- 1Department of Entomology, National Chung Hsing University, Taichung, Taiwan
- 2Department of Entomology, University of California, Riverside, Riverside, CA, United States
- 3Center for Marine Environmental Studies, Ehime University, Matsuyama, Japan
Both intensive farming of paddy field crops and conventional farm management techniques have been a major cause of environmental issues in recent years. A more ecosystem-based, sustainable agricultural methodology should be utilized to help solve this dilemma. However, the joint effects of landscape and farming on arthropod communities in paddy fields are unexplored. In eco-friendly paddy fields, we postulate the arthropod diversity to be higher in fields that use ecosystem-based methods. In this study, we collected arthropods monthly using a blower-vac during the first rice crop of 2019 in Taiwan. A total of 53,522 individuals of 14 orders, 96 families, and 445 morphospecies were collected. All arthropods were identified into four functional groups. The result showed that insect communities are different between sustainable and conventional fields. Results showed that sustainable fields had a higher abundance of arthropod fauna than conventional fields. It was also apparent that the arthropod community changed along with the developmental growth of rice and in different areas. Three functional groups, viz., herbivores, parasitoids, and detritivores, present the best model when the farming type was assigned as the fixed effect, while the predator reveal the best model when the farming type, landscape, and their interaction were assigned as the fixed effects. The arthropod communities have also been affected by the surrounding landscape. This study reveals that both agricultural management and landscape can have a joint effect on arthropod communities in paddy fields.
1 Introduction
Sustainable agriculture is a relatively new farming idea based on an ecological concept that embraces the interactions of organisms to achieve a balance and maintain the productivity of a given ecosystem. In contrast, in conventional agriculture, pesticides have been used intensively to control pests and diseases that maintain the crop yield loss and product quality (Aktar et al., 2009; Damalas, 2009). Although the usage of conventional agricultural methods can control the pest population, non-target organisms and their associated agroecosystem environments are highly threatened (Gibbs et al., 2009; Stanley, 2016). For example, the decline of arthropod predator diversity occurs (Frampton and Çilgi, 1994; Marie et al., 2018; Graf et al., 2019). Therefore, outcomes of sustainable agriculture are closely linked to the ecological environment and have resulted in several interactions being developed, such as eco-agriculture (McNeely and Scherr, 2003), natural farming (Fukuoka, 2010), organic agriculture (Geier, 2007), environmentally friendly farming (Novelli, 2018), and low-input agriculture (Daberkow and Reichelderfer, 1988). The combination of ecology and new technology can decrease the environmental costs of conventional agriculture. It is believed that the long-term application of such methods can improve the quality of resources and help preserve the ecological environment (Harwood, 1990).
The surrounding landscape of agroecosystems is also one of the important factors affecting the diversity of agroecosystem arthropods (Hendrickx et al., 2007; Mitchell et al., 2014). The whole farmland area may be separated by roads and low-density buildings. It is block-fragmented and may be surrounded by natural or semi-natural habitats such as vegetable gardens, orchards, woodlands, mountains, wastelands, streams, and artificial irrigation ditches (Verhagen et al., 2016; Haan et al., 2019). Baba and Tanaka (2016) found that ditches around fields can serve as non-rice cropping periods and overwintering habitats for Tetragnathidae spiders. Similarly, the combination of paddy fields and surrounding forests can increase the richness and abundance of grassland-dwelling spiders (Miyashita et al., 2012). Delettre and Morvan (2000) suggested that the distance from natural waterbodies affects the dispersal of Chironomidae species in agricultural areas. As the aforementioned studies suggest, different habitats around a farmland affect the make-up of the farmland’s arthropod diversity and preserve the habitats for natural enemies (Rusch et al., 2010; Holland et al., 2016). Diverse habitats surrounding the farmland also help maintain the biodiversity so that agroecosystems can maintain good ecosystem services for both humans and other organisms (Landis, 2017).
Arthropods play an important role in the human economy and ecological niches of the agroecosystem (Chakravarthy and Sridhara, 2016). They are not only preyed on by high trophic-level consumers, such as birds, frogs, and reptiles but also connect and interact with other arthropods in the food web of agroecosystems. Rice (Gramineae: Oryza sativa L.) is an important food crop in Asia and elsewhere. The production of approximately 770 million tons per year makes rice one of the most important agricultural products produced in the world (FAO, 2017). A paddy field is both a semi-humid and semi-arid habitat that consists of a unique ecosystem that combines the properties of both wetland and dryland areas that are common in the agricultural landscape of Asia. Paddy fields are flooded in the growing stage and become dry land before the harvest season; therefore, paddy fields are also a part of the wetland (Hook, 1993; Watanabe, 2018), attracting habitation of both aquatic and terrestrial arthropods. As Bambaradeniya and Amerasinghe (2004) discussed, in paddy field agroecosystems, the highest diversity of invertebrates is arthropods, most of which are insects. Arthropod communities develop, and their success coincides with rice planting stages. Arthropods in paddy field agroecosystems are highly diverse, susceptible to paddy field conditions, and can be regarded as indicators to help understand how paddy field agroecosystem management impacts organisms (Ueno, 2012; Morrison et al., 2018).
Most of the studies regarding agricultural management and agricultural landscape effects on arthropods focus on wheat fields in temperate biomes (Moreby et al., 1994; Clough et al., 2007; Diekötter et al., 2010; Tuck et al., 2014). Taiwan is an island located at the border of tropical and subtropical climate zones. We believe that our work is the first to assess the joint effects of farming and landscape on arthropod communities in paddy fields. Taiwan is narrow in area but densely populated; hence, the total potential area for cultivation is also limited. Small and quality agricultural areas are well-developed in Taiwan, and the arthropod community of paddy fields here is different compared to those of temperate and tropical zones. Therefore, we aim to examine and compare the effects of agricultural management and landscape factors on total and dominant arthropod abundance and diversity in a subtropical zone (i.e., northeastern Taiwan). This study examines the advantages and disadvantages of paddy field management systems that are currently in practice. It also compares the relationship between various arthropod communities and the landscape surrounding these management systems. This will help establish basic information that can serve as a reference for future evaluation of agricultural management strategies.
2 Materials and methods
2.1 Arthropod sampling
We selected four sampling areas, each more than 1 km in the Lanyang Plains of Taiwan (Figure 1). Each sampling area contained two paddy fields that were used for conventional agriculture (CA) and sustainable agriculture (SA), respectively (Table 1). The distance between these two fields was more than 100 m for decreasing the effect of the farming type. The major distinction between CA and SA is that CA uses chemical pesticides and fertilizers, and SA uses non-chemical materials. The four sampling areas were located in Jiaoxi (Jx), Zhuangwei (Zw), Luodong (Ld), and Sanxing (Sx). The farming information is shown in Table 1. Arthropods were collected monthly during the first crop season, i.e., from March to June 2019. A customized blower-vac (Arida and Heong, 1992; Buffington and Redak, 1998) was used for sampling arthropods on four randomly selected plots on each field (2.5 * 2.5 square meters). Arthropods were collected from the aboveground part of rice plants in each field. Each of the sampling plots was sampled for 90 s. The samplings were preserved in 34*45-cm zipper bags, brought back to the laboratory, and preserved in 70% ethanol for further sorting and identification. All specimens were identified to at least family level and categorized as morphospecies given a serial number, following the abbreviation of order, such as Hem-03 and Dip-08. Additionally, arthropods were grouped into functional guilds, herbivores, predators, parasitoids, and detritivores, for further analysis (Dominik et al., 2017). Supplementary data to this article can be found online at https://doi.org/10.13140/RG.2.2.10367.18082.
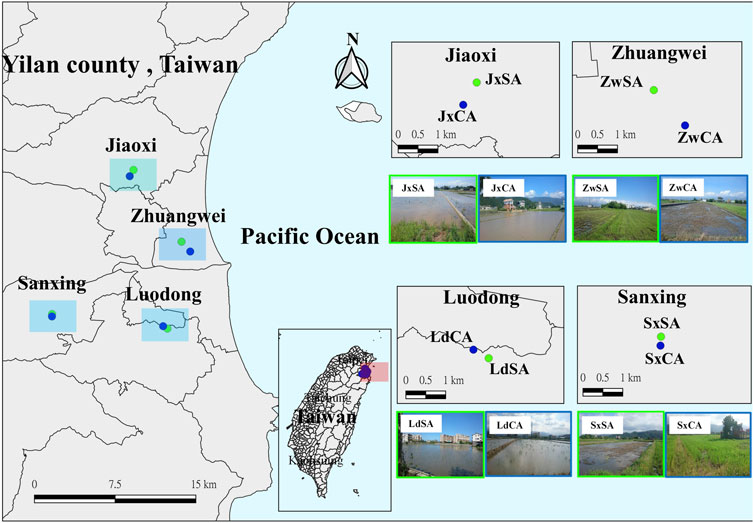
FIGURE 1. Location of the sample sites in four sampling areas in northeastern Taiwan. Green and dark blue points stand for sustainable and conventional farming fields, respectively. Jx, Jiaoxi; Zw, Zhuangwei; Ld, Luodong; Sx, Sanxing. SA, sustainable agriculture; CA, conventional agriculture.
2.2 Environmental data
For the environmental characteristics of each sampling area, we determined the spot directly between the sustainable and conventional sample areas and measured a 1-km radius from that point. Then, we measured the field size and surrounding landscape coverage using Google Earth Pro and ImageJ (v1.52r) software. We identified various landscape coverage, such as farmland, forest, ditch, cement (cement building and road), and stream. These ratios of coverage were presented by principal component analysis (PCA) to reveal the landscape characteristics of each sampling site (Figure 2). The Jx area possesses relatively more forests. Sx and Zw areas were occupied by paddy farms and some streams, while the Ld area has a large portion of buildings and some artificial ditches. Four sampling areas show different surrounding landscapes. In addition, the rice field size hectare, rice plant average interval, and width were measured.
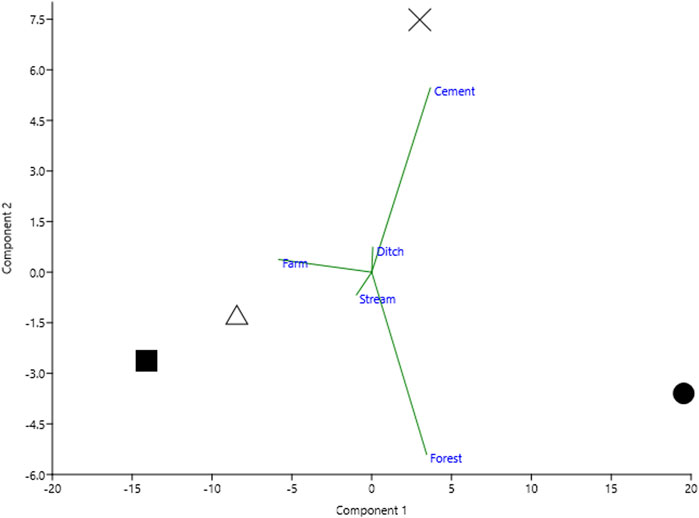
FIGURE 2. Four sampling sites characterized by five environmental data by principal component analysis. Four sampling areas are displayed by shape: circle, Jiaoxi (Jx); square, Zhuangwei (Zw); cross, Luodong (Ld); triangle, Sanxing (Sx).
2.3 Statistical analysis
We characterized the arthropod community structure by calculating the abundance, species richness, Shannon’s diversity index, Simpson’s diversity index, Pielou’s evenness index, and Margalef’s richness index for each field using Past 3.14 software (Hammer et al., 2001). To determine whether the arthropod communities were affected by the season, farming type, and landscape, we used permutational analysis of variance (PERMANOVA) performed by the package ‘vegan’ within the R statistical framework (version 4.0.0). The abundance of all arthropods was log-transformed prior to analyses to meet the assumptions of normality (Pinheiro et al., 2014). To measure the differences in the species structure among study sites, we performed non-metric multidimensional scaling (NMDS) ordinations after the computation of a Bray–Curtis dissimilarity matrix based on arthropod abundances.
To determine whether the abundance of each ecological group was affected by the farming type or landscape, we used generalized linear mixed models (GLMMs) performed by the lme4 package within the R statistical framework (version 4.0.0). The farming type, landscape, farming type + landscape, and farming type*landscape were assigned as fixed effects sequentially. The model with the lowest Akaike’s information criterion (AIC) is regarded as the best. In the GLMM, the farming system is a binary variable (either a conventional or sustainable system) in the model, while the landscape was represented by four sampling sites (Jx, Zw, Ld, and Sx, Figure 2). Other field characteristics, including the rice field size hectare, rice plant average interval, and width, were assigned as random effects. A stepwise regression procedure was used together with testing all variable combinations to determine the best-fitting model based on the lowest AICc score. The procedure was repeated separately for each response variable and spatial scale. Each response variable has a Poisson distribution in the model.
3 Results
In total, 53,522 individuals of 14 orders, 96 families, and 445 morphospecies were collected overall for sampling during the study period (Figure 3). The arthropod composition varied among different sampling sites (Figure 3). The arthropod communities located in Jx were characterized by Thysanoptera (35%) and Hemiptera (18%) in SA farming, while Diptera (43%) was the most abundant taxa in CA farming. In Zw, Hemiptera (54%) was the most dominant taxa in SA farming, while Diptera (38%) and Acariformes (26%) were more abundant in CA farming. In Ld, Diptera was seen in SA farming and CA farming (31% and 48%, respectively). Regarding Sx, both Thysanoptera and Hemiptera were the most dominant taxa in SA farming (34% and 39%, respectively) and CA farming (39% and 32%, respectively) (Figure 3). Dominant taxa (90% of all arthropods) presented by NMDS showed that each type of paddy field was separated by different dominant arthropods (Figure 4). Overall, more hemipterans (specifically Delphacidae and Cicadellidae) and thysanopterans (almost Thripidae) inhabit the SA farm, while more dipterans (specifically Chironomidae) occurred in the CA farm.
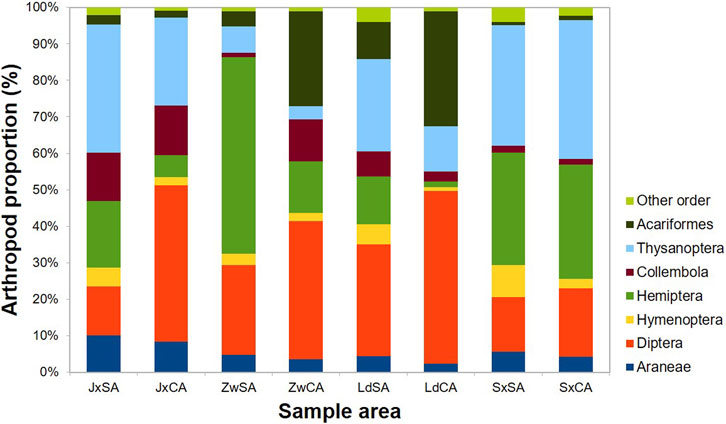
FIGURE 3. Fraction of abundance of order-level terrestrial arthropods in four sample areas in northeastern Taiwan. SA field, sustainable agricultural field; CA field, conventional agricultural field; Jx, Jiaoxi; Zw, Zhuangwei; Ld, Luodong; Sx, Sanxing.
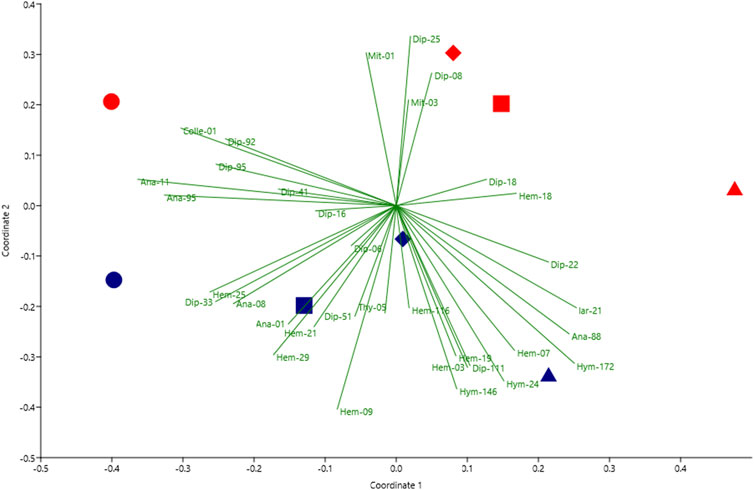
FIGURE 4. Non-metric multidimensional scaling (NMDS) analysis of the Bray–Curtis dissimilarity matrix represented by 34 dominant taxa (90% of all arthropods). Each point represents each paddy field. Sampling areas are displayed by shape: circle, Jiaoxi (Jx); square, Zhuangwei (Zw); diamond, Luodong (Ld); triangle, Sanxing (Sx). The farming type is marked by color: blue, sustainable agriculture (SA); red, conventional agriculture (CA) (stress = 0.091).
According to the PERMANOVA analysis, the arthropod abundance was significantly different among months (F = 6.55, p < 0.001), the farming type (F = 2.29, p < 0.05), and area (F = 1.95, p < 0.005). The arthropod composition varied along with the growing stages of the paddy field, from the seedling (March) to the mature stages (June). Diptera (specifically Chironomidae) was dominant in the seedling stage, while Hemiptera and Thysanoptera (major herbivores) were dominant in the latter stages. The arthropod community was more abundant under SA farming compared to CA farming (Table 2). Generally, the number of arthropod families, morphospecies, and species abundance in SA farming was higher than that in CA farming, except for the Ld sampling area (Table 2). Shannon’s Index, Simpson’s Index, Pielous’s Index, and Margelef’s Index indicate that the diversity was higher in SA farming than those in CA farming. LdSA has the highest diversity index, while LdCA has lowest (Table 2).
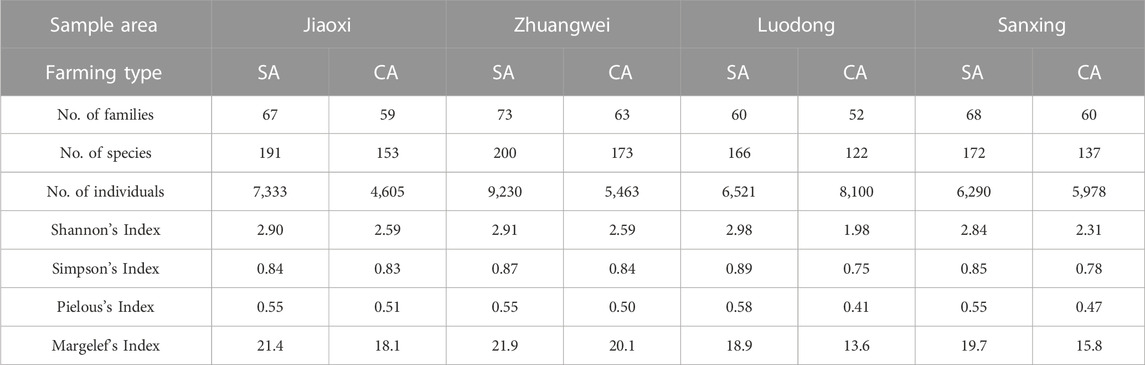
TABLE 2. Biodiversity indicator results of sample sites in four sample areas in northeastern Taiwan (SA, sustainable agriculture; CA, conventional agriculture).
Regarding the ecological guilds, herbivores accounted for 53.2% of the total arthropods collected and were dominated by white-backed planthoppers (Sogatella furcifera), leafhoppers (Macrosteles striifrons), and the rice thrips (Stenchaetothrips biformis). Predators contributed 7.5% of the total abundance and were mostly represented by long-jawed orb weavers (Tetragnathidae), orb-weaver spiders (Araneidae), Miridae, and Coccinellidae. The detritivores guild represented 35.3% of the total arthropod abundance and was mainly composed of chironomids and some collembolans. Finally, parasitoids accounted for 4.0% of the total abundance and were mainly represented by Mymaridae and Pteromalidae. According to the GLMM, the abundance of herbivores (AIC = 498.8, p < 0.001), parasitoids (AIC = 334.4, p < 0.001), and detritivores (AIC = 478.2, p < 0.001) presents the best model when only the farming type was assigned as the fixed effect, while the predators reveal the best model when the farming type, landscape, and their interaction were assigned as the fixed effects (AIC = 381.3, p < 0.05). Moreover, the abundance of herbivores, parasitoids, and predators is higher under SA farming but not detritivores (Figure 5).
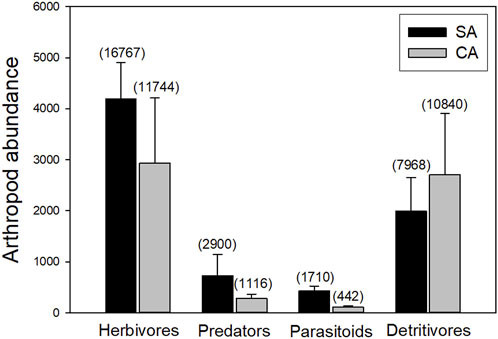
FIGURE 5. Comparison of arthropod abundance between SA and CA farming. The total arthropod abundance is shown in the parentheses.
4 Discussion
We recorded a total of 445 morphospecies of arthropods in the paddy field of northern Taiwan, which is higher than in other studies of the paddy field in the Philippines (Heong et al., 1991; Dominik et al., 2017). Overall, the abundance of arthropods in SA farming was higher than that of CA farming (Table 2). Conventional agroecosystems are not easily colonized by arthropod species as there is typically a lack of flora diversity (monocropping), and these areas are heavily disturbed (Brown, 1991; Benton et al., 2003). SA farming (eco-friendly agriculture) has a higher abundance of arthropods because of the limited use of pesticides.
Hemiptera, Thysanoptera, and Diptera are the three most dominant arthropod taxa in our study sites. Hemiptera, mostly Delphicidae, Cicadellidae, and Aphidae, and Thysanoptera are known major pests of rice in Taiwan (classified as herbivores). The abundance of these two taxa is significantly higher under SA farming compared to that in CA farming. This result is predictable because some major pesticides applied in the paddy field are specific to hemipteran and thysanopteran pests. However, another dominant group, Diptera (specifically Chironomidae), is classified as detritivores in this study, whose abundance is higher under CA farming. Their larvae are aquatic and feed on organic humus and algae in farmlands, ditches, and semi-aquatic environments (Stevens et al., 2006). Chironomids can endure water pollution and do not require good habitat quality (Al-Shami et al., 2010). In the early stages of rice farming, paddy fields are full of water. At this time, a large number of chironomids inhabit the paddy fields. They usually occur in the early stage of the paddy field, in which the pesticide spray is less compared to the later stage of the paddy field. This may cause some effects on chironomids by pesticides. On the other hand, Diptera abundance may be slightly affected by certain landscape factors, including ditch coverage. In this study, Chironomidae represented nearly half of the total arthropod abundance in the LdCA, which possess some coverage of ditches (Figure 2). The distance to the nearest waterbody also affects the distribution of chironomid populations (Delettre and Morvan, 2000). However, this phenomenon is not verified statistically and needs further study.
In this study, we also have found that the abundance of parasitoids, specifically parasitoid wasps of Hymenoptera, is higher in paddy fields under SA farming methodologies. This has seldom been mentioned in previous studies. The importance of other natural enemies in paddy fields has been well-documented, such as spiders and ground beetles (Tsutsui et al., 2016; Russell et al., 2017). These generalists play an important role in the suppression of pest populations (Settle et al., 1996). However, the significance of specialists, like parasitoid wasps, deserves more attention. Parasitoid wasps search for specific host species and effectively decrease the population of that host, which may or may not be considered a pest. The most dominant parasitoids in this study are Mymaridae, which are the major parasitoids of hemipterans (Jacob et al., 2006; Mutitu et al., 2013). It is observed that the most dominant herbivores in the paddy field are hemipterans. Both ecological guilds are significantly increased in the sustainable farming type (Birkhofer et al., 2016).
Other factors of the farming type may also be beneficial to the arthropod community, like loosening planting density. Many previous studies have pointed out that the rice planting density and interval are related to the field’s hemipteran population and disease occurrence (Denno and Roderick, 1991; Ishii-Eiteman and Power, 1997). Moreover, the density of rice plants may affect an arachnid’s ability to inhabit the paddy because dense planting would make web building more difficult, and the prey could remain hidden more easily (Foelix, 2011; Butt and Xaaceph, 2015). Roitberg (2018) also suggested that dense field planting may increase the difficulties for parasitoid wasps to find their host.
In this study, the surrounding landscape seems to not have any overall effect on the arthropod community, inferring that a simplified landscape may weaken the effect of the landscape. Dominik et al. (2017) suggested that arthropod composition is extremely different among different landscapes, which is less significant in our study. However, the sampling sites of Dominik et al. (2017) are separated by about 100 km, which makes the surrounding landscape completely different, including the terrain and flora. These abiotic and biotic differences cause the varied arthropod composition. In contrast, our four sampling sites are all located in the Lanyang Plains, which is an important area of rice production in northern Taiwan since the 18th century. After a long history of reclaim, more than 11,100 ha of area are paddy fields currently (AFA, 2023). The native vegetation has been removed in this area, which is a simplified landscape. The sampling areas of this study are separated by merely more than 1 km, which suggests that the arthropods moderately share the same surrounding landscape. The simplified landscape may result in a decrease in arthropod diversity; on the other hand, it highlights the importance of sustainable farming in the paddy field. More biodiversity in an agricultural ecosystem will bring more ecological service to humans. The arthropod diversity is more vulnerable in a simplified landscape without abundant vegetation. Moreover, the neighborhood effects of conventional farms cause negative effects on the sustainable farms nearby. We suggest that the policy of payments for ecosystem services (PES) should be promoted in Lanyang Plains to maintain the arthropod diversity.
5 Conclusion
In conclusion, our work suggests that the effects of the sustainable farming type on arthropod communities in paddy fields are beneficial to certain arthropod taxa, such as Hemiptera (major herbivores), Araneae (major predators), and Hymenoptera (major parasitoids). The spraying of pesticides mainly focuses on the hemipteran prey, and the major host of parasitoid wasps collected by this study is also hemipterans. To properly analyze and compare arthropod diversity and abundance with factors such as farming methodology and landscape, some important steps must be taken. First, a specific understanding of the diversity and taxonomic makeup of the ecosystems that are being examined must be developed. Once this is understood, taxa that are known to be more sensitive to factors that are being compared and focused on should be selected. Second, different farms located in varied landscapes need to be compared to understand the effect of landscapes on the arthropod community.
Data availability statement
The datasets presented in this study can be found in online repositories. The names of the repository/repositories and accession number(s) can be found in the article/supplementary material.
Ethics statement
The manuscript presents research on animals that do not require ethical approval for their study.
Author contributions
Conceptualization: J-HC, Y-CL, and M-MY; methodology and software: J-HC and M-CC; data collection and curation: J-HC and Y-CL; writing—original draft preparation: J-HC and Y-CL; supervision: Y-CL and M-MY; funding acquisition: M-MY. All authors contributed to the article and approved the submitted version.
Funding
This study was supported by grants from the Ministry of Science and Technology, Taiwan (Project ID: 107-2621-M-005-002).
Acknowledgments
The authors are grateful to several rice farmers Chien-Fu Yu, Sheng-Wen Yu, Wen-Chung Hsu, Chin-Kuo Tseng, and Wen-Huo Sung for providing paddy fields for our research; Chao-Nien Koh and Yung-Jen Lu for their kind help to contact farmers; and Person Huang for the loan of a customized blower-vac.
Conflict of interest
The authors declare that the research was conducted in the absence of any commercial or financial relationships that could be construed as a potential conflict of interest.
The handling editor JRL is currently organizing a Research Topic with the author M-CC.
Publisher’s note
All claims expressed in this article are solely those of the authors and do not necessarily represent those of their affiliated organizations, or those of the publisher, the editors, and the reviewers. Any product that may be evaluated in this article, or claim that may be made by its manufacturer, is not guaranteed or endorsed by the publisher.
References
AFA (2023). frame. Available at: https://agr.afa.gov.tw/afa/afa_frame.jsp.
Aktar, W., Sengupta, D., and Chowdhury, A. (2009). Impact of pesticides use in agriculture: their benefits and hazards. Interdiscip. Toxicol. 2, 1–12. doi:10.2478/v10102-009-0001-7
Al-Shami, S. A., Rawi, C. S. M., HassanAhmad, A., and Nor, S. A. M. (2010). Distribution of Chironomidae (insecta: diptera) in polluted rivers of the juru river basin, penang, Malaysia. J. Environ. Sci. 22, 1718–1727. doi:10.1016/s1001-0742(09)60311-9
Arida, G., and Heong, K. (1992). Blower-vac: a new suction apparatus for sampling rice arthropods. Int. Rice Res. Newsl. 17, 30–31.
Baba, Y. G., and Tanaka, K. (2016). Factors affecting abundance and species composition of generalist predators (Tetragnatha spiders) in agricultural ditches adjacent to rice paddy fields. Biol. Control 103, 147–153. doi:10.1016/j.biocontrol.2016.09.004
Bambaradeniya, C. N., and Amerasinghe, F. P. (2004). Biodiversity associated with the rice field agroecosystem in asian countries: a brief review. Colombo, Sri Lanka: International Water Management Institute.
Benton, T. G., Vickery, J. A., and Wilson, J. D. (2003). Farmland biodiversity: is habitat heterogeneity the key? Trends Ecol. Evol. 18, 182–188. doi:10.1016/s0169-5347(03)00011-9
Birkhofer, K., Arvidsson, F., Ehler, D., Mader, V. L., Bengtsson, J., and Smith, H. G. (2016). Organic farming affects the biological control of hemipteran pests and yields in spring barley independent of landscape complexity. Landsc. Ecol. 31, 567–579. doi:10.1007/s10980-015-0263-8
Brown, V. (1991). “The importance of habitat structure in the maintenance of insect species diversity,” in Diversidad biológica. Editors F. Pineda, M. Casado, M. Miguel, and J. Montalvo (Madrid: Editorial Centro de Estudios Ramón Areces S.A), 49–55.
Buffington, M., and Redak, R. (1998). A comparison of vacuum sampling versus sweep-netting for arthropod biodiversity measurements in California coastal sage scrub. J. Insect Conservation 2, 99–106. doi:10.1023/a:1009653021706
Butt, A., and Xaaceph, M. (2015). Functional response of Oxyopes javanus (araneidae: oxyopidae) to Sogatella furcifera (Hemiptera: Delphacidae) in laboratory and mesocosm. Pak. J. Zoology 47, 89–95.
Chakravarthy, A. K., and Sridhara, S. (2016). Economic and ecological significance of arthropods in diversified ecosystems: sustaining regulatory mechanisms. Singapore: Springer.
Clough, Y., Holzschuh, A., Gabriel, D., Purtauf, T., Kleijn, D., Kruess, A., et al. (2007). Alpha and beta diversity of arthropods and plants in organically and conventionally managed wheat fields. J. Appl. Ecol. 44, 804–812. doi:10.1111/j.1365-2664.2007.01294.x
Daberkow, S. G., and Reichelderfer, K. H. (1988). Low-input agriculture: trends, goals, and prospects for input use. Am. J. Agric. Econ. 70, 1159–1166. doi:10.2307/1241756
Damalas, C. A. (2009). Understanding benefits and risks of pesticide use. Sci. Res. Essays 4, 945–949.
Delettre, Y. R., and Morvan, N. (2000). Dispersal of adult aquatic Chironomidae (Diptera) in agricultural landscapes. Freshw. Biol. 44, 399–411. doi:10.1046/j.1365-2427.2000.00578.x
Denno, R. F., and Roderick, G. (1991). “Influence of patch size, vegetation texture, and host plant architecture on the diversity, abundance, and life history styles of sapfeeding herbivores,” in Habitat structure. Editors S. S. Bell, E. D. McCoy, and H. R. Mushinsky (Dordrecht: Springer), 169–196.
Diekötter, T., Wamser, S., Wolters, V., and Birkhofer, K. (2010). Landscape and management effects on structure and function of soil arthropod communities in winter wheat. Agric. Ecosyst. Environ. 137, 108–112. doi:10.1016/j.agee.2010.01.008
Dominik, C., Seppelt, R., Horgan, F. G., Marquez, L., Settele, J., and Václavík, T. (2017). Regional-scale effects override the influence of fine-scale landscape heterogeneity on rice arthropod communities. Agric. Ecosyst. Environ. 246, 269–278. doi:10.1016/j.agee.2017.06.011
FAO (2017). Food and agriculture organization of the united nations. Available at: http://www.fao.org/faostat/en/.
Frampton, G., and Çilgi, T. (1994). “Long-term effects of pesticides on carabidae in UK farmland: Some initial results from the “SCARAB” project,” in Carabid beetles: Ecology and evolution. Editors K. Desender, M. Dufrêne, M. Loreau, M. L. Luff, and J. Maelfait (Dordrecht: Springer), 433–438.
Fukuoka, M. (2010). The one-straw revolution: an introduction to natural farming. New York US: New York Review of Books. Review Books Classics.
Geier, B. (2007). “IFOAM and the history of the international organic movement,” in Organic farming: an international history. Editors W. Lockeretz (United Kingdom: Centre for Agriculture and Biosciences International), 175–186.
Gibbs, K. E., Mackey, R. L., and Currie, D. J. (2009). Human land use agriculture pesticides and losses of imperiled species. Divers. Distributions 15, 242–253. doi:10.1111/j.1472-4642.2008.00543.x
Graf, N., Battes, K. P., Cimpean, M., Dittrich, P., Entling, M. H., Link, M., et al. (2019). Do agricultural pesticides in streams influence riparian spiders? Sci. Total Environ. 660, 126–135. doi:10.1016/j.scitotenv.2018.12.370
Haan, N. L., Zhang, Y., and Landis, D. A. (2019). Predicting landscape configuration effects on agricultural pest suppression. Trends Ecol. Evol. 35, 175–186. doi:10.1016/j.tree.2019.10.003
Hammer, Ø., Harper, D., and Ryan, P. (2001). Past: paleontological statistics software package for education and data analysis. Available at: https://palaeoelectronica.org/2001_1/past/issue1_01.htm.
Harwood, R. R. (1990). “A history of sustainable agriculture,” in Sustainable agricultural systems. Editors C. A. Edwards, R. Lal, P. Madden, R. H. Miller, and G. House (US: Soil and Water Conservation Society), 3–19.
Hendrickx, F., Maelfait, J. P., Wingerden, W. V., Schweiger, O., Speelmans, M., Aviron, S., et al. (2007). How landscape structure, land-use intensity and habitat diversity affect components of total arthropod diversity in agricultural landscapes. J. Appl. Ecol. 44, 340–351. doi:10.1111/j.1365-2664.2006.01270.x
Heong, K., Aquino, G., and Barrion, A. (1991). Arthropod community structures of rice ecosystems in the Philippines. Bull. Entomological Res. 81, 407–416. doi:10.1017/s0007485300031977
Holland, J. M., Bianchi, F. J., Entling, M. H., Moonen, A. C., Smith, B. M., and Jeanneret, P. (2016). Structure, function and management of semi-natural habitats for conservation biological control: a review of European studies. Pest Manag. Sci. 72, 1638–1651. doi:10.1002/ps.4318
Hook, D. D. (1993). Wetlands: history, current status, and future. Environ. Toxicol. Chem. Int. J. 12, 2157–2166. doi:10.1002/etc.5620121202
Ishii-Eiteman, M. J., and Power, A. G. (1997). Reponse of green rice leafhoppers to rice-planting practices in northern Thailand. Ecol. Appl. 7, 194–208. doi:10.1890/1051-0761(1997)007[0194:rogrlt]2.0.co;2
Jacob, H. S., Joder, A., and Batchelor, K. L. (2006). Biology of Stethynium sp. (Hymenoptera: mymaridae), a native parasitoid of an introduced weed biological control agent. Environ. Entomol. 35, 630–636. doi:10.1603/0046-225x-35.3.630
Landis, D. A. (2017). Designing agricultural landscapes for biodiversity-based ecosystem services. Basic Appl. Ecol. 18, 1–12. doi:10.1016/j.baae.2016.07.005
Marie, A., Mangenot, A., Puech, C., Aviron, S., Plantegenest, M., Pétillon, J., et al. (2018). Farming system and landscape characteristics differentially affect two dominant taxa of predatory arthropods. Agric. Ecosyst. Environ. 259, 98–110. doi:10.1016/j.agee.2018.02.031
McNeely, J. A., and Scherr, S. J. (2003). Ecoagriculture: strategies to feed the world and save wild biodiversity. US: Island Press.
Mitchell, M. G., Bennett, E. M., and Gonzalez, A. (2014). Agricultural landscape structure affects arthropod diversity and arthropod-derived ecosystem services. Agric. Ecosyst. Environ. 192, 144–151. doi:10.1016/j.agee.2014.04.015
Miyashita, T., Chishiki, Y., and Takagi, S. R. (2012). Landscape heterogeneity at multiple spatial scales enhances spider species richness in an agricultural landscape. Popul. Ecol. 54, 573–581. doi:10.1007/s10144-012-0329-2
Moreby, S., Aebischer, N., Southway, S., and Sotherton, N. (1994). A comparison of the flora and arthropod fauna of organically and conventionally grown winter wheat in southern England. Ann. Appl. Biol. 125, 13–27. doi:10.1111/j.1744-7348.1994.tb04942.x
Morrison, W. R., Waller, J. T., Brayshaw, A. C., Hyman, D. A., Johnson, M. R., and Fraser, A. M. (2018). Evaluating multiple arthropod taxa as indicators of invertebrate diversity in old fields. Gt. Lakes. Entomologist 45, 7.
Mutitu, E. K., Garnas, J. R., Hurley, B. P., Wingfield, M. J., Harney, M., Bush, S. J., et al. (2013). Biology and rearing of Cleruchoides noackae (Hymenoptera: mymaridae), an egg parasitoid for the biological control of Thaumastocoris peregrinus (Hemiptera: thaumastocoridae). J. Econ. Entomology 106, 1979–1985. doi:10.1603/ec13135
Pinheiro, J., Bates, D., DebRoy, S., Sarker, D., and Team, R. C. (2014). nlme: linear and nonlinear mixed effects models. R package version 3.1-118. Vienna, Austria: R Project for Statistical Computing.
Roitberg, B. D. (2018). “Chemical communication,” in Insect behavior: from mechanisms to ecological and evolutionary consequences. Editors A. Córdoba-Aguilar, D. González-Tokman, and I. González-Santoyo (UK: Oxford University Press), 145.
Rusch, A., Valantin-Morison, M., Sarthou, J. P., and Roger-Estrade, J. (2010). “Biological control of insect pests in agroecosystems: Effects of crop management, farming systems, and seminatural habitats at the landscape scale: a review,” in Advances in agronomy. Editor D. L. Sparks (Amsterdam: Elsevier), 219–259.
Russell, M. C., Lambrinos, J., Records, E., and Ellen, G. (2017). Seasonal shifts in ground beetle (Coleoptera: carabidae) species and functional composition maintain prey consumption in Western Oregon agricultural landscapes. Biol. Control 106, 54–63. doi:10.1016/j.biocontrol.2016.12.008
Settle, W. H., Ariawan, A., Astuti, E. T., Cahyana, W., Hakim, A. L., Hindayana, D., et al. (1996). Managing tropical rice pests through conservation of generalist natural enemies and alternative prey. Ecology 77, 1975–1988. doi:10.2307/2265694
Stevens, M., Helliwell, S., and Cranston, P. (2006). Larval chironomid communities (Diptera: chironomidae) associated with establishing rice crops in southern New South Wales, Australia. Hydrobiologia 556, 317–325. doi:10.1007/s10750-005-1072-x
Tsutsui, M. H., Tanaka, K., Baba, Y. G., and Miyashita, T. (2016). Spatio-temporal dynamics of generalist predators (Tetragnatha spider) in environmentally friendly paddy fields. Appl. Entomology Zoology 51, 631–640. doi:10.1007/s13355-016-0440-5
Tuck, S. L., Winqvist, C., Mota, F., Ahnström, J., Turnbull, L. A., and Bengtsson, J. (2014). Land-use intensity and the effects of organic farming on biodiversity: a hierarchical meta-analysis. J. Appl. Ecol. 51, 746–755. doi:10.1111/1365-2664.12219
Ueno, T. (2012). Insect natural enemies as bioindicators in rice paddies. Korean J. Agric. Sci. 39, 545–553. doi:10.7744/cnujas.2012.39.4.545
Verhagen, W., Van Teeffelen, A. J., Compagnucci, A. B., Poggio, L., Gimona, A., and Verburg, P. H. (2016). Effects of landscape configuration on mapping ecosystem service capacity: a review of evidence and a case study in scotland. Landsc. Ecol. 31, 1457–1479. doi:10.1007/s10980-016-0345-2
Keywords: paddy field, sustainable farming, conventional farming, diversity, functional group
Citation: Chen J-H, Liao Y-C, Chiu M-C and Yang M-M (2023) Arthropod communities of rice agroecosystems can be shaped both by local agricultural practices and the surrounding landscape. Front. Environ. Sci. 11:1221247. doi: 10.3389/fenvs.2023.1221247
Received: 15 May 2023; Accepted: 08 August 2023;
Published: 25 August 2023.
Edited by:
Jhih-Rong Liao, Tokyo Metropolitan University, JapanReviewed by:
Hauchuan Liao, National Chung Hsing University, TaiwanFeng-Chuan Hsu, National Taiwan University, Taiwan
Copyright © 2023 Chen, Liao, Chiu and Yang. This is an open-access article distributed under the terms of the Creative Commons Attribution License (CC BY). The use, distribution or reproduction in other forums is permitted, provided the original author(s) and the copyright owner(s) are credited and that the original publication in this journal is cited, in accordance with accepted academic practice. No use, distribution or reproduction is permitted which does not comply with these terms.
*Correspondence: Yi-Chang Liao, ycliaopsyllids@gmail.com