- 1State Key Laboratory of Loess and Quaternary Geology, Institute of Earth Environment, Chinese Academy of Sciences, Xi’an, China
- 2School of Geography and Tourism, Shaanxi Normal University, Xi’an, China
Agricultural ecosystem is the largest artificial ecosystem on Earth and provide 66% of the world’s food supply. Soil microorganisms are an engine for carbon and nutrient cycling. However, the driving mechanism of soil microbial community structure and carbon and nitrogen transformation mediated by fertilization and planting pattern in rainfed agricultural ecosystems is still unclear. The research was conducted at the Changwu Agricultural Ecology Experimental Station in Shaanxi Province, China. Seven different fertilization and planting pattern were designed. The Phosphate fatty acids (PLFAs) were used to explore the effects of fertilization and plating pattern on the soil microbial community structure and the relationship with soil carbon and nitrogen transformation. The results showed that there were significant differences in soil physical and chemical properties among treatments. Organic fertilizer significantly increased the soil carbon and nitrogen and decreased the soil pH. The contents of total PLFAs and microbial groups in the wheat and corn rotation treatment were the highest. Compared with the change in planting pattern, organic fertilizer had a greater impact on PLFA content and soil ecological processes. The soil microbial community structure has a significantly positive correlation with soil organic carbon (SOC), total carbon (TC), total nitrogen (TN), and total phosphorus (TP). Compared with applying NP fertilizer, applying organic fertilizer significantly increased the soil respiration rate and mineralized nitrogen content while decreasing the soil microbial biomass carbon (MBC). The correlation analysis showed that soil respiration was significantly positively correlated with SOC and TP, and mineralized nitrogen was significantly positively correlated with SOC, nitrate nitrogen, TN and MBC. Structural equation modeling (SEM) showed that the soil respiration rate was significantly positively affected by TC and negatively affected by SWC and explained 63%, whereas mineralized nitrogen was significantly positively influenced by TN and explained 55% of the total variance.
1 Introduction
Soil microorganisms are not only widely involved in various biochemical reactions with element cycling but are also an important medium connecting plants and soil (Zhang Q. et al., 2022). The agricultural ecosystem provides nearly 66% of the food supply, and agricultural soil acts as an important matrix linking crops and underground ecosystem processes (Zhang J. Y. et al., 2022). Agricultural soil microorganisms are of great significance to soil fertility, and their biomass nitrogen and phosphorus are important sources of crop nutrients (Chen et al., 2017). The quality of the soil environment can directly affect the composition of the microbial community and thereby affect crop growth and health (Li et al., 2021). A suitable microbial community structure in agricultural ecosystems could promote crop growth, and the change in soil microbial community structure could be used as an important indicator of soil health and crop growth (Liu Z. H. et al., 2021).
Agricultural crop planting pattern affect soil physicochemical properties and microbial properties (Rashid et al., 2016). Agricultural crop planting pattern mainly include continuous cropping and crop rotation. Research has shown that the biotic and abiotic environments in the soil are significantly different between different crops under the same planting pattern or between different planting patterns of the same crop (Li et al., 2021). The reason is mainly because different planting patterns could affect the physical structure, nutrients, and microbial community structure of the soil (Aimaierjiang et al., 2022). Continuous cropping may lead to a decline in soil fertility and the transformation of soil microorganisms, causing abnormal crop growth (Ma et al., 2004; Pradeep et al., 2010). Crop rotation could balance the utilization of soil nutrients and increase the variety and quantity of beneficial microorganisms in the soil (Asuming-Brempong et al., 2008). For example, soybean rotation can significantly increase bacterial PLFA content, and avoid the process of microbial groups transforming to fungi caused by continuous cropping (Yao et al., 2015). Therefore, scientifically and reasonably selecting crop planting pattern is beneficial for improving the soil environment and promoting the healthy growth of crops.
Fertilization is an important factor affecting crop growth and soil health. Long-term application of chemical nitrogen and phosphorus fertilizers significantly increases soil organic carbon (SOC), total phosphorus (TP), and available phosphorus (AP) (Gao et al., 2021). Fertilization can also alter soil microbial properties, and organic fertilizers or organic‒inorganic combinations can effectively increase the number and activity of microorganisms (Li et al., 2005; Wu et al., 2020). In humid and warm climate conditions, organic fertilizer has a great effect on soil microbial properties and leads to greater microbial diversity and a more stable microbial community (Wang et al., 2017). The combination of organic and inorganic fertilizers increased the number of bacteria and decreased fungi (Nanda et al., 1998). Although chemical fertilizers can effectively improve crop productivity (Liu Z. et al., 2020; Liu J. A. et al., 2021), excessive application of inorganic fertilizers can cause damage to the soil environment, which not only leads to soil quality degradation but also has a negative impact on soil microecological balance (Tiziano et al., 2011; Kour et al., 2020; Yu et al., 2020). It is of great significance to explore the effects of fertilization on soil physicochemical and microbial properties to improve agricultural safety.
Soil organic carbon and nitrogen and their turnover processes determine soil fertility and sustainability (Morales-Rodriguez et al., 2019). Soil carbon and nitrogen mineralization is affected by environmental factors, and generally, the rate of soil organic carbon mineralization increases with increasing temperature (Ma et al., 2016). In addition to temperature, changes in planting and fertilization pattern also cause changes in soil carbon mineralization, which in turn affects crop growth. Nitrogen fertilizer application can prohibit soil carbon mineralization by inhibiting microbial biomass and extracellular enzyme activity (Keeler et al., 2009), while significantly improving soil microbial net nitrogen mineralization (NNM) (Wang et al., 2014). Organic fertilization could increase carbon mineralization by 52%–117% more than chemical inputs in soil, and significantly enhance NNM under no-tillage management in humid conditions (Wang et al., 2011). There has been a report that fertilization has a greater impact on soil microbial community structure than crop rotation (Guo et al., 2020). However, there are still few reports on the effects of fertilization and planting pattern on soil microorganisms and carbon and nitrogen mineralization in rainfed farmland.
The rainfed farmland in the Chinese Loess Plateau is an important agricultural ecosystem and produces vital food for the local people (Lian et al., 2021). To explore the effects of fertilization and planting pattern on soil microbial community structure and microbe-mediated ecological processes of carbon and nitrogen cycling. We used long-term field experiments to address these uncertainties and we hypothesized that 1) fertilization and planting pattern mainly affect the soil carbon and nitrogen contents and affect the microbial community structure and 2) compared with the change in planting pattern, fertilization had a greater influence on the structure and composition of the microbial community. To test these assumptions, the changes in the microbial community and carbon and nitrogen mineralization were tested by the traditional biochemical method. Determining the influence mechanism of fertilization and planting pattern on soil carbon and nitrogen mineralization and soil microbial properties will help to set an effective and safe fertilization pattern under different planting scenarios.
2 Materials and methods
2.1 Site description
The research site is selected in the Changwu Agricultural Ecology Experimental Station of the Chinese Academy of Sciences, Shaanxi Province, China. Geographic coordinates are 107°41′E, 35°14′N, with an altitude of 1,220 m. It belongs to a semihumid continental monsoon climate. The average annual precipitation is 580 mm, the average annual temperature is 9.1°C, and the frost-free period is 171 days. The loose and higher permeability soil is Heilu soil (Cumulic Haplustoll, USDA). The long-term field experiment in this research began in 1984. The initial soil nutrient status was as follows: organic matter = 10.50 g·kg−1, total nitrogen = 0.80 g·kg−1, total phosphorus = 1.26 g·kg−1, and pH = 8.10.
2.2 Experimental treatment and design
This experimental design had 7 different fertilization and planting pattern treatments: 1) wheat continuous cropping without fertilization (W-CK); 2) wheat continuous cropping with NP fertilizer (W-NP); 3) wheat continuous cropping with NP and organic fertilizer (W-NPM); 4) wheat and corn rotation with NP fertilizer (WC-NP); 5) wheat and corn rotation with NP and organic fertilizer (WC-NPM); 6) wheat, millet and pea rotation with NP fertilizer (WMP-NP); and 7) wheat, millet and pea rotation with NP fertilizer and organic fertilizer (WMP-NPM). The fertilization amounts of the three fertilizers were as follows: N (120 kg·ha−1·y−1 CH4N2O), P (90 kg·ha−1·y−1 P2O5), and M (7.5 × 104 kg·ha−1·y−1 manure with 10.6% organic matter content). Each treatment had 3 replicates with a 36 m2 (4 m × 9 m) plot area. Field activities such as weeding and spraying were carried out according to local field management habits.
2.3 Soil sample collection
In June 2019, soil samples were collected after crop harvest. After clearing the dead branches and leaves on the soil surface, a soil sample of 0–20 cm was taken using the five point sampling method. The same treated soil samples in each plot were mixed and bagged, stored at 4°C and taken back to the laboratory. After removing the visible sand, gravel, animal and plant residues, the soil was passed through a 2 mm stainless sieve. The screened soil sample was divided into 3 parts. One part was placed into a 4°C refrigerator to determine the soil microbial biomass and soil respiration, one part was stored at −86°C to test PLFAs, and the third part was used to determine the soil physical and chemical properties after air drying with protection from sunlight.
2.4 Measurement items and methods
2.4.1 Determination of soil physical and chemical properties
The soil water content (SWC) was determined using the oven drying method; total carbon (TC) in soil was measured using a carbon analyzer (Vario TOC, Elemental, Hanau, Germany); soil organic carbon (SOC) was measured using the potassium dichromate external heating method; total phosphorus (TP) in soil was measured using the Olsen method; a fully automatic Kjeldahl nitrogen analyzer was used to measure soil total nitrogen (TN); ammonium nitrogen (NH4+-N) and nitrate nitrogen (NO3−-N) in soil were extracted with K2SO4 solution and measured using a continuous flow analyzer (Autoanalyzer 3, Bran Luebbe, Germany); available phosphorus (AP) in soil was measured using the Olsen method; the organic phosphorus (OP) in the soil was determined by the high-temperature burning method; soil pH (water/soil at 2.5:1) was measured by a glass electrode pH meter; soil particle composition was measured using the Malvern 2000 laser particle size analyzer; and mineralized nitrogen was measured using the biological culture method (Bao, 2000).
2.4.2 Determination of soil microbial community structure and microbial indicators
The phosphate fatty acid (PLFA) method was used to determine the soil microbial community structure. 8 g of freeze-dried soil was weighed for the test. After operating with the protocol, phospholipid fatty acids were extracted and determined using a gas chromatograph (Agilent GC-7890B) combined with the MIDI microbial identification system Sherlock 6.2 (Frosteg et al., 1991). Bacteria were represented with 15:0, 17:0, 15:0iso, 15:0anteiso, 16:0iso, 16:1w7c, 17:0iso, 17:0anteiso, 17:0cycloω7c, 18:1ω7c and 18:1ω5c (Tunlid et al., 1989; Frosteg et al., 1993). Fungi were represented with 16:1ω5c, 18:1ω9c, 18:2ω6c and 20:1ω9c (Federle et al., 1986; Baath, 2003). Arbuscular mycorrhizal fungi (AMF) were represented with 16:1ω5c (Nordby et al., 1981; Olsson, 1999). Actinomycete were represented with 10Me16:0, 10Me17:0 and 10Me18:0 (Zelles, 1997). Gram-positive bacteria (G+) were represented with 15:0iso, 15:0anteiso, 16:0anteiso, 17:0iso, 17:1isoω9c, 18:0iso, 16:0iso, 17:0anteiso and 17:1anteisoω7c. Gram-negative bacteria (G−) were represented with 19:0cycloω7c, 12:1ω5c, 16:1ω7c, 18:1ω5c, 17:1ω8c, 12:1ω8c, 16:1ω9c, 17:0cycloω7c, 19:0cycloω7c and 21:1ω9c (Frosteg and Baath, 1996; Zelles, 1997).
Soil microbial biomass carbon (MBC) and microbial biomass nitrogen (MBN) were extracted and measured using the chloroform fumigation method. Soil respiration and mineralized nitrogen were measured by alkali absorption titration. The samples were incubated continuously at 25°C for 28 days and removed on days 1, 3, 7, and 14 to calculate the soil respiration rate during the incubation process by titration. At 28 days, the soil nitrate nitrogen (NO3−-N) and ammonium nitrogen (NH4+-N) contents before and after cultivation were measured using a continuous flow analyzer (Autoanalyzer 3, Bran Luebbe, Germany), and the soil mineralization nitrogen, soil net nitrification rate, and net mineralization rate were calculated as follows:
In the formula, d refers to the cultivation days of the soil used for measuring mineralized nitrogen, which in this research is 28 days. c(NO3−-N)1, c(NO3−-N)2, c(NH4+-N)1 and c(NH4+-N)2 represent the concentrations of NO3−-N and NH4+-N before and after incubation. Anit and Amin represent the net accumulation of nitrate nitrogen and mineralized nitrogen content, respectively. Vnit and Vmin represent the net nitrification rate and net mineralization rate of nitrogen, respectively.
2.5 Statistical analysis
Data Processing System (DPS) software was used for data analysis (Tang and Zhang, 2013). One-way ANOVA was performed with least significant difference (LSD) analysis. Structural equation modeling (SEM) was used to analyze the effect of different treatments on mineralized nitrogen and soil respiration by soil microbial community structure. The maximum likelihood estimation method was used with Amos V18.0 (IBM, Chicago, IL, United States). R software was used to draw figures. The data in this research are expressed as the average ± standard deviation.
3 Results
3.1 Soil physical and chemical properties under different treatments
Planting pattern and fertilization treatments had significant effects on soil physical and chemical properties (Table 1). Compared with the W-CK, SOC was significantly increased in the W-NPM and WC-NPM (p < 0.05), whereas TC was significantly decreased in the W-NP, WC-NP and WMP-NP (p < 0.05). All treatments significantly enhanced NO3−-N, while only W-NPM, WC-NP and WMP-NP increased NH4+-N and WC-NPM increased TN (p < 0.05). AP and TP were significantly higher than those in the W-CK in all other treatments, and OP was only significantly higher in the WMP-NP treatment (p < 0.05). The pH and SWC both significantly decreased in all the treatments, but the physical clay content only increased in the WC-NP treatment (p < 0.05).
In the wheat continuous treatment, SOC, TC, NH4+-N, TN, AP and TP were significantly higher in the organic fertilizer used plot whereas SWC had a reverse trend (p < 0.05). The wheat and corn rotation plot with organic fertilizer had significantly higher SOC, TC, NO3−-N, TN, AP and TP, and significantly lower NH4+-N, pH and SWC than the chemical fertilizer plot (p < 0.05). The plot with wheat, millet and pea rotation and organic fertilizer obviously enhanced AP, TP and SWC but abated NH4+-N, NO3−-N and OP (p < 0.05).
In the chemical fertilizer treatment, AP and TP significantly increased with the number of rotations whereas SWC decreased (p < 0.05). NH4+-N and NO3−-N were significantly influenced by rotational management with a reverse trend (p < 0.05). In the organic fertilizer plot, NH4+-N significantly decreased with the number of rotations, pH decreased first and then increased, and NO3−-N, TN and AP had a reverse trend (p < 0.05).
3.2 Soil microbial community structure in different treatments
Planting pattern and fertilization had a significant effect on the soil microbial community structure (Figure 1). Compared with W-CK, the total PLFA content was significantly decreased in W-NP and significantly increased in W-NPM, WC-NP and WC-NPM (p < 0.05). There was no obvious difference among the W-CK, WMP-NP and WMP-NPM (Figure 1A). The trend of bacteria, fungi, actinomycete, AMF, G+ and G− PLFAs all showed a similar pattern with the total PLFAs. The Fungal/Bacteria ratio was not significantly different.
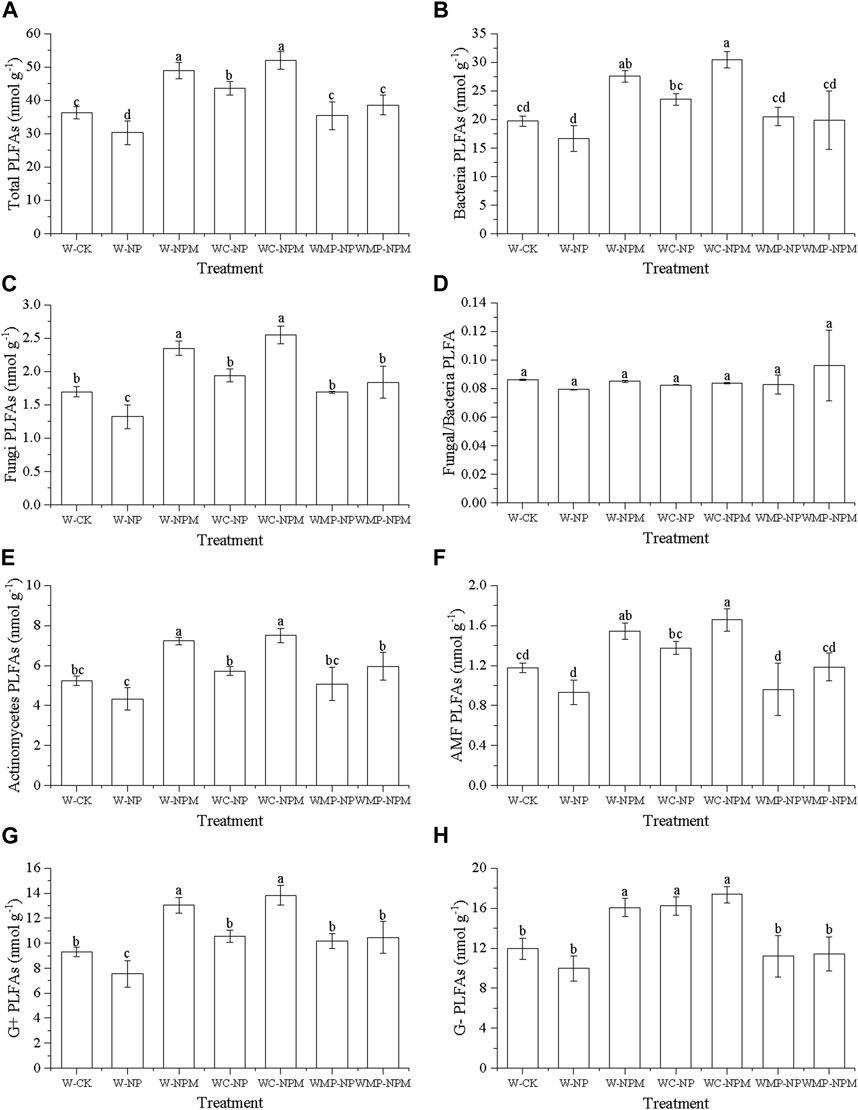
FIGURE 1. Trends of Total PLFAs (A), Bacteria PLFAs (B), Fungi PLFAs (C), Fungi/Bacteria PLFAs (D), Actinomycete PLFAs (E), AMF PLFAs (F), Gram positive bacteria PLFAs (G) and Gram negative bacteria PLFAs (H) in soil microbial communities under different treatments. The same letter above each column indicates no significantly difference (p > 0.05); different letters indicate significant difference (p < 0.05).
The plot with organic fertilizer had higher microbial PLFAs than the chemical fertilizer treatment, and this effect was weaked in the wheat, millet and pea rotation scenario. As the rotation number increased, all the microbial PLFAs first increased then decreased. Overall, the application of organic fertilizers has a greater impact on soil microbial PLFAs than rotation management.
A heatmap was used to show the correlation between soil physical and chemical properties and soil microbial communities (Figure 2). The PLFAs of bacteria, gram positive bacteria and fungi were significantly correlated with SOC, TC, TN, SWC and TP (p < 0.05). The PLFAs of actinomycete and AM fungi were significantly correlated with SOC, TC and TN (p < 0.05). Gram negative bacteria were only correlated with SOC and TN (p < 0.05).
3.3 Soil microbial biomass under different treatments
Fertilization and planting pattern have significant effects on soil microbial biomass. Compared with W-CK, W-NP and WMP-NP significantly increased MBC, whereas WC-NPM and WMP-NPM decreased MBC (p < 0.05). The organic fertilizer had a negative effect on MBC and the effect obviously increased as the rotation number increased (Figure 3A). The MBN was significantly decreased in the W-NP and WMP-NP treatments but increased in WC-NPM (p < 0.05). Contrary to MBC, MBN was positively influenced by organic fertilizer and the effect was lower in the wheat and corn rotation treatment (Figure 3B).
3.4 Mineralization of soil C and N under different treatments
3.4.1 Soil respiration rate of different treatments
Fertilization and rotation significantly affected the soil respiration rate (Figure 4A). Compared with the W-CK, the soil respiration rate was significantly increased in the W-NPM, WC-NPM, WMP-NP and WMP-NPM (p < 0.05). Under the same planting pattern, organic fertilizer significantly increased the soil respiration rate in the wheat continuous cropping, and wheat and corn rotation treatments (p < 0.05), whereas a converse trend was observed in the wheat, millet and pea rotation treatments, although it was not statistically significant. Under the chemical fertilizer scenario, the soil respiration rate increased as the rotation number increased, while this trend reversed when the organic fertilizer was applied.
There were significant differences in the soil respiration entropy among the different treatments (Figure 4B). The soil respiration entropy in the W-NPM, WC-NPM and WMP-NPM treatments was significantly higher than that in the W-CK treatment (p < 0.05). Under the same planting pattern, organic fertilizer significantly enhanced soil respiration entropy, and the wheat and corn rotation system was the most sensitive treatment (p < 0.05). Under the same fertilization treatment, chemical fertilizer had no obvious effect on the soil respiration entropy under different rotation plots, whereas the soil respiration entropy first increased and then decreased with increasing rotation number in the plot with organic fertilizer (p < 0.05).
The soil respiration rate was significantly positively correlated with SOC, NO3−-N and TP, and negatively correlated with pH and SWC (p < 0.05). Soil respiration entropy was significantly positively correlated with SOC, NO3−-N, TP and MBN, and negatively correlated with NH4+-N, pH and MBC, p < 0.05 (Figure 5).
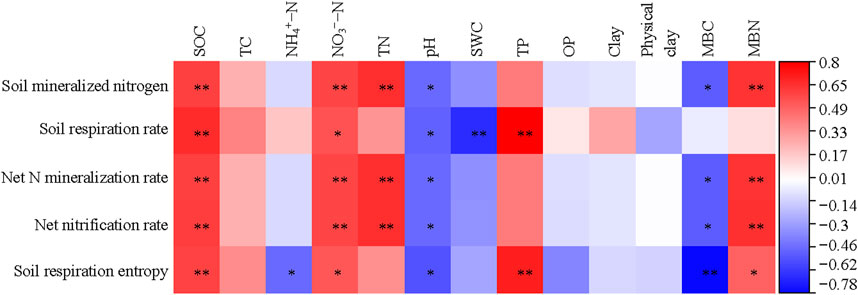
FIGURE 5. Correlation analysis of soil carbon and nitrogen mineralization with soil physiochemical and microbial properties.
3.4.2 Mineralization of soil nitrogen under different treatments
The field management practice, fertilization and planting rotation, significantly affected soil mineralized nitrogen, net nitrogen mineralization rate and net nitrification rate with a similar trend (Figure 6). Compared with the W-CK, the soil mineralized nitrogen, net nitrogen mineralization rate and net nitrification rate were all significantly increased in the W-NPM, WC-NP and WC-NPM (p < 0.05). Under the same planting pattern, the soil mineralized nitrogen, net nitrogen mineralization rate and net nitrification rate were significantly higher in the plot with organic fertilizer but this effect was not significant in the wheat, millet and pea rotation treatment (p < 0.05). Under the same fertilization treatment, the soil mineralized nitrogen, net nitrogen mineralization rate and net nitrification rate all increased from the wheat continuous cropping to wheat and corn rotation treatment, and then decreased in the wheat, millet and pea rotation treatment (p < 0.05).
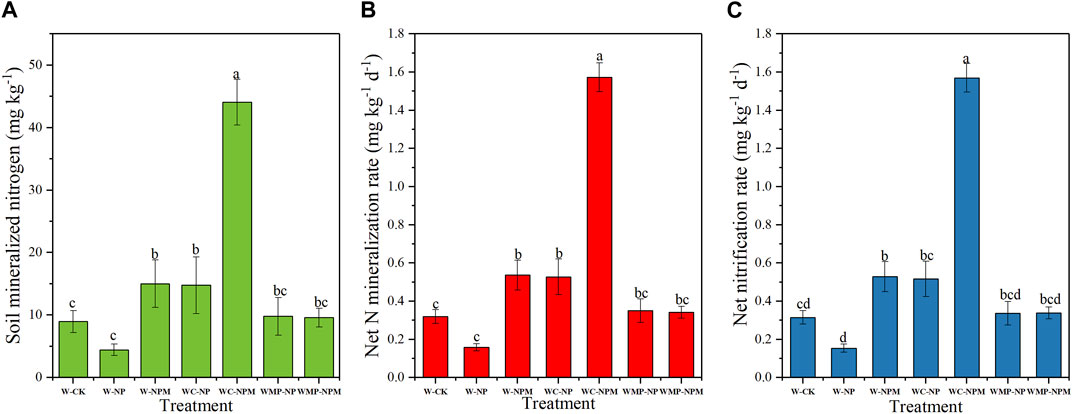
FIGURE 6. Soil mineralization nitrogen (A), net N mineralization rate (B) and net nitrification rate (C) in different treatments.
Soil mineralized nitrogen, net nitrogen mineralization rate and net nitrification rate were all significantly positively correlated with SOC, NO3−-N, TN and MBN, and negatively correlated with pH and MBC, p < 0.05, (Figure 5).
3.5 Effect of fertilization and planting pattern on soil microbial community structure and soil carbon and nitrogen mineralization
Based on structural equation modeling, the driving mechanisms of different treatments on mineralized nitrogen and soil respiration rate were analyzed (Figure 7, χ2 = 17.175, df = 10.000, p = 0.071, CFI = 0.916, GFI = 0.839, RMSEA = 0.189, AIC = 69.175). The results of the SEM showed that both TN and SOC directly positively affected total PLFAs and explaining 58% of the total variance with TC and SWC. The fungi/bacteria PLFAs were significantly negatively influenced by TN with −0.65 standardized path coefficients, and significantly positively affected by SOC with 0.63 standardized path coefficients. The combination of TC, TN, SOC and SWC explained 84% of its total variance. Mineralized nitrogen was significantly positively affected by TN, and 55% of the total variance was explained by soil environmental factors and soil microbial community structure. The soil respiration rate was significantly positively affected by TC and negatively affected by SWC and was explained 63% by soil physical, chemical and microbial properties.
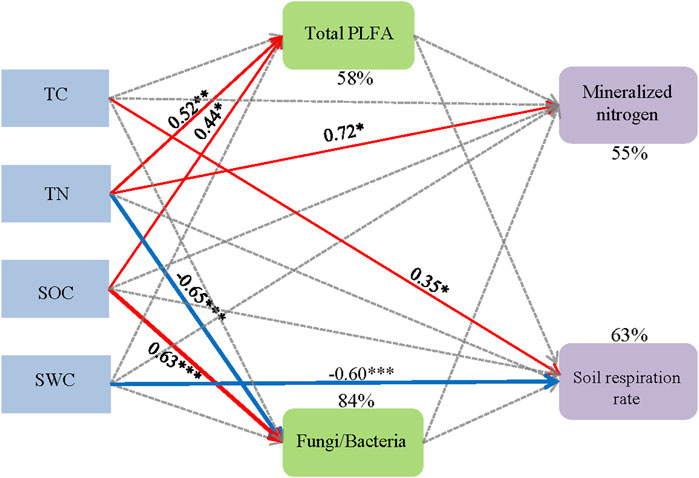
FIGURE 7. Structural equation model shows the effect of fertilization and planting pattern on soil microbial community structure and soil carbon and nitrogen mineralization. Note: The model fits: χ2 = 17.175, df = 10.000, p = 0.071, CFI = 0.916, GFI = 0.839, RMSEA = 0.189, AIC = 69.175. The numbers on the arrows are standardized path coefficients (correlation coefficients), and asterisks following the numbers indicate significant relationships (***p < 0.001, **p < 0.01, *p < 0.05). Red solid arrows indicate significant positive relationships, blue solid arrows indicate significant negative relationships, and gray dashed arrows indicate path coefficients >0.05. The width of the arrows shows the strength of the relationship. Percentages (R2) close to endogenous variables indicate the variance explained by the soil factors.
4 Discussion
4.1 Effects of fertilization and planting pattern on soil physical and chemical properties
Fertilization and planting pattern, as important field management practices, can affect soil nutrients and alter soil physicochemical characteristics (Wang W. et al., 2015; Cai et al., 2019). Soil nutrients are the main source for crop nutrition, and the level of soil nutrient content affects the survival and health of crops. Similar to other research, fertilization can significantly increase soil NH4+-N, NO3−-N, TN, AP, TP, and clay content, and reduce soil pH and SWC in the wheat continuous cropping treatment (Guo et al., 2010; Sun et al., 2014; Lusiba et al., 2017; Liu J. et al., 2020; Lin et al., 2022). The reason for this change in soil physicochemical properties may be that fertilizers contain a large amount of nitrogen and phosphorus. The application of fertilizers can quickly supplement the nutrient elements, thereby greatly increasing the available nitrogen and phosphorus. The increased available nutrients could enhance microbial activity and accelerate the transformation of nutrients (Zhang et al., 2013). The hydrolysis of urea causes a short-term and rapid decrease in soil pH, and lower pH and sufficient urea hydrolysates (NH4+-N) significantly stimulate nitrification in soils (Zhao et al., 2014). This is consistent with the significant increase in nitrate nitrogen content and the opposite trend of nitrate and ammonia nitrogen changes after fertilization in this study. Soil pH is an important factor affecting soil quality. Nitrogen in fertilizer promotes the absorption of soil cations by crop roots, resulting in the production of more hydrogen ions and a decrease in the soil pH value with soil acidification (Cai et al., 2019; Dong et al., 2021b). In addition, the H+ produced by nitrification will also reduce the pH (Dong et al., 2021a).
Compared with the application of inorganic fertilizers, the application of chemical with organic fertilizers can increase SOC and reduce SWC and pH. After being applied to the soil, organic fertilizers increase the rate of soil nutrient accumulation, improve the soil physical structure, and create a rich microenvironment, which is conducive to microbial reproduction and ultimately drives the carbon nitrogen cycle and is more conducive to the accumulation of organic carbon components (Chen et al., 2019). The decrease in pH may be due to the combination of organic and inorganic fertilizers regulating the carbon nitrogen ratio of soil, promoting microbial growth, increasing soil microbial diversity, stimulating soil enzyme activity, promoting organic matter degradation, and generating more low molecular organic acids such as acetic acid (CH3COOH) (Zhang et al., 2021). This research also found that under the same fertilizer conditions, the soil nutrient content was also affected by planting pattern. Under the same fertilization conditions, the SOC, TN, AP, and TP of the wheat corn rotation were higher than those of the wheat continuous cropping. This may be due to differences in nutrient absorption among different crops in rotation. Reasonable crop rotation can achieve balanced utilization of soil nutrients and achieve the effect of land nutrition. Meanwhile, compared to wheat continuous cropping, the SWC of wheat corn rotation decreases, possibly due to the more developed corn roots and high water demand, resulting in a decrease in SWC (Wu et al., 2021).
4.2 Effects of fertilization and planting pattern on microbial community structure
Soil microorganisms are an important component of soil ecosystems and play a notable role in maintaining soil ecosystem function and services. Fertilization significantly affects the abundance and diversity of soil microorganisms (Chinnadurai et al., 2014; Tamilselvi et al., 2015). In this research, compared with no fertilization, the application of chemical with organic fertilizer significantly increased the content of total PLFAs, bacteria, fungi, G+, G−, AMF and actinomycete PLFAs in the wheat continuous cropping treatment, whereas the NP fertilizer had the opposite effect. The application of organic fertilizer can provide a suitable survival environment for microorganisms (Wang et al., 2020). The application of organic fertilizers could increase the organic matter content of the soil, improve the soil physicochemical traits and the structure of the soil, improve the soil fertility, provide a suitable environment for microbial growth and promote microbial activity, and they also directly introduced microorganisms in organic fertilizers into the soil and played the roles of “inoculating” and “importing” (Zeng et al., 2007; Dong et al., 2014). Long-term application of inorganic fertilizers alters soil physicochemical traits and inhibits the activity of soil microorganisms; at the same time, exogenous organic carbon sources are insufficiently supplemented, and there is not enough energy to satisfy the growth and development of microorganisms, resulting in a decline in the number of microorganisms, while the input of organic fertilizers exactly complements this shortcoming, thereby increasing soil microbial PLFA contents (Li et al., 2020).
With the same fertilization, the PLFA content was different among the different rotation systems. In this research, the PLFA content in the wheat millet pea rotation treatment was lower than that in the wheat corn rotation treatment, indicating that rotation has a certain impact on the soil microbial community structure. Compared with the effect of fertilization, the planting pattern has a smaller impact on the soil microbial community structure, which may be because the application of organic fertilizer allows microorganisms to obtain a large amount of nutrients, or the presence of a large number of different microbial groups in the organic fertilizer. The differences in soil microbial community structure among different treatments are mainly related to whether organic fertilizers are applied, and although planting pattern have an impact on microbial community structure, the effect is relatively small.
4.3 Effects of fertilization and planting pattern on soil carbon and nitrogen mineralization
Soil microbial biomass serves as both a reservoir of available nutrients and a driving force for soil nutrient cycling and organic matter transformation (Yang et al., 2017). MBN is a key interconversion of inorganic and organic N in soils and often serves as a sensitive indicator for the evolution of soil fertility status and soil quality. Previous studies have shown that the application of chemical fertilizers can enhance MBC and MBN (Daniel and Kate, 2014). The results of this study differed in that, compared with no fertilization, the application of NP and organic manure had no significant effect on MBC and MBN, but the application of NP significantly increased the MBC content and decreased the MBN content. This may be due to the difference in the amount of NP applied compared to other studies, or it may be related to soil cultivation methods or background physicochemical indicators, resulting in the application of NP affecting microorganisms and causing a decrease in MBN. In addition, research has found that compared to applying NP, applying NP and organic fertilizer significantly reduces MBC content and increases MBN content. This may be due to unreasonable chemical fertilizer application, resulting in insufficient carbon sources. The increase in MBN may be due to the long-term input of exogenous organic materials, which can regulate the soil nitrogen supply (Liu et al., 2012).
Soil respiration is often used to measure the total activity of soil microorganisms and to evaluate soil fertility and is affected by fertilization and planting pattern. Compared with no fertilization, fertilization can significantly improve soil respiration, except when chemical fertilizer is applied in wheat continuous and wheat and corn rotation systems. This may be because fertilizer improves the availability of nitrogen in the soil, promotes the growth of crop roots and their secretions, and increases microbial respiration (Ding et al., 2006; Li et al., 2010). Compared with applying NP, applying NP and organic fertilizer can significantly improve soil respiration in wheat continuous and wheat and corn rotation systems. In addition, by comparing W-NP, W-NPM, WC-NP and WC-NPM, although rotation had an impact on soil respiration, the impact was far less than that of the combined application of organic fertilizer. The main reason may be that the combination of organic and inorganic fertilizers significantly increases the soil organic matter content and thus increases the number of soil microorganisms, thereby increasing CO2 emissions from microbial respiration (Han et al., 2008). Different soil microenvironments lead to different soil respiration (Raich and Tufekciogul, 2000), and changes in fertilization and planting pattern can change soil physical and chemical properties, which indirectly affect soil respiration by affecting the number and distribution of microorganisms (Zhou et al., 2011).
The nitrogen required by crops comes not only from the input of exogenous fertilizer but also from the inorganic nitrogen released by soil organic nitrogen mineralization. Therefore, studying the changes in soil nitrogen mineralization under different fertilization and planting pattern is crucial for the nitrogen supply to crops (Zhang et al., 2019). In this study, the trends of changes in soil mineralization nitrogen content, soil net nitrification rate, and soil net mineralization rate were the same among the different treatments. When the fertilization was the same, the wheat and corn rotation treatment had the highest soil mineralization nitrogen content, soil net mineralization rate, and soil net nitrification rate. The combination of organic fertilizer and wheat and corn rotation has a promoting effect on soil N transformation. According to the heatmap analysis in Figure 5, there is a highly significant positive correlation between soil mineralization nitrogen content and SOC, nitrate nitrogen, TN, and MBN, indicating that the richer the soil organic carbon, nitrate nitrogen, TN, and MBN content, the more substrates and more conducive to mineralization (He et al., 2005; Wang S. C. et al., 2015). Soil urease and protease are key enzymes for soil nitrogen conversion, and their activity is closely related to the intensity of soil nitrogen conversion and soil nitrogen supply capacity (Wang et al., 2010). It is speculated that the impact mechanism of fertilization and planting pattern on N mineralization is, on the one hand, by changing the mineralization substrate and the number of related microorganisms, and on the other hand, it may affect enzyme activity by changing soil physical and chemical properties, thereby affecting nitrogen mineralization.
5 Conclusion
There were significant differences in soil physicochemical factors and microbial community structure among the different fertilization and planting pattern. Compared with applying NP fertilizer, applying organic fertilizer significantly increased soil organic matter and nutrients and decreased SWC and soil pH. The wheat and corn rotation treatment had the highest content of total PLFAs and microbial groups. Applying organic fertilizer can significantly increase the content of PLFAs in the soil. Increasing organic fertilizer mainly affects the content of various microorganisms and total PLFAs in the soil by changing the content of SOC and TN. Compared with applying organic fertilizer, changes in the planting pattern had less impact on the structure of the soil microbial community. Both increasing organic fertilizer application and changing planting pattern can affect soil respiration and soil mineralized nitrogen content. Overall, increasing the application of organic fertilizers has a greater impact on soil ecological processes than changes in planting pattern. The soil microbial community structure (fungi:bacteria) was significantly negatively correlated with total nitrogen and positively correlated with soil organic carbon. Soil respiration was negatively affected by soil water content and positively affected by total carbon. Soil mineralized nitrogen was positively influenced by total nitrogen. In summary, compared to applying NP fertilizer, applying organic fertilizer has a more significant effect on improving soil quality in agricultural ecosystems. Compared with the planting pattern, the application of organic fertilizer has a higher impact on soil properties.
Data availability statement
The original contributions presented in the study are included in the article/Supplementary Material, further inquiries can be directed to the corresponding author.
Author contributions
YW conceptualized the experiment, wrote and edited the manuscript. QL wrote the first original draft. CL designed the research experiment and edited the manuscript. All authors contributed to the article and approved the submitted version.
Funding
This work was funded by the project of Science and Technology Department of Shaanxi Province (2022NY-074), the National Natural Science Foundation of China (41501255 and 41671269), the Xi’an Science and Technology Project (21NYYF0033) and the Fundamental Research Funds for the Central Universities (SYJS202224).
Acknowledgments
The authors would like to thank YX for performing the experiments and collecting the data.
Conflict of interest
The authors declare that the research was conducted in the absence of any commercial or financial relationships that could be construed as a potential conflict of interest.
Publisher’s note
All claims expressed in this article are solely those of the authors and do not necessarily represent those of their affiliated organizations, or those of the publisher, the editors and the reviewers. Any product that may be evaluated in this article, or claim that may be made by its manufacturer, is not guaranteed or endorsed by the publisher.
References
Aimaierjiang, A., Huang, J., Xu, H. Q., Chen, F. L., Xiao, J. X., Cheng, Z. J., et al. (2022). Effects of different planting patterns on soil physicochemical properties and microbial characteristics. J. South. Agric. 53, 3369–3379. doi:10.3969/j.issn.2095-1191.2022.12.008
Asuming-Brempong, S., Gantner, S., Adiku, S. G. K., Archer, G., Edusei, V., and Tiedje, J. M. (2008). Changes in the biodiversity of microbial populations in tropical soils under different fallow treatments. Soil Biol. biochem. 40, 2811–2818. doi:10.1016/j.soilbio.2008.08.010
Baath, E. (2003). The use of neutral lipid fatty acids to indicate the physiological conditions of soil fungi. Microb. Ecol. 45, 373–383. doi:10.1007/s00248-003-2002-y
Cai, A. D., Xu, M. G., Wang, B. R., Zhang, W. J., Liang, G. P., Hou, E. Q., et al. (2019). Manure acts as a better fertilizer for increasing crop yields than synthetic fertilizer does by improving soil fertility. Soil till. Res. 189, 168–175. doi:10.1016/j.still.2018.12.022
Chen, J., Liang, G. Q., Zhou, W., Wang, X. B., Sun, J. W., Liu, D. H., et al. (2019). Responses of soil organic carbon and nitrogen fractions to long-term organic fertilization under rice-wheat rotation. J. Plant Nutr. Fertilizers 25, 36–44. doi:10.11674/zwyf.18138
Chen, J., Zheng, M. J., Pang, D. W., Yin, Y. P., Han, M. M., Li, Y. X., et al. (2017). Straw return and appropriate tillage method improve grain yield and nitrogen efficiency of winter wheat. J. Integr. Agric. 16, 1708–1719. doi:10.1016/s2095-3119(16)61589-7
Chinnadurai, C., Gopalaswamy, G., and Balachandar, D. (2014). Impact of long-term organic and inorganic nutrient managements on the biological properties and eubacterial community diversity of the Indian semi-arid Alfisol. Arch. Agron. Soil Sci. 60, 531–548. doi:10.1080/03650340.2013.803072
Daniel, G., and Kate, M. S. (2014). Long-term effects of mineral fertilizers on soil microorganisms – a review. Soil Biol. biochem. 75, 54–63. doi:10.1016/j.soilbio.2014.03.023
Ding, W. X., Meng, L., Yin, Y. F., Cai, Z. C., and Zheng, X. H. (2006). CO2 emission in an intensively cultivated loam as affected by long-term application of organic manure and nitrogen fertilizer. Soil Biol. biochem. 39, 669–679. doi:10.1016/j.soilbio.2006.09.024
Dong, W. Y., Zhang, X. Y., Dai, X. Q., Fu, X. L., Yang, F. T., Liu, X. Y., et al. (2014). Changes in soil microbial community composition in response to fertilization of paddy soils in subtropical China. Appl. Soil Ecol. 84, 140–147. doi:10.1016/j.apsoil.2014.06.007
Dong, Y., Yang, J. L., Zhao, X. R., Yang, S. H., Jan, M., Peter, D., et al. (2021a). Seasonal dynamics of soil pH and N transformation as affected by N fertilization in subtropical China: An in situ15N labeling study. Sci. Total Environ. 816, 151596. doi:10.1016/j.scitotenv.2021.151596
Dong, Y., Yang, J. L., Zhao, X. R., Yang, S. H., and Zhang, G. L. (2021b). Contribution of different proton sources to the acidification of red soil with maize cropping in subtropical China. Geoderma 392, 114995. doi:10.1016/j.geoderma.2021.114995
Federle, T. W., Dobbins, D. C., Thornton-Manning, J. R., and Jones, D. D. (1986). Microbial biomass, activity, and community structure in subsurface soils. Groundwater 24, 365–374. doi:10.1111/j.1745-6584.1986.tb01013.x
Frosteg, R. D, Å., Tunlid, A., and Baath, E. (1991). Microbial biomass measured as total lipid phosphate in soils of different organic content. J. Microbiol. Methods. 14, 151–163. doi:10.1016/0167-7012(91)90018-l
Frosteg, R. D, Å., Tunlid, A., and Baath, E. (1993). Phospholipid fatty acid composition, biomass, and activity of microbial communities from two soil types experimentally exposed to different heavy metals. Appl. Environ. Microbiol. 59, 3605–3617. doi:10.1128/aem.59.11.3605-3617.1993
Frosteg, R. D. A., and Baath, E. (1996). The use of phospholipid fatty acid analysis to estimate bacterial and fungal biomass in soil. Biol. Fertil. Soils. 22, 59–65. doi:10.1007/bf00384433
Gao, X. F., Yan, B. S., Wu, C. X., and Wang, G. L. (2021). Effects of long-term fertilization on soil quality and millet yield on slope farmland in loess hilly areas. Agric. Res. Arid Areas 39, 76–83. doi:10.7606/j.issn.1000-7601.2021.05.10
Guo, J. H., Liu, X. J., Zhang, Y., Shen, J. L., Han, W. X., Zhang, W. F., et al. (2010). Significant acidification in major Chinese croplands. Science 327, 1008–1010. doi:10.1126/science.1182570
Guo, Z. B., Wan, S. X., Hua, K. K., Yin, Y., Chu, H. Y., Wang, D. Z., et al. (2020). Fertilization regime has a greater effect on soil microbial community structure than crop rotation and growth stage in an agroecosystem. Appl. Soil Ecol. 149, 103510. doi:10.1016/j.apsoil.2020.103510
Han, G. X., Zhou, G. S., and Xu, Z. Z. (2008). Research and prospects for soil respiration of farmland ecosys tems in China. Chin. J. Plant Ecol. 32, 719–733. doi:10.3773/j.issn.1005-264x.2008.03.022
He, F. Y., Yin, B., Cai, G. X., Jin, X. X., and Li, H. X. (2005). Comparison of nitrogen mineralization and nitrification in vegetable and dry grain soils. Chin. J. Soil Sci. 36, 41–44. doi:10.19336/j.cnki.trtb.2005.01.012
Keeler, B. L., Hobbie, S. E., and Kellogg, L. E. (2009). Effects of long-term nitrogen addition on microbial enzyme activity in eight forested and grassland sites: Implications for litter and soil organic matter decomposition. Ecosystems 12, 1–15. doi:10.1007/s10021-008-9199-z
Kour, D., Rana, K. L., Yadav, A. N., Yadav, N., Kumar, M., Kumar, V., et al. (2020). Microbial biofertilizers: Bioresources and eco-friendly technologies for agricultural and environmental sustainability. Biocatal. Agric. Biotechnol. 23, 101487. doi:10.1016/j.bcab.2019.101487
Li, J. M., Ding, W. X., and Cai, Z. C. (2010). Effects of nitrogen fertilization on soil respiration during maize growth season. Chin. J. Appl. Ecol. 21, 2025–2030. doi:10.13287/j.1001-9332.2010.0293
Li, L. R., Feng, J. L., Liu, M. M., Mei, H., Kang, Z. Y., and Cai, Q. N. (2021). Effect of crop planting patterns on soil microorganisms and crop pests in farmland. Chin. Agric. Sci. Bull. 37, 99–106. doi:10.11924/j.issn.1000-6850.casb2020-0789
Li, M. L., Chen, Y. T., Hong, X. F., Qiao, Y. Y., Wang, Q. X., Chen, X. J., et al. (2020). Effects of nitrogen management on soil microbial community structure in paddy fields. Acta Agric. Zhejiangensis 32, 308–316. doi:10.3969/j.issn.1004-1524.2020.02.15
Li, X. Y., Zhao, B. Q., Li, X. H., Li, Y. T., Sun, R. L., Zhu, L. S., et al. (2005). Effects of different fertilization systems on soil microbe and its relation to soil fertility. Sci. Agric. Sin. 38, 1591–1599. doi:10.3321/j.issn:0578-1752.2005.08.014
Lian, X., Piao, S., Chen, A., Huntingford, C., Fu, B., Li, L. Z. X., et al. (2021). Multifaceted characteristics of dryland aridity changes in a warming world. Nat. Rev. Earth Env. 2, 232–250. doi:10.1038/s43017-021-00144-0
Lin, X., Chen, X. L., Wan, S. M., Wang, S. A., Zhang, L., Wang, X. J., et al. (2022). Socket shield technique: A systemic review and meta-analysis. Soils Crops 11, 226–235. doi:10.11689/j.issn.2095-2961.2022.02.012
Liu, J. A., Shu, A. P., Song, W. F., Shi, W. C., Li, M. C., Zhang, W. X., et al. (2021a). Long-term organic fertilizer substitution increases rice yield by improving soil properties and regulating soil bacteria. Geoderma 404, 115287. doi:10.1016/j.geoderma.2021.115287
Liu, J., Li, C. Y., Xing, Y. W., Wang, Y., Xue, Y. L., Wang, C. R., et al. (2020a). Effects of long-term fertilization on soil organic phosphorus fractions and wheat yield in farmland of Loess Plateau. Chin. J. Appl. Ecol. 31, 157–164. doi:10.13287/j.1001-9332.202001.028
Liu, Y. R., Yu, J., Li, X., Xu, Y. C., and Shen, Q. R. (2012). Effects of combined application of organic and inorganic fertilizers on soil microbiological characteristics in a wheat-rice rotation system. J. Agro-Environment Sci. 31, 989–994. (in Chinese with English abstract).
Liu, Z., Guo, Q., Feng, Z. Y., Liu, Z. D., Li, H. Y., Sun, Y. F., et al. (2020b). Long-term organic fertilization improves the productivity of kiwifruit (Actinidia chinensis Planch) through increasing rhizosphere microbial diversity and network complexity. Appl. Soil Ecol. 147, 103426. doi:10.1016/j.apsoil.2019.103426
Liu, Z. H., Huang, F. Y., Li, J. L., Zhang, P., Yang, B. P., Ding, R. X., et al. (2021b). Effects of farmland mulching patterns on soil microbial diversity and community structure in dryland. Acta Ecol. Sin. 41, 2750–2760. doi:10.5846/stxb201904130739
Lusiba, S., Odhiambo, J., and Ogola, J. (2017). Effect of biochar and phosphorus fertilizer application on soil fertility: Soil physical and chemical properties. Arch. Agron. Soil Sci. 63, 477–490. doi:10.1080/03650340.2016.1218477
Ma, C. M., Tang, Y. Z., and Ji, S. N. (2004). Long term experimental study on crop positioning rotation system (II) - effect of different rotation methods on soil microbial biomass in soybean fields. J. Northeast Agric. Univ. 35, 645–650. doi:10.19720/j.cnki.issn.1005-9369.2004.06.001
Ma, T. E., Wei, Y. C., Yang, X. L., Wei, X. R., Wang, Y. H., Hao, M. D., et al. (2016). Mineralization characteristics of soil organic carbon under long-term fertilization management. Chin. J. Eco-Agriculture 24, 8–16. doi:10.13930/j.cnki.cjea.150740
Morales-Rodriguez, C., Anslan, S., Auger-Rozenberg, M.-A., Augustin, S., Baranchikov, Y., Bellahirech, A., et al. (2019). Forewarned is forearmed: Harmonized approaches for early detection of potentially invasive pests and pathogens in sentinel plantings. Neobiota 47, 95–123. doi:10.3897/neobiota.47.34276
Nanda, S. K., Das, P. K., and Behera, B. (1998). Effects of continuous manuring on microbial population, ammonification and CO2 evolution in a rice soil. Oryza 25, 413–416.
Nordby, H. E., Nemec, S., and Nagy, S. (1981). Fatty acids and sterols associated with citrus root mycorrhizae. J. Agric. Food Chem. 29, 396–401. doi:10.1021/jf00104a043
Olsson, P. A. (1999). Signature fatty acids provide tools for determination of the distribution and interactions of mycorrhizal fungi in soil. FEMS Microbiol. Ecol. 29, 303–310. doi:10.1111/j.1574-6941.1999.tb00621.x
Pradeep, K. S., Meenakshi, S., and Deepak, V. (2010). Biocontrol of fusarium wilt of chickpea using arbuscular mycorrhizal fungi and rhizobium leguminosorum biovar. Caryologia 63, 349–353. doi:10.1080/00087114.2010.10589745
Raich, J. W., and Tufekciogul, A. (2000). Vegetation and soil respiration: Correlations and controls. Biogeochemistry 48, 71–90. doi:10.1023/a:1006112000616
Rashid, M. I., Mujawar, L. H., Shahzad, T., Almeelbi, T., Ismail, I. M. I., and Oves, M. (2016). Bacteria and fungi can contribute to nutrients bioavailability and aggregate formation in degraded soils. Microbiol. Res. 183, 26–41. doi:10.1016/j.micres.2015.11.007
Sun, C. L., Xue, S., Liu, G. B., and Ding, S. N. (2014). Effects of long-term fertilization on soil particles and microaggregate distribution in the loess area. J. Plant Nutr. Fertilizers 20, 550–561. doi:10.11674/zwyf.2014.0305
Tamilselvi, S. M., Chinnadurai, C., Ilamurugu, K., Arulmozhiselvan, K., and Balachandar, D. (2015). Effect of long-term nutrient managements on biological and biochemical properties of semi-arid tropical Alfisol during maize crop development stages. Ecol. Indic. 48, 76–87. doi:10.1016/j.ecolind.2014.08.001
Tang, Q., and Zhang, C. (2013). Data Processing System (DPS) software with experimental design, statistical analysis and data mining developed for use in entomological research. Insect Sci. 20, 254–260. doi:10.1111/j.1744-7917.2012.01519.x
Tiziano, G., David, P., and Maurizio, G. P. (2011). Environmental impact of different agricultural management practices: Conventional vs. organic agriculture. Crit. Rev. Plant Sci. 30, 95–124. doi:10.1080/07352689.2011.554355
Tunlid, A., Hoitink, H. A., Low, C., and White, D. C. (1989). Characterization of bacteria that suppress rhizoctonia damping-off in bark compost media by analysis of fatty acid biomarkers. Appl. Environ. Microb. 55, 1368–1374. doi:10.1128/aem.55.6.1368-1374.1989
Wang, C. H., Zhu, F., Zhao, X., and Dong, K. H. (2014). The effects of N and P additions on microbial N transformations and biomass on saline-alkaline grassland of Loess Plateau of Northern China. Geoderma 213, 419–425. doi:10.1016/j.geoderma.2013.08.003
Wang, C. T., Long, R. J., Wang, Q. L., Liu, W., Jing, Z. C., and Zhang, L. (2010). Fertilization and litter effects on the functional group biomass, species diversity of plants, microbial biomass, and enzyme activity of two alpine meadow communities. Plant Soil 331, 377–389. doi:10.1007/s11104-009-0259-8
Wang, J. W., Zhang, G. Y., and Yu, C. Q. (2020). A Meta-analysis of the effects of organic and inorganic fertilizers on the soil microbial community. J. Resour. Ecol. 11, 298–303.
Wang, S. C., Zhou, J. B., Chen, Z. J., and Man, J. (2015a). Effects of temperature on soil nitrogen mineralization in solar greenhouses with different cultivation years. J. Plant Nutr. Fertilizers 21, 121–127. (in Chinese with English abstract).
Wang, W., Lai, D. Y. F., Wang, C., Pan, T., and Zeng, C. (2015b). Effects of rice straw incorporation on active soil organic carbon pools in a subtropical paddy field. Soil till. Res. 152, 8–16. doi:10.1016/j.still.2015.03.011
Wang, Y., Li, C., Tu, C., Hoyt, G. D., Deforest, J. L., and Hu, S. (2017). Long-term no-tillage and organic input management enhanced the diversity and stability of soil microbial community. Sci. Total Environ. 609, 341–347. doi:10.1016/j.scitotenv.2017.07.053
Wang, Y., Tu, C., Cheng, L., Li, C., Gentry, L. F., Hoyt, G. D., et al. (2011). Long-term impact of farming practices on soil organic carbon and nitrogen pools and microbial biomass and activity. Soil till. Res. 117, 8–16. doi:10.1016/j.still.2011.08.002
Wu, E., Sun, P. L., Sa, Q. R. G., Cao, C. L., Tian, X. Y., and He, Q. (2021). Effects of different rotation patterns on the physical and chemical properties and enzyme activities of Black soil in Northeast China. China Agric. Technol. Ext. 37, 70–75+80. doi:10.3969/j.issn.1002-381X.2021.06.027
Wu, R., Liu, S. J., Sun, H., Li, Y. X., Ma, L., and Bai, Y. (2020). Effects of long-term fertilization on soil fertility and microbial characteristics. Soil Fertilizer Sci. China 37, 12–16. (in Chinese with English abstract). doi:10.3760/cma.j.issn.1003-9406.2020.01.004
Yang, T., Adams, J. M., Shi, Y., He, J. S., Jing, X., Chen, L., et al. (2017). Soil fungal diversity in natural grasslands of the Tibetan plateau: Associations with plant diversity and productivity. New Phytol. 215, 756–765. doi:10.1111/nph.14606
Yao, Q., Xu, Y. L., Song, J., and Wei, W. (2015). Characteristics of phospholipid fatty acids of soil microorganism under different plant patterns of soybean. Soybean Sci. 34, 442–448. doi:10.11861/j.issn.1000-9841.2015.03.0442
Yu, C., Li, Y., Mo, R., Deng, W., Zhu, Z., Liu, D., et al. (2020). Effects of long-term straw retention on soil microorganisms under a rice-wheat cropping system. Arch. Microbiol. 202, 1915–1927. doi:10.1007/s00203-020-01899-8
Zelles, L. (1997). Phospholipid fatty acid profiles in selected members of soil microbial communities. Chemosphere 35, 275–294. doi:10.1016/s0045-6535(97)00155-0
Zeng, L. S., Liao, M., Chen, C. L., and Huang, C. Y. (2007). Effects of lead contamination on soil enzymatic activities, microbial biomass, and rice physiological indices in soil-lead-rice (Oryza sativa L) system. Ecotox. Environ. Safe. 67, 67–74. doi:10.1016/j.ecoenv.2006.05.001
Zhang, J. Y., Song, Y., and Wang, J. (2022a). Spatiotemporal patterns of gross ecosystem product across China's cropland ecosystems over the past two decades. Front. Ecol. Evol. 10-2022, 959329. doi:10.3389/fevo.2022.959329
Zhang, Q., Wang, C., Sun, Z. S., Li, S. Y., and Liang, Y. J. (2022b). Research progress on influencing factors of soil microbial biomass and diversity. North. Hortic. 503, 116–121. doi:10.11937/bfyy.20213763
Zhang, X., Wang, H., Hui, X. L., Wang, Z. H., and Liu, J. S. (2019). Effects of different fertilization and fallowing practices on soil carbon and nitrogen mineralization in a dryland soil with low organic matter. J. Soil Sci. Plant Nut. 19, 108–116. doi:10.1007/s42729-019-0016-x
Zhang, Y., Sheng, Z. E., Wang, Y. N., Su, S. M., Bai, L. Y., Wu, C. X., et al. (2021). Long-term manure application enhances the stability of aggregates and aggregate-associated carbon by regulating soil physicochemical characteristics. Catena 203, 105342. doi:10.1016/j.catena.2021.105342
Zhang, Z. S., Cao, C. G., Cai, M. L., and Li, C. F. (2013). Crop yield, P uptake and soil organic phosphorus fractions in response to short-term tillage and fertilization under a rape-rice rotation in central China. J. Soil Sci. Plant Nut. 13, 0–882. doi:10.4067/s0718-95162013005000069
Zhao, X., Wang, S. Q., and Xing, G. X. (2014). Nitrification, acidification, and nitrogen leaching from subtropical cropland soils as affected by rice straw-based biochar: Laboratory incubation and column leaching studies. J. Soil. Sediment. 14, 471–482. doi:10.1007/s11368-013-0803-2
Keywords: rainfed farmland, soil respiration, nitrogen mineralization, fertilization, cropping pattern, soil microbe
Citation: Wang Y, Li Q and Li C (2023) Organic fertilizer has a greater effect on soil microbial community structure and carbon and nitrogen mineralization than planting pattern in rainfed farmland of the Loess Plateau. Front. Environ. Sci. 11:1232527. doi: 10.3389/fenvs.2023.1232527
Received: 31 May 2023; Accepted: 10 July 2023;
Published: 20 July 2023.
Edited by:
Jianping Li, Ningxia University, ChinaReviewed by:
Junzhuo Liu, Chinese Academy of Sciences (CAS), ChinaYangquanwei Zhong, Northwestern Polytechnical University, China
Copyright © 2023 Wang, Li and Li. This is an open-access article distributed under the terms of the Creative Commons Attribution License (CC BY). The use, distribution or reproduction in other forums is permitted, provided the original author(s) and the copyright owner(s) are credited and that the original publication in this journal is cited, in accordance with accepted academic practice. No use, distribution or reproduction is permitted which does not comply with these terms.
*Correspondence: Chunyue Li, chunyue_li@snnu.edu.cn
†These authors have contributed equally to this work and share first authorship