- 1School of Engineering and Built Environment, Griffith University, Brisbane, QLD, Australia
- 2Department of Fisheries and Marine Science, Noakhali Science and Technology University, Noakhali, Bangladesh
- 3Analytical Chemistry Laboratory, Chemistry Division, Atomic Energy Centre Dhaka, Bangladesh Atomic Energy Commission, Dhaka, Bangladesh
- 4Materials Science Division, Atomic Energy Centre Dhaka, Bangladesh Atomic Energy Commission, Dhaka, Bangladesh
- 5Department of Zoology, College of Science, King Saud University, Riyadh, Saudi Arabia
- 6Environmental and Life Sciences Programme, Faculty of Science, Universiti Brunei Darussalam, Gadong, Brunei Darussalam
Microplastics (MPs), an emerging pollutant, have drawn attention on a global scale and have been found in various ecosystems. Nevertheless, there is currently a lack of information regarding the contamination levels of MPs in aquaculture ponds worldwide. In this study, sediment and water samples were collected from five types of fish ponds, namely, homestead ponds (S1), commercial aquaculture ponds (S2), ponds near a residential area (S3), ponds near a small-scale industrial area (S4), and ponds near a large-scale industrial area (S5), to identify, characterize, and assess the contamination risk of MPs. Stereomicroscopic and Fourier-transform infrared (FTIR) spectroscopy analyses revealed that the MPs ranged from 3.33 item/kg to 136.67 item/kg in sediment and 16.6 item/L to 100 item/L in water samples. Overall, the abundance of MPs was extremely high in S5, followed by S4, S2, S3, and S1, which clearly showed the levels increased with the intensity of human activities. The levels of MPs in both sediment and water showed significant differences (p < 0.05) within and between ponds, as well as in comparison between water and sediment samples. The concentrations of MPs surpassed those noted in aquaculture ponds of different nations. The majority of MPs consisted of fragments and films in both sediment and water samples. Furthermore, transparent and white-colored MPs were the prevailing types found in the sediment and water samples of the aquaculture ponds. Around 34% of MPs present in sediment and 30% in water fell within the size range of 0.5–1 mm. Polymers like polypropylene (PP), polyethylene (PE), and polyethylene terephthalate (PET) were prevalent in both sediment and water samples. The contamination factor reached exceptionally elevated levels (>30 for sediment and >3 for water), signifying that the sampled regions, particularly S4 and S5 (industrial sites), displayed significant MP contamination. Moreover, the pollution load index values of the sediment (3.0 ± 1.5) and water samples (1.5 ± 0.3) also indicated the areas were contaminated with MPs. Multivariate analysis indicated that the elevated concentration of MPs in the studied region could be attributed to the discharge of effluents and other human-induced activities.
1 Introduction
Plastics have enhanced the quality of life for millions of people throughout the world. Approximately 390.7 million tons of plastic products were generated globally in 2021, of which 4.8 and 12.7 million tons were discharged into aquatic environments each year (PEMRG, 2022). Due to the effects of wave-induced mechanical abrasion, photochemical oxidation, and biological processes, larger plastic items undergo fragmentation into smaller microscopic fragments (<5 mm in size), commonly denoted as “microplastics” (MPs) (Cole et al., 2011; Rilling, 2012; Corcoran et al., 2015; Banik et al., 2022). The buoyant nature, durability, widespread presence, resistance to weather, and chemicals are attributes that cause plastics to float at the water surface within aquatic ecosystems, where they tend to persist for extended periods (Geyer et al., 2017). MPs can be divided into two categories. Virgin pellets of pharmaceutical, cosmetic, or personal care items (glitters and microbeads) and cleaning products in the plastics industry are the primary types of MPs (Hossain et al., 2023a). Through processes of degradation or fragmentation, secondary MPs are created from bigger plastic debris (Barnes et al., 2009; Andrady, 2011). As MPs are hardly removed from polluted environments, launching a proper and an efficient waste management system to diminish the risk of MP pollution on aquatic ecosystems and human food web (Anderson et al., 2016; Estahbanati and Fahrenfeld, 2016; Banik et al., 2022) is necessary.
MPs are frequently recorded from three major ecosystems, i.e., marine, freshwater, and terrestrial ecosystems (Horton A. A. et al., 2017; Di and Wang, 2018; Karthik et al., 2018; Tang et al., 2018; Zhang and Liu, 2018; Zhu et al., 2018; Hossain et al., 2023b). However, it is approximated that the research addressing MP pollution in freshwater ecosystems is only a fraction constituting less than 4% of the attention given to marine environments (Klein et al., 2018; Lambert and Wagner, 2018). Roughly, 80% of MPs originate from terrestrial origins and find their way into freshwater habitats through activities such as laundering synthetic fabrics, usage of personal care items, tourism, urban development, and industrial operations (Browne et al., 2010; Browne et al., 2011). Agricultural runoffs, storm water or surface runoff, hand washing of fleece and shirts, and careless handling of covered landfills and plastic wastes can cause plastic waste to enter freshwater bodies (Mohapatra et al., 2016; Andrady, 2017; Ng et al., 2018; Atugoda et al., 2020). Wastewater treatment discharge, agricultural runoff, and sewage with sludge are three potential pathways of MPs entering into freshwater ecosystems (Eriksen et al., 2013). The infrastructure of a wastewater treatment plant (WWTP) is not designed to remove MPs fully from the discharge effluents, and these are discharged into aquatic systems directly or indirectly (Gatidou et al., 2019). Although the direct adverse impacts of microplastics on animals, fish, or humans remain unconfirmed, the extensive surface area and pronounced hydrophobic characteristics of MPs make them effective carriers of certain toxic elements including heavy metals, POPs (Van Cauwenberghe and Janssen, 2014; Ma et al., 2019; Hossain et al., 2023b). It is evident that these harmful substances are dangerous to aquatic life and humans in both the short and long term (Suja et al., 2009; Pramanik et al., 2015).
Aquaculture is one of the fastest growing industries in the world including Bangladesh. Bangladesh has gained fifth position in world aquaculture production and contributes 3.50% to the national GDP (Department of Fisheries, 2020). Almost every house in the suburban and village areas has a pond for fish culture, and the total area of ponds in Bangladesh is 397,775 ha, which contributes to 45.04% of whole fish production across the country (Department of Fisheries, 2020). The primary water sources used for culturing fish in ponds encompass rivers, streams, haors, baors, canals, and both small and large canals. These water bodies have been noted to transport plastic waste and MPs from diverse sources. Consequently, MPs show potential to amass within the water and sediment of aquaculture ponds, subsequently becoming incorporated into the bodies of fish. Thus, there is an imperative need to investigate the occurrence of MPs and to grasp their potential ramifications on freshwater aquaculture ponds, along with the associated health risks they might pose to both humans and the organisms residing within these environments. Limited research exists regarding MP contamination in aquaculture ponds worldwide (Bordós et al., 2019; Wang et al., 2020), and there has been no investigation conducted on MP occurrence, specifically in Bangladesh. In the context of Bangladesh, a country heavily reliant on aquaculture for sustenance and economic prosperity, the investigation of MPs in aquaculture ponds stands as a novel endeavor of paramount significance. Addressing this knowledge gap not only contributes to the broader understanding of MPs’ ecological impact but also sheds light on potential implications for food security and human health. Therefore, this pioneering study aims to identify, characterize, and assess the contamination risk of MPs in the sediments and water from five different types of ponds (homestead pond, commercial aquaculture, residential, and small- and large-scale industrial area ponds) from Noakhali coast, Bangladesh. The findings will offer a foundation for informed management strategies and future research directions tailored to this unique aquatic context.
2 Materials and methods
2.1 Study site
The study region Noakhali, located in the southeastern region of Bangladesh, is one of the coastal districts. It is bordered by the expansive estuarine systems of the Meghna estuary and the Bay of Bengal to its southern side. The geographical characteristics of this district comprise a vast, flat, coastal, and fertile deltaic terrain, forming the tidal floodplain of the Meghna River delta (Figure 1). The coastline rests approximately 9 m above the average sea level and is enveloped by alluvial plain on three sides, which receives annual inundation and nourishment from sediment deposits originating from the Meghna estuary. The typical annual temperature stands at 25.6°C, with an average annual rainfall of 2,980 mm. The strong currents flowing from the Himalayas bring not only river erosion but also a valuable load of fertile sediment. Upon reaching the Bay of Bengal, these sediments settle along the coastline, forming new land formations, locally known as “Char.” The climate of Noakhali is classified as tropical according to the Köppen–Geiger classification system due to its short dry season and frequent substantial rainfall. These climatic and geographical attributes have rendered the Noakhali region favorable for fish farming. Noakhali stands out as a prominent coastal district in Bangladesh due to its numerous homesteads and culture-based ponds, spanning an area of 1,068 ha and yielding a fish production of 1,438 metric tons (FRSS, 2018; Sarker et al., 2020). Furthermore, an industrial zone was established in 2006 under the Bangladesh Small and Cottage Industries Corporation (BSCIC) in Sadar Upazila, Noakhali. This industrial area generates significant quantities of chemical and plastic wastes. These waste materials are disposed directly or indirectly into nearby freshwater reservoirs, primarily ponds.
2.2 Sample collections
A total of 30 (15 sediment and 15 water) samples were collected from five different types of ponds (S1 to S5) in the post monsoon season from October to December in 2020 (Figure 1), each having triplicates. These five different kinds of ponds received surface runoff from catchments with various land uses, household garbage, and road dust. From these, one served as homestead ponds (S1), one was used for commercial culture purposes (S2), one served as residential areas (S3), and two served as both small- and large-scale industrial areas (S4 and S5). Every pond was around 10–15 years old and has a minimum amount of water (around 1m depth) all year round. Different kinds of plastic goods such as bucket, jug, plate, plastic pouches, refrigerator wraps, dishwashers, dishes, glasses, electronics and electrical equipment, irons, and tea or coffee makers may be the potential sources of MPs in both homestead and residential areas. Plastic coatings used in several aqua-industries (e.g., fertilizer, feed, aqua-drugs, transportation, and other chemicals) can also be contributed in the production of MPs. According to Wang J. et al. (2017), MP concentration can be reduced with the distance from urban areas. However, the land-use type can be influenced by the MP concentration, but it is difficult to narrate (Xu et al., 2021). There are a wide range of land-use classification, which may be the potential MP sources (e.g., a textile factory, paint industries, and other cosmetics industries), and this condition can be integrated and overlapped (Liu et al., 2008).
Sediment samples (top 10 cm) at 1 m water depth were collected using a Van Veen bottom grab sampler (10 × 10 cm) and were taken into an aluminum bag (Liu et al., 2019a). Sediment accumulation of each pond is assumed to be 5–7 years. The water samples were collected by filtering 60 L of surface water at a water depth of at least 0.5 m through a 330-µm mesh-sized manta net with cod-end. All water samples were performed in glass storage bottles with teflon-coated (polytetrafluoroethylene, PTFE) screw caps, and 5 mL of formalin was added to preserve each sample (Lusher et al., 2015; Zhang et al., 2017; Olesen et al., 2019).
2.3 Isolation procedure of MPs
With certain adjustments, the MP isolation procedure in this investigation was carried out in accordance with the approaches described by Banik et al. (2022) and Hossain et al. (2021). The water samples were subjected to drying in a hot air oven at a temperature of 90°C until complete dryness, along with 400 g of wet sediment samples from the bed. For 1 h, 400 mL of potassium metaphosphate (KO3P, Loba Chemie, India) was used to disaggregate all sediment samples (Masura et al., 2015). The dried sediment samples were then subjected to primary density separation using a salt solution of ZnCl2 (1.8 g cm-3) (Coppock et al., 2017). To remove native MPs from the samples, they were filtered using a cellulose nitrate filter paper of 5.0 µm before being added to all solutions, such as ZnCl2, FeSO4, and NaCl (Loba Chemie, India). By adding 20 mL of 30% H2O2 (Scharlab, Spain) and FeSO4 (0.05 M) solution, all the organic matter in the samples was eliminated. After that, the samples were moved to a secondary density separator and maintained overnight (Coppock et al., 2017). A cellulose nitrate filter paper (5.0 µm) (Minipore, India) with a 47-mm diameter was used to filter the supernatant from the separator (Bonello et al., 2018).
2.4 Morphological analysis and polymer identification of MPs
The MPs were identified and quantified from the filter paper using a stereomicroscope (Leica EZ4E, Germany) with ×8–×35 magnification. In order to count the MPs for these, the filter paper was divided into four sections, each of which pointed clearly toward the top (Lots et al., 2017). Using ImageJ software (version 2.0.0) and a high-resolution camera (DP software) mounted to the microscope, measurements of MPs were carried out (Hossain et al., 2021). A hot needle test was also performed for any suspicious plastic particles (Banik et al., 2022). The categorization of MP particles in terms of their types, shapes, colors, and sizes adhered to the existing literature guidelines (Hidalgo-Ruz et al., 2012; Frias and Nash, 2019; Hossain et al., 2021). Subsequently, larger-sized MP particles were separated from the filter papers to identify their chemical composition (polymer type). The specific details of the Fourier-transform infrared (FTIR) analysis can be found in Banik et al. (2022).
2.5 Control of contamination
To ensure control over contamination, rigorous precautions were taken during the entire experiment. Handling the H2O2-mixed solution necessitated special care due to its toxicity. Thus, the entire procedure was conducted within a fume hood. Measures were also implemented to prevent cross-contamination, especially with synthetic fibers from clothing and airborne pollutants. Thorough cleaning procedures, involving distilled water, followed by 70% alcohol, were consistently applied to the tools and work surfaces. For analyzing blank samples, the entire protocol from Banik et al. (2022) was followed. The MP size range investigated in this study spanned from 0.3 mm to 5 mm as the samples were sieved through a mesh with a 0.3-mm aperture (Hossain et al., 2021).
2.6 Contamination factor and pollution load index
The pollutant load index (PLI) and contamination factors (CFs) are used to assess the level of pollution in natural ecosystems (Tomlinson et al., 1980). The CF readings were divided into four groups, with CF < 1 denoting a low contamination level, 1–3 denoting a moderate contamination level, 3–6 denoting significant levels of contamination, and CF > 6 denoting extremely high contamination levels (Mmolawa et al., 2011; Rakib et al., 2022). On the contrary, the sampling area is contemplated to be polluted when PLI >1 (Tomlinson et al., 1980). However, the assessment model was defined as follows (Ranjani et al., 2021):
where
2.7 Statistical analysis
All mathematical calculations were performed in Microsoft Excel 2016 and PAST (Paleontological Statistics; Version 4.03). Moreover, SPSS 23 was used to conduct Kolmogorov–Smirnov and Shapiro–Wilk tests to test for normality of data distribution. In addition, Levene’s test was employed using SPSS 23 to identify the homogeneity of the variance of datasets with a significant level of 0.05. PAST software was employed to conduct one-way analysis of variance (ANOVA) and multivariate analysis, i.e., principal component analysis (PCA), which was used for determining the interconnection among the variables and their provable sources. Other univariate and multivariate analyses, such as Pearson correlation and cluster analysis, were conducted using Origin Pro 2021. Cluster analysis is used to reveal the frequency and variance of key determinants of the dataset (Wang et al., 2014). Moreover, all graphical components were extracted using Origin pro 2021 and GraphPad Prism 9. For the spatial distribution of MPs in sampling sites, QGIS 2.18 was used.
3 Results and discussion
3.1 Abundance and distribution of MPs in selected ponds’ sediment and water
The presence of MPs in sediment and water samples across the five ponds is illustrated in Figures 1, 2, respectively. Within the sediment, the levels of MPs ranged from 3.33 items/kg to 137 items/kg, with the highest concentration (102 ± 32 items/kg) recorded in S5. The mean sediment MP concentration exhibited a descending order as follows: S5 > S4 > S2 > S3 > S1. In contrast, the water sample exhibited a range of MP concentration varying from 16.67 items/L to 100 items/L, with the elevated concentration (61.11 ± 41.94 items/L) found in S5. The mean concentration of MPs in water samples followed the descending order of S5 > S4 > S2 > S3 > S1. Overall, the highest levels of MPs were sorted from the ponds located near a large industrial area (S5) and the lowest from the ponds near a small house (S1) (Figures 1, 2). The levels varied based on the intensities of human activities and settlement. The result of ANOVA showed that the concentration of the MPs in sediment and water samples was significantly different (p < 0.05). The adopted Kolmogorov–Smirnov and Shapiro–Wilk tests revealed that data variables followed the normal distributed pattern. However, many studies suggested different implications regarding uneven MP distribution, possibly due to hydraulic characteristics, the amount of municipal waste, and the surrounding environment (Islam and Chowdhury, 2014; Auta et al., 2017; Horton A. A. et al., 2017).
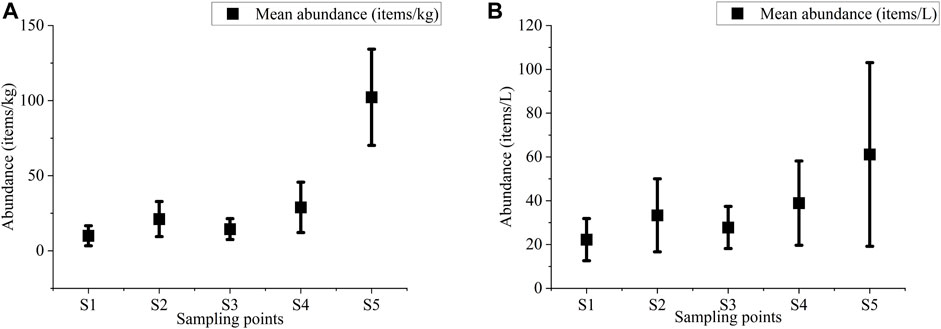
FIGURE 2. Microplastic abundance in water of five selective pond sediment samples (A) and in water (B).
Furthermore, inadequate and unplanned infrastructure can contribute to the elevated prevalence of MPs in open environment (Rakib et al., 2022). For instance, the absence of effective treatment methods for such effluents has led to a surge in municipal waste, escalating from 538 tons per day in 1999 to 1,890 tons per day in 2009 (Chowdhury et al., 2013).
There are only few reports available on MPs in pond ecosystems from around the world to compare our data (Table 1). Olesen et al. (2019) and Scopetani et al. (2019) documented a higher number of MPs than this study in the sediment samples of ponds. In contrast, Bordós et al. (2019) and Mercy et al. (2022) recorded a lower range of MPs than the present outcomes in the sediment of freshwater ponds. In addition, Olesen et al. (2019) recorded a higher concentration of MPs in water than the present study in stormwater retention ponds from Denmark and aquaculture ponds from Jakarta Bay (Priscilla and Patria, 2020). On the other hand, some studies documented a lower concentration of MPs than the current findings in fish ponds of the Carpathian basin and Changzhou (Bordós et al., 2019; Wang et al., 2020). Nonetheless, ponds typically collect rain-triggered surface runoff from the surrounding regions. As a result, the sediments and water samples within ponds function as reservoirs with a strong capacity for retaining MPs (Liu et al., 2019b; Campanale et al., 2022). Olesen et al. (2019) estimated the MP retention efficiency of ponds was 85%, indicating the function of pond’s habitat as a sink of MPs. Hence, MPs in the ponds needed more attention as these are ingested by aquatic organisms (Xu et al., 2020; Wootton et al., 2021; Zhang et al., 2021; Yagi et al., 2022). Regardless, further investigations regarding MP pollution in the ponds are essential, which certainly might be a source or path of MPs in the human body and terrestrial environments in future.
3.2 Morphological characteristics of MPs in sediment and water
In sediment, the fragments constituted the most prevalent form of MPs, accounting for 45% of the total identified MPs with an average concentration of 17.6 ± 22 items/kg at each sampling point. This was followed by 37.5% films, 15.1% fibers, 1.5% microbeads, and 0.9% foams (Figure 1; Figure 3A). The highest quantities of fragments (55.6 ± 22 items/kg), microbeads (7.78 ± 5.1 items/kg), and foams (4.4 ± 5.1 items/kg) were observed in the S5 sampling sites. However, no microbeads or foams were detected in other sediment samples. Conversely, MPs in pond water were predominantly composed of 50.2% films, with an average concentration of 17.8 ± 7.2 items/L at each sampling point. This was followed by 24% fibers, 12% fragments, 9.8% foams, and 4% microbeads (Figure 1; Figure 3B). The highest counts of fibers (16.7 items/L), fragments, and foams (11 ± 19 items/L) were documented in the S5 site.
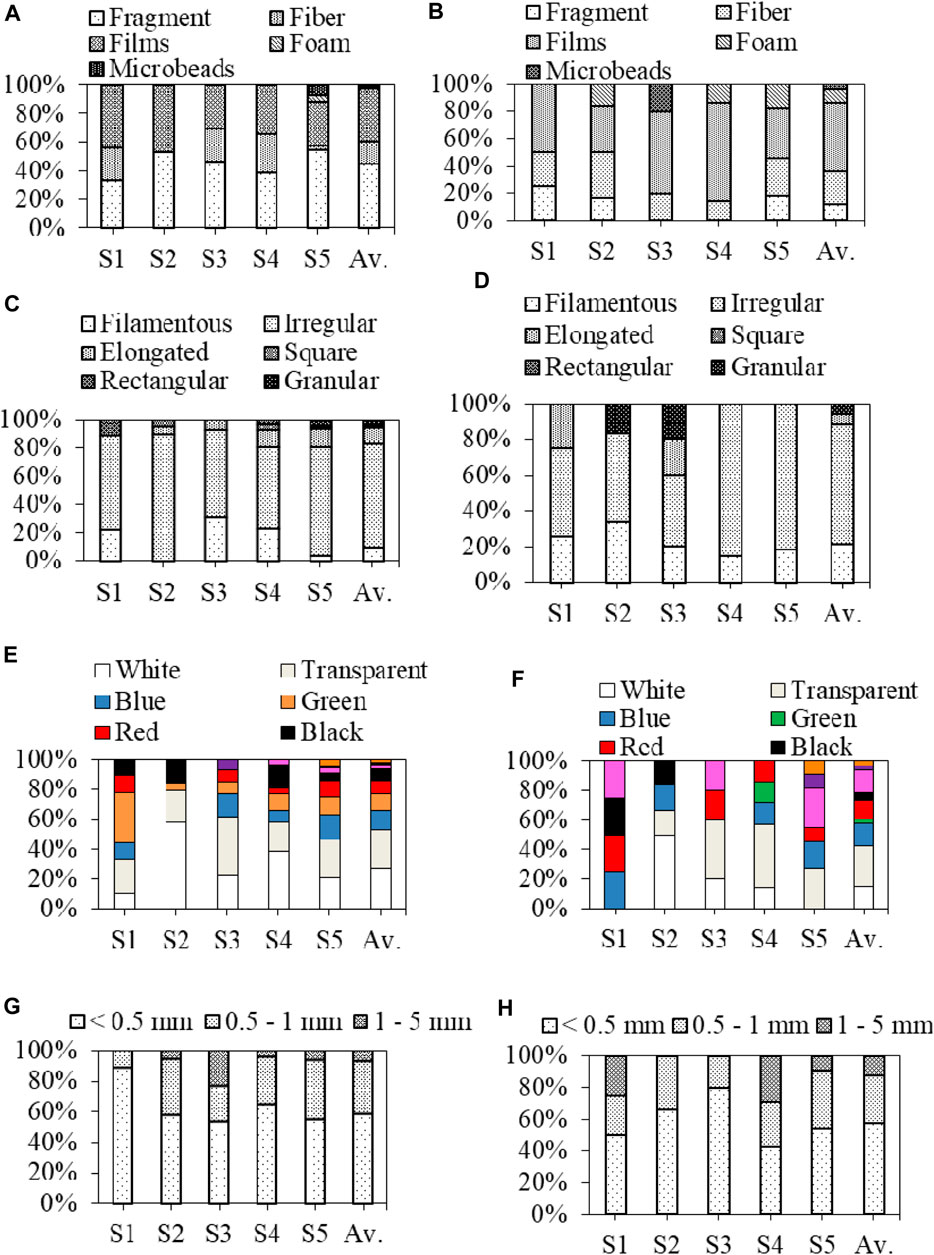
FIGURE 3. Morphological characteristics of MPs; types of MPs in sediment (A) and water (B); shapes of MPs in sediment (C) and water (D); color of MPs in sediment (E) and water (F); and size of MPs in sediment (G) and water (H). Here, S1–S5 denote the studied ponds, and Av. means the average values of all ponds.
The majority of MPs in both sediment and water samples displayed irregular shapes, contributing to 73.6% and 66.7% of the total MPs, respectively (Figures 3C, D). However, the fragments discovered in the pond sediment are likely to originate from plastic containers, bottles, and other household items that have undergone degradation due to environmental factors such as sunlight, wind, and water movement (Baldwin et al., 2016; Cesa et al., 2017; Huang et al., 2017; Wang et al., 2018). On the other hand, the prevalence of films and fibers in pond water samples could be attributed to the breakdown of packaging materials and the discharge of laundry effluents (De Falco et al., 2017). Additionally, the diverse array of MP types and shapes found in the pond near industrial area suggested a substantial impact of industrialization processes on the presence of MPs in the surrounding catchment areas (Hitchcock and Mitrovic, 2019). The distribution of MP colors in both sediment and water samples of different stations can be observed in Figures 3E, F. In the sediment of the pond, colored MPs constituted the majority (74.8%), while transparent MPs accounted for only 25.2%. The sequence of colors for MPs in sediment followed a descending order of white > blue > green > red > black > pink > orange > yellow > violet. A similar pattern was observed in water, with colored MPs making up 72.7% of the total and the remaining 27.3% being transparent. The color hierarchy for MPs in water was as follows: white > blue > pink > red > black > green > violet > orange. The diverse range of MP colors indicates their varying sources of origin. Notably, certain colors like red and blue from films and fibers might lose their original hues upon entering an aquatic environment due to the prolonged exposure to sunlight (Martí et al., 2020; Gela and Aragaw, 2022).
The size distribution of MPs measured in both sediment and water samples from the studied area is shown in Figures 3G, H. The majority of MPs were below 0.5 mm in both sediment (59%) and water (58%). In the sediment, 34% of MPs fell within the size range of 0.5–1 mm, and the remaining 7% of MPs were within the size range of 1–5 mm. Similarly, 30% of MPs in water were categorized in the 0.5–1-mm size range, while 12% were in the 1–5-mm size range. This phenomenon was possibly a result of larger plastic items breaking down into smaller MP particles due to various forces such as chemical, physical, and microbial activities (Su et al., 2016; Wang J. et al., 2017). These findings align with many other international studies conducted in locations such as Lake Hovsgol, Mongolia (Free et al., 2014); the Laurentian Great Lakes, United States (Eriksen et al., 2013); Wuhan urban lakes, China (Wang W. et al., 2017); and the Three Gorges Reservoir, China (Di and Wang, 2018), where smaller-sized MPs were predominantly identified. However, further research concerning the types, colors, and size ranges of MPs is needed to achieve a more conclusive understanding of their quantities and fate within the ponds.
3.3 Polymer composition
A total of 21 MP samples were verified through FTIR analysis, 12 MPs from sediment samples, and 9 MPs from water samples. The polymer type of MPs varied between sediment and water samples. In the sediment samples, MPs were dominated by polypropylene (41.7%), followed by polyethylene (33.3%), polyethylene terephthalate (16.7%), and cellulose (8.3%) (Supplementary Figure S1). On the contrary, in the water samples, PE was the most abundant polymer type with 44.4% of all MPs. The other polymer types included PP (33.3%) and PET (22.2%). However, our findings were in accordance with the previous studies where PE, PP, and PET were identified from pond ecosystems (Liu et al., 2019a; Liu et al., 2019b; Bordós et al., 2019; Olesen et al., 2019; Hu et al., 2020; Campanale et al., 2022). Ponds perform an indispensable role in the fate of these widely used polymers in consequence of the rain-induced runoff transmissions from the surface (Wang et al., 2022).
3.4 Potential sources of MPs in ponds
Pearson correlation analysis was employed to examine the relationships among the variables (Table 2; Figure 4A). Our observations revealed a mix of both positive and negative strong significant correlations among these variables. Notably, there were several instances of positive strong significant correlations, including fragment—films (r = 0.992), fragment—foams (r = 0.988), fragment—microbeads (r = 0.988), films—foams (r = 0.968), films—microbeads (r = 0.968), and the most notable, a strong positive correlation between foam and microbeads (r = 1). No negative correlations were found among the variables. These relationships prompted efforts to discern the origin of the MPs.
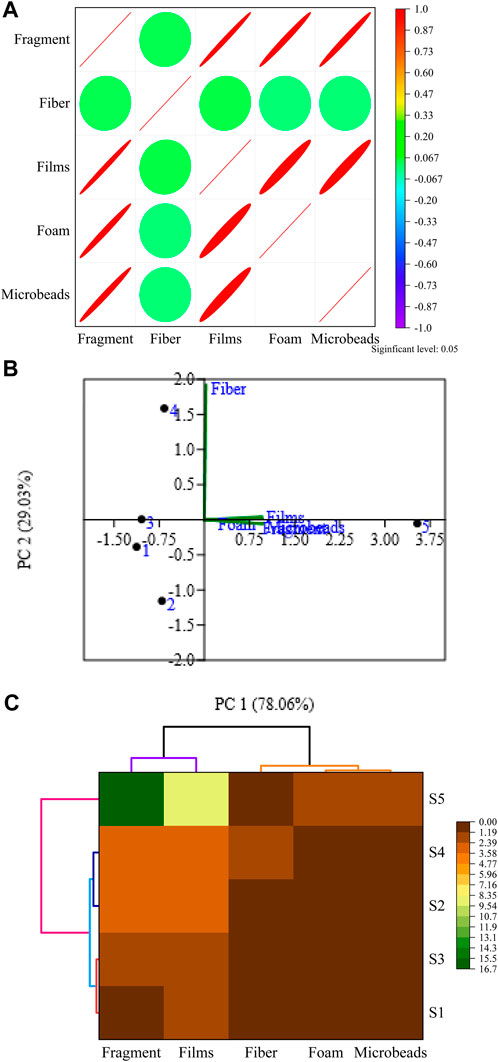
FIGURE 4. Correlation (A). Principal component analysis (B) of types of microplastics and two-way cluster analysis (C) among the types of microplastics and sites.
For source identification, principal component analysis (PCA) illuminates the relations among variables while considering the sampling areas (Hossain et al., 2023a). Additionally, PCA is commonly employed to qualitatively interpret the clustering tendencies and characteristics of potential features within the sampled materials. In our study, the application of PCA is particularly apt due to the availability of varying proportions of different plastic particles, which aids in obtaining a better comprehension (Rakib et al., 2022). Employing PCA helps us delve deeper into the loading values corresponding to the analyzed MPs as well. The resultant PCA outcomes, including eigenvalues and their corresponding loadings, are presented in both Table 2 and Figure 4B. The results of the PCA produced two associated factors, namely, PC 1 and PC 2, accounting for a cumulative variance of 99.09% Figure 4B. The primary component, PC 1, accounted for 78.06% of the variance, while PC 2 contributed 29.03% of the variance. Notably, substantial loadings were observed for fragment, foam, and microbeads, each contributing around 25% individually to the makeup of PC 1. Among them, fragments were the most dominant as the eigenvalue was greater than 1. In other words, PC 1 was greatly influenced by fragment particles. Thus, we can assume that particles like fragments, foam, and microbeads were available in our study area. On the other hand, significant loading was observed in PC 2 for fiber, representing 97% loading score. In other words, the fiber particle was the dominant factor in PC 2. Our PCA results denoted that the intrusion of MPs in the study area was from various anthropogenic activities such as unconscious fishing practices, household, industrial chemicals, wastage, urban discharges, and unplanned drainage systems. To identify the similar characteristics of MPs, it is obvious to conduct cluster analysis (CA).
One useful approach for illustrating a related set of variables along the sampling locationsvs. the experimental parameters in terms of unique variability is cluster analysis (Hossain et al., 2018). In this case, a similar collection of sites is shown in one cluster group, while a different set of sites was shown in another group to identify specific pollution hotspots (Sundaray et al., 2011). A two-way hierarchical cluster was generated in the current investigation using the ward-linkage approach and Euclidean distance (Figure 4C) When the distance (Dlink/Dmax) × 100 was less than 3, the dendrogram exhibited a distinct grouping in the vertical section, forming three identifiable clusters: clusters 1, 2, and 3. Cluster 1 included S1 and S3, while S2 and S4 were part of cluster 2, and S5 was associated with cluster 3. On the contrary, the horizontal site dendrogram followed the distance of (Dlink/Dmax) ×100 < 0.2 and represented similarly two clusters. Particles like fragments and films were in cluster 1, and fiber, foam, and microbeads were in cluster 2. The cluster classification varied significantly as the sampled sites in these clusters revealed similar features along with the anthropogenic sources. Moreover, discharge from industrial waste, the chemical used in agricultural lands, other anthropogenic actions, and municipal runoff were considered potential sources of these contents.
As reported by Julienne et al. (2019), over 90% of solid debris retains a size greater than 1 mm even after undergoing 25 units of water-induced weathering. Therefore, the aquaculture ponds need proper drainage facilities every 1 or 2 years. Another reason is that plastics discharged into the water might not have enough time to become smaller particles. The greater percentage of large particles in the study area indicated that there should be a specific fragmentation process. In addition, the large fraction of smaller particles implied that MPs would be weathered into smaller sizes.
There were two typical transport gateways of the attribution of MPs to the aquaculture ponds: 1) settling down in the bottom sediment of the ponds and 2) discharging MPs to the natural aquatic environment via different drainage systems (Xiong et al., 2021). In this concern, the aquaculture pond sediment is a crucial fate of MPs. Studies have suggested that high-nutrient contents might accelerate the MP sedimentation in different aquaculture ponds (Chen et al., 2019). Moreover, feed particles precipitating in the bottom sediment might accelerate the precipitation of MPs by co-precipitation and flocculation (Porter et al., 2018). In addition, large-scale ponds are susceptible to MP contamination due to their direct exposure to industrial effluents. These released MPs can be consumed by aquatic organisms, especially in cases where there is a substantial density of aquatic production, leading to the entrapment and eventual expulsion of MPs through feces (Katija et al., 2017). In instances of such densely populated aquatic production ponds, the sediment can be extracted through specialized drainage methods and utilized as enriched soil for agricultural applications. Thus, it can be considered one of the reverse processes compared with the knowledge of MP transportation from land to water (Siegfried et al., 2017). Although aquaculture ponds are one of the potential nutrient sources, MPs from the aquatic system can affect another natural water body that is close to the water body through a drainage system (Rico et al., 2013) because MPs in the natural water body can act as vectors of other pollutants like metals (Koelmans et al., 2016). In that case, ecological risk should be under concern in future studies.
3.5 Contamination level analysis in fish ponds
The CF values of the sediment and water samples of the studied areas followed the decreasing order of S5 > S4 > S2 > S3 > S1 (Figure 5). In case of sediment, the CF values of S5 (30.7 ± 9.6), S4 (8.7 ± 5.0), and S2 (6.3 ± 3.5) indicated that the sampling areas were highly contaminated. In contrast, the water of S5 (3.7 ± 2.5) had a considerable level of contamination, whereas the others were in a moderate contamination level. Moreover, the PLI values of sediment (3.0 ± 1.5) and water (1.5 ± 0.3) also indicated the aquaculture ponds were contaminated with MPs. Therefore, further research practices are recommended to recognize the fate of MPs and reduce the level of MP contamination in the freshwater ecosystems through proper waste disposal systems.
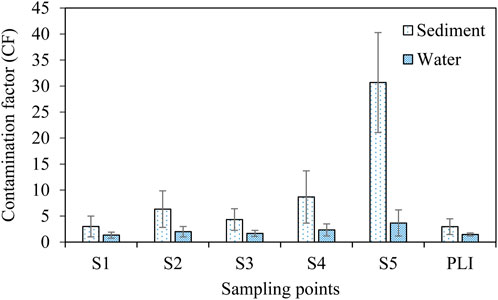
FIGURE 5. Contamination factor (CF) and the pollution load index (PLI) in the sediment and water samples from ponds.
4 Conclusion
This is the first study conducted to characterize and assess the contamination levels of MPs in different types of aquaculture ponds from Bangladesh. In the sediment samples, the lowest concentration (3.33 items/kg) of MPs was found in the homestead ponds (S1), while the highest concentration (102 items/kg) was observed in ponds located near a large-scale industrial area (S5). The concentration of MPs in water varied from 16.67 items/L to 100 items/L, with the highest levels detected in S5, reaching 61.11 items/L. Furthermore, a significant difference (p < 0.05) was observed in the abundance of MPs between water and sediment. The levels of MPs in this study contained a greater quantity of MPs compared to findings from other countries. The MPs identified displayed diverse characteristics, including the type, color, shape, and size. Predominantly, the particles consisted of fragments and films with transparent and white colors being prominent in water and sediment, respectively. Moreover, a significant portion of these particles exhibited irregular shapes with fragments and elongated forms being predominant. The size analysis revealed that the most common particles were within the range of 0.5–1 mm. Polymer characterization of MPs demonstrated that polypropylene (41.7%) and polyethylene (33.3%) were dominant in sediment, and PE was the most abundant (44.4%) in water. There was a strong significant correlation among the different types of MPs, indicating their same sources of origin. Values of contamination factor (>6) and pollution load index (>1) indicated that the aquaculture ponds, especially S4 and S5, were highly contaminated. Further studies are recommended to assess the ecological and human health risks due to MPs contamination.
Data availability statement
The original contributions presented in the study are included in the article/Supplementary Material. Further inquiries can be directed to the corresponding authors.
Author contributions
All authors listed have made a substantial, direct, and intellectual contribution to the work and approved it for publication.
Funding
This research was supported by the Researchers Supporting Project Number (RSP2023R436), King Saud University, Riyadh, Saudi Arabia. This study was also partially funded by Universiti Brunei Darussalam under the FOS Allied Fund (UBD/RSCH/1.4/FICBF(a)/2023).
Acknowledgments
The authors would like to acknowledge the support provided by the Researchers Supporting Project Number (RSP2023R436), King Saud University, Riyadh, Saudi Arabia.
Conflict of interest
The authors declare that the research was conducted in the absence of any commercial or financial relationships that could be construed as a potential conflict of interest.
Publisher’s note
All claims expressed in this article are solely those of the authors and do not necessarily represent those of their affiliated organizations, or those of the publisher, the editors, and the reviewers. Any product that may be evaluated in this article, or claim that may be made by its manufacturer, is not guaranteed or endorsed by the publisher.
Supplementary material
The Supplementary Material for this article can be found online at: https://www.frontiersin.org/articles/10.3389/fenvs.2023.1251158/full#supplementary-material
References
Anderson, J. C., Park, B. J., and Palace, V. P. (2016). Microplastics in aquatic environments: Implications for Canadian ecosystems. Environ. Pollut. 218, 269–280. doi:10.1016/j.envpol.2016.06.074
Andrady, A. L. (2011). Microplastics in the marine environment. Mar. Pollut. Bull. 62, 1596–1605. doi:10.1016/j.marpolbul.2011.05.030
Andrady, A. L. (2017). The plastic in microplastics: A review. Mar. Pollut. Bull. 119, 12–22. doi:10.1016/j.marpolbul.2017.01.082
Atugoda, T., Piyumali, H., Liyanage, S., Mahatantila, K., and Vithanage, M. (2020). Fate and behavior of microplastics in freshwater systems. Handb. Microplastics Environ., 1–31. doi:10.1007/978-3-030-10618-8_42-1
Auta, H. S., Emenike, C. U., and Fauziah, S. H. (2017). Distribution and importance of microplastics in the marine environment: A review of the sources, fate, effects, and potential solutions. Environ. Int. 102, 165–176. doi:10.1016/j.envint.2017.02.013
Baldwin, A. K., Corsi, S. R., and Mason, S. A. (2016). Plastic debris in 29 Great lakes tributaries: Relations to watershed attributes and hydrology. Environ. Sci. Technol. 50 (19), 10377–10385. doi:10.1021/acs.est.6b02917
Banik, P., Hossain, M. B., Nur, A. A. U., Choudhury, R. T., Liba, S. I., Yu, J., et al. (2022). Microplastics in sediment of Kuakata Beach, Bangladesh: Occurrence, spatial distribution, and risk assessment. Front. Mar. Sci. 9, 860989. doi:10.3389/fmars.2022.860989
Barnes, D. K., Galgani, F., Thompson, R. C., and Barlaz, M. (2009). Accumulation and fragmentation of plastic debris in global environments. Philos. Trans. R. Soc. B 364, 1985–1998. doi:10.1098/rstb.2008.0205
Bonello, G., Varrella, P., and Pane, L. (2018). First evaluation of microplastic content in benthic filter-feeders of the Gulf of La Spezia (Ligurian Sea). J. Aquat. Food Prod. Technol. 27 (3), 284–291. doi:10.1080/10498850.2018.1427820
Bordós, G., Urbányi, B., Micsinai, A., Kriszt, B., Palotai, Z., Szabó, I., et al. (2019). Identification of microplastics in fish ponds and natural freshwater environments of the Carpathian basin, Europe. Chemosphere 216, 110–116. doi:10.1016/j.chemosphere.2018.10.110
Browne, M. A., Crump, P., Niven, S. J., Teuten, E., Tonkin, A., Galloway, T., et al. (2011). Accumulation of microplastic on shorelines woldwide: Sources and sinks. Environ. Sci. Technol. 45, 9175–9179. doi:10.1021/es201811s
Browne, M. A., Galloway, T. S., and Thompson, R. C. (2010). Spatial patterns of plastic debris along estuarine shorelines. Environ. Sci. Technol. 44, 3404–3409. doi:10.1021/es903784e
Campanale, C., Galafassi, S., Savino, I., Massarelli, C., Ancona, V., Volta, P., et al. (2022). Microplastics pollution in the terrestrial environments: Poorly known diffuse sources and implications for plants. Sci. Total Environ. 805, 150431. doi:10.1016/j.scitotenv.2021.150431
Cesa, F. S., Turra, A., and Baruque-Ramos, J. (2017). Synthetic fibers as microplastics in the marine environment: A review from textile perspective with a focus on domestic washings. Sci. total Environ. 598, 1116–1129. doi:10.1016/j.scitotenv.2017.04.172
Chen, X., Xiong, X., Jiang, X., Shi, H., and Wu, C. (2019). Sinking of floating plastic debris caused by biofilm development in a freshwater lake. Chemosphere 222, 856–864. doi:10.1016/j.chemosphere.2019.02.015
Chowdhury, R. B., Sujauddin, M., Murakami, S., Chakraborty, P., and Alam, M. S. U. (2013). Current status of municipal solid waste management system in Chittagong, Bangladesh. Int. J. Environ. Waste Manag. 12 (2), 167–188. doi:10.1504/ijewm.2013.055592
Cole, M., Lindeque, P., Halsband, C., and Galloway, T. S. (2011). Microplastics as contaminants in the marine environment: A review. Mar. Pollut. Bull. 62, 2588–2597. doi:10.1016/j.marpolbul.2011.09.025
Coppock, R. L., Cole, M., Lindeque, P. K., Queirós, A. M., and Galloway, T. S. (2017). A small-scale, portable method for extracting microplastics from marine sediments. Environ. Pollut. 230, 829–837. doi:10.1016/j.envpol.2017.07.017
Corcoran, P. L., Norris, T., Ceccanese, T., Walzak, M. J., Helm, P. A., and Marvin, C. H. (2015). Hidden plastics of Lake Ontario, Canada and their potential preservation in the sediment record. Environ. Pollut. 204, 17–25. doi:10.1016/j.envpol.2015.04.009
De Falco, F., Gullo, M. P., Gentile, G., Di Pace, E., Cocca, M., Gelabert, L., et al. (2018). Evaluation of microplastic release caused by textile washing processes of synthetic fabrics. Environ. Pol. 236, 916–925. doi:10.1016/j.envpol.2017.10.057
Department of Fisheries (Dof), (2020). Yearbook of Fisheries Statistics of Bangladesh. Dhaka, Bangladesh: Department of Fisheries.
Di, M., and Wang, J. (2018). Microplastics in surface waters and sediments of the three Gorges reservoir, China. Sci. Total Environ. 616, 1620–1627. doi:10.1016/j.scitotenv.2017.10.150
Eriksen, M., Mason, S., Wilson, S., Box, C., Zellers, A., Edwards, W., et al. (2013). Microplastic pollution in the surface waters of the laurentian Great lakes. Mar. Pollut. Bull. 77, 177–182. doi:10.1016/j.marpolbul.2013.10.007
Estahbanati, S., and Fahrenfeld, N. L. (2016). Influence of wastewater treatment plant discharges on microplastic concentrations in surface water. Chemosphere 162, 277–284. doi:10.1016/j.chemosphere.2016.07.083
Free, C. M., Jensen, O. P., Mason, S. A., Eriksen, M., Williamson, N. J., and Boldgiv, B. (2014). High-levels of microplastic pollution in a large, remote, mountain lake. Mar. Pollut. Bull. 85 (1), 156–163. doi:10.1016/j.marpolbul.2014.06.001
Frias, J. P. G. L., and Nash, R. (2019). Microplastics: Finding a consensus on the definition. Mar. Pollut. Bull. 138, 145–147. doi:10.1016/j.marpolbul.2018.11.022
FRSS (2018). “Yearbook of fisheries statistics of Bangladesh,” in Bangladesh: Fisheries resources survey system (Dhaka, Bangladesh: Department of Fisheries), 129.
Gatidou, G., Arvaniti, O. S., and Stasinakis, A. S. (2019). Review on the occurrence and fate of microplastics in sewage treatment plants. J. Hazard Mater 367, 504–512. doi:10.1016/j.jhazmat.2018.12.081
Gela, S. M., and Aragaw, T. A. (2022). Abundance and characterization of microplastics in main urban ditches across the Bahir Dar City, Ethiopia. Front. Mar. Sci. 10, 831417.
Geyer, R., Jambeck, J. R., and Law, K. L. (2017). Production, use, and fate of all plastics ever made. Sci. Adv. 3, e1700782. doi:10.1126/sciadv.1700782
Hidalgo-Ruz, V., Gutow, L., Thompson, R. C., and Thiel, M. (2012). Microplastics in the marine environment: A review of the methods used for identification and quantification. Environ. Sci. Technol. 46, 3060–3075. doi:10.1021/es2031505
Hitchcock, J. N., and Mitrovic, S. M. (2019). Microplastic pollution in estuaries across a gradient of human impact. Environ. Pol. 247, 457–466. doi:10.1016/j.envpol.2019.01.069
Horton, A. A., Svendsen, C., Williams, R. J., Spurgeon, D. J., and Lahive, E. (2017a). Large microplastic particles in sediments of tributaries of the River Thames, UK–Abundance, sources and methods for effective quantification. Mar. Pollut. Bull. 114 (1), 218–226. doi:10.1016/j.marpolbul.2016.09.004
Horton, A. A., Walton, A., Spurgeon, D. J., Lahive, E., and Svendsen, C. (2017b). Microplastics in freshwater and terrestrial environments: Evaluating the current understanding to identify the knowledge gaps and future research priorities. Sci. Total Environ. 586, 127–141. doi:10.1016/j.scitotenv.2017.01.190
Hossain, M. B., Ahmed, A. S. S., Sarker, M., and Islam, S. (2018). Human health risks of Hg, As, Mn, and Cr through consumption of fish, Ticto barb (Puntius ticto) from a tropical river, Bangladesh. Environ. Sci. Pollut. Res. 25 (31), 31727–31736. doi:10.1007/s11356-018-3158-9
Hossain, M. B., Banik, P., Nur, A. A. U., and Rahman, T. (2020). Abundance and characteristics of microplastics in sediments from the world's longest natural beach, Cox's Bazar, Bangladesh. Mar. Pollut. Bull. 163, 111956. doi:10.1016/j.marpolbul.2020.111956
Hossain, M. B., Yu, J., Nur, A. A. U., Banik, P., Jolly, Y. N., Al-Mamun, M., et al. (2023b). Microplastics in surface water from a mighty subtropical estuary: First observations on occurrence, characterization, and contamination assessment. Environ. Res. 226, 115594. doi:10.1016/j.envres.2023.115594
Hossain, M. B., Yu, J., Nur, A. A. U., Banik, P., Jolly, Y. N., Al-Mamun, M., et al. (2023a). Distribution, characterization and contamination risk assessment of microplastics in the sediment from the world's top sediment-laden estuary. J. Environ. Manag. 344, 118472. doi:10.1016/j.jenvman.2023.118472
Hu, L., He, D., and Shi, H. (2020). Microplastics in inland small waterbodies. Berlin, Germany: Springer, 93–110. doi:10.1007/698_2019_445
Huang, D., Hu, C., Zeng, G., Cheng, M., Xu, P., Gong, X., et al. (2017). Combination of Fenton processes and biotreatment for wastewater treatment and soil remediation. Sci. Total Environ. 574, 1599–1610. doi:10.1016/j.scitotenv.2016.08.199
Islam, M. K., and Chowdhury, S. (2014). Karnophuli River front development, chittagong, Bangladesh. Am. J. Eng. Res. 3 (11), 46–54.
Julienne, F., Delorme, N., and Lagarde, F. (2019). From macroplastics to microplastics: Role of water in the fragmentation of polyethylene. Chemosphere 236, 124409. doi:10.1016/j.chemosphere.2019.124409
Karthik, R., Robin, R. S., Purvaja, R., Ganguly, D., Anandavelu, I., Raghuraman, R., et al. (2018). Microplastics along the beaches of southeast coast of India. Sci. Total Environ. 645, 1388–1399. doi:10.1016/j.scitotenv.2018.07.242
Katija, K., Choy, C. A., Sherlock, R. E., Sherman, A. D., and Robison, B. H. (2017). From the surface to the seafloor: How giant larvaceans transport microplastics into the deep sea. Sci. Adv. 3 (8), e1700715. doi:10.1126/sciadv.1700715
Klein, S., Dimzon, I. K., Eubeler, J., and Knepper, T. P. (2018). “Analysis, occurrence, and degradation of microplastics in the aqueous environment,” in Freshwater microplastics: Emerging environmental contaminants? Editors M. Wagner, and S. Lambert (Heidelberg, Germany: Springer), 51–67.
Koelmans, A. A., Bakir, A., Burton, G. A., and Janssen, C. R. (2016). Microplastic as a vector for chemicals in the aquatic environment: Critical review and model-supported reinterpretation of empirical studies. Environ. Sci. Technol. 50 (7), 3315–3326. doi:10.1021/acs.est.5b06069
Lusher, A., Tirelli, V., O'Connor, L., and Officer, R. (2015). Microplastics in surface waters and sediments of the three Gorges reservoir, China. Sci. Total Environ. 616–617, 1620–1627. doi:10.1016/j.scitotenv.2017.10.150
Lambert, S., and Wagner, M. (2018). “Microplastics are contaminants of emerging concern in freshwater environments: An overview,” in Freshwater microplastics: Emerging environmental contaminants? Editors M. Wagner, and S. Lambert (Heidelberg, Germany: Springer), 1–23.
Li, R., Yu, L., Chai, M., Wu, H., and Zhu, X. (2020). The distribution, characteristics and ecological risks of microplastics in the mangroves of Southern China. Sci. Total Environ. 708, 135025. doi:10.1016/j.scitotenv.2019.135025
Liu, F., Olesen, K. B., Borregaard, A. R., and Vollertsen, J. (2019a). Microplastics in urban and highway stormwater retention ponds. Sci. Total Environ. 671, 992–1000. doi:10.1016/j.scitotenv.2019.03.416
Liu, F., Vianello, A., and Vollertsen, J. (2019b). Retention of microplastics in sediments of urban and highway stormwater retention ponds. Environ. Pollut. 255, 113335. doi:10.1016/j.envpol.2019.113335
Liu, W., Zhang, D., Chen, B., Zhang, Z. y., Zhang, S. q., Xin, W. g., et al. (2008). Characteristics of China’s town-level land use in rapid urbanization stage. ACTA Geogr. Sin. 46, 301–304.
Lots, F. A., Behrens, P., Vijver, M. G., Horton, A. A., and Bosker, T. (2017). A large-scale investigation of microplastic contamination: Abundance and characteristics of microplastics in European beach sediment. Mar. Pollut. Bull. 123, 219–226. doi:10.1016/j.marpolbul.2017.08.057
Ma, B., Xue, W., Hu, C., Liu, H., Qu, J., and Li, L. (2019). Characteristics of microplastic removal via coagulation and ultrafiltration during drinking water treatment. Chem. Eng. J. 359, 159–167. doi:10.1016/j.cej.2018.11.155
Martí, E., Martin, C., Galli, M., Echevarría, F., Duarte, C. M., and Cózar, A. (2020). The colors of the ocean plastics. Environ. Sci. Technol. 54 (11), 6594–6601. doi:10.1021/acs.est.9b06400
Masura, J., Baker, J. E., Foster, G. D., Arthur, C., and Herring, C. (2015). “Laboratory methods for the analysis of microplastics in the marine environment: Recommendations for quantifying synthetic particles in waters and sediments,” in NOAA technical memorandum NOS-ORandR-48 (Washington, D.C., United States: National Oceanic and Atmospheric Administration).
Mercy, F. T., Alam, A. R., and andAkbor, M. A. (2022). Abundance and characteristics of microplastics in major urban wetlands of Dhaka, Bangladesh. Heliyon 9.
Mmolawa, K. B., Likuku, A. S., and Gaboutloeloe, G. K. (2011). Assessment of heavy metal pollution in soils along major roadside areas in Botswana. Afr. J. Environ. Sci. Technol. 5 (3), 186–196.
Mohapatra, D. P., Cledón, M., Brar, S. K., and Surampalli, R. Y. (2016). Application of wastewater and biosolids in soil: Occurrence and fate of emerging contaminants. Water Air Soil Pollut. 227 (3), 77. doi:10.1007/s11270-016-2768-4
Ng, E. L., Lwanga, E. H., Eldridge, S. M., Johnston, P., Hu, H., Geissen, V., et al. (2018). An overview of microplastic and nanoplastic pollution in agroecosystems. Sci. Total Environ. 627, 1377–1388. doi:10.1016/j.scitotenv.2018.01.341
Olesen, K. B., Stephansen, D. A., van Alst, N., and Vollertsen, J. (2019). Microplastics in a stormwater pond. WaterSwitzerl. 11, 1466. doi:10.3390/w11071466
PEMRG (Plastics Europe Market Research Group) (2021). Plastics–the Facts 2020: An analysis of European plastics production, demand and recovery for 2020. Brussels, Belgium: Plastic Europe Association of Plastic Manufacturers.
Porter, A., Lyons, B. P., Galloway, T. S., and Lewis, C. (2018). Role of marine snows in microplastic fate and bioavailability. Environ. Sci. Technol. 52 (12), 7111–7119. doi:10.1021/acs.est.8b01000
Pramanik, B. K., Pramanik, S. K., and Suja, F. (2015). A comparative study of coagulation,granular-and powdered-activated carbon for the removal of perfluorooctanesulfonate and perfluorooctanoate in drinking water treatment. Environ. Technol. 36 (20), 2610–2617. doi:10.1080/09593330.2015.1040079
Priscilla, V., and Patria, M. P. (2020). Comparison of microplastic abundance in aquaculture ponds of milkfish chanoschanos (forsskål, 1775) at muara kamal and marunda, Jakarta Bay. IOP Conf. Ser. earth Environ. Sci. 404 (1), 012027. doi:10.1088/1755-1315/404/1/012027
Ranjani, M., Veerasingam, S., Venkatachalapathy, R., Mugilarasan, M., Bagaev, A., Mukhanov, V., et al. (2021). Assessment of potential ecological risk of microplastics in the coastal sediments of India: A meta-analysis. Mar. Pollut. Bull. 163, 111969. doi:10.1016/j.marpolbul.2021.111969
Rakib, M., Jahan, R., Hossain, M. B., Kumar, R., Ullah, M., Al Nahian, S., et al. (2022). Spatial distribution and risk assessments due to the microplastics pollution in sediments of Karnaphuli River Estuary, Bangladesh. Sci. Rep. 12 (1), 8581–8615. doi:10.1038/s41598-022-12296-0
Rico, A., Geng, Y., Focks, A., and Van den Brink, P. J. (2013). Modeling environmental and human health risks of veterinary medicinal products applied in pond aquaculture. Environ. Toxicol. Chem. 32 (5), 1196–1207. doi:10.1002/etc.2153
Rillig, M. C. (2012). Microplastic in terrestrial ecosystems and the soil. Environ. Sci. Technol. 46, 6453–6454. doi:10.1021/es302011r
Sarker, M. M., Hossain, M. B., Islam, M. M., Mustafa Kamal, A. H., and Idris, M. H. (2021). Unravelling the diversity and assemblage of phytoplankton in homestead ponds of central coastal belt, Bangladesh. Aquac. Res. 52 (1), 167–184. doi:10.1111/are.14878
Scopetani, C., Chelazzi, D., Cincinelli, A., and andEsterhuizen-Londt, M. (2019). Assessment of microplastic pollution: Occurrence and characterisation in vesijärvilake and PikkuVesijärvi pond, Finland. Environ. Monit. Assess. 191 (11), 652–717. doi:10.1007/s10661-019-7843-z
Siegfried, M., Koelmans, A. A., Besseling, E., and Kroeze, C. (2017). Export of microplastics from land to sea. A modelling approach. Water Res. 127, 249–257. doi:10.1016/j.watres.2017.10.011
Su, L., Xue, Y., Li, L., Yang, D., Kolandhasamy, P., Li, D., et al. (2016). Microplastics in taihu lake, China. Environ. Pollut. 216, 711–719. doi:10.1016/j.envpol.2016.06.036
Suja, F., Pramanik, B. K., and Zain, S. M. (2009). Contamination, bioaccumulation and toxiceffects of perfluorinated chemicals (PFCs) in the water environment: A reviewpaper. Water Sci. Technol. 60 (6), 1533–1544. doi:10.2166/wst.2009.504
Sundaray, S. K., Nayak, B. B., Lin, S., and Bhatta, D. (2011). Geochemical speciation and risk assessment of heavy metals in the river estuarine sediments—A case study: Mahanadi basin, India. J. Hazard. Mater. 186 (2-3), 1837–1846. doi:10.1016/j.jhazmat.2010.12.081
Tang, G., Liu, M., Zhou, Q., He, H., Chen, K., Zhang, H., et al. (2018). Microplastics and polycyclic aromatic hydrocarbons (PAHs) in xiamen coastal areas: Implications for anthropogenic impacts. Sci. Total Environ. 634, 811–820. doi:10.1016/j.scitotenv.2018.03.336
Tomlinson, D. L., Wilson, J. G., Harris, C. R., and Jeffrey, D. W. (1980). Problems in the assessment of heavy-metal levels in estuaries and the formation of a pollution index. Helgol. Meeresunters. 33 (1-4), 566–575. doi:10.1007/bf02414780
Van Cauwenberghe, L., and Janssen, C. R. (2014). Microplastics in bivalves cultured for human consumption. Environ. Pollut. 193, 65–70. doi:10.1016/j.envpol.2014.06.010
Wang, F., Wang, B., Duan, L., Zhang, Y., Zhou, Y., Sui, Q., et al. (2020). Occurrence and distribution of microplastics in domestic, industrial, agricultural and aquacultural wastewater sources: A case study in Changzhou, China. Water Res. 182, 115956. doi:10.1016/j.watres.2020.115956
Wang, J., Peng, J., Tan, Z., Gao, Y., Zhan, Z., Chen, Q., et al. (2017a). Microplastics in the surface sediments from the beijiang river littoral zone: Composition, abundance, surface textures and interaction with heavy metals. Chemosphere 171, 248–258. doi:10.1016/j.chemosphere.2016.12.074
Wang, W., Ndungu, A. W., Li, Z., and Wang, J. (2017b). Microplastics pollution in inland freshwaters of China: A case study in urban surface waters of wuhan, China. Sci. Total Environ. 575, 1369–1374. doi:10.1016/j.scitotenv.2016.09.213
Wang, W., Yuan, W., Chen, Y., and Wang, J. (2018). Microplastics in surface waters of dongting lake and hong lake, China. Sci. Total Environ. 633, 539–545. doi:10.1016/j.scitotenv.2018.03.211
Wang, Y. B., Liu, C. W., Liao, P. Y., and Lee, J. J. (2014). Spatial pattern assessment of river water quality: Implications of reducing the number of monitoring stations and chemical parameters. Environ. Monit. Assess. 186 (3), 1781–1792. doi:10.1007/s10661-013-3492-9
Wang, Z., Dou, M., Ren, P., Sun, B., Jia, R., and Zhou, Y. (2022). Sedimentation of irregular shaped microplastics under steady and dynamic flow conditions. https://www.researchsquare.com/article/rs-252593/v1.
Wootton, N., Ferreira, M., Reis-Santos, P., and Gillanders, B. M. (2021). A comparison of microplastic in fish from Australia and Fiji. Front. Mar. Sci. 8, 690991. doi:10.3389/fmars.2021.690991
Xiong, X., Liu, Q., Chen, X., Wang, R., Duan, M., and Wu, C. (2021). Occurrence of microplastic in the water of different types of aquaculture ponds in an important lakeside freshwater aquaculture area of China. Chemosphere 282, 131126. doi:10.1016/j.chemosphere.2021.131126
Xu, X., Wong, C. Y., Tam, N. F., Lo, H. S., and Cheung, S. G. (2020). Microplastics in invertebrates on soft shores in Hong Kong: Influence of habitat, taxa and feeding mode. Sci. Total Environ. 715, 136999. doi:10.1016/j.scitotenv.2020.136999
Xu, Y., Chan, F. K. S., Johnson, M., Stanton, T., He, J., Jia, T., et al. (2021). Microplastic pollution in Chinese urban rivers: The influence of urban factors. Res. Conserv. Recycl. 173, 105686. doi:10.1016/j.resconrec.2021.105686
Yagi, M., Kobayashi, T., Maruyama, Y., Hoshina, S., Masumi, S., Aizawa, I., et al. (2022). Microplastic pollution of commercial fishes from coastal and offshore waters in southwestern Japan. Mar. Pollut. Bull. 174, 113304. doi:10.1016/j.marpolbul.2021.113304
Zhang, G. S., and Liu, Y. F. (2018). The distribution of microplastics in soil aggregate fractions in southwestern China. Sci. Total Environ. 642, 12–20. doi:10.1016/j.scitotenv.2018.06.004
Zhang, T., Sun, Y., Song, K., Du, W., Huang, W., Gu, Z., et al. (2021). Microplastics in different tissues of wild crabs at three important fishing grounds in China. Chemosphere 271, 129479. doi:10.1016/j.chemosphere.2020.129479
Zhang, W., Zhang, S., Wang, J., Wang, Y., Mu, J., Wang, P., et al. (2017). Microplastic pollution in the surface waters of the Bohai Sea, China. Environ. Pollut. 231, 541–548. doi:10.1016/j.envpol.2017.08.058
Zhao, J., Ran, W., Teng, J., Liu, Y., Liu, H., Yin, X., et al. 2018. Microplastic pollution in sediments from the bohai sea and the yellow sea, China, Sci. Total Environ. 640–641, 637–645. doi:10.1016/j.scitotenv.2018.05.346
Keywords: microplastics, homestead ponds, aquaculture ponds, polymers, contamination assessment
Citation: Hossain MB, Banik P, Nur A-A, Choudhury TR, Liba SI, Albeshr MF, Yu J and Arai T (2023) Microplastics in fish culture ponds: abundance, characterization, and contamination risk assessment. Front. Environ. Sci. 11:1251158. doi: 10.3389/fenvs.2023.1251158
Received: 30 June 2023; Accepted: 23 August 2023;
Published: 14 September 2023.
Edited by:
Jing Ding, Harbin Institute of Technology, ChinaReviewed by:
Akan Williams, Covenant University, NigeriaYing Zhang, Shandong Normal University, China
Copyright © 2023 Hossain, Banik, Nur, Choudhury, Liba, Albeshr, Yu and Arai. This is an open-access article distributed under the terms of the Creative Commons Attribution License (CC BY). The use, distribution or reproduction in other forums is permitted, provided the original author(s) and the copyright owner(s) are credited and that the original publication in this journal is cited, in accordance with accepted academic practice. No use, distribution or reproduction is permitted which does not comply with these terms.
*Correspondence: M. Belal Hossain, belal.hossain@nstu.edu.bd; Takaomi Arai, takaomi.arai@ubd.edu.bn