- 1Environmental and Biochemical Sciences Department, The James Hutton Institute, Aberdeen, United Kingdom
- 2Lancaster Environment Centre, Lancaster University, Lancaster, United Kingdom
- 3Department of Geography and Planning, School of Environmental Sciences, University of Liverpool, Liverpool, United Kingdom
- 4Department of Forest Ecology and Management, Swedish University of Agricultural Sciences, Uppsala, Sweden
- 5Department of Continental Ecology, Centre d’Estudis Avançats de Blanes (CEAB-CSIC), Girona, Spain
- 6Helmholtz-Centre for Environmental Research UFZ, Department of Hydrogeology, Leipzig, Germany
Riparian zones are critical interfaces to freshwater systems, acting as gateways for the conveyance and modification of macronutrient fluxes from land to rivers and oceans. In this paper, we propose that certain riparian conditions and processes (conceptually ‘Riparian Reactive Interfaces’) may be susceptible to environmental change with consequences of accelerating local nutrient cycling cascading to global impacts on the cycles of carbon (C), nitrogen (N), and phosphorus (P). However, we argue that this concept is insufficiently understood and that research has not yet established robust baseline data to predict and measure change at the key riparian ecosystem interface. We suggest one contributing factor as lack of interdisciplinary study of abiotic and biotic processes linking C, N, and P dynamics and another being emphasis on riparian ecology and restoration that limits frameworks for handling and scaling topography–soil–water–climate physical and biogeochemical observations from plot to large catchment scales. Scientific effort is required now to evaluate riparian current and future controls on global nutrient cycles through multi-nutrient (and controlling element) studies, grounded in landscape frameworks for dynamic riparian behaviour variation, facilitating scaling to catchment predictions.
1 Introduction
Freshwaters as conveyers of particulate and dissolved mass loads from land to oceans are a key component of global nutrient cycles. A decade ago, the ‘active pipe’ hypothesis by Cole et al. (2007) transformed the view of freshwaters also as reactors, transforming nutrients between environmental compartments (water–air, water–sediment, and physicochemical to biota) informing the debate on global carbon cycles. Both conveyance and transformations of nutrients are spatially and temporally dynamic, under considerable change pressures through superimposed land-use and climate change and, hence, highly topical. With respect to this, it is vital to consider that the freshwater continuum extends beyond the channel network into adjacent soils, floodplains, and wetlands (Abril and Borges, 2019) and to understand the pivotal and potential changing, processing roles for C, N, and P attributed to freshwater riparian zones.
Yet, our understanding of riparian zones in transforming nutrient cycles is lacking (Krause et al., 2017). Their importance may be likened to the role of the rhizosphere, a key ecotone in plant–soil domains. The main roots (cf. rivers) are relatively fast conduit pathways across the soil (landscapes), the fine root structures (streams) intimately connect with the adjacent rhizosphere (riparian zones, inclusive of hyporheic zones) that potentially and substantially magnify and modify explored soil and sediment volumes, residence times, and biogeochemical processing potential. Riparian interfaces are unique units at landscape scales because of the two-way exchanges of water, solutes, particulates, and energy with river channels (Covino, 2017). Recent upward revised estimates show global rivers occupy 0.58% of Earth’s non-glaciated surface area (Allen and Pavelsky, 2018). European estimates suggest 2% land coverage of riparian zones (Clerici et al., 2011). However, their limited local extent masks vastly expanded areas of drainage (both hillslope runoff and overbank river flow from up-catchment) passing through them. We propose the concept of reactive riparian interface (RRI) as having distinct physicochemical processes from the wider landscape and operating as a dynamic ‘gatekeeper’ of land–freshwater–ocean transfers, crucially affecting C, N, and P exports and ecosystem interactions.
2 Interdisciplinary study is needed spanning carbon, nitrogen, and phosphorus riparian processes
To understand change at the RRI within landscape spatio-temporal frameworks (e.g., catchments) necessitates a further interface, one of bringing expertise together across soil, biogeochemical, ecological, climate sciences (prediction and effects), geomorphological, and hydrological disciplines. Longstanding interest in ecological functions and restoration dominates riparian science (Riis et al., 2020). Figure 1 is a visualisation of the most prominent themes (clusters) and interdisciplinary links within over 8,000 publications screened on the topic of ‘riparian’ and at least one of ‘carbon’, ‘nitrogen’, and ‘phosphorus’ over the last 30 years. While a lot of research studies have been conducted within specific themes (soil phosphorus, especially management of diffuse pollution mitigation zones, soil biodiversity and quality, water and nutrients, and greenhouse gases), almost no links can be found in between the clusters. This constitutes an interdisciplinary gap for biogeochemical understanding across linked C, N, and P processes. It can be seen that factors of importance to our arguments here (e.g., wetland, soil profile, water table depth, hydrological functioning, drought, and climate change) appear small and/or unconnected in the sparse centre. Holistic studies are required into process connections across C, N, and P cycles and with additional element cycles mechanistically related to change (e.g., the role of Fe in sorption and redox). This is especially true given advances in compositional analysis of organic matter that allow the study of functional pools of C, N, and P related to ecosystem processes, reactivity, and sensitivities to key emerging transformational factors such as oxygen conditions (Lau and del Giorgio, 2020).
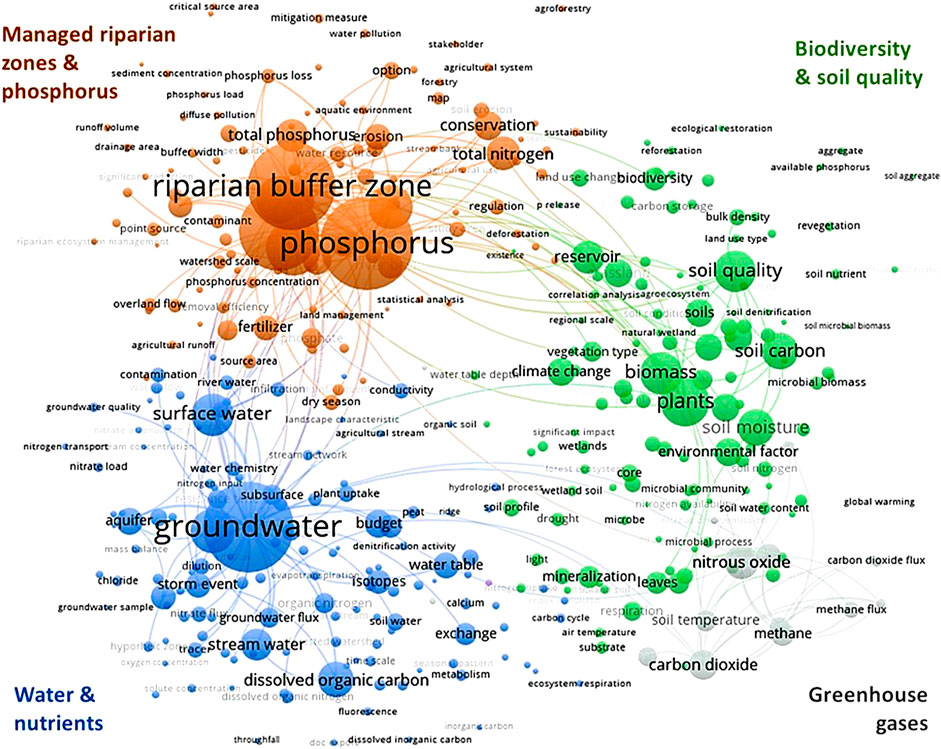
FIGURE 1. Intersectoral gap in riparian-related research generated with VOSviewer and based on 8,000 publications using the keywords “riparian, carbon,” “riparian, nitrogen,” and “riparian, phosphorus.” Each circle corresponds to a keyword found in the articles title and abstracts and is linked by a line if it co-occurs frequently in articles. The size of the circles corresponds to the number of occurrences of a keyword. Lines have been reduced by 50% for better visual clarity.
3 Spatial frameworks are required to represent functions and change sensitivity towards effecting scenario modelling across scales
Our RRI concept recognises the potential for an inherently high degree of spatio-temporal variation that is not yet sufficiently investigated through the necessary interdisciplinary study. Key variability concerns aspects of nutrient stocks, fluxes, passive or reactive conveyance and the sensitivity of these to climate and land change that separates (re)active RRI from wider passive riparian areas (c.f. broader freshwater passive and active pipe theory). Such theory has grounding in ‘hot-spots and hot-moments’ that conceptualized where landscape biogeochemical flux pathways converged in space and time with accumulation zones of complementary reactants (McClain et al., 2003; Bernhardt et al., 2017). Here, we focus this on the riparian interface, but consider holistically linked nutrient cycles and environmental change sensitivity. Our premise is that landscape controls of topography, soil hydrological pathways, geomorphology and related soil moisture and temperature regime, water table, and flooding can be represented in riparian units (RUs). In turn, these dynamic properties control physical and biogeochemical mechanisms governing nutrient stocks (source magnitude), mobilisation, and transport (connectivity to freshwater exports), with varying sensitivity to climate change. Furthermore, the landscape configuration of RU behaviour zones holds the key to representing basin-scale nutrient scenarios and the wider RRI influence on cascading local manifestations of climate change up to larger global nutrient cycles (Krause et al., 2017) where currently, validation and modelling toward scaling aggregate impacts of ‘ecosystem control points’ are found to be lacking (Bernhardt et al., 2017).
Figure 2 is a hypothetical example of how a spatial framework may be informed in practice using primary data to derive relative scales of influence of source-mobilisation-transport-change sensitivity of derived RU in a catchment. Three situations may be contrasted: (a) high importance for accelerating freshwater fluxes of one/more macronutrients resulting from moderate-to-high weightings in all factors (e.g., RU 1, 2; Figure 1), less importance for accelerating fluxes associated with (b) units where a high source term is accompanied by inactive transport or mobilisation and low sensitivity to change (RU 3), or (c) high source-mobilisation-transport terms may result in already high fluxes, but low sensitivity may mean that future change is negligible (RU n).
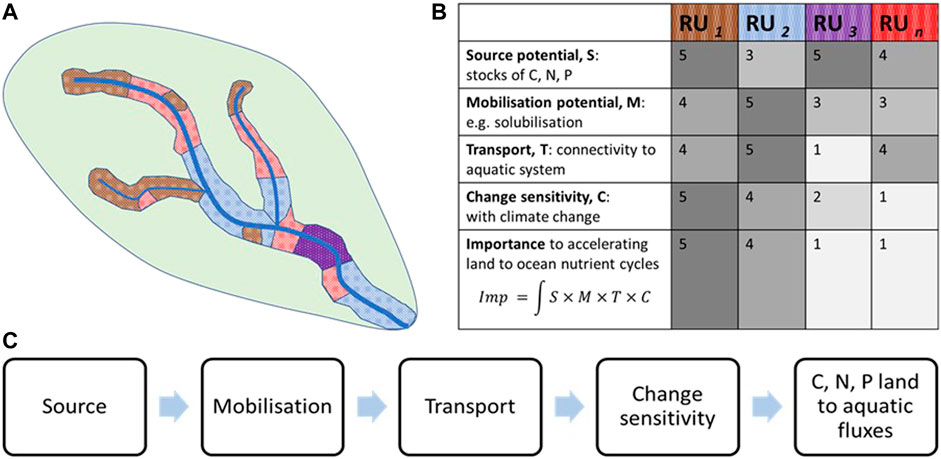
FIGURE 2. Hypothetical example of riparian units (RU 1 to n) used to scale observed riparian properties to catchment nutrient export modelling. (A) Spatial data and monitoring validation informs their landscape orientation, and then, collective unit behaviours in space and time inform catchment models. The conceptual RU differentiation in an example headwater is used to show how RUs may begin to be represented in spatial modelling to aggregate collective impacts. (B) Relative importance of units can be conceptually developed using weightings (or absolute rates informed by experimentation), here represented by a heatmap informing on key factors (see text for explanation) (C) that extend a recognised catchment concept of source-mobilisation-transport by addition of a further factor of sensitivity to climate change.
4 Future outlook and priorities
Rapid progress in riparian observations, data, and knowledge is needed to capture current baseline conditions in climate-sensitive riparian biogeochemical processes and hasten future change comparisons (Gómez-Gerner et al., 2020). To adequately test our arguments, we suggest three interdisciplinary action areas addressing the following:
(1) Landscape variability in stocks, mobilisation, and transport controlling C, N, and P cycling specific to riparian (and directly connected hillslope) zones and processes—using further conceptual development of the RRI critical interface zone, establishing riparian observatories of interdisciplinary study across contrasting landscapes and climate zones.
(2) Sensitivity to climate and environmental change through effects on abiotic and biotic processes destabilising C, N, and P—using experimentation linking laboratory and field-scale manipulations of isolated environmental controls (e.g., soil water-temperature regime), particularly addressing the lack of integration across C, N, and P by a simultaneous spatio-temporal dynamics into catchment modelling and future nutrient export scenarios—extending already developed frameworks for understanding and scaling land to water transfers in the C cycle (Aufdenkampe et al., 2011; Casas-Ruiz et al., 2023) for multi-nutrients; currently, frameworks with potential to facilitate riparian process integration are stronger for C, moderate for N (Pinay et al., 2018), weaker for P (limited to physical processes; Records et al., 2016), and at a starting point for C:N:P integration.
Data availability statement
The original contributions presented in the study are included in the article/supplementary material; further inquiries can be directed to the corresponding author.
Author contributions
All authors participated in the discussion leading to the paper and contributed to the conception and writing of the manuscript. MS took the lead in writing and overall organization of the manuscript. All authors contributed to the article and approved the submitted version.
Funding
MS, NB, JD, ZG, PH, AL, and ST received funding from the Natural Environment Research Council under award NE/V012460/1. Other authors are grateful for institutional support.
Conflict of interest
The authors declare that the research was conducted in the absence of any commercial or financial relationships that could be construed as a potential conflict of interest.
Publisher’s note
All claims expressed in this article are solely those of the authors and do not necessarily represent those of their affiliated organizations, or those of the publisher, the editors, and the reviewers. Any product that may be evaluated in this article, or claim that may be made by its manufacturer, is not guaranteed or endorsed by the publisher.
References
Abril, G., and Borges, A. V. (2019). Ideas and perspectives: Carbon leaks from flooded land: Do we need to replumb the inland water active pipe? Biogeosciences 16, 769–784. doi:10.5194/bg-16-769-2019
Allen, G. H., and Pavelsky, T. M. (2018). Global extent of rivers and streams. Science 361, 585–588. doi:10.1126/science.aat0636
Aufdenkampe, A. K., Mayorga, E., Raymond, P. A., Melack, J. M., Sc, Doney, Alin, S. R., et al. (2011). Riverine coupling of biogeochemical cycles between land, oceans, and atmosphere. Front. Ecol. Environ. 9, 53–60. doi:10.1890/100014
Bernhardt, E. S., Blaszczak, J. R., Ficken, C. D., Fork, M. L., Kaiser, K. E., and Seybold, E. C. (2017). Control points in ecosystems: Moving beyond the hot spot hot moment concept. Ecosystems 20, 665–682. doi:10.1007/s10021-016-0103-y
Casas-Ruiz, J. P., Bodmer, P., Bona, K. A., Butman, D., Couturier, M., Emilson, E. J. S., et al. (2023). Integrating terrestrial and aquatic ecosystems to constrain estimates of land-atmosphere carbon exchange. Nat. Comm. 14, 1571. doi:10.1038/s41467-023-37232-2
Clerici, N., Weissteiner, C., Paracchini, M-L., and Strobl, P. (2011). JRC Scientific and Technical Report 63959. Ispra, Italy: European Union Joint research Centre, Institute for Environment and Sustainability. https://publications.jrc.ec.europa.eu/repository/handle/JRC63959. Riparian zones: Where green and blue networks meet.
Cole, J. J., Prairie, Y. T., Caraco, N. F., McDowell, W. H., Tranvik, L. J., Striegl, R. G., et al. (2007). Plumbing the global carbon cycle: Integrating inland waters into the terrestrial carbon budget. Ecosystems 10, 172–185. doi:10.1007/s10021-006-9013-8
Covino, T. (2017). Hydrologic connectivity as a framework for understanding biogeochemical flux through watersheds and along fluvial networks. Geomorph 277, 133–144. doi:10.1016/j.geomorph.2016.09.030
Gómez-Gerner, L., Lupon, A., Laudon, H., and Sponseller, R. A. (2020). Drought alters the biogeochemistry of boreal stream networks. Nat. Comm. 38. doi:10.1038/s41467-020-15496-2
Krause, S., Lewandowski, J., Grimm, N. B., Hannah, D. M., Pinay, G., McDonald, K., et al. (2017). Ecohydrological interfaces as hot spots of ecosystem processes. Water Resour. Res. 53, 6359–6376. doi:10.1002/2016WR019516
Lau, M. P., and del Giorgio, P. (2020). Reactivity, fate and functional roles of dissolved organic matter in anoxic inland waters. Biol. Lett. 16, 20190694. doi:10.1098/rsbl.2019.0694
McClain, M. E., Boyer, E. W., Dent, C. L., Gergel, S. E., Grimm, N. B., Groffman, P. M., et al. (2003). Biogeochemical hot spots and hot moments at the interface of terrestrial and aquatic ecosystems. Ecosystems 6, 301–312. doi:10.1007/s10021-003-0161-9
Pinay, G., Bernal, S., Abbott, B. W., Lupon, A., Marti, E., Sabater, F., et al. (2018). Riparian corridors: A new conceptual framework for assessing nitrogen buffering across biomes. Front. Environ. Sci. 6, 47. doi:10.3389/fenvs.2018.00047
Records, R. M., Wohl, E., and Arabi, M. (2016). Phosphorus in the river corridor. Earth Sci. Rev. 158, 65–88. doi:10.1016/j.earscirev.2016.04.010
Keywords: riparian, macronutrient, environmental change, soil, landscape, spatio-temporal, global nutrient cycles
Citation: Stutter M, Baggaley NJ, Davies J, Gagkas Z, Janes-Bassett V, Laudon H, Lilly A, Lupon A, Musolff A, Trojahn S and Haygarth PM (2023) The riparian reactive interface: a climate-sensitive gatekeeper of global nutrient cycles. Front. Environ. Sci. 11:1213175. doi: 10.3389/fenvs.2023.1213175
Received: 27 April 2023; Accepted: 30 May 2023;
Published: 12 June 2023.
Edited by:
Dipankar Dwivedi, Berkeley Lab (DOE), United StatesReviewed by:
Merrin Macrae, University of Waterloo, CanadaCopyright © 2023 Stutter, Baggaley, Davies, Gagkas, Janes-Bassett, Laudon, Lilly, Lupon, Musolff, Trojahn and Haygarth. This is an open-access article distributed under the terms of the Creative Commons Attribution License (CC BY). The use, distribution or reproduction in other forums is permitted, provided the original author(s) and the copyright owner(s) are credited and that the original publication in this journal is cited, in accordance with accepted academic practice. No use, distribution or reproduction is permitted which does not comply with these terms.
*Correspondence: Marc Stutter, bWFyYy5zdHV0dGVyQGh1dHRvbi5hYy51aw==