- 1Ecole Nationale d’Ingénieurs de Monastir, Université de Monastir, Monastir, Tunisia
- 2Ecole Supérieure des Sciences et de Technologie de Hammam Sousse, Université de Sousse, Sousse, Tunisia
In Tunisia, this sector will occupy the first place in terms of energy consumption in a few years. To remedy this, we must move towards renewable energy sources that partially or totally replace fossil fuels such as oil, coal, etc., and developing ways to make the building more energy efficient in order to save and reduce energy consumption while working to ensure better comfort for the occupant. This work deals with a numerical study based on the nodal method using software (DIGITAL Visual FORTRAN95) to predict the effect of integrating a PCM layer on the thermal behavior of a two-zone building. The effect of the location of the PCM in the wall on its thermal behavior is demonstrated, and the thermal performance of the PCM is demonstrated by considering energy indicators based on the indoor air temperature and the indoor and outdoor surface temperature of the building. It was concluded that the incorporation of PCM into the building envelope increased its thermal inertia, directly influencing the perception of thermal comfort. This indicates that the use of PCM can contribute to improved thermal performance and a more comfortable indoor environment.
1 Introduction
In recent years, there has been a growing interest in utilizing phase change materials (PCMs) in the construction industry, particularly in relation to building envelopes. PCMs offer a promising solution to enhance the thermal inertia of buildings. A study conducted by Al-Yasiri and Marta (2022) in Al-Amarah, Iraq, focused on the application of PCMs in a hot climate. The researchers constructed and tested two chambers: one incorporated PCM in its design, while the other did not. Several parameters were investigated during the construction of the PCM chamber, including the optimal position and thickness of the PCM layer in the roof, as well as the most thermally efficient PCM concrete capsules. These parameters were crucial in determining the overall thermal efficiency of the PCM room. The experimental findings demonstrated that the integration of PCM in the building envelope can effectively improve its thermal performance when exposed to high outdoor temperatures. By incorporating PCM, the building envelope exhibited enhanced thermal inertia, which helped to regulate indoor temperatures and reduce the dependence on mechanical cooling systems. Overall, this study highlights the significant potential of using PCM in building construction, particularly in hot climates like Al-Amarah, Iraq. By considering factors such as the appropriate placement and thickness of PCM layers in the roof and utilizing efficient PCM concrete capsules, the thermal performance of buildings can be greatly enhanced, leading to improved energy efficiency and comfort for occupants. Pappu et al. (2022) studied the effectiveness of incorporating Phase Change Materials (PCMs), insulation, natural ventilation, and night ventilation techniques to improve the thermal performance of buildings situated in different climatic regions. The study aims to assess the energy efficiency and indoor comfort achieved by utilizing this techniques. The research methodology involves a combination of theoretical analysis, computer simulations, and practical experiments. Different types of PCMs are considered and integrated into building components such as walls, ceilings, and floors. Various insulation materials are evaluated for their thermal resistance properties. Natural ventilation strategies are explored, including the design of openings, window configurations, and airflow patterns. Night ventilation techniques are examined, focusing on optimal timings, window operation, and thermal mass utilization. The study evaluates the performance of these techniques in representative climates, including hot and arid, hot and humid, cold and dry, and cold and wet regions. Key performance indicators such as indoor temperature, energy consumption, thermal comfort, and potential cost savings are analyzed and compared across different climatic conditions.
Adnan et al. (2021) focused on the use of phase change materials (PCM) to improve the energy efficiency of buildings. PCMs are materials capable of absorbing and releasing large amounts of heat when changing from a solid state to a liquid state and vice versa. This capability helps regulate the temperature inside buildings, reducing the need for conventional heating or air conditioning systems. The authors present a study on the development of improved buildings through the incorporation of PCM in their structure. They discuss the different properties of PCMs and their impact on the energy performance of buildings. Potential benefits of using PCMs include reduced energy demand, lower heating and cooling costs, and improved thermal comfort for occupants. The article also discusses the different types of PCMs available on the market, their potential applications in buildings, and the challenges associated with their implementation. The authors highlight the importance of proper thermal management system design and proper integration of PCMs into the building structure. In conclusion, the article highlights the potential of PCMs to reduce energy consumption in the buildings. It provides an overview of current research in this area and highlights the importance of an integrated approach to maximize the benefits of PCMs in building design. In their study, Luisa et al. (2007) developed and evaluated a novel type of concrete. Their objective was to investigate and compare the thermal characteristics of this innovative composite material in comparison to traditional concrete. The experimental measurements conducted by the researchers demonstrated that the new concrete exhibited the capability to decrease the magnitude of temperature fluctuations within its interior throughout the day. Castell et al. (2010) employed a similar methodology to assess the performance of macro-encapsulated Phase Change Material (PCM) incorporated into brick construction, specifically aimed at cooling during summer. The researchers conducted experiments in real outdoor weather conditions and observed that the utilization of PCM exhibited promising potential in mitigating fluctuations in indoor temperatures as well as reducing power consumption. Kissock and Limas (2006) conducted a simulation to analyze the transient heat transfer in a south-facing multi-layer wall in Dayton, Ohio. The wall consisted of an insulating layer positioned between two layers of paraffin-impregnated concrete, with a thickness of 30.4 cm. The paraffin content in the concrete was 10% by weight. The results of the simulation revealed a reduction of 19% in peak cooling loads and 13% in annual cooling loads. Additionally, peak heating loads decreased by 11%, while annual heating loads saw a slight reduction of 1%. Zwanzig et al. (2013) performed simulations on a single-zone building situated in Minneapolis, MN, Louisville, KY, or Miami, Florida. The building had conventional multi-layer construction, with 6-inch-thick vertical walls and a 4-inch-thick ceiling. Each of these surfaces included a layer of plasterboard that was impregnated with 25% paraffin, with a phase change temperature ranging between 25°C and 27.5°C. The study findings demonstrated that the incorporation of paraffin-based Phase Change Material (PCM) in the walls and ceiling led to a greater reduction in the building’s thermal load during the summer months compared to the winter months. Additionally, the orientation of the building also affected the extent of energy flux reduction through the composite walls (with PCM), owing to variations in heat flux resulting from incident solar radiation. Fraise et al. (2002) developed a precise construction model using thermoelectric analogy. They initially investigated the conversion of a multilayer wall into three resistors and four capacitors (3R4C) and compared it to other electrical models (3R2C and R2C) through frequency analysis. Henri and Darren Robinson (2007) proposed a simplified model for simulating heat flow in two-zone buildings. They utilized a resistance-capacitance type model to study energy exchange through radiation and convection between multilayer walls and the ambient air. The results demonstrated the accuracy of the bizone model for various typical wall constructions. Jinghua and Yu (2023) examined the thermal performance of a ventilation roof incorporating a form-stabilized phase change material (SPCM). They developed a dynamic heat transfer model by combining the number of transfer units (NTU) heat exchange model with the resistance-capacitance dynamic heat network model. The study indicated that PCM and night ventilation exhibited significant energy-saving potential, with the PCM layer achieving an increased average latent heat utilization rate and a reduced cumulative cooling load compared to the PCM roof and reference roof, respectively. Al-Yasiri and Márta (2023) used EnergyPlus software to numerically investigate the passive utilization of PCM in thin building envelopes within a very hot climate. They evaluated the thermal contribution of PCM to indoor thermal comfort by considering parameters such as average temperature fluctuation reduction (ATFR), thermal load leveling reduction (TLLR), and operating temperature reduction (OTR). The study also discussed metrics like total average heat gain reduction (AHGR), equivalent CO2 emissions, and electricity savings (ECS) to quantify energy efficiency. The integration of PCM in thin envelope elements proved to significantly enhance building performance in extremely hot locations. Chaoen et al. (2023) founded that using biomass-derived carbon as a support material is an inexpensive and environmentally friendly way to make composite MCPs with exceptional performance; Adopted multi-PCMs are an important way to solve the “seasonally adaptable problem.” Finally, crucial findings and future recommendations are also provided to guide researchers in seeking further improvements. Madeeha et al. (2022) carried out a numerical calculation in building simulation software, EnergyPlus. Initially, fifteen suitable PCMs are evaluated for a one-room reference house. CrodaTherm24 having a melting temperature of 24°C with a thickness of 40 mm proves to be the optimal PCM choice when placed on the interior sides of building envelopes. It is then integrated into typical single-storey and two-storey multi-zone residential buildings. For a one-storey building, the average monthly energy saving of 44.9% is achieved in Islamabad, 35% in Karachi, 32% in Lahore, 35% in Peshawar and 49.6% in Quetta, while for two-storey buildings, the average monthly energy savings of 12%, 21.4%, 15.5%, 12.9%, and 13.5% are achieved, respectively. The objective of this work is to use an intelligent material (PCM Phase Change Materials) to improve the thermal efficiency of building envelopes by using the method of apparent capacities, a fixed and regular mesh to model the thermal behavior of the material at phase change and several different room configurations have been studied. The results showed that the addition of a PCM in the vertical walls improves the thermal inertia of the building.
2 Analysis and modeling
2.1 Position of the problem
Our research focuses on investigating the thermal performance of a two-zone building, as illustrated in Figure 1. The building comprises two zones, namely, Zone 1 and Zone 2, with identical walls. The total surface area of the walls is S = 30 m2, and the volume of the building is V = 300 m3, with dimensions of height, length, and width measuring 3, 10, and 10 m, respectively. The two zones are separated by a party wall located on the East side.
To study the impact of thermal behavior, we have chosen a commercial paraffin called RT25 (Stéphane et al., 2014). This paraffin has a melting temperature of 26°C, which is close to the desired comfort temperature of 25°C during the summer season. The paraffin exhibits a specific temperature range for its phase change, indicating the temperatures at which it transitions between the liquid and solid states.
In our investigation, we have incorporated a 20 mm layer of paraffin into the walls of the building envelope. The paraffin layer can be positioned either on the exterior or the interior side of the walls, as depicted in Figure 2 and Tables 1–3.
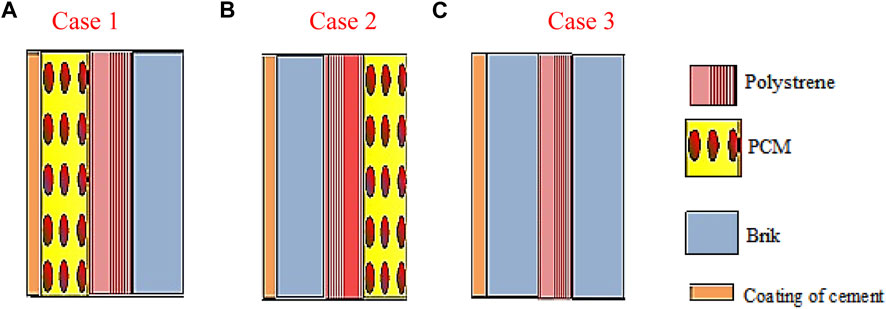
FIGURE 2. Description of the walls of the envelope, (A) reference case, (B) PCM on the exterior side, (C) PCM on the interior side.
2.1.1 The vertical walls (North-South–East-West)
We integrate a PCM in all the vertical faces of the envelope:
• Case 1: The PCMs are placed on the exterior faces of all the vertical walls.
• Case 2: The PCMs are placed on the interior faces of all the vertical walls.
• Case 3: A reference wall
2.2 Mathematical model
2.2.1 Modeling of phase change materials
Among the thermal models of PCM in the literature, we chose the apparent heat capacity method (Ben Tahar et al., 2019) at a fixed and regular mesh. It is a method that meets our requirements and is easy to implement. Given the above assumptions, the enthalpy form of the energy conservation equation is as follows:
With:
The volume enthalpy H can still be written with the term volume fraction of fluid in the following form:
We can obtain the equation of the apparent heat capacity (or equivalent heat capacity) of the medium by using the mathematical properties on the exact total derivatives, which is defined by:
So, the Eq. 4 becomes:
2.2.2 Assumptions
We cite the simplifying assumptions in order to be able to perform the calculations:
- Heat transfer is one-dimensional.
- The convection mode is negligible.
- Conduction in the solid and liquid phases is taken into account.
- The liquid phase is Newtonian and incompressible.
- Contact is assumed to be perfect between the two phases (no contact resistance).
- The thermal conductivity depends only on the temperature.
- The expansion of PCM is negligible and the phase change is isothermal.
2.2.3 Equation of the problem
In our study, the heat balance equation of node i is written:
2.3 Discretization of the model and solving method
In order to analyze the thermal and mass behavior of the two-zone building, the researchers employed the nodal method as a numerical approach. This method incorporates the concept of fictitious nodes and offers the advantage of not requiring a highly refined mesh, which is particularly beneficial in thermal building simulations. The nodal method divides the system into multiple elements, with each element represented by a node that is influenced by the temperature and thermo-physical properties of that specific element. In this study, a total of 81 nodes were utilized in the model. The thermoelectricity method, illustrated in Figure 3, was employed in the model and was validated through analytical methods (Lajimi and Boukadida, 2015; Ben Tahar et al., 2019; Lajimi et al., 2022). This approach facilitated the prediction and assessment of the energy advantages offered by the walls and the resulting composite materials containing PCM (Phase Change Material). The model demonstrated ease of use and provided accurate evaluations of the energy benefits associated with the walls and PCM composite materials.
2.4 Conditions of thermal boundaries
The values of meteorological temperature and outside air are provided by the Sousse local meteorological station, as shown in Figure 4A for the months of July. For the months of July, Figure 4B depicts the variation in the density of incident solar flux on the exterior vertical face (South, North, Est, and West). On the 15th of the month, which is considered a typical day, direct and diffuse solar density fluxes are calculated hourly.
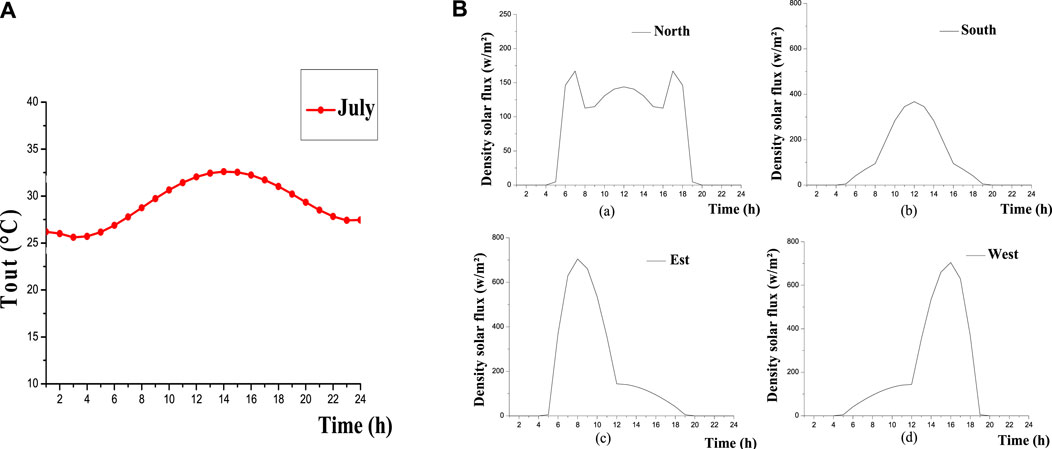
FIGURE 4. Outside air temperature (A) and variation in the density of the incident solar flux (B) for the months July.
3 Results and interpretation
3.1 Evolution of the indoor air temperature according to the position of the MCP
The Figure 5 shows that the indoor air temperature for the three cases studied (cases 1, 2, and 3) follows the outdoor temperature but it generates a phase shift of 2 h for the case reference (case 3) and 3 h for cases 1 and 2. The temperature profile corresponding to cases 1 and 2 shows a low damping between the daytime period and the night period, compared to that of the reference case. We find that when the faces of the envelope are equipped with a PCM (case 1: The PCMs are placed on the exterior faces of all the vertical walls), the latter generates a reduction in temperature amplitudes which is good for thermal comfort in summer.
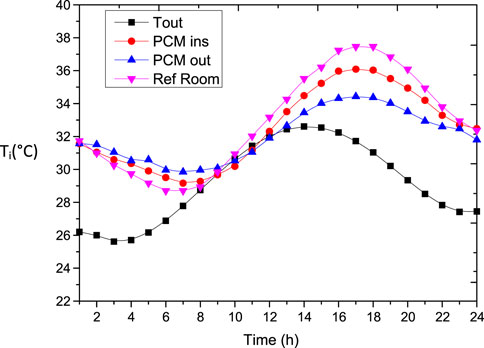
FIGURE 5. Evolution of indoor air temperature according to the position of PCM for the months of July.
3.2 Evolution of the temperature of the internal faces of the envelope
Figure 6 illustrates the evolution of the temperature of the interior faces of the vertical walls (North, South, East, and West), for the 2 rooms (case1: The PCMs are placed on the exterior faces of all the vertical walls and case3: A Reference wall) for July.
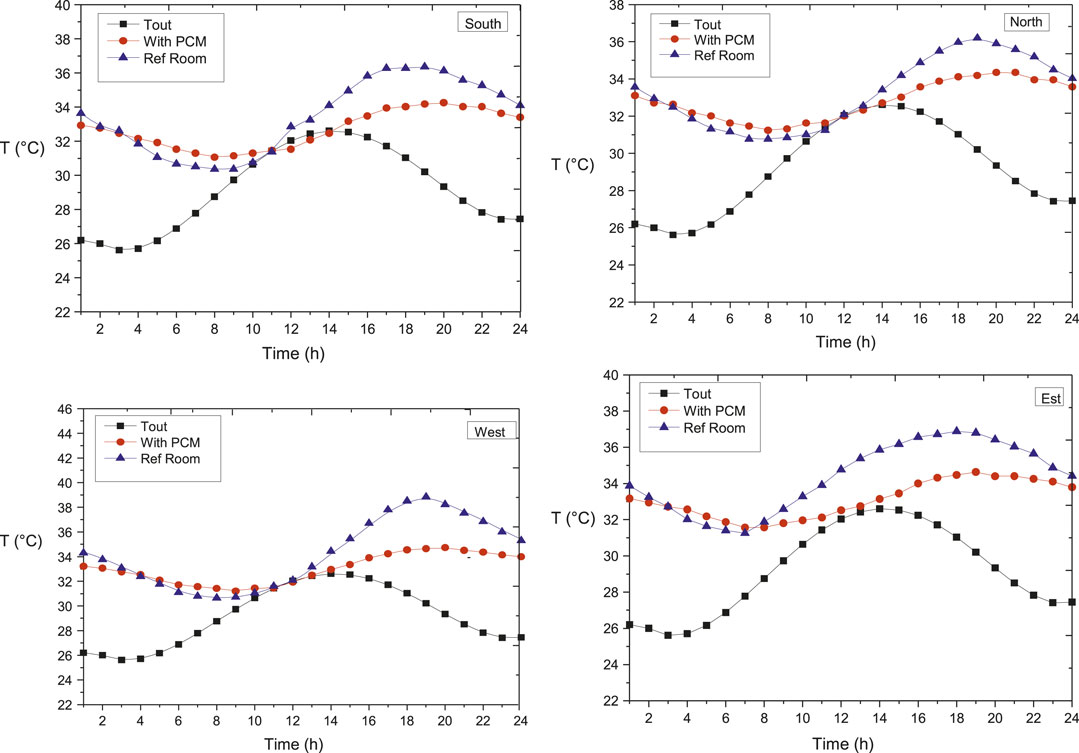
FIGURE 6. Hourly evolution of the temperature of the interior faces of a room during the summer season (July).
In case 1 where the walls are provided with PCM on the exterior side. The temperature profiles of the interior walls are around 32°C. In July, the PCMs reduce temperature fluctuations on the South face by 5°C, for the North face by 3°C, for the East face by 3.5°C and by approximately 10°C for the West face (Figure 6).
In case 3 where the case of a reference wall, the temperature profiles corresponding to the different faces represent a remarkable difference; during the month of July (Figure 6), it is around 33°C for the North and South faces and 34°C for the East and West faces. The difference between the maximum value of the temperature found in the case of reference and this is 2°C for the North face and the East face, 4°C for the South face and 5°C for the West face.
3.3 PCM thermal performance indicators
3.3.1 Room maximum temperature reduction (RMTR)
RMTR indicates how significant the temperature reduction inside the test room is for each PCM model during peak hours. This indicator clearly demonstrates the storage capacity of each PCM thickness as well as if or not the PCM is fully melted. RMTR is calculated in this manner by comparing the maximum temperature in the room of the PCM model to the reference room using Eq. 7, as shown below.
Where: Ti,maw.,ref, Ti,max.,PCM are the maximum temperature of the reference room (case3) and PCM rooms (case1) respectively.
We found that the maximum of the RTMR is obtained in the case in Figure 7 where the west wall is equipped with PCM placed on the outer side is around 13% still compare with the case without PCM (case3).
3.2.2 Decrementation factor and time phase shift
Figure 8A illustrates the relationship between the decrementation factor and the volume fraction of PCM, as described by Eq. 8. This factor quantifies the reduction in cyclic temperature between the inner and outer surfaces of the wall. Numerous studies have defined the decrementation factor as the ratio between the temperature oscillation amplitudes at the inner and outer surfaces of the wall, as expressed in reference (Thiele et al., 2017)
Where
4 Conclusion
In this study, the thermoelectric analogy method was employed to investigate the impact of phase change material (PCM) on the thermal load transmitted through a building envelope under realistic weather conditions in Sousse, Tunisia. The findings of the study demonstrated that:
- When PCMs were applied to the external side of the vertical walls through fixing, they effectively reduced the thermal fluctuations of the interior temperatures during hot periods,
- The proposed method utilized various PCM performance indicators, including indoor surface temperature, RMTR (Room maximum temperature reduction), decrement factor, and time lag, to evaluate the influence of PCM on the building,
- The study concluded that the incorporation of PCM in the building envelope increased its thermal inertia, directly influencing the perception of thermal comfort. This indicates that the utilization of PCM can contribute to improved thermal performance and a more comfortable indoor environment. As a perspective study, we wish to extend our work also with the help of an experimental study of the wall containing an adequate phase change material which can make it possible to validate the numerical results.
Data availability statement
The original contributions presented in the study are included in the article/Supplementary Material, further inquiries can be directed to the corresponding author.
Author contributions
NL integrated the MCP heat balance into the building model. He completed the discretization of the physical problem equation, the numerical simulation, the analysis and the interpretation of the obtained results. The work is based on what has been studied by NL who used the same building model and the same numerical method (nodal method) in previous work to evaluate the energy performance of buildings with a MCP integrated in the roof wall. NB contributed with his correction of the present paper is the supervisor of this team. All authors contributed to the article and approved the submitted version.
Conflict of interest
The authors declare that the research was conducted in the absence of any commercial or financial relationships that could be construed as a potential conflict of interest.
Publisher’s note
All claims expressed in this article are solely those of the authors and do not necessarily represent those of their affiliated organizations, or those of the publisher, the editors and the reviewers. Any product that may be evaluated in this article, or claim that may be made by its manufacturer, is not guaranteed or endorsed by the publisher.
References
Adnan, K., and Ghaida, A. (2021). Muhammad Basha Developing building enhanced with PCM to reduce energy consumptionJournal of Building. Engineering 48 (1), 103923. doi:10.1016/j.jobe.2021.103923
Al-Yasiri, Q., and Marta, S. (2022). Energetic and thermal comfort assessment of phase change material passively incorporated building envelope in severe hot Climate: An experimental study. Appl. Energy 314 (2), 118957. doi:10.1016/j.apenergy.2022.118957
Al-Yasiri, Q., and Marta, S. (2023). Numerical analysis of thin building envelope-integrated phase change material towards energy-efficient buildings in severe hot location. Sustain. Cities Soc. 89, 104365. doi:10.1016/j.scs.2022.104365
Ben Tahar, N., Lajimi, N., and Boukadida, N. (2019). Evaluation of the effect of a phase-change material on the thermal response of a bizone building under the climatic conditions of Tunisia. Comptes Rendus Phys. 20, 503–603. doi:10.1016/j.crhy.2019.01.004
Biwole, P. H., Eclache, P., and Kuznik, F. (2013). Phase-change materials to improve solar panel’s performance. Energy Build. 62, 59–67. doi:10.1016/j.enbuild.2013.02.059
Castell, A., Martorell, I., Medrano, M., Pérez, G., and Cabeza, L. F. (2010). Experimental study of using PCM in brick constructive solutions for passive cooling. Energy Build. 42 (4), 534–540. doi:10.1016/j.enbuild.2009.10.022
Chaoen, L., Xiaodong, W., Wei, C., Hang, Y., and Dongjing, L. (2023). Phase change material for passive cooling in building envelopes: A comprehensive review. J. Build. Eng. 65, 105763. doi:10.1016/j.jobe.2022.105763
Fraise, G., Viardot, C., Lafabrie, O., and Achard, G. (2002). Development of a simplified and accurate building model based on electrical analogy. Energy Build. 34, 1017–1031. doi:10.1016/S0378-7788(02)00019-1
Henri, J., and Darren Robinson, K. (2007). A simplified thermal model to support analysis of urban resource flows. Energy Build. 39, 445–453. doi:10.1016/j.enbuild.2006.09.002
Jinghua, Yu a. (2023). The energy saving potential of a new ventilation roof with stabilized phase change material in hot summer region. Renew. Energy. doi:10.1016/j.renene.2023.05.012
Kissock, K., and Limas, S. (2006). Diurnal load reduction through phase-change building components. ASHRAE Trans., 509–517.
Lajimi, N., Ben Taher, N., and Boukadida, N. (2022). Numerical study of the energy efficiency of the building envelope containing multi-alveolar structures under Tunisian weather conditions. Int. J. Nonlinear Sci. Numer. Simul. 23, 931–946. doi:10.1515/ijnsns-2021-0090
Lajimi, N., and Boukadida, N. (2015). Numerical study of the thermal behavior of bi-zone buildings. Comptes Rendus Phys. 16 (8), 708–720. doi:10.1016/j.crhy.2015.08.010
Luisa, F. C., Cecilia, C., Miquel, N., Marc, M., Ron, L., and Oihana, Z. (2007). Use of microencapsulated PCM in concrete walls for energy savings. Energy Build. 39 (2), 113–119. doi:10.1016/j.enbuild.2006.03.030
Madeeha, K., Muhammad, A., Muhammad, I., Naseem, A., Ilyas, K., Mohamed, M., et al. (2022). Energy performance enhancement of residential buildings in Pakistan by integrating phase change materials in building envelopes. Energy Rep. 8, 9290–9307. doi:10.1016/j.egyr.2022.07.047
Pappu, A., Velraj, R., and Pandiyarajan, V. (2022). Effective PCM, insulation, natural and/or night ventilation techniques to enhance the thermal performance of buildings located in various climates. A Rev. Build. 258 (1), 111840. doi:10.1016/j.enbuild.2022.111840
Stéphane, G., Frédéric, M., Dimitri, B., and Horry, B. (2014). A thermal model for phase change materials in a building roof for a tropical and humid climate: Model description and elements of validation. Energy Build. 70, 71–80. University of Reunion, France. doi:10.1016/j.enbuild.2013.11.079
Thiele, A. M., Ligett, R. S., Sant, G., and Pilon, L. (2017). Simple thermal evaluation of building envelopes containing phase change materials using a modified admittance method. Energy Build. 145, 238–250. doi:10.1016/j.enbuild.2017.03.046
Zwanzig, S. D., Lian, Y., and Brehob, E. G. (2013). Numerical simulation of phase change material composite wallboard in a multi-layered building envelope. Energy Convers. Manage 69, 27–40. doi:10.1016/j.enconman.2013.02.003
Nomenclature
Keywords: numerical method, heat transfer, phase change material, solar flux, building
Citation: Lajimi N and Boukadida N (2023) Effect of the position of the PCM on the thermal behavior of a multilayer wall of a building in summer period. Front. Environ. Sci. 11:1226454. doi: 10.3389/fenvs.2023.1226454
Received: 21 May 2023; Accepted: 27 July 2023;
Published: 09 August 2023.
Edited by:
Sandip K. Saha, Indian Institute of Technology Bombay, IndiaReviewed by:
Selvaraj Jegadheeswaran, Bannari Amman Institute of Technology (BIT), IndiaKarolina Matuszek, Monash University, Australia
Surendra Poonia, Central Arid Zone Research Institute (ICAR), India
Copyright © 2023 Lajimi and Boukadida. This is an open-access article distributed under the terms of the Creative Commons Attribution License (CC BY). The use, distribution or reproduction in other forums is permitted, provided the original author(s) and the copyright owner(s) are credited and that the original publication in this journal is cited, in accordance with accepted academic practice. No use, distribution or reproduction is permitted which does not comply with these terms.
*Correspondence: Nour Lajimi, bm91cmxhamltaUB5YWhvby5mcg==