- 1Helmholtz Centre for Environmental Research—UFZ, Department of Analytical Chemistry, Leipzig, Germany
- 2Norwegian Institute for Air Research (NILU), Tromsø, Norway
- 3Aix-Marseille Univ., Toulon Univ., CNRS, IRD, Mediterranean Institute of Oceanography (M I O), UM 110, Marseille, France
- 4Institute for Analytical Chemistry, University of Leipzig, Leipzig, Germany
Tire wear particles (TWPs) represent one of the major anthropogenic pools of particles ending up in the environment. They contain a large variety of chemicals, a part of which may be released into the environment through leaching, although the influence of sunlight and other environmental factors during this process is still unclear. This laboratory study compares the leaching of organic compounds from TWP in seawater in the dark and under artificial sunlight for 1) cryo-milled tire tread (CMTT), 2) ‘virgin’ crumb rubber (VCR) and 3) crumb rubber immersed in the sea for ≥12 months prior to the experiments (WCR). Leachates were analyzed for dissolved organic carbon (DOC) and 19 tire-derived chemicals, benzothiazoles and phenylguanidines as well as phenylendiamines by liquid chromatography-high resolution-mass spectrometry. For DOC and most chemicals, the amounts released decreased in the order CMTT > VCR > WCR and increased when leaching occurred under artificial sunlight. sunlight also led to the formation of 23 transformation processes related to 1,3-diphenylguanidine (DPG). In contrast, 4-hydroxydiphenylamine (4-HDPA) and N-(1,3-dimethylbutyl)-N′-phenyl-p-phenylenediamine quinone (6-PPDQ) were found in lower amounts upon sunlight exposure. The 19 quantified chemicals, however, did only account for 6%–55% of the DOC in the leachates; most of the DOC, thus, remained unexplained. This study highlights that the amount of chemicals leached from tire particles depends upon their aging history and may be modulated by environmental conditions.
1 Introduction
Tire wear particles (TWPs) originate from the abrasive friction between roads and the treads of tires from car, truck, bicycle or e-scooter tires. The particles originating from this process are usually further referred to as tire and road wear particles (TRWP), since they include road materials as well as the original tire material.
Tires are commonly made of styrene-butadiene-rubber (SBR) and butadiene rubber (BR) and can contain high amounts of filling agents, vulcanization agents (e.g., benzotriazoles and benzothiazoles) and other additives, such as zinc (Wagner et al., 2018). Zn has therefore previously been proposed as an elemental marker for the detection of TWP in particulate samples (Klöckner et al., 2019). Currently, a widely used antioxidant, N-(1,3-dimethylbutyl)-N′-phenyl-p-phenylenediamine (6-PPD) and its derivative 6-PPD quinone (6-PPDQ) receive growing attention, since a study reported 6-PPDQ to induce acute mortality in juvenile coho salmon (Oncorhynchus kisutch) (Tian et al., 2021), with a revised LC50 level of 95 ng/L (Tian et al., 2022). Interestingly, a follow-up study confirmed that short-term (<24 h) exposure to TWP leachate was acutely lethal in adult coho salmon, but not in adult chum salmon (Oncorhynchus keta), a closely related species, raising questions concerning the mechanisms behind these inter-species differences (McIntyre et al., 2021). Environmental data on TWP additives are still scarce and laboratory scale experiments have been used to describe the chemical composition of tire leachables (Müller et al., 2022). A study investigating TWP additives in surface waters of an urban tributary in Australia reported a maximum total concentration of 2,760 ng/L for 15 chemicals after a storm event, with 1,3-diphenylguanidine (DPG) being the dominant compound (1,079 ng/L). While a correlation between the presence of SBR, BR and additives was observed, the authors noted that for most of the detected chemicals other sources than tires exist and might have contributed to the observed concentrations (Rauert et al., 2022).
Estimations on the amount of TRWP generated by automotive traffic are available (Baensch-Baltruschat et al., 2020; Wagner et al., 2022), but the percentage to which it reaches the marine environment is not clear, yet. It, certainly, depends on regional aspects such as the distance between urban source regions and the receiving ocean and on their connectivity. The leaching of chemicals from tire particles can be influenced by biotic (biodegradation) and abiotic (e.g., oxidation, mechanical degradation) factors (Wagner et al., 2022). Photodegradation studies on TWP have been performed to investigate the influence of photoaging on both particles and related chemicals (Unice et al., 2015; Fohet et al., 2023). These studies were, however, performed in dry conditions and dedicated studies simulating marine environmental conditions have not been published, yet.
Therefore, our objective was to investigate the leaching behavior of three different TWP materials in natural seawater when exposed to artificial sunlight to simulate processes expected to occur when tire particles reach the marine environment. This study was performed with cryo-milled tire tread (CMTT) and end-of-life tires ground into crumb rubber granulate for use in artificial sports fields.
2 Material and methods
2.1 Chemicals
All chemicals were of analytical grade and used as received without further purification. Methanol, acetonitrile, and formic acid for UPLC-MS analysis were provided by Biosolve (Valkenswaard, Netherlands) whereas ultrapure water was obtained from a Merck MilliQ Integral 5 system (Merck, Darmstadt, Germany). All standards used are listed in Supplementary Table S1, with purity grade and supplier information.
2.2 Artificial sunlight exposure
Surface seawater was collected in the Bay of Marseille (France) in July 2021 using pre-combusted glass bottles, then filtered in the laboratory through a 0.22 μm polyethersulfone (PES) filter pre-rinsed with Milli-Q water and filled into 50 mL quartz tubes containing 1 g/L of the test material; i.e., 50 ± 2 mg per tube. The samples were exposed in laboratory conditions to artificial sunlight using a Suntest CPS + system (ATLAS) equipped with a daylight filter according to (Para et al., 2010). Irradiance intensity was set at 300 W/m2 (300–800 nm) and temperature at 22°C ± 2°C, both representing environmental conditions as encountered in Marseille (France) during summer (reference data from July 2021: monthly mean solar radiation = 298 W/m2, monthly mean surface seawater temperature = 22°C). The quartz tubes were hereby partially submerged in a basin connected to a Minichiller® (Huber Kältemaschinenbau AG) inducing a constant water flow to ensure the maintenance of the desired temperature. In parallel, samples were kept under dark conditions by using the same experimental set-up and temperature, but in complete darkness. At each sampling timepoint, three replicates of UV exposed and dark condition samples were sacrificed, each. Hereby, 10 mL were collected for dissolved organic carbon (DOC) analysis, acidified (20 μL of 85% H3PO4 acid) and stored in glass vials in the dark at 4°C until analysis within a month by using a TOC-5000 total carbon analyzer (Sohrin and Sempéré, 2005). The natural seawater collected at the surface in the Bay of Marseille exhibited a DOC value of 76.8 (±16.4) µM, which is consistent with DOC concentrations previously measured in the same area (72.5–90 µM in summer-fall; Paluselli et al., 2018). This background DOC concentration from the seawater was subtracted from the DOC values observed in the leachates, to further investigate the DOC which derived from the test materials.
The remaining volume was stored in amber glass vials in the dark at 4°C until analysis of organic compounds using UPLC-TOF-MS (see section 2.5). Samples were collected at three sampling timepoints: after (t1) 66 h, (t2) 158 h and (t3) 336 h, representing 6, 14.4 and 30.6 simulated days, respectively. Simulated days were calculated using the following equation from Gewert et al. (2018):
where the total irradiance exposed is the product of the intensity (in W/m2) and the number of hours of exposure (in h) and the European mean irradiance equals 1,200 kWh/m2 per year
2.3 Test material
Three types of test material were used for this study. The “CMTT” comprises a mix of tire tread from 20 different, new tires (see Supplementary Table S2), which were homogenized through a ball milling in cryogenic conditions (Mixer Mill MM 400, Retsch, Germany) and represents small particles as they can be found in road runoff. The size distribution and particle shape images are provided in Supplementary Figure S1. The two other test materials are made of crumb rubber granulate as used in artificial sports fields or playgrounds. The original material (hereafter called “virgin crumb rubber”, VCR) was sourced from a sports field in Tromsø (Norway) prior to application on the field and has previously been used for leaching and toxicity testing (Halsband et al., 2020).
Finally, part of the VCR was exposed in situ to natural seawater in Tromsø for 12–18 months (hereafter called “weathered crumb rubber”, WCR) to create a test material of high environmental relevance. For this, the particles were placed in a cylindric stainless-steel container covered with a mesh and submerged in surface waters (3–4 m depth). The outside of the container was mechanically cleaned at regular intervals to remove algal and bivalve growth and permit the sunlight to transpierce. While the choice of stainless steel limited the exposure of the contained particles to natural sunlight, it was considered as preferable to plastic materials to avoid a chemical contamination of the test material. The particles exposed for 12 and for 18 months were mixed together to have sufficient material to perform the experiment. After recovery from the sea, the WCR was not modified (i.e., no removal of the biofilm or other cleaning), but stored in dry conditions prior to use.
2.4 QA/QC
All glassware was heated (450°C, 6 h) prior to use and covered with burnt aluminum foil. Plastic and rubber materials were avoided at all times. All sample processing steps were conducted in an ISO class 6 clean room equipped for organic trace analysis. Cotton lab coats and nitrile gloves were worn during sample handling. Instrument and water media blank controls performed during the sample analysis were below LOD/LOQ levels or otherwise subtracted from the sample values.
2.5 Analysis with UPLC-TOF-MS
Aqueous samples were analyzed for 19 tire-related chemicals (Supplementary Table S1) by ultra-performance liquid chromatography time-of-flight mass-spectrometry (LC-TOF-MS). The instrument consisted of an ACQUITY UPLC-System (HSS T3 column; 100 × 2.1 mm, 1.7 μm) coupled to a XEVO XS Q-TOF-MS (Waters GmbH, Eschborn, Deutschland) as described elsewhere (Klöckner et al., 2021; Klöckner et al., 2020). A flow rate of 0.45 μL/min, a column temperature of 45°C and a capillary voltage of 0.7 kV (positive ionization mode) were applied. The source temperature was set at 140°C, while the desolvation temperature was set at 550°C. The sampling cone voltage was 20 V and the source offset was 50 V. Nitrogen was used as a cone gas and argon as a collision gas. The desolvation gas flow was set at 950 L/h and the scan time at 0.15 s. A collision energy of 4 eV (molecular ions) and 15–35 eV (fragment analysis) was applied. The mobile phase consisted of A) water with 0.1% formic acid and B) methanol with 0.1% formic acid. The solvent gradient was as follows: 0 min 2% B, 12.25 min 99% B, 15.00 min 99% B, 15.10 min 2% B, 17.00 min 2% B. A volume of 10 µL of the leachates was directly injected into the LC-HRMS system and the first minute was forwarded to waste, not to the MS. The target substances (Supplementary Table S1) were quantified using an external calibration, which was comparable to seawater (checked separately). Therefore, TargetLynx was used with 0.01 Da mass accuracy and 0.1 min accurate retention time and a calibration range from 0.03 to 30 μg/L.
2.6 Identification of transformation products
Identification of transformation products (TPs) was done by evaluating the RPLC-MS data in a retention time window of 1–10 min and a mass range of m/z 50–1,200. MarkerLynx was used to perform the peak picking for the molecular ion trace (function 1) with 0.1 min deviation in retention time and 0.01 Da deviation in exact mass. The marker table with relative intensities (to the total marker intensity) was exported to Excel and all further evaluations were performed there. The peaks with a relative intensity above 1 and present in the leachates compared to controls were selected. Sum formulas were assigned by using a mass tolerance of 5 ppm and an elemental composition of C (0–100), H (0–100), N (0–20), O (0–20), S (0–3) and Na (0–2), and by taking into account the fragment ions. The fragment ions were used, if available, to assign the elemental composition. For DPG related TPs the fragments were screened for those containing m/z 212.118 (C13H14N3), 195.0922 (C13H11N2), 131.0599 (C8H7N2) and/or 119.06 (C7H7N2), 106.0648 (C7H8N). DCH related TPs were identified by having the fragment m/z 180.1755 (C12H22N). The integration of the areas was done in TargetLynx.
3 Results and discussion
3.1 Release of dissolved organic carbon
All three test materials released DOC into the seawater in the range of 20–500 μg/g within 14 days (Figure 1). Under dark conditions CMTT released the highest amount of organic carbon (140 μg/g; Figure 1A), followed by WCR and VCR (23–28 μg/g). The stronger DOC release from CMTT may in part be due to a higher content of low molecular weight additives in that material (see section 3.2) and its significantly lower mean particle size (180 µm) compared to VCR and WCR (3 mm). A higher DOC concentration (316 μg/g) was previously reported for a freshly pulverized crumb rubber after 3 days of leaching, after which the concentration decreased (Selbes et al., 2015). The authors suggested that freshly generated particles would release more DOC; this may explain their higher values compared to the crumb rubber in this study.
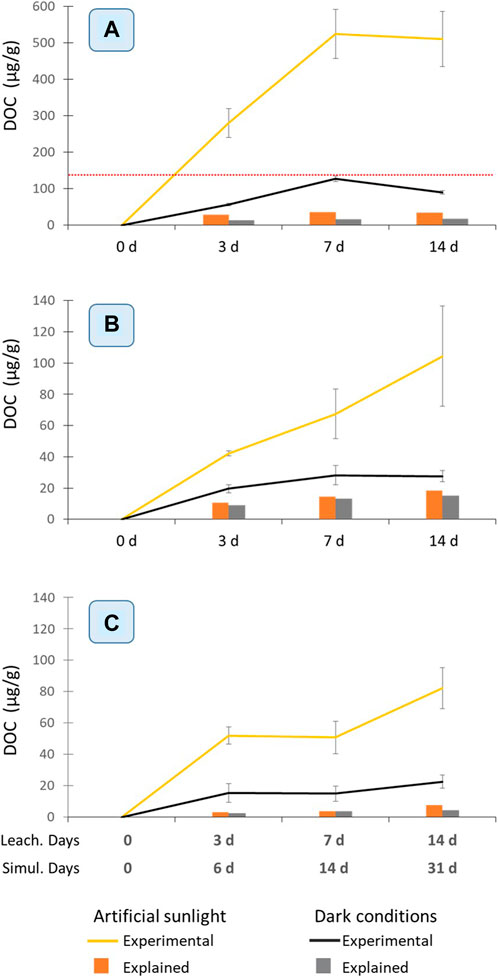
FIGURE 1. DOC release from (A) CMTT (B) VCR and (C) WCR exposed to artificial sunlight (yellow lines) and dark conditions (black lines) over 14 days. This time corresponds to a simulated sunlight exposure of 31 days. The red dotted line in (A) indicates the y-axis limit used in graphs (B) and (C) for better comparison. Error bars indicate the standard deviation (n = 3) of independent samples. The columns report the amount of DOC in the artificial sunlight exposed samples (orange columns) and in dark conditions (grey columns), which can be explained by the quantified organic compounds.
It is noteworthy that the VCR released only slightly higher amounts of organic carbon (20%–25%) than the same material after being exposed to marine water for about 1 year. This suggests that the pool of organic compounds that can be released from crumb rubber is large and is not much depleted within 1 year. The release rate of organic compounds from the rubber into the surrounding water is determined by the (low) diffusion speed of these compounds from the interior of the particles towards the surface (Huntink, 2003). A part of the organic matter released from WCR may, however, also be natural organic matter adsorbed to it during the exposure period.
For CMTT there is a slight decrease in average DOC concentrations from day 7 to day 14 (Figure 1A), which may reflect the onset of biodegradation of some of the organic chemicals released from the material, as the experiments were not run under sterile conditions.
For all three materials parallel exposure to artificial sunlight drastically increased the release of organic matter over 14 days by a factor of 3–5 compared to the dark conditions (Figure 1.). Several hypotheses might be made to explain this observation: 1) The UV exposure could induce an aging and loosening of the rubber matrix, which would increase its accessibility for water and speed up leaching of organic compounds. 2) A sunlight induced transformation of tire constituents with a relatively lower tendency for leaching into TPs of lower molecular weight and higher polarity, with a stronger leaching tendency might occur. 3) While the temperature within the irradiation chamber was controlled (22°C ± 2°C), the black color of the particles might have led to a higher temperature of the particles themselves exposed to artificial sunlight, supporting the leaching compared to the dark conditions. A combination of these aspects as well as other factors may be responsible for the enhancing effect of sunlight exposure.
While the leaching of chemicals from tire material has been studied (Halsband et al., 2020; Tian et al., 2021; Jeong et al., 2022; Müller et al., 2022), the total amount of organic matter measured as DOC has been reported so far only for crumb rubber (Selbes et al., 2015) but not for a smaller dimensional size range of tire particles.
3.2 Leachate composition
A total of 19 tire-related chemicals were quantified in the leachates based on LC-HRMS analysis (Supplementary Table S1). Among them were the vulcanization accelerators 2-mercaptobenzothiazole (2-MBT), benzothiazole (BT), benzothiazole-2-sulfonic acid (BTSA), 2-hydroxybenzothiazole (2-OHBT), 1,3-diphenylguanidine (DPG) and its degradation product phenylguanidine (PG), and dicyclohexylamine (DCH), as well as the antioxidant N-(1,3-dimethylbutyl)-N′-phenyl-p-phenylenediamine (6-PPD) and its hydrolysis and oxidation products 4-hydroxydiphenylamine (4-HDPA) and 6-PPD quinone (6-PPDQ), respectively.
3.2.1 Vulcanization accelerators
Benzothiazoles were the most abundant substances found in the leachates (from 58 to 342 μg/g and from 111 to 589 μg/g in dark and sunlight exposed conditions, respectively). 2-MBT is a prominent transformation product of the vulcanization accelerators found in tires. Similar amounts were released from the three materials, even after 1 year of exposure to marine waters (WCR). This suggests that the reservoir of 2-MBT in the materials is large enough not to be exhausted in this period of time. For all three materials 2-MBT leaching was lower during sunlight exposure, likely due to direct or indirect photodegradation of 2-MBT (Zajíčková and Párkányi, 2008). This photodegradation may have occurred either on the particle surface before 2-MBT was released or in solution after its release.
BT, BTSA and 2-OHBT all are putative transformation products of MBT. For BT and BTSA, the leached amounts were significantly higher from CMTT, compared to VCR and WCR, especially under sunlight exposure (CMTT 160–350 μg/g, VCR and WCR 6–50 μg/g). CMTT with its much smaller particle size offers more surface per mass. This enhances both, release of chemicals from the surface as well as transformation reactions on the surface. And it might explain why much higher amounts of BT and BTSA were detected in CMTT samples exposed to artificial sunlight (Figures 2B,C), even though the amount of the parent compound, 2-MBT, in solution under dark conditions was even lower than for VCR and WCR samples (Figure 2A).
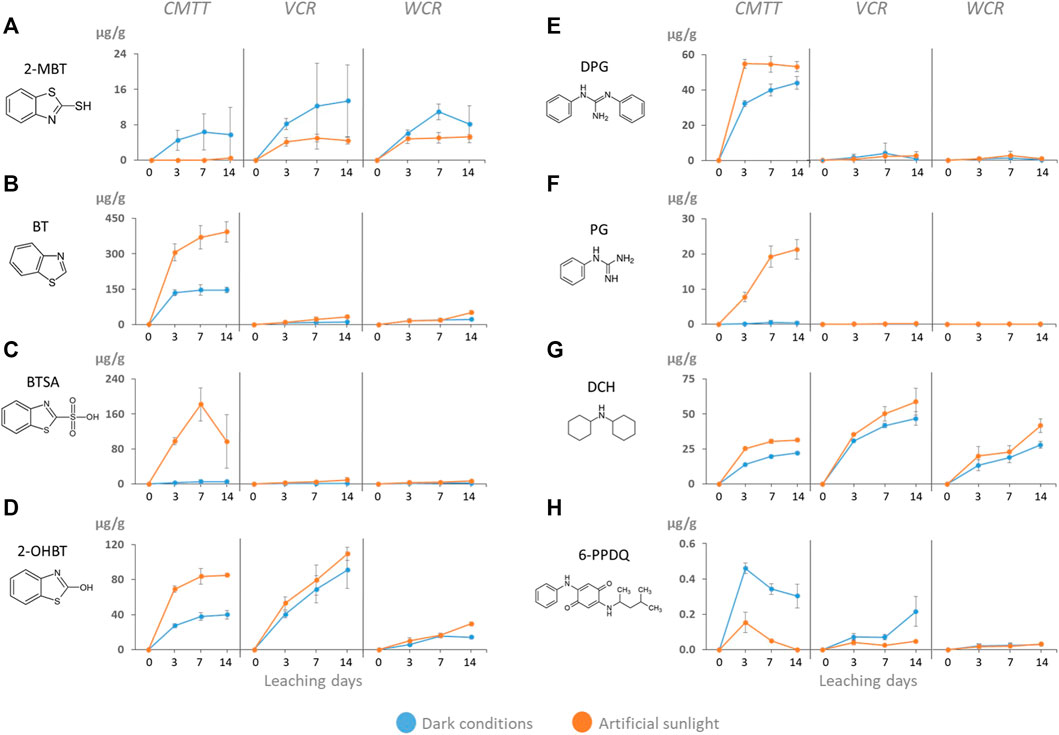
FIGURE 2. Amounts of (A) 2-mercaptobenzothiazole (2-MBT), (B) benzothiazole (BT), (C) benzothiazole-2-sulfonic acid (BTSA), (D) 2-hydroxybenzothiazole (2-OHBT), (E) 1,3-diphenylguanidine (DPG), (F) phenylguanidine (PG), (G) dicyclohexylamine (DCH), (H) 6-PPD quinone (6-PPDQ) released from CMTT, VCR and WCR by leaching over 14 days in the dark (blue lines) and under artificial sunlight (orange lines). Error bars represent standard deviation (n = 3) of independent replicates.
BT was also detected in 14-day leachates of crumb rubber in seawater, at concentrations of 80 ± 46 μg/g (Halsband et al., 2020) when considering the same crumb rubber concentration as used in our study (1 g/L). This is comparable to our results for VCR and WCR after 14 days of leaching (11–50 μg/g, depending on the conditions).
Furthermore, 2-aminobenzothiazole (NH2-BT) was determined in the leachates (0.2–2 μg/g). As for BTSA and BT, sunlight exposure increased the release of NH2-BT from CMTT more strongly than from VCR and WCR (Supplementary Figure S2).
For 2-OHBT the difference between CMTT and the other materials is less strong (WCR) or even absent (VCR); also, the difference between dark and light leaching conditions is less pronounced (Figure 2D). This, likely, indicates that the formation of 2-OHBT occurred inside the tire particles and is only partially due to sunlight exposure during the leaching. The amount of 2-OHBT released from WCR was approximately 20% of that released from the VCR material (Figure 2D). It appears that a significant proportion of 2-OHBT leached out of the WCR during the 1 year of weathering in marine waters. Such a strong difference between WCR and VCR was not visible for BT and BTSA, supporting the assumption that they are formed by different processes than 2-OHBT. On a molar basis, the enhancing effect of sunlight exposure, however, was equally strong for VCR (+118 nM) and WCR (+100 nM) probably due to their similar particle size range, while it was three times higher for CMTT (+300 nM).1,3-diphenylguanidine (DPG) is another curing agent and a prominent chemical in tire rubber and tire leachates (Schulze et al., 2019; Zahn et al., 2019; Johannessen et al., 2021; Müller et al., 2022). This was visible for CMTT with 40–50 μg/g being released under dark and sunlight conditions (Figure 2E). Release of DPG from VCR and WCR was one order of magnitude lower, with 3–4 μg/g. The transformation of DPG to PG is a hydrolytic process. Nevertheless, sunlight exposure appears to support this transformation, as the PG concentration in the CMTT leachate continuously increased from 0.3 to 21 μg/g in artificial sunlight conditions (Figure 2E). Besides PG, a large number of transformation products have previously been reported to occur in tire leachates (Müller et al., 2022), Fohet et al. (2023) reported two DPG TPs, one being more stable (C19H23N3, 13 days) and the other less stable (C19H23N3O, 4 days) than DPG (8 days) under photoaging conditions.
To search for additional DPG related TPs a screening was performed in the LC-HRMS data of the CMTT leachates based on characteristic fragment ions (see section 2.6). In total, 23 putative TPs were detected in the leachate after 14 days. Just one of these TPs (DPG + C3H3O2) corresponds to those reported by (Müller et al., 2022); all the others have not been reported before. Among others, five isomers with the formula DPG + C2H2O2 and three isomers corresponding to DPG + C5H6O2 were detected (Figure 3; details provided in the Supplementary Table S4). Such sets of isomers point at their formation by radical processes, as those are often of limited regio-selectivity. It is not clear, if these 23 TPs were formed directly from DPG, or if they were formed from DPG related transformation products already present in the tire material. It has been shown that only 50% of the initial amount of DPG in a starting material may remain unaltered after rubber curing (Unice et al., 2015). Artificial sunlight strongly supported the formation of these TPs, as they were hardly detectable under dark conditions (Figures 3A, B).
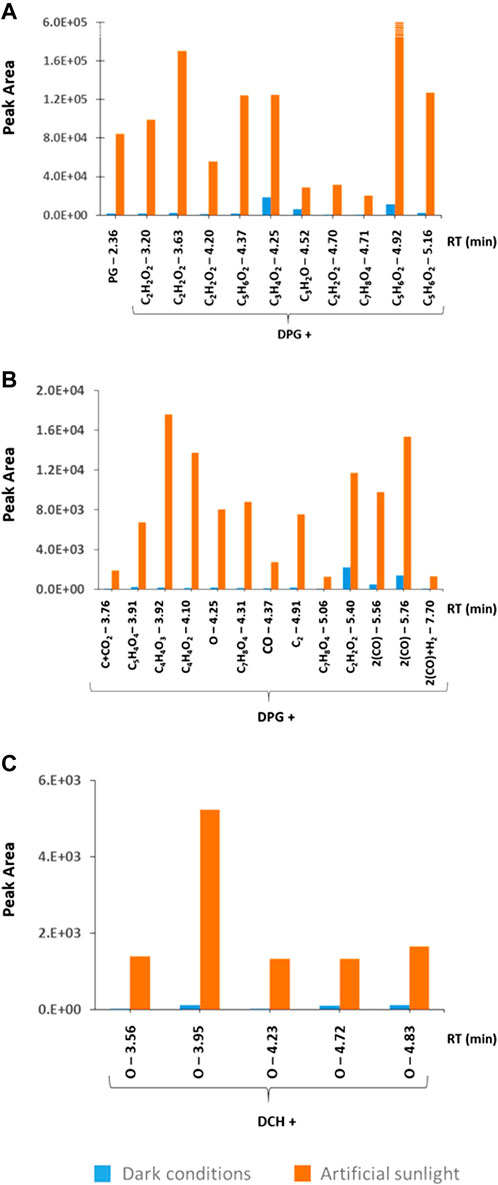
FIGURE 3. Peak areas of DPG transformation products (TPs) detected in the leachates of CMTT after 14 days (A) at high intensity and (B) low intensity, and (C) TPs of DCH. The peak intensities in the artificial sunlight exposed samples (orange color) are compared with the dark condition samples (blue color), highlighting the impact of UV irradiance on the formation of TPs from the considered parent compounds.
DCH was the amine with the highest intensity found in all the leachates and is suspected to be formed by hydrolysis from different vulcanization accelerators, such as N,N-dicyclohexylbenzothiazole-2-sulphenamide (Seiwert et al., 2020). An amount of 20–50 μg/g was released from the three tested materials (Figure 2G). The lack of a difference between the three materials is unexpected. However, the concentration of a chemical detected in the leachate is not only a consequence of its concentration in the test material, but governed by several parallel processes such as the migration from the particles interior to its surface, the release from the particles into solution, the formation of precursor compounds and its transformation to TPs, either in the particles or in solution. For all three materials, artificial sunlight led to slightly increasing concentrations of DCH of approx. 25% (Figure 2G). Furthermore, a screening for DCH TPs was performed in the LC-HRMS data. Indeed, five isomeric TPs were detected in the sunlit leachate, which all carried an additional hydroxy group (Figure 3C). These TPs were hardly detected in the dark condition samples.
Another chemical often reported is hexamethoxymethylmelamine (HMMM) (Alhelou et al., 2019). It was detected in all leachates (0.5–1.8 μg/g), exhibiting a relatively stable behavior in all samples (CMTT, VCR and WCR), with comparable concentrations both in dark and artificial sunlight conditions (Supplementary Table S3).
3.2.2 Antioxidants
Among the antioxidant compounds detected, 4-hydroxydiphenylamine (4-HDPA), a hydrolysis product of 6-PPD (Unice et al., 2015), was most abundant, with higher amounts released from CMTT than from VCR and WCR (Supplementary Figure S1). Since 6-PPD and diphenylamine react rapidly with reactive oxygen species and as 4-HDPA is also reactive (Seiwert et al., 2022), the 4-HDPA concentrations in the CMTT leachates under sunlight exposure were extremely low, with near-zero concentration values during the whole experiment.
The 6-PPD oxidation product 6-PPDQ (Tian et al., 2021) was detected in all leachates, with the amounts following the order CMTT (0.4 μg/g) > VCR (0.2 μg/g) > WCR (0.03 μg/g) (Figure 2H). The concentrations were always lower in the samples exposed to artificial sunlight, according to the reactivity of 6-PPDQ towards oxidative species. However, 6-PPDQ quantification was compromised by its adsorption to the PES membrane filters (>50%) used during the experimental procedure (Supplementary Figure S3). This data therefore needs to be considered with caution.
3.3 Contributions of target compounds to DOC
For the 19 tire related chemicals quantified in the leachates (Supplementary Table S1), their contribution to the DOC amount was calculated, grouped into vulcanization accelerators (15 compounds, including thiazoles and guanidines) and antioxidants (4 phenylenediamine compounds) (Table 1). For all three tire materials, the explainable DOC is always significantly higher for the leachate generated in the dark than under sunlight exposure. This corresponds to the much higher DOC in the leachate with artificial sunlight. Obviously, sunlight exposure leads to the release of larger amounts of yet unknown compounds. sunlight exposure transforms parent chemicals into TPs, many of which are either unknown or not available as reference compounds and are, therefore, not considered in this balance. A typical example for this are the 23 TPs of DPG, which were detected by LC-HRMS screening in the sunlit CMTT leachate, but could not be quantified (Figure 3, Supplementary Table S4). The oxidative transformation of 6-PPD has been shown to lead to an even larger number of TPs (Seiwert et al., 2022), three of which are available as standards and have been included in this study.
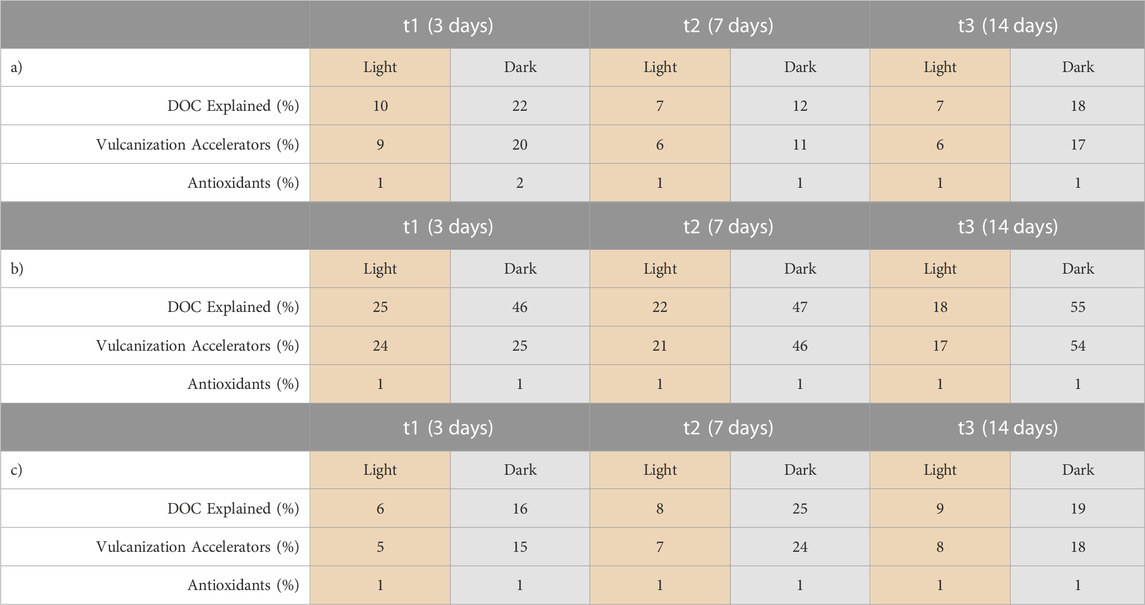
TABLE 1. Percentages of the DOC contributions which can be explained by the quantified compounds under artificial sunlight (light orange) and dark conditions (grey) by CMTT a), VCR b) and WCR c).
In all three tire materials, vulcanization accelerators played a predominant role in the explainable DOC. On the contrary, antioxidants explain only 1% of DOC in most cases. This is not only because of the lower number of quantified antioxidant compounds, but also due to the fast degradability of antioxidants through hydrolysis and oxidation, which may lead to an extremely high number of possible TPs (Seiwert et al., 2022).
For the CMTT leachates (Table 1), the explainable DOC during the UV exposure was 10% in the first timepoint but then decreased to 7% as the amount of DOC significantly increased. In dark conditions, the explainable DOC corresponds to almost the double compared to the UV exposed samples. While the smaller particle size of the CMTT favors the release of all chemicals compared to the coarser VCR and WCR material, this effect can be expected to be stronger for chemicals with a lower diffusion coefficient, i.e., compounds of higher molecular weight, e.g., oligomers of HMMM and poly (1,2-dihydro-2,2,4-trimethylquinoline) (TMQ). These compounds were not included in the target list, which may explain the lower percentage of explained DOC for CMTT.
The WCR material, which has been weathered in marine water for about 1 year, may additionally release natural organic matter into the leachate, which was taken up during this long-time exposure to seawater. As a consequence of these different trends, the highest percentage of “explainable” DOC was obtained for VCR in the dark (55%) and the lowest percentages for CMTT and WCR under sunlight exposure (7%–8%).
4 Conclusion
This study investigated the leaching of tire materials (CMTT, VCR and WCR) while simulating marine environmental conditions as encountered in Europe during summer. DOC concentrations found in leachates increased from WCR over VCR to CMTT. For all three materials DOC increased significantly upon sunlight exposure, highlighting that sunlight favors the release of organic compounds from tire materials. Likely, particle aging and transformation of chemicals into more soluble products contribute to this enhanced leaching.
Of the 19 tire-derived chemicals that were quantified, BT, BTSA, 2-OHBT, DPG and DCH were released in largest amounts. Exposure of the tire materials to artificial sunlight during leaching increased the release of TPs such as BT, BTSA, and PG and led to the occurrence of 23 TPs of DPG and 5 TPs of DCH in the CMTT leachate, most of which were absent in the dark condition leachate. Accordingly, highly reactive chemicals, such as 2-MBT, 4-HDPA and 6-PPDQ, decreased in concentration. Although a total of 19 tire related chemicals were quantified, the identity of the largest proportion of the organic carbon released from the three tire materials remained unknown.
This study outlines that the nature of chemicals released from tire material depends on the history of the tire particles and the leaching conditions. It indicates that the release of chemicals from larger tire particles, such as crumb rubber (3 mm mean diameter), can be a slow process taking several years.
Data availability statement
The original contributions presented in the study are included in the article/Supplementary Material, further inquiries can be directed to the corresponding author.
Author contributions
AF, NS, DH, RS, and TR contributed to the conception and design of the study. AF and BS performed the chemical analysis. AF and NS wrote the first draft of the manuscript. All authors contributed to the article and approved the submitted version.
Acknowledgments
We thank Catherine Guigue and the PACEM-MIO platform for DOC analysis and Laure Papillon for seawater collection. The research was conducted as part of the JPI Oceans ANDROMEDA project. We thank the French Agence Nationale de Recherche (ANR-19-383 JOCE-0002-01), the German Ministry for Education and Research (BMBF; FKz 03F0850A) and the Norwegian Research Council (311313/E40) for funding.
Conflict of interest
The authors declare that the research was conducted in the absence of any commercial or financial relationships that could be construed as a potential conflict of interest.
Publisher’s note
All claims expressed in this article are solely those of the authors and do not necessarily represent those of their affiliated organizations, or those of the publisher, the editors and the reviewers. Any product that may be evaluated in this article, or claim that may be made by its manufacturer, is not guaranteed or endorsed by the publisher.
Supplementary material
The Supplementary Material for this article can be found online at: https://www.frontiersin.org/articles/10.3389/fenvs.2023.1206449/full#supplementary-material
References
Alhelou, R., Seiwert, B., and Reemtsma, T. (2019). Hexamethoxymethylmelamine – a precursor of persistent and mobile contaminants in municipal wastewater and the water cycle. Water Res. 165, 114973. doi:10.1016/j.watres.2019.114973
Baensch-Baltruschat, B., Kocher, B., Stock, F., and Reifferscheid, G. (2020). Tyre and road wear particles (TRWP) - a review of generation, properties, emissions, human health risk, ecotoxicity, and fate in the environment. Sci. Total Environ. 733, 137823. doi:10.1016/j.scitotenv.2020.137823
Fohet, L., Andanson, J. M., Charbouillot, T., Malosse, L., Leremboure, M., Delor-Jestin, F., et al. (2023). Time-concentration profiles of tire particle additives and transformation products under natural and artificial aging. Sci. Total Environ. 859, 160150. doi:10.1016/j.scitotenv.2022.160150
Gewert, B., Plassmann, M., Sandblom, O., and Macleod, M. (2018). Identification of chain scission products released to water by plastic exposed to ultraviolet light. Environ. Sci. Technol. Lett. 5, 272–276. doi:10.1021/acs.estlett.8b00119
Halsband, C., Sørensen, L., Booth, A. M., and Herzke, D. (2020). Car tire crumb rubber: Does leaching produce a toxic chemical cocktail in coastal marine systems? Front. Environ. Sci. 8, 1–15. doi:10.3389/fenvs.2020.00125
Huntink, N. M. (2003). Durability of rubber products, PhD thesis. Enschede, Netherlands: University of Twente.
Jeong, Y., Lee, S., and Woo, S. H. (2022). Chemical leaching from tire wear particles with various treadwear ratings. Int. J. Environ. Res. Public Health 19, 6006. doi:10.3390/ijerph19106006
Johannessen, C., Helm, P., and Metcalfe, C. D. (2021). Detection of selected tire wear compounds in urban receiving waters. Environ. Pollut. 287, 117659. doi:10.1016/j.envpol.2021.117659
Klöckner, P., Reemtsma, T., Eisentraut, P., Braun, U., Ruhl, A. S., and Wagner, S. (2019). Tire and road wear particles in road environment – quantification and assessment of particle dynamics by Zn determination after density separation. Chemosphere 222, 714–721. doi:10.1016/j.chemosphere.2019.01.176
Klöckner, P., Seiwert, B., Eisentraut, P., Braun, U., Reemtsma, T., and Wagner, S. (2020). Characterization of tire and road wear particles from road runoff indicates highly dynamic particle properties. Water Res. 185, 116262. doi:10.1016/j.watres.2020.116262
Klöckner, P., Seiwert, B., Weyrauch, S., Escher, B. I., Reemtsma, T., and Wagner, S. (2021). Comprehensive characterization of tire and road wear particles in highway tunnel road dust by use of size and density fractionation. Chemosphere 279, 130530. doi:10.1016/j.chemosphere.2021.130530
McIntyre, J. K., Prat, J., Cameron, J., Wetzel, J., Mudrock, E., Peter, K. T., et al. (2021). Treading water: Tire wear particle leachate recreates an urban runoff mortality syndrome in coho but not chum salmon. Environ. Sci. Technol. 55, 11767–11774. doi:10.1021/acs.est.1c03569
Müller, K., Hübner, D., Huppertsberg, S., Knepper, T. P., and Zahn, D. (2022). Probing the chemical complexity of tires: Identification of potential tire-borne water contaminants with high-resolution mass spectrometry. Sci. Total Environ. 802, 149799. doi:10.1016/j.scitotenv.2021.149799
Paluselli, A., Fauvelle, V., Schmidt, N., Galgani, F., Net, S., and Sempéré, R. (2018). Distribution of phthalates in Marseille Bay (NW mediterranean sea). Sci. Total Environ. 621, 578–587. doi:10.1016/j.scitotenv.2017.11.306
Para, J., Coble, P. G., Charrière, B., Tedetti, M., Fontana, C., and Sempéré, R. (2010). Fluorescence and absorption properties of chromophoric dissolved organic matter (CDOM) in coastal surface waters of the northwestern Mediterranean Sea, influence of the Rhône River. Biogeosciences 7, 4083–4103. doi:10.5194/bg-7-4083-2010
Rauert, C., Charlton, N., Okoffo, E. D., Stanton, R. S., Agua, A. R., Pirrung, M. C., et al. (2022). Concentrations of tire additive chemicals and tire road wear particles in an Australian urban tributary. Environ. Sci. Technol. 56, 2421–2431. doi:10.1021/acs.est.1c07451
Schulze, S., Zahn, D., Montes, R., Rodil, R., Quintana, J. B., Knepper, T. P., et al. (2019). Occurrence of emerging persistent and mobile organic contaminants in European water samples. Water Res. 153, 80–90. doi:10.1016/j.watres.2019.01.008
Seiwert, B., Klöckner, P., Wagner, S., and Reemtsma, T. (2020). Source-related smart suspect screening in the aqueous environment: Search for tire-derived persistent and mobile trace organic contaminants in surface waters. Anal. Bioanal. Chem. 412, 4909–4919. doi:10.1007/s00216-020-02653-1
Seiwert, B., Nihemaiti, M., Troussier, M., Weyrauch, S., and Reemtsma, T. (2022). Abiotic oxidative transformation of 6-PPD and 6-PPD quinone from tires and occurrence of their products in snow from urban roads and in municipal wastewater. Water Res. 212, 118122. doi:10.1016/j.watres.2022.118122
Selbes, M., Yilmaz, O., Khan, A. A., and Karanfil, T. (2015). Leaching of DOC, DN, and inorganic constituents from scrap tires. Chemosphere 139, 617–623. doi:10.1016/j.chemosphere.2015.01.042
Sohrin, R., and Sempéré, R. (2005). Seasonal variation in total organic carbon in the northeast Atlantic in 2000-2001. J. Geophys Res. Oceans 110, C10S90–11. doi:10.1029/2004JC002731
Tian, Z., Gonzalez, M., Rideout, C. A., Zhao, H. N., Hu, X., Wetzel, J., et al. (2022). 6PPD-Quinone: Revised toxicity assessment and quantification with a commercial standard. Environ. Sci. Technol. Lett. 9, 140–146. doi:10.1021/acs.estlett.1c00910
Tian, Z., Zhao, H., Peter, K. T., Gonzalez, M., Wetzel, J., Wu, C., et al. (2021). A ubiquitous tire rubber–derived chemical induces acute mortality in coho salmon. Science 371, 185–189. doi:10.1126/science.abd6951
Unice, K. M., Bare, J. L., Kreider, M. L., and Panko, J. M. (2015). Experimental methodology for assessing the environmental fate of organic chemicals in polymer matrices using column leaching studies and OECD 308 water/sediment systems: Application to tire and road wear particles. Sci. Total Environ. 533, 476–487. doi:10.1016/j.scitotenv.2015.06.053
Wagner, S., Hüffer, T., Klöckner, P., Wehrhahn, M., Hofmann, T., and Reemtsma, T. (2018). Tire wear particles in the aquatic environment - a review on generation, analysis, occurrence, fate and effects. Water Res. 139, 83–100. doi:10.1016/j.watres.2018.03.051
Wagner, S., Klöckner, P., and Reemtsma, T. (2022). Aging of tire and road wear particles in terrestrial and freshwater environments – a review on processes, testing, analysis and impact. Chemosphere 288, 132467. doi:10.1016/j.chemosphere.2021.132467
Zahn, D., Mucha, P., Zilles, V., Touffet, A., Gallard, H., Knepper, T. P., et al. (2019). Identification of potentially mobile and persistent transformation products of REACH-registered chemicals and their occurrence in surface waters. Water Res. 150, 86–96. doi:10.1016/j.watres.2018.11.042
Keywords: photodegradation, transformation products (TPs), ocean, automobile, additives, antioxidants, vulcanization accelerators, plastic
Citation: Foscari A, Schmidt N, Seiwert B, Herzke D, Sempéré R and Reemtsma T (2023) Leaching of chemicals and DOC from tire particles under simulated marine conditions. Front. Environ. Sci. 11:1206449. doi: 10.3389/fenvs.2023.1206449
Received: 15 April 2023; Accepted: 12 June 2023;
Published: 22 June 2023.
Edited by:
Tania Martellini, University of Florence, ItalyReviewed by:
Cassandra Rauert, The University of Queensland, AustraliaShangwei Zhang, Beijing Normal University, China
Copyright © 2023 Foscari, Schmidt, Seiwert, Herzke, Sempéré and Reemtsma. This is an open-access article distributed under the terms of the Creative Commons Attribution License (CC BY). The use, distribution or reproduction in other forums is permitted, provided the original author(s) and the copyright owner(s) are credited and that the original publication in this journal is cited, in accordance with accepted academic practice. No use, distribution or reproduction is permitted which does not comply with these terms.
*Correspondence: Thorsten Reemtsma, thorsten.reemtsma@ufz.de
†These authors have contributed equally to this work and share first authorship