height, there has been a remarkable increase in the frequency of these storms. In the past, an average of around 5 storms per year was recorded during the mid-20th century, while today, this figure has risen to 20 annual storms (Martínez et al., 2018).
The coasts of Latin America are exposed to coastal erosion due to natural causes and extreme hydrometeorological events. These frequent events result in economic losses in coastal tourism (Silva et al., 2014). Phenomena such as hurricanes and intense swells have led to the loss of beaches and flooding in Latin American cities. The southern region, particularly the Atlantic coast, has a higher exposure to cyclonic surges (Losada et al., 2013). Changes in wave conditions have been observed in various regions, with increases in average annual wave height on the Pacific coast of Mexico, southern Brazil, Uruguay, and northern Argentina (CEPAL, 2015a). Limited knowledge of coastal dynamics and scarcity of local data on waves and atmospheric conditions pose challenges for coastal risk management in Latin America (Reguero et al., 2013; Silva et al., 2014). Coastal erosion represents a significant threat to communities and economies along the Latin American coast. It is crucial to understand the relationship between this issue and climate change to develop better adaptation and mitigation strategies that protect vulnerable communities from the adverse effects of erosion. Different coastal regions in Latin America heavily rely on economic activities such as tourism, fishing, aquaculture, among others, further exacerbating the socio-economic consequences associated with coastal erosion, including infrastructure loss, community displacement, and economic disruption.
To conduct a systematic review focus of the available literature to analyze the impacts of hydrodynamics processes on coastal erosion in Latin America. To the best of our knowledge, there have been no literature reviews specifically addressing this relationship. Therefore, this study aims to answer the following research question: How have hydrodynamic processes influenced coastal erosion impact in Latin America? A systematic literature review methodology will be employed to identify the findings and trends reported in the existing scientific literature. The study contains following sections: 1) Introduction, 2) Systematic review methodology, 3) Climate, environmental, and geomorphological characteristics: Latin America context 4) Identification of key drivers of coastal erosion, 5) Cases studies of coastal erosion influenced by hydrodynamic processes in different coastal zones, 6) Data sources, tools and methods commonly use in studies of coastal erosion in Latin America, 7) Discussion. This systematic review is expected to contribute to scientific knowledge by providing a synthesis of existing studies on local cases of coastal erosion in Latin America caused by hydrodynamic processes amplified by external factors. Additionally, it aims to identify the most used techniques for studying shoreline changes in the region, as well as potential topics for future research related to coastal erosion. The findings of this study could be valuable for coastal managers, decision-makers, and other stakeholders interested in developing effective mitigation and adaptation strategies against coastal erosion in the region.
2 Systematic review methodology
The methodology used to collect and select studies on coastal erosion related to variations in hydrodynamic processes in Latin America corresponds to a systematic review. This review was conducted following the guidelines of the Preferred Reporting Items for Systematic Reviews (PRISMA) (Moher et al., 2009; Yepes-Nuñez et al., 2021). The PRISMA literature review guide provides a series of guidelines throughout the review process, primarily ensuring the establishment of a specific research question that allows for the search of a defined topic. Figure 1 illustrates the process of scientific article selection using the PRISMA systematic review methodology. Additionally, these guidelines ensure the establishment of different exclusion and inclusion criteria to obtain results that are limited to the topic of interest. The procedure executed for the presented systematic review is described in the following sections.
The systematic review was conducted in April 2023, with a cut-off date for document extraction set on 14 April 2023. The consulted databases were Scopus and Web of Science (WoS), as they are widely used and known for their reliability in providing diverse literature. A search was performed in these databases to identify general topics related to coastal erosion and its relationship with hydrodynamic processes, to detect the most frequently recurring keywords in various worldwide publications. This process resulted in the construction of a list of relevant keywords for the research. After selecting the keywords to be used for the systematic review, a search equation was developed and tested through multiple selection tasks in Scopus and WoS. In the provided search equation, different terms related to hydrodynamic processes and coastal hazards were combined, along with terms associated with coastal erosion on sandy beaches. The selected countries for this research defined the study area within Latin America. Additionally, Boolean operators (i.e., OR, AND) were used to establish the defined associations, and an asterisk (*) was employed for word truncation to account for variant endings in the keywords when necessary.
During this preliminary revision, keywords that did not significantly affect the quantity of retrieved data were identified and eliminated, ensuring that unnecessary terms were not included in the search. The keywords equation used in the Scopus and WoS databases were as follows ((“Tide*” OR “Current*” OR “Wave climate” OR “Wave condition*” OR “Wave direction*” OR “Storm surge” OR “Coastal dynamics” OR “Sea level rise” OR “Coastal flood*” OR “Coastal process*” OR “Coastline retreat” OR “Coastal hazard*” OR “Shoreline retreat” OR “Shoreline chang*” OR “Shoreline evolution” OR “Sandy beach*” OR “Sandy shoreline” OR “Climate change” OR “El Niño-Southern Oscillation” OR “ENSO”) AND (“Coastal erosion” OR “Beach erosion” OR “Shoreline erosion”) AND (“Latin America” OR “Central America” OR “South America” OR “Mexico” OR “Guatemala” OR “El Salvador” OR “Honduras” OR “Nicaragua” OR “Costa Rica” OR “Panama” OR “Colombia” OR “Ecuador” OR “Peru” OR “Chile” OR “Venezuela” OR “Brazil” OR “Uruguay” OR “Argentina”)).
The selection criteria for this research involved considering all types of documents within the Scopus and Web of Science (WoS) databases (e.g., research articles, conference papers, books, book chapters, etc.), with a limitation to documents written in English and Spanish. The database search yielded a total of 701 identified records, of which 405 were from Scopus and 296 were from WoS. Upon comparing both databases, 172 duplicate records were removed as they appeared in both Scopus and WoS. This resulted in a total of 529 records for review to determine if they met the selection criteria. The exclusion criteria established were as follows: records corresponding to areas outside the selected study zones were removed (eliminating 135 records), records not directly related to the research objective based on the abstract were excluded (eliminating 147 records), and records were eliminated due to lack of access to information (eliminating 15 records). After applying these selection criteria, a total of 232 articles remained for a comprehensive review. Subsequently, an additional selection criterion was applied, excluding articles that were not directly related to the research objectives (eliminating 134 records). This left a total of 94 records to be evaluated for eligibility, excluding 18 without defined causes of coastal erosion and 14 records focused on atmospheric and oceanographic factors. Finally, a total of 62 records addressing case studies of coastal erosion associated with hydrodynamic processes were included for this review.
3 Geomorphology, climate, and population of coastal zones in Mexico, Central and South America
Mexico is one of the countries in the Latin American region with a large coastline both on the Pacific and Atlantic sides. The Pacific coast of Mexico has a longer extension than the Atlantic coast and features a straight shape along with a rugged and mountainous landscape, due to the presence of numerous sierras and mountain ranges that descend towards the sea. On the other hand, the Atlantic coast of Mexico has two distinct characteristics compared to the Pacific coast. Firstly, it is divided into two regions: the Gulf of Mexico and the Caribbean Sea. Secondly, the extent of this coast is shorter and exhibits a more sinuous shape due to the presence of peninsulas and bays. The beaches on this coast include extensive stretches of sand and coral reefs, making it home to various coastal ecosystems (CEPAL, 2015a).
The coastal zones of Central America exhibit a higher concentration of peninsulas, gulfs, and bays, as well as intertidal areas and sandy barrier systems. Central America is one of the world’s most seismically active regions due to the interaction of six tectonic plates: North America, Caribbean, Cocos, Panama, Nazca, and South America (CEPAL, 2015a). Along the Pacific coast, well-developed cliffs can be observed in Costa Rica and partially in El Salvador, Nicaragua, and Panama. In contrast, the Atlantic coast tends to be flatter, with limited presence of cliffs (except in Guatemala). The Pacific coasts of Central America are characterized by intricate and rocky landscapes alternating with sandy beaches, deltas, estuaries, and rich ecosystems such as mangroves and coral reefs. On the other hand, the Caribbean coasts are generally sandy and elongated, featuring coral reefs such as the Mesoamerican Barrier Reef System, found in southern Costa Rica and the Bocas del Toro and San Blas archipelagos in Panama. Additionally, the region is home to extensive wetlands that include estuarine ecosystems, defining the transition between riverine plains and the Caribbean Sea (Bergoeing, 2015; Quesada-Román et al., 2022).
In South America the Pacific coast is characterized by a mountainous relief due to the presence of the Andes Mountains, which is why the Pacific coastal shelf is relatively narrow and features small drainage basins. The coastline is marked by elevated landforms, alternating with embedded sandy beaches, rocky coastlines, and mangrove areas. The Atlantic coast of South America exhibits a lower relief and a broader coastal shelf. This coast is characterized by extremely large drainage basins (Blanco-Chao et al., 2014; Psuty and Mizobe, 2014). Along the coast, one can find embedded beaches, pebble beaches with various rock fragments, and long sandy beaches extending from Rio Grande do Sul to the northeastern region of Uruguay (Psuty and Mizobe, 2014). The countries in South America with the longest stretches of sandy beaches include Mexico, Brazil, Argentina, Chile, and Peru, with Brazil having the most urbanized sandy coastlines.
In general, Latin America is characterized by the presence of straight beaches that are more susceptible to sea-level changes and wave intensity. Bay beaches are also common in this region and are influenced by changes in the dominant wave direction (CEPAL, 2015d). Straight beaches are predominant along most of Mexico’s coasts (especially in the Gulf of Mexico) and in Brazil, while bay beaches dominate the coasts of Chile (CEPAL, 2015b). Sandy beaches in Latin America are influenced by hydrodynamic processes, which contribute to a variety of wave energy environments. In the Pacific, during spring tides, tidal amplitudes can reach up to 6 m, as can be observed in places such as Puerto Montt (Chile) and in Balboa in the Bay of Panama (Panama). In the Caribbean, these significant amplitudes are recorded in specific zones during spring tides, such as Comodoro Rivadavia and Punta Loyola (Argentina) (Figure 2) (National Oceanic and Atmospheric Administration, 2020, Historic Tide and Tidal Current Tables). The Caribbean coasts are characterized by microtidal ranges, with spring tides of less than 1 m (Blanco-Chao et al., 2014). Dominant winds can generate waves up to 3 m high on the Atlantic coast, whereas wave heights are generally lower on the Pacific coast (CEPAL, 2015a). The level of risk differs between the Caribbean and Pacific coasts, with the former being more susceptible to sea-level changes and the latter facing higher risks due to variations in wave intensity (CEPAL, 2015c).
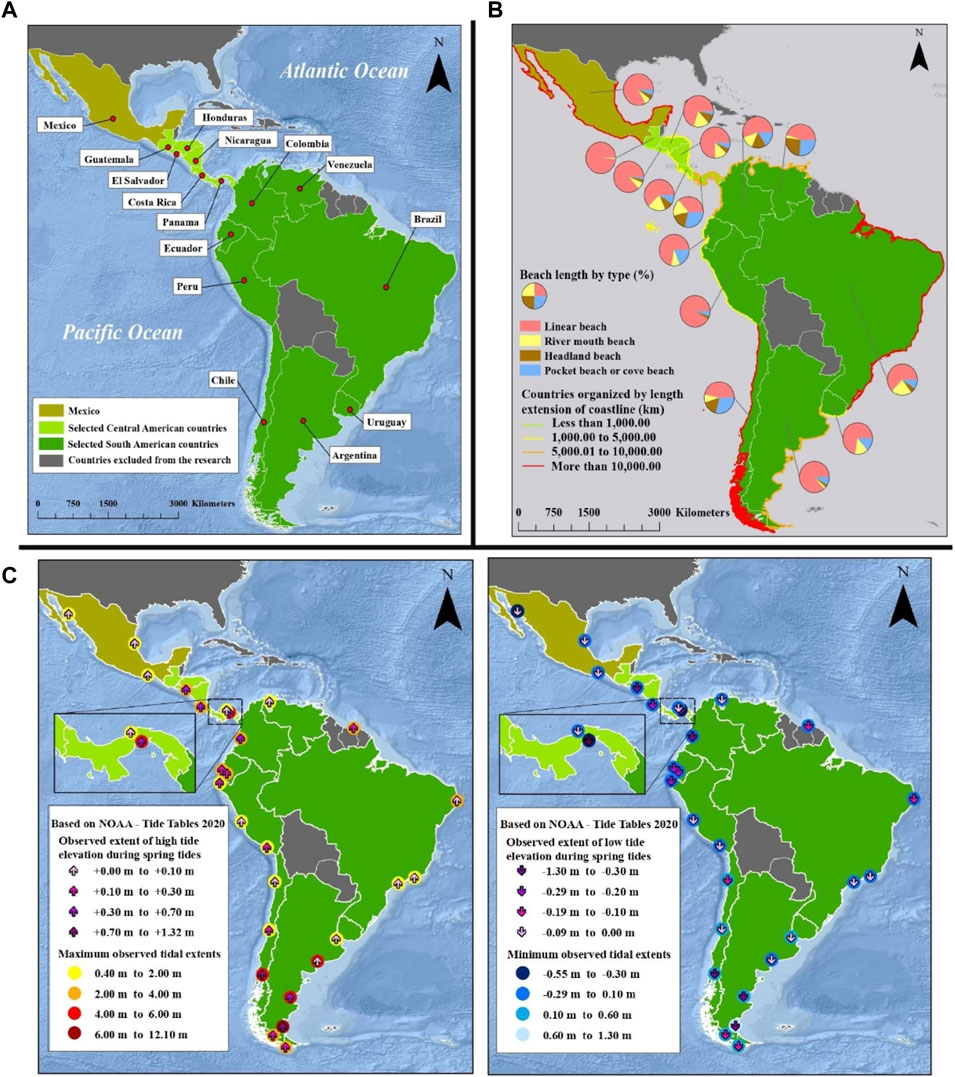
FIGURE 2. (A) Countries of continental Latin America included in the search equation for the systematic review are indicated. (B) Data on total coastline length per country, as well as the categorization by beach type and percentage per country, are shown based on data presented in IHCantabria—CEPAL 2015d. Note: Linear beach—refers to beaches with a straight shape without significant curves or irregularities in their orientation, River mouth beach—refers to beaches adjacent to the mouth of a river, Headland beach—considers beaches that have rigid confinement at one of their ends, Pocket beach or cove beach—includes all beaches that have rigid confinement at both ends. (C) Approximate maximum and minimum tidal data observed in the tidal table provided by the National Oceanic and Atmospheric Administration (NOAA) for the year 2020, as well as the additional approximate increase or decrease in tidal levels during the occurrence of spring tides.
The climatic conditions of Mexico, Central America, and parts of South America is influenced by the El Niño-Southern Oscillation (ENSO), which causes alternating cycles of intense drought and precipitation. Some of the effects of ENSO are related to seasonal changes in mean sea level in Colombia and severe impacts from wave action in coastal areas of Ecuador. Additionally, this region is affected by cold fronts, the migration of the Intertropical Convergence Zone (ITCZ), and trade winds (Garcia et al., 2009; Quesada-Román et al., 2022). Both Mexico and Central America are vulnerable to the presence of tropical storms and hurricanes that form in both the Pacific and Atlantic. However, South America is rarely affected by tropical cyclones, which are generally limited to the Atlantic and occur mainly at low latitudes along the coasts of Colombia and Venezuela (Garcia et al., 2009; Calil et al., 2017). On the other hand, a phenomenon known as “sudestada” is observed in South America, characterized by strong southeast winds that primarily affect the eastern coast of Buenos Aires province in Argentina and areas adjacent to the Río de la Plata. This meteorological event occurs when specific atmospheric conditions combine, including the presence of a high-pressure system over southern Argentina and the Atlantic Ocean, along with a low-pressure system to the north of Buenos Aires, Uruguay, and southern Brazil. These conditions generate intense southeast winds that can result in adverse maritime conditions, coastal flooding, and changes in water levels in the Río de la Plata (Garcia et al., 2009).
Coastal zones of Latin America face numerous climate-related challenges, including erosion and increased vulnerability. With a collective population of around 610 million, most coastal states have more than three-quarters of their population living within 200 km of the coast (Ayyam et al., 2019). It is important to highlight that countries such as Brazil, Colombia, Venezuela, Costa Rica, El Salvador, Panama, and Ecuador have over 30% of their population concentrated in the Low Elevation Coastal Zone (LECZ), this zones are characterized by having an altitude of less than 10 m above sea level along the coastline, making them particularly susceptible to flooding, rising sea levels and other impacts of climate events (McGranahan et al., 2007; Calil et al., 2017). Latin America is expected to experience significant population growth in the next century, becoming the second-largest population increase globally. Furthermore, it is estimated that the coastal population residing in low-lying coastal areas will increase by 80% for the next century (Silva et al., 2014). This rapid population growth poses additional challenges for coastal management and planning, requiring proactive measures to address the growing vulnerability of coastal communities to climate events (Villamizar et al., 2017).
4 Identification of key drivers of coastal erosion in sandy beaches
The identification of various factors that contribute to coastal erosion is presented in this section. These factors have been classified in an interdependent manner, as shown in Figure 3, which also indicates the relationship that each factor has with coastal erosion. To visualize this information, the Ishikawa diagram or cause and effect diagram has been used, which is used to present a central problem and the different causes associated with it. To support this research, previous studies conducted by Codignotto, 1997; Silva et al., 2014 have been considered, who analyzed case studies in coastal areas of the South American region and identified erosion drivers. In this study, we have expanded the identification of driving factors of coastal erosion in the region through the analysis of 62 case studies.
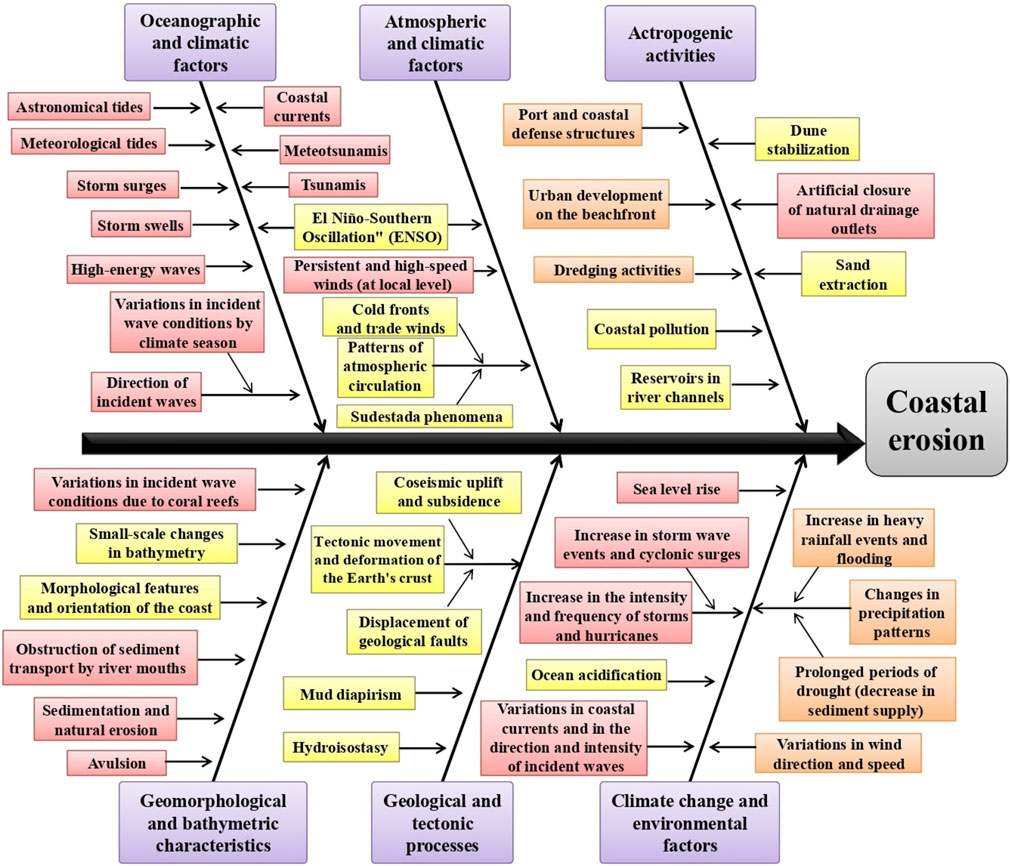
FIGURE 3. Identification and classification of coastal erosion drivers into six main categories (represented by purple), according to different factors categorized into primary and secondary levels based on their direct influence (represented in red), indirect influence (represented in yellow), and mixed influence (represented in orange).
4.1 Oceanographic and climatic factors
Hydrodynamic processes such as tides, waves, and currents play a fundamental role in the shape of sandy beaches. However, they can also have a negative effect on beaches, such as meteorological tides associated with weather systems like cold fronts or storms (Parise et al., 2009). This includes positive cyclonic surges that raise water levels and generate more energetic waves (Diez et al., 2007; Pousa et al., 2013). Another phenomenon included in Argentina is known as “meteorological tsunamis,” which induce coastal erosion and are generated by atmospheric processes such as atmospheric waves, rapid pressure changes, or wind gusts. However, their probability of occurrence is low and limited to specific locations such as the region between Mar de Ajo and Quequen on the southeast coast of Argentina (Dragani et al., 2014). On the other hand, tsunamis generated by movements in the seafloor can erode coastal systems. During these events, sediments are suspended and loosely compacted, making them susceptible to removal or deposition by the action of waves, tides, or coastal currents (Soto et al., 2015). Additionally, littoral currents parallel to the coast derived from wave action also have a direct impact on sediment transport and contribute to wave transformation, intensifying erosion, or sediment accumulation processes (Oliveira et al., 2020). However, the influence of currents can be enhanced or disrupted by the presence of port structures, river mouths, and the coastal morphology itself, which affects sediment transport.
In the South American region, numerous studies have identified wave action as the main cause of coastal erosion. High-energy waves, the angle of incidence on the coast, and transformations caused by natural obstacles (such as islands and coral reefs), as well as artificial obstacles (such as breakwaters or seawalls) and bathymetry, have a severe and short-term impact on sandy beaches (Gomes and Da Silva, 2014; Martins and Pereira, 2014; Fernandino et al., 2018). Variability in wave direction and intensity due to seasonal climate changes can amplify erosion and accretion processes in the area, especially during extreme events like storms or hurricanes. During these events, high-energy wave surges impact the coast, eroding the beach profile and prolonging its recovery time (Mulcahy et al., 2016; Rangel-Buitrago et al., 2020; Pinheiro et al., 2021). Additionally, during years when the El Niño-Southern Oscillation (ENSO) occurs, greater severity in coastal erosion has been observed due to its influence on the coastal climate of the region (Martínez et al., 2022; Vallarino-Castillo et al., 2022).
4.2 Atmospheric and climatic factors
During the occurrence of ENSO, precipitation patterns in Central America can experience notable effects. In Panama, a relationship has been observed between coastal erosion and the lack of sediment input from rivers that flow into the studied beaches. This climatic phenomenon also influences coastal currents in the Bay of Panama, which could worsen the problem of coastal erosion (Vallarino-Castillo et al., 2022). In the South American region, a significant relationship has also been observed during El Niño episodes with variations in precipitation that affect the river flow in the Andean basins of Chile (Martínez et al., 2022).
In the Intertropical Convergence Zone (ITCZ) in the Caribbean region of Colombia, during the dry season, trade winds generate high-energy swells (Cueto et al., 2022). In the Caribbean region of Costa Rica, the high energy in swells is intensified by tropical storms, resulting in significant variations in the beach profile between winter and summer (Barrantes-Castillo et al., 2021). In the Yucatan Peninsula of Mexico, tropical cyclones formed in the Atlantic Ocean and local winds from the east and northeast affect hydrodynamic processes such as currents and wave propagation, which in turn influence coastal erosion (Escudero-Castillo et al., 2018). In the Mar del Plata area, southeast winds raise the water level, creating discrepancies between predicted and observed tides (Pousa et al., 2007; Pousa et al., 2013). Similarly, the swells recorded in the southernmost region of Brazil are closely related to south winds caused by the passage of cold fronts, resulting in high-energy swells in the region (Esteves et al., 2003).
4.3 Anthropogenic activities
Anthropogenic interventions on the coast have caused significant changes in the coastal landscape. Activities such as beach sand extraction, construction of coastal structures, and urban development in areas near the beach have altered the natural dynamics and created imbalances in sediment transport (Pousa et al., 2007; Codignotto et al., 2011). In some cases, dune stabilization has been carried out to prevent sediment loss. However, this has led to the formation of sandbanks at port entrances, affecting sediment supply to the beaches and causing coastal erosion (Merlotto et al., 2014; Medina et al., 2016). Furthermore, the artificial closure of natural drainage systems, sand mining activities, dredging, and the construction of upstream dams in rivers affect the sediment balance in coastal areas. The closure of natural drains during road construction, which are only temporarily opened during heavy rainfall, releases an excess of sediments, disrupting the dynamic equilibrium of the coast (Esteves et al., 2003; Dillenburg et al., 2004). These anthropogenic interventions have resulted in long-term changes that have led to a situation of coastal compression or reduction of available beach space (coastal squeeze). Narrow beaches provide less protection against storms, increasing the risk of coastal erosion (Ortega et al., 2013). In environments where beaches remain in a natural state without human intervention, they tend to naturally recover after events such as storms (Pousa et al., 2007).
4.4 Geomorphological and bathymetric characteristics
The morphology of the coastline and the bathymetry influence coastal erosion processes. Straight beaches are prone to erosion due to hydrodynamic processes compared to beaches in protected bays (Fernandez et al., 2011). Bathymetry can induce coastal erosion by causing wave convergence due to small-scale changes (Dillenburg et al., 2004). These areas where small-scale changes in bathymetry occur are known as “bathymetric lenses,” a concept proposed by (Speranski and Calliari, 2001), referring to areas on the seafloor with convex characteristics that act as lenses. Additionally, the presence of artificial reefs can also alter wave direction and increase energy in specific coastal areas (Gomes and Da Silva, 2014; Martins and Pereira, 2014; Fernandino et al., 2018). These areas where wave energy is concentrated on the coast are called “focuses of surf” a concept proposed by Glushkov in 1935 and often associated with erosive phenomena or changes in coastal morphology (Speranski and Calliari, 2001).
Another cause, that leads to erosion on the coast is variations in river mouths, which act as hydraulic barriers blocking littoral drift. When the flow of rivers is altered due to increased water and sediment input, sediment accumulation occurs at the river mouth, disrupting the dynamic equilibrium of the coastal system (Addad and Martins-Neto, 2000). Additionally, phenomena such as “avulsion,” which refers to a sudden and natural change in the course of a river, can also alter the dynamic equilibrium of the coast, causing erosion and flooding in areas that normally did not experience these events (Coca-Domínguez and Ricaurte-Villota, 2019).
4.5 Geological and tectonic processes
Tectonic movements such as uplift and subsidence of the Earth’s crust can generate erosion events on the coast, as they disrupt the dynamic equilibrium (Martínez et al., 2022). Compressional tectonic activity that influences the formation of diapirs, which is a process where subterranean material is uplifted to the surface through cracks and fractures in the Earth’s crust, can also alter coastal morphology and stability due to sediment obstruction (Correa, 1990; Vernette and Gayet, 1992; Restrepo-Ángel et al., 2021). Additionally, changes in the morphology of some regions in South America are related to slow subsidence due to hydroisostasy. This refers to changes in sea level caused by the redistribution of water masses due to sediment loading or unloading or changes in the elevation of the Earth’s crust (Rostami et al., 2000; Correa and Vernette, 2004).
4.6 Climate change and environmental factors
Climate change has contributed to an increase in the frequency and intensity of weather phenomena such as storms and variations in wind speed in some regions. In the northern coast of Argentina, Uruguay, and the southern coast of Brazil, the increase in sea surface temperature (SST) has led to a higher frequency and duration of storms from the southeast. Additionally, in the southern coast of Brazil (an area dominated by north winds), a similar pattern associated with cold fronts and strong south winds has generated high-energy waves that induce changes in beach morphology (Dillenburg et al., 2004; Ortega et al., 2013). The climate variability associated with climate change is still a topic that requires further research; however, various studies conclude that in the future, there will be an increase in the frequency and intensity of extreme events such as hurricanes and wave climate (Lopez, 2014; Mulcahy et al., 2016; Camus et al., 2017). This could significantly increase the impacts on coastal areas, mainly on beach profiles, as their recovery largely depends on the time between these events (Mulcahy et al., 2016). ENSO events will become more intense, and in scenarios of high greenhouse gas emissions, the precipitation variability in the central and eastern tropical Pacific is expected to increase significantly (Cai et al., 2021; Liberto, 2021). Additionally, ocean acidification will amplify its negative impact on coral reefs, also affecting organisms that sustain vital coastal habitats such as mangroves and seagrass meadows. As a result, it is possible that new areas will be exposed to erosive processes that could cause flooding in the future. This, along with the rise in sea level, will also contribute to the transgression of coastal dunes. During periods of sea level rise, waves and tides can advance inland, surpassing the dunes and depositing sediments in previously stable areas (Dillenburg et al., 2004; Losada et al., 2013; IPCC, 2022).
5 Cases studies of coastal erosion influenced by hydrodynamic processes in Latin America
5.1 Argentina
On the east and south Atlantic coast of Argentina, storm surges have been identified as the main driver of coastal erosion (Merlotto et al., 2014). In the Mar del Plata region, southeast winds raise the water level, creating differences between predicted and observed tide levels. These effects are amplified in conjunction with wave action due to the bathymetry of the area and coastal landscape degradation (Pousa et al., 2007; Pousa et al., 2013). As a result, erosion rates exceeding −0.40 m/year have been recorded in areas such as Necochea, Bahía de los Vientos, and Quequén, while in areas like Pinamar, Villa Gesell, and Balneario Parque Mar Chiquita (Figure 4), erosion rates can exceed −2 m/year (Merlotto et al., 2014). In the case of the Rio de la Plata region, the most severe impacts are generated by positive cyclonic storm surges. These are caused by the southeast winds that impede drainage into the Atlantic and result in flooding events known as “sudestadas” (Pousa et al., 2013). Additionally, weather tsunamis in this area tend to be more frequent compared to cyclonic storm surges. When both events occur simultaneously, the impacts on the coast increase, reaching the upper sandy beach and affecting areas in the province of Buenos Aires (Dragani et al., 2014).
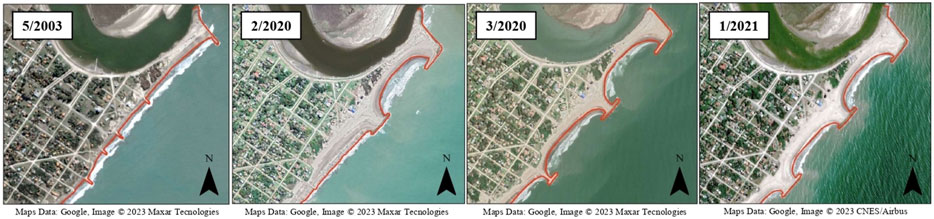
FIGURE 4. Example of alteration of shoreline (represented in red) due to the presence of coastal defense structures in Balneario Mar Chiquita, Argentina.
The Rio de la Plata region and the east and south Atlantic coast of Argentina are influenced by climatic and oceanographic processes that generate positive storm surges in one area and negative storm surges in another. An example of this is the storm events in July 2009, during which positive storm surges with large waves were recorded on the coast of the east and south Atlantic front of Argentina. The same event generated negative storm surges in the Rio de la Plata region due to southwest winds (Pousa et al., 2013). Although progress has been made in the study of these events, it is still not possible to accurately quantify their impact due to the degree of human intervention along the coast, which hinders the quantification of natural processes. Therefore, in Samborombón Bay, being an area without human intervention, is a potential location for studying coastal erosion due to natural causes such as variations in wave height and direction, “sudestadas,” and sea-level rise (Codignotto et al., 2011; Bacino et al., 2019; Lipp, 2019).
5.2 Brazil
The coasts of Brazil, both in the north and south, experience coastal erosion processes. In the south, “hot spots” have been identified where hydrodynamic processes are more relevant to shoreline loss. On the coast of Rio Grande do Sul (RS), erosion of 41% has been recorded, mainly affecting the beaches of Hermenegildo and Faro de Conceição (Alves, 2009; Nicolodi et al., 2021). Northeast waves in summer and southwest waves in winter contribute to coastal instability in this area, along with cyclonic storm surges that sometimes exceed 1.50 m in height and intensify erosion processes when they coincide with high tides (Esteves et al., 2003; Silva et al., 2016). Additionally, the configuration of the RS coastline affects sediment transport and the distribution of wave energy and incidence angle (Esteves et al., 2006).
The beaches of Hermenegildo and Faro de Conceição in Rio Grande do Sul (RS), Brazil, undergo erosive processes due to the concentration of wave energy generated by small-scale changes that result in the convergence of wave rays at certain points along the coast known as “focuses of surf” (Esteves et al., 2002; 2003; Calliari et al., 2003; Dillenburg et al., 2004; Nicolodi et al., 2021). These bathymetric effects extend from Faro de Conceição to Cabo de Polonio in Uruguay. Furthermore, increased storm magnitude during certain periods can increase the severity of coastal erosion in the Faro de Conceição area (Barletta and Calliari, 2003). During different stages of storm system evolution, wave convergence may remain stable, resulting in areas with a focus on migrating high waves (Speranski and Calliari, 2001). At Cassino Beach, also in RS, severe erosion processes were recorded between June 2006 and July 2007 due to the occurrence of cyclone events generated in southern Uruguay. These erosive processes depend not only on the intensity and magnitude of extreme events but also on the previous state of the coastal profile (Parise et al., 2009). Other erosion points in the northernmost part of RS, such as Praia do Farol and Mostardense, are mainly due to the direction and energy of incident waves, with erosion rates ranging from −1 m/year to −6 m/year. Similar to the rates at Faro de Conceição and the beaches of Hermenegildo, which range from −0.5 m/year to −3.6 m/year (Figure 5) (Calliari et al., 2003; Esteves et al., 2008; Simões et al., 2022).
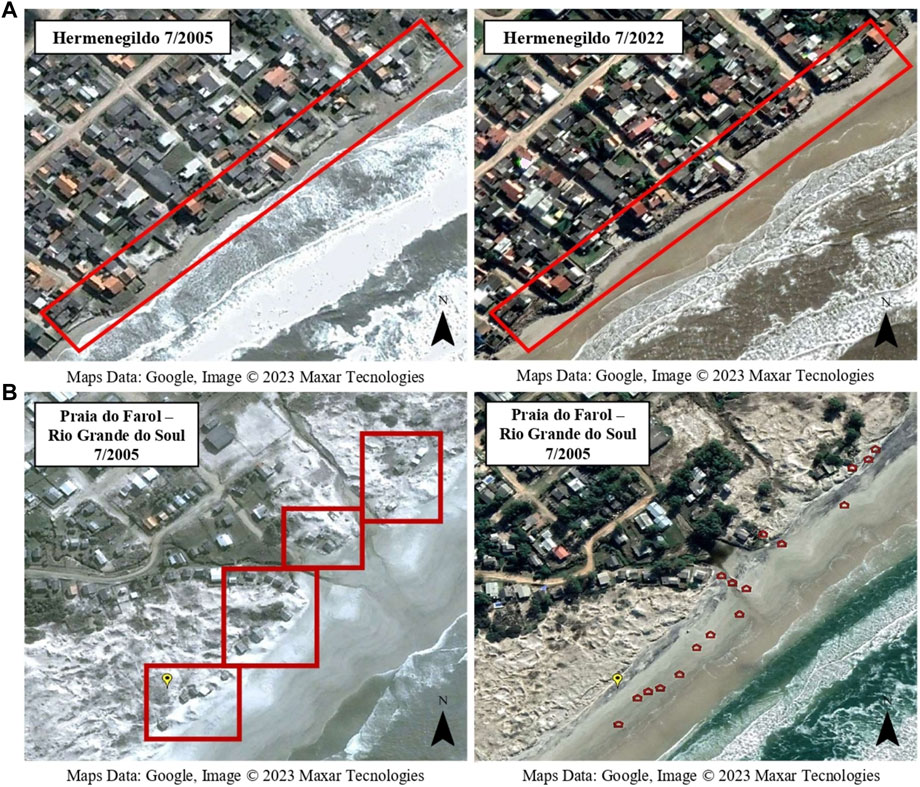
FIGURE 5. (A) Hermenegildo Beach - Increase installation of coastal protection in front of houses (red box). (B) Praia do Farol—Loss of properties along the beachfront (red box).
In Praia da Barra in Santa Catarina, Brazil, areas of erosion caused by waves generating longitudinal currents parallel to the coast have been identified. This has caused damage to coastal structures built to mitigate the impact of these hydrodynamic processes. However, these structures hinder sediment transport, resulting in a decrease in beach profile due to wave reflection and an increase in current intensity in the area (Oliveira et al., 2020).
In Rio de Janeiro, areas of erosion have been identified due to morphological features in the form of arcs, especially between Cabo Buzios and Cabo Frio, and between Rio das Ostras and Macaé. In these areas, the northern part tends to erode during storm events, while the southern part tends to sediment (Fernandez et al., 2011). In the Bay of Ilha Grande, erosion has been observed in places such as São Gonçalo, Taquari, Prainha, and Jabaquará, due to the combination of storm waves, tides, and a low sediment transport rate. These areas are less exposed to wave action but experience severe effects during high-energy wave events, such as flooding, dune retreat, and loss of urban and coastal infrastructure (Pinheiro et al., 2021).
The presence of coral reefs can contribute to beach loss due to the alteration of incident waves. This is the case of Tinharé-Boipeba Archipelago, waves arrive nearly perpendicular to the coast in the southern part of Boipeba Island due to the coral reefs. This also occurs in the central-southern region of Tinharé, where waves diffract and arrive nearly perpendicular to the coast. Coral reefs can increase wave energy and coastal currents, even when they are not directly in front of the beach, as in the case of Garapuá and Moreré (Elliff et al., 2019). In the state of Bahia, areas of erosion have been identified due to the presence of coral reefs, such as Pau Amarelo Beach and Porto Seguro (Martins and Pereira, 2014; Fernandino et al., 2018). A notable case is Candeias Beach, where the formation of reefs off the coast created an opening known as “Abreus Bar,” which generated a deepwater channel and exposed the beach to high sediment transport. Although a coastal structure was built to remedy the situation, it had to be modified due to its impact on the dynamic equilibrium of the beach (Figure 6) (Gomes and Da Silva, 2014). Other cases of coastal erosion due to high-energy waves include Maria Farinha Beach, where erosion problems occur due to high-energy waves, and Casa Caiada and Rio Doce beaches, where groins and parallel breakwaters were constructed to combat erosion but altered the dynamic equilibrium of the area and created the formation of small bays along the beach (Pereira et al., 2006; Mallmann and Pereira, 2014).
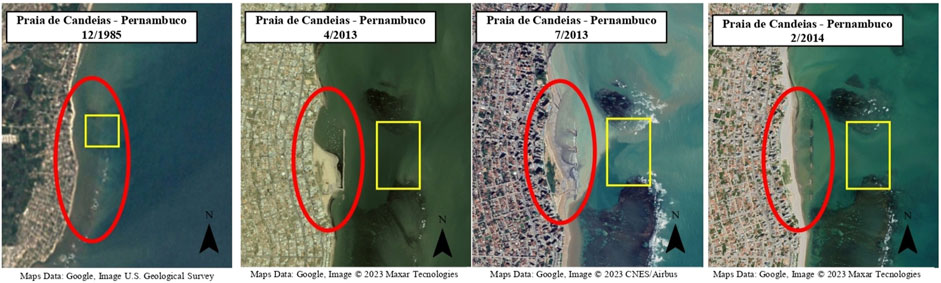
FIGURE 6. Case Study at Candeias Beach, Pernambuco, Brazil. This image depicts the significant influence of reef formations on wave transformation, resulting in notable changes to the beach morphology (highlighted in red). These alterations are attributed to an opening in the reef line known as the “Abreus Bar” (highlighted in yellow).
5.3 Colombia
The Atlantic and Pacific coasts of Colombia present differences in tidal variations, with the Atlantic being microtidal and the Pacific ranging from mesotidal. The Atlantic coast faces erosion issues due to extreme events such as hurricanes, cold fronts, and sea-level rise, resulting in infrastructure damage and coastline retreat (Cueto et al., 2022). In Cartagena, approximately 71.7% of the coast experiences severe erosion problems, with setbacks of up to 50 m due to extreme events (Rangel-Buitrago et al., 2020; Cueto et al., 2022). Furthermore, extreme events like hurricanes that generate strong winds and storm surges along the coasts are intensified by trade winds from the east-northeast, prolonging beach recovery time (Rangel-Buitrago et al., 2020).
In Caribbean region of Colombia, multiple causes of coastal erosion related to hydrodynamic processes are identified in different departments. These include sand retention and sediment movement difficulties due to groins in La Guajira, high erosion due to fractures and faults in Magdalena, large-scale sand migration and bathymetric features in Atlántico, wave energy concentration and sea-level rise in Bolívar, sedimentary imbalances due to coastal protection structures in Sucre, coastal retreat due to sediment deficiency in Córdoba, factors such as sea-level rise and tectonic movements in Antioquia, and sedimentary imbalances due to ecosystem destruction in the San Andrés and Providencia Islands (Rangel-Buitrago et al., 2015).
Although, in the Pacific region of Colombia, specifically in the La Barra area, coastal erosion is attributed to an avulsion process that generated a new river mouth and altered the dynamics of the coastal system (Figure 7). This resulted in erosion towards the west of the littoral bar and fluvial erosion towards the east. The opening of this new river mouth accelerated the retreat of the coastline, affecting houses and kiosks. These processes are attributed to changes in tidal flow dynamics, exposure to waves, changes in intertidal bars, and episodes of high tides. These erosions have had a significant impact on the communities of La Barra, which are in an area influenced by the hydrodynamics of the San Juan River delta (Coca-Domínguez and Ricaurte-Villota, 2019).
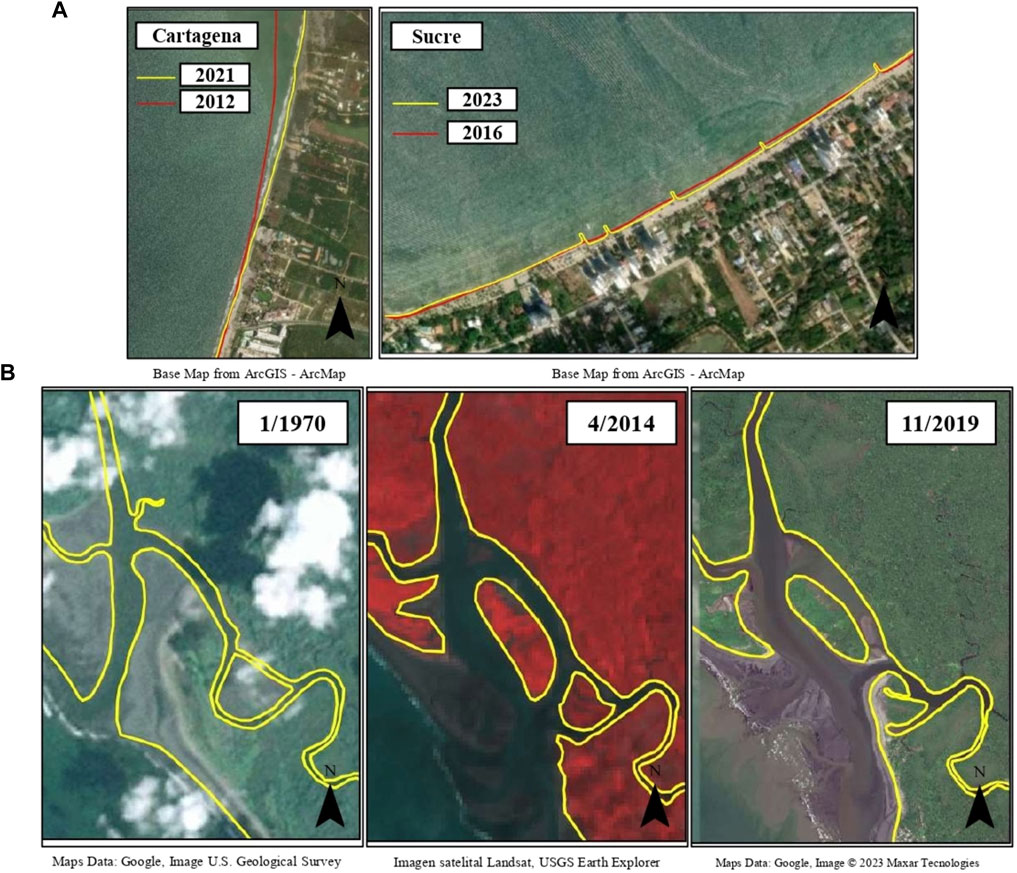
FIGURE 7. (A) Atlantic Coast of Colombia - variations in the shoreline in Cartagena and Sucre (highlighted in red and yellow). (B) Pacific Coast of Colombia—avulsion process due to the formation of a new river mouth in La Barra, Buenaventura, Colombia.
5.4 Costa Rica
The case of Cieneguita Beach in the Atlantic coast of Costa Rica, hydrodynamics especially wave height and energy, play a key role in coastal erosion processes. During winter, wave intensity increases due to trade winds and tropical storms, resulting in concave beach profiles. In contrast, during spring, wave activity decreases, allowing for beach recovery and convex profiles. In summer similar profiles are maintained, but with lower wave heights, favoring more extensive beaches. Other natural factors that could contribute to erosion along the Caribbean coast of Costa Rica encompass sea-level rise, alterations in land use, rip currents, and local geodynamic effects resulting from earthquakes (Figure 8) (Barrantes-Castillo et al., 2021; Barrantes-Castillo and Ortega-Otárola, 2023).
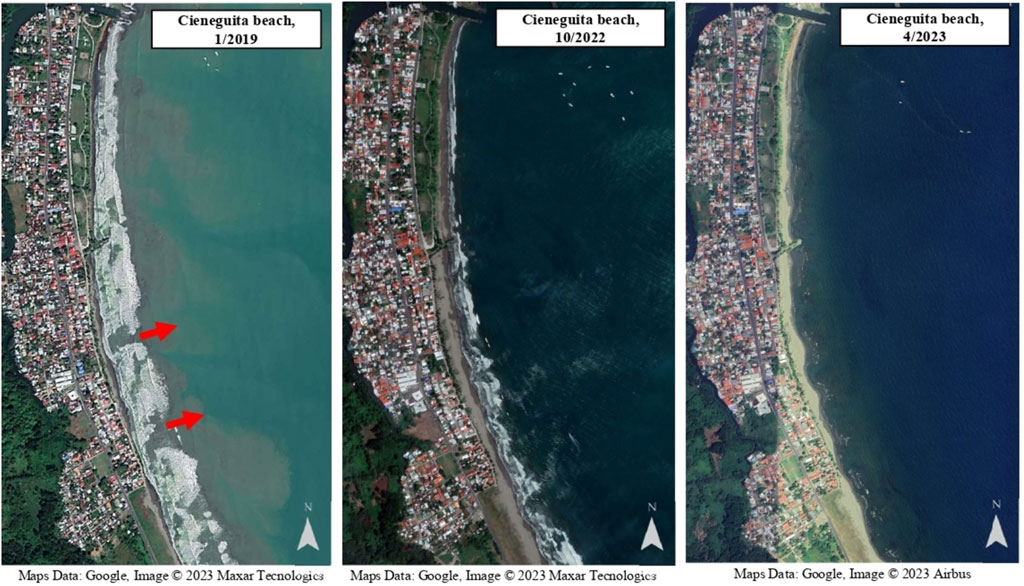
FIGURE 8. Seasonal variations in Cieneguita Beach, Costa Rica. Image 1/2019 depicts the formation of rip currents (represented by red arrows), image 10/2022 shows the beach condition during the winter season and image 4/2023 shows the beach condition during the summer season.
5.5 Chile
It has been identified that the beaches in the southwest of the Arauco Gulf experience erosion due to high-energy waves. However, it has been observed that Santa María Island acts as a protective barrier in certain areas by dissipating wave energy through wave diffraction (Villagrán et al., 2023). In addition to hydrodynamic processes, tectonic movements associated with earthquakes also contribute to changes in coastal morphology along the Chilean coasts. The movements and more frequent storms have made the situation worse and made it harder for affected beaches to recover (Martínez et al., 2018; Martínez et al., 2022; Villagrán et al., 2023). Events such as earthquakes and tsunamis, like the one that occurred in 2010, have had a significant impact on the beaches, causing sediment scarcity, destruction of coastal dunes, and erosion. In Pichilemu Beach, natural regeneration was not evident until 2014 due to limitations in sediment supply (Figure 9) (Soto et al., 2015). Additionally, an earthquake in 2010 caused a 1.4-m uplift in the Tubul Beach area in the Arauco Gulf, altering the dynamic conditions of the system and making it an area of interest for studying the effects of tectonic movements and coastal evolution (Villagrán et al., 2023).
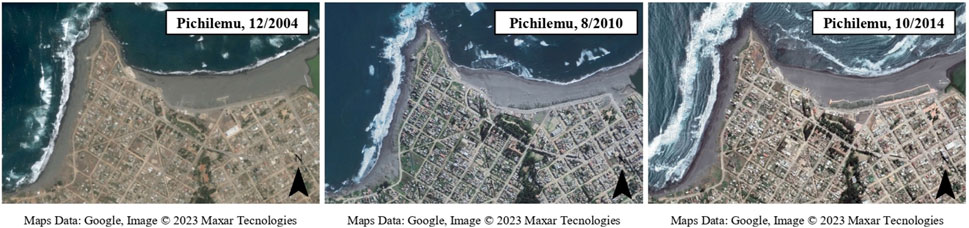
FIGURE 9. Variations in the coastal area of Pichilemu, Chile. Image 12/2004: beach condition before the 2010 earthquake and tsunami. Image 8/2010: beach condition after the 2010 tsunami. Image 10/2014: subsequent beach recovery with changes in the coastline.
5.6 Mexico
The Caribbean coast of Yucatan Peninsula is a coastal region that is continuously impacted due to hydrodynamic processes and extreme events that cause erosion. Anticyclonic systems known as “Nortes” generate cold fronts and intense wind waves in autumn and winter. Tropical cyclones, such as depressions and hurricanes, form in summer and autumn, generating powerful wind waves in the region. “Suradas” are storms that produce waves from the southeast in spring. These challenging conditions affect the coast of the Yucatan Peninsula (Escudero-Castillo et al., 2018). In the Quintana Roo coast, erosion has increased due to strong storms and hurricanes, possibly intensified by global warming (Lopez, 2014). The northern coast is characterized by low-energy waves, while the eastern coast experiences higher energy due to the Caribbean Sea swells. Sediment transport is directed from east to west influenced by waves and the orientation of the coastline (Appendini et al., 2012). In Cancun, storms generate fast wave currents that intensify erosion. Additionally, divergent sediment transport is an important phenomenon in the area, especially in areas such as Punta Nizuc and near Punta Cancun when waves come from the southeast (Escudero-Castillo et al., 2018). The lack of sediment supplies due to the flat coastal zone without surface rivers and the construction of tourist infrastructure on dunes worsen erosion, affecting coastal structures. Human intervention disrupts sediment transport and supply, generating critical erosion problems in urbanized areas (Appendini et al., 2012; Silva-Casarin et al., 2012). The characteristic white sand of the Mexican Caribbean beaches is biogenically sourced and moves along the beach through waves and currents, with a dominant south to north movement. However, during strong storms, the sand is dragged towards the sea and deposited on the seafloor, making its natural return to the coast difficult (due to differences in bathymetry affecting wave currents distribution and magnitude) (Appendini et al., 2012; Lopez, 2014; Escudero-Castillo et al., 2018).
In Chelem, Quintana Roo, and Isla del Carmen, Campeche, coastal defense structures and factors such as urban growth and hydrological changes have influenced coastal evolution. The Progreso pier in Chelem acts as a protective barrier but generates uneven sediment transport gradients and contributes to coastal erosion. When waves come from the northwest, the pier does not dissipate the waves, allowing beach erosion due to sediment transport perpendicular to the coast (Lira-Pantoja et al., 2012). In Isla del Carmen, poorly designed breakwaters and groins block sediment transport to the beach, causing widespread coastal retreat, such as in the Sabancuy estuary. Emergency measures implemented have not solved the problem and have increased currents and wave energy in some areas (Escudero et al., 2014).
The location of the Yucatan Peninsula is vulnerable to hurricanes as they typically pass through the Caribbean Sea and Gulf of Mexico. Numerous hurricanes were recorded in the Cancun region between 1948 and 2007, some reaching significant wave heights exceeding 10 m, with four exceeding 12 m in height (Silva-Casarin et al., 2012). Hurricanes like Gilbert and Wilma caused severe damage and erosion in the beaches of Cancun, exacerbated by high anthropogenic intervention in these areas (Escudero-Castillo et al., 2018). Hurricane Wilma in 2005 caused severe impacts in the region, including the case of the Capitan Laffite Hotel, which lost 20 m of beach, submerging its foundations in the sea. Subsequently, the land was acquired to build the Grand Velas Riviera Maya Hotel, investing in beach recovery and stabilization measures (Lopez, 2014). Hurricane Wilma generated significant wave heights and storm surges (Figure 10). However, coral reefs dissipated more than 80% of the wave energy (Mulcahy et al., 2016). In addition to hurricanes Gilbert and Wilma, Hurricane Allen in 1980 recorded an average wave height of approximately 8.60 m, while Hurricane Roxanne in 1995 generated waves around 10.50 m high. Hurricane Emily in 2005 had wave heights of approximately 12.30 m. These events have demonstrated the significant impact of hurricanes on the coastal conditions of Cancun, as its location northwest of the Yucatan Peninsula makes it an especially energetic area in the Gulf of Mexico and the Caribbean Sea (Silva-Casarin et al., 2012).
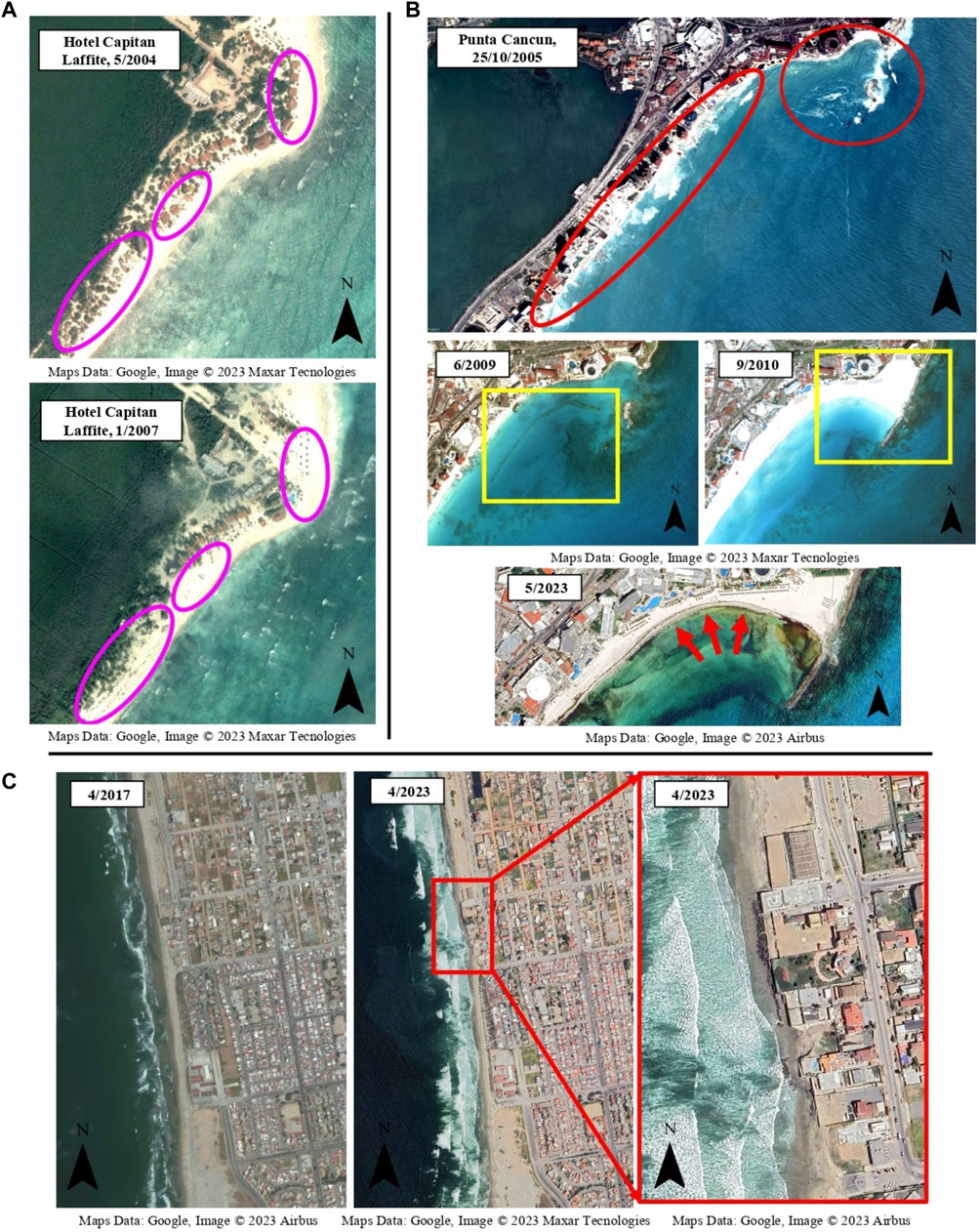
FIGURE 10. (A) Case of Capitan Laffite Hotel in Cancun, which suffered severe damage and infrastructure loss due to the passage of Hurricane Wilma in 2005. (B) Google Earth Pro images captured during the passage of Hurricane Wilma in Punta Cancun, showing its impact on the coastal environment (highlighted in red), and the subsequent remediation measures taken in the area, including the installation of geotextile tubes for wave dissipation, construction of a new breakwater, and artificial beach nourishment (highlighted in yellow). However, it is evident from the image of 5/2023 that the area is currently experiencing shoreline retreat (indicated by red arrows). (C) The “coastal squeeze” can be observed on Bahía Todos Santos beach.
Anthropogenic factors have also contributed to the vulnerability of the coastal area of Cancun, in addition to the effects of hurricanes. Inadequate construction of coastal structures, uncontrolled urban growth, and alteration of sediment flows have weakened beach stability and increased susceptibility to erosion. A notable example is Hurricane Dean in 2007, which impacted an already altered and adapting coastal system. Despite expectations of minor damage, the fragility of the coastal system resulted in significant sand removal. The waves generated by the hurricane caused changes in beach shape, retreat in the coastline, and the formation of submerged bars in the northern part of Cancun (Martell-Dubois et al., 2012; Mulcahy et al., 2016). However, hurricanes will remain one of the main drivers of short-term coastal erosion in the Cancun area, and climate change could increase the intensity of extreme events, leading to a more significant impact on the evolution of Cancun’s beaches. The frequency of wave-induced flooding could increase, and storm recurrence intervals could shorten, making it challenging for beaches to fully recover between successive events. Additionally, although not included in the systematic review of case studies, the study conducted by (Guido-Aldana et al., 2009) is recommended due to its extensive information on the impacts of Hurricane Wilma and Hurricane Dean on the coastal zone of Cancun and the subsequent mitigation measures taken in the area following these events.
In Bahía de Todos Santos (Figure 10), on the Baja California Peninsula on the Pacific coast of Mexico, there is evidence of coastal erosion derived from various factors such as coastal geology, wave climate, sea level, sediment supply, and human intervention. During the period from 1978 to 1983, strong winter storms caused intense erosion in the bay. Extratropical storms generated higher and more erosive waves, affecting Playitas Beach and destroying homes in the coastal area. In January 1987, a severe storm event led to a rise in sea level along the coast. However, the changes in the beach during this event in Bahía de Todos Santos were not documented. Additionally, the area is crossed by geological faults that can cause significant movements in the coastline (Lizarraga-Arciniega and Fischer, 1998). In the upper part of the Gulf of California, on the Baja California Peninsula, climate change and sea-level rise are causing severe damage to cultural heritage sites. The archaeological site “Conchero 4” was devastated in 2018 due to a combination of tides and storm surges generated by storms. Hurricane Rosa also impacted the area, causing significant damage to coastal developments and archaeological sites along the coast (Vellanoweth et al., 2020).
The research by (Valderrama-Landeros et al., 2019) is recommended for addressing both environmental and morphological characteristics, as well as coastal dynamics and erosion and accretion processes along the coasts of Mexico. This study presents evidence of various erosion and accretion processes along the Pacific and Atlantic coasts of Mexico, with a particular focus on vegetation-deprived coastal areas, especially beaches. The Mexican states most affected by coastal erosion, ranked by the average erosion length in meters during the period from 1970/81 to 2015, are Campeche (−683.0 m), Sinaloa (−214.0 m), Jalisco (−130.40 m), Colima (−112.50 m), and Guerrero (−110.70 m). Although this study does not provide specific causes for these changes, it offers insights into potential “hot spots” along the Mexican coasts. Other research, such as that conducted by (Escudero et al., 2014), may help link coastal erosion to specific causes. For instance, in Isla del Carmen (Campeche state), poorly designed defense structures like breakwaters and groins have disrupted sediment transport to the beach. Additionally, urban expansion in Ciudad del Carmen, construction of buildings on the beachfront and hydrological changes have exacerbated the problem. Additionally, the study conducted by (Godwyn-Paulson et al., 2021), which focuses on the analysis of the coast of the state of Oaxaca, provides various conclusions regarding potential causes of erosive processes, including cases associated with “El Niño” events. These events promote the occurrence of more frequent storm wave conditions and create a unimodal wave climate that tends to predominantly move perpendicularly to the coast, resulting in reduced energy dissipation.
5.7 Panama
In the study Pacific Coast of Panama, the cause of coastal deterioration is not clear, but there are several factors contributing to erosion. These factors can be of human origin, such as coastal construction and sand extraction, or natural origin, such as tides and waves. The negative impact of human intervention is observed in the beaches of Malibu, Gorgona, Serena, Coronado, and Teta where high-energy waves and tidal variations erode the coastline. Cliffs in Punta Barco Viejo also show similar effects (Figure 11). Sand extraction and terrace construction have deteriorated the beach’s defense capacity against extreme events. Additionally, bathymetry causes wave convergence, similar to what has been observed on Brazilian beaches. It is important to study the effects of “El Niño” events on coastal currents and sediment transport in the Gulf of Panama because this could increase coastal erosion problems (Vallarino-Castillo et al., 2022).
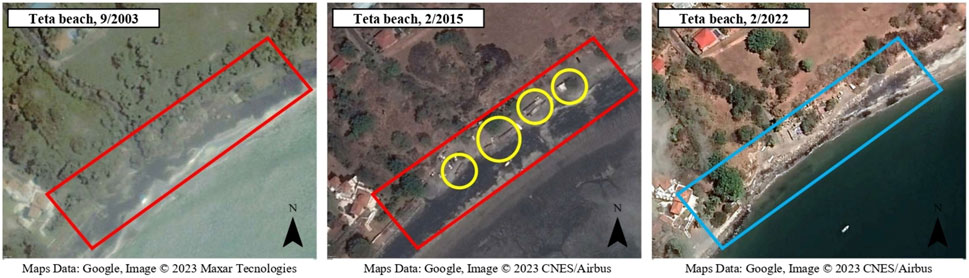
FIGURE 11. Modification of the coastal landscape and disappearance of urban infrastructure in Playa Teta, Panama. Image 9/2003: Previous state of the beach before modification of the beach area (red). Image 2/2015: Modified beach state with human settlements (yellow). Image 2/2022: Modification of the position of human settlements, installation of rocks as a coastal protection measure, and reduction of the beach strip (blue).
5.8 Uruguay
In the case of Uruguay’s coastlines, the methodology proposed by Solari et al. (2018) in their study of beach profiles along the Uruguayan coast determined that beach profiles have a retreat potential 3 to 10 times greater on the Atlantic-facing coast compared to the coast bordering the Río de la Plata estuary. This is because this area is primarily influenced by wave climatic conditions, which increase its destructive capacity during extreme wave conditions. In some areas of the Atlantic coast, such as the stretch from Barra del Chuy to Cabo Polonio, local changes in bathymetry have a significant impact on wave convergence, resulting in increased wave energy and erosion issues (Figure 12) (Speranski and Calliari, 2001). Another evidence of the influence of hydrodynamic processes on coastline evolution is observed in the case of Balneario Solís beach, as presented in (Alonso et al., 2014). Here, the intensification of beach erosion and the change in the direction of sandbar growth appear to be related to changes in local bathymetry. However, despite the magnitude of these bathymetric changes and their proximity to the coast, the lack of a detailed bathymetric map limited the available information for linking it to the evolution of the sandbar and coastline in the study area. On the other hand, climate variability, which has led to an increase in storm frequency, has caused changes in coastal dynamics (Ortega et al., 2013). Other studies examining a possible increase in the frequency of “El Niño” and “La Niña” extreme events, along with rising sea levels and a greater number of storms, could trigger more frequent events and higher erosion rates along Uruguay’s Atlantic coast in general (Orlando et al., 2019). It is important to note that adaptations have been observed on some beaches in Arachania and Barra del Chuy. In reflective beaches, there has been an increase in slope and the width of the swash zone, improving their wave-reflecting capacity. However, this also increases the erosion risk since these beaches are more sensitive to wave height and energy. In contrast, in dissipative beaches, not only has an increase in swash zone width been observed but also an overall decrease in beach slope, which enhances their ability to disperse and dissipate wave energy in these areas (Ortega et al., 2013).
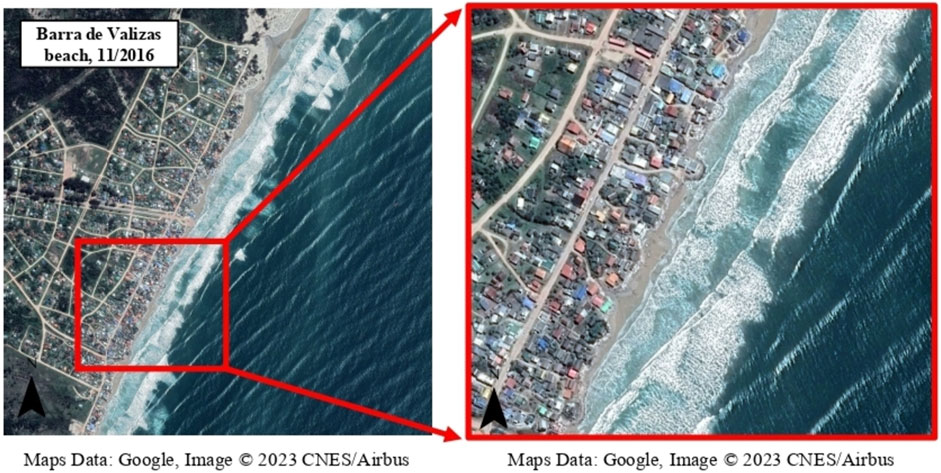
FIGURE 12. Image depicting strong waves reaching the residential urban area at Barra de Valizas Beach near to the Cabo Polonio zone in Uruguay.
5.9 Ecuador and Perú, the influence of ENSO on erosion events on coasts
The coasts of Ecuador and Peru face threats primarily exacerbated by the ENSO phenomenon, with significant impacts attributed to the climate variability triggered by “El Niño” episodes. During these events, there are notable anomalies in the frequency and intensity of precipitation, particularly in the coastal plains (Losada et al., 2013; Rollenbeck et al., 2022). Furthermore, these episodes exert a significant influence on sea levels, with fluctuations that can reach up to ±30 cm, intensifying changes in coastal morphology (Martínez et al., 2018). However, it is important to emphasize the need for local measurements, as there is evidence that the figures obtained at local stations can differ significantly from global observations, as noted in the study by Jigena-Antelo et al. (2023). This study found that IPCC estimates for the period between 2006 and 2015 indicated a sea-level rise of 3.60 mm/year. However, measurements taken at stations in Peru showed significantly higher rates of sea-level rise, ranging from 8.30 to 13.30 mm/year. Regarding impacts related to “El Niño,” in the Bay of Callao (Peru), a decrease in beach stability in the area of the Rímac river has been observed due to reduced sediment accumulation transported by marine currents in recent years (Muñoz et al., 2022). In the beaches of Libertador Bolívar and Playa Bruja (Ecuador), erosion events in this region are primarily attributed to the action of waves and currents predominantly coming from the southwest, resulting in erosion rates of approximately 0.64 m/year (Nativí-Merchán et al., 2021) (Figure 13). Furthermore, in the coastal area of Esmeraldas, there is evidence of destructive effects that can be triggered by “El Niño” events, especially during the 1997–1998 period, which included severe damage to the coastal road, the loss of structures (such as houses), and cliff retreat (Federici and Rodolfi, 2001).
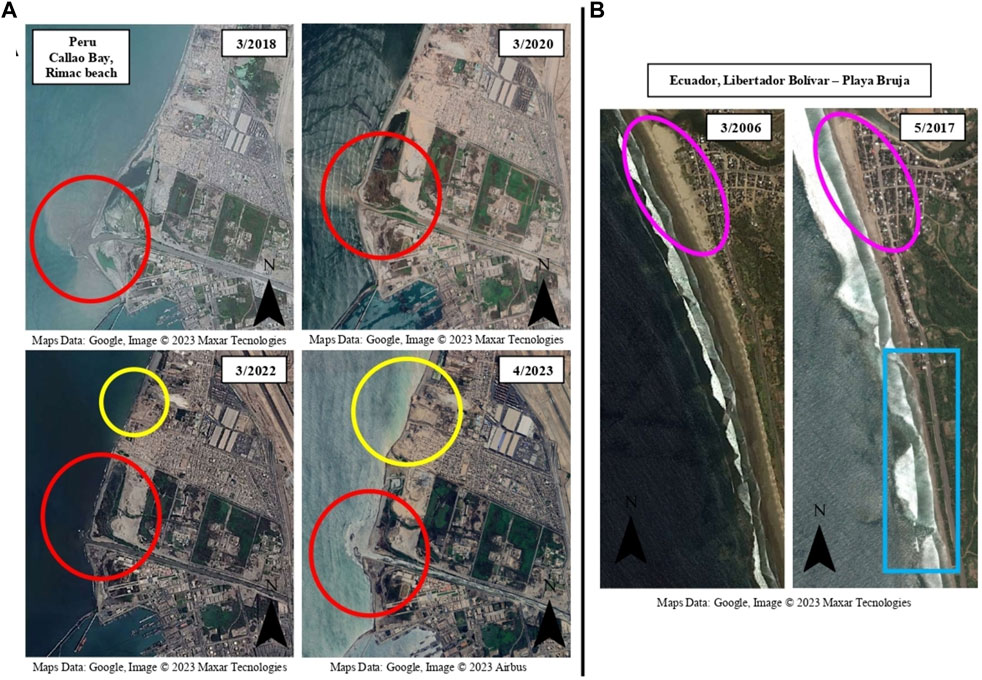
FIGURE 13. (A) The case of Playa Rimac in Peru is an example of how variations in sediment supply from the Rimac River (marked in red), combined with the influence of currents and waves, have caused alterations in the coastline (indicated in yellow). (B) In Libertador Bolivar and Playa Bruja area, a retreat of the beach has been observed (highlighted in fuchsia), which increases the risk of damage to houses and roads due to the action of strong waves (highlighted in light blue).
6 Analyses of data sources, tools and methods commonly use in studies of coastal erosion in Latin America
The research methodology used in the different case studies varies depending on the availability of information and the defined scope. Using the 62 selected case studies for this literature review, six (6) clusters have been defined that encompass different techniques, data sources, and digital tools, interdependent and employed for the study of changes in coastal areas associated with hydrodynamic processes (Figure 14). These different clusters are defined as follows.
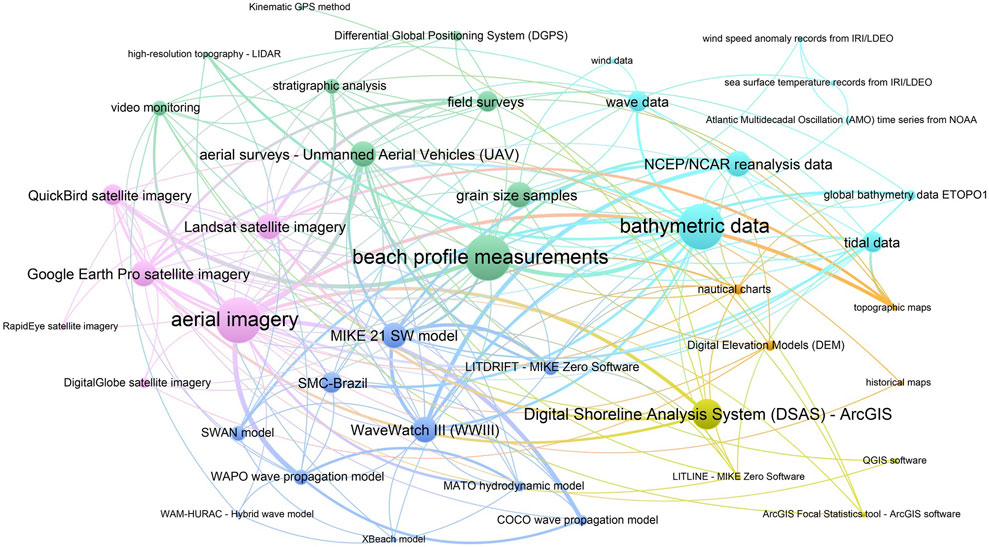
FIGURE 14. Methods, tools, and data sources identified as relevant in the 62 case studies. Different clusters are presented classified according to colors: cluster 1, cartographic data (orange); cluster 2, fieldwork (green); cluster 3, hydrodynamic process modeling (blue); cluster 4, shoreline change analysis (olive green); cluster 5, aerial and satellite images (pink); cluster 6, marine and atmospheric conditions data (turquoise).
6.1 Cluster 1: Cartographic data
Cartographic data is collected to visualize the characteristics of the coastal zone using historical maps, topographic maps, and nautical charts (Fernandino et al., 2018). Topographic maps provide information about the shape, orientation, and elevation of the coast. Additionally, gathering historical records of topographic maps provides necessary data to understand the evolution of the coastline over time. It also helps to identify elements near the coastal environment such as rivers, lakes, mountains, and volcanoes (Barrantes-Castillo et al., 2021; Vallarino-Castillo et al., 2022). Furthermore, nautical charts are used to create wave concentration maps at specific points in the area and complement the information on deep-water bathymetry (Addad and Martins-Neto, 2000; Speranski and Calliari, 2001; Calliari et al., 2003; Dillenburg et al., 2004; Pousa et al., 2007; Merlotto et al., 2014; Oliveira et al., 2020).
6.2 Cluster 2: Fieldwork
Focused on the activities in the study area that involve the collection of data in the field, such as measurements of beach profiles, collection of grain size samples and stratigraphic analysis (Pousa et al., 2007; Ortega et al., 2013; Medina et al., 2016). It also includes the generation of information through the use of unmanned aerial vehicles (UAVs) to obtain high-resolution images and videos of the beach conditions (Parise et al., 2009). These tools are used in conjunction with global positioning systems (DGPS and GPS) to obtain the precise position of the beach line, which can subsequently be compared with historical cartographic data (Dillenburg et al., 2004; Esteves et al., 2006; Fernandez et al., 2011; Coca-Domínguez and Ricaurte-Villota, 2019; Martínez et al., 2022). They also include the use of Lidar to obtain high-resolution data of the topography of the area and field surveys to know the perception of the population and data related to impacts on infrastructures (Cueto et al., 2022; Simões et al., 2022; Vallarino-Castillo et al., 2022). However, the widely used technique recognized in most case studies has been the measurement of beach profiles, as it allows obtaining information at the local level.
6.3 Cluster 3: Hydrodynamic process modeling
Centered on the modeling of hydrodynamic processes in the coastal zone using specialized software. Among the tools are WaveWatch III, Mike 21 Spectral Wave, SWAN and XBeach to simulate currents, waves and coastal tides at different depths (Codignotto et al., 2011; Fernandez et al., 2011; Escudero-Castillo et al., 2018; Cueto et al., 2022). In some studies, the use of WaveWatch III has been observed, which is a global model used for deep waters and its output has been used as input to model the propagation of waves in shallow waters with MIKE 21 SW (Fernandez et al., 2011; Appendini et al., 2012; Silva et al., 2016). Another example is the SWAN model in conjunction with the XBeach model, to analyze the propagation of waves from deep waters to the coast (SWAN) and subsequently to simulate the morphological response of the coast to hydrodynamic processes (XBeach) (Cueto et al., 2022). Also, in Brazil the SMC-Brasil model is often used which provides information on the characteristics of the waves and bathymetric data. It is also used to perform analyses of the propagation of waves towards the coast (Fernandino et al., 2018; Elliff et al., 2019; Oliveira et al., 2020). WAPO, COCO and MATO models are also used to analyze the transformation of waves during their propagation. The WAPO model calculates the linear propagation of waves, and consequently the output of this model is used as input in the COCO or MATO model that solves the nonlinear equations in shallow waters (Silva-Casarin et al., 2012; Mallmann and Pereira, 2014; Martins and Pereira, 2014; Escudero-Castillo et al., 2018). For the calculation of coastal currents and sediment transport, the LITDRIFT model from MIKE Zero Software is used. However, although the models are advantageous, it is important to calibrate and compare the results of these models with measurements made in field work or with available data (Lira-Pantoja et al., 2012).
6.4 Cluster 4: Shoreline change analysis
Includes software associated with Geographic Information Systems (GIS) to evaluate erosion, sedimentation, and changes in coastal morphology. The QGIS and ArcGIS software, along with the Digital Shoreline Analysis System (DSAS) extension, are widely used tools in regional studies of shoreline change (Esteves et al., 2003; Rangel-Buitrago et al., 2020; Simões et al., 2022). These tools allow comparing data obtained from field measurements, images, and historical maps to calculate rates of erosion and/or accretion (Rangel-Buitrago et al., 2015; Coca-Domínguez and Ricaurte-Villota, 2019; Vallarino-Castillo et al., 2022). In addition, ArcGIS - Focal Statistics is used to perform neighborhood comparisons and calculate the loss at a site after an erosion event (Vellanoweth et al., 2020).
6.5 Cluster 5: Aerial and satellite images
Data obtained from aerial and satellite images is one of the most used current tools to carry out studies of variations in the shoreline. These provide detailed historical and current data on the elements of the study area, such as vegetation, urban areas, and water bodies. These images are especially useful when fieldwork is limited or when specific cartographic material is not available. In addition, they are combined with the use of software for shoreline change analysis to know the variations in the position and rates of erosion and sedimentation in a study area (Rangel-Buitrago et al., 2020; Martínez et al., 2022; Simões et al., 2022). For this task, high-precision aerial and satellite images are commonly used, such as Google Earth Pro, QuickBird and Landsat. Less frequently, the use of RapidEye images and other images offered by the DigitalGlobe company was indicated (Merlotto et al., 2014; Mulcahy et al., 2016; Escudero-Castillo et al., 2018). Additionally, high-precision images at the local level are sometimes obtained by researchers or research institutions by unmanned aerial vehicles (UAVs) (Silva-Casarin et al., 2012; Escudero et al., 2014). On the other hand, Google Earth Pro satellite images have been used to establish the location and contours of artificial reefs, in combination with specialized software for wave propagation analysis (Fernandino et al., 2018). These data sources complement each other to obtain better results at a large scale, an example is the limitation of images available in Google Earth Pro for the case of avulsion in La Barra in Buenaventura, Colombia, which could be supplemented with Landsat images to show the coastline change produced in this area (Pousa et al., 2007; Rangel-Buitrago et al., 2015; Coca-Domínguez and Ricaurte-Villota, 2019; Vallarino-Castillo et al., 2022).
6.6 Cluster 6: Marine and atmospheric conditions data
The data included in this section includes global reanalysis information from NCEP/NCAR, used to study wave and tide conditions, coastal currents, and climatic data. These data can be used in conjunction with software such as SWAN for local coastal studies (Codignotto et al., 2011). Additionally, they have been used in studies on meteorological tsunamis, extratropical cyclones, and climatic anomalies (Parise et al., 2009; Dragani et al., 2014). Another example is the Atlantic Multidecadal Oscillation (AMO) time series, which is used to represent large-scale processes. These are combined with sea surface temperature anomalies (SSTA) and wind speed anomalies (WSA) data obtained from the IRI/LDEO Climate Data Library to analyze climatic anomalies that influence the study area in countries like Uruguay (Ortega et al., 2013). Furthermore, in Brazil, SMC-Brasil is used to obtain global reanalysis information on sea states (Fernandino et al., 2018). Specialized institutions and fieldwork are also relied upon to gather local data on marine and bathymetric conditions, which have been collected through tide gauge stations, echo sounders, and/or buoys (Addad and Martins-Neto, 2000; Pousa et al., 2007; Pousa et al., 2013; Oliveira et al., 2020; Cueto et al., 2022). Bathymetric data is combined with nautical charts and Google Earth Pro to add information about artificial reefs. Bathymetry data can also be obtained from global databases like ETOPO1 (https://www.ncei.noaa.gov/products/etopo-global-relief-model) (Amante and Eakins, 2009) or through the digitization of nautical charts (Appendini et al., 2012; Silva et al., 2016; Elliff et al., 2019).
7 Discussion
Coastal erosion in different regions of Mexico, Central America, and South America is closely related to hydrodynamic processes that may be directly or indirectly associated with other processes such as those identified in Figure 3. Therefore, it is necessary to consider the possibility of interaction between different driving mechanisms, since these can vary from extreme climatic events such as storms and hurricanes, to small-scale changes in bathymetry such as the case of Brazil. These factors can have synergistic effects and exacerbate the problem of coastal erosion, increasing the vulnerability of populations in these areas to events such as coastal flooding. In addition, the acceleration of coastal erosion processes has been influenced not only by natural factors, but also by the influence of human intervention. Because of uncontrolled urban growth, the lack of adequate planning in construction near the coast and activities such as sand extraction have contributed to this problem. The degradation of coastal ecosystems can generate a significant negative impact on the economy of the local population, especially in activities related to tourism and fishing. Therefore, understanding of coastal erosion processes and the identification of the main drivers are essential for the development of effective adaptation and mitigation strategies. It is for this reason the need to increase the number of investigations in this field of study, to generate data through continuous monitoring in the evaluation of the state of coastal areas and predict possible future scenarios. Collaboration between different stakeholders such as scientists, government authorities, local communities and non-governmental organizations is essential to address these challenges.
7.1 Scientific production and international cooperation
The scientific production selected for this literature review focuses on the study of coastal erosion associated with hydrodynamic processes in the coastal zone. The results show that Brazil is the country with the highest number of research in this area, with a total of 22 selected records, followed by Mexico and Argentina. Regarding international cooperation, it has been observed that institutions from the United States and Spain have had a greater collaboration in the selected case studies, followed by institutions from the United Kingdom, New Zealand, Portugal and to a lesser extent Australia, Germany, the Netherlands and Italy. This is presented in Figure 15, which shows a map of cooperation created from (TU Delft, 2013, VOSViewer map). Cases have also been identified in which researchers from international affiliations show interest in the study of coastal erosion in the region (without the cooperation of local researchers), which represents opportunities for international cooperation for the creation of a network between different institutions. Although, while most of the selected case studies have researchers affiliated with institutions in the study country, collaborations between researchers from the region have also been observed.
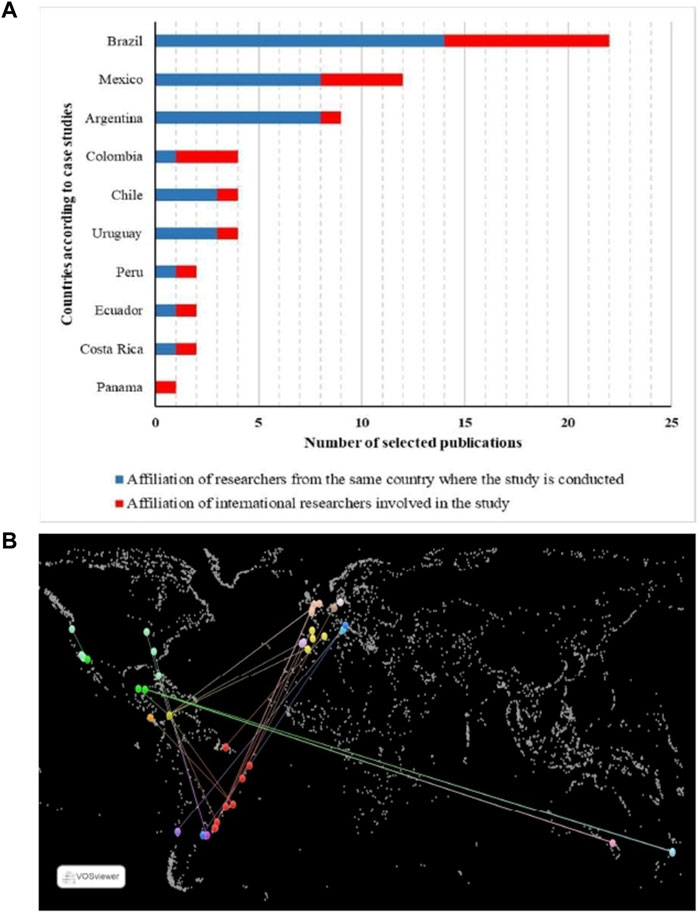
FIGURE 15. (A) Number of research studies per country and affiliations of national or international researchers, as per the case study. (B) Map of international cooperation between countries according to the selected coastal erosion cases, created based on TU Delft map VOSViewer.
International cooperation plays an important role in advancing research in coastal areas of the region. A notable example is the cooperation between Brazil and Spain, which has led to the development of the Coastal Modeling System for Brazil (SMC-Brasil) by the Institute of Environmental Hydraulics of the University of Cantabria (IH-Cantabria) (Quetzalcóatl et al., 2019). This software allows modeling time series of waves at any point on the coast and calculating the littoral drift, flood levels and the direction of the mean wave energy flow.
Therefore, it would be interesting to analyze in more depth local and international cooperation to identify the potential opportunities for the study of coastal erosion between Latin America and other countries worldwide.
7.2 Potential future research in Latin America
Coastal regions of Mexico, Central America, and South America, where coastal erosion occurs have been identified, both directly and indirectly related to hidrodynamics. However, there is still a need to better understand how these events, such as cyclonic surges and intense waves during storms, occur and their impact (Dillenburg et al., 2004). This is particularly important because over 3% of the population in these regions lives within a 5.00 km coastal zone (Barragán and de Andrés, 2015; CEPAL, 2015d). It is also necessary to investigate in detail the relationship between sediment transport by coastal currents and the effects of natural obstacles, such as river mouths, as well as in areas heavily impacted by human activities (Appendini et al., 2012).
In the current context of global climate change, it is crucial to identify variations in wave energy and direction at both regional and local scales (Fernandino et al., 2018; Bacino et al., 2019). This will provide a clearer understanding of the future impacts on sandy beaches, which are particularly vulnerable to wind-generated waves and currents caused by wave breaking in shallow waters. This has been observed in cases in Brazil, Argentina, Mexico and Chile. Additionally, we need to delve into how small-scale changes in bathymetry (bathymetric lenses), coral reefs, islands, and coastal orientation influence scenarios with variations in climate, tides, and currents. It is worth noting that there is evidence that seafloor morphology and coral reefs can intensify the effects of coastal erosion in certain cases by transforming incident waves (Esteves et al., 2006; Fernandino et al., 2018).
Coastal erosion in some regions has been exacerbated by climatic factors, as seen in Argentina and Uruguay. Variability in the North Atlantic and changes in temperature patterns in the Southwest Atlantic have resulted in coastal dynamics variations in both countries. This raises uncertainties about the future impact of increased storm intensity (Ortega et al., 2013). On the other hand, in the Pacific region, although there is evidence that El Niño-Southern Oscillation (ENSO) can intensify the effects of coastal erosion due to its influence on storm surges and precipitation patterns that, in turn, affect river discharge and sediment transport to the coast, a clear relationship between this phenomenon and changes in coastal areas has not yet been established (Martínez et al., 2022).
A common limitation identified in various selected studies is the lack of local information available to carry out studies on coastal dynamics. This has led to many institutions and researchers conducting field studies to collect data and then conducting research on shoreline changes. This approach can significantly delay scientific production in the study of coastal areas in the countries of the region. In some cases, researchers opt to use global databases and specialized coastal climate modeling software to generate data and obtain results. This requires prior knowledge in the use of these programs and in the calibration and validation of the models. Often, the collaboration of multidisciplinary professionals with experience in areas of knowledge such as global atmospheric patterns, oceanography, coastal engineering, and geology, is required to obtain results that are closer to reality.
Additionally, human intervention in coastal areas experiencing erosion complicates the analysis of natural-driven shoreline changes. Therefore, it is necessary to identify areas that have not been significantly altered to evaluate the effects of climate change on coastal dynamics and to make meaningful comparisons that help us understand the impact of coastal erosion. In Argentina, the Samborombón Bay has been identified as a site of interest to study changes in the coast related to hydrodynamic processes (including weather events) (Codignotto et al., 2011).
It is crucial to investigate the perception of the population in urbanized areas exposed to erosion processes associated with extreme events. An example is the research conducted by Esteves et al. (2003) that indicate in Hermenegildo, Rio Grande do Sul (an identified erosion “hot spot”), despite the damages suffered and constant investments in protective structures for residential properties, residents show resistance in acknowledging the problems associated with living in that area. Additionally, changes in coastal dynamics also affect coastal biodiversity, recreational values of beaches, and cultural heritage sites. Therefore, multidisciplinary research is needed to develop conservation strategies for these natural ecosystems, as well as regulations and strategies guiding future urban development in erosion-prone coastal areas (Silva-Casarin et al., 2012; Merlotto et al., 2014; Barragán-Muñoz, 2020; Vellanoweth et al., 2020; Winckler et al., 2023).
Data availability statement
The original contributions presented in the study are included in the article/supplementary material, further inquiries can be directed to the corresponding author.
Author contributions
RV: Conceptualization, Formal Analysis, Investigation, Methodology, Writing–original draft, Writing–review and editing. VN: Conceptualization, Funding acquisition, Supervision, Writing–review and editing. JC: Supervision, Writing–review and editing, Conceptualization, Funding acquisition.
Funding
The authors declare financial support was received for the research, authorship, and/or publication of this article. This work was supported by the Fundación Agustín de Betancourt established at the Escuela Técnica Superior de Ingenieros de Caminos, Canales y Puertos of the Universidad Politécnica de Madrid. Author RV has received research support from the National Secretary of Science, Technology, and Innovation (SENACYT) of Panama.
Acknowledgments
The authors wish to express their gratitude for the accessibility of historical images generously provided by Google Earth and its collaborators. Furthermore, we wish to extend our gratitude to the National Oceanic and Atmospheric Administration (NOAA) for the provision of tide prediction data derived from historical records (NOAA, Historic Tide and Tidal Current Tables).
Conflict of interest
The authors declare that the research was conducted in the absence of any commercial or financial relationships that could be construed as a potential conflict of interest.
Publisher’s note
All claims expressed in this article are solely those of the authors and do not necessarily represent those of their affiliated organizations, or those of the publisher, the editors and the reviewers. Any product that may be evaluated in this article, or claim that may be made by its manufacturer, is not guaranteed or endorsed by the publisher.
References
Addad, J., and Martins-Neto, M. A. (2000). Deforestation and coastal erosion: a case from east Brazil. J. Coast Res. 16, 423–431.
Allan, J. C., and Komar, P. D. (2002). Extreme storms on the pacific northwest coast during the 1997-98 El Niño and 1998-99 La Niña. J. Coast Res. 18, 175–193.
Alonso, R., López, G., Mosquera, R., Solari, S., and Teixeira, L. (2014). Coastal erosion in Balneario Solís, Uruguay. J. Coast Res. 71, 48–54. doi:10.2112/SI71-006.1
Alves, A. R. (2009). Long-term erosional hot spots in the southern Brazilian coast. J. Geophys Res. Oceans 114, C02020. doi:10.1029/2008JC004933
Amante, C., and Eakins, B. W. (2009). ETOPO1 1 arc-minute global relief model: procedures, data sources and analysis, NOAA technical memorandum NESDIS NGDC. Available at: https://repository.library.noaa.gov/view/noaa/1163 (Accessed August 24, 2023).
Appendini, C. M., Salles, P., Mendoza, E. T., López, J., and Torres-Freyermuth, A. (2012). Longshore sediment transport on the northern coast of the yucatan peninsula. J. Coast Res. 28, 1404–1417. doi:10.2112/JCOASTRES-D-11-00162.1
Ayyam, V., Palanivel, S., and Chandrakasan, S. (2019). Coastal ecosystems of the tropics-adaptive management. Berlin, Germany: Springer.
Bacino, G. L., Dragani, W. C., and Codignotto, J. O. (2019). Changes in wave climate and its impact on the coastal erosion in Samborombón Bay, Río de la Plata estuary, Argentina. Estuar. Coast Shelf Sci. 219, 71–80. doi:10.1016/j.ecss.2019.01.011
Barletta, R., and Calliari, L. J. (2003). An assessment of the atmospheric and wave aspects determining beach morphodynamic characteristics along the central coast of RS state, southern Brazil. J. Coast Res. 3, 300–308.
Barnard, P. L., Hoover, D., Hubbard, D. M., Snyder, A., Ludka, B. C., Allan, J., et al. (2017). Extreme oceanographic forcing and coastal response due to the 2015-2016 El Niño. Nat. Commun. 8, 14365. doi:10.1038/ncomms14365
Barnard, P. L., Short, A. D., Harley, M. D., Splinter, K. D., Vitousek, S., Turner, I. L., et al. (2015). Coastal vulnerability across the pacific dominated by El niño/southern oscillation. Nat. Geosci. 8, 801–807. doi:10.1038/ngeo2539
Barragán, J. M., and de Andrés, M. (2015). Analysis and trends of the world’s coastal cities and agglomerations. Ocean. Coast Manag. 114, 11–20. doi:10.1016/j.ocecoaman.2015.06.004
Barragán-Muñoz, J. M. (2020). Progress of coastal management in Latin America and the Caribbean. Ocean. Coast Manag. 184, 105009. doi:10.1016/j.ocecoaman.2019.105009
Barrantes-Castillo, G., and Ortega-Otárola, K. (2023). Coastal erosion and accretion on the Caribbean coastline of Costa Rica long-term observations. J. South Am. Earth Sci. 127, 104371. doi:10.1016/j.jsames.2023.104371
Barrantes-Castillo, G., Valverde-Calderón, J., Rojas-Jiménez, D., Badilla-Ramos, N., Panigua-Jiménez, D., and Carvalho-da Silva, A. L. (2021). Seasonal changes in the beach profile of Cieneguita, Limón, Costa Rica. Rev. Geogr. Chileerra Aust. 57, 12–28. doi:10.23854/07199562.2021571esp.Barrantes12
Bergoeing, J.-P. (2015). Geomorphology of Central America: a syngenetic perspective. Netherlands: Elsevier.
Blanco-Chao, R., Pedoja, K., Witt, C., Martinod, J., Husson, L., Regard, V., et al. (2014). Chapter 10 the rock coast of South and Central America. Geol. Soc. Mem. 40, 155–191. doi:10.1144/M40.10
Cai, W., Santoso, A., Collins, M., Dewitte, B., Karamperidou, C., Kug, J. S., et al. (2021). Changing El niño–southern oscillation in a warming climate. Nat. Rev. Earth Environ. 2, 628–644. doi:10.1038/s43017-021-00199-z
Calil, J., Reguero, B. G., Zamora, A. R., Losada, I. J., and Méndez, F. J. (2017). Comparative coastal risk index (CCRI): A multidisciplinary risk index for Latin America and the caribbean. PLoS One 12, e0187011. doi:10.1371/journal.pone.0187011
Calliari, L., Boukareva, I., Pimenta, F., and Speranski, N. (2003). Classification of the southern Brazilian coast according to storm wave patterns and geomorphologic evidence of coastal erosion. J. Coast Res. 3, 339–342.
Camus, P., Losada, I. J., Izaguirre, C., Espejo, A., Menéndez, M., and Pérez, J. (2017). Statistical wave climate projections for coastal impact assessments. Earths Future 5, 918–933. doi:10.1002/2017EF000609
CEPAL (2015a). Efectos del cambio climático en la costa de América Latina y el Caribe - dinámicas, tendencias y variabilidad climática. Available at: www.cepal.org/dmaah.
CEPAL (2015b). Efectos del cambio climático en la costa de América Latina y el Caribe - impactos. Available at: www.cepal.org/ddsah.
CEPAL (2015c). Efectos del cambio climático en la costa de América Latina y el Caribe - riesgos. Available at: www.cepal.org/ddsah.
CEPAL (2015d). Efectos del cambio climático en la costa de América Latina y el Caribe - vulnerabilidad y Exposición. Available at: www.cepal.org/ddsah.
CHW Platform (2023). Coastal hazard wheel app. Available at: https://chw-app.coastalhazardwheel.org/ (Accessed May 25, 2023).
Coca-Domínguez, O., and Ricaurte-Villota, C. (2019). Análisis de la evolución litoral y respuesta de las comunidades afro-descendientes asentadas en la zona costera: caso de estudio La Barra, Buenaventura, pacífico colombiano. Entorno Geográfico 19, 7–26. doi:10.25100/eg.v0i17.7918
Codignotto, J., Dragani, W., Martín, P., Campos, M., Alonso, G., Simionato, C., et al. (2011). Erosion at the Samborombón Bay and changes in wind direction, province of Buenos Aires, Argentina. Rev. del Mus. Argent. Ciencias Nat. 13. doi:10.22179/revmacn.13.217
Codignotto, J. (1997). “Geomorfología y dinámica costera,” in El mar agentino y sus recursos pesqueros (Argentina: Instituto Nacional de Investigación y Desarrollo Pesquero), 89–105.
Correa, I. D., and Vernette, G. (2004). Introducción al problema de la erosión litoral en Urabá (sector Arboletes-Tubo) Costa Caribe Colombiana. Bol. Investig. Mar. Costeras - INVEMAR 33, 5–26. doi:10.25268/bimc.invemar.2004.33.0.245
Correa, I. (1990). “Inventario de erosión y acreción litoral (1793-1990) entre Los Morros y Galerazamba. Departamento de Bolívar, Colombia,” in Seminario andino de Geología ambiental (Armenia, Colombia: Conferencia Colombiana de Geología Ambiental), 129–142.
Cueto, J. E., Otero-Díaz, L. J., Ospino-Ortiz, S. R., and Torres-Freyermuth, A. (2022). The role of morphodynamics in predicting coastal flooding from storms on a dissipative beach with sea level rise conditions. Nat. Hazards Earth Syst. Sci. 22, 713–728. doi:10.5194/nhess-22-713-2022
Diez, P. G., Perillo, G. M. E., and Piccolo, M. C. (2007). Vulnerability to sea-level rise on the coast of the Buenos Aires Province. J. Coast Res. 23, 119–126. doi:10.2112/04-0205.1
Dillenburg, S. R., Esteves, L. S., and Tomazelli, L. J. (2004). A critical evaluation of coastal erosion in Rio Grande do Sul, Southern Brazil. Acad Bras Cienc 76, 611–623. doi:10.1590/s0001-37652004000300014
Dragani, W. C., D’Onofrio, E. E., Oreiro, F., Alonso, G., Fiore, M., and Grismeyer, W. (2014). Simultaneous meteorological tsunamis and storm surges at Buenos Aires coast, southeastern South America. Nat. Hazards 74, 269–280. doi:10.1007/s11069-013-0836-2
Elliff, C. I., Silva, I. R., Cánovas, V., and González, M. (2019). Wave attenuation and shoreline protection by a fringing reef system. Anu. do Inst. Geociencias 42, 87–94. doi:10.11137/2019_1_87_94
Escudero, M., Silva, R., and Mendoza, E. (2014). Beach erosion driven by natural and human activity at Isla del Carmen Barrier Island, Mexico. J. Coast Res. 71, 62–74. doi:10.2112/SI71-008.1
Escudero-Castillo, M., Felix-Delgado, A., Silva, R., Mariño-Tapia, I., and Mendoza, E. (2018). Beach erosion and loss of protection environmental services in Cancun, Mexico. Ocean. Coast Manag. 156, 183–197. doi:10.1016/j.ocecoaman.2017.06.015
Esteves, L. S., Rocha De Oliveira, U., Puccinelli Da Silva, A. R., Paquete Vranja, M., Alejandra Gomez Pivel, M., Vanz, A., et al. (2003). Seasonal changes in beach profile inducing the response of beachfront owners in southern Brazil. J. Coast Res. 3, 557–563.
Esteves, L. S., Teixeira, P., and Williams, J. J. (2008). Managing coastal erosion: from long-term coastal evolution to seasonal shoreline changes. in Sediment Dynamics in Changing Environments Procededings of a symposium held in Christchurch. New Zealand, December 2008, IAHS.
Esteves, L. S., Toldo, E. E., Dillenburg, S. R., and Tomazelli, L. J. (2002). Long-and short-term coastal erosion in southern Brazil. J. Coast. Res. 36, 273–282. doi:10.2112/1551-5036-36.sp1.273
Esteves, L. S., Williams, J. J., and Dillenburg, S. R. (2006). Seasonal and interannual influences on the patterns of shoreline changes in Rio Grande do Sul, southern Brazil. J. Coast Res. 22, 1076–1093. doi:10.2112/04-0227R.1
Federici, P. R., and Rodolfi, G. (2001). Rapid shoreline retreat along the Esmeraldas coast, Ecuador: natural and man-induced processes. J. Coast Conserv. 7, 163–170. doi:10.1007/bf02742478
Fernandez, G., Bulhoes, E., and da Rocha, T. (2011). Impacts of severe storm ocurred in April 2010 along Rio de Janeiro coast, Brazil. J. Coast. Res. 64.
Fernandino, G., González, M., Cánovas, V., Tanajura, C. A. S., and Silva, I. R. (2018). Erosional patterns induced by coral reefs in the eastern coast of Brazil. Pesqui. em Geociencias 45. doi:10.22456/1807-9806.91391
Garcia, N. O., Ferreira, R. N., and Latrubesse, E. M. (2009). Climate and geomorphologic-related disasters in Latin America. Dev. Earth Surf. Process. 13, 1–27. doi:10.1016/S0928-2025(08)10001-3
Godwyn-Paulson, P., Jonathan, M. P., Roy, P. D., Rodríguez-Espinosa, P. F., Muthusankar, G., Muñoz-Sevilla, N. P., et al. (2021). Evolution of southern Mexican pacific coastline: responses to meteo-oceanographic and physiographic conditions. Reg. Stud. Mar. Sci. 47, 101914. doi:10.1016/j.rsma.2021.101914
Gomes, G., and Da Silva, A. C. (2014). Coastal erosion case at Candeias beach (NE-Brazil). J. Coast Res. 71, 30–40. doi:10.2112/SI71-004.1
Guido-Aldana, P., Ramírez-Camperos, A., Godínez-Orta, L., Cruz-León, S., and Juárez-León, A. (2009). Estudio de la erosión costera en Cancún y la riviera Maya, México. Av. Recur. Hidraúlicos 20, 41–56.
Hinkel, J., Nicholls, R. J., Tol, R. S. J., Wang, Z. B., Hamilton, J. M., Boot, G., et al. (2013). A global analysis of erosion of sandy beaches and seasea-level rise: an application of DIVA. Glob. Planet Change 111, 150–158. doi:10.1016/j.gloplacha.2013.09.002
Intergovernmental Panel on Climate Change (IPCC) (2022). “Sea level rise and implications for low-lying islands, coasts and communities,” in The Ocean and cryosphere in a changing climate: special report of the intergovernmental Panel on climate change (Cambridge: Cambridge University Press), 321–446. doi:10.1017/9781009157964.006
Jigena-Antelo, B., Estrada-Ludeña, C., Howden, S., Rey, W., Paz-Acosta, J., Lopez-García, P., et al. (2023). Evidence of sea level rise at the Peruvian coast (1942–2019). Sci. Total Environ. 859, 160082. doi:10.1016/j.scitotenv.2022.160082
Komar, P. D., and Enfield, D. B. (1987). “Short-term sea-level changes and coastal erosion,” in Sea-level fluctuation and coastal evolution (United States: SEPA), 17–27. doi:10.2110/pec.87.41.0017
Komar, P. D., and Holman, R. A. (1986). Coastal processes and the development of shoreline erosion. Available at: www.annualreviews.org.
Liberto, T. (2021). ENSO and climate change: what does the new IPCC report say? Available at: https://www.climate.gov/news-features/blogs/enso/enso-and-climate-change-what-does-new-ipcc-report-say (Accessed July 12, 2023).
Lipp, D. O. (2019). “The impact of the increase of the level of the sea in the Argentine coastal areas. Current evidence and future scenarios,” in Extreme weather events and human health: international case studies (Berlin, Germany: Springer International Publishing), 333–353. doi:10.1007/978-3-030-23773-8
Lira-Pantoja, A., Torres-Freyermuth, A., Appendini, C. M., Fernández, D., Salles, P., Mendoza, E. T., et al. (2012). “Chronic beach erosion induced by coastal structures in Chelem, Yucatán,” in Coastal engineering proceedings (Berlin, Germany: Springer). doi:10.9753/icce.v33.sediment.125
Lizarraga-Arciniega, R., and Fischer, D. W. (1998). Coastal erosion along the Todos Santos bay, ensenada, Baja California, Mexico: an overview. J. Coast Res. 14, 1–9.
Lobeto, H., Menendez, M., and Losada, I. J. (2021). Future behavior of wind wave extremes due to climate change. Sci. Rep. 11, 7869. doi:10.1038/s41598-021-86524-4
Lopez, R. (2014). Beach restoration at Grand Velas Hotel, riviera Maya, Mexico. J. Coast Res. 71, 86–92. doi:10.2112/SI71-010.1
Losada, I. J., Reguero, B. G., Méndez, F. J., Castanedo, S., Abascal, A. J., and Mínguez, R. (2013). Long-term changes in sea-level components in Latin America and the Caribbean. Glob. Planet Change 104, 34–50. doi:10.1016/j.gloplacha.2013.02.006
Luijendijk, A., Hagenaars, G., Ranasinghe, R., Baart, F., Donchyts, G., and Aarninkhof, S. (2018). The state of the world’s beaches. Sci. Rep. 8, 6641. doi:10.1038/s41598-018-24630-6
Mallmann, D. L. B., and Pereira, P. S. (2014). Coastal erosion at Maria Farinha beach, Pernambuco, Brazil: possible causes and alternatives for shoreline protection. J. Coast Res. 71, 24–29. doi:10.2112/SI71-003.1
Martell-Dubois, R., Mendoza-Baldwin, E., Mariño-Tapia, I., Silva-Casarín, R., and Escalante-Mancera, E. (2012). Impactos de corto plazo del huracán Dean sobre la morfología de la playa de Cancún, México. Tecnol. Ciencias del Agua 3, 89–111.
Martell-Dubois, R., Silva-Casarin, R., Mendoza-Baldwin, E. G., Muñoz-Pérez, J. J., Cerdeira-Estrada, S., Escalante-Mancera, E., et al. (2018). Spectral bimodality of waves produced by hurricanes in the Caribbean coastal zone off Mexico. Cienc. Mar. 44, 33–48. doi:10.7773/cm.v44i1.2717
Martínez, C., Contreras-López, M., Winckler, P., Hidalgo, H., Godoy, E., and Agredano, R. (2018). Coastal erosion in central Chile: A new hazard? Ocean. Coast Manag. 156, 141–155. doi:10.1016/j.ocecoaman.2017.07.011
Martínez, C., Winckler-Grez, P., Agredano-Martín, R., Esparza-Acuña, C., Torres, I., and Contreras-López, M. (2022). Coastal erosion in sandy beaches along a tectonically active coast: the Chile study case. Prog. Phys. Geogr. 46, 250–271. doi:10.1177/03091333211057194
Martins, K. A., and Pereira, P. S. (2014). Coastal erosion at Pau Amarelo beach, northeast of Brazil. J. Coast Res. 71, 17–23. doi:10.2112/SI71-002.1
McGranahan, G., Balk, D., and Anderson, B. (2007). The rising tide: assessing the risks of climate change and human settlements in low elevation coastal zones. Environ. Urban 19, 17–37. doi:10.1177/0956247807076960
Medina, R. A., Martínez, A. L., Mormeneo, L., and Richiano, S. M. (2016). Cambios morfosedimentarios causados por la construcción de un espigón en Camet Norte, Provincia de Buenos Aires, Argentina. Rev. Asoc. Geol. Argent. 73, 270–279.
Merlotto, A., Bértola, G. R., Isla, F. I., Cortizo, L. C., and Piccolo, M. C. (2014). Short and medium-term coastal evolution of Necochea Municipality, Buenos Aires province, Argentina. Environ. Earth Sci. 71, 1213–1225. doi:10.1007/s12665-013-2525-6
Moher, D., Liberati, A., Tetzlaff, J., Altman, D. G., and Group, T. P. (2009). Preferred reporting Items for systematic reviews and meta-analyses: the PRISMA statement. PLoS Med. 6, e1000097. doi:10.1371/journal.pmed.1000097
Mulcahy, N., Kennedy, D. M., and Blanchon, P. (2016). Hurricane-induced shoreline change and post-storm recovery: Northeastern Yucatan Peninsula, Mexico. J. Coast. Res. 75, 1192–1196. doi:10.2112/SI75-239.1
Muñoz, A., Mendoza, L., Guzmán, E., and Ramos, C. (2022). “Analysis of coastline evolution using Landsat and sentinel 2 images from 2001 to 2020 in Callao bay, Peru,” in International conference on geographical information systems theory, applications and management, GISTAM - proceedings (United Kingdom: Science and Technology Publications), 115–122. doi:10.5220/0011037500003185
National Aeronautics and Space Administration (NASA) (2023). Living Ocean. Available at: https://science.nasa.gov/earth-science/oceanography/living-ocean/#:∼:text=There%20are%20about%20620%2C000%20kilometers%20%28372%2C000%20miles%29%20of,devastating%20tsunami%20in%20the%20Indian%20Ocean%20in%202004 (Accessed May 25, 2023).
National Aeronautics and Space Administration (NASA) (2019). Ocean surface topography from space. Available at: https://sealevel.jpl.nasa.gov/data/vital-signs/el-nino/#:∼:text=During%20an%20El%20Ni%C3%B1o%20event%2C%20the%20trade%20winds,Pacific%20and%20colder%20water%20in%20the%20eastern%20Pacific (Accessed May 25, 2023).
National Oceanic and Atmospheric Administration (NOAA) (2020). Historic tide and tidal current tables. Available at: https://www.tidesandcurrents.noaa.gov/historic_tide_tables (Accessed August 29, 2023).
National Snow and Ice Data Center (NSIDC) (2023). Ice shelves - why ice shelves matter. National aeronautics and space administration (NASA). Available at: https://nsidc.org/learn/parts-cryosphere/ice-shelves/why-ice-shelves-matter (Accessed May 25, 2023).
Nativí-Merchán, S., Caiza-Quinga, R., Saltos-Andrade, I., Martillo-Bustamante, C., Andrade-García, G., Quiñonez, M., et al. (2021). Coastal erosion assessment using remote sensing and computational numerical model. Case of study: libertador bolivar, Ecuador. Ocean. Coast Manag. 214, 105894. doi:10.1016/j.ocecoaman.2021.105894
Nicolodi, J. L., de Figueiredo, S. A., Toldo, E., and Calliari, L. J. (2021). Regional coastline changes and future predicted scenario on southern Brazil. Rev. Geogr. Chileerra Aust. 57, 76–95. doi:10.23854/07199562.2021571esp.Nicolodi76
Oliveira, J. F., Scarelli, F. M., Portantiolo-Manzolli, R., Portz, L. C., and Barboza, E. G. (2020). Geomorphological Responses Due to Storm Wave Events at Praia da Barra, Garopaba - Santa Catarina State, Southern Brazil. J. Coast Res. 95, 474–478. doi:10.2112/SI95-092.1
Orlando, L., Ortega, L., and Defeo, O. (2019). Multi-decadal variability in sandy beach area and the role of climate forcing. Estuar. Coast Shelf Sci. 218, 197–203. doi:10.1016/j.ecss.2018.12.015
Ortega, L., Celentano, E., Finkl, C., and Defeo, O. (2013). Effects of climate variability on the morphodynamics of Uruguayan sandy beaches. J. Coast Res. 29, 747–755. doi:10.2112/JCOASTRES-D-13-00003.1
Parise, C. K., Calliari, L. J., and Krusche, N. (2009). Extreme storm surges in the south of Brazil: atmospheric conditions and shore erosion. Braz J. Oceanogr. 57, 175–188. doi:10.1590/s1679-87592009000300002
Pereira, L. C. C., Medeiros, C., Jiménez, J. A., and da Costa, R. A. M. (2006). Topographic changes in two highly sheltered beaches, Casa Caiada and Rio Doce PE (Brazil). J. Coast Res. 6, 644–647.
Pinheiro, A. B., da Silva, A. L. C., and Neto, J. A. B. (2021). Dynamics and storm records on sheltered beaches: paraty, southeast coast of Brazil. J. Sediment. Environ. 6, 647–663. doi:10.1007/s43217-021-00075-2
Pousa, J. L., D’Onofrio, E. E., Fiore, M. M. E., and Kruse, E. E. (2013). Environmental impacts and simultaneity of positive and negative storm surges on the coast of the Province of Buenos Aires, Argentina. Environ. Earth Sci. 68, 2325–2335. doi:10.1007/s12665-012-1911-9
Pousa, J., Tosi, L., Kruse, E., Guaraglia, D., Bonardi, M., Mazzoldi, A., et al. (2007). Coastal processes and environmental hazards: the Buenos Aires (Argentina) and Venetian (Italy) littorals. Environ. Geol. 51, 1307–1316. doi:10.1007/s00254-006-0424-9
Psuty, N. P., and Mizobe, C. (2014). “South America, coastal morphology,” in Beaches and coastal geology. Encyclopedia of Earth sciences series (Berlin, Germany: Springer-Verlag), 765–770. doi:10.1007/0-387-30843-1_429
Quesada-Román, A., Torres-Bernhard, L., Ruiz-Álvarez, M. A., Rodríguez-Maradiaga, M., Velázquez-Espinoza, G., Espinosa-Vega, C., et al. (2022). Geodiversity, geoconservation, and geotourism in Central America. Land (Basel) 11, 48. doi:10.3390/land11010048
Quetzalcóatl, O., González, M., Cánovas, V., Medina, R., Espejo, A., Klein, A., et al. (2019). SMCε, a coastal modeling system for assessing beach processes and coastal interventions: application to the Brazilian coast. Environ. Model. Softw. 116, 131–152. doi:10.1016/j.envsoft.2019.03.001
Rangel-Buitrago, N. G., Anfuso, G., and Williams, A. T. (2015). Coastal erosion along the caribbean coast of Colombia: magnitudes, causes and management. Ocean. Coast Manag. 114, 129–144. doi:10.1016/j.ocecoaman.2015.06.024
Rangel-Buitrago, N., Neal, W. J., and de Jonge, V. N. (2020). Risk assessment as tool for coastal erosion management. Ocean. Coast Manag. 186, 105099. doi:10.1016/j.ocecoaman.2020.105099
Reguero, B. G., Losada, I. J., and Méndez, F. J. (2019). A recent increase in global wave power as a consequence of oceanic warming. Nat. Commun. 10, 205. doi:10.1038/s41467-018-08066-0
Reguero, B. G., Méndez, F. J., and Losada, I. J. (2013). Variability of multivariate wave climate in Latin America and the Caribbean. Glob. Planet Change 100, 70–84. doi:10.1016/j.gloplacha.2012.09.005
Restrepo-Ángel, J. D., Mora-Páez, H., Díaz, F., Govorcin, M., Wdowinski, S., Giraldo-Londoño, L., et al. (2021). Coastal subsidence increases vulnerability to sea level rise over twenty first century in Cartagena, Caribbean Colombia. Sci. Rep. 11, 18873. doi:10.1038/s41598-021-98428-4
Rollenbeck, R., Orellana-Alvear, J., Bendix, J., Rodriguez, R., Pucha-Cofrep, F., Guallpa, M., et al. (2022). The coastal El Niño event of 2017 in Ecuador and Peru: A weather radar analysis. Remote Sens. (Basel) 14, 824. doi:10.3390/rs14040824
Rostami, K., Peltier, W. R., and Mangini, A. (2000). Quaternary marine terraces, sea-level changes and uplift history of patagonia, Argentina: comparisons with predictions of the ICE-4G (VM2) model of the global process of glacial isostatic adjustment. Quat. Sci. Rev. 19, 1495–1525. doi:10.1016/s0277-3791(00)00075-5
Silva, F. G., de Oliveira Sousa, P. H. G., and Siegle, E. (2016). Longshore transport gradients and erosion processes along the Ilha Comprida (Brazil) beach system. Ocean. Dyn. 66, 853–865. doi:10.1007/s10236-016-0956-9
Silva, R., Martínez, M. L., Hesp, P. A., Catalan, P., Osorio, A. F., Martell, R., et al. (2014). Present and future challenges of coastal erosion in Latin America. J. Coast Res. 71, 1–16. doi:10.2112/SI71-001.1
Silva-Casarin, R., Ruiz-Martinez, G., Mariño-Tapia, I., Posada-Vanegas, G., Mendoza-Baldwin, E., and Escalante-Mancera, E. (2012). Manmade vulnerability of the Cancun beach system: the case of hurricane Wilma. Clean. (Weinh) 40, 911–919. doi:10.1002/clen.201100677
Simões, R. S., Calliari, L. J., de Figueiredo, S. A., de Oliveira, U. R., and de Almeida, L. P. M. (2022). Coastline dynamics in the extreme south of Brazil and their socio-environmental impacts. Ocean. Coast Manag. 230, 106373. doi:10.1016/j.ocecoaman.2022.106373
Small, C., and Nicholls, R. J. (2003). A global analysis of human settlement in coastal zones. J. Coast Res. 19, 584–599.
Solari, S., Alonso, R., and Teixeira, L. (2018). Analysis of coastal vulnerability along the Uruguayan coasts. J. Coast. Res. 85, 1536–1540. doi:10.2112/SI85-308.1
Soto, M.-V., Arriagada, J., Castro-Correa, C. P., Ibarra, I., and Rodolfi, G. (2015). Condiciones geodinámicas derivadas del terremoto y tsunami de 2010 en la costa de Chile central: El caso de Pichilemu. Rev. Geogr. Norte Gd. 60, 79–95. doi:10.4067/s0718-34022015000100005
Speranski, N., and Calliari, L. (2001). Bathymetric lenses and localized coastal erosion in southern Brazil. J. Coast Res. 1, 209–215.
Toimil, A., Losada, I. J., Camus, P., and Díaz-Simal, P. (2017). Managing coastal erosion under climate change at the regional scale. Coast. Eng. 128, 106–122. doi:10.1016/j.coastaleng.2017.08.004
TU Delft (2013). Case 21: TU Delft top collaborators. Available at: https://www.tudelft.nl/library/research-analytics/case-21-tu-delft-top-collaborators-2 (Accessed July 13, 2023).
Valderrama-Landeros, L. H., Martell-Dubois, R., Ressl, R., Silva-Casarín, R., Cruz-Ramírez, C. J., and Muñoz-Pérez, J. J. (2019). Dynamics of coastline changes in Mexico. J. Geogr. Sci. 29, 1637–1654. doi:10.1007/s11442-019-1679-x
Vallarino-Castillo, R., Negro-Valdecantos, V., and Moreno-Blasco, L. (2022). Shoreline change analysis using historical multispectral Landsat images of the pacific coast of Panama. J. Mar. Sci. Eng. 10, 1801. doi:10.3390/jmse10121801
Vellanoweth, R. L., Porcayo-Michelini, A., Guttenberg, R. B., Hayden, W., Ainis, A. F., and Hernández-Estrada, R. L. (2020). Spring tides, storm surges, and the destruction of coastal middens: A case study from the upper gulf of California, méxico. J. Isl. Coast. Archaeol. 17, 420–431. doi:10.1080/15564894.2020.1782539
Vernette, G., Gayet, J., Bobier, C., and Briceno, L. (1992). Mud diapirism, fan sedimentation and strike-slip faulting, Caribbean Colombian Margin. Tectonophysics 202, 335–349. doi:10.1016/0040-1951(92)90118-p
Villagrán, M., Gómez, M., and Martínez, C. (2023). Coastal erosion and a characterization of the morphological dynamics of Arauco gulf beaches under dominant wave conditions. Water (Basel) 15, 23. doi:10.3390/w15010023
Villamizar, A., Gutiérrez, M. E., Nagy, G. J., Caffera, R. M., and Leal Filho, W. (2017). Climate adaptation in South America with emphasis in coastal areas: the state-of-the-art and case studies from Venezuela and Uruguay. Clim. Dev. 9, 364–382. doi:10.1080/17565529.2016.1146120
Vousdoukas, M. I., Ranasinghe, R., Mentaschi, L., Plomaritis, T. A., Athanasiou, P., Luijendijk, A., et al. (2020). Sandy coastlines under threat of erosion. Nat. Clim. Chang. 10, 260–263. doi:10.1038/s41558-020-0697-0
Winckler, P., Agredano-Martín, R., Esparza, C., Melo, O., Sactic, M. I., and Martínez, C. (2023). Projections of beach erosion and associated costs in Chile. Sustain. Switz. 15, 5883. doi:10.3390/su15075883
Keywords: coastal erosion, coastal hydrodynamics, erosion drivers, shoreline change, beach morphodynamics, coastal hazards, Latin America
Citation: Vallarino Castillo R, Negro Valdecantos V and del Campo JM (2023) Understanding the impact of hydrodynamics on coastal erosion in Latin America: a systematic review. Front. Environ. Sci. 11:1267402. doi: 10.3389/fenvs.2023.1267402
Received: 26 July 2023; Accepted: 20 September 2023;
Published: 04 October 2023.
Edited by:
Celso Santos, Federal University of Paraíba, BrazilReviewed by:
Edgar Mendoza, National Autonomous University of Mexico, MexicoJuan Jose Munoz-Perez, University of Cádiz, Spain
Copyright © 2023 Vallarino Castillo, Negro Valdecantos and del Campo. This is an open-access article distributed under the terms of the Creative Commons Attribution License (CC BY). The use, distribution or reproduction in other forums is permitted, provided the original author(s) and the copyright owner(s) are credited and that the original publication in this journal is cited, in accordance with accepted academic practice. No use, distribution or reproduction is permitted which does not comply with these terms.
*Correspondence: Ruby Vallarino Castillo, ruby.vallarino@alumnos.upm.es