- 1Escuela de Ciencias Químicas, Pontificia Universidad Católica del Ecuador, Quito, Ecuador
- 2Escuela de Gestión Ambiental, Pontificia Universidad Católica del Ecuador, Esmeraldas, Ecuador
- 3Yemanyá–Agua y Conservación, Esmeraldas, Ecuador
- 4Departamento de Recursos Hídricos y Ciencias Ambientales, Universidad de Cuenca, Cuenca, Ecuador
- 5Grupo de Ingeniería de las Reacciones Químicas, Catálisis y Tecnologías del Medio Ambiente, Departamento de Biociencias, Facultad de Ciencias Químicas, Universidad de Cuenca, Cuenca, Ecuador
- 6Laboratorio Nacional de Referencia del Agua, Universidad Regional Amazónica Ikiam, Tena, Ecuador
- 7Laboratorio de Productos Naturales, Universidad Regional Amazónica Ikiam, Tena, Ecuador
- 8Biomolecules Discovery Group, Universidad Regional Amazónica Ikiam, Tena, Ecuador
Introduction: Emerging contaminants (ECs) are substances with widely diverse chemical structures that may pose a risk to the environment and human beings. The limited scope of water treatment facilities, particularly in low and middle-income countries, allows ECs to be continuously introduced to the environment and become part of the cycle again for potabilization. In this work, we study for the first time the presence of these compounds in the drinking water of five Ecuadorian cities.
Methods: The compounds of interest were mainly pharmaceutical substances commonly present in prescription and over-the-counter medicines, along with caffeine, a known coadjuvant in some of these preparations. Samples were collected from Quito, Guayaquil, Cuenca, Ibarra, and Esmeraldas, considering each city’s distribution systems, and, after solid-phase extraction, analyzed by LC-MS/MS ESI+.
Results and discussion: Results showed a high occurrence of caffeine, the only analyte present in all cities, with concentrations ranging from <6.35 to 201 ngL-1 and an occurrence from 11% in Quito to 77% in Cuenca. The highest median was found in Cuenca, followed by Esmeraldas. Our observations regarding concentrations are comparable to other studies around the globe. Although in other cities, some pharmaceuticals appeared at levels below our detection limits. These findings highlight the ubiquitous nature of emerging contaminants while pointing out the need for regulatory frameworks that facilitate the implementation of treatment technologies at the source and wastewater level. These actions will safeguard public and environmental health in the long term.
1 Introduction
Emerging contaminants (ECs) are naturally occurring or synthetic compounds that are not commonly monitored in the environment and pose potential risks to human and environmental health (Naidu et al., 2016). There are several types of ECs, including pharmaceutical products (e.g., antibiotics, painkillers, anti-inflammatories) and associated substances. Medicaments reach drinking water systems through the introduction of wastewater effluents into rivers, which serve as sources for water treatment facilities. Current technologies employed in these plants are not effective in removing pharmaceutical compounds before distribution (Furlong et al., 2017).
Over the past 2 decades, emerging compounds have garnered significant attention from the scientific community due to their impact on human health and ecosystems (Ramírez-Malule et al., 2020). While some compounds may undergo changes when exposed to the environment, their continuous introduction offsets such effects. Studies have also demonstrated the influence of socioeconomic factors in the occurrence of ECs, such as their higher concentrations in regions with higher values of gross domestic product per capita and human development index (Santos et al., 2020). Furthermore, the constant consumption of pharmaceuticals can lead to bacterial resistance (Tell et al., 2019) and their physicochemical properties, such as high water solubility and low biodegradability (Gil, Soto, Usma and Gutiérrez, 2013), can result in bioaccumulation increasing the risk associated with these substances (Du et al., 2014; Muir et al., 2017). Additionally, there is evidence of environmental risks, particularly to marine fauna. Li and Lin (2015) found that a 2-h exposure to a mix of pharmaceutical compounds including sulfamethoxazole, caffeine, diclofenac, and acetaminophen, led to increased mortality and abnormal behaviors in fish. The concentrations used in the study (3.9 mgL−1 each, previously exposed to sunlight) were similar to those found in local hospital raw wastewater.
Most of the investigations on ECs have been conducted in North America and Europe, where monitoring of pharmaceuticals, perfluorinated chemicals, estrogenic hormones, detergents, microplastics, and inorganic elements in surface and drinking waters has been carried out (Furlong et al., 2017; Sousa et al., 2018). The United States, China, Spain, Italy, and Canada have emerged as the top five nations with the most published works in this field (Ramírez-Malule et al., 2020). However, limited research has focused on the presence of ECs in drinking water in Latin America, with findings reported only in Brazil, Colombia, Venezuela, and Chile, representing less than one-third of the countries in the region (Peña-Guzmán et al., 2019). Besides the limited information, the lack of regulation and monitoring, along with the poor water treatment and purification processes result in an increased presence of these compounds in all bodies of water (Vargas-Berrones et al., 2020). Pinos-Velez et al. (2019) made a comprehensive review of ECs occurrence in Latin America and reported that caffeine was the most prevalent and concentrated compound, although triclosan, cocaine, non-ylphenol, bisphenol A, atrazine, ibuprofen, among others, were also detected in estuaries and drinking water.
In Ecuador, less than 25% of wastewater receives treatment before being discharged into rivers and seas, leading cities like Guayaquil and Esmeraldas to receive industrial and domestic residual effluents in their drinking water sources (Pinos Velez et al., 2019). Studies have found caffeine, sulfamethoxazole, venlafaxine, o-desmethylvenlafaxine, and some steroidal estrogens throughout the San Pedro, Guayllabamba, and Esmeraldas River basins (Voloshenko-Rossin et al., 2015), as well as caffeine, paracetamol, and trimethoprim in the north of the Ecuadorian Amazon (Capparelli et al., 2021). Since ECs have already been detected in water bodies in Ecuador, and considering that most municipalities directly discharge wastewater into various river basins, it becomes imperative to determine whether these compounds are reaching the drinking water systems of urban areas. We hypothesize that they are and that this information may shed light on the environmental impact of ECs on the national ecosystem. Therefore, our research aims to answer the following questions: Are there emerging compounds present in the drinking water of five representative cities in Ecuador, namely: Quito, Guayaquil, Cuenca, Esmeraldas, and Ibarra? At what concentration are they found?
2 Materials and methods
2.1 Study area
As detailed in Table 1, the three most populated cities of Ecuador were selected for this study, namely: Quito, Guayaquil, and Cuenca. We also included two smaller cities that are within the top 10% in terms of population. Esmeraldas, a small coastal city, has previous reports of emerging contaminants in the namesake river (Voloshenko-Rossin et al., 2015) which is also the source for water potabilization and in other water bodies that surround the city (Cipriani-Ávila et al., 2023). Besides, the province of Esmeraldas, where the city is located, has low indexes of potabilization and wastewater treatment. In contrast, Ibarra is the capital city of Imbabura, one of the provinces with most potabilization and wastewater treatment facilities, as well as one with the highest compliance with the technical norm NTE 1 108:2011, which establishes drinking water requirements (INEC, 2016).
The sampling points in each city, detailed in Figure 1, were chosen based on their respective drinking water production systems, their distribution networks and their main parishes. In Quito, for each of the water distribution subsystems (Bellavista, Puengasí, El Troje, Northwest Treatment Plant) three neighborhoods were randomly selected, within each neighborhood three sampling points were picked out. In the case of Guayaquil, the city was divided into northern, central, and southern sectors. Samples were taken from five neighborhoods in each sector; Alborada and Urdesa neighborhoods were considered twice, as they cover two sectors of the city, Cuenca’s drinking water system is divided into three main subsystems named after the rivers from which the water is drawn: Tomebamba, Machángara, and Yanuncay. For each of these, sampling points were selected in their distribution zones in Cuenca city.
In Esmeraldas 2-3 random sampling points were selected per area, namely: south, center, high neighborhoods, low neighborhoods, Las Piedras and Tachina; these areas were established according to the water distribution system and the regularity of supply. In Ibarra, we worked in the five urban parishes (El Sagrario, San Francisco, Alpachaca, Priorato and Caranqui), where three sampling points were chosen. At each sampling point, one water sample was taken, adding to a total of: 45 samples in Quito, 42 in Guayaquil, 15 in Cuenca, 15 in Ibarra and 16 in Esmeraldas.
2.2 Sampling and sample preparation
Sampling was carried out with the methodology described by the International Standardization Organization NTC-ISO 5667–1:2008. Five hundred milliliters of each sample were placed in an amber glass bottle with screw cap and subsequently stored at 4°C until processed at the lab. The time between sampling and processing was not longer than 24 h in all cases.
Samples underwent the methodology by Glassmeyer et al. (2017). Analytes were separated from the water matrix by solid-phase extraction (SPE) using a vacuum pump (Millipore, WP6111560), a manifold (27 × 17 × 9.5 cm), and OASIS HLB cartridges (Waters, 200 mg, 6 ml). To condition the cartridges, they were treated with 6 ml of reagent water after 4 ml of methanol at a flow rate of 10 ml/min. After eluting 500 ml of sample, the cartridges were dried under vacuum for 10 min before analytes were eluted into glass tubes using 6 ml of methanol. Using nitrogen, extracts were concentrated to dryness and reconstituted to 0.5 ml with methanol, filtered through a 0.22 μm filter, and then placed into vials for chromatographic analysis. As part of measures to assure the quality of the results, a field blank was collected and analyzed to assess that sampling and extraction procedures were performed correctly. In addition, a spiked sample of the mean concentration level was used to gauge the recovery rates of each component (Table 2). All analytical standards had a purity >96.8% and were obtained from Supelco or Sigma-Aldrich.
2.3 Instrumental analysis
Five of the most consumed pharmaceutical compounds in Ecuador were selected for this study, namely: caffeine (stimulant), acetaminophen (analgesic), sodium diclofenac (anti-inflammatory), sulfamethoxazole (antibiotic), and trimethoprim (antibiotic). The information was obtained by request of the authors in May 2019 from the National Undersecretariat of Public Health Governance (Subsecretaría Nacional de Gobernanza de la Salud Pública del Ecuador, 2019).
These compounds were analyzed by UPLC-Qtof (Waters Model I-class liquid chromatograph coupled to a Waters Xevo G2 QTOF) under the conditions described in Cipriani Avila et al. (2023). Briefly, acetonitrile/formic acid in a 99.9%/0.1% ratio (solvent B) and 0.1% formic acid in water (solvent A) were the solvents utilized as the mobile phase; the elution gradient was: 5% B over 1 min, 5%–100% B over 9 min, 100%–5% B over 2 min, and then a column re-equilibration at 5% B during 3 min. A C18 column (Waters Acquity BEH 1.7 μm, 100 mm 2.1 mm i. d.) operating at 25°C was used with a flow rate of 0.3 ml min−1. For the electrospray ionization (ESI-MS), a positive mode with a capillary voltage of 0.5 kV, 30 L h−1 cone gas flow, 900 L h−1 desolvation gas flow, 120°C source temperature, 450°C desolvation temperature with sampling cone and source compensation at 40 and 80 V, were employed. Further fractionation (MS/MS) was conducted with ramp collision energy 20–30 eV. The range of m/z was 50–1,000 Da. Because the acquisition method provides complete information, the analytes were quantified using the precursor or product ion with the highest intensity. The method’s performance was determined according to Eurolab España and Morillas (2016).
2.4 Statistical analyses
For the statistical analysis, the software R version 4.2.1 (RRID:SCR_001905) was used with the R-Studio interface. Non-parametric Kruskal–Wallis statistics were performed to determine significant differences in caffeine content in water by city at a significance level of 0.05. Once statistically significant differences were found, the pairwise Wilcox test was applied.
3 Results
Table 2 indicates the main figures of merit of the analytical method employed for the emerging contaminants in this study. The highest sensitivity was obtained for sulfamethoxazole, although the recovery was lower compared to the other CEs. Trimethoprim showed the highest recovery among the five tested compounds but also the highest limits of detection and quantitation.
Figure 2 shows the results of the five studied compounds in each city. Caffeine was found in all cities with an overall concentration range of 1.4–201 ngL−1. It is also observed that acetaminophen was only found in site 20 (Bellavista) of Quito with 13.2 ngL−1 (occurrence 2.2%).
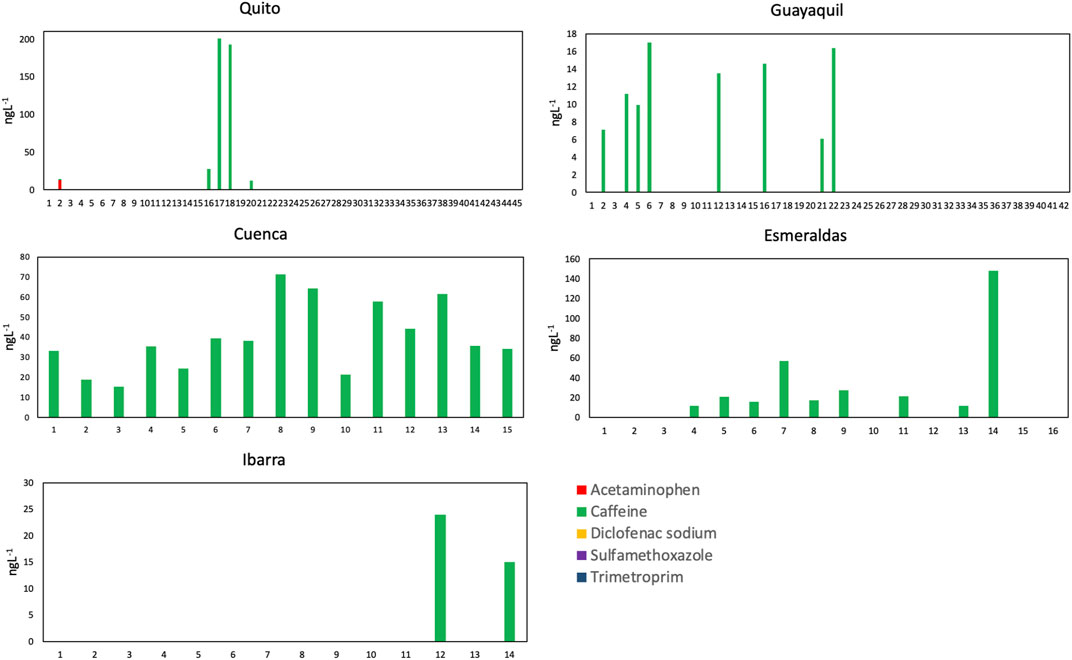
FIGURE 2. Concentration of acetaminophen, caffeine, sodium diclofenac, sulfamethoxazole, and trimethoprim by sampling site in Quito, Esmeraldas, Ibarra, Guayaquil and Cuenca.
In Table 3 it is observed that the occurrence of caffeine varies from 11% in Guayaquil to 100% in Cuenca. The highest and lowest concentrations were found in Quito with 201 and 1.4 ngL−1, respectively. In the cities with higher occurrence, Cuenca and Esmeraldas, where more than half of the samples contained caffeine, the variation in concentration ranged from 11.6 to 148 ngL−1. Sodium diclofenac, sulfamethoxazole, and trimethoprim were not found in any sample.
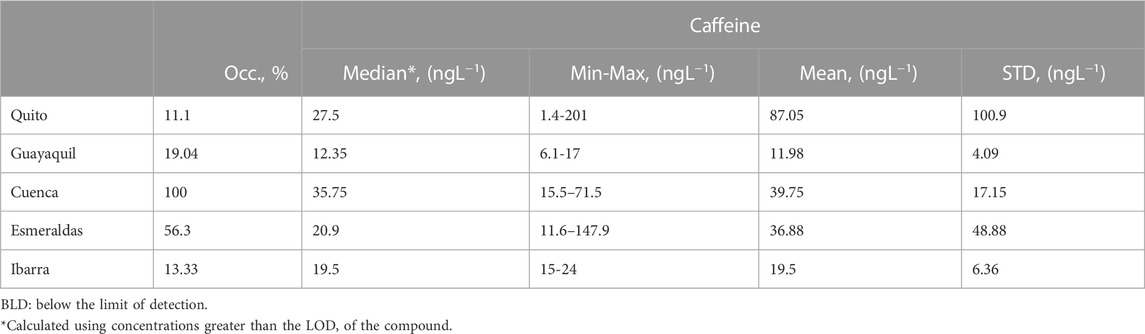
TABLE 3. Occurrence (%) and concentrations (ngL−1) of the caffeine in urban drinking water in Ecuador. Min: minimal quantifiable value, Max: maximal value.
In Figure 3 the notch boxplot of caffeine per city is presented. The highest median was found in Cuenca, approximately 36 ngL−1 with 95% of confidence. Esmeraldas, Guayaquil, Ibarra, and Quito show outliers. Kruskal–Wallis found statistically significant differences between medians (chi-squared = 62.3, p < 0.001). Through the pairwise Wilcox test for comparison of median, differences were found between Cuenca and all the other cities with Cuenca with the highest median. Also, differences were found between Esmeraldas with Guayaquil and Quito where Esmeraldas presented the highest median. Moreover, differences were found between Ibarra with Guayaquil and Quito where Ibarra has the highest median.
To contextualize the results of our research, in Table 4 we provide data from similar studies conducted in drinking water (after potabilization process) around the globe. In all of these cases, the methodology was similar to ours, although some minor differences in certain references are noted later in the discussion. The number of analyzed samples has a wide range, from 8 to 179; this study is the one that presents the higher value albeit comprising a broader geographical distribution. Regarding the analytes, it can be seen that caffeine and sulfamethoxazole are reported more frequently, followed by diclofenac and acetaminophen; except for one, the concentrations are in the same order of magnitude as ours but in some cases, the values are below the detection limits of our study.
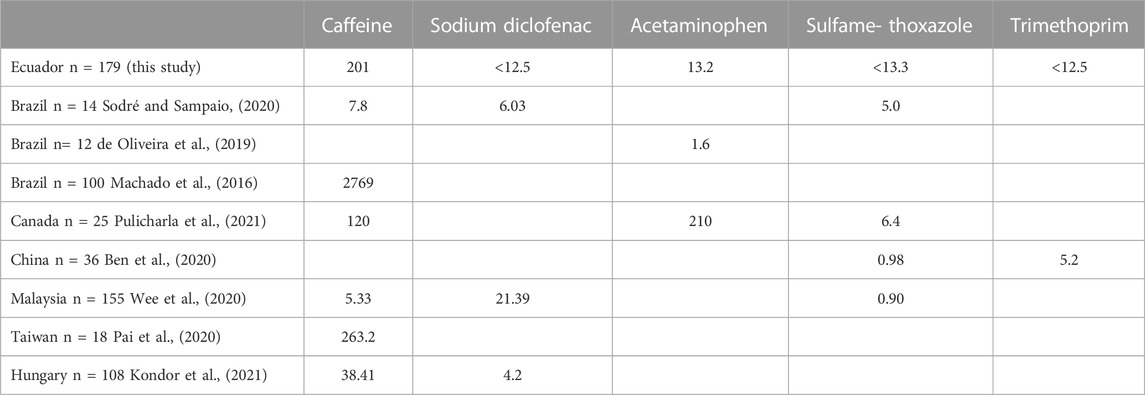
TABLE 4. Comparison of pharmaceutical compounds concentrations (ngL−1) in drinking waters around the world. Only the highest reported value is shown.
4 Discussion
This is the first study to show the concentration ranges of high-occurrence contaminants in the drinking water supplies of Ecuadorian cities: Quito, Guayaquil, Cuenca, Esmeraldas, and Ibarra. These compounds were determined using SPE followed by UPLC/ESI-MS; the method provided satisfactory analytical performance for the study of all five substances. The findings revealed the presence of two of the five compounds investigated, with caffeine having the highest occurrence, ranging from 11% of samples in Quito to 77% in Cuenca. Concentrations were comparable to those reported in other cities worldwide, hence adding to the evidence that there is an increasing need for regulation and management of these compounds in bodies of water.
According to Figure 2 and Table 3, caffeine was the emerging contaminant with the highest occurrence in drinking water samples, being found in 11% of the Quito samples, 19% in those of Guayaquil, 13% of Ibarra’s, 56% of Esmeraldas’, and 100% of Cuenca’s. However, as shown in Figure 3, despite the low occurrence, Quito had one of the highest variations in concentrations, as caffeine ranged from 1.4 ngL−1 to 201 ngL−1. When comparing with other countries of the continent, these values are intermediate between those reported for Canada and Brazil; in them, maximum values of 120 ngL−1 and 2769 ngL−1 were found (Machado et al., 2016; Pulicharla et al., 2021). Given the high frequency of caffeine-containing samples, there were significant differences between the results for Cuenca and the other four cities. It is not possible to pinpoint the causes for the increased caffeine concentration observed in Cuenca; however, we think that the tourism and cattle-raising activities near the catchment waters at the Cajas National Park might be a key influence. Lodging and restaurants in the Cajas National Park do not have a wastewater treatment plant or garbage disposal systems, which is why wastewater infiltrations and a clandestine garbage dump have been detected (Beltran, 2023). The drinking water in Esmeraldas showed a narrower distribution compared to Cuenca while presenting a higher overall concentration than Quito, Guayaquil, and Ibarra. This may be explained by the quality of the source water. Esmeraldas River receives the wastewater, treated and not, from the cities in the mountain region, being Quito the biggest one. As previously reported in the study by Voloshenko-Rossin et al. (2015), caffeine was among the emerging contaminants detected in this river, whose waters are the intake for the potabilization process in the city.
Caffeine is a commonly used psychoactive ingredient in free trade medicine and daily beverages, and although a large part of the intake is metabolized, it is accepted as an indicator of anthropogenic contributions to water bodies (Daneshvar et al., 2012; Li et al., 2020). The presence of this compound in wastewater in Ecuador has been reported by Capparelli et al. (2021) and Arcentales-Ríos et al. (2022); the latter group of researchers also demonstrate that only 50% of caffeine concentration is decreased with traditional technologies employed in Ecuador. In the best-case scenario, this treated water is released into the environment and eventually is used as a source for drinking water treatment. In other cases, untreated wastewater, rich in emerging contaminants, is directly discharged into these sources. Indeed, treated and untreated wastewater are the largest source of emerging pollutants in the environment since these are discharged to surface waters, distributing them in natural environments where they endanger flora and fauna (Pinos-Vélez et al., 2019). Currently, conventional primary and secondary treatments such as activated sludge, up-flow anaerobic sludge blanket reactor, stabilization ponds, wetlands, and trickling filters only contribute to a small portion of caffeine removal (Zhou et al., 2010; Martín et al., 2012). Organic matter removal mechanisms are unsuitable for compounds with more stable chemical structures like caffeine and other emerging pollutants (Arcentales-Ríos et al., 2022). For this reason, the incorporation of provisional or definitive ternary processes (like adsorption or advanced oxidation processes) into wastewater treatment plants is crucial to eliminate emerging contaminants (Morin-Crini et al., 2022).
Studies show that pharmaceuticals are also inefficiently removed in conventional potabilization treatments (Padhye et al., 2014; McKie et al., 2016), This is evidenced in the results of various studies, such as those presented in Table 4. Our results back up these findings, as caffeine was found in the drinking water of all the studied cities. Although the caffeine concentrations we found in drinking waters do not represent a risk to human health, they put in evidence the ineffectiveness of water treatment plants to eliminate emerging contaminants, both in wastewater and drinking water processing. Considering that with the current lifestyle, introduction rates, as well as EC diversity, tend to increase, it is important to take action to stop the introduction of these compounds into our water sources. For instance, alternative procedures have been proposed that allow the complete elimination of emerging compounds in drinking water, such as activated carbon processes, advanced oxidation processes, ozonation, among others (Kim et al., 2007; Yang et al., 2017; OECD, 2019).
The occurrence of acetaminophen was very low in Ecuadorian cities. Only one sample in Quito showed a quantifiable value for this compound with a concentration of 13.20 ngL−1. As detailed in Table 4, higher values have been reported by Pulicharla et al. (2021). The results found in our study may be due to the transformation of paracetamol during the chlorination of water in the treatment processes, where the main reaction products are hydroquinone and two types of chlorinated compounds: monochlorinated acetaminophen and dichlorinated acetaminophen (Cao et al., 2016). It is also possible that the concentrations in the analyzed samples were similar to those reported by De Oliveira et al. (2019), thus below the detection limit of our analytical method. As for the health impact of acetaminophen, the Minnesota Department of Health recommends a maximum value in drinking water of 200 ppb, equivalent to 200 µgL−1, pointing out that the liver might be the organ most prone to damage by chronic exposure (Minnesota Health Department, 2014). Our results are more than an order of magnitude below this threshold.
Although sodium diclofenac, sulfamethoxazole, and trimethoprim were not determined in the drinking water samples from the Ecuadorian cities, some previous studies show their presence in this matrix in other territories. Kondor et al. (2021) indicated a maximum concentration of 4.2 ng sodium diclofenac per liter of drinking water in Hungary. Sulfamethoxazole was frequently found in the samples of a study carried out in the United States where 51 emerging compounds were analyzed (Benotti et al., 2009), as well as in the drinking water of cities in Brazil and Spain with values less than 10 ngL−1 (Gros et al., 2012; Monteiro et al., 2017). As with acetaminophen, this compound may have been chemically transformed during potabilization, due to the high reactivity of sulfonamides with chlorine or chlorine dioxide (Huber et al., 2005; Gaffney et al., 2016). The absence of trimethoprim in the samples can be explained by a lack of sensitivity of the selected analytical methodology, as detailed below.
The publications by Machado et al. (2016) and Gros et al. (2012) show results of LC-MS/MS methods for several emerging compounds, obtaining method LODs of 1, 0.3, 0.8, 0.1, and 0.1 ngL−1 for caffeine, sodium diclofenac, acetaminophen, sulfamethoxazole, and trimethoprim, respectively. Wee et al. (2020) also report lower method detection limits. In contrast, as detailed in Table 2, the LOD values obtained for this research were higher for all analytes. These differences can be attributed to method development. For instance, we focused on sensitivity rather than resolution for spectral scanning optimization. On the other hand, our analyses were conducted in positive ionization mode, whereas, Wee et al. (2020) and Gros et al. (2012) use ionization in both positive and negative modes, probably because certain compounds exhibit higher ionization in the latter, thereby yielding greater analysis sensitivity and, consequently, lower detection limits. Furthermore, Gros et al. (2012) applied ESI- along with acetonitrile/ammonium acetate buffer as mobile phase for trimethoprim analysis. Considering that in a study carried out in China a maximum concentration of 5.2 ngL−1 of trimethoprim was found in drinking water (Ben et al., 2020), it could be inferred that our method did not present adequate sensitivity for the determination of this compound.
Internationally, European countries are leading legislative actions concerning emerging contaminants. The European Commission has established a watch list of substances that may pose a risk to the aquatic environment but whose current information is limited to conclude its significance. Among the chemical compounds listed are two of those included in this study: sulfamethoxazole and trimethoprim (Implementing Decision (EU), 2022/1,307). Diclofenac was previously listed, in 2015 (OECD, 2019), and is currently regulated in Switzerland, with a maximum concentration of 50 ngL−1 in surface waters (Swiss Federal Law, 2023).
In Ecuador, our results point towards several steps that must be taken to ensure water quality. One of the most important is the increase of wastewater treatment plants and the provision of various technologies to effectively remove or reduce polluting substances, including emerging contaminants. Wingfield et al. (2021) point out the importance of sewage treatment in ensuring water access in Ecuador, while also advocating for increased legislative support for ecosystem preservation. Along the same lines, Martínez-Moscoso et al. (2018) provide an overview of the current water laws landscape in Ecuador, emphasizing that water provision, particularly drinking water, shall be a public service. However, their study demonstrates that in practice, to comply with this precept, local governments pass on operating costs to final users, resulting in an increased cost to the population in rural areas. If wastewater treatment plants are installed in these municipalities, the cost of water services will rise, and if more advanced treatments are installed, the cost will rise even further. Hence, financial support from other sources is an important aspect to consider along with the cost of advanced treatment technologies. The Organization for Economic Co-operation and Development (2019) reports that from the currently available methods for pharmaceuticals removal from water, reverse osmosis and ozone have similar efficiency, but the latter has half of the cost per cubic meter.
The results from 179 samples collected in five Ecuadorian cities indicate that caffeine is one of the most prevalent emerging compounds in drinking water. We have pointed out the growing need to monitor ECs in various bodies of water to anticipate and effectively mitigate the environmental risks that derive from them. Protection measures for drinking water sources should include strict regulations for houses, hotels, restaurants, and entertainment venues nearby. Of paramount importance are the implementation of adequate garbage disposal and wastewater treatment plants to avoid infiltration of liquids that endanger the quality of the source water. An update of the water treatment systems is also necessary, especially in the coastal cities that take river water as a source of water to be made drinkable. Future research should also consider extended monitoring campaigns and including drug metabolites in the analysis, which may appear due to reactivity in treatment plants, metabolism in the human body, or environmental degradation. These details will be critical for taking informed legislative action at the national level.
Data availability statement
The datasets presented in this study can be found in online repositories. The names of the repository/repositories and accession number(s) can be found below: https://osf.io/2mg87/?view_only=ea727d3ae65a40b0b81bad3cc1ee8327
Author contributions
EJ-N: methodology, investigation, formal analysis, writing–original draft, writing–review and editing. IC-A: conceptualization, methodology, investigation, formal analysis, funding acquisition, supervision, writing–original draft, writing–review and editing. JM: methodology, investigation, formal analysis, validation, supervision, writing–original draft, writing–review and editing. VP-V: methodology, formal analysis, funding acquisition, writing–original draft, writing–review and editing. SA-L: methodology, investigation, supervision, writing–original draft, writing–review and editing. MC: methodology, validation, writing–review and editing. EM-V: methodology, formal analysis, writing–review and editing. DL-A: methodology, investigation, writing–original draft. AP: investigation, writing–original draft. OM: investigation, writing–original draft. NM: funding acquisition, supervision, writing–original draft, writing–review and editing. All authors contributed to the article and approved the submitted version.
Funding
Corporación Ecuatoriana para el Desarrollo de la Investigación y Academia-CEDIA: Project CEPRA-XIV-2020-09 "Determinación del impacto y ocurrencia de Contaminantes Emergentes en ríos de la Costa Ecuatoriana y propuestas de tratamiento para su remoción".Pontificia Universidad Católica del Ecuador: Project QINV0151-IINV529020200.
Acknowledgments
The authors thank the Corporación Ecuatoriana para el Desarrollo de la Investigación y Academia—CEDIA for their contribution in innovation, through the CEPRA projects. The Pontificia Universidad Católica del Ecuador Sede Esmeraldas granted access to the Laboratory of Environmental Management during the field campaign.
Conflict of interest
The authors declare that the research was conducted in the absence of any commercial or financial relationships that could be construed as a potential conflict of interest.
Publisher’s note
All claims expressed in this article are solely those of the authors and do not necessarily represent those of their affiliated organizations, or those of the publisher, the editors and the reviewers. Any product that may be evaluated in this article, or claim that may be made by its manufacturer, is not guaranteed or endorsed by the publisher.
References
Arcentales-Ríos, R., Carrión-Méndez, A., Cipriani-Ávila, I., Acosta, S., Capparelli, M., Moulatlet, G., et al. (2022). Assessment of metals, emerging contaminants, and physicochemical characteristics in the drinking water and wastewater of Cuenca, Ecuador. J. Trace Elem. Minerals 2, 100030. doi:10.1016/j.jtemin.2022.100030
Beltran, J. (2023). Un botadero clandestino crecía en el Parque Nacional Cajas. Available at: https://www.primicias.ec/noticias/sociedad/botadero-clandestino-parque-nacional-cajas/.
Ben, Y., Hu, M., Zhang, X., Wu, S., Wong, M. H., Wang, M., et al. (2020). Efficient detection and assessment of human exposure to trace antibiotic residues in drinking water. Water Res. 175, 115699. doi:10.1016/j.watres.2020.115699
Benotti, M. J., Trenholm, R. A., Vanderford, B. J., Holady, J. C., Stanford, B. D., and Snyder, S. A. (2009). Pharmaceuticals and endocrine disrupting compounds in U.S. drinking water. Environ. Sci. Technol. 43 (3), 597–603. doi:10.1021/es801845a
Cao, F., Zhang, M., Yuan, S., Feng, J., Wang, Q., Wang, W., et al. (2016). Transformation of acetaminophen during water chlorination treatment: Kinetics and transformation products identification. Environ. Sci. Pollut. Res. 12, 23(12), 12303–12311. doi:10.1007/S11356-016-6341-X
Capparelli, M. V., Cipriani-Avila, I., Jara-Negrete, E., Acosta-López, S., Acosta, B., Pérez-González, A., et al. (2021). Emerging contaminants in the northeast andean foothills of amazonia: The case of study of the city of tena, napo, Ecuador. Bull. Environ. Contam. Toxicol. 107 (1), 2–10. doi:10.1007/s00128-021-03275-8
Cipriani-Avila, I., Molinero, J., Cabrera, M., Medina-Villamizar, E. J., Capparelli, M. V., Jara-Negrete, E., et al. (2023). Occurrence of emerging contaminants in surface water bodies of a coastal province in Ecuador and possible influence of tourism decline caused by COVID-19 lockdown. Sci. Total Environ. 866, 161340. doi:10.1016/j.scitotenv.2022.161340
Climate-Data.org (2023). Cuenca climate (Ecuador). Climate data. Available at: https://en.climate-data.org/south-america/ecuador/provincia-del-azuay/cuenca-875185/.
Daneshvar, A., Aboulfadl, K., Viglino, L., Broséus, R., Sauvé, S., Madoux-Humery, A. S., et al. (2012). Evaluating pharmaceuticals and caffeine as indicators of fecal contamination in drinking water sources of the Greater Montreal region. Chemosphere 88 (1), 131–139. doi:10.1016/J.CHEMOSPHERE.2012.03.016
De Oliveira, J. M. R., Izeppi, L. J. P., Loose, R. F., Muenchen, D. K., Prestes, O. D., and Zanella, R. (2019). A multiclass method for the determination of pharmaceuticals in drinking water by solid phase extraction and ultra-high performance liquid chromatography-tandem mass spectrometry. Anal. Methods 11 (17), 2333–2340. doi:10.1039/c9ay00289h
Du, B., Haddad, S. P., Luek, A., Scott, W. C., Saari, G. N., Kristofco, L. A., et al. (2014). Bioaccumulation and trophic dilution of human pharmaceuticals across trophic positions of an effluent-dependent wadeable stream. Philosophical Trans. R. Soc. B 369 (1656), 20140058. doi:10.1098/rstb.2014.0058
Empresa de Telecomunicaciones, Agua Potable, Alcantarillado y Saneamiento de Cuenca [ETAPA EP] (2023). Plantas de potabilización. Available at: https://www.etapa.net.ec/principal/agua-potable/operacion-y-mantenimiento/plantas-de-potabilizacion.
Empresa Pública Mancomunada de Agua Potable y Saneamiento de Esmeraldas [EPMAPSE] (2021). Informe de rendición de cuentas. Available at: https://epmapse.gob.ec/wp-content/uploads/2023/05/informe_rc_2021_final_aprobado-signed.pdf.
Empresa Pública Metropolitana de Agua Potable y Saneamiento Quito [EPMAPS] (2023). Captación. Available at: https://www.aguaquito.gob.ec/captacion/.
España, Eurolab, and Morillas, P. (2016). Guía eurachem: La adecuación al uso de los métodos analíticos – una guía de laboratorio para la validación de métodos y temas relacionados. Available at: https://www.eurachem.org/images/stories/Guides/pdf/MV_guide_2nd_ed_ES.pdf.
Furlong, E., Batt, A., Glassmeyer, S., Noriega, M., Kolpin, D., Mash, H., et al. (2017). Nationwide reconnaissance of contaminants of emerging concern in source and treated drinking waters of the United States: Pharmaceuticals. Sci. Total Environ. 579, 1629–1642. doi:10.1016/j.scitotenv.2016.03.128
Gaffney, V. de J., Cardoso, V. V., Benoliel, M. J., and Almeida, C. M. M. (2016). Chlorination and oxidation of sulfonamides by free chlorine: Identification and behaviour of reaction products by UPLC-MS/MS. J. Environ. Manag. 166, 466–477. doi:10.1016/J.JENVMAN.2015.10.048
Gil, M. J., Soto, A. M., Usma, J. I., and Gutiérrez, O. D. (2013). Contaminantes emergentes en aguas, efectos y posibles tratamientos. Producción+ limpia 7 (2).
Glassmeyer, S. T., Furlong, E. T., Kolpin, D. W., Batt, A. L., Benson, R., Boone, J. S., et al. (2017). Nationwide reconnaissance of contaminants of emerging concern in source and treated drinking waters of the United States. Sci. Total Environ. 581–582, 909–922. doi:10.1016/J.SCITOTENV.2016.12.004
Gros, M., Rodríguez-Mozaz, S., and Barceló, D. (2012). Fast and comprehensive multi-residue analysis of a broad range of human and veterinary pharmaceuticals and some of their metabolites in surface and treated waters by ultra-high-performance liquid chromatography coupled to quadrupole-linear ion trap tandem mass spectrometry. J. Chromatogr. A 1248, 104–121. doi:10.1016/J.CHROMA.2012.05.084
Huber, M. M., Korhonen, S., Ternes, T. A., and Von Gunten, U. (2005). Oxidation of pharmaceuticals during water treatment with chlorine dioxide. Water Res. 39 (15), 3607–3617. doi:10.1016/J.WATRES.2005.05.040
Implementing Decision (EU) (2022/1307). Watch list of substances for Union-wide monitoring in the field of water policy pursuant to Directive 2008/105/EC of the European Parliament and of the Council. Available at: http://data.europa.eu/eli/dec_impl/2022/1307/oj.
Instituto Nacional de Estadísticas y Censos [INEC] (2016). Estadística de Información Ambiental Económica en Gobiernos Autónomos Descentralizados Municipales 2015 (Agua y Alcantarillado). Available at: https://www.ecuadorencifras.gob.ec/documentos/web-inec/Encuestas_Ambientales/Municipios_2015/Documento_Tecnico-Gestion_de_Agua_y_Alcantarillado_2015.pdf.
Instituto Nacional de Meteorología e Hidrología [INAMHI] (2023). Series mensuales de datos metereológicos 2010-2023 - varias estaciones.
Interagua (2023). ¿Cómo se dotará de agua a Guayaquil en el 2041? Available at: https://www.interagua.com.ec/sites/default/files/6_interagua_pranc_neptuno_1.pdf.
Japanese International Cooperation Agency [JICA] (2005). Informe del estudio de diseño básico sobre el proyecto para el mejoramiento del sistema de agua potable para el Cantón Ibarra en la República del Ecuador. Available at: https://openjicareport.jica.go.jp/pdf/11809829_01.pdf.
Kim, S. D., Cho, J., Kim, I. S., Vanderford, B. J., and Snyder, S. A. (2007). Occurrence and removal of pharmaceuticals and endocrine disruptors in South Korean surface, drinking, and waste waters. Water Res. 41 (5), 1013–1021. doi:10.1016/j.watres.2006.06.034
Kondor, A. C., Molnár, É., Vancsik, A., Filep, T., Szeberényi, J., Szabó, L., et al. (2021). Occurrence and health risk assessment of pharmaceutically active compounds in riverbank filtrated drinking water. J. Water Process Eng. 41, 102039. doi:10.1016/J.JWPE.2021.102039
Li, S., and Lin, A. (2015). Increased acute toxicity to fish caused by pharmaceuticals in hospital effluents in a pharmaceutical mixture and after solar irradiation. Chemosphere 139, 190–196. doi:10.1016/j.chemosphere.2015.06.010
Li, S., Wen, J., He, B., Wang, J., Hu, X., and Liu, J. (2020). Occurrence of caffeine in the freshwater environment: Implications for ecopharmacovigilance. Environ. Pollut. 263, 114371. doi:10.1016/J.ENVPOL.2020.114371
Machado, K. C., Grassi, M. T., Vidal, C., Pescara, I. C., Jardim, W. F., Fernandes, A. N., et al. (2016). A preliminary nationwide survey of the presence of emerging contaminants in drinking and source waters in Brazil. Sci. Total Environ. 572, 138–146. doi:10.1016/J.SCITOTENV.2016.07.210
Martín, J. F., Camacho-Muñoz, D., Santos, J. E. P., Aparicio, I., and Alonso, E. (2012). Occurrence of pharmaceutical compounds in wastewater and sludge from wastewater treatment plants: Removal and ecotoxicological impact of wastewater discharges and sludge disposal. J. Hazard. Mater. 239 (240), 40–47. doi:10.1016/j.jhazmat.2012.04.068
Martínez-Moscoso, A., Feijó, V. G. A., and Silva, T. V. (2018). The vital minimum amount of drinking water required in Ecuador. Resources 7 (1), 15. doi:10.3390/resources7010015
McKie, M. J., Andrews, S. A., and Andrews, R. C. (2016). Conventional drinking water treatment and direct biofiltration for the removal of pharmaceuticals and artificial sweeteners: A pilot-scale approach. Sci. Total Environ. 544, 10–17. doi:10.1016/J.SCITOTENV.2015.11.145
Minnesota Health Department. (2014, ). Acetaminophen in drinking water. Human health-based water guidance table. Retrieved May 29, 2023, Available at: https://www.health.state.mn.us/communities/environment/risk/docs/guidance/dwec/acetamininfo.pdf
Monteiro, M., Spisso, B., Ferreira, R., Pereira, M., Grutes, J., de Andrade, B., et al. (2017). Development and validation of liquid chromatography-tandem mass spectrometry methods for determination of beta-lactams, macrolides, fluoroquinolones, sulfonamides and tetracyclines in surface and drinking water from Rio de Janeiro, Brazil. J. Braz. Chem. Soc. doi:10.21577/0103-5053.20170203
Morin-Crini, N., Sharma, V. K., Fourmentin, M., Ribeiro, A. C., Noutsopoulos, C., Mapelli, F., et al. (2022). Removal of emerging contaminants from wastewater using advanced treatments. A review. Environ. Chem. Lett. 20 (2), 1333–1375. doi:10.1007/s10311-021-01379-5
Muir, D. C. G., Simmons, D. B., Wang, X., Peart, T., Villella, M., Miller, J., et al. (2017). Bioaccumulation of pharmaceuticals and personal care product chemicals in fish exposed to wastewater effluent in an urban wetland. Sci. Rep. 7 (1), 16999. doi:10.1038/s41598-017-15462-x
Naidu, R., España, V. A. A., Liu, Y., and Jit, J. (2016). Emerging contaminants in the environment: Risk-based analysis for better management. Chemosphere 154, 350–357. doi:10.1016/j.chemosphere.2016.03.068
Organization for Economic Co-operation and Development [OECD] (2019). Pharmaceutical Residues in freshwater: Hazards and policy responses, OECD studies on water. Paris: OECD Publishing. doi:10.1787/c936f42d-en
Padhye, L. P., Yao, H., Kung’u, F. T., and Huang, C. H. (2014). Year-long evaluation on the occurrence and fate of pharmaceuticals, personal care products, and endocrine disrupting chemicals in an urban drinking water treatment plant. Water Res. 51, 266–276. doi:10.1016/J.WATRES.2013.10.070
Pai, C. W., Leong, D., Chen, C., and Wang, G. (2020). Occurrences of pharmaceuticals and personal care products in the drinking water of Taiwan and their removal in conventional water treatment processes. Chemosphere 256, 127002. doi:10.1016/j.chemosphere.2020.127002
Peña-Guzmán, C., Ulloa-Sánchez, S., Mora, K. S. C., Helena-Bustos, R., Barrera, E. a. L., Alvarez, J. M., et al. (2019). Emerging pollutants in the urban water cycle in Latin America: A review of the current literature. J. Environ. Manag. 237, 408–423. doi:10.1016/j.jenvman.2019.02.100
Pinos-Vélez, V. N., Esquivel-Hernández, G., Cipriani-Avila, I., Mora-Abril, E., Cisneros, J. C., Alvarado, A., et al. (2019). Emerging contaminants in trans-American waters. Revista Ambiente Água 14 (6), 1. doi:10.4136/ambi-agua.2436
Pulicharla, R., Proulx, F., Behmel, S., Sérodes, J., and Rodriguez, M. J. (2021). Occurrence and seasonality of raw and drinking water contaminants of emerging interest in five water facilities. Sci. Total Environ. 751, 141748. doi:10.1016/j.scitotenv.2020.141748
Ramírez-Malule, H., Quiñones-Murillo, D. H., and Manotas-Duque, D. (2020). Emerging contaminants as global environmental hazards. A bibliometric analysis. Emerg. Contam. 6, 179–193. doi:10.1016/j.emcon.2020.05.001
Santos, A., Couto, C. F., Lebron, Y. a. R., Moreira, V. R., Foureaux, A. F. S., Reis, E. G., et al. (2020). Occurrence and risk assessment of pharmaceutically active compounds in water supply systems in Brazil. Sci. Total Environ. 746, 141011. doi:10.1016/j.scitotenv.2020.141011
Sodré, F. F., and Sampaio, T. H. (2020). Development and application of a SPE-LC-QTOF method for the quantification of micropollutants of emerging concern in drinking waters from the Brazilian capital. Emerg. Contam. 6, 72–81. doi:10.1016/j.emcon.2020.01.001
Sousa, J. M. C., Ribeiro, A. C., Barbosa, M. O., Pereira, M. F. R., and Silva, A. M. (2018). A review on environmental monitoring of water organic pollutants identified by EU guidelines. J. Hazard. Mater. 344, 146–162. doi:10.1016/j.jhazmat.2017.09.058
Subsecretaría Nacional de Gobernanza de la Salud Pública del Ecuador (2019). Reporte de ventas de productos farmacéuticos 2008-2013.
Swiss Federal Law (2023). Swiss federal law 814.201: 2023. Waters protection ordinance (WPO). Available at: https://fedlex.data.admin.ch/eli/cc/1998/2863_2863_2863.
Tell, J., Caldwell, D. J., Häner, A., Hellstern, J., Hoeger, B., Journel, R., et al. (2019). Science-based targets for antibiotics in receiving waters from pharmaceutical manufacturing operations. Integr. Environ. Assess. Manag. 15 (3), 312–319. doi:10.1002/ieam.4141
Vargas-Berrones, K., Bernal-Jácome, L., Díaz de León-Martínez, L., and Flores-Ramírez, R. (2020). Emerging pollutants (EPs) in Latin américa: A critical review of under-studied EPs, case of study -nonylphenol-. Sci. Total Environ. 726, 138493. doi:10.1016/j.scitotenv.2020.138493
Voloshenko-Rossin, A., Gasser, G., Cohen, K., Gun, J., Cumbal-Flores, L., Parra-Morales, W., et al. (2015). Emerging pollutants in the Esmeraldas watershed in Ecuador: Discharge and attenuation of emerging organic pollutants along the san pedro–guayllabamba–esmeraldas rivers. Environ. Sci. Process. Impacts 17 (1), 41–53. doi:10.1039/C4EM00394B
Wee, S. Y., Haron, D. E. M., Aris, A. Z., Yusoff, F. M., and Praveena, S. M. (2020). Active pharmaceutical ingredients in Malaysian drinking water: Consumption, exposure, and human health risk. Environ. Geochem. Health 42 (10), 3247–3261. doi:10.1007/s10653-020-00565-8
Wingfield, S., Martínez-Moscoso, A., Quiroga, D., and Ochoa-Herrera, V. (2021). Challenges to water management in Ecuador: Legal authorization, quality parameters, and socio-political responses. Water 13 (8), 1017. doi:10.3390/w13081017
Yang, Y., Ok, Y. S., Kim, K. H., Kwon, E. E., and Tsang, Y. F. (2017). Occurrences and removal of pharmaceuticals and personal care products (PPCPs) in drinking water and water/sewage treatment plants: A review. Sci. Total Environ. 596, 303–320. –597. doi:10.1016/J.SCITOTENV.2017.04.102
Keywords: emerging contaminants, drinking water, Ecuador, pharmaceuticals, caffeine
Citation: Jara-Negrete E, Cipriani-Avila I, Molinero J, Pinos-Vélez V, Acosta-López S, Cabrera M, Medina-Villamizar EJ, Leiva-Andrade D, Pozo A, Martínez O and Mogollón NGS (2023) Pharmaceutical compounds in urban drinking waters of Ecuador. Front. Environ. Sci. 11:1232405. doi: 10.3389/fenvs.2023.1232405
Received: 31 May 2023; Accepted: 31 July 2023;
Published: 17 August 2023.
Edited by:
Rajendra Prasad Singh, Southeast University, ChinaReviewed by:
Tengyi Zhu, Yangzhou University, ChinaSunil Kumar, Academy of Scientific and Innovative Research (AcSIR), India
Yajun Wang, Lanzhou University of Technology, China
Amit Kumar, Nanjing University of Information Science and Technology, China
Copyright © 2023 Jara-Negrete, Cipriani-Avila, Molinero, Pinos-Vélez, Acosta-López, Cabrera, Medina-Villamizar, Leiva-Andrade, Pozo, Martínez and Mogollón. This is an open-access article distributed under the terms of the Creative Commons Attribution License (CC BY). The use, distribution or reproduction in other forums is permitted, provided the original author(s) and the copyright owner(s) are credited and that the original publication in this journal is cited, in accordance with accepted academic practice. No use, distribution or reproduction is permitted which does not comply with these terms.
*Correspondence: Isabel Cipriani-Avila, ecipriani111@puce.edu.ec