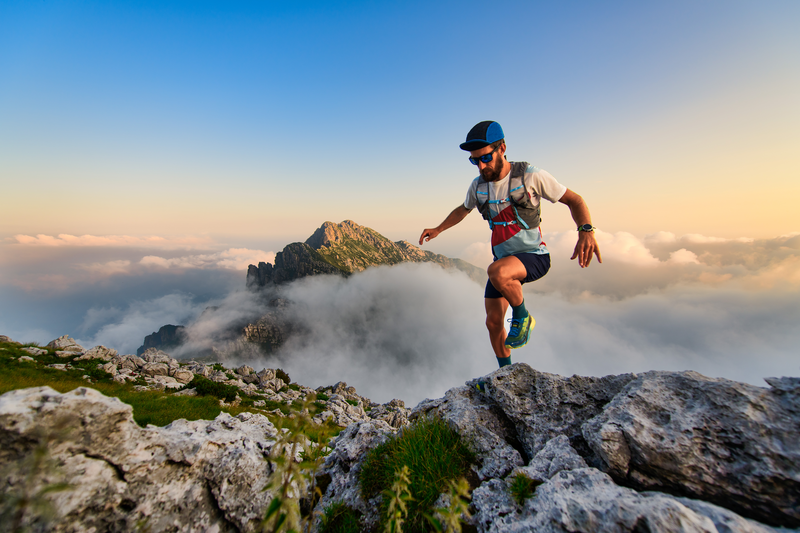
95% of researchers rate our articles as excellent or good
Learn more about the work of our research integrity team to safeguard the quality of each article we publish.
Find out more
EDITORIAL article
Front. Environ. Sci. , 19 April 2023
Sec. Water and Wastewater Management
Volume 11 - 2023 | https://doi.org/10.3389/fenvs.2023.1187335
This article is part of the Research Topic Advanced Technologies for Industrial Wastewater Reclamation View all 10 articles
Editorial on the Research Topic
Advanced technologies for industrial wastewater reclamation
Humankind is facing the significant challenge of water scarcity (Martins et al., 2020). Since 1950, the world population has doubled, and global water consumption has increased by more than 600% (Martins et al., 2020). A report by the United Nations demonstrated that two-thirds of the world’s population would face water stress by the year 2025 (Ahmed, 2006). A significant fraction of the increased water consumption was attributed to the rapid growth in the industrial developments (Sathya et al., 2022). Nearly all water consumed in industries ends up as industrial wastewater (IWW). The release of IWW into the environment may adversely affect the environment and pose a health threat to humans and our ecosystem (Martins et al., 2020).
Innovative solutions are therefore needed to provide adequate treatment of IWW to meet the increasingly stringent discharge standards and explore options to obtain sufficient water to support industrial developments (Cai et al., 2021). Treating and reclaiming treated industrial effluent can be an effective option to meet both challenges. Nonetheless, IWW is far more complex than domestic sewage, containing numerous organic and inorganic pollutants and exhibiting much greater variability in wastewater characteristics (Sathya et al., 2022). As a result, employing state-of-the-art biological treatment technologies alone is often inadequate for meeting stringent discharge standards, not to mention the ability to meet water reclamation requirements (Samaei et al., 2018; Sathya et al., 2022). Alternatives such as advanced oxidation processes (AOPs, e.g., ozonation (Deng et al., 2021a; Jothinathan et al., 2021), Fenton (Cai et al., 2020a; Cai et al., 2021; Sathya et al., 2022) and photocatalytic oxidation (Thiruvenkatachari et al., 2008; Autin et al., 2013) and separation processes (e.g., membrane filtration (Deng et al., 2021b; Liu et al., 2021) and adsorption (Ambaye et al., 2020) have been used for treating recalcitrant organics. However, AOPs typically require high energy (e.g., O3-generation in the ozonation process and UV-irradiation in the photocatalytic process) and chemical (e.g., oxidants and catalysts in the Fenton process and persulphate-based oxidation process) consumptions and therefore are expensive options (Loh et al., 2021; Wu et al., 2021; Jothinathan et al., 2022). Separation processes, on the other hand, have been used for pollutant removal and resource recovery, but innovative strategies are still needed to reduce their costs (Ambaye et al., 2020; Liu et al., 2021). Thus, there is a need to develop innovative technologies and build upon our knowledge of existing technologies for IWW treatment and reclamation.
Beyond the development of innovative technologies, combinations of the existing technologies could also obtain considerable performance with reduced energy and chemical consumption. Deng et al. (2021b) combined catalytic ozonation processes with a membrane bioreactor (MBR) for the treatment of phenolic wastewater produced by the petrochemical industry. This combination achieved total organic carbon (TOC) and phenolic compounds removals of 98.0% and 99.4%, respectively, and decreased the membrane fouling rate of MBR by 88.2%. The pretreatment by catalytic ozonation in the combined process reduced the acute biotoxicity of the wastewater by 79.2% and increased the 5-day biochemical oxygen demand to chemical oxygen demand ratio (BOD5/COD) by 2.45-folds, which significantly contributed to the performance enhancement and membrane fouling mitigation of MBR (Deng et al., 2021b). Tong et al. (2018) combined the adsorption processes by ligntie-activated coke (LAC-adsorption) and immobilized biological filter (IBF) in the treatment of heavy oil wastewater on a pilot-scale system. The combined process obtained a dissolved organic carbon (DOC) removal of 85.9%, where most biorefractory compounds were removed by the pre-LAC-adsorption process and benefitted the subsequent IBF, and the post-LAC-adsorption process contributed to amides removal (Tong et al., 2018). Cai et al. (2020b) combined the fluidized bed reactor-based Fenton processes (FBR-Fenton) with a biological activated carbon (BAC) system for the reclamation of reverse osmosis concentrate and obtained a COD removal of 69% with a low average effluent COD of 26 mg/L. In the combined process, the FBR-Fenton processes markedly contributed to the degradation of humic acid and fulvic acid and improved the BOD5/COD ratio by 4.2-10.0 times, which strengthened the performance of the BAC system (Cai et al., 2020b).
In addition to the IWW treatment and effluent reuse, the recovery of valuable resources is also essential under the global trends of the circular economy (Goglio et al., 2019; Soltangheisi et al., 2019; Cheng et al., 2022). Wastewater is a rich source of organic carbon, nutrients (e.g., nitrogen N) and phosphorus P) and metals (e.g., potassium K), copper (Cu) and silver (Ag). Concerning energy generation, Heidrich et al. precisely determined the energy content of domestic wastewater (DWW) through freeze-drying samples to minimize the loss of volatiles and organic matters. Their results indicated that DWW contains an energy generation potential of 7.6 kJ/L (Heidrich et al., 2011). Wang et al. also estimated that the energy content of DWW related to COD was 23 W per capita (Wang et al., 2017). Similar assessments on IWW are lacking, but a significant energy recovery potential of organic IWW can be estimated due to their normally much higher organic carbon concentration. In terms of nutrients, it has been estimated that more than 20% of consumed P from minerals is excreted by human activities (Cordell et al., 2009). Robles et al. estimated that the global resource recovery from waste streams could essentially retrieve 50% of the P consumed by humans. Additionally, an N to P mass ratio of over 3 has been widely observed in research done on various waste streams, which indicated that more N than P is lost during the wastewater treatment (Batstone et al., 2015; Robles et al., 2020). Some kinds of IWW contain extremely high concentrations of N and P compared to DWW (Bokun et al., 2020; Deng et al., 2022). For example, in coking wastewater, the ammonia concentration is usually in a high range of 500-2,000 mg N/L, and it could further research the range of 3,500-10,000 mg N/L in the semi-coking wastewater when the pyrolytic temperature of coal was reduced to 600°C-800°C (Ma et al., 2017; Bokun et al., 2020; Huang et al., 2021). P concentration is usually high in the waste streams, such as fertilizer production wastewater, paper pulping and making wastewater and some of the chemical industry wastewater (Bian et al., 2011; Hutnik et al., 2013; Gentili & Sveriges, 2014). Specifically, the P concentration in the wastewater from the phosphorus mineral fertilizers industry (Hutnik et al., 2013) and the wastewater from PolyTH production industry (Bian et al., 2011) could reach 445 mg P/L and 15,000-18,000 mg P/L, respectively. Metal ions are also rich in IWW, such as K and Mg are rich in dairy wastewater and manure wastewater (Goglio et al., 2019), and Ag and Cu are rich in deplating wastewater (Gu et al., 2020). Based on the abovementioned information, IWW is an abundant source of energy, nutrients and metals to be recovered and reused, which could significantly contribute to the circular economy. Furthermore, the worldwide increase in consumption and the gradual depletion of natural resources have emphasized the need for resource recovery from waste streams (Goglio et al., 2019; Soltangheisi et al., 2019). Therefore, instead of releasing resource-rich waste streams into the water bodies increasing the environmental risk, this resource recovery can lead to multiple benefits, such as generating valuable products, greatly improving wastewater treatment processes, reclaiming wastewater, maintaining the ecological balance of aquatic environments and reducing the carbon footprint in wastewater disposal. In order to fulfill this vision, novel technologies for resource recovery from IWW need to be developed by the research community.
This Research Topic focuses on novel technologies for IWW treatment for the purpose of resource reclamation. This includes the dissemination of knowledge generated from studies regarding the development, optimization, and applications of advanced technologies aiming at the treatment and resource recovery of IWW. It also aims to address an important aspect of sustainable water management with an emphasis on meeting the needs of the industrial sector from a global perspective.
We hope this Research Topic can contribute significantly to both the research and the engineering communities for managing IWW treatment and reclamation.
All authors listed have made a substantial, direct and intellectual contribution to the work, and approved it for publication.
The authors declare that the research was conducted in the absence of any commercial or financial relationships that could be construed as a potential conflict of interest.
All claims expressed in this article are solely those of the authors and do not necessarily represent those of their affiliated organizations, or those of the publisher, the editors and the reviewers. Any product that may be evaluated in this article, or claim that may be made by its manufacturer, is not guaranteed or endorsed by the publisher.
Ahmed, A. M. (2006). UNDP, 2006, beyond scarcity: Power, poverty and global water circes human development report. Ahfad J. 23 (2), 150.
Ambaye, T. G., Vaccari, M., van Hullebusch, E. D., Amrane, A., and Rtimi, S. (2020). Mechanisms and adsorption capacities of biochar for the removal of organic and inorganic pollutants from industrial wastewater. Int. J. Environ. Sci. Technol. (Tehran) 18 (10), 3273–3294. doi:10.1007/s13762-020-03060-w
Autin, O., Hart, J., Jarvis, P., MacAdam, J., Parsons, S. A., and Jefferson, B. (2013). The impact of background organic matter and alkalinity on the degradation of the pesticide metaldehyde by two advanced oxidation processes: UV/H2O2 and UV/TiO2. Water Res. Oxf. 47 (6), 2041–2049. doi:10.1016/j.watres.2013.01.022
Batstone, D. J., Hulsen, T., Mehta, C. M., and Keller, J. (2015). Platforms for energy and nutrient recovery from domestic wastewater: A review. Chemosphere 140, 2–11. doi:10.1016/j.chemosphere.2014.10.021
Bian, D., Ai, S., Liu, J., Zuo, Y., and Tian, X. (2011). Treatment of phosphorus waste water using crystallization method. J. Environ. Sci. 23, S106–S109. doi:10.1016/s1001-0742(11)61088-7
Bokun, C., Siyu, Y., Yangyang, W., Miyangzi, S., and Yu, Q. (2020). Intensified phenols extraction and oil removal for industrial semi-coking wastewater: A novel economic pretreatment process design. J. Clean. Prod. 242, 118453. doi:10.1016/j.jclepro.2019.118453
Cai, Q. Q., Jothinathan, L., Deng, S. H., Ong, S. L., Ng, H. Y., and Hu, J. Y. (2021). “11 - Fenton- and ozone-based AOP processes for industrial effluent treatment,” in Advanced oxidation processes for effluent treatment plants. Editor M. P. Shah (Elsevier), 199–254.
Cai, Q. Q., Wu, M. Y., Hu, L. M., Lee, B. C. Y., Ong, S. L., Wang, P., et al. (2020a). Organics removal and in-situ granule activated carbon regeneration in FBR-Fenton/GAC process for reverse osmosis concentrate treatment. Water Res. Oxf. 183, 116119. doi:10.1016/j.watres.2020.116119
Cai, Q. Q., Wu, M. Y., Li, R., Deng, S. H., Lee, B. C. Y., Ong, S. L., et al. (2020b). Potential of combined advanced oxidation – biological process for cost-effective organic matters removal in reverse osmosis concentrate produced from industrial wastewater reclamation: Screening of AOP pre-treatment technologies. Chem. Eng. 389, 123419. doi:10.1016/j.cej.2019.123419
Cheng, D., Ngo, H. H., Guo, W., Chang, S. W., Nguyen, D. D., Zhang, S., et al. (2022). Impact factors and novel strategies for improving biohydrogen production in microbial electrolysis cells. Bioresour. Technol. 346, 126588. doi:10.1016/j.biortech.2021.126588
Cordell, D., Drangert, J.-O., and White, S. J. G. e. c. (2009). The story of phosphorus: Global food security and food for thought. Glob. food Secur. food thought 19 (2), 292–305. doi:10.1016/j.gloenvcha.2008.10.009
Deng, S., Jothinathan, L., Cai, Q., Li, R., Wu, M., Ong, S. L., et al. (2021a). FeOx@GAC catalyzed microbubble ozonation coupled with biological process for industrial phenolic wastewater treatment: Catalytic performance, biological process screening and microbial characteristics. Water Res. Oxf. 190, 116687. doi:10.1016/j.watres.2020.116687
Deng, S., Peng, S., Ngo, H. H., Oh, S. J.-A., Hu, Z., Yao, H., et al. (2022). Characterization of nitrous oxide and nitrite accumulation during iron (Fe(0))- and ferrous iron (Fe(II))-driven autotrophic denitrification: Mechanisms, environmental impact factors and molecular microbial characterization. Chem. Eng. J. 438, 135627. doi:10.1016/j.cej.2022.135627
Deng, S., Wang, Q., Cai, Q., Ong, S. L., and Hu, J. (2021b). Efficient bio-refractory industrial wastewater treatment with mitigated membrane fouling in a membrane bioreactor strengthened by the micro-scale ZVI@GAC galvanic-cells-initiated radical generation and coagulation processes. Water Res. 209, 117943. doi:10.1016/j.watres.2021.117943
Gentili, F. G., and Sveriges, l. (2014). Microalgal biomass and lipid production in mixed municipal, dairy, pulp and paper wastewater together with added flue gases. Bioresour. Technol. 169, 27–32. doi:10.1016/j.biortech.2014.06.061
Goglio, A., Marzorati, S., Rago, L., Pant, D., Cristiani, P., and Schievano, A. (2019). Microbial recycling cells: First steps into a new type of microbial electrochemical technologies, aimed at recovering nutrients from wastewater. Bioresour. Technol. 277, 117–127. doi:10.1016/j.biortech.2019.01.039
Gu, J. N., Liang, J., Chen, C., Li, K., Zhou, W., Jia, J., et al. (2020). Treatment of real deplating wastewater through an environmental friendly precipitation-electrodeposition-oxidation process: Recovery of silver and copper and reuse of wastewater. Sep. Purif. Technol. 248, 117082. doi:10.1016/j.seppur.2020.117082
Heidrich, E. S., Curtis, T. P., and Dolfing, J. (2011). Determination of the internal chemical energy of wastewater. Environ. Sci. Technol. 45 (2), 827–832. doi:10.1021/es103058w
Huang, H., Li, B., Dai, J., Wang, W., Zhang, M., and Ou, Y. (2021). Ammonia nitrogen removal from coking wastewater and high quality gypsum recovery by struvite recycling by using calcium hydroxide as decomposer. J. Environ. Manag. 292, 112712. doi:10.1016/j.jenvman.2021.112712
Hutnik, N., Kozik, A., Mazienczuk, A., Piotrowski, K., Wierzbowska, B., and Matynia, A. (2013). Phosphates (V) recovery from phosphorus mineral fertilizers industry wastewater by continuous struvite reaction crystallization process. Water Res. 47 (11), 3635–3643. doi:10.1016/j.watres.2013.04.026
Jothinathan, L., Cai, Q. Q., Ong, S. L., and Hu, J. Y. (2022). Fe-Mn doped powdered activated carbon pellet as ozone catalyst for cost-effective phenolic wastewater treatment: Mechanism studies and phenol by-products elimination. J. Hazard. Mater. 424, 127483. doi:10.1016/j.jhazmat.2021.127483
Jothinathan, L., Cai, Q. Q., Ong, S. L., and Hu, J. Y. (2021). Organics removal in high strength petrochemical wastewater with combined microbubble-catalytic ozonation process. Chemosphere Oxf. 263, 127980. doi:10.1016/j.chemosphere.2020.127980
Liu, Y., Liu, H., and Shen, Z. (2021). Nanocellulose based filtration membrane in industrial waste water treatment: A review. Materials 14 (18), 5398. doi:10.3390/ma14185398
Loh, W. H., Cai, Q. Q., Li, R., Jothinathan, L., Lee, B. C. Y., Ng, O. H., et al. (2021). Reverse osmosis concentrate treatment by microbubble ozonation-biological activated carbon process: Organics removal performance and environmental impact assessment. Sci. total Environ. 798, 149289. doi:10.1016/j.scitotenv.2021.149289
Ma, X., Wang, X., Liu, Y., Gao, J., and Wang, Y. (2017). Variations in toxicity of semi-coking wastewater treatment processes and their toxicity prediction. Ecotoxicol. Environ. Saf. 138, 163–169. doi:10.1016/j.ecoenv.2016.09.031
Martins, R. C., Quinta-Ferreira, R. M., Rasteiro, M. G., Dantas, R., and Gmurek, M. (2020). Editorial: Advanced processes for wastewater treatment and water reuse. Front. Environ. Sci. 8. doi:10.3389/fenvs.2020.611159
Robles, A., Aguado, D., Barat, R., Borras, L., Bouzas, A., Gimenez, J. B., et al. (2020). New frontiers from removal to recycling of nitrogen and phosphorus from wastewater in the Circular Economy. Bioresour. Technol. 300, 122673. doi:10.1016/j.biortech.2019.122673
Samaei, S. M., Gato-Trinidad, S., and Altaee, A. (2018). The application of pressure-driven ceramic membrane technology for the treatment of industrial wastewaters – a review. Sep. Purif. Technol. 200, 198–220. doi:10.1016/j.seppur.2018.02.041
Sathya, K., Nagarajan, K., Carlin Geor Malar, G., Rajalakshmi, S., and Raja Lakshmi, P. (2022). A comprehensive review on comparison among effluent treatment methods and modern methods of treatment of industrial wastewater effluent from different sources. Appl. water Sci. 12 (4), 70–27. doi:10.1007/s13201-022-01594-7
Soltangheisi, A., Withers, P. J. A., Pavinato, P. S., Cherubin, M. R., Rossetto, R., Do Carmo, J. B., et al. (2019). Improving phosphorus sustainability of sugarcane production in Brazil. Glob. change Biol. Bioenergy 11 (12), 1444–1455. doi:10.1111/gcbb.12650
Thiruvenkatachari, R., Vigneswaran, S., and Moon, I. S. (2008). A review on UV/TiO2 photocatalytic oxidation process (Journal Review). Korean J. Chem. Eng. 25 (1), 64–72. doi:10.1007/s11814-008-0011-8
Tong, K., Zhang, Z., Lin, A., Song, Q., Ji, G., Wang, D., et al. (2018). Treatment of super heavy oil wastewater by a combined process of lignite-activated coke adsorption and immobilized biological filter degradation: Performance and the relevant microbial community analysis. J. Chem. Technol. Biotechnol. 93 (10), 2942–2951. doi:10.1002/jctb.5650
Wang, K., Sheng, Y., Cao, H., Yan, K., and Zhang, Y. (2017). Impact of applied current on sulfate-rich wastewater treatment and microbial biodiversity in the cathode chamber of microbial electrolysis cell (MEC) reactor. Chem. Eng. J. 307, 150–158. doi:10.1016/j.cej.2016.07.106
Keywords: industrial wastewater, pollutants disposal, resource reclamation, effluent reuse, energy generation, nutrient and mineral recovery
Citation: Deng S, Hu J, Ong S-L, Li Q and Han J (2023) Editorial: Advanced technologies for industrial wastewater reclamation. Front. Environ. Sci. 11:1187335. doi: 10.3389/fenvs.2023.1187335
Received: 15 March 2023; Accepted: 14 April 2023;
Published: 19 April 2023.
Edited and reviewed by:
Paolo Perona, Platform of Hydraulic Constructions (PL-LCH), IIC, ENAC, EPFL, SwitzerlandCopyright © 2023 Deng, Hu, Ong, Li and Han. This is an open-access article distributed under the terms of the Creative Commons Attribution License (CC BY). The use, distribution or reproduction in other forums is permitted, provided the original author(s) and the copyright owner(s) are credited and that the original publication in this journal is cited, in accordance with accepted academic practice. No use, distribution or reproduction is permitted which does not comply with these terms.
*Correspondence: Jiangyong Hu, aHVqaWFuZ3lvbmdAbnVzLmVkdS5zZw==
Disclaimer: All claims expressed in this article are solely those of the authors and do not necessarily represent those of their affiliated organizations, or those of the publisher, the editors and the reviewers. Any product that may be evaluated in this article or claim that may be made by its manufacturer is not guaranteed or endorsed by the publisher.
Research integrity at Frontiers
Learn more about the work of our research integrity team to safeguard the quality of each article we publish.