- 1Institute of Veterinary Medicine, Federal University of Pará, Castanhal, Pará, Brazil
- 2Institute of Health and Animal Production, Federal Rural University of Amazon, Belém, Pará, Brazil
- 3Institute of Food and Agricultural Science, University of Florida, Gainsville, FL, United States
- 4Research and Development Department, Brazilian Agricultural Research Corporation, Rio Branco, Acre, Brazil
- 5Institute of Agrarian Science, Federal University of Jequitinhonha and Mucuri Valleys, Unaí, Minas Gerais, Brazil
- 6Department of Animal Science, São Paulo State University, São Paulo, São Paulo, Brazil
- 7Department of Animal Science, Federal University of Ceará, Fortaleza, Ceará, Brazil
Methane emissions (CH4) from the soil increase according to changes made in forest soils and adverse edaphoclimatic factors. Soil temperature and nutrients will impact the activity of microorganisms, depending on land use. The objective of this study was to evaluate the impacts of land use, temperature, and nitrogen application on CH4 emissions from soils within the Amazon region. Three experiments were conducted in a completely randomized design. Each experiment consisted of five replicates to measure CH4 emissions. The variables examined in these experiments were: 1) three distinct land uses (forest, pasture, or agriculture; 2) soil temperatures (25, 30, 35, or 40°C); and 3) input of nitrogen to the soil (0, 90, 180, or 270 kg of N ha−1). In this study, the highest emissions occurred in pasture soils, with values of 470 μg of CH4 g−1 of dry soil, while forest and agricultural soils suffer the effects of CH4 oxidation. Temperature is a factor that contributes to CH4 emissions, and temperatures above 30°C tended to reduce gas emissions in the systems studied, since the highest emission was observed in pasture soil kept at 25°C (∼1,130 μg of CH4 g−1 of dry soil). Nitrogen fertilization in pasture soils reduces CH4 emitted nearly 140% as the dose increased. As a result, the pasture soils tended to emit higher concentrations of CH4 into the atmosphere. However, reducing these emissions from the pasture management employed is possible.
1 Introduction
The Amazon rainforest is considered one of the most important reservoirs of biodiversity on Earth (Heckenberger et al., 2007), providing various ecosystem services such as water and nutrient cycling, climate regulation, and carbon storage (Carvalho et al., 2017; Córdoba et al., 2019). Several factors have contributed to the expansion of deforestation and land use change in the Amazon, such as the use of more areas for agricultural purposes, logging, mining, burning, and population growth in the region, which has been increasing since the 70s (Fearnside, 2018; Gay and Sánchez, 2019; Paiva et al., 2019; Souza Filho et al., 2020; Souza et al., 2021; Andrade et al., 2022; Silveira et al., 2022). Management, in which humans interfere with nature, causes several changes in the earth’s surface. As the changes intensify, it is necessary to pay greater attention to the environment. Furthermore, soils in the Amazon Biome are methane sinkholes because of their high moisture content (Zhao et al., 2019). However, the changes in land use that have occurred have converted these areas to sources of CH4 because of the impacts caused by the bacterial communities of the soil (Steudler et al., 1996; Fernandes et al., 2002; Meyer et al., 2017), which will alter nutrient cycling and, consequently, the emission of greenhouse gases, such as CH4 (Garcia-Montiel et al., 2001; Khan et al., 2019).
Soil temperature is a key variable that influences greenhouse gas emissions because it regulates growth and microbial activity in the soil and influences O2 availability, porosity, and soil water content (Dai et al., 2020; Cardoso et al., 2020). Moreover, previous studies in temperate regions found that the elevation of ambient temperature potentiates the emission of CH4 into the atmosphere because of the greater efficiency of methanotrophic microorganisms present in the soil (Liikanen et al., 2002; Zheng et al., 2018).
The presence of nitrogen in the soil has a direct effect on the emission of CH4 into the atmosphere because it accelerates the process of senescence and death of plant tissues and the tillering rate, thus increasing the litter layer present in the areas and the vegetation cover and accelerating the consumption of methanotrophic microorganisms (Fan et al., 2020; Cui, et al., 2022). However, the source of nitrogen fertilizer can promote high oscillations in the fluxes of CH4 soon after its application (Raposo et al., 2020), correlating mainly with the content of mineral nitrogen in the soil owing to the inhibitory effect of ammonium in the process of methanotrophy, which is responsible for the oxidation of CH4 in the soil (Corrêa et al., 2021).
The effect of land use changes on gas emissions in diverse ecosystems throughout South America has been the focus of several studies; however, studies in the Amazon region remain scarce. For example, Metay et al. (2007) and Siqueira Neto et al. (2020) studied CH4 emissions in Cerrado soils, Lourenço et al. (2022) performed analyses in the Caatinga, and Vasconcelos et al. (2018) conducted studies in Brazilian pampas, where all authors observed changes in soil methane emissions when changing land cover, from forest to pasture or agriculture. Physical and chemical differences in the soil can modify gas emissions in the Amazon biome, making it necessary to conduct regional studies to understand how emissions occur in different ecosystems to seek mitigation strategies for CH4 in these areas.
This study tested the hypotheses that a change from forest areas to pasture or agricultural areas increases the emission of CH4 into the atmosphere, and that the temperature or nitrogen increases changes the CH4 flux of the soil, with the aim of identifying the causes of the increase in gas emissions into the atmosphere and seeking mitigation strategies for the systems. This research objective was to characterize the CH4 emissions in different land uses (forest, pasture, or agriculture) subjected to elevated soil temperature or increased nitrogen in the soil.
2 Material and methods
2.1 Location and soil characteristics
To conduct the incubations, areas of similar soil characteristics were selected in the municipality of Nova Esperança do Piriá, Pará, Brazil (2°15′S, 46°58′W; altitude 73 m). The local climate is classified as a tropical monsoon (Am) type according to the Köppen classification, with a short dry season and heavy rains during the rest of the year (Alvares et al., 2013). In the year of the collect the total precipitation is 3,000 mm and mean temperature is 27.7°C, with maximum and minimum temperature of 33.8°C and 22.6°C, respectively. The soil was collected at a depth of 20 cm from three land-use systems (forest, pasture, and agriculture), approximately 2 km from each other. The soil characteristics are presented in Table 1. The incubations were conducted in the foraging laboratory at the State University of São Paulo, Jaboticabal, São Paulo, Brazil.
The systems (Figure 1) were chosen based on a diagnosis conducted in the municipality of Nova Esperança do Piriá, Pará, Brazil, because the region’s economy revolves around black pepper production. Pasture systems (2°19′34″S; 46°56′22″W) invest little in the system’s intensification. The native forest (2°19′14″S; 46°56′26″W) area represents a reference of the original ecosystem of the region. The pasture used for soil collection was formed in 2007 after burning with the implantation of Urochloa brizantha cv. Marandú. A swidden process is performed annually to remove invasive plants. The production system is extensive, with cattle production using a continuous stocking system at various stocking rates. The last fertilization was carried out in 2018 using natural reactive phosphate (90 kg P2O5 ha−1). The area corresponding to agriculture (2°19′38″S; 46°55′40″W) has cultivated black pepper (Piper nigrum), which was opened in 2012 through burning. The planting was 5 years old and in the third collection cycle. At the time of collection, the crop was in its fifth year of cultivation and received annual fertilization of 130 kg ha–1 of nitrogen, 80 kg ha–1 of phosphorus (P2O5), and 200 kg ha–1 of potassium (KCl).
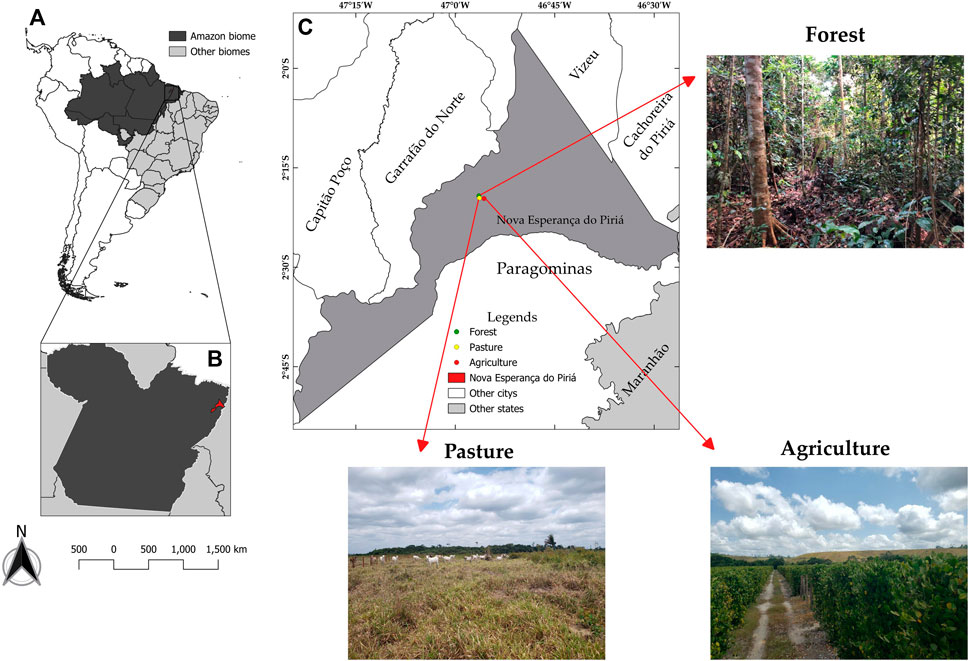
FIGURE 1. Location map of soils collection areas. (A) Representation of the delimitation of the Amazon biome. (B) Location of Nova Esperança do Piriá, PA. (C) Soils collection areas in Nova Esperança do Piriá, PA.
Soil samples were collected, air-dried for 72 h, homogenized, and mechanically macerated using a roller to break the clods.
2.2 Experimental design
Three incubations were carried out to determine the CH4 emissions and flux in each land-use system, as well as their interactions with temperature variations and soil nitrogen input.
a) Incubation 1: A completely randomized design consisting of three treatments corresponding to land use (forest, pasture, and agriculture) with five replicates, totaling 15 experimental units.
b) Incubation 2: An experiment was conducted in a 3 × 4 factorial scheme with a completely randomized design, with five replicates, totaling 60 experimental units. The first factor was land use (forest, pasture, and agriculture), and the second was the elevation in soil temperature (25, 30, 35, and 40°C). The temperature of 25°C is due to the average temperature of the region’s climate (Alvares et al., 2013).
c) Incubation 3: An experiment in a 3 × 4 factorial scheme was conducted out in a completely randomized design, with five replicates, totaling 60 experimental units. It was tested the effects of different land uses (forest, pasture, and agriculture) and nitrogen input into the soil (0, 9, 180, and 270 kg N ha-1).
2.3 Incubation of treatments
The study was conducted under controlled conditions: 100 g of dry soil (forest, pasture, or agriculture) was added to flask (500 mL), and the soil moisture was maintained at 33% of the water retention capacity in all soil systems. In incubations where the effect of temperature was not tested, 25.0°C ± 1.0°C was maintained. In incubation 1 and 2, 0.018 mg g−1 soil, corresponding to 50 kg of N ha−1, was added in each soil in the form of a liquid solution to stimulate the soil’s microbial population, wheres in incubation 3, the addition of nitrogen was an evaluating factor.
a) Soil temperature elevation treatment
Greenhouses with forced air circulation were maintained at a constant temperature corresponding to the defined values. The vials were kept at 25°C, 30°C, 35°C, and 40°C and withdrawn only for gas collection.
b) Soil nitrogen input treatment
Urea solutions with predefined nitrogen concentrations were prepared. To nitrogen concentrations of 90, 180, and 270 kg of N ha−1, 0.032, 0.064, and 0.096 mg g−1 soil, respectively, were added. For the treatment of 0 kg of N ha−1, only water was added to the vials to maintain the desired humidity.
2.4 CH4 measurement and soil analysis
To quantify the emissions of CH4, the technique of a closed static chamber consisting of 400 mL of free space was used. The sampling routine was between 9 a.m. and 10 a.m., as described by Cardoso et al. (2017). The flux of CH4 emissions was monitored for 25 days in incubation 1 and 28 days in incubations 2 and 3. Samples were collected every day during the first week and at 2-day until the end of the incubation period.
To measure CH4 production, bottle caps were sealed for 30 min and the change in free-space concentration was quantified. At the end of the incubation period (T30), air samples were collected using 50 mL polypropylene syringes. Additionally, ambient gas samples (T0) were collected from the five chambers before sealing to measure the initial CH4 concentration. The chambers used for the initial sample collection were rotated for each collection.
The gas samples were transferred to 20 mL pre-evacuated vials (Shimadzu vials) for quantitative analysis using gas chromatography (Shimadzu Green House Gas Analyzer GC-2014; Kyoto, Japan) to measure CH4. The analysis was performed under the following conditions: injector temperature of 250°C, column temperature of 80°C, N2 carrier gas flow rate of 30 mL min−1, and flame ionization detector (FID) temperature set at 280 °C.
The CH4 flux (μg CH4 m−2 h−1) was determined by calculating the change in concentration over the sampling period, assuming a linear increase in CH4 concentration. The calculations were performed using the following equations (Cardoso et al., 2019):
Where, P is the air pressure at the sampling site, R denotes the gas constant, T is the temperature inside the chamber, V is the volume of the chamber, A is the surface area of the sampling soil, and ΔC/Δt is the linear slope of the concentration change during the sampling period.
Daily flux data were plotted over time to generate cumulative emissions by applying a linear interpolation between sampling days. This approach allowed for data integration. To determine the CH4 flux attributed to the treatments, the cumulative emissions were subtracted from the ambient gas collected on the same day.
Soil mineral nitrogen content was assessed at the end of the incubation period by extracting it with 2 M KCl and conducting colorimetric analysis to determine the levels of ammonium (Forster, 1995) and nitrate (Doane and Horwath, 2003).
2.5 Statistical analysis
To verify the normality of the residuals and homogeneity of variances, the data were analyzed using the Shapiro-Wilk and Levene tests, respectively. When the presence of outliers was detected, they were excluded from the analysis. Analysis of variance (ANOVA) was performed for the three incubation tests. When ANOVA was significant for land use, the means were compared using Tukey’s test, considering p < 0.05 as a significant difference. When the temperature and nitrogen variations were significant; regression adjustment was performed to identify the effects of the treatments. All statistical analyses were performed using the statistical program R (version 4.0.2; R Core Team, 2014).
3 Results
3.1 Mineral N
The soil with agricultural use of 0 and 90 kg N ha−1 exhibited the lowest ammonium content (7.50 mg N-NH4 kg−1 of dry soil), whereas the forest treatment at 25°C showed the highest ammonium content (30.0 mg N-NH4 kg−1 dry soil). Conversely, the lowest nitrate content was observed in the forest treatment (0.30 mg N-NO3 kg−1 of dry soil), whereas the highest nitrate content was found in the pasture treatment at 180 kg N ha−1 (12.5 mg N-NO3 kg−1 of dry soil). Table 2 presents the results of soil mineral nitrogen analysis.
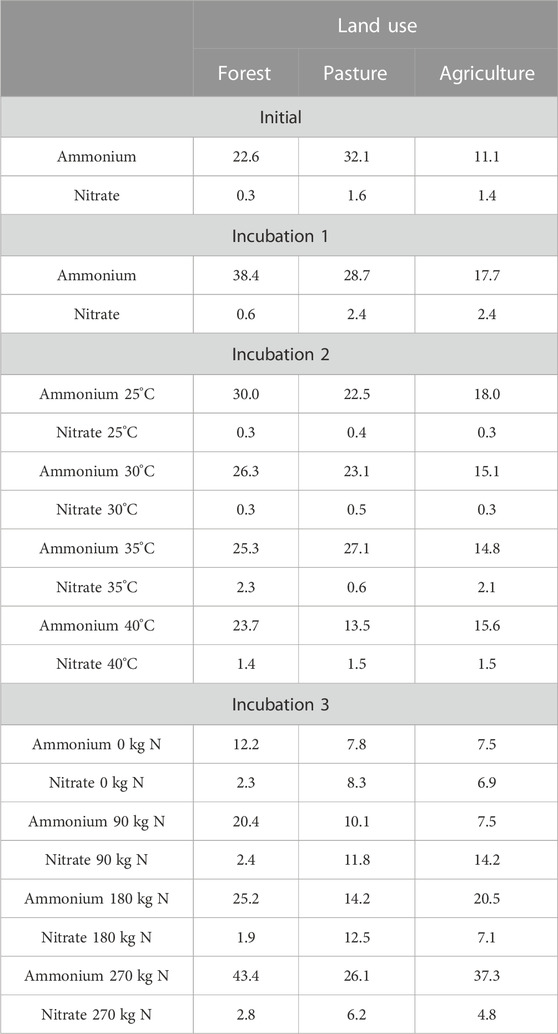
TABLE 2. Ammonium concentrations (mg N-NH4 kg−1 of dry soil) and nitrate (mg N-NO3 kg−1 of dry soil) in soils of different land uses submitted to temperature of incubations or nitrogen addition.
3.2 Effects of land use on CH4 emissions
When evaluating the effect of land use, higher total emissions of CH4 (p < 0.001) were observed in pasture soil (470 μg CH4 g−1 of dry soil; Figure 2) compared the soils of the other two systems. In contrast, there was a process of oxidation of CH4 in tropical rainforest soils (−303 μg CH4 g−1 of dry soil) and agriculture (−267 μg CH4 g−1 of dry soil), which did not differ (p > 0.05) between them. Therefore, these values are considered negative. Throughout the 25 days of incubation, the highest CH4 yields occurred between days 2 and 3 in the pasture soils, ranging from 263 to 275 ng CH4 g−1 of dry soil day−1. After this period, the emissions remained at zero until the end of the experimental period. CH4 emissions from forest soils and agricultural land resulted in an almost undetectable flux over the 25 days of incubation (Figure 3).
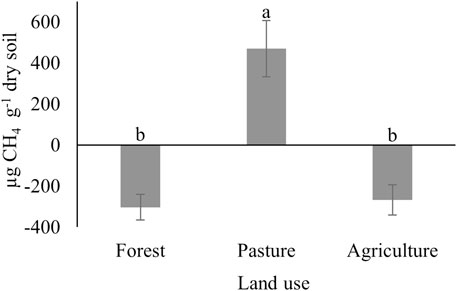
FIGURE 2. Total CH4 emissions (μg CH4 g−1 of dry soil day−1) in soils of different land use systems in the Brazilian Amazon. Different capital letters differ by Tukey’s test (p < 0.05).
3.3 Effects of incubation temperature on CH4 emissions
An interaction effect between the land-use system and temperature (p < 0.001) was observed. The forest and agriculture treatments presented the lowest (p < 0.001) emissions of CH4 among the land uses, except at the temperature of 40°C, where the lowest production was observed only in the agricultural soil (p > 0.05). In the pasture soil, a negative quadratic effect (p < 0.001) was observed in gas emissions as a function of temperature, where the lowest gas production was observed at temperatures of 35°C. Forest and agricultural soils did not vary (p > 0.05) in the emissions of CH4 with the increase in incubation temperature. Pasture soils showed the highest methane emissions, regardless of the temperature applied, while Forest and agricultural soils showed no statistical difference (p > 0.05; Table 3).
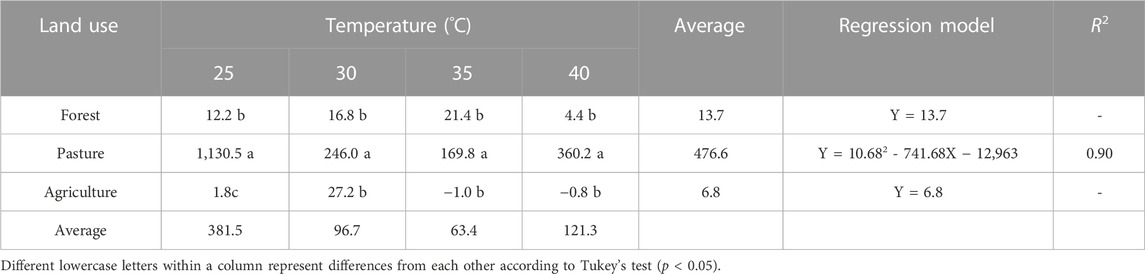
TABLE 3. Total CH4 emissions (μg CH4 g−1 of dry soil) in different land use systems, subjected to temperature levels.
Temperature variation altered the flux of CH4 in forest soils (Figure 4A). At temperatures of 30°C, 35°C, and 40°C, the flux began on the first day of incubation, whereas at 25°C, it began only on the third day. At each studied temperature, peak production occurred at different times. At 25°C, the peak was observed on the seventh day, producing ∼103 ng CH4 g−1 day−1. At 30°C, the peak was on day 21, with ∼109 ng CH4 g−1 day−1. At 35°C on the ninth day, with ∼62 ng CH4 g−1 day−1, and at 40°C, two peaks were observed on the ninth day (∼135 ng CH4 g−1 day−1) and 19th day (∼146 ng CH4 g−1 day−1). Production began on the first day in pasture soil (Figure 4B). However, a higher flux was observed at 25°C, with peak production reaching ∼12,160 ng CH4 g−1 day−1 on the 25th day of incubation. In the agricultural soil (Figure 4C), higher fluxes were observed at two temperatures of 25°C, with two production peaks on the seventh day (165 ng CH4 g−1 day−1) and on the 11th day (205 ng CH4 g−1 day−1) and at 40°C, a peak on the sixth day (148 ng CH4 g−1 day−1). The CH4 fluxes at 30°C and 35°C remained unchanged throughout the incubation period.
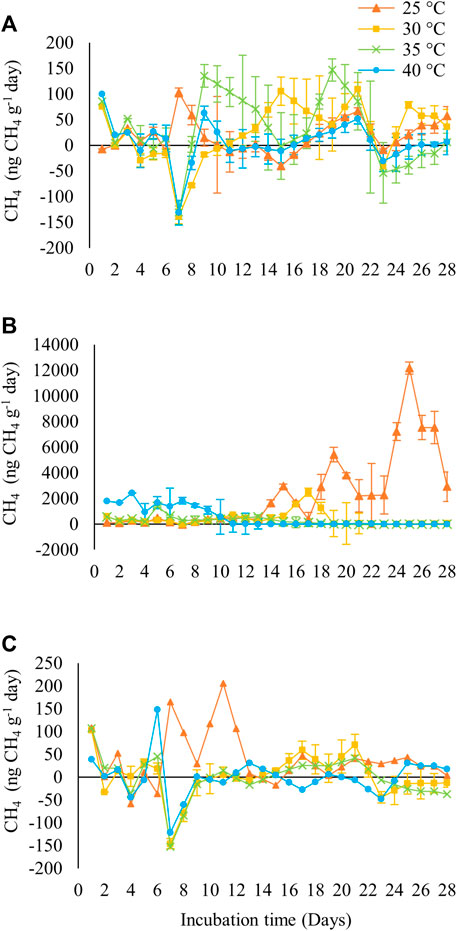
FIGURE 4. CH4 fluxes (ng CH4 g−1 of dry soil−1 day−1) in different land use systems of use of (A) forest; (B) pasture; (C) agriculture in the Brazilian Amazon, subjected to different soil incubation temperatures.
3.4 Effect of soil nitrogen addition on CH4 emissions
An interaction (p < 0.001) between land use and soil nitrogen on the total emission of CH4 was observed (Table 4). The addition of nitrogen to the soil affected (p < 0.05) the total emission of CH4 in all soils. A quadratic effect was observed for total emissions of CH4 in forest soils (p = 0.005), with higher values observed in soils that received 180 kg N ha−1. In pasture soils, a decreasing linear effect was observed (p = 0.01), whereas agricultural soils showed an increasing linear effect (p = 0.04) as the nitrogen concentration in the soil increased. Pasture soils showed higher total emissions of CH4, regardless of soil nitrogen concentration, with values ranging from 129.2 to 52.8 μg CH4 g−1.
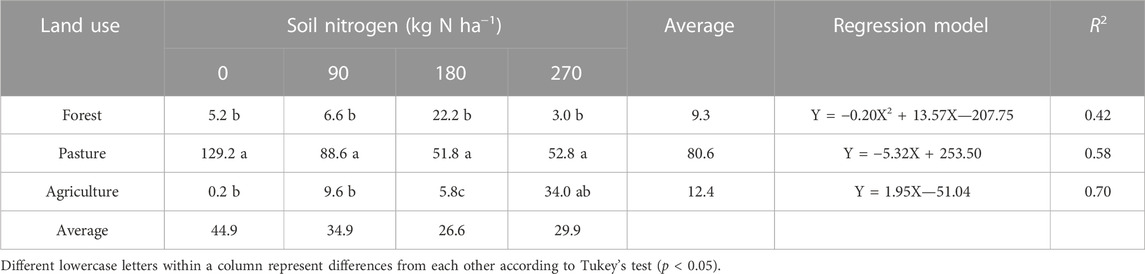
TABLE 4. Total CH4 emissions (μg CH4 g−1 of dry soil) in different land use systems subjected to nitrogen addition in the soil.
Nitrogen rates did little to alter the flux of CH4 in forest soils (Figure 5A) or in agricultural soils (Figure 5C) with incubation time. In forest soils, only one large production peak was observed on the third day of incubation (∼390 ng CH4 g−1 day−1), whereas agricultural soils showed peak production on the sixth day of incubation (∼1,285 ng CH4 g−1 day−1). In contrast, the pasture soil (Figure 5B) presented greater variation between the applied doses, with higher (>600 ng CH4 g−1) fluxes observed when urea was not applied to the soil. When nitrogen fertilizer was applied, production peaks decreased as the dose increased.
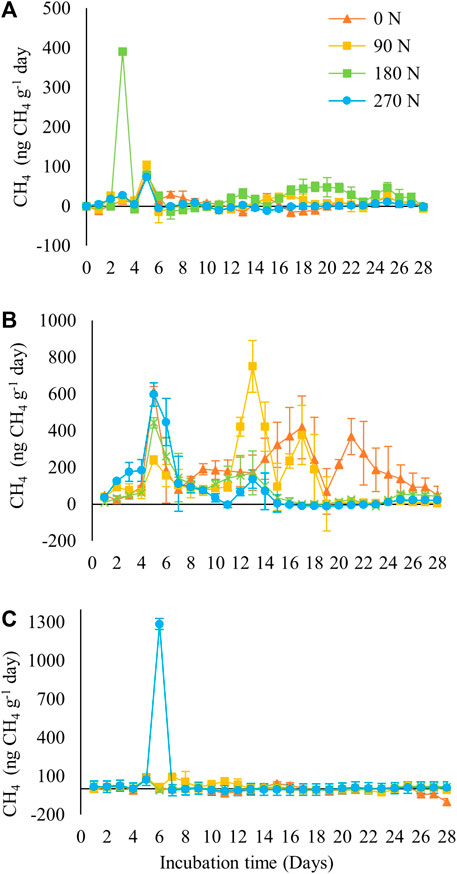
FIGURE 5. CH4 fluxes (ng CH4 g−1 of dry soil day−1) in different land uses systems (A) forest; (B) pasture; (C) agriculture in the Brazilian Amazon, subject to the addition of nitrogen in the soil.
4 Discussion
4.1 Effect of land use on CH4 emission
When different land use types were evaluated, higher emissions of CH4 in pasture soils were found (Figure 1). According to several studies (Potter et al., 1996; Azevedo et al., 2018; Machacova et al., 2020), forest soils are sinks for CH4 due to the vast methanotrophic communities present in the soil. Land-use change tends to cause changes in the soil microbial community, stimulating methanogenic Archaea (Kroeger et al., 2021). These changes were due to changes in the botanical community, soil pH, nutrient cycling, and allocation of carbon in the soil. This set of factors alters the oxidation of CH4 in the soil, causing the area to emit greater amounts of gas (Tate, 2015).
CH4 is produced in the soil by the decomposition of organic matter (Ye et al., 2015). Pasture soils are in a constant cycle of tiller appearance and senescence, thus increasing the rate of litter deposition in the soil, which enters the decomposition process (Chianese et al., 2009). The agricultural soil studied was characterized by a large spacing between lines (2 m × 2 m), being a well-ventilated soil, providing greater oxidation of CH4 (Kirschke et al., 2013; Broucek, 2014).
The bacterial community is also responsible for CH4 cycling and several factors affect the methanogenic community. The conversion from areas by forest to pasture changes the soil microbial community, increasing the abundance and action of methanogenic archaea, as a consequence of this, pasture tends to emit more methane into the atmosphere (Alves et al., 2022). Among the factors, soil moisture and the addition of nutrients may be the largest contributors to CH4 emissions (Knief, 2019). CH4 production in pasture soils and oxidation in forest and agricultural soils occur during the same period because of the addition of nitrogen and water to the soil.
4.2 Effect of incubation temperature on CH4 emission
Temperature variations are important in studies of greenhouse gas emissions, as global warming tends to increase the temperatures of the globe over the years. Therefore, understanding how these variations alter CH4 emission patterns today will help in the research for alternatives in the future.
CH4 emissions from forest soils and agriculture are independent of temperature because forest soils are considered resilient. Therefore, they are less affected by adverse conditions, such as temperature variations, thereby reducing CH4 emissions in the system (Peri et al., 2017). A study that evaluated 41 peer-reviewed publications observed that a temperature rise did not much affect CH4 emissions from forested areas (Dijkstra et al., 2012). In turn, the agricultural soils studied are black pepper, a crop that stands out for its growth habit, of the creeper type. The spacing used in the study was large, about 2 m × 2 m, thus decreasing the content of organic matter present in the soil and keeping the soil more aerated, which causes the methanogenic archaea communities to decrease (Abduh et al., 2020).
In pasture soils, the highest emissions at 25°C are due to the ability of the soil to maintain its moisture for longer than at temperatures of 30°C and 35°C, causing methanogenic bacteria to carry out methanogenesis (Conrad, 2009; Dijkstra et al., 2012). In pasture soils, significant variation in the levels of ammonium before incubation was observed, showing an increase as the temperature increased (except at 40°C). Another important point to be observed is the presence of bovine feces in the area, in which authors show that greater emissions occur above 20°C, however, increasing this temperature beyond 25°C decreases the activities of methanogenic bacteria (Cárdenas et al., 2021; Rennie et al., 2021).
As seen in the previous study, land-use changes directly impact CH4 emissions, as at all temperatures studied, CH4 emissions were higher in pasture soils. Moreover, the change in land use alters the patterns of the soil, whether microbiological, structural, or biodiversity, present in the environment (Ball, 2013; Kroeger et al., 2021). In addition, the lower emissions of CH4 from agricultural soil (black pepper) concerning pastures show that more aerated soils with low litter deposition emit less CH4 into the atmosphere (Kirschke et al., 2013; Valenzuela et al., 2017).
The flux in the pasture soil can be well observed because the variations over time in the N-NH4+ contents are greater, thus favoring the oxidation of soil CH4. However, the peaks observed in the forest and agricultural soils can be explained precisely by the moments at which the soil presented an environment conducive to gas production.
4.3 Effect of soil nitrogen addition on CH4 emission
Agriculture and livestock contribute significantly to greenhouse gas emissions owing to practices that alter soil carbon and nitrogen dynamics (Bento et al., 2018). Fertilization can affect these emissions. Nitrogen fertilization contributes greatly to soil quality and increases crop yield and nutritional value (Delevatti et al., 2019; Santos et al., 2020). However, the source, dose, and application form must be considered because inappropriate use increases greenhouse gas emissions (Raposo et al., 2020; Corrêa et al., 2021; Cardoso et al., 2022).
The fertilizer used in the treatment was urea, an ammoniated fertilizer that increases ammonium levels in pasture soils as the dose increases. Ammonium directly affects methanotrophy, thereby increasing CH4 oxidation in the system (He et al., 2019). The same pattern was not observed in the agricultural soils. However, emissions matched only at higher nitrogen doses.
The variation found in the forest soils was due to the peak observed at a dose of 180 kg N ha−1 on the third day of evaluation. However, the soil quickly returned to the emission patterns, indicating the resilience of forest soils (Peri et al., 2017). A similar pattern was observed in agricultural soils at a dose of 270 kg N ha−1.
The peak observed in pasture soils at all doses was due to urea being converted to ammonia only between days 4 and 6 in the soil (He et al., 2019), which explains the drop in CH4 yields after the sixth day of evaluation.
5 Conclusion
CH4 emissions from tropical rainforest soils and agriculture were lower and less affected by temperature and nitrogen availability. Pasture soils emit more CH4 than forest soils and black pepper cultivation in the Brazilian Amazon, and different production peaks can be observed when pasture soil is subjected to temperature variation. Applying urea as a nitrogen fertilizer tends to reduce CH4 emissions from pasture soils. Given this, the intensification of pasture use can be a management strategy in the face of climate change. Future work is required, especially regarding in situ achievements in the field of study.
Data availability statement
The raw data supporting the conclusion of this article will be made available by the authors, without undue reservation.
Author contributions
Conceptualization: NL, AC, JA, ARê; Data Curation: NL, AC, and JA; Formal Analysis: NL and AC; Funding Acquisition: AC, ARu, RR, and ARê; Investigation: NL, AC, and JA; Methodology: NL, AC, and JA; Project Administration: NL, AC, ARu, RR, and ARê; Supervision: AC; FD, CF, TS, and ARê; Validation: NL, AC, JA, VM, FD, CF, TS, and ARê; Visualization: NL, AC, VM, FD, CF, TS, ARu, RR, and ARê; Writing–original draft Preparation: NL, AC, and ARê. Writing–review and editing: NL, AC, VM, and ARê. All authors contributed to the article and approved the submitted version.
Funding
This study was financed in part by the Coordination for the Improvement of Higher Education Personnel—Brasil (CAPES)—Finance Code 001 and PDPG—Amazônia Legal (013/2020); Amazon Foundation for Studies and Research in the State of Pará—FAPESPA (2019/25234-0) and by the grants 2017/11272-5 and 2018/16273-0, São Paulo Research Foundation (FAPESP).
Acknowledgments
We would like to thank the Study Group on Ruminants and Forage Production of the Amazon (GERFAM) and the Study Groups on Forage (UnespFor) for their support in conducting the experiments. To Federal University of Pará (UFPA) for the financial of Qualified Publication Support Program (PAPQ/UFPA—02/2023). We would like to thank Editage (www.editage.com) for English language editing.
Conflict of interest
Author VM was employed by Brazilian Agricultural Research Corporation.
The remaining authors declare that the research was conducted in the absence of any commercial or financial relationships that could be construed as a potential conflict of interest.
Publisher’s note
All claims expressed in this article are solely those of the authors and do not necessarily represent those of their affiliated organizations, or those of the publisher, the editors and the reviewers. Any product that may be evaluated in this article, or claim that may be made by its manufacturer, is not guaranteed or endorsed by the publisher.
References
Abduh, A. M., Hanudin, E., Purwanto, B. H., and Utami, S. N. H. (2020). Effect of plant spacing and organic fertilizer doses on methane emission in organic rice fields. Environ. Nat. Resour. J. 18, 66–74. doi:10.32526/ennrj.18.1.2020.07
Alvares, C. A., Stapa, J. L., Sentelhas, P. C., Gonçalves, J. L. M., and Sparovek, G. (2013). Köppen's climate classification map for Brazil. Meteorol. Z. 22, 711–728. doi:10.1127/0941-2948/2013/0507
Alves, K. J., Pyrlo, V. S., Nakayama, C. R., Vital, V. G., Taketani, R. G., Santos, D. G., et al. (2022). Methanogenic communities and methane emissions from enrichments of Brazilian Amazonia soils under land-use change. Microbiol. Res. 265, 127178. doi:10.1016/j.micres.2022.127178
Andrade, F. W. C., Pinto, T. I., Moreira, L. S., Ponte, M. J. M., Lobato, T. C., Sousa, J. T. R., et al. (2022). The legal roundwood market in the Amazon and its impact on deforestation in the region between 2009–2015. Forest 13, 558. doi:10.3390/f13040558
Azevedo, T. R., Costa Junior, C., Brandão Junior, A., Crème, M. S., Piatto, M., Tsai, D. S., et al. (2018). Data Descriptor: SEEG initiative estimates of Brazilian greenhouse gas emissions from 1970 to 2015. Sci. data 5, 180045. doi:10.1038/sdata.2018.45
Ball, B. C. (2013). Soil structure and greenhouse gas emissions: a synthesis of 20 years of experimentation. Eur. J. Soil. Sci. 64, 357–373. doi:10.1111/ejss.12013
Bento, C. B., Filoso, S., Pitombo, L. M., Cantarella, H., Rosseto, L. M., Carmo, J. B., et al. (2018). Impacts of sugarcane agriculture expansion over low-intensity cattle ranch pasture in Brazil on greenhouse gases. J. Environ. Manage. 206, 980–988. doi:10.1016/j.jenvman.2017.11.085
Broucek, J. (2014). Production of methane emissions from ruminant husbandry: a review. J. Environ. Prot. 5, 1482–1493. doi:10.4236/jep.2014.515141
Cárdenas, A., Ammon, C., Schumacher, B., Stinner, W., Hermann, C., Schneider, M., et al. (2021). Methane emissions from the storage of liquid dairy manure: influences of season, temperature and storage duration. Waster Manag. 121, 393–402. doi:10.1016/j.wasman.2020.12.026
Cardoso, A. S., Junqueira, J. B., Reis, R. A., and RUggieri, A. C. (2020). How do greenhouse gas emissions vary with biofertilizer type and soil temperature and moisture in a tropical grassland?. Pedosphere 30, 607–617. doi:10.1016/S1002-0160(20)60025-X
Cardoso, A. S., Longhini, V. Z., Berça, A. S., Ongaratto, F., Siniscalchi, D., Reis, R. A., et al. (2022). Pasture manegement and greenhouse gases emissions. Biosci. J. 39, e38099. doi:10.14393/BJ-v38n0a2022-60614
Cardoso, A. S., Quintana, B. G., Janusckiewicz, E. R., Brito, L. F., Morgado, E. S., Reis, R. A., et al. (2017). N2O emissions from urine-treated tropical soil: effects of soil moisture and compaction, urine composition, and dung addition. Catena 157, 325–332. doi:10.1016/j.catena.2017.05.036
Cardoso, A. S., Quintana, B. G., Janusckiewicz, E. R., Brito, L. F., Morgado, E. S., Reis, R. A., et al. (2019). How do methane rates vary with soil moisture and compaction, N compound and rate, and dung addition in a tropical soil? Int. J. Biometeorol. 63, 1533–1540. doi:10.1007/s00484-018-1641-0
Carvalho, T., Domingues, E., and Horridge, J. (2017). Controlling deforestation in the Brazilian Amazon: regional economic impacts and land-use change. Land Use Policy 64, 327–341. doi:10.1016/j.landusepol.2017.03.001
Chianese, D. S., Rotz, C. A., and Richard, T. L. (2009). Whole-farm greenhouse gas emissions: a review with application to a Pennsylvania dairy farm. Appl. Eng. Agric. 25, 431–442. doi:10.13031/2013.26895
Conrad, R. (2009). The global methane cycle: recent advances in understanding the microbial processes involved. Environ. Microbiol. Rep. 1, 285–292. doi:10.1111/j.1758-2229.2009.00038.x
Córdoba, D., Juen, L., Selfa, T., Peredo, A. M., Montag, L. F. A., Sombra, D., et al. (2019). Understanding local perceptions of the impacts of large-scale oil palm plantations on ecosystem services in the Brazilian Amazon. For. Policy. Econ. 109, 102007–102011. doi:10.1016/j.forpol.2019.102007
Corrêa, D. C. C., Cardoso, A. S., Ferreira, M. R., Siniscalchi, D., Toniello, A. D., Lima, G. C., et al. (2021). Are CH4, CO2, and N2O emissions from soil affected by the sources and doses of nitrogen warm-season pasture? Glob. Change Biol. 26, 5267–5276. doi:10.3390/atmos12060697
Cui, J., Lam, S. K., Xu, S., and Lai, D. Y. F. (2022). The response of soil-atmosphere greenhouse gas exchange to changing plant litter inputs in terrestrial forest ecosystems. Sci. Total Environ. 838, 155995. doi:10.1016/j.scitotenv.2022.155995
Dai, Z., Yu, M., Chen, H., Zhao, H., Huang, Y., Su, W., et al. (2020). Improved quantification of microbial CH&lt;sub&gt;4&lt;/sub&gt; oxidation efficiency in arctic wetland soils using carbon isotope fractionation. Biogeosciences 10, 2539–2552. doi:10.5194/bg-10-2539-2013
Delevatti, L. M., Cardoso, A. S., Barbero, R. P., Leite, R. G., Romanzini, E. P., Ruggieri, A. C., et al. (2019). Effect of nitrogen application rate on yield, forage quality, and animal performance in a tropical pasture. Sci. Rep. 19, 7596. doi:10.1038/s41598-019-44138-x
Dijkstra, F. A., Prior, S. A., Réunion, G. B., Torbert, H. A., Tian, H., Lu, C., et al. (2012). Effects of elevated carbon dioxide and increased temperature on methane and nitrous oxide fluxes: evidence from field experiments. Front. Ecol. Environ. 10, 520–527. doi:10.1890/120059
Doane, T. A., and Horwath, W. R. (2003). Spectrophotometric determination of nitrate with a single reagent. Anal. Lett. 36, 2713–2722. doi:10.1081/AL-120024647
Fan, J., Luo, R., McConkey, B. G., and Ziadi, N. (2020). Effects of nitrogen deposition and litter layer management on soil CO2, N2O, and CH4 emissions in a subtropical pine forestland. Sci. Rep. 10, 8959. doi:10.1038/s41598-020-65952-8
Fearnside, P. (2018). Deforestation of the Brazilian Amazon. Environ. Sci. 18. doi:10.1093/acrefore/9780199389414.013.102
Fernandes, S. A. P., Bernoux, M., Cerri, C. C., Feigl, B. J., and Piccolo, M. C. (2002). Seasonal variation of soil chemical properties and CO2 and CH4 fluxes in unfertilized and P-fertilized pastures in an Ultisol of the Brazilian Amazon. Geoderma 107, 227–241. doi:10.1016/S0016-7061(01)00150-1
Forster, J. C. (1995). “Soil nitrogen,” in Methods in applied soil microbiology and biochemistry. Editors K. Alef, and P. Nannipieri 1st edn (London: Academic Press), 79–87.
Garcia-Montiel, D. C., Steudler, P. A., Piccolo, M. S., Melillo, J. M., Neill, C., and Cerri, C. C. (2001). Controls on soil nitrogen oxide emissions from forest and pastures in the Brazilian Amazon. Glob. Biogeochem. Cycles 15, 1021–1030. doi:10.1029/2000GB001349
Gay, J. S., and Sánchez, L. E. (2021). The outbreak of illegal gold mining in the Brazilian Amazon boosts deforestation. Reg. Environ. Change. 21, 28. doi:10.1007/s10113-021-01761-7
He, D., Zhang, L., Dumont, M. G., He, J. S., Ren, L., and Chu, H. (2019). The response of methanotrophs to additions of either ammonium, nitrate or urea in alpine swamp meadow soil as revealed by stable isotope probing. FEMS Microbiol. Ecol. 95, fiz077. doi:10.1093/femsec/fiz077
Heckenberger, M. J., Russell, J. C., Toney, J., and Schmidt, M. J. (2007). The legacy of cultural landscapes in the Brazilian Amazon: implications for biodiversity. Philos. Trans. R. Soc. B 362, 197–208. doi:10.1098/rstb.2006.1979
Khan, M. A. W., Bohannan, B. J. M., Nüsslein, K., Tiedje, J. M., Tringe, S. G., Parlade, E., et al. (2019). Deforestation impacts network co-occurrence patterns of microbial communities in Amazon soils. FEMS Microbiol. Ecol. 95, fiy230. doi:10.1093/femsec/fiy230
Kirschke, S., Bousquet, P., Ciais, P., Saunois, M., Canadell, J. G., Dlugokencky, E. J., et al. (2013). Three decades of global methane sources and sinks. Nat. Geosci. 6, 813–823. doi:10.1038/ngeo1955
Knief, C. (2019). Diversity of methane-cycling microorganisms in soils and their relation to oxygen. Mol. Biol. 33, 23–56. doi:10.21775/cimb.033.023
Kroeger, M. E., Meredith, L. K., Meyer, K. M., Webster, K. D., Camargo, P. B., Souza, L. F., et al. (2021). Rainforest-to-pasture conversion stimulates soil methanogenesis across the Brazilian Amazon. ISME J. 15, 658–672. doi:10.1038/s41396-020-00804-x
Liikanen, A., Huttunen, J. T., Valli, K., and Martikainen, P. J. (2002). Methane cycling in the sediment and water column of mid-boreal hyper-eutrophic Lake Kevätön, Finland. Arch. Hydrobiol. 154, 585–603. doi:10.1127/archiv-hydrobiol/154/2002/585
Lourenço, E. R. C., Souza, B. I., D’Andrea, A. F., and Souza, JJLL (2022). Temporal variation of soil CO2 emission in different land uses in the Caatinga. Appl. Geogr. 140, 102661. doi:10.1016/j.apgeog.2022.102661
Machacova, K., Borak, L., Agyei, T., Schindler, T., Soosaar, K., Mander, U., et al. (2020). Trees as net sinks for methane (CH4) and nitrous oxide (N2O) in the lowland tropical rain forest on volcanic Réunion Island. New Phytol. 229, 1983–1994. doi:10.1111/nph.17002
Metay, A., Oliver, R., Scopel, E., Douzet, J. M., Moreira, J. A. A., Maraux, F., et al. (2007). N2O and CH4 emissions from soils under conventional and no-till management practices in Goiânia (Cerrados, Brazil). Geoderma 141, 78–88. doi:10.1016/j.geoderma.2007.05.010
Meyer, K. M., Klein, A. M., Rodrigues, J. L. M., Nusslein, K., Tringe, S. G., Mirza, B., et al. (2017). Conversion of Amazon rainforest to agriculture alters community traits of methane-cycling organisms. Mol. Ecol. 26, 1547–1556. doi:10.1111/mec.14011
Paiva, P. F. P., Ruivo, M. L. P., Silva, J., de Nazaré Martins Maciel, M., Braga, T. G. M., de Andrade, M. M. N., et al. (2019). Deforestation in protect areas in the Amazon: a threat to biodiversity. Biodivers. Conserv. 29, 19–38. doi:10.1007/s10531-019-01867-9
Peri, P. L., López, D. R., Rusch, V., Rosas, Y. M., Pastur, G. M., and Martínez Pastur, G. (2017). State and transition model approach in native forests of Southern Patagonia (Argentina): linking ecosystem services, thresholds and resilience. Int. J. Biodivers. Sci. Ecosyst. Serv. Manag. 13, 105–118. doi:10.1080/21513732.2017.1304995
Potter, C. S., Davidson, E. A., and Verchot, L. V. (1996). Estimation of global biogeochemical controls and seasonality in soil methane consumption. Chemosphere 32, 2219–2246. doi:10.1016/0045-6535(96)00119-1
Raposo, E., Brito, L. Z., Janusckiewicz, E. R., Oliveira, L. F., Versuti, J., Assumpção, F., et al. (2020). Greenhouse gases emissions from tropical grasslands affected by nitrogen fertilizer management. Agron. J. 112, 4666–4680. doi:10.1002/agj2.20385
Rennie, T. J., Grant, B. B., Gordon, R. J., Smith, W. N., and VanderZaag, A. C. (2021). Regional climate influences manure temperature and methane emissions – a pan-Canadian modelling assessment. Sci. Total Environ. 750, 142278. doi:10.1016/j.scitotenv.2020.142278
Santos, H. C. A., Lima Junior, J. A., Silva, A. L. P., Castro, G. L. S., and Gomes, R. F. (2020). Yield of fertigated bell pepper under different soil water tensions and nitrogen fertilization. Rev. Caatinga. 33, 172–183. doi:10.1590/1983-21252020v33n119rc
Silveira, M. V. F., Silva Junior, C. H. L., Anderson, L. O., and Aragão, LEOC (2022). Amazon fires in the 21st century: the year of 2020 in evidence. Glob. Ecol. Biogeogr. 31, 2026–2040. doi:10.1111/geb.13577
Siqueira Neto, M., Popin, G. V., Piccolo, M. C., Scopel, E., Camargo, P. B., Bernoux, M., et al. (2020). Impacts of land use and cropland management on soil organic matter and greenhouse gas emissions in the Brazilian Cerrado. Eur. J. Soil Sci. 72, 1431–1446. doi:10.1111/ejss.13059
Souza, L. E. V., Fetz, M., Zagatto, B. P., and Pinho, N. S. (2021). Violence and illegal deforestation: the crimes of “environmental militias” in the Amazon forest. Cap. Nat. Soc. 33, 5–25. doi:10.1080/10455752.2021.1980817
Souza Filho, P. W. M., Lobo, F. L. L., Cavalcante, R. B. L., Mota, J. Á., Junior, N., Santos, D. C., et al. (2020). Land-use intensity of official mineral extraction in the Amazon region: linking economic and spatial data. Land Degrad. Dev. 32, 1706–1717. doi:10.1002/ldr.3810
Steudler, P. A. M., Melillo, J. M., Feigl, B. J., Neill, C., Piccolo, M. C., and Cerri, C. C. (1996). Consequence of forest-to-pasture conversion on CH4 fluxes in the Brazilian Amazon Basin. J. Geophys. Res. 101, 18547–18554. doi:10.1029/96JD01551
Tate, K. R. (2015). Soil methane oxidation and land-use change – from process to mitigation. Soil Biol. biochem. 82, 260–272. doi:10.1016/j.soilbio.2014.10.010
Valenzuela, E. I., Prieto-Davó, A., López-Lozano, N. E., Hernández-Eligio, A., Veja-Alvarado, L., Juárez, K., et al. (2017). Anaerobic methane oxidation driven by microbial reduction of natural organic matter in a tropical wetland. Appl. Environ. Microbiol. 83, 006455–e717. doi:10.1128/AEM.00645-17
Vasconcelos, K., Farinha, M., Bernardos, L., Lampert, V. N., Gianezini, M., Costa, J. S., et al. (2018). Livestock-derived greenhouse gas emissions in a diversified grazing system in the endangered Pampa biome, Southern Brazil. Land Use Pol. 75, 442–448. doi:10.1016/j.landusepol.2018.03.056
Ye, R., Doane, T. A., Morris, J., and Howarth, W. R. (2015). The effect of rice straw on the priming of soil organic matter and methane production in peat soils. Soil Biol. biochem. 81, 98–107. doi:10.1016/j.soilbio.2014.11.007
Zhao, J. F., Peng, S., Chen, M. P., Wang, G. Z., Cui, Y. B., Liao, L. G., et al. (2019). Tropical forest soils serve as substantial and persistent methane sinks. Sci. Rep. 9, 16799. doi:10.1038/s41598-019-51515-z
Keywords: arc of deforestation of the Amazon, CH4 drivers, land use change, nitrogen, temperature
Citation: Lage Filho NM, Cardoso AdS, Azevedo JCd, Macedo VHM, Domingues FN, Faturi C, Silva TCd, Ruggieri AC, Reis RA and do Rêgo AC (2023) How does land use change affect the methane emission of soil in the Eastern Amazon?. Front. Environ. Sci. 11:1244152. doi: 10.3389/fenvs.2023.1244152
Received: 21 June 2023; Accepted: 21 November 2023;
Published: 04 December 2023.
Edited by:
Alban Kuriqi, University of Lisbon, PortugalReviewed by:
Sangeeta Lenka, Indian Institute of Soil Science (ICAR), IndiaGabriel Brito Costa, Federal University of Western Pará, Brazil
Celso H. L. Silva-Junior, Instituto de Pesquisa Ambiental da Amazonia (IPAM), Brazil
Luana Santamaria Basso, Max Planck Institute for Biogeochemistry, Germany
Copyright © 2023 Lage Filho, Cardoso, Azevedo, Macedo, Domingues, Faturi, Silva, Ruggieri, Reis and do Rêgo. This is an open-access article distributed under the terms of the Creative Commons Attribution License (CC BY). The use, distribution or reproduction in other forums is permitted, provided the original author(s) and the copyright owner(s) are credited and that the original publication in this journal is cited, in accordance with accepted academic practice. No use, distribution or reproduction is permitted which does not comply with these terms.
*Correspondence: Aníbal Coutinho do Rêgo, YW5pYmFsY3JAdWZjLmJy