- 1Department of Chemistry and Biochemistry, School of Sciences, Jain University, Bangalore, India
- 2Department of Biotechnology, School of Life Sciences, Dr. Bhimrao Ambedkar University, Agra, Uttar Pradesh, India
- 3Department of Biotechnology, Maharani Lakshmi Ammanni College for Women, Bengaluru, India
- 4Redox Regulation Laboratory, Department of Zoology, College of Basic Science and Humanities, Odisha University of Agriculture and Technology, Bhubaneswar, India
- 5Department of Biotechnology, President Science College, Ahmedabad, India
- 6Department of Forensic Science, Garden City University, Bangalore, India
- 7Department of Life Sciences, Jain School of Science, Jain University, Bengaluru, Karnataka, India
- 8Department of Biochemistry, Maharani Lakshmi Ammanni College for Women, Bengaluru, India
- 9Botany and Biotechnology, Keral Verma Subharti College of Sciences, Swami Vivekanand Subharti University, Meerut-, India
- 10Department of Biochemistry, Avinashilingam Institute for Home Science and Higher Education for Women, Coimbatore, India
- 11Department of Veterinary Clinical Sciences, College of Veterinary Medicine, Iowa State University, Iowa, IA, United States
Fish are among the best-studied aquatic animals due to their economic and ecological values. Fish meat is the most affordable protein source for the economically weaker section of people. The environment of almost all aquatic ecosystems has a specific influential role on or by fishes. Therefore, studying their stress biology, especially oxidative stress, is vital because it can influence their growth, production, reproduction, etc. To review the above topic, peer-reviewed electronic databases, including Web of Science, science direct, PubMed, Google Scholar, Scopus, and AGRICOLA, were searched with specific keywords associated with fish, oxidative stress, diseases, etc. The influence of abiotic stress, such as the effects of water dissolved oxygen, temperature, salinity, water hardness, alkalinity, pH, pollutants, heavy metals, and anthropogenic activities, was reviewed in the current article to draw a conclusion on the updated relation that exists between fish physiology, disease, and abiotic stressors. Oxidative stress and redox regulatory levels under the above parameters were reviewed as the stress or anti-stress responses differ in various fish models. Undoubtedly, the reviewed abiotic factors modulate fish oxidative health status to a greater extent, and therefore, these factors must be considered on a priority basis to improve the general health and immunity status of fish. The statement above remains valid in both saline and freshwater habitats.
1 Introduction
Environmental fluctuations occur in the aquatic environment, mainly due to abiotic stress generated by extremities in pH, salinity, temperature, dissolved oxygen (DO), acidity, pollution load, etc. (Masson et al., 2002; Moon et al., 2003; Wu et al., 2018; Bernhardt et al., 2020; Song and Choi, 2021; Booth et al., 2023). The primary causes of abiotic stress in aquatic organisms are anthropogenic activities, including metal pollution, petrochemicals, domestic wastes, and other contaminants (Bal et al., 2022; Bal and Paital, 2023). Different bioindicators are commonly used in detecting stress caused by pollutants and physicochemical factors in water. Numerous studies have been conducted to investigate and comprehend the impact of external environmental elements on livestock and aquatic animals, specifically focusing on the abiotic stress phenomena (Howden et al., 2008; Tripathi and Sindhi, 2020; Mansou et al., 2023). Fish are prone to stress under climatic changing conditions. And fish are largely affected due to abiotic stress conditions in the aquatic environment. Due to this, globally, fish production is predicted to decline by 10% in 2050 (Boraiah et al., 2021). Various strategies and methods must be employed to combat the abiotic stress-inducing factors because these factors can generate internal cellular stress, including oxidative stress caused by over-produced reactive oxygen species (ROS) in fish and other organisms (Paital, 2013, 2014).
Variations in environmental factors, use of certain medicines, electromagnetic spectrum involving UV, visible rays, etc., can indeed contribute to the production of free radicals (Biller and Takahashi, 2018). Oxidative stress mainly occurs due to the accumulation of ROS in cells and tissues of organisms; hence it is ubiquitous in nature. In aquatic animals, there are different cellular strategies adapted by which they show defence against oxidative stress. Mainly it is with the scavenging effect of small antioxidants and antioxidant enzymes (Diler et al., 2022). But due to anthropogenic activities and increased environmental factors such as pH, temperature, and chemical pollutants, damage incurred to the cellular antioxidant system (Valavanidis et al., 2006). Various pollutants, such as pesticides, organochlorines, organophosphates, etc., are hazardous to organisms, including fish as well as to the environment. Some of the effects of these pollutants on fish are alteration in DNA, antioxidant enzyme inhibition, altered gene expression, and the generation of heat shock proteins (Arantes et al., 2016; Biller and Takahashi, 2018). It leads to hampering the normal physiology of fish. The analysis of these fish phenomena is of utmost importance due to their significant contribution to the economies of various countries, particularly developing nations such as India, China, Brazil, and others.
The annual economic outcome from oceanic sectors is around $1.5 trillion, and it is predicted that the value may reach (OECD, 2021). As a major contributor to the above sector, aquaculture sectors produced 178 tonnes in 2020, of which captured fisheries contributed 90 million tonnes, with a “first sale” with a total estimated cost of US$ 406 billion. The total cost constitutes US$ 141 billion from captured fisheries sector and US$ 265 billion from the global aquaculture sector in 2021. And the later value has been raised to US$ 289.6 billion in 2022. According to the Food and Agriculture Organization of the United Nations (FAO) data, marine water capture was 63% while 37% in inland waters (FAO, 2020, 2021). The aquatic ecosystem (blue carbon) is of paramount importance for fostering economic growth and ensuring food security. Moreover, these ecosystems play a crucial role in mitigating climate change by effectively regulating carbon balance, surpassing even the carbon sequestration capacity of forests (World Bank, 2021). Reduction in the balance of aquatic ecosystems is mainly due to the accumulation of various pollutants, leading to stress induction in the animals living in the water. Oxidative stress is extensively studied in aquatic species, especially in fish. Variations in physicochemical parameters such as pH, temperature, etc., and pollutants such as crude oil, pesticides, and other contaminants cause severe abiotic stress, including oxidative stress in aquatic species. Affected inhabitants in highly polluted habitats can be considered bio-indicators, especially the infected fish population, to evaluate the state and health of an ecosystem. Monitoring variations in the antioxidant enzymes and small antioxidants can be useful in predicting environmental contamination (Regoli et al., 2011). So, the study of oxidative stress in hydrobionts was already performed decades back (Lushchak, 2010, 2011), and reports have already highlighted the development, pathology, and aging perspective in aquatic organisms under the changing water physicochemical conditions (Zang et al., 2011; Paital, 2014; Zhou et al., 2019; Xing et al., 2022).
Oxidative stress is an important physiological response in all animals that influence their reproduction, growth, quality, and production quantity in farmed/cultured animals, including fishes (Paital, 2013). These phenomena are influenced by abiotic factors in fishes both under artisanal and open sea/river culture systems. Therefore, it was hypothesized that oxidative stress must be emphatically contributing in fish to manage their health and economic value. The results of this review will be useful in managing the physiological role of oxidative stress in aquatic organisms in general and fish in particular. All major databases are searched with different terminology to establish any relation between oxidative stress and fishes in relation to abiotic stress.
2 Materials and methods
For an updated review on the topic “oxidative stress in fish in relation to abiotic stress”, key terms such as fish, oxidative stress, abiotic stress, salinity, pH, temperature, pollution, etc., were searched alone or in combination with terms such as redox regulation, antioxidants, “physiology”, “stress”, “biochemical”, “molecular mechanism”, environmental response, etc., in the key databases such as Web of Science, Scopus, PubMed, AGRICOLA, Google scholar, etc. Only peer-reviewed published articles were included in this review using traditional manual methods. Literature published in English was only included in the study, whereas any other local manuals, articles, books, etc., obtained as a search result were excluded from this study.
2.1 Sources of data and strategy for search
PubMed/MEDLINE, AGRICOLA, Scopus, Web of Science, science direct, and Google Scholar are a few of the largest databases for peer-reviewed published literature. Google Scholar, a large database in all fields of science, may not contain peer-reviewed articles. So, care was taken to check manually before including any articles from this database. PubMed contains peer-reviewed articles in biomedicine, while both peer-reviewed and non-peer-reviewed articles are eventually stored in the Google search engine. The literature (about 6 million) in AGRICOLA pertains to the field of agriculture and allied sciences. It is necessary to emphasize that fishes are exclusively included in this database as they are available in cultivated as well as in natural saline, brackish, and freshwater bodies. Scopus is a database that evaluates journals on certain criteria. Therefore, articles were also searched from this database. Similarly, the Web of Science includes journals with their articles based on quite highly stringent peer-review criteria. The aforementioned factors were considered crucially in choosing the above databases for searching the related articles. The peer-reviewed English papers on fish published from the above-cited databases related to oxidative stress physiology were screened. Search terms such as abiotic and biotic stressors, antioxidant enzymes, oxidative stress in special relation to dissolved oxygen, temperature, salinity, hardness and alkalinity, pH, heavy metals pollutants and anthropogenic activity in relation to in fish health were explored in the above databases electronically.
2.2 Study selection
Besides the published peer-reviewed articles in the above databases in the form of books, journals, periodicals, and various authentic reputed webpages such as https://www.fao.org/home/en and various other authentic databases that are published till February of 2023 were also included in this study. Articles without any terms mentioned in Section 2.1 were excluded. Articles matching within the subject area of the fish and oxidative stress physiology were included in the review, whereas any axillary articles in which the above term(s) were merely mentioned were excluded from the review. Out of 4,294 articles found in PubMed, 1,970 articles in AGRICOLA on the search term fish oxidative stress antioxidants and 20,500 articles from Google Scholar were filtered manually to fix the search in relation to DO, temperature, salinity, hardness and alkalinity, pH, heavy metals pollutants and anthropogenic activity. All the data from the published papers were extracted independently and unbiasedly, irrespective of the geographical region, institution, or person.
2.3 Data extraction
In order to streamline the entire review process securely and to ensure reliability and consistency, we have covered various causes and effects (such as disease) of oxidative stress in fish. Studies on antioxidant enzymes such as superoxide dismutase, catalase, glutathione peroxidase, glutathione reductase, and glutaredoxin system, small antioxidants such as the reduced glutathione, oxidised glutathione, ascorbic acid, total antioxidant capacity, reactive oxygen species scavenging activity, oxidative stress markers such as lipid peroxidation, protein carbonylation and reactive oxygen species markers such as hydrogen peroxide, superoxide radicals, hydroxyl radicals, hydroperoxides in fish under abiotic factors were manually selected and included in this review.
2.4 Synthesis and analyses
Once the authors were convinced about the peer-review and authenticity of the website in the electronic sources, a total of <280 articles were included avoiding duplications among all databases. The content under the subject of each article was extracted and categorized under 1) dissolved oxygen, 2) temperature, 3) salinity, 4) hardness and alkalinity, 5) pH, 6) pollutants including heavy metals, and 7) anthropogenic activity in relation to oxidative stress physiology parameters in fish. As previously indicated, the review did not include articles that only referenced the aforementioned category phrases or search terms.
3 Current updates on fish production
One should have a brief idea of the current status of fish and fisheries sectors and why this aspect must be studied emphatically. China tops the list among fish-producing nations (Table 1). The fish production world also increased in a steady state. The total fish production increased from 33,643,331 metric tonnes in 1960 to 212,766,144 in 2022 (World Bank, 2023a). Among the most developing countries, such as India, has steadily increased its fish output (Tractor junction, 2023). In 2019–2020, India produced approximately 14 million metric tonnes making it the second largest producer. The country encompasses ocean, freshwater, and brackish aquaculture. The Indian government has encouraged aquaculture, fish farming infrastructure, and the fishing sector in recent years. It has increased fish output, mostly through aquaculture, and can be an example for other countries for fresh and marine water aquaculture (Jellyman et al., 2003; Jellyman, 2007). Overfishing, habitat damage, disease outbreaks, and climate change threaten fish-producing businesses in most developing countries (DOF, 2023d); therefore, sustainable fish farming is needed by adapting various modern technologies (Banan et al., 2020a).
It is worth noting that these figures are subject to change and that the ranking of countries in fish production may differ depending on the source and the year. Nevertheless, these countries collectively account for a large portion of global fish production, highlighting the importance of the fishing industry in these countries and the need for sustainable management of fish populations and aquatic ecosystems (World Bank, 2023a). Cultured aquatic organisms are needed to be farmed in an organised fashion. The cultivated organism must be predetermined to be an aquatic species by its very nature. It is comprised of finfish, such as Tilapia, carp, trout, milkfish, bait minnow, yellowtail, mullet, and catfish, etc., captured from the sea or cultivated in human-made set-ups (Worldfishcenter, 2023). Shrimp, prawns, oysters, mussels, pearl oysters, etc., are the second group known as shellfish (Table 2). Water chestnuts (Trapa natans), Japanese red alga, or Norie (Porphyra), Filipino and American red algae (Eucheuma), Japans brown alga called Wakame (Undaria) are plants products of aquaculture (FAO, 1987).
Considering the international status of aquaculture crops (Table 2), the Japanese Resource Council, Science and Technology Agency has defined aquaculture as follows: Aquaculture is the industrial process of raising aquatic organisms to final commercial production within properly partitioned aquatic areas, controlling the environmental factors and administering the life history of the organism, and it has to be considered as a separate industry from the fisheries hitherto. The largest fish producer is Asia, contributing to about 84% of the world’s total aquaculture production.
Fish are essential components of aquatic ecosystems; they not only play an essential part in the food web but also ensure that these ecosystems remain in a state of delicate equilibrium to provide a stress-free environment for fish (Craig et al., 2016). Therefore, understanding the impact of the changing water physicochemical factors on (oxidative) stress in fish is crucial for several reasons, including for their aquaculture and judicious Exploitation (Sotoudeh et al., 2019). To understand, for example, fish health and survival under stress (Chowdhury and Saikia, 2020; Sharifi-Rad et al., 2020), for the balance of the aquatic ecosystem (Allgeier et al., 2013; Layman et al., 2013), for judicious consumption to maintain human health and nutrition (Mozaffarian et al., 2005), and to use them as a model organism for oxidative stress and other stress studies (for example, Danio rerio, Martos-Sitcha et al., 2020), it is necessary to have a thorough perspective on the subject matter.
Besides the above, the economic and social importance of fish with special reference to developing countries is very important in developing countries, including India (DOF, 2023a; 2023c), and it contributed to US$ 6,957 million export values only by marine fisheries in 2021–22 only in India (PIB, 2023). Keeping the export values and internal demand and use, countries have already proposed various programs for the judicious culture, production, and consumption of fish and fisheries products (DOF, 2023a, 2023b; DOF, 2023b; Eparlib, 2023; NFDB, 2023; Pmmsy, 2023; Reogma, 2023; Sinha, 2023; Tractorjunction, 2023). The increased consumption of fish and fisheries products in various fields, including biotechnology, research models in genetics, etc., also demanded studies on their habitat’s effects (Conley and Barden, 2016). For example, use of Atlantic salmon AquAdvantage salmon eggs with a growth hormone gene and an ocean pout antifreeze protein promoter, genetically modified farmed Tilapia, carps, Barramundi, Trout production, production of Fish oil and Omega-3 supplements, production of Surimi, etc. (Hicks, 2016; Munshi and Sharma, 2018; Jerry et al., 2022; MPEDA, 2023; ODS, 2023). Owing to the importance of fish in both ecosystems and society, the route of their decline in production needs to be studied. It has been noticed that stress and disease management are two key components that contribute to the quality and quantity of products in fisheries sectors.
4 Stressors and diseases behind loss of fish and fisheries product
It is noteworthy to mention that pisciculture is dependent on multiple abiotic and biotic factors, which may impart their role alone or in combination to control fish physiology. Finally, it may lead to exert (oxidative) stress in fish that can be a marker for improper growth and production in fish (Paital and Chainy, 2012). When such factors combine with disease(s) as a cause or effect, it greatly impairs fish physiology (Bal et al., 2021a; 2021b). Bacterial and viral infections in fish can cause huge losses in fisheries sectors, which might vary depending on the species, habitat, and illness, making it difficult to precisely assess fish losses. Nevertheless, bacterial and viral illnesses can decimate fish populations and the fishing sector. These losses can diminish productivity, fish yields, and economic losses (Sindermann, 1984; Plumb and Hanson, 2010). Many such diseases, such as ichthyophthiriasis which is also known as Ich (Ekanem et al., 2004), columnaris disease caused by Flavobacterium columnare (LaFrentz et al., 2022), septicaemia, skin and fin rot, and systemic infections caused by Aeromonas hydrophila (Toranzo et al., 2005), viral diseases that may influence molecular signalling (Sun et al., 2020), feeding and, swimming behaviour (Crane and Hyatt, 2011), inflammation and hematopoiesis (Crane and Hyatt, 2011), necrosis (Crane and Hyatt, 2011) are noted. Similarly, parasitic diseases caused by Dactylogyrus, Gyrodactylus, tapeworms, such as Lernaea and Dicrocoelium (Iyaji and Eyo, 2009), an epizootic ulcerative syndrome caused by Aphanomyces invadans and A. hydrophila, (Mishra, 2017), catfish nervous necrosis virus disease (Mishra, 2017), white spot disease caused by white spot syndrome virus (WSSV) (Mishra, 2017), etc., can ultimately influence the stress factor in fishes.
Therefore, pisciculture more or less relies on fish microbiology, which studies fish microbes in natural and cultivated habitats (Noble and Summerfelt, 1996). It is to be noted that the infection and mode of action of the pathogens in fish are more regulated by (oxidative) stress and environmental factors. So, besides diseases, various abiotic and biotic stress factors are also responsible for generating internal cellular stress, including oxidative stress in fish that lead to economic loss in fish aquaculture (Bal et al., 2022). Experiencing oxidative stress due to environmental insults is one of the examples for which the production of fish and other aquaculture animals is proven to be reduced (Lackner, 1998; Paital and Chainy, 2012; Castro et al., 2020; Dong et al., 2022).
5 Oxidative stress physiology and fish
Fish oxidative stress genes and their environmental connections can be discovered using molecular biology markers. Oxidative stress harms fish health and industrial output. Fish genes expressed during experiencing oxidative stress can disclose reasons and lead to novel therapies. Transcriptomics, proteomics, and genomes are revealing fish oxidative stress genes and pathways and developing new management strategies.
A few of the following genes are examples; Expression of genes that eliminate free radicals are controlled by erythroid 2-related factor 2 (Nrf2). Tripeptide glutathione (GSH) and other associated enzymes remove ROS and other toxins (Srikanth et al., 2013; Branco et al., 2014). Glutathione peroxidase (GPx) antioxidant family enzymes neutralise ROS and protect cells from oxidative damage (Do et al., 2019). Thioredoxin (Trx) proteins eliminate ROS and other reactive chemicals to minimise oxidative damage (Branco et al., 2012). Adaptor protein-14-3-3 regulates cellular signalling pathways, including oxidative stress responses (Pennington et al., 2018). PTEN-induced putative kinase (PINK1) gene is associated with oxidative stress pathology in Zebrafish (Priyadarshini et al., 2013).
Oxidative stress is a major factor affecting healthy fish longevity (Paital et al., 2016). It happens when there is a difference between how much ROS are produced, accumulated and how well the fish can get rid of them by antioxidants or fix the damage they do. Studying oxidative stress in fish is important because it can give clues about the causes and effects of oxidative stress in aquatic ecosystems and how fish have adapted to it. Oxidative stress can alter respiratory chain enzyme function and reduce energy generation. Oxidative stress can alter glucose metabolism genes and impair energy generation and storage. Oxidative stress alters fish gene expression for energy metabolism, maturation, reproduction, immunological and antioxidant enzymes, and other cellular defence proteins (Jomova et al., 2023). If oxidative stress levels are too high, DNA, proteins, and lipids can be damaged, leading to cellular malfunction and even death (Sharifi-Rad et al., 2020). Oxidative stress-based altered expression of genes for glucose metabolism, fatty acid metabolism, and the citric acid cycle could result in a change in the energy balance of the fish. This can have deleterious effects on fish development, reproduction, and survival; understanding the complicated oxidative stress physiology and gene expression relationship is essential for aquaculture.
5.1 Oxidative stress has negative effects on fish immunity
Immunity is one of the important defences in fish to protect against diseases that indirectly influence their growth, production, and reproduction. When fish are stressed by factors like high levels of contaminants or low levels of DO, their natural defence system, the immune system, can become overwhelmed (Bols et al., 2001). Due to stress, their weakened immune system makes them more vulnerable to sickness and infection (Lushchak, 2011). Also, the accumulation of ROS in the fish can damage lipids, proteins, and DNA through oxidation (Marcos-López et al., 2010; Giefing-Kröll et al., 2015). This can further weaken their immune system and make them more likely to get sick. As a result, it is essential to uphold favourable environmental circumstances, such as ideal water quality and temperature, in order to lessen the effects of oxidative stress and boost fish immunity.
Oxidative stress research on fish can lead to new feed additives, biotechnology products, and ways to raise healthier and more productive fish. Studying oxidative stress in fish helps in learning about the ecology, biology, and physiology of aquatic species to come up with new tools and ways to protect them and their habitats. Such information can also be used to control and protect fish populations and their habitats.
5.2 Abiotic stress, oxidative stress and fish health
Abiotic stress refers to the negative effects of physical and chemical factors in the environment on aquatic organisms. In the context of aquaculture, abiotic stress marks noteworthy effects on the health, survival, and growth of farmed fish, as well as on the productivity and sustainability of the aquaculture industry. Some of the most common abiotic stress factors in aquaculture include water temperature, salinity, pH, DO, etc. It has been noticed that water temperature (Kozak, 2005; Brander, 2007; Dalvi et al., 2009; Anttila et al., 2013; Claësson et al., 2016), pollution (Austin, 1998), salinity (Dominguez et al., 2004; Abou Anni et al., 2016; Vargas-Chacoff et al., 2018; Bal et al., 2021a), pH (Randall and Tsui, 2002), light availability (Villamizar et al., 2014; Tian et al., 2015; Martínez-Chávez et al., 2021) influence the (oxidative) stress physiology in fish. Therefore, these factors need special attention for pisciculture management.
The influence of light intensity and photoperiod on fish behaviour, physiology, and health has been observed (Bani et al., 2009). It was also observed that the colour of the tank affects fish physiology and stress pattern, as studied in the fish Huso huso (Banan et al., 2011). Fish nutrition, circadian rhythm, eating behaviour, metabolism, reproduction, and development can be affected by light intensity (Tian et al., 2015). In some fish, the higher light intensity can cause phototoxicity, oxidative stress, fish cell damage, and influence development, while too little light might slow development by impairing nutrition and metabolism (Martínez-Chávez et al., 2021). Light intensity regulates fish circadian rhythms, which regulate digestion and metabolism. Hence, optimum light intensity in aquaculture systems is essential for fish health. Aquaculture systems need adequate light intensity to keep fish healthy and maximum yield (Villamizar et al., 2014; Tian et al., 2015; Martínez-Chávez et al., 2021).
In addition to environmental factors, the spread of disease, the accumulation of waste, and the presence of predators can also cause stress to farmed fish and negatively impact the health of the fish population. Maintaining adequate water quality, temperature, and other environmental variables in aquaculture operations reduces abiotic stress. This may require proper water management, aeration, water quality monitoring, and disease control to avoid disease spread. Reduced stress in farmed fish improves production, growth, and survival and promotes aquaculture sustainability. Most fish prefer a pH of 7, while 6.5 to 8.5 is optimum. Most fish require water temperatures between 15°C and 25°C. At a minimum, fish require 5 mg/L of dissolved oxygen; however, 7 mg/L is optimal. As warmer water holds less dissolved oxygen, species and water temperature affect the exact needed levels.
Water parameters must be monitored periodically and maintained at appropriate levels for the type of fish produced to avoid substantial health risks and economic losses to the aquaculture business. The scale and location of the fish business in India, the kinds of fish being grown, and the reason for the loss makes it difficult to calculate the sector’s economic losses. The industry’s financial success can be affected by disease outbreaks, environmental circumstances, and changes in market demand. Diversifying portfolios and capitalising on the rising demand for fish and seafood may be done by investing in the fish market. The advent of technology has undeniably facilitated the aquaculture industry, which serves as a prominent source of fish production. This technological advancement has brought about certain conveniences and enhanced ecological sustainability to a certain extent. However, it is imperative to recognize the significance of comprehending the impact of distinct environmental factors on the oxidative stress biology of aquatic organisms at the cellular level. Such understanding holds immense value in guiding future research endeavors within this domain.
5.2.1 Dissolved oxygen, oxidative stress and fish health
Aquaculture requires a precise dissolved oxygen (DO) level (Rajwa et al., 2014). DO is affected by aeration, temperature, photosynthesis, organic waste decomposition, respiration, salinity, pressure, and other factors. Consequently, studying and implementing oxygen production and introduction have been a crucial focus in current research (Yadav et al., 2023). Aquatic hypoxia and hyperoxia affect fish physiology due to several variables. DO affect freshwater fish physiology. It can impart stress on various behavioural and physiological changes in fish, such as swimming, eating, respiration, growth, reproduction, disease management, survival, health parameters, and immunity. Additionally, DO is essential for the survival and health of fish. Fish obtain the oxygen they need to breathe from the water they swim in, and if DO levels in the water are insufficient; it can have detrimental effects on their health. Swingle (1969) developed a DO scale for warm-water fish (Table 3).
Carlson and Siefert (1974) examined the effects of low oxygen on lake trout (Salvelinus namaycush) and largemouth bass (Micropterus salmoides) eggs and larvae. The capacity of fish to tolerate hypoxia and swim under conditions of chronic hypoxia is enhanced by certain remodelling of the gill, heart, and swim muscles. Furthermore, increased hematocrit, higher oxygen binding affinity of haemoglobin, augmented anaerobic capacity, and increased cardiac output (Wilson and Egginton, 1994; Chippari-Gomes et al., 2005; Svendsen et al., 2010) are remaining factors affecting the hypoxia tolerance in fish.
The flocculating oxygen level, especially hypoxia, along with temperature, can also influence the lives of a few inhabitants. At 7 and 10°C, lake trout development from fertilisation to first feeding commenced at all decreased oxygen saturations (≤50% lower level), but survival was negatively impacted at all concentrations except at 50% saturation at 7°C. Largemouth bass survived with 35% oxygen saturation at 20 and 23°C habitat water temperatures. Fish growth was inhibited at 70% oxygen saturation; at 50% and lower, hatching was premature, and first feeding was delayed. DO was also found to hamper the growth of fish in different ways (Table 4).
Similarly, atmospheric pressure along with DO was also found to affect the life of fish as observed in black barbel catfish Pelteobagrus vachelli (Fu et al., 2009), common carp Cyprinus carpio (Zhang et al., 2010), and Crucian carp (Zhang et al., 2012). Adult salmonids migrate upstream with low dissolved oxygen (Ali and Mishra, 2022). Lack of dissolved oxygen can stress, stunt, or kill fish. Chemical oxygen demand (COD) and biological oxygen demand (BOD) are typical approaches to assessing water quality and its impact on aquatic life. The parameters of COD and BOD are utilized to quantify the amount of oxygen that aquatic species necessitate for respiration. Organic pollutants lead to COD, and BOD can reduce water’s dissolved oxygen (APHA, 1992). Total Dissolved Solids (TDS) may also impair farmed fish health. The TDS in a particular volume of water includes salts, minerals, and other inorganic substances. Fish can die from osmotic stress produced by high TDS levels. Temperature, salinity, air pressure, and other dissolved substances all impact water’s ability to hold DO (APHA, 1992).
Zebrafish of any life stage are a highly suitable model for studying the impacts of oxidative stress in vertebrates due to several factors (Villamizar et al., 2014). These include their transparent embryos, cost-effectiveness, genomic similarity to humans, straightforward developmental processes, high number of offspring per spawning, and other advantageous characteristics (Chowdhury and Saikia, 2022). So, it is also a valuable model organism for studying oxidative stress and its impacts on health and behaviour. Their small size, rapid development, and genetic tractability make them ideal organisms for investigating the underlying mechanisms of oxidative stress and developing new treatments to mitigate its negative climatic impacts (Villamizar et al., 2014; Chowdhury and Saikia, 2022). Studies on zebrafish have shown that DO levels can significantly impact their physiology and behaviour. For example, exposure to low DO levels can cause oxidative stress and impair the immune system, increasing disease susceptibility. Conversely, exposure to high DO levels can result in oxidative stress by higher accumulation of ROS. Fish require oxygen in order to metabolize food and convert it into energy. Consequently, insufficient amounts of DO in their environment can potentially lead to starvation. This can cause slow development, impaired immunity, and death in fish. Low DO stresses fish, weakening their immune systems and making them more susceptible to illnesses. Stressed fish may slow down, cease eating, or swim towards water having high dissolved oxygen (Zhou et al., 2019; Ali and Mishra, 2022).
Low DO levels affect fish reproduction. In several species, low DO levels impair egg production or quality. Low DO levels make fish waste-produced ammonia more toxic. Maintaining enough DO, organic contaminant reduction, and water quality monitoring may improve farm cultivation. Fish generally show high hypoxia tolerance due to decreased oxygen demand and environmental oxygen tension. In nature, quick swimming is probably more common than continuous swimming at maximum sustainable speeds and depends on DO (Ali and Mishra, 2022). When exposed to low DO levels, fish exhibit reduced swimming abilities but typically swim at moderate rates under normal DO conditions (Zhou et al., 2019). At normal DO concentrations, fish can continue to swim at a modest speed. Nevertheless, in specific atypical circumstances, ingesting insufficient oxygen can result in the generation of ROS and the onset of oxidative stress in fish. These situations may arise from either aberrant health conditions of the inhabitants or diminished O2 content in the aquatic environment (Paital et al., 2016). Oxidative stress refers to a state of imbalance between the generations of ROS and the ability of biological systems to eliminate the reactive by-products or restore the consequent damage to proteins, lipids, and DNA.
According to Wedemeyer et al. (1976), the condition of hypoxia has the potential to inflict harm upon the immune systems of fish, thereby rendering them more susceptible to contracting diseases. According to Mellergaard and Nielsen (1995), the presence of hypoxia exacerbates the pathogenicity of specific endemic diseases. The sensitivity of fish to low concentrations of DO is subject to variation based on a multitude of factors, including but not limited to species, life stage (such as eggs, larvae, and adults), life processes (such as feeding, growth, and reproduction), and types of activity (such as resting, swimming, and digesting). Oxidative stress and immunological function are closely related in fish. Fish incur oxidative stress when ROS production exceeds antioxidant defences. Nevertheless, ROS from high DO concentrations may cause oxidative stress (with high levels of ROS) in fish. ROS can cause cellular damage, leading to various health issues, including impaired immune function. ROS destroys lipids, proteins, and DNA, causing cellular dysfunction leading to contracting diseases or death. ROS formation rises with high DO level, temperatures, pollution, or metabolic stress. Oxidative stress can cause several cellular and physiological changes that endanger fish. It stunts growth, weakens the immune system, increases sickness risk, and oxidises tissues and organs (Chainy et al., 2016).
Such conditions have been reported in intensive aquaculture systems, which are known to maintain high fish populations (Akoll and Mwanja, 2012). Fish experience stress due to reduced DO levels, which can be caused by overcrowding or exposure to chemical pollutants. The available evidence suggests that the circumstances are conducive to the proliferation of endemic illnesses in the aquatic environment, as documented by Hershberger et al. (1999) and Carls et al. (1998). The significance of DO levels in pond water lies in its correlation with disease outbreaks, as evidenced by studies conducted by Null et al. (2017), Domenici et al. (2017), and Gallage et al. (2016, 2017). The occurrence of hypoxia-induced disease outbreaks has been found to have a negative impact on fish development and productivity, as reported by Abdel-Tawwab et al. (2019) and Shoemaker et al. (2000).
Oxygen is an essential component for the survival of all living organisms. However, the quantity of oxygen required by different organs may vary. Oxidative stress induced by O2 metabolites can cause additional damage to cellular biomolecules. As seen in Zebrafish, that exhibit oxidative stress resulting from exposure to a range of environmental stressors, including but not limited to chemicals, radiation, nanoparticles, pesticides, and heavy metals. According to Chowdhury and Saikia (2022), this animal model presents a higher degree of realism and reliability for exploring oxidative mechanisms in the domains of medicine, ecology, and the environment.
Avoiding oxidative stress and reducing ROS generation helps farm-raised fish thrive. When freshwater DO drops below 5–6 mg/L, aquatic organisms enter hypoxia (Dong et al., 2011). Anoxia and hypoxia, which promote stress, poor appetite, sluggish development, sickness susceptibility, and death (Abdel-Tawwab et al., 2019), reduce fish quantity, variety, and catch (Breitburg, 2002). Moderate hypoxia reduced blood oxygen saturation and pO2. At all times, acute and chronic moderate and extreme hypoxia decreased blood pH, pO2, total oxygen content, plasma Na+, and Cl− (Aboagye and Allen, 2018).
Several genes are involved in oxidative stress responses in fish. Due to its negative effects on fish health and production, oxidative stress poses a significant concern for the fishing industry (Isaak et al., 2012). Investigating genes implicated in oxidative stress in fish is a significant area of scholarly inquiry, as it can provide insight into the fundamental mechanisms of oxidative stress and stimulate innovative strategies for its mitigation. The antioxidant enzymes, Superoxide dismutase (SOD), Catalase (CAT), GPx, and glutathione reductase (GR) play a crucial role in the neutralisation of ROS and safeguarding cells against oxidative stress, as reported by Zhang et al. (2018).
Haemoglobin, an erythrocyte protein, is crucial in transporting oxygen throughout the circulatory system. Haemoglobin in fish has been observed to exhibit a potential function in scavenging ROS and safeguarding cells against oxidative stress. Heat shock proteins (HSPs) are a group of molecular chaperones that play a crucial role in the cellular stress response by safeguarding cells against damage during periods of stress, such as oxidative stress. The HSPs are known to have a crucial function in the process of refolding proteins that have been damaged or misfolded, particularly in response to oxidative stress (Szyller and Bil-Lula, 2021). Molecular biology methods allow researchers to uncover fish oxidative stress-reducing genes and their interactions with each other and the environment. Researchers currently employ transcriptomics, proteomics, and genomes to uncover fish oxidative stress genes and pathways and develop new management measures. Researchers are leveraging molecular biology to develop novel medications, technologies, and nutritional and environmental measures to minimize oxidative stress in fish.
5.2.3 Temperature, oxidative stress and fish health
In aquatic environments, the temperature is one of the abiotic factors that are crucial for maintaining fish growth, reproduction, behaviour, and health (Pereira et al., 2021, Table 5; Figure 1). The temperature has an impact on metabolic rates and biochemical reactions as well. Fish experience oxidative stress by changes in the temperature of the water, which may eventually lead to a disease outbreak.
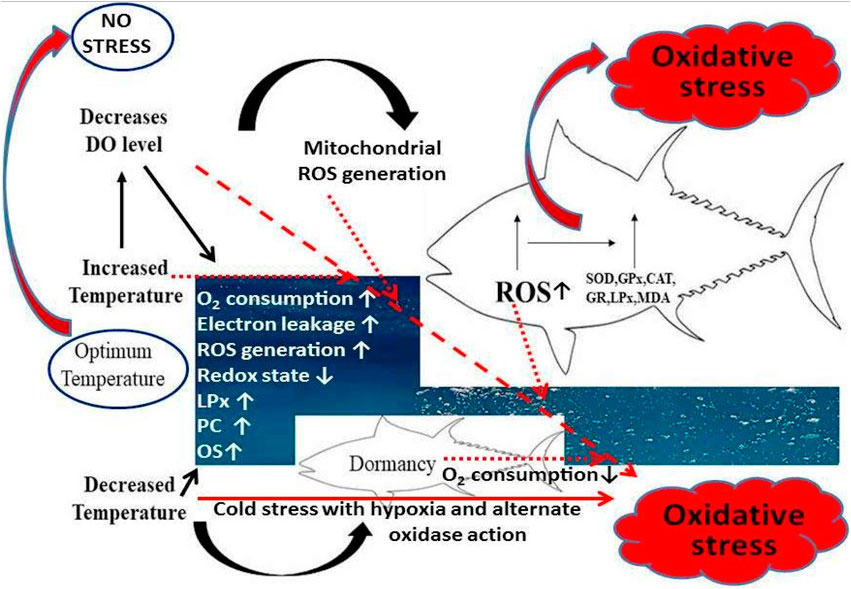
FIGURE 1. Effect of temperature on oxidative stress in fishes. In both salt and fresh water, temperature induces oxidative stress by increasing the rate of O2 consumption, electron leakage in electron transport chain in mitochondria that leads to increase in reactive oxygen (ROS) generation, the reduced redox state (lowing the activities of antioxidant enzymes such as superoxide dismutase, i.e., SOD, glutathione peroxidase, i.e., GPx and catalase and elevated lipid oxidation (LPx)or malondialdehyde (MDA) level, protein carbonylation (PC) and oxidative stress (OS).
Extremely high or low water temperatures influence the physiological processes of fish, like feeding habits, swimming, and immunity, and ultimately affect fish health and metabolism. In some species, continuous pathophysiological disturbances may cause the death of fish and cause significant economic losses in the aquaculture industry (Kozak, 2005). Abiotic stress, such as temperature extremes, can disrupt normal physiological processes in fish, leading to reduced growth, decreased survival, and even death (Dalvi et al., 2009). The high mortality rates and decreased production resulting from temperature-related stress can have a major impact on the profitability of fish farms and can cause significant losses for the aquaculture industry. Additionally, temperature extremes can also make fish more susceptible to disease, further exacerbating the economic losses in the industry. It is, therefore, important for fish farmers to monitor and regulate water temperatures to maintain optimal conditions for fish health and to prevent economic losses in the aquaculture industry (Brander, 2007; Dalvi et al., 2009; Anttila et al., 2013; Claësson et al., 2016).
It has been discovered that the temperature of the water and its DO content are sprightly correlated. It is well known that warm water has less DO than cold water. The rate of fish growth and feeding will be hampered because of low DO contents. The level of DO in water is indirectly proportional to the water temperature (Chakraborty and Krishnani, 2022). Temperature changes can cause the generation of ROS, impairing the immunity and antioxidant capability of the Tambaqui fish species from the Amazon (Pereira et al., 2021). The phenomenon in question has been postulated to occur through the modulation of mitochondrial O2 consumption rates in aquatic organisms (Paital and Chainy, 2014). According to a study on Dicentrarchus labrax fish to relate DO and temperature, Vinagre et al. (2012) found that CAT activity and lipid peroxidation (LPx) levels are tremendously complex to environmental temperature, especially at temperatures outside the optimal range of fish species. At 24 °C, also considered as the thermal optimum temperature of the species, CAT enzyme activity, and malondialdehyde (MDA) concentrations were at their low levels. The maximum levels of CAT activity and MDA concentration were noted in the fishes directed to temperatures above or below their optimum temperature, indicating that the heat stress influences how young D. labrax respond to ROS in their cells (Vinagre et al., 2012). Fish at temperatures above or below their thermal optimum had been seen to have more MDA levels than normal and enzymatic (CAT) activity, demonstrating a relationship between the stressors related to temperature, ROS, and the cells of D. labrax.
Major antioxidant enzymes SOD and CAT were tremendously upregulated in response to an elevated temperature. Other parameters, such as lysozyme and phagocytosis, were upregulated in response to the 18 °C temperature (Kim et al., 2017). A study by Kim et al. (2017) revealed that the temperature has an impact on the ability of young sablefish (A. fimbria) to grow and that extreme temperature can compromise the immune and antioxidant system in the same (Kim et al., 2017). The oxidative damage leading to LPx has been linked to long-term temperature stress. Long-term temperature exposures of fish result in upregulated antioxidants such as SOD, CAT, GPx, GR, and glutathione-S-transferase (GST), which are more apparent under heat stress than cold stress. This suggests that physiological pathways are being triggered to scavenge the ROS formed during heat-expedited stress levels (Madeira et al., 2013; Topal et al., 2021).
In Carassius auratus L. (also called goldfish), activities of glucose-6-phosphate dehydrogenase (G6PDH), SOD, CAT, GPx, GST, and GR were recorded depending on the exposure of temperature, i.e., high temperature (35°C) for 1–12 h and low temperature (21°C). The enzyme activity level of SOD was found to be increased at 35°C in all types of tissues (brain, liver, kidney), though it did not follow the pattern at repossession. After an hour of heat shock exposure, the SOD, CAT, GPx, and G6PDH activities in some tissues drastically dropped, supporting the theory that heat likely inactivated those enzymes. In several instances, enzyme activities are seen after 4 hours of exposure. Further same study from Lushchak and Bagnyukova (2006) correlated SOD and LPx. This indicated that these parameters might be up-scaled and help in antioxidant defence (Lushchak and Bagnyukova, 2006).
A study by Pereira et al. (2021) suggested that immunological function, the antioxidant system, and oxidative stress are all affected by the relationship between photoperiod and temperature in the fish Tambaqui bernacchii. The development of species is observed to be impeded when subjected to prolonged photoperiods or when such conditions are coupled with elevated temperatures, as these circumstances potentiate cellular damage and potentially compromise the immune system. The outcomes of this study, therefore, suggest that the cultivation of T. bernacchii ought to be conducted under photoperiods shorter than 18L:6D and within the temperature range of 29°C–32°C (Pereira et al., 2021). According to a study by Enzor and Place (2014), antioxidant enzymes (SOD, CAT) can be correlated for sea fishes using different parameters like treatments and tissues. The low pH and higher temperature of the seawater pushed ROS significantly high for a shorter duration. Interestingly, long-term acclimatisation made no visible changes in antioxidant levels in T. bernacchii, though there were no cellular damages seen. Their findings indicated that notothenioid fish counteract ocean conditions using their antioxidant system (Enzor and Place, 2014).
Temperature and DO have a significant part in acclimatisation adjustments during vigorous exercise. The ability of marine species in Antarctica to adapt to temperature fluctuation is thought to be quite constrained (Paital et al., 2016). Robinson and Davison (2008) demonstrated that this theory does not apply to the Antarctic notothenioid fish Pagothenia borchgrevinki. With a 5°C temperature increase, P. borchgrevinki was able to adapt its resting metabolic rate and resting breathing frequency. The pattern of oxygen use was not seen as significant either in cold- or warm-acclimated fish (Robinson and Davison, 2008). The oxygen-utilizing patterns were found similar in cold or warm acclimated fishes.
Dormancy, or reduced activity and fasting, is observed in temperate fish during cold temperatures or overwintering (Figure 1). This is similar to what is seen in many centrarchid sunfishes, such as cunner, American eel, and brown bullhead (Ameiurus nebulosus) (Reeve et al., 2022). The hormone level of orexin, also a regulator of feeding behaviours, is seen increasing at higher temperatures in Carassius auratus and Gadus morhua. The study also indicated that water temperature directly affects fish-feeding hormones. In brain CART and leptin, PYY hormone in the intestine also gets influenced by the decrease in temperature.
A comparative study between Gadus morhua and Amphiprion ocellaris shows that food intake decreases if water temperature drops. Whereas change in A. ocellaris feeding behaviours was noticed at 32°C (higher) than fish reared at 28°C. The red-spotted grouper and other fish species exhibited a commensurate response, whereby food consumption was elevated within the temperature range of 15°C–20°C (Volkoff and Ronnestad, 2020). Intestinal cholecystokinin (CCK) expression and temperature are correlated in fish species. Vertebrate digestive physiology depends heavily on CCK, which is produced and secreted by enteroendocrine cells in response to food in the gastrointestinal tract. An increase in CCK level was noticed with increasing temperature. Their study was comparable to the findings of Butt et al. (2019). Their group also indicated that the increase in temperature can be correlated with the food eating pattern. The same was noticed in black tetra for intestinal CCK expression (Butt et al., 2019).
Additionally, studies indicate that thermal pollution significantly increased the total copies of the Vibrio gene and the proportion of the marine fish pathogen Photobacterium damselae in the P. sexlineatus hindgut microbiome. These findings support the hypothesis that increased water temperature causes estuarine fish gut dysbiosis. P. damselae damselae is supposed to engage in unscrupulous proliferation in estuarine fish guts under stress induced by temperature changes, but there was never any P. damselae damselae in seawater through the learning and thermal pollution ought to not affect the microbial communities or copiousness of the Vibrionaceae linked with the water sample obtained from an ocean (Suzzi et al., 2023). Research on the impact of temperature on fish sensory systems caused by the human state high temperatures change how the central nervous system processes sensory information, speeds up neuron signalling, and produces more ROS.
Temperature also affects the pH of water, and low pH disrupts otolith growth, impairs vision and smell, and interferes with central nervous system operation are well studied (Lefevre et al., 2021). In their studies, Lu et al. (2005) indicated that the high temperatures speed up neuron signalling, change how the central nervous system processes sensory information, and increase ROS levels. Low oxygen inhibits the synthesis of energy, slows down nerve conduction, impairs eyesight, and induces apoptosis in the brain indicated previously by Rummer et al. (2013) and Damsgaard et al. (2020).
5.2.4 Hardness, oxidative stress and fish health
Hardness is the total concentration of mainly Ca2+ and Mg2+, i.e., divalent cations. It is commonly advocated for aquaculture to have some water hardness (25–100 mg/L as Ca2+) because of the fundamental functions and effects of Ca2+ ions. It is a crucial factor that influences the absorption and elimination of metals within aquatic systems. The bioavailability of metals and, as a result, their toxicity in aquatic species is significantly influenced by hardness. According to Romano et al. (2020), the bioavailability of metals rises in soft water, making them more hazardous than in hard water. There was a notable augmentation in hardness, which correspondingly signified a reduction in the activity of Na+/K+-ATPase in fish (Romano et al., 2020). The enzyme in question experienced a significant decrease in activity primarily as a result of Cd exposure, thereby leading to a notable decline in major hardness. Though Mg and Na ions were found to have significantly changed, infecting the ion exposure in various water conditions, such as hard and soft, also affected Na+/K+-ATPase activities in gills. Interestingly, increased fish mortality in soft water indicated its direct impact on hard water conditions (Saglam et al., 2013).
An exceptional study by Romano et al. (2020) established that water hardness is not required for Micropterus salmoides juveniles. Those studies’ benefits were yet to be confirmed, which may lead to exemplary fewer feeding costs (Romano et al., 2020). At 900 ppm water hardness, Poecilia reticulata significantly increased in size (p = 0.005). The fertility and gonadosomatic index of P. reticulata were both considerably greater. Water hardness is understood to harm breeding in Betta splendens species. The study demonstrated varied effects of water hardness on the test species P. reticulata and B. splendens intern, significantly improving larval growth (Krishnakumar et al., 2020).
In a study, the regulation of caspase-3 and metallothionein activity, activity of NO, and overall antioxidant activities were noticed as stress indicators in goldfish (C. auratus) exposed to various Zn concentrations and hardness of water for 14 days. Results from the TUNEL assay indicate that high water hardness can inhibit apoptosis and accumulation of Zn. The authors concluded that high water hardness can affect how much zinc is absorbed, and lowering the hardness can lessen the toxic stress that zinc causes (Choi et al., 2022). According to research, even though channel catfish grow poorly in water hardness up to 1,250 mg/L CaCO3, it can counteract the growth-inhibiting effects of high environmental ammonia (HEA). This was partially attributed to 1,250 mg/L CaCO3 shielding the gills and reducing HEA-induced disruption of the ion- and ammonia-regulatory systems. Elevated hardness, however, might be less effective at reducing catfish (Ictalurus punctatus) salinity stress (Sinha et al., 2022). Oppositely, water hardness of nearly 1000 ppm may be considered as protective factor in the cat fish Heteropneustes fossilis against low salinity (3 to 9 ppt) induced stress (Bal et al., 2021b).
According to research on the fish Ictalurus punctatus, when exposed to high hardness levels, fish diminish their ability to absorb calcium through their gills (Ca2+-ATPase and Na+/K+-ATPase activity). An unexpected rise in muscle water in fish subjected to 4,000 mg/L CaCO3 showed that the osmoregulatory capacity was substantially impaired. The same was true for blood levels between 3,000 and 4,000 mg/L, which resulted in an iron deficit and a corresponding reduction in haematological indices of respiratory dysfunction (HCt and Hb value) (Limbaugh et al., 2021). Under very hard water, Capoeta fusca is protected from the toxicity of Co and Ni, whereas the fish are more vulnerable to it in hard water (Pourkhabbaz et al., 2011). According to a study, increasing or cumulative H2O hardness stays an effective way to lessen the stress retort in goldfish (Carassius auratus) brought on by Zn (Li Z. et al., 2022). Research on Danio rerio embryos states that adding humic substances below lower hardness/alkalinity states can support plummeting toxicity and is hazardous to the fish (Liu et al., 2021).
Investigations were also conducted on fish treated with ethoxylate and the impact of cations on ethoxylate toxicity. Compared to soft water (SW), ethoxylate is slightly less harmful in hard water (HW). The evidence suggests that the toxicity of ethoxylate is independent of the cation composition of a particular hardness. The vulnerability of fishes to non-ionic ethoxylate detergents is unaffected by their capacity to adjust to different water hardness (Tovell et al., 1974). The number of hazardous effects that anionic detergents in solution have on fish mostly depends on how hard the water is that the fish are being treated in. The HW absorbs detergent more quickly than SW, and salmon and goldfish are more sensitive to sodium lauryl sulphate in HW than in SW. It has been established that the toxicity of sodium lauryl sulphate is directly influenced by the concentration of bivalent cations. Acclimatization studies have shown that the hardness of the water used to treat the fish, as well as the hardness of the water used to acclimate the fish in the first place, both affect the toxicity of sodium lauryl sulphate (Tovell et al., 1974).
Abiotic variables in freshwater and ocean environments are rapidly changing due to human activities. Along with oxidative stress, salinity, and hardness-induced stress are key stress factors in aquatic species that eventually affect normal physiology. As a result, ecological studies are greatly influenced by oxidative stress and the physiology it generates. Both the production of ROS and the functions of the enzymes depend on the species and tissue. Osmotic stress caused by salinity accelerates physiological procedures such as preserving ionic and osmotic equilibrium in the specialised regions of distinct excretory organs. The control of ions depends on gills in addition to gas exchange. The organism-level reflexes to cellular responses, such as the ability to survive and reproduce, altered transcriptional levels of various enzymes, and altered gene expression, are connected to the salinity shift-induced altered physiology.
5.2.5 Alkalinity, oxidative stress and fish health
Another important characteristic regularly evaluated to gauge water quality is total alkalinity, which includes all acidimetric titratable species down to pH 4. Importantly, the bicarbonate/carbonate concentrations have been identified as the main contributors to total alkalinity. This factor enables a crucial environmental context for comprehending phenomena like acidification, the spread of organisms, and the water quality suitable for ingestion by humans (Wiorek et al., 2021). Carbonate alkalinity, a significant environmental stressor that can swiftly cause oxidative stress and hypo immunity in fish, substantially impacts the structure of aquatic feed. In addition to exerting effects on the growth, immunity, and disease resistance of aquatic organisms, prolonged exposure to carbonate alkalinity stress can also influence the in-vivo nutrition metabolism of fish (Fan et al., 2021, 2022).
When water carbonate alkalinity increases significantly, it decreases SOD and T-AOC activities in the intestine and significantly increases MDA contents in fish. In contrast to carbonate alkalinity of 0 mmol/L, carbonate alkalinity of 15 mmol/L can significantly reduce SOD and T-AOC activities while increasing the MDA content (Fan et al., 2021, 2022). A study on the combined effects of alkalinity and salinity on Luciobarbus capito found that an elevation in alkalinity with different concentrations from 15 mM to 60 mM significantly increased plasma potassium ions and urea nitrogen concentrations but had no impact on both plasma osmotic pressure and gill filament ATPase activity. In conclusion, salinity was found to be the factor that had the greatest impact on osmotic pressure, followed by alkalinity (Geng et al., 2016).
The alkalinity-induced stress for longer periods shows that metabolic profiles are significantly impacted by alkalinity exposure. In fish exposed to alkalinity, metabolites related to amino acid metabolism pathways such as serine, phenylalanine, glycine, tyrosine, threonine, methionine, cysteine, and also pyruvate, butanoate metabolism were found to have differentially expressed levels. Seven different metabolite structures were found to be significant indicators of exposure to alkalinity (Sun et al., 2018). When the pesticide -trifluoromethyl-4-nitrophenol (TFM) was used to control Petromyzon marinus (invasive sea lamprey) populations in the Laurentian Great Lakes, TFM caused non-target mortality of lake sturgeon (Acipenser fulvescens). The study found that as alkalinity increased from a low concentration of 50 mg/L to a medium concentration of 150 mg/L, TFM uptake decreased before reaching a concentration of 250 mg/L as CaCO3. 3–6 h after TFM exposure, all fish at low alkalinity perished. TFM-exposed fishes with only medium alkalinity had slower death.
In fish (A. ful and P. marinus), survival was approximately 75% between 4.5 and 7.0 h after exposure, then it decreased to slightly more than 50% after 15 h, and there was no further mortality after that. But because alkalinity typically rises with water pH, the correlation between TFM toxicity and alkalinity is more complex. Nonetheless, the association between TFM tolerance of sea lamprey larvae and alkalinity is overdone when connected with pH (Hepditch et al., 2019). In many cellular responses to various environmental stressors, HSPs play a crucial role. Concerning gill alkalinity stress and salinity shift, it was found that the coordinated expression of LmHsps in Lateolabrax maculatus (spotted sea bass) occurred. The results showed that under various regulatory situations, the effects of alkalinity stress might be triggered by the LmHsp40-70–90 recruitment of co-chaperons adaptively, as HSPs are expressed in a variety of ways in L. maculatus (Li et al., 2022b). The current data in hardness imply that there is an additional grey area that needs to be studied concerning ROS. The osmoregulatory patterns must be evaluated using a variety of ecologically relevant concentrations of the examined substances, like ion concentrations, with a type of water.
5.2.6 Salinity, oxidative stress and fish health
Aquatic species may undergo physiological changes and experience stress when their natural habitat is impacted by several variables, including temperature fluctuations, oxygen levels, pH levels, pollution, salinity, and other environmental stressors. It generally triggers them to fight these stress responses by adapting to changes (Chainy et al., 2016; Paital et al., 2016; Fras-Espericueta et al., 2022). When there is a change in the amount of dissolved salts or ions in the surrounding water, fish endure salinity stress, and when salinity is combined with other factors, it can be manifested changes at biochemical and histopathological levels in fish (Banan et al., 2020b, 2022). This can happen naturally, like when the tides change or in an estuary, or it can be caused by people, like when runoff from farms or land-salted water gets into the river, ponds, or other freshwater sources (Xing et al., 2022).
Fish that are regularly exposed to a certain range of salinity levels endure stress if the salinity levels alter significantly in a sudden manner (Li et al., 2022c, Figure 2). For example, a fish that normally lives in freshwater may experience salinity stress if it is suddenly exposed to salt water. Long-term exposure to high or low salinity levels can cause electrolyte imbalances and associated difficulties. Similarly, polluted water or having certain diseases can also cause salinity stress. Understanding these causative factors and adopting adequate measures to counteract the salinity stress, either to stop or lessen it, can help ensure that fish in aquatic environments are healthy and happy, which in turn will avoid economic loss (Moniruzzaman et al., 2022).
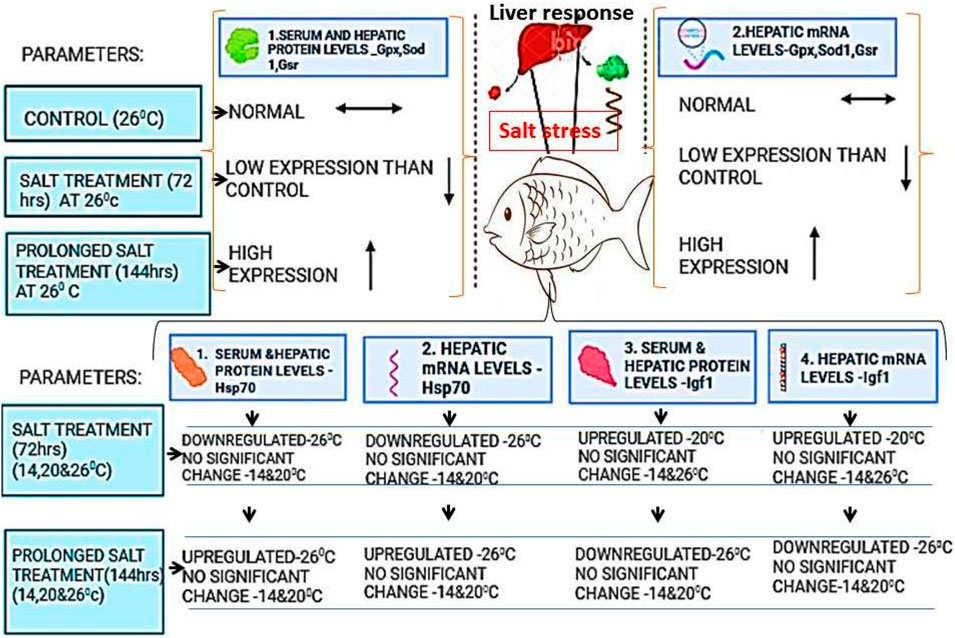
FIGURE 2. The marker protein and mRNA expression in liver and blood serum under salt stress conditions. Under short term or long term salinity stress, molecular markers such as transcript and translation level of Hsp70, Igf1 are found to be differentially regulated as shown in the figure in fish hepatic tissues. Thus they can be used as biomarkers of salt stress in fish.
Salt stress can cause changes in osmotic pressure, problems with a fish’s metabolism, stunted growth, and fewer offspring, all of which hurt the profitability of fish farming in the long run. Nile tilapia can handle less-than-ideal growing conditions like low oxygen and high salt levels better than other finfish (Dawod et al., 2021; Worldfishcenter, 2023). They examined Nile tilapia in the laboratory for 4 weeks under 0, 10, or 20% salt, and findings represented that Nile tilapia grows slower in high salinity. The salinity also causes inflammation in the gills, intestines, and liver tissue, which causes less functioning of organs. Conversely, in normoxia, Nile tilapia could endure 10% salinity, but in hypoxia, 20% of salinity produced oxidative stress, apoptosis, and inflammation in the intestines, gills, and livers. Furthermore, the detection of inflammatory gene transcription, IL-8, IL-1, pro-inflammatory genes (IFN-γ, TNF-α), and the caspase-3 gene for apoptosis, highlighted the effect of high salinity on functionality and reduced Nile tilapia productivity, resulting in significant economic loss.
A change in temperature and pH may lead to the salinity of the water. Abnormal changes in salinity levels can impact the osmoregulatory system of fish, leading to stress and reduced growth. Water salinity is a major abiotic element affecting fish’s humoral defense and survival. The fish’s development, reproduction, and health might suffer if the water’s salinity is too high. In extreme cases, high or low salinity can lead to mortality. Salinity variations in aquaculture can alter the microbial ecology, increasing disease risk and water quality. High salinity also increases heavy metals and other hazardous compounds in the water, harming fish. Hence, salt levels in fish production systems must be monitored and controlled to reduce the possibility of negative effects on fish health and aquaculture economics (Dominguez et al., 2004; Abou Anni et al., 2016; Vargas-Chacoff et al., 2018).
Salinity is one of the important factors that, alone or with multiple stressors, influence redox regulation, like the superoxide dismutase activity in aquatic animals (Paital and Chainy, 2013). An increase in water salinity affects oxidative stress and allied physiology in the survival of freshwater fish species. The feed conversation ratio and specific growth factors are found to be elevated and alleviated in the freshwater fish when exposed to higher salinity of 9 ppt than its natural freshwater (Bal et al., 2021b). Besides the above, gain in body weight, increase in total body length, standard length, length of snout to pectoral fin, caudal fin length, length of caudal peduncle, dorsal to ventral body height are also decreases when the fish was exposed to a gradual increase in salinity from 0 to, 6 and 9 ppt. The total basophil, haemoglobin, and RBC content were also alleviated with the rise in salinity. All these changes were found to be accompanied by histological damage in the fish at the liver, gills, and muscle levels. Finally, the tissues were also found to have a gradual hike in lipid peroxidation levels as a function of salinity (Bal et al., 2021b).
Hormones released by the organs are responsible for feeling more (orexigenic) or less (anorexigenic) hunger. Their release is controlled by a complex network that uses signals from the brain and other organs (Conde-Sieira et al., 2018). Some of the most important hormones for controlling hunger are neuropeptide Y (Npy), cholecystokinin (Cck), peptide YY (Pyy), and cocaine and amphetamine-regulated transcription factor (Cart) (Rønnestad et al., 2017). Martins et al. (2022) showed that under the influence of high or low salinity, the expression of genes for hunger is changed. This change and other adaptive responses could have led to a drop in body mass index (BMI), specific growth rate (SGR), and final weight. Blood cells were also found to be damaged by oxidation when there was much salt in the body.
Salinity stress is a major environmental factor that declines the health and growth of fish, damaging the aquaculture economy. Scientific investigation to determine the signals of stressors due to global warming at the earliest is essential; thus, expression of stress-associated biomarkers was evaluated by Eissa et al. (2017) in yellow perch, who quantified the serum and hepatic protein levels Hsp 70, IGF-1, GPx, SOD1, and Gsr and their mRNA levels in the liver tissues. Exposure of yellow perch with salt caused a temporary reverse effect on the biomarkers chosen for the study. The expression level of Igf1 is upregulated and hsp70 is downregulated under salinity stress up to 3 days and the results are reversed under long term salinity stress (Eissa et al., 2017, Figure 2). These enzyme levels in fish samples thus could be potential biomarkers for the diagnosis of early signs of salt stress that negatively impacts fish survival in aquaculture, which may enable us to address the stress and enhance the economic profitability of fisheries.
5.2.7 pH, oxidative stress and fish health
Farmed fish need acceptable water quality, comprising appropriate pH, dissolved O2, CO2, NH3, and nitrogen compounds within species-specific limitations. High pollution, dissolved oxygen, and toxin levels can stress and harm fish. Unionized ammonia passes through most cellular membranes but not ionised ammonium. Similarly, fish gills retain endogenous ammonia or passively diffuse it depending on pH (Evans et al., 2005). Concentration and pH determine ammonia toxicity leads to a toxic pH level that can damage fish gills and induce respiratory distress. Abrupt pH changes can stress fish and decrease their capacity to resist diseases. A maximum number of fish species prefer pH between 6.5 and 9.0; others can tolerate a greater range. Low pH can injure fish gills, skin, and eyes by disrupting their sensitive ion balance. Yet, a pH level too high (basic) might prevent fish from absorbing oxygen and induce stress or death. It was found that acute ammonia intoxication causes neurological disorders in fish (Yavuzcan Yildiz et al., 2017).
Acidification in the freshwater environment has become a global issue due to increased CO2 levels, acid rain, soil acid leaching, pollution, and anthropogenic activities in oceans (Schindler, 1988; Harding, 2012). Changes in pH induce oxidative stress in aquatic species and affect development and growth, physiological processes, immune system, and changes at the molecular level in fishes, which can lead to oxidative stress accumulation (Figure 3). Hydrogen ion concentration present in water makes water acidic, alkaline, or neutral; this is an important parameter in quality assessment of water (Boyd et al., 2011) for fish growth. The decrease in pH level is primarily caused by the change of ionized NH4+ to NH3+ after excretion from animals, and the reason for the increase in pH level is mainly by carbon dioxide release (Duarte et al., 2013). A change in pH has a negative effect on larval health and early development in Fishes (Kikkawa et al., 2003; Baumann et al., 2012). Some of the attributes, such as larval deformities, nonviability of eggs, prolonged incubation time, etc., have already been reported in many studies (Ferreira et al., 2001; Reynalte-Tataje et al., 2020).
The alteration of carbon chemistry and the increase in anthropogenic CO2 emissions have caused 30% of CO2 to be released into the atmosphere and subsequently absorbed by aquatic environments (Doney et al., 2009), resulting in the acidification of oceans and adversely affecting fish physiology. Specifically, the influx of H+ ions through the gills has led to a decrease in the ability of haemoglobin to effectively bind with oxygen (Fromm, 1980a). Consequently, this has resulted in haematological disorders and disrupted levels of magnesium, glucose, haematocrit, glutamic-oxaloacetic transaminase, and glutamic pyruvic transaminase in fish (Ultsch et al., 1981; Scott et al., 2003; Das et al., 2006). The current oceanic water pH is 8.2 and is anticipated to further decrease to 7.7–7.8 by 2,100 and 7.4–7.5 by 2,300 due to acidification (Caldeira and Wickett, 2003), which in turn could affect physiological homeostasis in fish as changes in ocean pH cause increase in reactive oxygen species and induces oxidative stress among the fish population (Lee et al., 2022). High pH also causes deleterious effects on fish health as it hinders oxygen transport to tissues (Aride et al., 2007). The pH above 9.5 causes increased ammonia concentration in plasma, followed by mortality (Ghanbari et al., 2012). The activity of superoxide dismutase and glutathione–S-transferase, two key enzymes involved in oxidative stress, are reported to be altered with changes in pH (Kim et al., 2019; Kim et al., 2023).
Though antioxidant enzymes such as SOD and CAT scavenge the ROS, under stressful environment activity of these enzymes is decreased/inhibited, which may result in DNA destruction, activation of caspases, and impaired protein functions in aquatic animals (Lesser, 2003). The pH change can also cause oxidative stress in fish (Figure 3). Sudden change in pH values of water also affects immune response by altering cellular and humoral response among fishes due to susceptibility to acidification and/or alkalization of their surroundings (Zhao et al., 2010). IgM is considered a critical immune biomarker to evaluate toxic effects due to pH and other environmental changes (Li and Chen, 2008; Kim J. H. and Kang J. C., 2016, Kim N. and Kang, S. W. 2016). Other parameters such as glucose levels and count of WBC (white blood cells) and RBC (Red blood cells) have also been evaluated to have fluctuated with changes in the pH of water in Danio rerio (Zahangir, 2015).
Significant increase in ROS affects DNA, Proteins, and lipids and causes hereditary diseases in aquatic animals (Wang et al., 2002; Kim et al., 2019) due to increased Calcium ion influx from the extracellular environment into the cell, as ROS also acts as sensors which activate the Ca2+ influx inside the cell leading to chromatin damage and subsequent cell death. Acidification has also been reported to induce P53-mediated Apoptosis in tilapia (Ermak and Davies, 2002; Mai et al., 2010).
5.2.8 Heavy metals, OS, and fish health
Many populations worldwide rely on fish for daily meals because fish and seafood are beneficial components of human nutrition and provide necessary nutrients like proteins, vitamins and minerals, and polyunsaturated omega-3 fatty acids (FAO, 2023). In some places, fish can serve as a primary food source and contribute considerably to food security (FAO, 2023). Yet, fish and other marine animals are impacted by the ecology in which they live. Living beings can take in both useful and harmful environmental minerals and metals through the water, sediment, and food they ingest (Ebdon et al., 2001). Although eating fish and shellfish has many health benefits, it can potentially drastically jeopardise consumer health due to the pollutants. Metals and metalloids are the most frequently accumulated toxins in fish and shellfish that could result in health issues when taken in amounts over the advised safe quantity (Llobet et al., 2003; Falcó et al., 2006).
The existence of heavy metals in aquatic environments is a subject of significant concern due to their propensity to deposit in aquatic organisms, for example, in the fish Aplocheilus panchax (Rath et al., 2021; Paital et al., 2022). It is noted that the accumulation of heavy metals has a negative impact on aquatic life. The accumulation of heavy metals leads to several adverse consequences, such as oxidative stress, inflammation, histological damage, and genetic anomalies (Amoatey and Baawain, 2019; Qi et al., 2022). The toxic levels rise as heavy metals enter the body through permeable membranes (such as the gills) and inhibit several activities, such as by substituting the native required metal ions of the metabolic enzymes, disrupting protein structures, and creating DNA-crosslinks which ultimately affects the cell cycle (Garai et al., 2021; Dey et al., 2023). Seafood is becoming more popular despite its toxicity, and consuming heavy metals containing aquatic organisms can have similar effects on people, including cardiovascular and peripheral vascular diseases and harm to the brain system, the liver, the kidneys, and reproduction (Engwa et al., 2019). Since the spread of HMs in the food chain cannot be stopped, effective methods must be found to remove heavy metals from aquatic species before consumption. Metals in the aquatic environment can be found in dissolved, particulate, or chelated/combined forms in water, sediment, or aquatic creatures (Naggar et al., 2018; Amankwaa et al., 2021).
5.2.9 Water pollutants, OS, and fish health
According to Alrumman et al. (2016), water pollution happens when undesirable pollutants enter bodies of water, change the water’s quality, and impact ecosystems and human health (Briggs, 2003). Water is a precious natural resource used for drinking and other purposes related to development. Moreover, it is vital to the recycling of nutrients. Normally, sewage and hazardous waste are disposed of, recycled, and any overflow is drained into the ocean via aquatic systems. The abuse of water resources for various anthropogenic activities such as agriculture, industrial processes, and thermal generating plants to fulfill the needs of the enormous population results in much less of its assimilative volume due to the rise in pollution levels. The dual strain placed on the water bodies eventually causes the existing biological populations to perish.
Water pollution can harm fish, causing the fish business to lose money (Figure 4). Polluted water can include dangerous chemicals, poisonous compounds, and infections that can kill fish, decrease growth, and cause sickness. Water quality degradation reduces dissolved oxygen, which fish need to survive. Hence, water contamination can affect fish growth, yields, and industrial expenses. Severe water pollution may wipe off fish populations, hurting the fish industry. Wastewater treatment, agriculture best practices, and plastic waste minimization boost profit in the fish business by reducing disease risk and improving fish health and production by safeguarding aquaculture water quality (Austin, 1998).
One of the most important aquatic populations for people often includes fish species. Any inadvertent alteration to an ecosystem’s natural characteristics brought on by adjustments to its physical, chemical, and biological components is referred to as “pollution” (Subhendu, 2000). Aquatic ecosystems are vulnerable and at great risk as a result of a large number of pollutants coming from home, urban, and industrial sources, as well as various farming practices that leak contaminants into the river system (Byrne et al., 2015; Kaur and Dua, 2015; Pinto et al., 2015).
Heavy metals and insecticides are the most prevalent pollutants, especially in aquatic ecosystems (Khoshnood, 2016). The main ways pesticides used in agricultural activities are directly released into the open environment are drift spray, volatilization, and wind erosion of soil (Qiu et al., 2004). Certain pesticides that are present in aquatic ecosystems may have an impact on the life cycles of aquatic organisms (Ventura et al., 2008). It has been discovered that some aquatic organisms can concentrate the hazardous solutes from their surroundings without visible harm to themselves, acting as pollution amplifiers and giving access to predators to dangerously high concentrations of the toxic substances. Environmental pollutants’ harmful impacts on fish health and consumers have been discussed in a few specific contexts. As a result of the heavy toll that increased human activity is taking on the aquatic ecosystem, there is an urgent need for research into the harmful impacts of water pollution and the potential risks they pose to aquatic organisms and their ecosystems. Several scientific investigations have shown that different types of water pollution negatively affect the aquatic populations that inhabit water bodies. The aquatic ecosystem is expected to undergo significant changes due to diminishing fish numbers and a partial stop to commercial fishing (Hinton and Lauren, 1990).
The fish population is one of the most sought-after sources of high-quality protein for humans. The aquatic organism’s altered shape, tissues, and metabolic profile highlight the numerous types of stress and adjustments to the surrounding ecology. For instance, some fish species respond swiftly and cause several alterations in various body parts and organs, primarily the gills, kidneys, and liver (Bukola et al., 2015). Several histological changes in different fish species have been noticed and validated as biomarkers to gauge the extent of pollution. Due to a variety of factors, including expanding human populations, industrialization, urbanisation, the clearing of forests, a lack of environmental awareness among society, the failure to put policies, rules, and regulations into place, and the discharge of effluent from various industries, aquatic ecosystem pollution ultimately leads to the loss of aquatic organisms. The contaminants from various industrial discharges and sewage sink into the earth, contaminating groundwater supplies and damaging rivers and reservoirs’ surface waters.
Aquatic habitats are burdened by pollution due to rising urbanisation and an increased rate of population (Edokpayi and Nkwoji, 2000; Nkwoji et al., 2010). The pollutants affect physicochemical, flora, and hydrological characteristics and pose major risks to aquatic ecosystems (Nkwoji et al., 2010). The most serious issue facing developing nations is the improper treatment of the enormous amounts of waste materials created by various anthropogenic activities. Their main challenge is the hazardous discharge of these effluents into freshwater bodies, like rivers and reservoirs, which can no longer be used as primary or secondary natural resources. Pollutants have an impact on aquatic organisms’ behaviour both directly and indirectly (Zala and Penn, 2004; Saaristo et al., 2018), particularly fish species (Robinson, 2009; Sloman and McNeil, 2012). Various pollutants, encompassing both inorganic and organic substances, exert an influence on a diverse array of behavioral patterns, including but not limited to feeding habits, reproductive activities, and social interactions, encompassing both amicable and aggressive behaviors. Certain pollutants may have caused changes in the neurotransmitter, hormone, and cholinesterase activities of several fish species (Brodin et al., 2014; Vindas et al., 2017).
Pollution-induced alterations in fish behaviour have the potential to increase pollutant exposure even further and produce positive feedback loops that point to the detrimental impacts of pollution on fish health. Water contamination has a consistent impact on a variety of spatial behaviour patterns, including activity, exploration, and avoidance. Aquatic creatures that spent most of their lives in environments with significant metal contamination (such as lead and cadmium) had reduced exploratory inclinations (Grunst et al., 2019). The diminished exploratory tendencies of fish have adversely affected their ability to assess the quality of their habitat. Exploration plays a crucial role in enabling people to gather data and indicators pertaining to their activities. Water contamination consistently affects various spatial behavior patterns, such as curiosity and avoidance, among others.
Aquatic animals that lived most of their lives in habitats with heavy metal contamination (such as lead and cadmium) had a decreased propensity for exploration (Grunst et al., 2019). Such contaminations have affected the ability of fish to judge the quality of their habitat because exploration is the main trait that enables individuals to receive information and clues about their surrounding ecosystem (Reader, 2015). Moreover, these pollutants frequently change community interactions that reduce social learning and information gathering from their conspecifics (Brown and Laland, 2003). The capacity for learning and spatial memory is both significantly impacted by pollutants. For instance, aluminium pollution in Atlantic salmon affected their ability to learn in a labyrinth test as well as how they processed information and responded to unfamiliar situations (Grassie et al., 2013). Moreover, pesticides have some effects on the spatial memory of Gobiocypris rarus and Danio rerio.
5.2.10 Anthropogenic activity, OS and fish health
Various anthropogenic inputs leading to salinity fluctuations and a stressful aquatic environment have been reviewed (Bal et al., 2022). Major anthropogenic activities led to many insults, including oxidative stress in fish (Figure 5; Table 6). Ecotoxicological studies to assess the impact of contamination by human activities in aquatic environments worldwide–both marine and freshwater - used oxidative stress biomarkers in fish (Nwabueze et al., 2020; Makuvara et al., 2022; Filice et al., 2023; Hore et al., 2023). Exposure of haarder fish to tributyltin chloride (TBTC), a chemical contaminant, resulted in decreased antioxidant enzymatic activity and increased malondialdehyde (Zhao et al., 2022). Transcriptomic studies on Volatile organic compounds (VOCs) such as benzene in BTEX mixture from vehicle exhaust and industrial emissions it has been shown that 1 ppm benzene dysregulated genes of oxidative stress in zebrafish (Wu et al., 2022). Ferrocene exposure in rainbow trout exhibited lipid peroxidation and antioxidant enzyme activities inhibition; thus, increased iron levels as a result of mining and industrial activities in freshwater ecosystems need monitoring (Özgeriş et al., 2023). Pharmaceuticals - another anthropogenic contaminant - studied by Diclofenac exposure in Gasterosteus aculeatus through metabolomics-based approaches showed that the antioxidant system is impacted in male sticklebacks (Lebeau-Roche et al., 2023). Herbicides and OA group toxins - okadaic acid and dinophysis toxin-1 have been shown to affect the juvenile/larval forms of freshwater African catfish and medaka, respectively, by altering antioxidant systems (Anih et al., 2023; Figueroa et al., 2023).
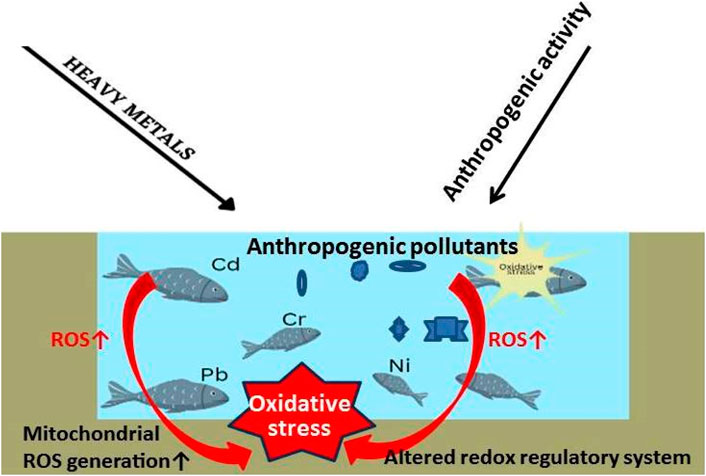
FIGURE 5. Anthropogenic induced heavy metals and other pollutants for induction of ROS and oxidative stress in fish.
Microplastics (polyethylene (PE), polystyrene (PS), polyvinyl chloride (PVC)) and microfibres represent another major anthropogenic contaminant in water bodies that can induce oxidative stress responses (Thakur et al., 2022; Subaramaniyam et al., 2023). Microfiber plastics released by cloth washing and wastewater treatment plants cause oxidative stress and cell damage in medaka fish exposed to 500 or 1,000 polyester fibres/L of for 21 days, evidenced by increased mRNA expression of SOD, CAT enzymes from day 10 and plasma malondialdehyde content from day 7 respectively (Kim et al., 2023). In another study, PE induced oxidative stress in carp gills and increased expression of NF-kappaB and NLRP3, causing apoptosis and inflammation (Cao et al., 2023). Interaction of microplastics with other environmental irritants also poses a serious threat to aquatic organisms. Zebrafish exposed to polystyrene nanoplastic particulates and nonylphenol for a period of 45 days induced oxidative stress by reducing CAT activity and GSH levels (Aliakbarzadeh et al., 2023). Antioxidant enzyme activity and lipid peroxidation levels in the liver of the PVC-diet-treated Clarias gariepinus decreased and elevated in a time-dependent manner, respectively (Iheanacho and Odo, 2020).
Oil pollution in marine bodies, especially mazut exposure, generated oxidative stress in developing embryos of fish (Rudneva, 2022; Rudneva, 2023). Increased enzymatic activities for catalase, rhodanese, and glutathione S-transferase enzymes in the liver, muscles, and gills of Nile tilapia and Mullet fish collected from some crude oil-polluted rivers in Nigeria again implicated oxidative stress (George et al., 2023).
Studies on cyanotoxins, mainly microcystins released from blooms as a result of human eutrophication, have been found to cause oxidative stress in fish (Falfushynska et al., 2023). Recent studies have shown that bioaccumulated silver nanoparticles (AgNPs) from sewage/wastewater treatment plants discharge can generate oxidative stress in fish (Zaheer Ud Din et al., 2023). Lower antioxidant responses were observed in Lusitanian toadfish males when exposed to boat noise, an in-situ study (Amorim et al., 2022).
Here we discussed multiple stressors directly or in combination with other factors that lead to increased oxidative stress and damage in fish species and the use of oxidative stress biomarkers to assess the impact of anthropogenic activities in aquatic environments globally (Panda et al., 2022). The aforementioned studies collectively and intriguingly illustrate the imperative nature of on-going surveillance of aquatic ecosystems in order to enhance the wellbeing of fish populations by means of assessing oxidative stress biomarkers, ultimately yielding advantages for human beings.
Every organism is susceptible to oxidative stress under various external and internal stresses in its natural environment (Figure 5). Accumulation of higher oxidative stress leads to hampering various economic and ecological values of an aquatic organism. The values include their growth, production, reproduction, immunity, disease susceptibility and resistance. Like all other aquatic organisms, fish also experience oxidative stress against various environmental insults generated in their natural environment. Higher generation of ROS and oxidative stress under the impaired function of their redox regulatory systems are prominent under environmental insults. The environmental insults include low oxygen levels, pollutants, chemicals, heavy metals, extreme temperature, salinity, pH, hardness, turbidity, etc. Such abiotic stress damages lipids, proteins, and DNA in many fish and, therefore, needs to be controlled as far as possible in their culture places, especially in artisanal fisheries.
Although antioxidant enzymes such as superoxide dismutase, catalase, and glutathione peroxidase and antioxidant helping enzymes such as glutathione reductase, glutathione-S-transferase, glutaredoxin system, etc., are expressed at variable levels, are found to be unable to counter the stress (ROS and oxidation of biomolecules) in fish. Although fish are found to develop various strategies to withhold the changing environments to challenge high ROS and oxidative stress, their production is greatly hampered under such stress. So, antioxidant-rich food supplements may be one of the options for handling environmentally induced oxidative stress in fish in artisanal fisheries.
The Pacific and Atlantic Oceans have been found to contain HMs, both dissolved and particulate, including Pb, Fe, Mn, Ni, Zn, and Cr (Benson et al., 2018). These chemicals have also been discovered in significant concentrations in freshwater bodies (such as rivers and lakes).
Owing to the importance of fish and fisheries products, the control of (oxidative) stress and diseases in fish has been given due importance (Paital, 2013, 2014; Mishra et al., 2020). Major challenges in the fisheries industry are solved using biotechnology (blue biotechnology). Some of the approaches are the development of vaccines, diagnostic tools, and treatments to prevent and control diseases in fish (Mugunthan et al., 2023). Genetic improvement techniques are useful for developing new and improved fish varieties that are faster-growing, disease-resistant, and better adapted to different environmental conditions (Nguyen, 2015). This significantly increases fish production and improves the quality of their products. Developing improved fish nutrition could enhance the growth, health, and productivity of fish. Molecular methods are improving the nutritional value of fish, making it more nutritious and appealing to consumers (Pradhan et al., 2019). Biotechnology can be used to develop more convenient and consumer-friendly methods for preserving fish, such as stress resistance can extend the shelf life of fish products. Strategic aquaculture management could be obtained using improved seed, water quality and controlling pests and diseases (Paital, 2018).
Several genetically modified (GM) fish species that have been developed and are under investigation for commercial purposes. Scientists and companies are using biotechnology to make vaccines and other things that can help fish fight diseases like vibriosis, furunculosis, and bacterial kidney disease, by making fish resistant to getting sick. Vaccinations and immune-boosters could help to increase fish production and the aquaculture’s population (Paital, 2018). Due to its significant value, there has been a notable increase in the presence of numerous commercial entities engaged in the manufacture and distribution of fish protein products (Table 7). Many other companies offer similar products, and the selection of available products can vary depending on the location and availability. The aquaculture industry in India is constantly growing and evolving, and new companies are being established every year to meet the demand at a very high rate for fish and fish-based products (Paital, 2018; Pradhan et al., 2019).
It is worth noting that the commercialization of GM fish is controversial, and many people are concerned about the safety, environmental impact, and ethical implications of genetically modifying fish. Debate and research are continuing on GM fish; meanwhile, some of the most well-known GM fish are already approved by the Food, drug, and cosmetic act (FD & C Act) or other laws (FDA, 2023).
6 Future prospective and conclusion
Studying oxidative stress health in fish is an emerging area of research as it has gained much interest in recent years. Being an aquatic organism, various environmental insults such as the changing pH, salinity, temperature, turbidity, dissolved oxygen, pollution, chemicals, and anthropogenic pressures are found to impose various cellular disturbances in fish. Many biochemical and molecular strategies, such as upregulated antioxidant genes and proteins and upregulation of antioxidant transcribing systems such as NRF2-Keap1 system, are adapted by fish to challenge the environmental stressors; they may not be sufficient under the high fluctuation of environmental factors. So, understanding the role and regulation or expression patterns of each redox regulating enzyme/molecule in fish under the changing earth’s climate may help to make suitable resilient strategies to save and increase fish population/growth. Understanding the exact role of supplementary antioxidants in fish physiology can help to develop strategies to improve fish (oxidative stress) health and reduce disease susceptibility. Such studies should be done in individual fish species under exposure to the individual or combined climatic or aquatic physicochemical factors to have common or individual strategies to improve fish oxidative stress health. Identifying individual biomarkers in fish under the above aquatic stress factors can be another scope of such studies. All such studies can lead to the application in aquaculture and fisheries management of fish on the one hand and in the conservation of aquatic habitats on the other hand.
Author contributions
Conceptualization; Data curation; Formal analysis; Funding acquisition; Investigation; Methodology; Project administration; Resources; Software; Supervision; Validation; Visualization; Roles/Writing - original draft; Writing–review and editing by BP. Concept, Writing–original draft; Writing–review and editing by SM and DS, Writing–original draft, editing–AK, SMi, SMa, RJ, AK, TU, KH, SD, NR, US, and MA. All authors contributed to the article and approved the submitted version.
Funding
Schemes (number ECR/2016/001984 from Science Engineering Research Board, DST, Govt. of India and 1188/ST, Bhubaneswar, dated 01.03.17, ST- (Bio)-02/2017 from Department of Biotechnology, DST, Govt. of Odisha, India) to BP are acknowledged.
Acknowledgments
Encouragements by the honourable Vice Chancellor, Odisha University of Agriculture and Technology Bhubaneswar, and the Central Instrumentation facility are duly acknowledged.
Conflict of interest
The authors declare that the research was conducted in the absence of any commercial or financial relationships that could be construed as a potential conflict of interest.
Publisher’s note
All claims expressed in this article are solely those of the authors and do not necessarily represent those of their affiliated organizations, or those of the publisher, the editors and the reviewers. Any product that may be evaluated in this article, or claim that may be made by its manufacturer, is not guaranteed or endorsed by the publisher.
References
Abdel-Tawwab, M., Monier, M. N., Hoseinifar, S. H., and Faggio, C. (2019). Fish response to hypoxia stress: Growth, physiological, and immunological biomarkers. Fish. Physiol. Biochem. 45, 997–1013. doi:10.1007/s10695-019-00614-9
Aboagye, D. L., and Allen, P. J. (2018). Effects of acute and chronic hypoxia on acid-base regulation, hematology, ion, and osmoregulation of juvenile American paddlefish. J. Comp. Physiol. B 188, 77–88. doi:10.1007/s00360-017-1104-7
Abou Anni, I. S., Bianchini, A., Barcarolli Varela, A. S., Robaldo, A. B., Tesser, A. B., Sampaio, L. A., et al. (2016). Salinity influence on growth, osmoregulation and energy turnover in juvenile pompano Trachinotus marginatus Cuvier 1832. Aquacul 455, 63–72. doi:10.1016/j.aquaculture.2016.01.010
Akoll, P., and Mwanja, W. (2012). Fish health status, research and management in east africa: Past and present. Afric. J. Aquat. Sci. 37, 117–129. doi:10.2989/16085914.2012.694628
Ali, B. A., and Mishra, A. (2022). Effects of dissolved oxygen concentration on freshwater fish: A review. Int. J. Fish. Aqua. Stud. 10, 113–127. doi:10.22271/fish.2022.v10.i4b.2693
Aliakbarzadeh, F., Rafiee, M., Khodagholi, F., Khorramizadeh, M. R., Manouchehri, H., Eslami, A., et al. (2023). Adverse effects of polystyrene nanoplastic and its binary mixtures with nonylphenol on zebrafish nervous system: From oxidative stress to impaired neurotransmitter system. Environ. Pollu. 317, 120587. doi:10.1016/j.envpol.2022.120587
Allgeier, J. E., Yeager, L. A., and Layman, C. A. (2013). Consumers regulate nutrient limitation regimes and primary production in seagrass ecosystems. Ecol 94, 521–529. doi:10.1890/12-1122.1
Alrumman, S. A., El-kott, A. F., and Kehsk, M. A. (2016). Water pollution: Source and treatment. Amer. J. Environ. Engin. 6 (3), 88–98. doi:10.5923/j.ajee.20160603.02
Amanajás, R. D., Silva, J. M., and Val, A. L. (2018). Growing in the dark warmth: The case of amazonian fish Colossoma macropomum. Front. Mar. Sci. 5. doi:10.3389/fmars.2018.00492
Amankwaa, G., Yin, X., Zhang, L., Huang, W., Cao, Y., Ni, X., et al. (2021). Spatial distribution and eco-environmental risk assessment of heavy metals in surface sediments from a crater lake (Bosomtwe/Bosumtwi). Environ. Sci. Pollu. Res. 28, 19367–19380. doi:10.1007/s11356-020-12112-0
Amoatey, P., and Baawain, M. S. (2019). Effects of pollution on freshwater aquatic organisms. Water Environ. Res. 91, 1272–1287. doi:10.1002/wer.1221
Amorim, M. C. P., Vieira, M., Meireles, G., Novais, S. C., Lemos, M. F. L., Modesto, T., et al. (2022). Boat noise impacts Lusitanian toadfish breeding males and reproductive outcome. Sci. Total Environ. 830, 154735. doi:10.1016/j.scitotenv.2022.154735
Anih, L. N., Atama, C. I., Chiejina, C. O., Ezeani, S. I., Chinwe Ezinwa, H., Chukwu, M. N., et al. (2023). Long term integrated biomarker responses in freshwater African catfish Clarias gariepinus exposed to a new brand of herbicide fluazifop-p-butyl. Drug Chem. Toxicol., 1–13. doi:10.1080/01480545.2023.2174987
Antonucci, M., Belghit, I., Truzzi, C., Illuminati, S., and Araujo, P. (2019). Modeling the influence of time and temperature on the levels of fatty acids in the liver of Antarctic fish Trematomus bernacchii. Polar Biol. 42, 2017–2030. doi:10.1007/s00300-019-02577-2
Anttila, K., Dhillon, R. S., Boulding, E. G., Farrell, D. P., Glebe, D. B., Elliott, J. A. K., et al. (2013). Variation in temperature tolerance among families of Atlantic salmon (Salmo salar) is associated with hypoxia tolerance, ventricle size and myoglobin level. J. Exp. Biol. 216, 1183–1190. doi:10.1242/jeb.080556
Apha, (1992). Standard methods for the examination of water and wastewater. 18. Washington DC: American Public Health Association APHA, American Water Works Association AWWA and Water Pollution Control Federation WPCF.
Arantes, F. P., Savassi, L. A., Santos, H. B., Gomes, M. V. T., and Bazzoli, N. (2016). Bioaccumulation of mercury, cadmium, zinc, chromium, and lead in muscle, liver, and spleen tissues of a large commercially valuable catfish species from Brazil. An. Acad. Bras. Cienc. 88, 137–147. doi:10.1590/0001-3765201620140434
Aride, P. H. R., Roubach, R., and Val, A. L. (2007). Tolerance response of tambaqui Colossoma macropomum (Cuvier) to water pH. Aquac. Res. 38, 588–594. doi:10.1111/j.1365-2109.2007.01693.x
Arrieche, D., Maeda-Martínez, A. N., Acosta-Balbás, V., Freites, L., Acosta-Salmón, H., and Lodeiros-Seijo, C. (2020). Optimum temperature for growth of an invasive green mussel Perna viridis population from Venezuela, determined in an open-flow system. Aquacult. Rep. 16, 100284. doi:10.1016/j.aqrep.2020.100284
Austin, B. (1998). The effects of pollution on fish health. J. Appl. Microbiol. 85, 234S–242S. doi:10.1111/j.1365-2672.1998.tb05303.x
Bal, A., and Paital, B. (2023). Anthropization, salinity and oxidative stress in animals in the coastal zone. Environ. Sci. Proc. 2023, 25. doi:10.3390/ECWS-7-14228
Bal, A., Panda, F., Pati, S. G., Anwar, T. N., Das, K., and Paital, B. (2022). Influence of anthropogenic activities on redox regulation and oxidative stress responses in different phyla of animals in coastal water via changing in salinity. Water 14, 4026. doi:10.3390/w14244026
Bal, A., Panda, F., Pati, S. G., Das, K., Agrawalc, P. K., and Paital, B. (2021a). Modulation of physiological oxidative stress and antioxidant status by abiotic factors especially salinity in aquatic organisms. Comp. Biochem. Physiol. C 241, 108971. doi:10.1016/j.cbpc.2020.108971
Bal, A., Pati, S. G., Panda, F., Mohanty, L., and Paital, B. (2021b). Low salinity induced challenges in the hardy fish Heteropneustes fossilis; future prospective of aquaculture in near coastal zones. Aquaculture 543, 737007. doi:10.1016/j.aquaculture.2021.737007
Banan, A., Forouharmehr, A., Kalbassi, M. R., Esmaeilbeigi, M., Bahmani, M., Sadati, M. Y., et al. (2022). Salinity gradients exacerbate the genotoxicity and bioaccumulation of silver nanoparticles in fingerling Persian sturgeon (Acipenser persicus). Regional Stud. Mar. Sci. 52, 102264. doi:10.1016/j.rsma.2022.102264
Banan, A., Nasiri, A., and Taheri-Garavand, A. (2020a). Deep learning-based appearance features extraction for automated carp species identification. Aquac. Eng. 89, 102053. doi:10.1016/j.aquaeng.2020.102053
Banan, A., Kalbassi, M. R., Bahmani, M., and Sadati, M. A. Y. (2011). Effects of colored light and tank color on growth indices and some physiological parameters of juvenile beluga (Huso huso). J. Appl. Ichthyology 27 (2), 565–570. doi:10.1111/j.1439-0426.2011.01682.x
Banan, A., Kalbassi, M. R., Bahmani, M., Sotoudeh, E., Johari, S. A., Ali, J. M., et al. (2020b). Salinity modulates biochemical and histopathological changes caused by silver nanoparticles in juvenile Persian sturgeon (Acipenser persicus). Environ. Sci. Pollut. Res. 27, 10658–10671. doi:10.1007/s11356-020-07687-7
Bani, A., Tabarsa, M., Falahatkar, B., and Bananshkan, A. (2009). Effects of different photoperiods on growth, stress and haematological parameters in juvenile great sturgeon Huso huso. Aquac. Res. 40 (16), 1899–1907. doi:10.1111/j.1365-2109.2009.02321.x
Baumann, H., Talmage, S. C., and Gobler, C. J. (2012). Reduced early life growth and survival in a fish in direct response to increased carbon dioxide. Nat. Clim. Change. 2, 38–41. doi:10.1038/nclimate1291
Benson, N. U., Adedapo, A. E., Fred-Ahmadu, O. H., Williams, A. B., Udosen, E. D., Ayejuyo, O. O., et al. (2018). A new method for assessment of sediment-associated contamination risks using multivariate statistical approach. Methods x. 5, 268–276. doi:10.1016/j.mex.2018.03.005
Bernhardt, J. R., Connor, M. I. O., Sunday, J. M., Gonzalez, A., and Bernhardt, J. R. (2020). Life in fluctuating environments. Philos. Trans. R. Soc. B Biol. Sci. 375, 2019045. doi:10.32942/OSF.IO/DGMR4
Biller, J. D., and Takahashi, L. S. (2018). Oxidative stress and fish immune system: Phagocytosis and leukocyte respiratory burst activity. An. Acad. Bras. Ciênc. 90, 3403–3414. doi:10.1590/0001-3765201820170730
Bols, N. C., Brubacher, J. L., Ganassin, R. C., and Lee, L. E. (2001). Ecotoxicology and innate immunity in fish. Dev. Comp. Immunol. 25 (8-9), 853–873. doi:10.1016/s0145-305x(01)00040-4
Booth, J. M., Giomi, F., Daffonchio, D., McQuaid, C. D., and Fusi, M. (2023). Disturbance of primary producer communities disrupts the thermal limits of the associated aquatic fauna. Sci. Tot. Environ. 872, 162135. doi:10.1016/j.scitotenv.2023.162135
Boraiah, K. M., Basavaraj, P. S., Harisha, C. B., Kochewad, S. A., Khapte, P. S., Bhendarkar, M. P., et al. (2021). Abiotic stress tolerant crop varieties, livestock breeds and fish species. Technical report. Available from: http://niam.res.in/sites/default/files/pdfs/TB-livestock%20breeds%20and%20fish%20species.pdf (Accessed August 15, 2023).
Boyd, C. E., Tucker, C. S., and Viriyatum, R. (2011). Interpretation of pH, acidity, and alkalinity in aquaculture and fisheries. N. Am. J. Aquac. 73, 403–408. doi:10.1080/15222055.2011.620861
Branco, V., Canário, J., Lu, J., Holmgren, A., and Carvalho, C. (2012). Mercury and selenium interaction in vivo: Effects on thioredoxin reductase and glutathione peroxidase. Free Radic. Biol. Med. 52, 781–793. doi:10.1016/j.freeradbiomed.2011.12.002
Branco, V., Godinho-Santos, A., Gonçalves, J., Lu, J., Holmgren, A., and Carvalho, C. (2014). Mitochondrial thioredoxin reductase inhibition, selenium status, and Nrf-2 activation are determinant factors modulating the toxicity of mercury compounds. Free Radic. Biol. Med. 73, 95–105. doi:10.1016/j.freeradbiomed.2014.04.030
Brander, K. M. (2007). Global fish production and climate change. Proc. Nat. Acad. Sci. 104, 19709–19714. doi:10.1073/pnas.0702059104
Breitburg, D. (2002). Effects of hypoxia, and the balance between hypoxia and enrichment, on coastal fishes and fisheries. Estuaries 25, 767–781. doi:10.1007/BF02804904
Briggs, D. (2003). Environmental pollution and the global burden of disease. Br. Med. Bull. 68, 1–24. doi:10.1093/bmb/ldg019
Brodin, T., Piovano, S., Fick, J., Klaminder, J., Heynen, M., and Jonsson, M. (2014). Ecological effects of pharmaceuticals in aquatic systems impacts through behavioural alterations. Philos. Trans. R. Soc. Lond., B, Biol. Sci. 369, 20130580. doi:10.1098/rstb.2013.0580
Brown, C., and Laland, K. N. (2003). Social learning in fishes: A review. Fish. Fish. 4, 280–288. doi:10.1046/j.1467-2979.2003.00122.x
Bukola, D., Zaid, A., Olalekan, E. I., and Falilu, A. (2015). Consequences of anthropogenic activities on fish and the aquatic environment. Poult. Fish. Wildl. Sci. 3, 1–12. doi:10.4172/2375-446X.1000138
Butt, Z. D., O'Brien, E., and Volkoff, H. (2019). Effects of fasting on the gene expression of appetite regulators in three Characiformes with different feeding habits (Gymnocorymbus ternetzi, Metynnis argenteus and Exodon paradoxus). Comp. Biochem. Physiol. A Mol. Integr. Physiol. 227, 105–115. doi:10.1016/j.cbpa.2018.10.002
Byrne, S., Miller, P., Waghiyi, V., Buck, C. L., von Hippel, F. A., and Carpenter, D. O. (2015). Persistent organochlorine pesticide exposure related to a formerly used defense site on st. Lawrence island, Alaska: Data from sentinel fish and human sera. J. Toxicol. Environ. Health, Part A 78, 976–992. doi:10.1080/15287394.2015.1037412
Caldeira, K., and Wickett, M. E. (2003). Oceanography: Anthropogenic carbon and ocean pH. Nature 425, 365. doi:10.1038/425365a
Cao, J., Xu, R., Wang, F., Geng, Y., Xu, T., Zhu, M., et al. (2023). Polyethylene microplastics trigger cell apoptosis and inflammation via inducing oxidative stress and activation of the NLRP3 inflammasome in carp gills. Fish. Shellfish Immunol. 132, 108470. doi:10.1016/j.fsi.2022.108470
Carls, M. G., Marty, G. D., Meyers, T. R., Thomas, R. E., and Rice, R. D. (1998). Expression of viral hemorrhagic septicemia virus in prespawning Pacific herring (Clupea pallasi) exposed to weathered crude oil. Can. J. Fish. Aqua. Sci. 55, 2300–2309. doi:10.1139/f98-116
Carlson, A. R., and Siefert, R. E. (1974). Effects of reduced oxygen on the embryos and larvae of lake trout (Salvelinus namaycush) and largemouth bass (Micropterus salmoides). J. Fish. Res. Board Can. 31, 1393–1396. doi:10.1139/f74-165
Castro, J. S., Braz-Mota, S., Campos, D. F., Souza, S. S., and Val, A. L. (2020). High temperature, pH, and hypoxia cause oxidative stress and impair the spermatic performance of the Amazon fish Colossoma macropomum. Front. Physiol. 11, 772. Published 2020 Jul 8. doi:10.3389/fphys.2020.00772
Chainy, G. B. N., Paital, B., and Dandpat, J. (2016). An overview of seasonal changes in oxidative stress and antioxidant defence parameters in some invertebrate and vertebrate species. Scientifica 2016, 1–8. Article number 6126570. doi:10.1155/2016/6126570
Chakraborty, P., and Krishnani, K. K. (2022). Emerging bioanalytical sensors for rapid and close-to-realtime detection of priority abiotic and biotic stressors in aquaculture and culture-based fisheries. Sci. Total Environ. 838 (2), 156128. doi:10.1016/j.scitotenv.2022.156128
Chippari-Gomes, A. R., Gomes, L. C., Lopes, N. P., Val, A. L., and Almeida-Val, V. M. F. (2005). Metabolic adjustments in two Amazonian cichlids exposed to hypoxia and anoxia. Comp. Biochem. Physiology Part B Biochem. Mol. Biol. 141, 347–355. doi:10.1016/j.cbpc.2005.04.006
Choi, C. Y., Li, Z., Song, J. A., and Park, S. (2022). Water hardness can reduce the accumulation and oxidative stress of zinc in goldfish, Carassius auratus. Antioxidants 11, 715. doi:10.3390/antiox11040715
Chowdhury, S., and Saikia, S. K. (2020). Oxidative stress in fish: A review. J. Scien. Res. 12, 145–160. doi:10.3329/jsr.v12i1.41716
Chowdhury, S., and Saikia, S. K. (2022). Use of zebrafish as a model organism to study oxidative stress: A review. Zebrafish 19, 165–176. doi:10.1089/zeb.2021.0083
Claësson, D., Wang, T., and Malte, H. (2016). Maximal oxygen consumption increases with temperature in the European eel (Anguilla anguilla) through increased heart rate and arteriovenous extraction. Conserv. Physiol. 4, cow027. cow027. doi:10.1093/conphys/cow027
Conde-Sieira, M., Chivite, M., Míguez, J. M., and Soengas, J. L. (2018). Stress effects on the mechanisms regulating appetite in teleost fish. Front. Endocrinol. (Lausanne). 23 (9), 631. doi:10.3389/fendo.2018.00631
Conley, D., and Barden, L. (2016). Aqua advantage salmon: Climate-smart aquaculture. Available from: https://aquabounty.com/wp-content/uploads/2016/10/FFE_2016_EAS-Aquabounty.pdf (Accessed on March 11, 2023).
Craig, C. A., Kollaus, K. A., Behen, K. P. K., and Bonner, T. H. (2016). Relationships among spring flow, habitats, and fishes within evolutionary refugia of the Edwards Plateau. Ecosphere 7 (2), 1075. doi:10.1002/ecs2.1205
Crane, M., and Hyatt, A. (2011). Viruses of fish: An overview of significant pathogens. Viruses 3, 2025–2046. doi:10.3390/v3112025
Dalvi, R. S., Pal, A. K., Tiwari, L. R., Das, T., and Baruah, K. (2009). Thermal tolerance and oxygen consumption rates of the catfish Horabagrus brachysoma (Günther) acclimated to different temperatures. Aquaculture 295, 116–119. doi:10.1016/j.aquaculture.2009.06.034
Damsgaard, C., Lauridsen, H., Harter, T. S., Kwan, G. T., Thomsen, J. S., Funder, A. M. D., et al. (2020). Author response: A novel acidification mechanism for greatly enhanced oxygen supply to the fish retina. Elife, 91–20. doi:10.7554/eLife.58995.sa2
Das, P. C., Ayyappan, S., and Jena, J. K. (2006). Haematological changes in the three Indian major carps, Catla catla (Hamilton), Labeo rohita (Hamilton) and Cirrhinus mrigala (Hamilton) exposed to acidic and alkaline water pH. Aquaculture 256, 80–87. doi:10.1016/j.aquaculture.2006.02.019
Davison, A. (2008). Fish and Amphibian herpesviruses. Encycl. Virology (3), 205–212. doi:10.1016/B978-012374410-4.00399-X
Dawood, M. A. O., Noreldin, A. E., and Sewilam, H. (2021). Long term salinity disrupts the hepatic function, intestinal health, and gills antioxidative status in Nile tilapia stressed with hypoxia. Ecotoxicol. Environ. Saf. 1, 112412. doi:10.1016/j.ecoenv.2021.112412
Dey, A., Flajšhans, M., Pšenička, M., and Gazo, I. (2023). DNA repair genes play a variety of roles in the development of fish embryos. Front. Cell. Dev. Biol. 11, 1119229. doi:10.3389/fcell.2023.1119229
Diler, O., Özil, O., Nane, I. D., Nazıroğlu, N., Minaz, M., Aslankoç, R., et al. (2022). The effects of bisphenol A on oxidative stress, antioxidant defence, histopathological alterations and lysozyme activity in narrow-clawed crayfish (pontastacus leptodactylus). Turk. J. Fish. Aquat. Sci. 22 (10), TRJFAS19877. doi:10.4194/TRJFAS19877
Do, T. D., Thi Mai, N., Duy Khoa, T. N., Abol-Munafi, A. B., Liew, H. J., Kim, C-B., et al. (2019). Molecular characterization and gene expression of glutathione peroxidase 1 in tor tambroides exposed to temperature stress. Evol. Bioinf. 15, 117693431985358. doi:10.1177/1176934319853580
Dof, (2023b). Blue revolution. Available from: https://www.dof.gov.in/blue-revolution (Accessed on May 02, 2023).
Dof, (2023d). Department of fisheries. Available from: https://dof.gov.in/inland-fisheries; https://dof.gov.in/marine-fisheries (Accessed on May 02, 2023).
Dof, (2023a). Inland fisheries in India. Available from: https://dof.gov.in/inland-fisheries (Accessed on May 02, 2023).
Dof, (2023c). Marine fisheries. Available from: https://dof.gov.in/marine-fisheries.Marine Fisheries (Accessed on May 02, 2023).
Domenici, P., Steffensen, J. F., and Marras, S. (2017). The effect of hypoxia on fish schooling. Philosophical Trans. R. Soc. B Biol. Sci. 372, 20160236. doi:10.1098/rstb.2016.0236
Dominguezm, M., Takemura, A., Tsuchiya, M., and Nakamura, S. (2004). Impact of different environmental factors on the circulating immunoglobulin levels in the Nile tilapia, Oreochromis niloticus. Oreochromis Niloticus. Aquac. 241, 491–500. doi:10.1016/j.aquaculture.2004.06.027
Doney, S. C., Fabry, V. J., Feely, R. A., and Kleypas, J. A. (2009). Ocean acidification: The other CO2 problem. Annu. Rev. Mar. Sci. 1, 169–192. doi:10.1146/annurev.marine.010908.163834
Dong, X. Y., Qin, J. G., and Zhang, X. M. (2011). Fish adaptation to oxygen variations in aquaculture from hypoxia to hyperoxia. J. Fish. Aquacul. 2 (2), 23–28.
Dong, Y., Li, L., Xia, T., Wang, L., Xiao, L., Ding, N., et al. (2022). Oxidative stress can Be attenuated by 4-PBA caused by high-fat or ammonia nitrogen in cultured spotted seabass: The mechanism is related to endoplasmic reticulum stress. Antioxidants (Basel) 28;11 (7), 1276. doi:10.3390/antiox11071276
Duarte, R. M., Ferreira, M. S., Wood, C. M., and Val, A. L. (2013). Effect of low pH exposure on Na+ regulation in two cichlid fish species of the Amazon. Comp. Biochem. Physiol. Part A Mol. Integr. Physiol. 166, 441–448. doi:10.1016/j.cbpa.2013.07.022
Dülger, N., Kumlu, M., Türkmen, S., Ölçülü, A., Tufan, E. O., Asuman, Y. H., et al. (2012). Thermal tolerance of European Sea Bass (Dicentrarchus labrax) juveniles acclimated to three temperature levels. J. Therm. Biol. 37 (1), 79–82. doi:10.1016/j.jtherbio.2011.11.003
Ebdon, L., Quevauviller, P., Pitts, L., Cornelis, R., Crews, H., and Donard, O. F. X. (Editors) (2001). “Elemental speciation - science topic,” Trace element speciation for environment, food and health (London, United Kingdom: Royal Soc. Chem.). doi:10.1039/9781847552204
Edokpayi, C. A., and Nkwoji, J. A. (2000). Annual changes in the physico-chemical and microbenthic invertebrate characteristics of the Lagos lagoon sewage dump site at Iddo, Southern Nigeria. Ecol. Environ. Conserv. 13 (1), 13–18.
Eissa, N., Wang, H. P., Yao, H., Shen, Z. G., Shaheen, A. A., and Abou-ElGheit, E. N. (2017). Expression of Hsp70, Igf1, and three oxidative stress biomarkers in response to handling and salt treatment at different water temperatures in yellow perch, Perca flavescens. Front. Physiol. 12 (8), 683. doi:10.3389/fphys.2017.00683
Ekanem, A. P., Obiekezie, A., Kloas, W., and Knopf, K. (2004). Effects of crude extracts of Mucuna pruriens (Fabaceae) and Carica papaya (Caricaceae) against the protozoan fish parasite Ichthyophthirius multifiliis. Ichthyophthirius Multifiliis. Parasitol. Res. 92, 361–366. doi:10.1007/s00436-003-1038-8
Engwa, G. A., Ferdinand, P. U., Nwalo, F. N., and Unachukwu, M. N. (2019). Mechanism and health effects of heavy metal toxicity in humans. Poisoning Mod. World 10, 70–90. doi:10.5772/intechopen.82511
Enzor, L. A., and Place, S. P. (2014). Is warmer better? Decreased oxidative damage in notothenioid fish after long-term acclimation to multiple stressors. J. Exp. Biol. 217, 3301–3310. Pt 18. doi:10.1242/jeb.108431
Eparlib, (2023). Will the Minister of agriculture be pleased to state. Available from: https://www.eparlib.nic.in/bitstream/123456789/642315/1/141835.pdf.Will the Minister of agriculture be pleased to state (Accessed on May 02, 2023).
Ermak, G., and Davies, K. J. (2002). Calcium and oxidative stress: From cell signaling to cell death. Mol. Immunol. 38, 713–721. doi:10.1016/S0161-5890(01)00108-0
Falcó, G., Llobet, J. M., Bocio, A., and Domingo, J. L. (2006). Daily intake of arsenic, cadmium, mercury, and lead by consumption of edible marine species. J. Agric. Food Chem. 54, 6106–6112. doi:10.1021/jf0610110
Falfushynska, H., Kasianchuk, N., Siemens, E., Henao, E., and Rzymski, P. (2023). A review of common cyanotoxins and their effects on fish. Toxics 11 (2), 118. doi:10.3390/toxics11020118
Fan, Z., Wu, D., Li, J., Li, C., Zheng, X., and Wang, L. (2022). Phosphorus nutrition in songpu mirror carp (Cyprinus carpio songpu) during chronic carbonate alkalinity stress: Effects on growth, intestinal immunity, physical barrier function, and intestinal microflora. Front. Immunol. 13, 900793. doi:10.3389/fimmu.2022.900793
Fan, Z., Wu, D., Zhang, Y., Li, J., Xu, Q., and Wang, L. (2021). Carbonate alkalinity and dietary protein levels affected growth performance, intestinal immune responses and intestinal microflora in Songpu mirror carp (Cyprinus carpio Songpu). Aquacul 545, 737135. doi:10.1016/j.aquaculture.2021.737135
FAO (2023). Fisheries and aquaculture. Available from: http://www.fao.org/fishery/sofia/en (Accessed on May 02, 2023).
FAO (1987). Introduction to aquaculture, based on lectures given by V. G. Jhingran. Available from https://www.fao.org/3/ac169e/ac169e00.htm (accessed on March 11, 2023).
FAO (2020). The state of world fisheries and aquaculture 2020, sustainability in action. Rome: Food and Agriculture Organization, 244. doi:10.4060/ca9229en
FAO (2021). The state of world fisheries and aquaculture. Available from: https://www.fao.org/publications/home/fao-flagship-publications/the-state-of-world-fisheries-and-aquaculture/en (Accessed on May 02, 2023).
Fda, (2023). Federal food, drug, and cosmetic act (FD&C act). Available from: https://www.fda.gov/regulatory-information/laws-enforced-fda/federal-food-drug-and-cosmetic-act-fdc-act (Accessed on May 02, 2023).
Ferreira, Arthur, Nuñer, Alex, and Esquivel, Juan (2001). Influência do pH sobre ovos e larvas de jundiá, Rhamdia quelen (Osteichthyes, Siluriformes). Acta Sci. Biol. Sci. 23. doi:10.4025/actascibiolsci.v23i0.2705
Figueroa, D., Ríos, J., Araneda, O. F., Contreras, H. R., Concha, M. L., and García, C. (2023). Oxidative stress parameters and morphological changes in Japanese medaka (Oryzias latipes) after acute exposure to OA-group toxins. Life 13 (1), 15. doi:10.3390/life13010015
Filice, M., Reinero, F. R., Cerra, M. C., Faggio, C., Leonetti, F. L., Micarelli, P., et al. (2023). Contamination by trace elements and oxidative stress in the skeletal muscle of Scyliorhinus canicula from the central tyrrhenian sea. Antioxidants 12, 524. doi:10.3390/antiox12020524
Franklin, C. E., Davison, W., and Seebacher, F. (2007). Antarctic fish can compensate for rising temperatures: Thermal acclimation of cardiac performance in Pagothenia borchgrevinki. J. Experimen. Biol. 210, 3068–3074. Pt 17. doi:10.1242/jeb.003137
Frías-Espericueta, M. G., Bautista-Covarrubias, J. C., Osuna-Martínez, C. C., Delgado-Alvarez, C., Bojórquez, C., Aguilar-Juárez, M., et al. (2022). Metals and oxidative stress in aquatic decapod crustaceans: A review with special reference to shrimp and crabs. Aqua. Toxicol. 242, 106024. doi:10.1016/j.aquatox.2021.106024
Fromm, P. O. (1980a). A review of some physiological and toxicological responses of freshwater fish to acid stress. Environ. Biol. Fishes 5, 79–93. doi:10.1007/bf00000954
Fromm, P. O. (1980b). A review of some physiological and toxicological responses of freshwater fish to acid stress. Environ. Biol. Fish. 5, 79–93. doi:10.1007/BF00000954
Fu, S. J., Zeng, L. Q., Li, X. M., Pang, X., Cao, Z. D., Peng, J. L., et al. (2009). The behavioural, digestive and metabolic characteristics of fishes with different foraging strategies. J. Exp. Biol. 212, 2296–2302. doi:10.1242/jeb.027102
Gallage, S., Katagiri, T., Endo, M., Futami, M., Endo, M., and Maita, M. (2016). Influence of moderate hypoxia on vaccine efficacy against Vibrio anguillarum in Oreochromis niloticus (Nile tilapia). Fish. Shellfish Immunol. 51, 271–281. doi:10.1016/j.fsi.2016.02.024
Gallage, S., Katagiri, T., Endo, M., and Maita, M. (2017). Comprehensive evaluation of immunomodulation by moderate hypoxia in S. agalactiae vaccinated Nile tilapia. Fish. Shellfish Immunol. 66, 445–454. doi:10.1016/j.fsi.2017.05.041
Garai, P., Banerjee, P., Mondal, P., and Saha, N. C. (2021). Effect of heavy metals on fishes: Toxicity and bioaccumulation. J. Clin. Toxicol. S 18.
Geng, L., Tong, G., Jiang, H., and Xu, W. (2016). Effect of salinity and alkalinity on Luciobarbus capito gill Na+/K+-ATPase enzyme activity, plasma ion concentration, and osmotic pressure. Biomed. Res. Int. 2016, 1–7. doi:10.1155/2016/4605839
George, M. S., Nwaichi, E. O., and Monago-Ighorode, C. (2023). Enzyme activities in tissues of nile Tilapia (Oreochromis niloticus), mullet fish (Liza falcipinus) and crab (Callinectes amnicola) from oil impacted abuloma and woji jetties, rivers state Nigeria. Int. J. Biochem. Res. Rev. 32, 1–8. doi:10.9734/ijbcrr/2023/v32i1792
Ghanbari, M., Jami, M., Domig, K. J., and Kneifel, W. (2012). Long-term effects of water pH changes on hematological parameters in the common carp (Cyprinus carpio L.) Afr. J. Biotechnol. 11, 3153–3159. doi:10.5897/AJB10.1244
Giefing-Kröll, C., Berger, P., Lepperdinger, G., and Grubeck-Loebenstein, B. (2015). How sex and age affect immune responses, susceptibility to infections, and response to vaccination. Aging Cell. 14, 309–321. doi:10.1111/acel.12326
Grassie, C., Braithwaite, V. A., Nilsson, J., Nilsen, T. O., Teien, H. C., Handeland, S. O., et al. (2013). Aluminum exposure impacts brain plasticity and behavior in Atlantic salmon (Salmo salar). J. Exp. Biol. 216, 3148–3155. doi:10.1242/jeb.083550
Grunst, A. S., Grunst, M. L., Daem, N., Pinxten, R., Bervoets, L., and Eens, M. (2019). An important personality trait varies with blood and plumage metal concentrations in a free-living songbird. Environ. Sci. Technol. 53, 10487–10496. doi:10.1021/acs.est.9b03548
Hamre, K., Kolås, K., and Sandnes, K. (2010). Protection of fish feed, made directly from marine raw materials, with natural antioxidants. Food Chem. 119, 270–278. doi:10.1016/j.foodchem.2009.06.024
Harding, C. (2012). “Technical Report: Impacts of climate change on water resources in South Australia,” in Phase 4 volume 1: First order assessment and prioritisation of water-dependent ecosystems (Adelaide: Department for Water). Available from: https://www.waterconnect.sa.gov.au/Content/Publications/DEW/DFW_TR_2012_07.pdf (Accessed on August 15, 2023).
Hepditch, J., Tessier, S. L., Wilson, L. R., Birceanu, J. M., and Wilkie, M. P. (2019). Mitigation of lampricide toxicity to juvenile lake sturgeon: The importance of water alkalinity and life stage. Conserv. Physiol. 7, coz089. doi:10.1093/conphys/coz089
Hernández, A., García García, B., Jordán, M. J., and Hernández, M. D. (2014). Natural antioxidants in extruded fish feed: Protection at different storage temperatures. Anim. Feed Sci. Technol. 195, 112–119. doi:10.1016/j.anifeedsci.2014.06.003
Hershberger, P., Kocan, R., Elder, N., Meyers, T., and Winton, J. (1999). Epizootiology of viral hemorrhagic septicemia virus in Pacific herring from the spawn-on-kelp fishery in Prince William Sound, Alaska, USA. Dis. Aqua. Org. 37, 23–31. doi:10.3354/dao037023
Hicks, D. (2016). Seafood safety and quality: The consumer's role. Foods 5, 71. doi:10.3390/foods5040071
Hinton, D. E., and Lauren, D. J. (1990). “Liver structural alterations accompanying chronic toxicity in fishes. Potential biomarkers of exposure,” in Biomarkers of environmental contamination, 17–57. Available from: https://www.osti.gov/biblio/7018192 (Accessed on August 15, 2023).
Hore, M., Saha, R., Bhaskar, S., Mandal, S., Bhattacharyya, S., and Roy, S. (2023). Oxidative stress responses in Puntius sarana collected from some environmentally contaminated areas of River Mahananda, Malda, West Bengal. Ecotoxicol 32, 211–222. doi:10.1007/s10646-023-02630-1
Howden, S. M., Crimp, S. J., and Stokes, C. J. (2008). Climate change and Australian livestock systems: Impacts, research and policy issues. Aus. J. Exp. Agric. 48, 780–788. doi:10.1071/EA08033
Iheanacho, S. C., and Odo, G. E. (2020). Dietary exposure to polyvinyl chloride microparticles induced oxidative stress and hepatic damage in Clarias gariepinus (Burchell, 1822). Environ. Sci. Poll. Res. 27, 21159–21173. doi:10.1007/s11356-020-08611-9
Isaak, D. J., Wollrab, S., Horan, D., and Chandler, G. (2012). Climate change effects on stream and river temperatures across the northwest U. S. from 1980-2009 and implications for salmonid fishes. Clim. Chang. 113, 499–524. doi:10.1007/s10584-011-0326-z
Iyaji, F., and Eyo, J. (2009). Parasites and their freshwater fish host. Bio-Research 6. doi:10.4314/br.v6i1.28660
Jellyman, D., Bonnett, M. L., and SykesJulian Johnstone, P. (2003). “Contrasting use of daytime habitat by two species of freshwater eel Anguilla spp,” in New Zealand rivers (Grand Rapids, Michigan: Amer. Fish. Soc. Symp.), 63–78.
Jellyman, D. J. (2007). Status of New Zealand fresh-water eel stocks and management initiatives. ICES J. Mar. Sci. 7, 1379–1386. doi:10.1093/icesjms/fsm073
Jerry, D. R., Jones, D. B., Lillehammer, M., Massault, C., Loughnan, S., Cate, H. S., et al. (2022). Predicted strong genetic gains from the application of genomic selection to improve growth related traits in barramundi (Lates calcarifer). Aquaculture 549, 737761. doi:10.1016/j.aquaculture.2021.737761
Jomova, K., Raptova, R., Alomar, S. Y., Alwasel, S. H., Nepovimova, E., Kuca, K., et al. (2023). Reactive oxygen species, toxicity, oxidative stress, and antioxidants: Chronic diseases and aging. Arch. Toxicol. 97 (10), 2499–2574. doi:10.1007/s00204-023-03562-9
junction, Tractor (2023). Top 10 fish farming states in India - largest fish producing states. Available from: https://www.tractorjunction.com/blog/top-10-fish-farming-states-in-india/ (Accessed on May 02, 2023).
Karavolias, N. G., Horner, W., Abugu, W. N., and Evanega, S. N. (2021). Application of gene editing for climate change in agriculture. Front. Sust. Food Sys. 5. doi:10.3389/fsufs.2021.685801
Kaur, R., and Dua, A. (2015). Scales of freshwater fish Labeo rohita as bioindicators of water pollution in Tung Dhab Drain, Amritsar, Punjab, India. J. Toxicol. Environ. Health Part A 78, 388–396. doi:10.1080/15287394.2014.990072
Khoshnood, Z. (2016). Using biomarkers in ecotoxicology: What and why? Focus Sci. 2 (2), 1–10. doi:10.20286/focsci-020237
Kikkawa, T., Ishimatsu, A., and Kita, J. (2003). Acute CO2 tolerance during the early developmental stages of four marine teleosts. Environ. Toxicol. 18, 375–382. doi:10.1002/tox.10139
Kim, J. A., Kim, M. J., Song, J. A., and Choi, C. Y. (2023). Effects of microfiber exposure on medaka (Oryzias latipes): Oxidative stress, cell damage, and mortality. Comp. Biochem. Physiol. Part - C. Toxicol. Pharmacol. 265, 109535. doi:10.1016/j.cbpc.2022.109535
Kim, J. H., and Kang, J. C. (2016a). The toxic effects on the stress and immune responses in juvenile rockfish, Sebastes schlegelii exposed to hexavalent chromium. Environ. Toxicol. Pharmacol. 43, 128–133. doi:10.1016/j.etap.2016.03.008
Kim, J. H., Kim, S. K., and Hur, Y. B. (2019). Hematological parameters and antioxidant responses in olive flounder Paralichthys olivaceus in biofloc depend on water temperature. J. Therm. Biol. 82, 206–212. doi:10.1016/j.jtherbio.2019.04.013
Kim, J. H., Park, H. J., Kim, K. W., Hwang, I. K., Kim, D. H., Oh, C. W., et al. (2017). Growth performance, oxidative stress, and non-specific immune responses in juvenile sablefish, Anoplopoma fimbria, by changes of water temperature and salinity. Fish. Physiol. Biochem. 43 (5), 1421–1431. doi:10.1007/s10695-017-0382-z
Kim, N., and Kang, S. W. (2016b). Older and more engaged: The mediating role of age-linked resources on work engagement. Hum. Resour. Manag. J. 56, 731–746. doi:10.1002/hrm.21802
Kozak, W. (2005). “Temperature and toxicology: An integrative, comparative, and environmental approach by Christopher J. Gordon,” in Int. J. Toxicol. (Florida, United States: CRC Press, Taylor & Francis Group), 24, 471–472. ISBN: 0-8493-3024-6. 338 pages. doi:10.1080/10915810500368787
Krieger, J. R., Beaudreau, A. H., Heintz, R. A., and Callahan, M. W. (2020). Growth of young-of-year sablefish (Anoplopoma fimbria) in response to temperature and prey quality: Insights from a life stage specific bioenergetics model. J. Exp. Mar. Biol. Ecol. 526, 151340. doi:10.1016/j.jembe.2020.151340
Krishnakumar, A., Patrick Anton, E. S., and Jayawardena, U. A. (2020). Water hardness influenced variations in reproductive potential of two freshwater fish species; Poecilia reticulata and Betta splendens. BMC Res. Notes 13, 542. doi:10.1186/s13104-020-05382-x
Kuhn, J., Azari, S., and Volkoff, H. (2023). Effects of temperature on food intake and the expression of appetite regulators in three Characidae fish: The black-skirted tetra (Gymnocorymbus ternetzi), neon tetra (Paracheirodon innesi) and Mexican cavefish (Astyanax mexicanus). Comp. Biochem. Physiol. Part - C. Toxicol. Pharmacol. 275, 111333. doi:10.1016/j.cbpa.2022.111333
Lackner, R. (1998). ““Oxidative stress” in fish by environmental pollutants,” in Fish ecotoxicology. EXS. Editors T. Braunbeck, D. E. Hinton, and B. Streit (Basel: Birkhäuser), 86. doi:10.1007/978-3-0348-8853-0_6
LaFrentz, B. R., Králová, S., Burbick, C. R., Alexander, T. L., Phillips, T. W., Griffin, M. J., et al. (2022). The fish pathogen Flavobacterium columnare represents four distinct species: Flavobacterium columnare, Flavobacterium covae sp. nov., Flavobacterium davisii sp. nov. and Flavobacterium oreochromis sp. nov., and emended description of Flavobacterium columnare. Syst. Appl. Microbiol. 45, 126293. doi:10.1016/j.syapm.2021.126293
Layman, C. A., Allgeier, J. A., Yeager, L. A., and Stoner, E. W. (2013). Thresholds of ecosystem response to nutrient enrichment from fish aggregations. Ecol 94, 530–536. doi:10.1890/12-0705.1
Lebeau-Roche, E., Daniele, G., Fildier, A., Bonnefoy, C., Turiès, C., Bado-Nilles, A., et al. (2023). Time and dose-dependent impairment of liver metabolism in Gasterosteus aculeatus following exposure to diclofenac (DCF) highlighted by LC-HRMS untargeted metabolomics. Sci. Total Environ. 858, 159801. doi:10.1016/j.scitotenv.2022.159801
Lee, D-W., Choi, Y-C., Park, H-C., Park, Y-S., and Choi, C. Y. (2022). Effect of low pH and salinity conditions on the antioxidant response and hepatocyte damage in juvenile olive flounder Paralichthys olivaceus. Mar. Environ. Res. 175, 105562. ISSN 0141-1136. doi:10.1016/j.marenvres.2022.105562
Lefevre, S., Wang, T., and McKenzie, D. J. (2021). The role of mechanistic physiology in investigating impacts of global warming on fishes. J. Exp. Biol. 224, jeb238840. doi:10.1242/jeb.238840
Lesser, M. P., Kruse, V. A., and Barry, T. M. (2003). Exposure to ultraviolet radiation causes apoptosis in developing sea urchin embryos. J. Exp. Biol. 206, 4097–4103. doi:10.1242/jeb.00621
Li, C. C., and Chen, J. C. (2008). The immune response of white shrimp Litopenaeus vannamei and its susceptibility to Vibrio alginolyticus under low and high pH stress. Fish. Shellfish Immunol. 25, 701–709. doi:10.1016/j.fsi.2008.01.007
Li, X., Liu, S., Wang, Y., Lu, W., Zhang, Q., and Chengm, J. (2022b). Genomic and transcriptomic landscape and evolutionary dynamics of heat shock proteins in spotted sea bass (Lateolabrax maculatus) under salinity change and alkalinity stress. Biol 11, 353. doi:10.3390/biology11030353
Li, X., Shen, Y., Bao, Y., Wu, Z., Yang, B., Jiao, L., et al. (2022c). Physiological responses and adaptive strategies to acute low-salinity environmental stress of the euryhaline marine fish black seabream (Acanthopagrus schlegelii). Aquacul 554, 738117. doi:10.1016/j.aquaculture.2022.738117
Li, Z., Song, J. A., Kim, M. J., and Choi, C. Y. (2022a). Water hardness alleviates the stress response caused by waterborne zinc in goldfish Carassius auratus. Gen. Comp. Endocrinol. 327, 114095. doi:10.1016/j.ygcen.2022.114095
Limbaugh, N., Romano, N., Egnew, N., Shrivastava, J., Bishop, W. M., and Sinha, A. K. (2021). Coping strategies in response to different levels of elevated water hardness in channel catfish (Ictalurus punctatus): Insight into ion-regulatory and histopathological modulations. Comp. Biochem. Physiol. Part - C. Toxicol. Pharmacol. 260, 111040. doi:10.1016/j.cbpa.2021.111040
Liu, D., Pellicer, A. M., Brüggmann, A., Kiggen, M., Behrens, S., Good, C., et al. (2021). Effect of water hardness/alkalinity and humic substances on the toxicity of peracetic acid to zebrafish embryos and pathogenic isolates. Aquacul. Rep. 21, 100900. doi:10.1016/j.aqrep.2021.100900
Llobet, J. M., Falco, G., Casas, C., Teixido, A., and Domingo, J. L. (2003). Concentrations of arsenic, cadmium, mercury, and lead in common foods and estimated daily intake by children, adolescents, adults, and seniors of Catalonia, Spain. J. Agric. Food Chem. 51, 838–842. doi:10.1021/jf020734q
Lu, Y. T., Mak, S. M., Wai, W. H., Kwong, M., Fang, A., James, D., et al. (2005). Hypoxia-induced differential apoptosis in the central nervous system of the sturgeon (Acipenser shrenckii). Res. Tech. 68, 258–263. doi:10.1002/JEMT.20243
Lushchak, V. (2010). Environmentally induced oxidative stress in aquatic animals. Aquat. Toxicol. 101, 13–30. doi:10.1016/j.aquatox.2010.10.006
Lushchak, V. (2011). Environmentally induced oxidative stress in aquatic animals. Aqua. Toxicol. 101, 13–30. doi:10.1016/j.aquatox.2010.10.006
Lushchak, V. I., and Bagnyukova, T. V. (2006). Temperature increase results in oxidative stress in goldfish tissues. 2. Antioxidant and associated enzymes. Comp. Biochem. Physiol. Part - C. Toxicol. Pharmacol. 143, 36–41. doi:10.1016/j.cbpc.2005.11.018
Lutz, G. C. (2023). The use of CRISPR in aquaculture. Available from: https://thefishsite.com/articles/the-use-of-crispr-in-aquaculture-gene-editing-in-fish (Accessed on March 10, 2023).
Madeira, D., Narciso, L., Cabral, H. N., Vinagre, C., and Diniz, M. S. (2013). Influence of temperature in thermal and oxidative stress responses in estuarine fish. Comp. Biochem. Physiol. Mol. Int. Physiol. 166, 237–243. doi:10.1016/j.cbpa.2013.06.008
Mai, W., Yan, J., Wang, L., Zheng, Y., Xin, Y., and Wang, W. (2010). Oxidative stress in fish: Review. Aquat. Toxicol. 100, 271–281. doi:10.1016/j.aquatox.2010.07.025
Makuvara, Z., Marumure, J., Chapungu, L., Machingura, J., and Siwela, A. H. (2022). Comparison of oxidative stress biomarkers in Oreochromis mossambicus in minimally and highly disturbed aquatic environments in the Matabeleland region, Zimbabwe. Afr. J. Aqua. Sci. 47, 499–506. doi:10.2989/16085914.2022.2086846
Mansou, A. T., Ashour, M., Ruiz, C. E., Kumar, N., and Esteban, M. A. (2023). Editorial: Physiological performance of aquatic animals under farming-induced stress conditions. Front. Physiol. 14, 1136611. doi:10.3389/fphys.2023.1136611
Marcos-López, A., Gale, P., Oidtmann, B. C., and Peeler, E. J. (2010). Assessing the impact of climate change on disease emergence in freshwater fish in the United Kingdom. Transboun. Emer. Dis. 57, 293–304. doi:10.1111/j.1865-1682.2010.01150.x
Martínez-Chávez, C. C., Navarrete-Ramírez, P., Parke, D. V., and Migaud, H. (2021). Effects of continuous light and light intensity on the growth performance and gonadal development of Nile tilapia. Rev. Bras. Zootec. 50. doi:10.37496/rbz5020180275
Martins, A. W. S., Dellagostin, E. N., Blödorn, E. B., Silveira, T. L. R., Sampaio, L. A., Komninou, E. R., et al. (2022). Exposure to salinity induces oxidative damage and changes in the expression of genes related to appetite regulation in Nile tilapia (Oreochromis niloticus). Front. Genet. 8 (13), 948228. doi:10.3389/fgene.2022.948228
Martos-Sitcha, J. A., Mancera, J. M., Prunet, P., and Magnoni, L. J. (2020). Editorial: Welfare and stressors in fish: Challenges facing aquaculture. Front. Physiol. 11, 162. doi:10.3389/fphys.2020.00162
Masson, N., Guerold, F., and Dangles, O. (2002). Use of blood parameters in fish to assess acidic stress and chloride pollution in French running waters. Chemosphere 47, 467–473. doi:10.1016/S0045-6535(02)00055-3
Mellergaard, S., and Nielsen, E. (1995). Impact of oxygen deficiency on the disease status of common dab Limanda limanda. Dis. Aqua. Org. 22, 101–114. doi:10.3354/dao022101
Mishra, S., Paital, B., Sahoo, H. S., Pati, S. G., Tripathy, D., and Debata, N. B. (2020). A discrete Cu2(Pd-bpy)2L2 heterometallic compound with superoxide dismutase enzyme like activity. Dalton Trans. 49 (26), 8850–8854. doi:10.1039/d0dt00920b
Mishra, S. S., Das, R., Dhiman, R., Choudhary, P., and Debbarma, J. (2017). Present status of fish disease management in freshwater aquaculture in India: State-of-the-Art-Review. J. Aquacul. Fish. 1, 003. doi:10.24966/AAF-5523/100003
Moniruzzaman, M., Mukherjee, M., Kumar, S., and Chakraborty, S. B. (2022). Effects of salinity stress on antioxidant status and inflammatory responses in females of a "near threatened" economically important fish species notopterus chitala: A mechanistic approach. Environ. Sci. Pollut. Res. Int. 29, 75031–75042. doi:10.1007/s11356-022-21142-9
Moon, B-H., Seo, G-T., Lee, T-S., Kim, S-S., and Yoon, C-H. (2003). Effects of salt concentration on floc characteristics and pollutants removal efficiencies in treatment of seafood wastewater by SBR. Water Sci. Technol. 47, 65–70. doi:10.2166/wst.2003.0017
Mozaffarian, D., Ascherio, A., Hu, F. B., Stampfer, M. J., Willett, W. C., Siscovick, S. D., et al. (2005). Interplay between different polyunsaturated fatty acids and risk of coronary heart disease in men. Circulation 111, 157–164. doi:10.1161/01.CIR.0000152099.87287.83
MPEDA (2023). Tilapia (GIFT). Available from: https://mpeda.gov.in/?page_id=764 (Accessed on August 05, 2023).
Mugunthan, S. P., Kannan, G., Chandra, H. M., and Paital, B. (2023). Infection, transmission, pathogenesis and vaccine development against mycoplasma gallisepticum. Vaccines (Basel). 11 (2), 469. doi:10.3390/vaccines11020469
Munshi, A., and Sharma, V. (2018). Safety and ethics in biotechnology and bioengineering. Omics Technol. Bio-Eng., 577–590. doi:10.1016/B978-0-12-804659-3.00025-7
Naggar, A. l. Y., Khalil, M. S., and Ghorab, M. A. (2018). Environmental pollution by heavy metals in the aquatic ecosystems of Egypt. Open Acc. J. Toxicol. 3, 555603. doi:10.19080/OAJT.2018.03.555603
NFDB (2023). The national fisheries development board. Available from: https://nfdb.gov.in/ (Accessed on May 02, 2023).
Nguyen, N. H. (2015). Genetic improvement for important farmed aquaculture species with a reference to carp, tilapia and prawns in Asia: Achievements, lessons and challenges. Fish. Fish. 17, 483–506. doi:10.1111/faf.12122
Nkwoji, J. A., Yakub, A., Ajani, G. E., Balogun, K. J., Renner, K. O., Igbo, J. K., Ariyo, A. A., Bello, B. O., et al. (2010). Seasonal variations in the water chemistry and benthic macroinvertebrates of a South Western Lagoon, Lagos, Nigeria. J. Anim. Sci. 6, 85–92.
Noble, A. C., and Summerfelt, S. T. (1996). Diseases encountered in rainbow trout cultured in recirculating systems. Annu. Rev. Fish. Dis. 6, 65–92. doi:10.1016/S0959-8030(96)90006-X
Null, S. E., Mouzon, N. R., and Elmore, L. R. (2017). Dissolved oxygen, stream temperature, and fish habitat response to environmental water purchases. J. Environ. Manag. 197, 559–570. doi:10.1016/j.jenvman.2017.04.016
Nwabueze, J. C., Sogbanmu, T. O., and Ugwumba, A. A. A. (2020). Physicochemical characteristics, animal species diversity and oxidative stress responses in dominant fish from an impacted site on the Lagos Lagoon, Nigeria. Ife J. Sci. 22, 81–93. doi:10.4314/ijs.v22i2.8
ODS (2023). Omega-3 fatty acids. Available from: https://ods.od.nih.gov/factsheets/Omega3FattyAcids-HealthProfessional/ (Accessed on August 05, 2023).
OECD (2021). Organization for economic cooperation development. Available from: https://www.oecd.org/about/ (Accessed on June 22, 2023).
Özgeriş, F. B., Yeltekin, A. Ç., Ucar, A., Çağlar, Ö., Parlak, V., Arslan, M. E., et al. (2023). Toxic releases and exposure assessment: A multi-endpoint approach in fish for ferrocene toxicity. Process Saf. Environ. Prot. 169, 636–645. doi:10.1016/j.psep.2022.11.052
Paital, B. (2013). Antioxidant and oxidative stress parameters in brain of Heteropneustes fossilis under air exposure condition; role of mitochondrial electron transport chain. Ecotoxicol. Environ. Saf. 95, 69–77. doi:10.1016/j.ecoenv.2013.05.016
Paital, B., and Chainy, G. B. N. (2012). Biology and conservation of the genus Scylla in India subcontinent. J. Environ. Biol. 33 (5), 871–9.
Paital, B., and Chainy, G. B. N. (2014). Effects of temperature on complexes I and II mediated respiration, ROS generation and oxidative stress status in isolated gill mitochondria of the mud crab Scylla serrata. J. Therm. Biol. 41, 104–111. doi:10.1016/j.jtherbio.2014.02.013
Paital, B., and Chainy, G. B. N. (2013). Modulation of expression of SOD isoenzymes in mud crab (Scylla serrata): Effects of inhibitors, salinity and season. J. Enz. Inhib. Med. Chem. 28, 195–204. doi:10.3109/14756366.2011.645239
Paital, B. (2014). Modulation of redox regulatory molecules and electron transport chain activity in muscle of air breathing fish Heteropneustes fossilis under air exposure stress. J. Comp. Physiol. B 184 (1), 65–76. doi:10.1007/s00360-013-0778-8
Paital, B. (2018). Nutraceutical values of fish demand their ecological genetic studies: A short review. J. Basic Appl. Zool. 79 (16), 16–11. doi:10.1186/s41936-018-0030-x
Paital, B., Panda, S. K., Hati, A. K., Mohanty, B., Mohapatra, M. K., Kanungo, S., et al. (2016). Longevity of animals under reactive oxygen species stress and disease susceptibility due to global warming. World J. Biol. Chem. 7 (1), 110–127. doi:10.4331/wjbc.v7.i1.110
Paital, B., Pati, S. G., Panda, F., Jally, S. K., and Agrawal, P. K. (2022). Changes in physicochemical, heavy metals and air quality linked to spot Aplocheilus panchax along Mahanadi industrial belt of India under COVID-19-induced lockdowns. Environ. Geochem Health 45, 751–770. doi:10.1007/s10653-022-01247-3
Panda, F., Pati, S. G., Sa-manta, L., and Paital, B. (2022). Seasonal variation of water quality modulated redox regulatory system in the apple snail pila globosa and its use as a bioindicator species in freshwater ecosystems across India. Water 14 (20), 3275. doi:10.3390/w14203275
Pennington, K., Chan, T., Torres, M., and Andersen, J. (2018). The dynamic and stress-adaptive signaling hub of 14-3-3: Emerging mechanisms of regulation and context-dependent protein–protein interactions. Oncogene 37, 5587–5604. doi:10.1038/s41388-018-0348-3
Pereira, L. A. L., Amanajás, R. D., de Oliveira, A. M., Paulada Silva, M. N., and Val, A. L. (2021). Health of the Amazonian fish tambaqui (Colossoma macropomum): Effects of prolonged photoperiod and high temperature. Aquacul 541, 736836. doi:10.1016/j.aquaculture.2021.736836
Pham, L. P., Nguyen, M. V., Jordal, A. O., and Rønnestad, I. (2022). Metabolic rates, feed intake, appetite control, and gut transit of clownfish Amphiprion ocellaris exposed to increased temperature and limited feed availability. Comp. Biochem. Physiology Part A Mol. Integr. Physiology 274, 111318. doi:10.1016/j.cbpa.2022.111318
PIB (2023). Indian fisheries: NeeliKranti to ArthKranti. Available from: https://pib.gov.in/FeaturesDeatils.aspx?NoteId=151155&ModuleId%20=%202 (Accessed on March 07, 2023).
Pinto, M. F., Louro, H., Costa, P. M., Caeiro, S., and Silva, M. J. (2015). Exploring the potential interference of estuarine sediment contaminants with the DNA repair capacity of human hepatoma cells. J. Toxicol. Environ. Health A 78, 559–570. doi:10.1080/15287394.2015.1006712
Plumb, J. A., and Hanson, L. A. (2010). Health maintenance and principal microbial diseases of cultured fishes. New Jersey, United States: Wiley-Blackwell. doi:10.1002/9780470958353
Pmmsy, (2023). Pradhan mantri matsya sampada yojana. Available from: https://pmmsy.dof.gov.in/.Pradhan mantri matsya sampada yojana (Accessed on May 02, 2023).
Pourkhabbaz, A., Khazaei, T., Behravesh, S., Ebrahimpour, M., and Pourkhabbaz, H. (2011). Effect of water hardness on the toxicity of cobalt and nickel to a freshwater fish, Capoeta fusca. Biomed. Environ. Sci. 24, 656–660. doi:10.3967/0895-3988.2011.06.010
Pradhan, M., Guru, P., and Paital, B. (2019). Daily dietary nutrition and nutraceutical intake in agricultural laborers of hirakud command area, sambalpur, Odisha, India. J. Drug Deliv. Ther. 9 (5), 56–61. doi:10.22270/jddt.v9i5.3443
Priyadarshini, M., Orosco, L. A., and Panula, P. J. (2013). Oxidative stress and regulation of Pink1 in zebrafish (Danio rerio). PLoS ONE 8, e81851. doi:10.1371/journal.pone.0081851
Qi, Q., Hu, C., Lin, J., Wang, X., Tang, C., Dai, Z., et al. (2022). Contamination with multiple heavy metals decreases microbial diversity and favors generalists as the keystones in microbial occurrence networks. Environ. Poll. 306, 119406. doi:10.1016/j.envpol.2022.119406
Qiu, X., Zhu, T., Li, J., Pan, Li Q., Miao, G., Gong, J., et al. (2004). Organochlorine pesticides in the air around the Taihu Lake, China. Environ. Sci. Technol. 38, 1368–1374. doi:10.1021/es035052d
Rajwa, A. A., Rowinski, P. M., Bialik, R. J., and Karpinski, M. (2014). “Stream diurnal profiles of dissolved oxygen - case studies,” in 3rd IAHR Europe Congress, Book of Proceedings, Porto, Portugal, April 14th-16th 2014.
Rath, S., Bal, A., and Paital, B. (2021). Heavy metal and organic load in Haripur creek of Gopalpur along the Bay of Bengal, east coast of India. Environ. Sci. Poll. Res. 28, 28275–28288. doi:10.1007/s11356-021-12601-w
Reader, S. M. (2015). Causes of individual differences in animal exploration and search. Top. Cognitive Sci. 7, 451–468. doi:10.1111/tops.12148
Reeve, C., Rowsey, L. E., and Speers-Roesch, B. (2022). Inactivity and the passive slowing effect of cold on resting metabolism as the primary drivers of energy savings in overwintering fishes. J. Exper. Biol. 225, jeb243407. doi:10.1242/jeb.243407
Regoli, F., Giuliani, M. E., Benedetti, M., and Arukwe, A. (2011). Molecular and biochemical biomarkers in environmental monitoring: A comparison of biotransformation and antioxidant defense systems in multiple tissues. Aquat. Toxicol. 105 (3–4), 56–66. doi:10.1016/j.aquatox.2011.06.014
Reogma, (2023). Fish industry in India to reach US$ 25.39B by 2023. Available from: https://www.reogma.com/industry-reports/fish-industry-in-india-to-reach-us-25-39b-by-2023 (Accessed on May 02, 2023).
Reynalte-Tataje, D. A., Lopes, C. A., Massaro, M. V., Hartmann, P. B., Sulzbacher, R., Sanots, J. A., et al. (2020). State of the art of identification of eggs and larvae of freshwater fish in Brazil. Acta Limnol. Bras. 32. e6. doi:10.1590/s2179-975x5319
Robinson, E., and Davison, W. (2008). The Antarctic notothenioid fish Pagothenia borchgrevinki is thermally flexible: Acclimation changes oxygen consumption. Polar Biol. 31, 31317–31326. doi:10.1007/s00300-007-0361-4
Robinson, P. D. (2009). Behavioural toxicity of organic chemical contaminants in fish: Application to ecological risk assessments (ERAs). Can. J. Fish. Aqua. Sci. 66, 1179–1188. doi:10.1139/F09-069
Romano, N., Egnew, N., Quintero, H., Kelly, A., and Sinha, A. K. (2020). The effects of water hardness on the growth, metabolic indicators and stress resistance of largemouth bass Micropterus salmoides. Aquacul 527, 735469. doi:10.1016/j.aquaculture.2020.735469
Rønnestad, I., Gomes, A. S., Murashita, K., Angotzi, R., Jönsson, E., and Volkoff, H. (2017). Appetite-controlling endocrine systems in teleosts. Front. Endocrinol. (Lausanne). 18, 73. doi:10.3389/fendo.2017.00073
Rudneva, I. I. (2022). Assessment of mazut toxicity for embryos of two sea fish species. Ecol. Saf. Coast. Shelf Zones Sea 2, 118–127. doi:10.22449/2413-5577-2022-2-118-127
Rudneva, I. I. (2023). Interspecies Peculiarities of Biomarkers Response of Marine Fish Embryos to Oil Pollution. Pollution 9, 243–253. doi:10.22059/POLL.2022.345522.1530
Rummer, J. L., McKenzie, D. J., Innocenti, A., Supuran, C. T., and Brauner, C. J. (2013). Root effect hemoglobin may have evolved to enhance general tissue oxygen delivery, 340, 1327–1329. doi:10.1126/science.1233692
Saaristo, M., Brodin, T., Balshine, S., Bertram, M. G., Brooks, B. W., Ehlman, S. M., et al. (2018). Direct and indirect effects of chemical contaminants on the behaviour, ecology and evolution of wildlife. Proceeding B 285, 20181297. doi:10.1098/rspb.2018.1297
Sagla, D., Atli, G., and Canli, M. (2013). Investigations on the osmoregulation of freshwater fish (Oreochromis niloticus) following exposures to metals (Cd, Cu) in differing hardness. Ecotoxicol. Environ. Saf. 92, 79–86. doi:10.1016/j.ecoenv.2013.02.020
Schindler, D. W. (1988). Effects of acid rain on freshwater ecosystems. Science 239, 149–157. doi:10.1126/science.239.4836.149
Scott, D. M., Lucas, M. C., and Wilson, R. W. (2003). The effect of high pH on ion balance, nitrogen excretion and behaviour in freshwater fish from an eutrophic lake: A laboratory and field study. Aquat. Toxicol. 73, 31–43. doi:10.1016/j.aquatox.2004.12.013
Sharifi-Rad, M., Anil Kumar, N. V., Zucca, P., Varoni, E. M., Dini, L., Panzarini, E., et al. (2020). Lifestyle, oxidative stress, and antioxidants: Back and forth in the pathophysiology of chronic diseases. Fron. Physiol. 11, 694. doi:10.3389/fphys.2020.00694
Shoemaker, C. C., Evans, J. J., and Klesius, P. H. (2000). Density and dose: Factors affecting mortality of Streptococcus iniae infected tilapia (Oreochromis niloticus). Aquacul 188, 229–235. doi:10.1016/S0044-8486(00)00346-X
Sindermann, S. J. (1984). Disease in marine aquaculture. Helgoländer Meeresunters. 37, 505–530. doi:10.1007/BF01989327
Sinha, A. K., Limbaugh, N., Renukdas, N., Bishop, W. M., and Romano, N. (2022). Modulating effect of elevated water hardness on growth performance, ammonia dynamics and ion-regulatory capacity in channel catfish (Ictalurus punctatus) following chronic challenge with high environmental ammonia and salinity stress. Aquacul 560, 738489. doi:10.1016/j.aquaculture.2022.738489
Sinha, D. K. (2023). 9 major fish production centers in India. Available from: https://www.yourarticlelibrary.com/agriculture/9-major-fish-production-centers-in-india/42314 (Accessed on May 07, 2023).
Sloman, K. A., and McNeil, P. L. (2012). Using physiology and behaviour to understand the responses of fish early life stages to toxicants. J. Fish Biol. 81, 2175–2198. doi:10.1111/j.1095-8649.2012.03435.x
Song, J. A., and Choi, C. Y. (2021). Temporal changes in physiological responses of Bay Scallop: Performance of antioxidant mechanism in Argopecten irradians in response to sudden changes in habitat salinity. Antioxidants (Basel) 10, 1673. doi:10.3390/antiox10111673
Sotoudeh, E., Feizollahi, S., Banan, A., Morshedi, V., and Hosseini, A. (2019). Oxidative status and digestive histology of barramundi perch in response to different tank colors. J. Aquatic Animal Health 31 (2), 139–146. doi:10.1002/aah.10060
Srikanth, K., Pereira, E., Duarte, A. C., and Ahmad, I. (2013). Glutathione and its dependent enzymes' modulatory responses to toxic metals and metalloids in fish-a review. Environ. Sci. Poll. Res. 20, 2133–2149. doi:10.1007/s11356-012-1459-y
Subaramaniyam, U., Allimuthu, R. S., Vappu, S., Ramalingam, D., Balan, R., Paital, B., et al. (2023). Effects of microplastics, pesticides and nano-materials on fish health, oxidative stress and antioxidant defense mechanism. Front. Physiology 14, 1–24. 1217666. doi:10.3389/fphys.2023.1217666
Subhendu, D. (2000). Effects of aquatic pollution on fish and fisheries. Pollution-an International problem for fisheries. Can. J. Fish. Aqua. Sci. 66, 400–480.
Sun, J., Wang, J., Li, L., Wu, L., Chen, X., and Yuan, Y. (2020). ROS induced by spring viraemia of carp virus activate the inflammatory response via the MAPK/AP-1 and PI3K signaling pathways. Fish. Shellfish Immunol. 101, 216–224. doi:10.1016/j.fsi.2020.03.056
Sun, Y. C., Wu, S., Du, N. N., Song, Y., and Xu, W. (2018). High-throughput metabolomics enables metabolite biomarkers and metabolic mechanism discovery of fish in response to alkalinity stress. RSC Adv. 8 (27), 14983–14990. doi:10.1039/c8ra01317a
Suzzi, A. L., Stat, M., Gaston, T. F., Siboni, N., Williams, N. L., Seymour, J. R., et al. (2023). Elevated estuary water temperature drives fish gut dysbiosis and increased loads of pathogenic vibrionaceae. Environ. Res. 219, 115144. doi:10.1016/j.envres.2022.115144
Svendsen, J. C., Tudorache, C., Jordan, A. D., Steffensen, S., Aarestrup, K., and Domenici, P. (2010). Partition of aerobic and anaerobic swimming costs related to gait transitions in a labriform swimmer. J. Exp. Biol. 213, 2177–2183. doi:10.1242/jeb.041368
Swingle, H. S. (1969). Methods of analysis for waters, organic matter and pond bottom soils used in fisheries research. Auburn, Ala: Auburn Univ., 119.
Szyller, Z., and Bil-Lula, L. (2021). Heat shock proteins in oxidative stress and ischemia/reperfusion injury and benefits from physical exercises: A review to the current knowledge. Oxidative Med. Cell. Long. 2021, 1–12. doi:10.1155/2021/6678457
Thakur, S., Mathur, S., Patel, S., and Paital, B. (2022). Microplastic accumulation and degradation in environment via biotechnological approaches. Water 14 (24), 4053. doi:10.3390/w14244053
Tian, H-Y., Zhang, D-D., Xu, C., Wang, F., and Liu, W-B. (2015). Effects of light intensity on growth, immune responses, antioxidant capability and disease resistance of juvenile blunt snout bream Megalobrama amblycephala. Fish. Shellfish Immunol. 47, 674–680. doi:10.1016/j.fsi.2015.08.022
Topal, A., Özdemir, S., Arslan, H., and Çomaklı, S. (2021). How does elevated water temperature affect fish brain? (A neurophysiological and experimental study: Assessment of brain derived neurotrophic factor, cFOS, apoptotic genes, heat shock genes, ER-stress genes and oxidative stress genes). Fish. Shellfish Immunol. 115, 198–204. doi:10.1016/j.fsi.2021.05.002
Toranzo, A. E., Magariños, B., and Romalde, J. L. (2005). A review of the main bacterial fish diseases in mariculture systems. Aquaculture 246, 37–61. doi:10.1016/j.aquaculture.2005.01.002
Torrecillas, S., Caballero, M. J., Mompel, D., Montero, D., Zamorano, M. J., Robaina, L., et al. (2017). Disease resistance and response against Vibrio anguillarum intestinal infection in European seabass (Dicentrarchus labrax) fed low fish meal and fish oil diets. Fish. Shellfish Immunol. 67, 302–311. doi:10.1016/j.fsi.2017.06.022
Tovell, P., Newsome, C., and Howes, D. (1974). Effect of water hardness on the toxicity of an anionic detergent to fish. Water Res. 8 (5), 291–296. doi:10.1016/0043-1354(74)90091-8
Tripathi, A. K., and Sindhi, S. (2020). Droughts, Heatwaves and Agricultural Adaptation - A Historical Account for India. Econ. political Wkly. 55, 26–27.
Ultsch, G. R., Ott, M. E., and Heisler, N. (1981). Acid base and electrolyte status in carp (Cyprinus carpio) exposed to low environmental pH. J. Exp. Biol. 93, 65–80. doi:10.1242/jeb.93.1.65
Valavanidis, A., Vlahogianni, T., Dassenakis, M., and Scoullos, M. (2006). Molecular biomarkers of oxidative stress in aquatic organisms in relation to toxic environmental pollutants. Ecotoxicol. Environ. Saf. 64, 178–189. doi:10.1016/j.ecoenv.2005.03.013
Vargas-Chacoff, L., Regish, A. M., Weinstock, A., and McCormick, S. D. (2018). Effects of elevated temperature on osmoregulation and stress responses in Atlantic salmon Salmo salar smolts in fresh water and seawater. J. Fish. Biol. 93, 550–559. doi:10.1111/jfb.13683
Ventura, B. C., Angelis, D. F., Maria, A. M., and Morales, M. (2008). Mutagenic and genotoxic effects of the Atrazine herbicide in Oreochromis niloticus (Perciformes, Cichlidae) detected by the micronuclei test and the comet assay. Pesticide Biochem. Physiology 90, 42–51. doi:10.1016/j.pestbp.2007.07.009
Villamizar, N., Vera, L. M., Foulkes, N. S., and Sánchez-Vázquez, S. J. (2014). Effect of lighting conditions on zebrafish growth and development. Zebrafish 11, 173–181. doi:10.1089/zeb.2013.0926
Vinagre, C., Madeira, D., Narciso, L., Cabral, H. N., and Diniz, M. (2012). Effect of temperature on oxidative stress in fish: Lipid peroxidation and catalase activity in the muscle of juvenile seabass, Dicentrarchus labrax. Dicentrarchus Labrax. Ecol. Ind. 23, 274–279. doi:10.1016/j.ecolind.2012.04.009
Vindas, M. A., Gorissen, M., Höglund, E., Flik, G., Tronci, V., Damsgard, B., et al. (2017). How do individuals cope with stress? Behavioural, physiological and neuronal differences between proactive and reactive coping styles in fish. J. Exp. Biol. 220, 1524–1532. doi:10.1242/jeb.153213
Volkoff, H., and Rønnestad, I. (2020). Effects of temperature on feeding and digestive processes in fish. Temp. Multidiscip. Biomed. J. 7, 307–320. doi:10.1080/23328940.2020.1765950
Wang, C., Jiang, C., Gao, T., Peng, X., Ma, S., Sun, Q., et al. (2022). Improvement of fish production and water quality in a recirculating aquaculture pond enhanced with bacteria-microalgae association. Aquacul 547, 737420. doi:10.1016/j.aquaculture.2021.737420
Wang, W. N., Wang, A. L., Chen, L., Liu, Y., and Sun, R. Y. (2002). Effects of pH on survival, phosphorus concentration, adenylate energy charge and Na+–K+ ATPase activities of Penaeus chinensis Osbeck juveniles. Aquat. Toxicol. 60, 75–83. doi:10.1016/S0166-445X(01)00271-5
Wedemeyer, G. A., Meyer, F. P., and Smith, L. S. (1976). Diseases of fishes: Environmental stress and fish diseases, 1–192.
Wilson, R. W., and Egginton, S. (1994). Assessment of maximum sustainable swimming performance in rainbow trout (Oncorhynchus mykiss). J. Exp. Biol. 192, 299–305. doi:10.1242/jeb.192.1.299
Wiorek, A., Hussain, G., Molina-Osorio, A. F., Cuartero, M., and Crespo, G. A. (2021). Reagentless acid-base titration for alkalinity detection in seawater. Anal. Chem. 93 (42), 14130–14137. doi:10.1021/acs.analchem.1c02545
World Bank (2021). Blue economy. Available from: www.worldbank.org (Accessed on May 22, 2023).
World bank (2023a). Data World bank. Available from: https://data.worldbank.org/indicator/ER.FSH.PROD.MT?locations=Z4 (Accessed on March 09, 2023).
World Bank (2023b). Total fisheries production (metric tons). Available from: https://data.worldbank.org/indicator/ER.FSH.PROD.MT (Accessed on May 02, 2023).
World Bank (2023c). Total fisheries production (metric tons). Available from: https://data.worldbank.org/indicator/ER.FSH.PROD.MT?end=2020&start=1960&view=chart (Accessed on May 02, 2023).
Worldfishcenter, (2023). Tilapia aquaculture: Potential for India in meeting food, nutritional and livelihood security. Available from: https://worldfishcenter.org/blog/tilapia-aquaculture-potential-india-meeting-food-nutritional-and-livelihood-security (Accessed on May 05, 2023).
Wu, C. C., Blount, J. R., Haimbaugh, A., Heldman, S., Shields, J. N., and Baker, T. R. (2022). Evaluating phenotypic and transcriptomic responses induced by low-level VOCs in Zebrafish: Benzene as an example. Toxics 10, 351. doi:10.3390/toxics10070351
Wu, F., Huang, W., Liu, Q., Xu, X., Zeng, J., Cao, L., et al. (2018). Responses of antioxidant defense and immune gene expression in early life stages of large yellow croaker (pseudosciaena crocea) under methyl mercury exposure. Front. Physiol. 9, 1436. doi:10.3389/fphys.2018.01436
Xing, S. Y., Li, Z. H., Li, P., and You, H. A. (2022). A mini-review of the toxicity of pollutants to fish under different salinities. Bull. Environ. Contam. Toxicol. 108 (6), 1001–1005. doi:10.1007/s00128-022-03528-0
Yadav, V. K., Choudhary, N., Inwati, G., Rai, A., Singh, B., Solanki, B., et al. (2023). Recent trends in the nanozeolites-based oxygen concentrators and their application in respiratory disorders. Front. Med. 10, 1–13. 1147373. doi:10.3389/fmed.2023.1147373
Zahangir, M., Haque, F., Mostakim, F. M., and Islam, S. (2015). Secondary stress responses of zebrafish to different pH: Evaluation in a seasonal manner. Aquacult. Rep. 2, 91–96. ISSN 2352-5134. doi:10.1016/j.aqrep.2015.08.008
Zaheer, Ud D. S., Shah, K., Bibi, N. H., Mahboub, H., and Kakakhel, M. A. (2023). Recent insights into the silver nanomaterials: An overview of their transformation in the food webs and toxicity in the aquatic ecosystem. Water Air Soil Poll. 234, 114. doi:10.1007/s11270-023-06134-w
Zala, S. M., and Penn, D. J. (2004). Abnormal behaviours induced by chemical pollution: A review of the evidence and new challenges. Anim. Behav. 68, 649–664. doi:10.1016/j.anbehav.2004.01.005
Zang, C., Huang, S., Wu, M., Du, S., Scholz, M., Gao, F., et al. (2011). Comparison of relationships between pH, dissolved oxygen and chlorophyll a for aquaculture and non-aquaculture waters. Water Air Soil Pollut. 219, 157–174. doi:10.1007/s11270-010-0695-3
Zhang, C., Wang, N., Xu, Y., Tan, H-Y., Li, S., and Feng, Y. (2018). Molecular mechanisms involved in oxidative stress-associated liver injury induced by Chinese herbal medicine: An experimental evidence-based literature review and network pharmacology study. Int. J. Mol. Sci. 19, 2745. doi:10.3390/ijms19092745
Zhang, W., Cao, Z., Peng, J. L., Chen, B. J., and Fu, S. J. (2010). The effects of dissolved oxygen level on the metabolic interaction between digestion and locomotion in juvenile southern catfish (Silurus meridionalis Chen). Com. Biochem. Physiol. Part A Mol. Int. Physiol. 157, 212–219. doi:10.1016/j.cbpa.2010.06.184
Zhang, W., Cao, Z-D., and Fu, S-J. (2012). The effects of dissolved oxygen levels on the metabolic interaction between digestion and locomotion in Cyprinid fishes with different locomotive and digestive performances. J. Comp. Physiol. B 182, 641–650. doi:10.1007/s00360-012-0644-0
Zhao, C., Zhang, Y., Suo, A., Mu, J., and Ding, D. (2022). Toxicity of tributyltin chloride on haarder (Liza haematocheila) after its acute exposure: Bioaccumulation, antioxidant defense, histological, and transcriptional analyses. Fish. Shellfish Immunol. 130, 501–511. doi:10.1016/j.fsi.2022.09.050
Zhao, W., Liang, M., and Zhang, P. (2010). Effect of yeast polysaccharide on the immune function of juvenile sea cucumber, Apostichopus japonicus Selenka under pH stress. Aquac. Int. 18, 777–786. doi:10.1007/s10499-009-9300-4
Keywords: abiotic factors, disease, economic value, fish health, oxidative stress, physiology, redox regulation, water physicochemical factors
Citation: Menon SV, Kumar A, Middha SK, Paital B, Mathur S, Johnson R, Kademan A, Usha T, Hemavathi KN, Dayal S, Ramalingam N, Subaramaniyam U, Sahoo DK and Asthana M (2023) Water physicochemical factors and oxidative stress physiology in fish, a review. Front. Environ. Sci. 11:1240813. doi: 10.3389/fenvs.2023.1240813
Received: 15 June 2023; Accepted: 01 September 2023;
Published: 15 September 2023.
Edited by:
Wei Li, Dongguan University of Technology, ChinaCopyright © 2023 Menon, Kumar, Middha, Paital, Mathur, Johnson, Kademan, Usha, Hemavathi, Dayal, Ramalingam, Subaramaniyam, Sahoo and Asthana. This is an open-access article distributed under the terms of the Creative Commons Attribution License (CC BY). The use, distribution or reproduction in other forums is permitted, provided the original author(s) and the copyright owner(s) are credited and that the original publication in this journal is cited, in accordance with accepted academic practice. No use, distribution or reproduction is permitted which does not comply with these terms.
*Correspondence: Biswaranjan Paital, Ymlzd2FyYW5qYW5wYWl0YWxAZ21haWwuY29t, YnJwYWl0YWxAb3VhdC5hYy5pbg==; Dipak Kumar Sahoo, ZHNhaG9vQGlhc3RhdGUuZWR1, ZGlwYWtzYWhvbzExQGdtYWlsLmNvbQ==