- 1Department of Biological, Geological and Environmental Sciences, Alma Mater Studiorum University of Bologna, Bologna, Italy
- 2Istituto di Scienze Marine ISMAR CNR, National Research Council of Italy (CNR), Area Della Ricerca di Bologna, Bologna, Italy
- 3Department of Ecosustainable Marine Biotechnology, Stazione Zoologica Anton Dohrn, Napoli, Italy
Introduction: Municipal Solid Waste Incineration (MSWI) plants generate significant amounts of solid end-products, such as bottom ash (BA), containing potentially toxic elements like Cr, Ni, As, Cd, and Pb, base elements (e.g., Si, Al, Fe, Ti, Cu, and Zn), and other technology-critical elements (TCE), such as Co, Ga, Mg, Nb, P, Sb, Sc, V, Li, Sr, and REE. The accurate determination of these elements in anthropogenic wastes and the assessment of their removal are crucial for the circular economy.
Methods: This paper aims to characterize BA samples from two Italian MSWI plants (named FE and FC) by X-ray fluorescence (XRF) and comparatively assess the removal of a selection of elements using the aqua regia digestion (ARD) method, followed by Inductively Coupled Plasma Mass Spectrometry (ICP-MS) analytical determination.
Results and discussion: According to the XRF analysis, Ca, Fe, Al, Mg, and Na had high concentrations in BA, and their contents increased with decreasing particle size in both FE and FC samples. The Enrichment Factor (EF) based on the upper continental crust’s average values of Zn, Cu, and Pb was high (EF > 30), while Cr, Ni, and As were scarcely enriched (EF > 1), and REE enrichment was very low (EF < 1). In both FE and FC plants, the Degree of Elements Extractability (DE) was high (>80%), especially in the fine-grained fractions of MSWI bottom ash. The Enrichment Factor (EF) based on the upper continental crust average values of Zn, Cu, and Pb was high (EF > 30), while Cr, Ni, and As were scarcely enriched (EF > 1), and REE enrichment was very low (EF < 1). The bibliometric analysis helped highlight research trends in the assessment and treatment of MSWI-BA, discriminating the literature impact on environment/health issues and recovery/recycling strategies for the circular economy associated with the MSWI-BA material.
Conclusion: Although higher data coverage is needed, the present study suggests ARD as an effective method for better understanding the environmental impact and recoverability of useful elements from anthropogenic materials like MSWI bottom ash.
1 Introduction
Most European countries are concerned with moving toward a sustainable recycling society and keen to minimize waste production and exploit certain types of waste as a resource. Municipal Solid Waste Incineration (MSWI) is a fundamental base of the circular economy in terms of its significance in waste management and power generated with Waste-to-Energy (WtE) technology (Malinauskaite et al., 2017). MSWI solid residues are increasing with rapid urbanization, and notably, the incineration end-product contains valuable elements for urban mining (Pan et al., 2013; Funari et al., 2015; Zhao et al., 2022; Funari et al., 2023) including critical raw materials for the EU (European Commission, 2020). BA is a heterogeneous solid material containing hard pebbles, metals, ceramics, cullet glass, slag, and unburnt materials, in a variety of mineralogical phases (Bayuseno and Schmahl, 2010; Mantovani et al., 2021). BA has a different chemical and mineralogical composition, resulting in potential environmental impacts (Chen et al., 2020; Nikravan et al., 2020). The BA composition can vary among different incinerators, depending on the country, seasonality, incinerator facility and technology, and the efficiency of waste collection (He et al., 2004; Hyks and Astrup, 2009; Wang et al., 2019). However, elemental concentrations generally fall within a narrow range (Funari, 2022).
There is increasing interest in this subject in the literature according to a bibliometric analysis conducted for this study. China contributes more to MSWI-BA treatment and elemental resource recovery than any other country, followed by the United States and Italy (Supplementary Figure S1). Bibliometric analysis was used to visualize and reveal the complete indicators of MSWI management from accessible scientific publications present in the Scopus database (Shukla et al., 2019). The bibliometric analysis also showed the direction of future studies on MSW management and the role of MSWI ash from a circular economy perspective to minimize waste and resource management and to identify the solutions to create better inventories and develop economic flows (Zeller et al., 2019; Tsai et al., 2020; Ranjbari et al., 2021).
In some countries, BA can be used as a secondary raw material for road construction or manufacturing in the ceramic industry because its composition is similar to that of basaltic rocks and other geological materials (Chimenos et al., 2000). For example, studies assessed the reuse of raw BA and characterized selected elements in bulk and grain size BA samples (Yao et al., 2013; Abramov et al., 2018). Before recycling as a secondary raw material or disposal in landfills, BA is characterized and possibly treated for potentially toxic element (As, Cd, Cr, Ni, and Pb) remediation and for the recovery of valued elements (base metals: Si, Al, Fe, Ti, Cu, and Zn; technology-critical elements (TCE): Ba, Co, Ga, Mg, Nb, P, Sb, Sc, V, Li, Sr, and REE). The BA residues have significant potential for metal recovery (Muchova et al., 2009; Allegrini et al., 2015; Šyc et al., 2020). However, the recovery of Al, Zn, Cu, and Au is currently limited to a handful of specialized MSWI plants (Šyc et al., 2020), whereas the recovery of other elements of economic interest is overarchingly low. Yet, it is of great importance to assess the content of potentially toxic elements and recoverable elements from MSWI-BA to benefit from urban solid waste streams and avoid ill-controlled landfilling. The MSWI BA contains several metals originating from metallic scraps and smaller metallic objects, resulting in minor to trace metals hosted by a variety of mineral phases (Bunge, 2015). Small particles (<2 mm) are anticipated to contain a significant amount of precious non-ferrous metals (Bunge, 2015), and some elements are known to be concentrated in specific BA grain-size fractions (Yao et al., 2017; Chen et al., 2020; Nikravan et al., 2020; Li et al., 2022). Therefore, it is crucial to separate small to large particles for effective metal recovery from MSWI-BA because the particle size of BA has a significant impact on the separation efficiency of non-ferrous metals, which varies depending on the separation process (Šyc et al., 2018). A research gap still exists in the detailed characterization of BA in the published literature, i.e., a complete combination of metal leaching, elemental composition, and environmental impact is needed to reflect the importance of the utilization of MSWI-BA grain-size fractions. Studies related to the separation of the MSWI-BA fractions with different sizes for metal recovery are limited, and the utilization of small (fine) to large particles (bulk) of MSWI-BA has become a critical waste resource for efficient metal recovery. A complete characterization of MSWI-BA might make it possible to evaluate and use not only a particular fraction but all the fractions in the most effective way. Hence, this study could help to identify the most metal-abundant fraction in a comparison of grain sizes of MSWI-BA, based on the recovery of sustainable metals, and to effectively reduce environmental risks by removing potentially toxic elements.
The present study comparatively characterizes BA samples from two Italian MSWI plants (named FE and FE hereafter) and assesses the relative abundance of selected elements in bulk BA samples (bulk <8 mm) and in selected grain-size fractions (coarse 4–8 mm, medium 1–2 mm, and fine 0.5–1 mm) by Enrichment Factor (EF) and elemental Degree of Extraction (DE) after pseudo-total digestion. We used a bibliometric analysis to discuss the findings and research trends from the literature to encourage MSWI-BA sustainable management using existing or transfer-ready technologies as a helpful tool for decision-making in planning investment and advancing research fields.
2 Methods and materials
2.1 MSWI BA sampling and preparation
The sampling campaign of BA samples was conducted in 2020 at the FE and FC MSWI plants in northern Italy. Both MSWI plants are WtE systems that generally burn domestic household solid wastes (more than 90%) and special waste (10%). The FE MSWI plant was built in 1993 and upgraded in 2007. The FE incinerator plant has the potential to operate 142,000 tons of waste per year. The FC MSWI plant was built in 1976 and upgraded in 2008 (Branchini, 2012). The maximum operating capacity of FC MSWI plant is 60,000 to 120,000 tons per year of urban waste. Storage points and processing stages of MSWI facilities are described elsewhere (Funari et al., 2016). The BA stockpile consists of melted phases and remaining unburnt and noncombustible materials, and the pile is dry if it cools down at the exit and wet if water quenched (Berkhout et al., 2011). The heap of several tons of BA material, which was representative of the first quarter of 2020 production according to the facility managers, was sampled from the BA storage site following a previous approach (Funari et al., 2015). The primary samples of BA from both incinerators were homogeneously mixed and quartered to obtain representative samples (total 16 samples). The BA samples were sieved by shaking with stainless steel mesh sieves to make grain-size fractions, following the standard procedure adopted elsewhere (Yao et al., 2013). Different grain-size fractions of MSWI-BA were prepared for both sites, including bulk (<8 mm, samples FEB, FCB), coarse (4–8 mm, samples FEC, FCC), medium (1–2 mm, samples FEM, FCM), and fine (0.5–1 mm, samples FEF, FCF). All the BA samples were oven-dried at 40°C for 24 h and, after separation pre-treatment, thoroughly ground and milled to powder form (>40 µm) by an agate vibratory disc mill.
2.2 Experimental activity and chemical analysis
An aqua regia digestion (ARD) was performed on the BA samples using a microwave digester (Milestone MLS 1200 Mega). Exactly 0.5 g of dried and milled BA samples were dissolved with aqua regia solution (12 mL) in closed Teflon bombs up to 200°C. The ARD method is a quick, secure, and effective digestion technique and is resistant to heavy metal losses (especially volatile metals). It is reported that ARD is effective in assessing the potential recovery of metals (Fabricius et al., 2020) and proved an economically feasible option to understand the recoverability of base metals and TCE from MSWI-BA and other types of matrices. The solutions from ARD were analyzed by ICP-MS ELAN DRC-e (Perkin Elmer), and blank samples were included in the analysis to check for eventual contamination during the experiment. Further details can be found in the Supplementary Materials. Sample digestion analysis was carried out in duplicate. Certified Reference Material (CRM) of MSWI fly ash (BCR-CRM176) from the Community Bureau of Reference, European Commission, was analyzed as an unknown sample to assess the accuracy and precision of the analytical procedure. The CRM was also used to show a comparison of the recovery of certified and measured values of elements extracted in the same ARD method.
The Loss on Ignition (LOI) of all the BA samples was gravimetrically estimated after overnight heating at 950°C in open platinum crucibles (Heiri et al., 2001). The total concentration of major and trace elements (Supplementary Table S1 and Supplementary Figure S2) was determined on thin-layer powder pellets using a wavelength dispersive X-ray fluorescence (XRF) spectrometer, Panalytical Axios 4,000, equipped with an Rh tube. The same CRM (BCR-CRM176) was included in the analytical work to monitor the long-term homogeneity and reproducibility within different analytical batches (see Supplementary Table S1). The reproducibility of major elements was found to be better than 5%, whereas it was better than 10% for trace elements on average. The absolute accuracy of the validated CRM values was frequently within the reproducibility range. The detection limits of all major and trace elements are presented in Supplementary Table S1.
2.3 Evaluation of metal enrichment and extractability
Enrichment factors (EFs) were calculated to compare the relative abundance of a given element in the BA samples, relative to the same element in a reference material. Specifically, the EF is calculated as the measured concentration of an element (X) in the BA sample divided by the same element’s mean concentration in the Upper Continental Crust (UCC) (Rudnick et al., 2014). We used titanium mass fraction for the normalization purpose as a reference element for MSWI-BA. The EF was calculated using the following Equation 1:
Where (X) sample and (Ti) sample are the concentrations of analyte element and Ti in the sample, and (X) crust and (Ti) crust are their concentrations of average compositions in the UCC, as reported by Rudnick et al. (2014).
Degree of Extraction (DE) was calculated (Eq. 2) to assess the extractability of elements based on pseudo-total (ARD) and total composition (Toller et al., 2022):
Where Ci ARD represents the results from ARD and Ci RTM is the total concentration from XRF analysis.
Descriptive statistics, including minimum, maximum, mean, and standard deviation, were done using Microsoft Excel 365. Figures were plotted using Microsoft Excel 365 and Sigmaplot (version 14.0).
2.4 Scopus bibliometric data analysis
In the present study, Scopus bibliometric resources (using articles retrieved from 2010 to 2022) were analyzed using VOSviewer software (version 1.6.18, Leiden University, Leiden, Netherlands) as a reference to constrain our findings and possibly find literature trends and/or gaps. VOSviewer is used to construct and visualize bibliometric networks (van Eck et al., 2006), including journals, scholars/researchers, citations-based maps, bibliographic connections, and co-authorship relationships.
The data retrieval query included typical municipal solid waste-related keywords (such as thermal treatment, incinerator ash, and bottom ash), and various keywords (such as municipal solid waste incineration plants, waste to energy, municipal solid waste residues, bottom ash characterization, metal recovery, etc.) as shown in the Supplementary Material. All the keywords were connected by logical aggregation operators. Research articles, review articles, and conference paper categories of scientific publications were selected for bibliometric analysis. For the word cloud chart analysis (Supplementary Figure S1), the CSV file from the VOSviewer query results was used in wordclouds.com to construct a word cloud chart of country names in different font sizes. The size of each word conveys its significance and its frequency of use in the literature related to the query (Supplementary Material).
3 Results and discussion
3.1 Characterization of BA samples
3.1.1 Total composition
Supplementary Table S1 and Supplementary Figure S3 show the total element composition in FE-BA and FC-BA as indicated by XRF. The most abundant elements in both plants are Si and Ca, with similar bulk concentrations of Si (about 120,000 mg/kg) and slightly higher Ca in FE-BA (221,890 mg/kg) than FC-BA (208,280 mg/kg). Ca and Si were observed with elevated concentrations, at (228,510 and 198,000 mg/kg) and (77,740 and 100,340 mg/kg), in fine fractions (FEF and FCF) of FE-BA and FC-BA, respectively. It is likely that Si and Ca persist in BA after the incineration process due to their low volatility and high boiling temperature (Nikravan et al., 2020). Moderate Fe content, at (42,060 mg/kg in FE) vs. (59,230 mg/kg in FC), was recorded in bulk samples, with higher concentrations in fine fractions (58,660 mg/kg in FE, 60,810 mg/kg in FC). Similarly, Al was observed at around 40,520 mg/kg in bulk BA of FE and 42,370 mg/kg of FC, with higher concentration in the fine fractions in both FE-BA and FC-BA (see Supplementary Table S1). Base metals like Zn and Cu showed high abundance (>4,500 mg/kg and >2,500 respectively), while Co (>40 mg/kg), Pb (>900 mg/kg), Cr (>500 mg/kg), Ni (>120 mg/kg), and As (>30 mg/kg) were at moderate to high concentrations in all the grain sizes for both plants (Supplementary Figure S3). The concentration of Cu, Ni, Rb, Ga, and Pb were in agreement with the previous determinations for Italian incineration BA collected in May 2013 (Funari et al., 2015). However, the concentrations of TCE, such as Ba, V, Ga, Sr, and Nb, were observed as being higher than the previously reported values from grain size BA from MSWI plants in northern Italy (Mantovani et al., 2023). The LOI contents (ca. 18% on average) were similar between FE-BA and FC-BA, with slightly higher values in their fine-grained samples (Supplementary Table S1).
The two plants show a rather similar composition among the different grain sizes considered (Figure 1) even if only for some elements (Zn, Pb, Cu, Co, As). The other TCE elements (Ba, Ga, Mg, Nb, P, and V) showed less fluctuation in the composition between the two plants. The total chemical compositions of BA samples from FE and FC are consistent with the average composition of BA from Italian MSWI plants (Funari et al., 2015; Funari et al., 2016). There is fluctuation for Zn, Co, V, As, and Ce (also among grain-size fractions) compared to the average Italian BA (Supplementary Figure S3). Variation in trace element composition is likely to occur (Nikravan et al., 2020) as a primary consequence of the heterogeneous input waste. Overall, the concentrations of Ca, Al, Mg, Co, and Cr were lower than the reported values of MSWI-BA for other countries (Abramov et al., 2018; Loginova et al., 2019). The different behavior of elements like Pb and Co among the grain-size fractions, especially for coarse and fine fractions, could be explained by the intrinsic property of the metal species, which would alter their partitioning during the thermal treatment (Yao et al., 2013). The concentration of other elements, such as As, Cd, Cu, Ni, and Zn, is somewhat different from the reported values in other studies (Allegrini et al., 2014; Nikravan et al., 2020).
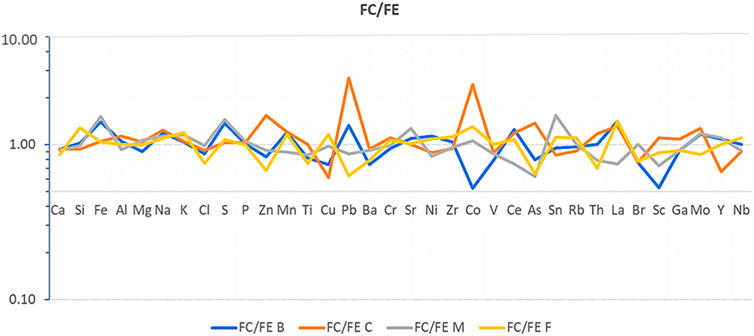
FIGURE 1. Comparison of total element composition by XRF in BA grain sizes from FE and FC. Ratios higher than 1 means enrichment in FC samples, lower than 1 depletion.
These elements could be bounded to non-silicate minerals in combination with Fe and Zn or may be found in different metallic inclusions (Wei et al., 2011) as well as in other glassy phases (Mantovani et al., 2021) in BA. These elements occur in BA in different oxidation states (Rissler et al., 2020), and they are frequently bounded to carbonates, chlorides, aluminosilicates, oxides, and hydroxides. Metallic forms of Zn and Cu are possibly associated with their combination in brass and with their association with sulfates (Bayuseno and Schmahl, 2010). Carbonates and iron-manganese oxide forms, organic bound forms, and exchange and residual forms could be bounded with elements in BA (Xiang et al., 2022). BA also primarily contains silicate-based glass phases and mineral phases including spinels, melilites, pseudowollastonite, metallic inclusions (heavy metal-bearing phases), and ceramics. Non-silicate mineral phases like spinel group minerals, hercynite, magnetite, calcium-rich mineral phases such as Calcite (CaCO3), Anorthite (Ca(Al2Si2O8)), Akermanite (Ca2Mg(Si2O7)), Gehlenite (Ca2Al(AlSiO7)), Vaterite (CaCO₃), Portlandite (Ca(OH)2), and Larnite (Ca2SiO4) (Mantovani et al., 2023), as well as refractory phases (quartz and plagioclase) are frequently found in BA with great proportion (Wei et al., 2011), possibly controlling element occurrence in MSWI-BA.
3.1.2 Aqua regia digestion
The composition of FE and FC MSWI-BA determined by ICP-MS after the ARD method is reported in Table 1 and Supplementary Figure S4. The variation in concentrations was observed for both bulk FE and FC samples and their fine, medium, and coarse grain-size fractions. The comparison between certified and measured values shows the quality and accuracy of the acid digestion performed on the CRM and BA samples.
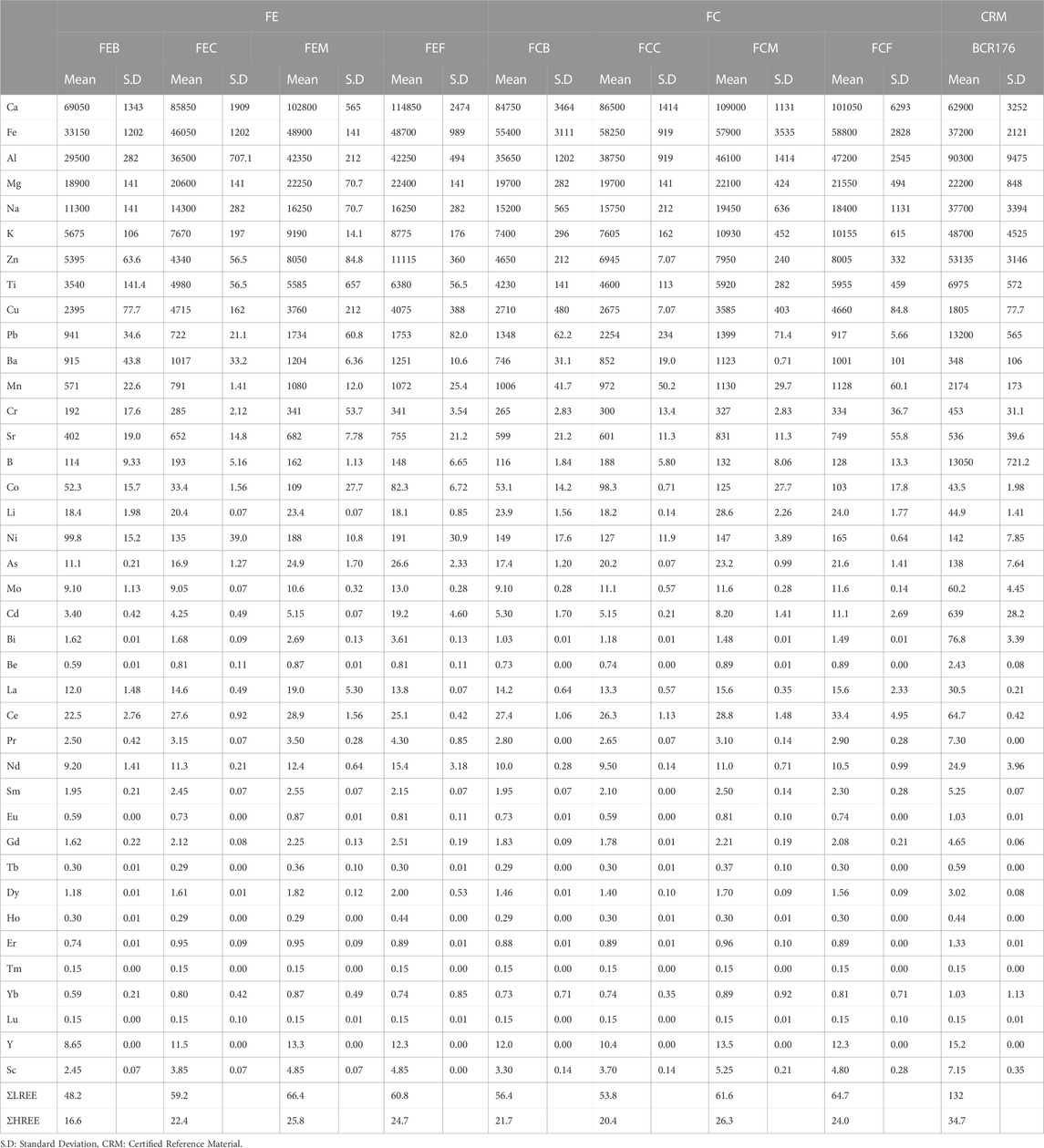
TABLE 1. Descriptive statistics of selected elements concentration (mg/kg), analyzed by ICP-MS after aqua regia digestion in FE- and FC-BA, as compared to CRM.
On the contrary, the recovery of potentially toxic elements (As, Cd, Cr, and Pb) and other TCE (Ba, Ga, Nb, Sc, V, and Li) was relatively low in BA grain-size fractions compared to the measured reference values after the same technique. Similar results were reported in the previous study by Allegrini et al. (2014). The low abundance of potentially toxic elements, such as As, Cd, Ni, and Pb, in both bulk samples (FEB and FCB) in comparison with the other fractions, confirms the hypothesis of selective enrichment in grain-size fractions. Similar results were observed in a study by Pan et al. (2013). The concentrations of Na, Mg, and Fe were higher, whereas K and Al were observed with low concentrations, which is similar to the findings of the previous study on the bulk composition of BA from the MSWI plant in Zhejiang province, China (Yao et al., 2017). These findings, with high extractions by microwave digestion, reported by Han et al. (2021) substantiate that elements in MSWI bottom and fly ash are associated with acid-soluble phases and can be easily dissolved by ARD.
Some elements showed an increasing trend with decreasing grain sizes (Table 1 and Supplementary Figure S4) for both MSWI plants. In particular, the contents of Ca, Al, Mg, Fe, Na, Cu, Co, Cr, Pb, Ni, Cd, As, and Zn were higher in the fine-grained BA fractions (FEF and FCF) compared to the other fractions. Similar results were reported by Yao et al. (2013), who showed that Cu, Zn, and Cd were higher in the fine-sized fractions of BA. Several researchers have proposed that fine-sized BA constitutes a greater hazard due to its high surface area, enabling the absorption and capture of more environmental pollutants and chemicals (Chimenos et al., 1999). Therefore, the finest fractions represent an environmental hazard. However, these elements (Cu and Zn), as well as several potentially toxic elements and other TCEs, are economically valuable, and the fine fractions with grain size 0.5–1 mm could be viable sources for the recovery of such elements.
Regarding valuable elements, Cu, Zn, and Co showed the greatest fluctuation amongst grain sizes. Other elements, like Ba, Bi, Mo, and REE (especially Tb, Dy, Ho, Er, Tm, Yb, and Lu), showed less fluctuation (Supplementary Figure S5). The sum of Light Rare Earth Elements (ΣLREE) concentrations was quite comparable, ranging from 48.2 to 66.4 mg/kg (FE-BA) and 53.8–64.7 mg/kg (FC-BA), while the sum of Heavy Rare Earth Elements (ΣHREE) concentration ranged between 16.6 and 24.7 mg/kg (FE-BA) and 20.4 and 26.3 mg/kg (FC-BA). Among REE, the highest concentration was observed for Ce of FEF and FCF with mean concentrations of 25.1 and 33.4 mg/kg, respectively. Similarly, La and Y reached > 10 mg/kg for FEF and FCF, while the LREE (Nd, Pr, Sm, Eu, and Gd) and other HREE (Dy, Ho, Sm, Er, Tm, Yb, and Lu) showed the lowest (<1 mg/kg) concentrations. In both FE-BA and FC-BA, the concentrations by ICP-MS after the ARD method of most elements, including potentially toxic elements, REE, and those of significant importance for the circular economy, were in decreasing order in fine > medium > coarse > bulk samples (Table 1). Most REE in both FE-BA and FC-BA measured by ICP-MS after ARD are comparable and in agreement with previous studies (Funari et al., 2015; Westerhoff et al., 2015).
In general, REE behavior in the incinerator can be influenced by the melting temperature and input composition of the combustor (Zhao et al., 2008). In Figure 2, the chondrite-normalized patterns of REE (McDonough and Sun, 1995) in all BA samples were compared to the UCC (Supplementary Table S2), to highlight fluctuation from geogenic materials. The HREE of FE-BA and FC-BA patterns exhibit weak enrichment with respect to the LREE and UCC. The general trend of REE in BA showed similarity with the averages of crustal samples. Eu, Ho, Tm, and Tb anomalies may be visible. Overall, the chondrite-normalized patterns of all FE-BA were distinguishable from the FC-BA.
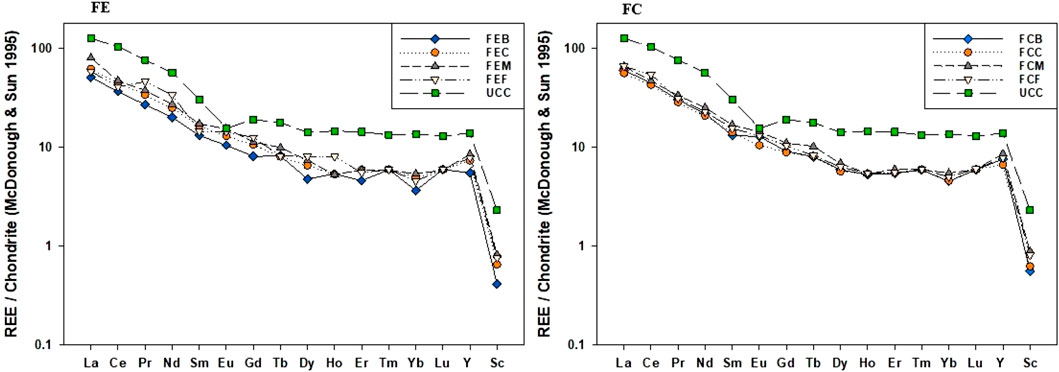
FIGURE 2. Chondrite-normalized composition of REE in FE-BA, and FC-BA based on calculation of the Chondrite averages by (McDonough and Sun, 1995).
3.2 Evaluation of metal enrichment for the circular economy
The EFs were calculated to identify the enrichment of selected elements in both plants (Figure 3) using aqua regia data. Notably, valuable elements, like Zn and Cu, and some potentially toxic elements (Pb and Cd) were moderately enriched in all samples from both plants. The EF of Zn, Cu, and Pb were comparatively lower than the values (EF < 100) reported by Fujimori et al. (2004). Westerhoff et al. (2015) reported lower Cu and Pb enrichments and Lanzerstorfer (2017) also reported lower enrichments. These elements are extensively used in industrial materials, explaining the high enrichments in MSWI-BA (Westerhoff et al., 2015). Cd showed comparable EF values, which were particularly high in fine-sized BA (Figure 3). Chromium and Ni were scarcely enriched (EF > 1) in all grain sizes of BA, and in the same enrichment range, As, Co, Ba, and Sr were also present. According to Figure 3, REE was depleted compared to the UCC reference. TCE, like REE, has a wide range of industrial applications, particularly in high technology material, but their enrichment in MSWI BA is nearly negligible (Verplanck et al., 2005; Rabiet et al., 2009), likely due to the importance of specific recovery routes.
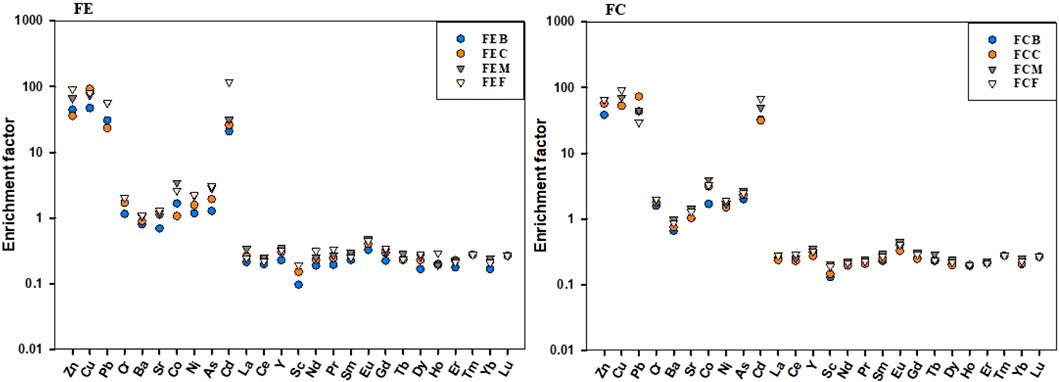
FIGURE 3. EF based on the upper continental crust averages for selected elements in BA bulk samples (B) and their sized fractions (C = coarse; M = medium; F = fine). FE and FC are the two MSWI plants considered.
Overall, several elements are more enriched in fine-sized fractions (FEF and FCF) compared to other fractions. Although the BA samples originated from burning varied amounts and kinds of input waste and are from two different incinerators, a substantial homogeneity within BA is visible (Figure 3).
The general variability of metal recovery in the present study of FE-BA and FC-BA is noticeably attributed to sample heterogeneity, resulting from possible uncertainties in primary sample size, different kinds of waste input, specific ash characteristics behavior, condensation, treatment, and combustion conditions of the two incineration facilities (Li et al., 2004; Loginova et al., 2019). The results of EF showed that a range of elements can be recovered from bulk or grain size MSWI-BA fractions for the circular economy, for example, with advanced separation techniques.
3.2.1 Metal enrichment in BA vs. BA treatment trend from 2010 to 2023
In the present study, the recovery of metal enrichment in BA was comparatively assessed in past studies from 2010 to 2023 (via clustering map analysis). To date, the majority of research studies have focused on aspects related to understanding utilization and recycling (Morf et al., 2013; Joseph et al., 2021) with an emphasis on leaching behaviors and metal recovery (Astrup et al., 2016; Weibel et al., 2017). According to a bibliometric clustering map analysis of research articles from 2010 to 2023, three clusters can be identified: blue, green, and red (Supplementary Figure S6). The bibliometric clustering map analysis was done based on the query mentioned in Supplementary Material section 1.2. The choice of timeframe for publication search was based on the assumption of technological advancement of incinerators and the utilization of MSWI-BA for the recovery of base elements and TCE, the need for which has increased in the circular economy since 2010.
The red cluster suggests a substantial interest in BA treatment with the recovery of elements of economic importance (base metals: Si, Al, Fe, Ti, Cu, and Zn; TCE: Ba, Co, Ga, Mg, Nb, P, Sb, Sc, V, Li, Sr, and REE). The most relevant elements are Cu, Cr, and Zn. On the contrary, the observed low score (small red circles in Supplementary Figure S6) of Ti, Sr, Ba, and Sn suggests they did not attract much interest in the past, likely due to their abundance (Table 1) being too low to be of mineable grade. Although the REE concentrations were low for all grain-size fractions (Table 1), trace elements like REE have received slightly more attention in past studies because their recovery attempts are important for the circular economy and because of their potential environmental impact. The blue cluster (blue circles) indicates the “principal components” of MSW, showing “MSW”, “Solid waste”, “Waste to energy”, “Zn compounds”, “Cu compounds”, “Circular economy”, and “Waste management”, indicating the utilization and recycling of MSWI-BA. This cluster encompasses the importance of management strategies in the utilization of BA in terms of the circular economy. The green cluster (green circles) refers to the terms “Aluminum compounds”, “Calcium compounds”, “Sulfur compounds”, “Sodium compounds”, “Chloride”, “Sulfate”, “Silicates”, “Aluminum oxides”, “Calcium oxides”, and “Calcium carbonate”. The recovery attempts of these elements and compounds are higher in BA due to the high affinity of volatile compounds, silicates, and oxide minerals. The presence of elements like As, Cd, Pb, and Tl in the red cluster (red circles) suggests an affinity with the terms “remediation” and “potentially toxic elements”. This VOSviewer analysis demonstrates that the urban waste stream of BA in MSWI can be utilized to efficiently gain potential outputs with industrial and environmental benefits and to avoid landfilling.
In the present study, less discussed elements such as Ba, Ti, Sr, and Sn were selected to add data coverage and help decision-makers plan investments for new lines of treatment. Their recovery from MSWI-BA is crucial for the circular economy, as suggested by the vicinity and connections of the corresponding clusters (Supplementary Figure S6). Furthermore, our results were found to be consistent with the previous study of bibliometric analysis by identifying the research trends of waste-to-energy incineration from 1999 to 2015 (Wang et al., 2016). They found the most searched terms “Bottom ash” and “Heavy metals” in “Cluster 1” nodes, showing the high research priority of the treatment of MSWI-BA for metal recovery. Other terms, like “Waste management” and “Recycling”, appeared to be in central nodes of the cluster, indicating management area. Few studies of bibliometric analysis indicated that the leaching behavior of heavy metals in MSWI-BA has been a substantial concern. For instance, a similar study of bibliometric analysis of emerging trends (from 1994 to 2018) in MSWI ash showed that “Heavy metals” appeared to be the most critical and frequently used keyword in the cluster analysis (Wong et al., 2020; Mostafa Hatami et al., 2022) came to the same conclusion analysing the bibliometric record from 2010 to 2021. It is noteworthy that the keywords of metals “Cu”, “Zn”, and “Pb” appeared mostly with larger nodes in the VOSviewer map. The occurrence of these valuable metals in previous bibliometric analysis showed consistency with the research trend of our present results that recovery and removal of Cu, Zn, and potentially toxic elements (Pb, Cd, As) in MSWI ash like BA has received significantly more attention than other metals.
3.3 Degree of elements extractability
ARD data from ICP-MS analysis and total concentrations of selected analytes attained by XRF data were compared by calculating the DE (%), as reported in Table 2. High (>75%), moderate (30%–75%), and low DE (<30%) values are presented in red, green, and blue, respectively. The results showed that most of the elements had high DE (>80%) in both FE-BA and FC-BA. For seized fractions, Fe, Al, Na, Mg, Ba, Cu, Co, Ti, Sr, Mo, Pb, Ni, Zn, and Y had a high DE of 80%–100% in both FE-BA and FC-BA. These findings, with high extractions by microwave digestion, reported by Han et al. (2021) substantiate that these elements in MSWI bottom and fly ash are associated with acid-soluble phases and can be easily dissolved by ARD.
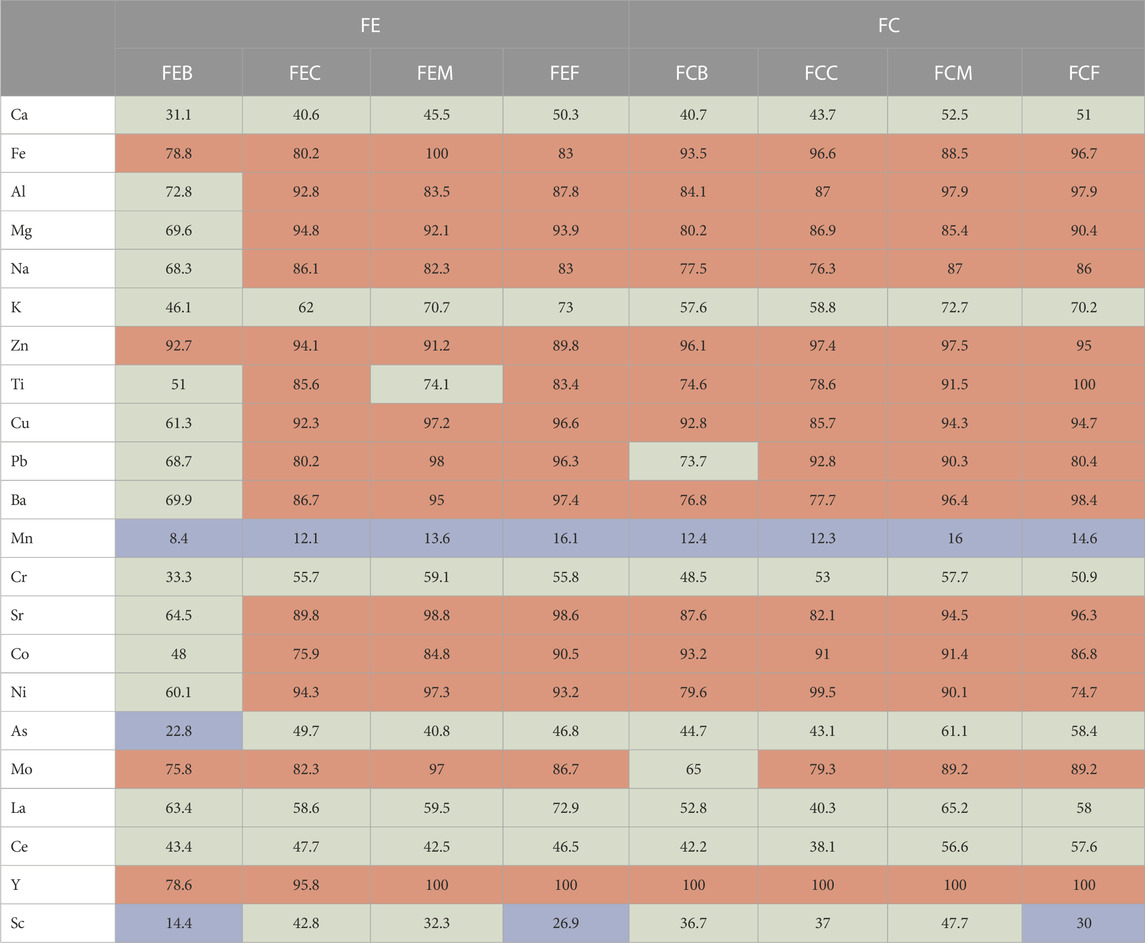
TABLE 2. Degree of extraction (%) of total concentration by ARD and XRF analyzed elements for FE- and FC-BA.
A first group with high DE of Na and Mg (>75%) in all the grain-size fractions except FEB and FCB (<70%) can be compared to other studies (Yao et al., 2010; Abramov et al., 2018). Na and Mg, possibly from carbonates, soluble salts of sodium chloride, and sodium hydroxide were highly extracted in all the fractions (Hong et al., 2000; Quina et al., 2008). Similarly, K was extracted with moderate to high DE (40%–75%), likely attributed to soluble salts of potassium chloride or soluble compounds with Na (Quina et al., 2008; Zhang et al., 2016). The BA can contain both NaCl and KCl, which may be removed by the washing process (Ahn et al., 2006). Fe and Al dissolved with high DE (70%–100%) in all grain sizes of BA. The base elements, Zn and Cu, were highly extracted with DE (>85%), and Ba was extracted with high DE (>70%) from the majority of BA samples. The base elements (especially Cu) can occur in different oxidation states in BA (Rissler et al., 2020), bounded to aluminosilicates, carbonates, chlorides, oxides, hydroxides, and Ba possibly due to its frequent association with sulfates (Bayuseno and Schmahl, 2010).
A second group of elements showed moderate DE (30%–75%) (Table 2), including Ca (DE < 60%), likely due to the occurrence of calcite (CaCO3), anorthite (Ca(Al2Si2O8)), akermanite (Ca2Mg(Si2O7)), gehlenite (Ca2Al(AlSiO7)), vaterite (CaCO₃), portlandite (Ca(OH)2), and larnite (Ca2SiO4) in the original BA, which were previously identified by Mantovani et al. (2023), who studied XRD mineralogical analysis of BA obtained from the same incinerator plants (FE and FC). The elements (Co, Sr, Ti, As, Ni, Pb, and Cr) with moderate to high DE (30%–95%), are mainly associated with non-silicate minerals in combination with Fe and Zn or found in different metallic inclusions (Wei et al., 2011) and other glassy phases (Mantovani et al., 2021) in BA.
The last group of elements with low DE (<30%) included Mn, likely in spinels (Wei et al., 2011). In REE, Y had the highest DE (78%–100%). La and Ce showed moderate DE (38%–73%). Their low extractability suggests that they may have limited potential for circular economy, even if La and Ce are essential constituents for new and green technologies (Chakhmouradian and Wall, 2012). Overall, the DE of fine-sized BA (FEF and FCF) was higher compared to the other BA samples, following a trend of enrichment in fine > medium > coarse > bulk samples.
4 Conclusion
This study reported a characterization of MSWI-BA of two incinerator plants (FE and FC). Statistical methods were employed to interpret and highlight the current trends toward the recoverability of a range of metals. The total composition of the most valuable and potentially toxic elements showed moderate-to-high abundance levels, while REE showed low abundance compared to crustal values. The contents of some valuable elements, including REE, increased as the particle size decreased in all BA samples from the two MSWI plants studied. However, REE was at a low concentration compared to the crustal averages. The chondrite-normalized patterns of REE confirmed anthropogenic enrichments or fluctuation for some elements, especially heavy REE. The EF for selected elements, which are considered critical by the European Commission, showed a moderate-to-high enrichment in BA, suggesting its potential contribution in a circular economy through MSWI ash management. Most valuable elements, such as base and TCE, showed high extractability (DE > 80%) for FEC, FCC, FEM, FCM, FEF, and FCF, but not FEB and FCB, in both FE-BA and FC-BA, confirming that these elements were well dissolved by ARD. Bibliometric analysis supported the discussion, showing clues and research trends that are particularly helpful for decision-makers to identify future research branches for investments. With the recent breakthrough in analytical capacity and the increasing number of nature-based solutions tested on the bench scale, an area of fast development is identifiable in the hybridization of treatments with biological processes and in targeting metals characterized by conductive properties to allow magnetic purification. The findings of the present study show that the recovery of valuable elements could produce enough benefits to balance capital costs and environmental impacts.
Data availability statement
The raw data supporting the conclusions of this article will be made available by the authors, without undue reservation.
Author contributions
Conceptualization: VF; Methodology: JG, VF, ED, and ST; Writing–original draft preparation: JG; Writing–review and editing: JG, VF, ED, ST, Supervision: VF, ED. All authors contributed to the article and approved the submitted version.
Funding
VF acknowledges the administrative support of the National Research Council of Italy and the financial support from PNRR MUR project ECS_00000033_ECOSISTER.
Acknowledgments
The authors are grateful to Yago Nestola of CNR-ISMAR and the engineers (Valeria Dercole and Giovanni Lombardi) and staff of the FE and FC Incinerator plants for their help and support in collecting the MSWI residual ash. We also acknowledge the valuable suggestions of the reviewer who helped to improve the quality of this manuscript.
Conflict of interest
The authors declare that the research was conducted in the absence of any commercial or financial relationships that could be construed as a potential conflict of interest.
Publisher’s note
All claims expressed in this article are solely those of the authors and do not necessarily represent those of their affiliated organizations, or those of the publisher, the editors and the reviewers. Any product that may be evaluated in this article, or claim that may be made by its manufacturer, is not guaranteed or endorsed by the publisher.
Supplementary material
The Supplementary Material for this article can be found online at: https://www.frontiersin.org/articles/10.3389/fenvs.2023.1252313/full#supplementary-material
References
Abramov, S., He, J., Wimmer, D., Lemloh, M. L., Muehe, E. M., Gann, B., et al. (2018). Heavy metal mobility and valuable contents of processed municipal solid waste incineration residues from Southwestern Germany. Waste manage 79, 735–743. doi:10.1016/j.wasman.2018.08.010
Ahn, J. W., Um, N. I., Han, G. C., You, K. S., and Cho, H. C. (2006). Effect of carbonation in removal of chloride from municipal solid waste incineration bottom ash. Geosystem Eng. 9 (4), 87–90. doi:10.1080/12269328.2006.10541260
Allegrini, E., Maresca, A., Olsson, M. E., Holtze, M. S., Boldrin, A., and Astrup, T. F. J. W. M. (2014). Quantification of the resource recovery potential of municipal solid waste incineration bottom ashes. Waste manage 34, 1627–1636. doi:10.1016/j.wasman.2014.05.003
Allegrini, E., Vadenbo, C., Boldrin, A., and Astrup, T. F. (2015). Life cycle assessment of resource recovery from municipal solid waste incineration bottom ash. J. Environ. Manage. 151, 132–143. doi:10.1016/j.jenvman.2014.11.032
Astrup, T., Muntoni, A., Polettini, A., Pomi, R., Van Gerven, T., and Van Zomeren, A. (2016). Treatment and reuse of incineration bottom ash. Environ. Mater. Waste 2016, 607–645. doi:10.1016/b978-0-12-803837-6.00024-x
Bayuseno, A., and Schmahl, W. W. (2010). Understanding the chemical and mineralogical properties of the inorganic portion of MSWI bottom ash. Waste manage 30 (8-9), 1509–1520. doi:10.1016/j.wasman.2010.03.010
Berkhout, S. P., Oudenhoven, B. P., and Rem, P. C. (2011). Optimizing non-ferrous metal value from MSWI bottom ashes. J. Environ. Prot. 2 (05), 564–570. doi:10.4236/jep.2011.25065
Branchini, L., De Pascale, A., Falchetti, M., and Fiore, P. (2012). Advanced waste-to-energy steam cycles. Energy Procedia 45, 1205–1214. doi:10.1016/j.egypro.2014.01.126
Bunge, R. (2015). “Recovery of metals from waste incinerator bottom ash,” in Removal, treatment and utilisation of waste incineration bottom ash. Editors O. Holm, and E. Thome-Kozmiensky (Switzerland: UMTEC), 63–143.
Chakhmouradian, A. R., and Wall, F. (2012). Rare earth elements: minerals, mines, magnets (and more). Elements 8 (5), 333–340. doi:10.2113/gselements.8.5.333
Chen, L., Liao, Y., Ma, X., and Lu, S. (2020). Heavy metals chemical speciation and environmental risk of bottom slag during co-combustion of municipal solid waste and sewage sludge. J. Clean. Prod. 262, 121318. doi:10.1016/j.jclepro.2020.121318
Chimenos, J., Fernandez, A., Nadal, R., and Espiell, F. (2000). Short-term natural weathering of MSWI bottom ash. J. Hazard. Mat. 79 (3), 287–299. doi:10.1016/s0304-3894(00)00270-3
Chimenos, J., Segarra, M., Fernández, M., and Espiell, F. J. (1999). Characterization of the bottom ash in municipal solid waste incinerator. J. Hazard. Mat. 64 (3), 211–222. doi:10.1016/s0304-3894(98)00246-5
European Commission (2020). Critical raw materials resilience: charting a path towards greater security and sustainability. Brussels, Germany: EESC.
Fabricius, A. L., Renner, M., Voss, M., Funk, M., Perfoll, A., Gehring, F., et al. (2020). Municipal waste incineration fly ashes: from a multi-element approach to market potential evaluation. Environ. Sci. Eur. 32 (1), 88–14. doi:10.1186/s12302-020-00365-y
Fujimori, E., Minamoto, K., Iwata, S., Chiba, K., and Haraguchi, H. J. (2004). Enrichment of elements in industrial waste incineration bottom ashes obtained from three different types of incinerators, as studied by ICP-AES and ICP-MS. J. Mat. Cycles Waste Manag. 6, 73–79. doi:10.1007/s10163-003-0106-6
Funari, V. (2022). Sustainability assessment of bioleaching for mineral resource recovery from MSWI ashes. Circular Econ. Sustain. 2, 419–445. doi:10.1016/b978-0-12-821664-4.00023-6
Funari, V., Bokhari, S. N. H., Vigliotti, L., Meisel, T., and Braga, R. (2016). The rare earth elements in municipal solid waste incinerators ash and promising tools for their prospecting. J. Hazard. Mat. 301, 471–479. doi:10.1016/j.jhazmat.2015.09.015
Funari, V., Braga, R., Bokhari, S. N. H., Dinelli, E., and Meisel, T. (2015). Solid residues from Italian municipal solid waste incinerators: a source for “critical” raw materials. Waste Manage 45, 206–216. doi:10.1016/j.wasman.2014.11.005
Funari, V., Toller, S., Vitale, L., Santos, R. M., and Gomes, H. I. (2023). Urban mining of municipal solid waste incineration (MSWI) residues with emphasis on bioleaching technologies: a critical review. Environ. Sci. Pollut. Res. 30, 59128–59150. doi:10.1007/s11356-023-26790-z
Han, S., Ju, T., Meng, Y., Du, Y., Xiang, H., Aihemaiti, A., et al. (2021). Evaluation of various microwave-assisted acid digestion procedures for the determination of major and heavy metal elements in municipal solid waste incineration fly ash. J. Clean. Prod. 321, 128922. doi:10.1016/j.jclepro.2021.128922
He, P. J., Zhang, H., Zhang, C. G., and Lee, D. J. (2004). Characteristics of air pollution control residues of MSW incineration plant in Shanghai. J. Hazard. Mat. 116 (3), 229–237. doi:10.1016/j.jhazmat.2004.09.009
Heiri, O., Lotter, A. F., and Lemcke, G. J. (2001). Loss on ignition as a method for estimating organic and carbonate content in sediments: reproducibility and comparability of results. J. Paleolimnol. 25, 101–110. doi:10.1023/a:1008119611481
Hong, K. J., Tokunaga, S., and Kajiuchi, T. (2000). Extraction of heavy metals from MSW incinerator fly ashes by chelating agents. J. Hazard. Mat. 75 (1), 57–73. doi:10.1016/s0304-3894(00)00171-0
Hyks, J., and Astrup, T. (2009). Influence of operational conditions, waste input and ageing on contaminant leaching from waste incinerator bottom ash: a full-scale study. Chemosphere 76 (9), 1178–1184. doi:10.1016/j.chemosphere.2009.06.040
Joseph, B., James, J., Kalarikkal, N., and Thomas, S. (2021). Recycling of medical plastics. Adv. Ind. Eng. Polym. 4 (3), 199–208. doi:10.1016/j.aiepr.2021.06.003
Lanzerstorfer, C. (2017). Grate-fired biomass combustion plants using forest residues as fuel: enrichment factors for components in the fly ash. Waste biomass valor 8 (1), 235–240. doi:10.1007/s12649-016-9565-6
Li, H., Sun, J., Gui, H., Xia, D., and Wang, Y. (2022). Physiochemical properties, heavy metal leaching characteristics and reutilization evaluations of solid ashes from municipal solid waste incinerator plants. Waste Manage 138, 49–58. doi:10.1016/j.wasman.2021.11.035
Li, M., Xiang, J., Hu, S., Sun, L. S., Su, S., Li, P. S., et al. (2004). Characterization of solid residues from municipal solid waste incinerator. Fuel 83 (10), 1397–1405. doi:10.1016/j.fuel.2004.01.005
Loginova, E., Volkov, D., Van De Wouw, P., Florea, M., and Brouwers, H. (2019). Detailed characterization of particle size fractions of municipal solid waste incineration bottom ash. J. Clean. Prod. 207, 866–874. doi:10.1016/j.jclepro.2018.10.022
Malinauskaite, J., Jouhara, H., Czajczyńska, D., Stanchev, P., Katsou, E., Rostkowski, P., et al. (2017). Municipal solid waste management and waste-to-energy in the context of a circular economy and energy recycling in Europe. Energy 141, 2013–2044. doi:10.1016/j.energy.2017.11.128
Mantovani, L., De Matteis, C., Tribaudino, M., Boschetti, T., Funari, V., Dinelli, E., et al. (2023). Grain size and mineralogical constraints on leaching in the bottom ashes from municipal solid waste incineration: a comparison of five plants in northern Italy. Front. Environ. Sci. 11, 587. doi:10.3389/fenvs.2023.1179272
Mantovani, L., Tribaudino, M., Matteis, C. D., and Funari, V. J. S. (2021). Particle size and potential toxic element speciation in municipal solid waste incineration (MSWI) bottom ash. Sustainability 13 (4), 1911. doi:10.3390/su13041911
McDonough, W. F., and Sun, S. S. (1995). The composition of the Earth. Chem. Geol. 120 (3-4), 223–253. doi:10.1016/0009-2541(94)00140-4
Morf, L. S., Gloor, R., Haag, O., Haupt, M., Skutan, S., Di Lorenzo, F., et al. (2013). Precious metals and rare earth elements in municipal solid waste–sources and fate in a Swiss incineration plant. Waste manage 33 (3), 634–644. doi:10.1016/j.wasman.2012.09.010
Mostafa Hatami, A., Sabour, M., and Nikravan, M. (2022). A bibliometric analysis on incineration ash during 2000 to 2020. Amirkabir J. Civ. Eng. 54 (8), 3025–3040. doi:10.22060/ceej.2022.20084.7339
Muchova, L., Bakker, E., and Rem, P. (2009). Precious metals in municipal solid waste incineration bottom ash. Water Air Soil Pollut. Focus 9 (1), 107–116. doi:10.1007/s11267-008-9191-9
Nikravan, M., Ramezanianpour, A. A., and Maknoon, R. (2020). Study on physiochemical properties and leaching behavior of residual ash fractions from a municipal solid waste incinerator (MSWI) plant. J. Environ. Manage. 260, 110042. doi:10.1016/j.jenvman.2019.110042
Pan, Y., Wu, Z., Zhou, J., Zhao, J., Ruan, X., Liu, J., et al. (2013). Chemical characteristics and risk assessment of typical municipal solid waste incineration (MSWI) fly ash in China. J. Hazard. Mat. 261, 269–276. doi:10.1016/j.jhazmat.2013.07.038
Quina, M. J., Bordado, J. C., and Quinta-Ferreira, R. M. (2008). Treatment and use of air pollution control residues from MSW incineration: an overview. Waste Manage 28 (11), 2097–2121. doi:10.1016/j.wasman.2007.08.030
Rabiet, M., Brissaud, F., Seidel, J., Pistre, S., and Elbaz-Poulichet, F. (2009). Positive gadolinium anomalies in wastewater treatment plant effluents and aquatic environment in the Hérault watershed (South France). Chemosphere 75 (8), 1057–1064. doi:10.1016/j.chemosphere.2009.01.036
Ranjbari, M., Saidani, M., Esfandabadi, Z. S., Peng, W., Lam, S. S., Aghbashlo, M., et al. (2021). Two decades of research on waste management in the circular economy: insights from bibliometric, text mining, and content analyses. J. Clean. Prod. 314, 128009. doi:10.1016/j.jclepro.2021.128009
Rissler, J., Klementiev, K., Dahl, J., Steenari, B. M., and Edo, M. (2020). Identification and quantification of chemical forms of Cu and Zn in MSWI ashes using XANES. Energ. Fuel 34 (11), 14505–14514. doi:10.1021/acs.energyfuels.0c02226
Rudnick, R., Gao, S., Holland, H., and Turekian, K. (2014). Composition of the continental crust. From: treatise on geochemistry. 2nd Edn, Editor K. K. Turekian, and H. D. Holland (New York, NY: Elsevier Inc.), Chap. 2, Vol. 4, 1–51. doi:10.1016/b978-0-08-095975-7.00301-6
Shukla, A. K., Janmaijaya, M., Abraham, A., and Muhuri, P. K. (2019). Engineering applications of artificial intelligence: a bibliometric analysis of 30 years (1988–2018). Eng. Appl. Artif. Intell. 85, 517–532. doi:10.1016/j.engappai.2019.06.010
Šyc, M., Krausová, A., Kameníková, P., Šomplák, R., Pavlas, M., Zach, B., et al. (2018). Material analysis of Bottom ash from waste-to-energy plants. Waste Manage 73, 360–366. doi:10.1016/j.wasman.2017.10.045
Šyc, M., Simon, F. G., Hykš, J., Braga, R., Biganzoli, L., Costa, G., et al. (2020). Metal recovery from incineration bottom ash: state-of-the-art and recent developments. J. Hazard. Mat. 393, 122433. doi:10.1016/j.jhazmat.2020.122433
Toller, S., Funari, V., Zannoni, D., Vasumini, I., and Dinelli, E. (2022). Sediment quality of the Ridracoli fresh water reservoir in Italy: insights from aqua regia digestion and sequential extractions. Sci. Total Environ. 826, 154167. doi:10.1016/j.scitotenv.2022.154167
Tsai, F. M., Bui, T. D., Tseng, M. L., Lim, M. K., and Hu, J. (2020). Municipal solid waste management in a circular economy: a data-driven bibliometric analysis. J. Clean. Prod. 275, 124132. doi:10.1016/j.jclepro.2020.124132
van Eck, N. J., Waltman, L., van den Berg, J., and Kaymak, U. J. I. C. I. M. (2006). Visualizing the computational intelligence field [Application Notes]. IEEE Comput. Intell. Mag. 1 (4), 6–10. doi:10.1109/mci.2006.329702
Verplanck, P. L., Taylor, H. E., Nordstrom, D. K., and Barber, L. B. (2005). Aqueous stability of gadolinium in surface waters receiving sewage treatment plant effluent, Boulder Creek, Colorado. Environ. Sci. Technol. 39 (18), 6923–6929. doi:10.1021/es048456u
Wang, P., Hu, Y., and Cheng, H. (2019). Municipal solid waste (MSW) incineration fly ash as an important source of heavy metal pollution in China. Environ. Pollut. 252, 461–475. doi:10.1016/j.envpol.2019.04.082
Wang, Y., Lai, N., Zuo, J., Chen, G., and Du, H. (2016). Characteristics and trends of research on waste-to-energy incineration: a bibliometric analysis, 1999–2015. Renew. Sust. Energy Rev. 66, 95–104. doi:10.1016/j.rser.2016.07.006
Wei, Y., Shimaoka, T., Saffarzadeh, A., and Takahashi, F. J. (2011). Mineralogical characterization of municipal solid waste incineration bottom ash with an emphasis on heavy metal-bearing phases. J. Hazard. Mat. 187 (1-3), 534–543. doi:10.1016/j.jhazmat.2011.01.070
Weibel, G., Eggenberger, U., Schlumberger, S., and Mäder, U. K. (2017). Chemical associations and mobilization of heavy metals in fly ash from municipal solid waste incineration. Waste manage 62, 147–159. doi:10.1016/j.wasman.2016.12.004
Westerhoff, P., Lee, S., Yang, Y., Gordon, G. W., Hristovski, K., Halden, R. U., et al. (2015). Characterization, recovery opportunities, and valuation of metals in municipal sludges from US wastewater treatment plants nationwide. Environ. Sci. Technol. 49 (16), 9479–9488. doi:10.1021/es505329q
Wong, S., Mah, A. X. Y., Nordin, A. H., Nyakuma, B. B., Ngadi, N., Mat, R., et al. (2020). Emerging trends in municipal solid waste incineration ashes research: a bibliometric analysis from 1994 to 2018. Environ. Sci. Pollut. Res. 27, 7757–7784. doi:10.1007/s11356-020-07933-y
Xiang, J., Qiu, J., Li, Z., Chen, J., and Song, Y. (2022). Eco-friendly treatment for MSWI bottom ash applied to supplementary cementing: mechanical properties and heavy metal leaching concentration evaluation. Constr. Build. Mat. 327, 127012. doi:10.1016/j.conbuildmat.2022.127012
Yao, J., Kong, Q., Zhu, H., Long, Y., and Shen, D. (2013). Content and fractionation of Cu, Zn and Cd in size fractionated municipal solid waste incineration bottom ash. Ecotoxicol. Environ. Saf. 94, 131–137. doi:10.1016/j.ecoenv.2013.05.014
Yao, J., Li, W. B., Tang, M., Fang, C. R., Feng, H. J., and Shen, D. S. (2010). Effect of weathering treatment on the fractionation and leaching behavior of copper in municipal solid waste incinerator bottom ash. Chemosphere 81 (5), 571–576. doi:10.1016/j.chemosphere.2010.08.038
Yao, J., Qiu, Z., Kong, Q., Chen, L., Zhu, H., Long, Y., et al. (2017). Migration of Cu, Zn and Cr through municipal solid waste incinerator bottom ash layer in the simulated landfill. Ecol. Eng. 102, 577–582. doi:10.1016/j.ecoleng.2017.02.063
Zeller, V., Towa, E., Degrez, M., and Achten, W. M. (2019). Urban waste flows and their potential for a circular economy model at city-region level. Waste manage 83, 83–94. doi:10.1016/j.wasman.2018.10.034
Zhang, Y., Cetin, B., Likos, W., and Edil, T. (2016). Impacts of pH on leaching potential of elements from MSW incineration fly ash. Fuel 184, 815–825. doi:10.1016/j.fuel.2016.07.089
Zhao, C., Lin, S., Zhao, Y., Lin, K., Tian, L., Xie, M., et al. (2022). Comprehensive understanding the transition behaviors and mechanisms of chlorine and metal ions in municipal solid waste incineration fly ash during thermal treatment. Sci. Total Environ. 807, 150731. doi:10.1016/j.scitotenv.2021.150731
Keywords: combustion residues, aqua regia digestion, circular economy, environmental impact, metal recovery
Citation: Ghani J, Toller S, Dinelli E and Funari V (2023) Impact and recoverability of metals from waste: a case study on bottom ash from municipal solid waste incineration plants. Front. Environ. Sci. 11:1252313. doi: 10.3389/fenvs.2023.1252313
Received: 03 July 2023; Accepted: 30 October 2023;
Published: 24 November 2023.
Edited by:
Oladele Ogunseitan, University of California, Irvine, United StatesReviewed by:
Min-Hao Yuan, China Medical University, TaiwanPiero Lattanzi, National Research Council (CNR), Italy
Copyright © 2023 Ghani, Toller, Dinelli and Funari. This is an open-access article distributed under the terms of the Creative Commons Attribution License (CC BY). The use, distribution or reproduction in other forums is permitted, provided the original author(s) and the copyright owner(s) are credited and that the original publication in this journal is cited, in accordance with accepted academic practice. No use, distribution or reproduction is permitted which does not comply with these terms.
*Correspondence: Valerio Funari, valerio.funari@bo.ismar.cnr.it