- Department of Engineering for Environment, Land and Infrastructure, Politecnico di Torino, DIATI, Turin, Italy
Hydropower (HP) is a key source of renewable energy, but also poses significant environmental challenges, including habitat fragmentation, hydropeaking and perturbations of flow and sediment regime. This study analyzed how Italian companies managing HP plants, and registered to the European Eco-Management and Audit Scheme (EMAS) in 2022, evaluated their sustainability and publicly disclosed related data. The analysis was based on the environmental statements (ESs) of 206 hydropower plants (29% of Italian HP production in 2019). The applied methodology involved an inventory of the plants and their categorization about technical features and the reporting of technical, environmental, and social aspects. The results of the analysis revealed that the companies rarely described the environmental state of the site, even less frequently adopting quantitative indicators. “Soil contamination”, “biodiversity”, “waste production”, “risk of environmental accidents”, “water pollution and flow management”, and “noise emissions” were the aspects considered most significant. No correlation was found among the significance of an aspect, the number of indicators used to describe the associated impacts, and the number of objectives set for reducing those impacts. “Biodiversity” was mostly described through irrelevant indicators (i.e., total site area) and 76% of the allocated budget was assigned to technical aspects without a clear connection with any environmental improvement. In particular, the specific impacts on the aquatic ecosystems were scarcely reported, mitigation measures were rarely mentioned and with few details. In conclusion, the results of the study highlight the need for specific guidelines, directed to the HP sector, for effectively reporting the environmental performances and the effectiveness of the adopted Environmental Management Systems, while providing valuable information to policymakers and researchers.
1 Introduction
Global energy consumption is rapidly increasing (AlQattan et al., 2018; Ma et al., 2021), and supplying environmentally friendly, fair, affordable, and secure energy is fundamental for sustainable development (Vera and Langlois, 2007). The United Nations’ 2030 Agenda for Sustainable Development (United Nations, 2015) dedicated Sustainable Development Goal No. 7 to “ensuring access to affordable, reliable, sustainable, and modern energy for all”. The production of heat and electricity is the main responsible for global greenhouse gas (GHG) emissions, and in 2019 it accounted for 33% of total emissions (Climate Watch, 2019) due to the high share of fossil fuels (Ouda et al., 2016). Decreasing the carbon intensity of energy production is of foremost importance to reach carbon neutrality by 2050, defined “the world’s most urgent mission” by the United Nations (United Nations, 2020) and “the heart of the European Green Deal” by the European Commission (European Commission, 2019). The European Green Deal acknowledges the key role played by renewable energy sources to achieve the objectives set for the reduction of GHG emissions, and defined a 40% minimum share of renewables in the EU energy mix as target for 2030.
Among renewable energy sources, hydropower (HP) is paramount; it has long tradition and significance in Europe, particularly in the Alps due to the presence of steep slopes and high water availability (Wagner et al., 2019; Vassoney et al., 2020). In the EU, HP accounted for 35.4% of total renewable energy production in 2020 (Vassoney et al., 2017), with 151 GW of installed capacity (IRENA, 2022). In Italy, 48.2 TWh of energy were produced from HP in 2020, corresponding to 42% of the national renewable energy production (IRENA, 2022). Italy’s total installed HP capacity grew from 21.9 GW in 2012 to 22.7 GW in 2020 (+0.5% per year on average) (IRENA, 2022). Compared to other EU countries, Italy’s increase was slower than global growth (2.8%/year from 2012 to 2020 on average) (IRENA, 2022) because water resources have been already extensively exploited for HP, and the interest in new plants has been mostly focused on small-scale run-of-the-river (RoR) installations (Vassoney et al., 2019; Algieri et al., 2020).
HP is generally characterized by limited GHG emissions throughout its life cycle (Almeida et al., 2019; Kumar et al., 2021), and is also a safe and reliable energy source, with low operating costs and long plants’ operational life (Saw and Ji-Qing, 2019; Majumder et al., 2020; Quaranta et al., 2021). HP is also characterized by great flexibility, since dam reservoirs can store high amounts of energy at low cost (Hunt et al., 2020), and the associated plants can rapidly increase or decrease energy production to match the demand (hydropeaking) or to counterpart intermittent energy sources (Danso et al., 2021; Zhang et al., 2022). The capacity to store water in reservoirs can play an essential role in water supply for irrigation, residential and industrial use, flood regulation, and inland navigation (Hogeboom et al., 2018; Hecht et al., 2019).
However, HP faces various environmental challenges, as dams and weirs threaten numerous fish species (van Puijenbroek et al., 2019; He et al., 2021) by interrupting river continuity (Pini Prato et al., 2011; Calles et al., 2012), and thus hindering a crucial element for natural aquatic environments (European Commission, 2000). Fish migration can be enabled through fishways, but their application is not always feasible (e.g., high head dams), and design criteria are often based only on the swimming abilities of species with high socio-economic value (e.g., salmon) (Wilkes et al., 2019; Sánchez-Pérez et al., 2022). Furthermore, fishways’ effectiveness is often below 70% for upstream passages and even lower for downstream passages (Noonan et al., 2012; Calles et al., 2013; Hershey, 2021). Regulated reservoirs also perturbate the natural flow regime and sediment transport, resulting in reservoir siltation, riverbank erosion, habitat degradation, and loss of biodiversity (Espa et al., 2019; Koutrakis et al., 2019; Vassoney et al., 2019). Reservoirs siltation is often tackled with sediment flushing, which can trigger undesired ecological effects (Nukazawa et al., 2020; Doretto et al., 2021). The flexibility in electricity production allowed by dam reservoirs induces hydropeaking, leading to significant changes to the hydrology, hydraulics, and sediment regime of the river on very short time scales, also affecting the river ecosystem (Bruder et al., 2016; Bejarano et al., 2018). Although RoR HP plants are perceived as more environmentally friendly than large HP plants with regulated reservoirs, they have been associated with numerous environmental impacts, especially when the river flow is diverted (Anderson et al., 2017; Kuriqi et al., 2021). Another critical challenge for the HP sector is the setting of ecological flows maintained downstream of the water withdrawal sites. Traditionally, the minimum in-stream flow (MIF) was defined as “the minimum amount of water downstream of any diversion that allows for the protection of the aquatic ecosystem” (Alecci and Rossi, 2020). In contrast, the ecological flow (EF) is described in the European Guidance on Ecological Flows as “the amount of water required for the aquatic ecosystem to continue to thrive and provide the services we rely upon” (European Commission and Directorate-General for Environment, 2016). The ecological flow follows a more holistic approach and focuses on maintaining the health and function of the aquatic ecosystem, which requires consideration of its biological requirements varying over time, the natural flow regimes, and hydrological patterns (Alpine Convention, 2009; Vezza et al., 2014). The constant minimum in-stream flow is primarily focused on ensuring a minimum level of water in the river downstream the dam to maintain the health of the aquatic ecosystem, while ecological flows also encompass a series of minimum flows variable through time to mimic the natural river flow variability.
Given the environmental concerns of the HP sector and the associated ongoing and increasing interest, especially in developing countries (Couto and Olden, 2018; Pracheil et al., 2019), the capacity to assess the overall environmental sustainability of HP plants remains remarkably limited. This study analyzes how the Italian companies managing HP plants and registered to the European Eco-Management and Audit Scheme (EMAS) in 2022 evaluated their sustainability. EMAS is the voluntary international standard for implementing environmental management systems (European Commission and Council of the EU, 2009), along with ISO 14001 (ISO, 2015). Despite the similarities between the two schemes, EMAS places more emphasis on public disclosure of environmental performance data and stakeholder engagement, requiring registered organizations to publish each year the relevant environmental information in an Environmental Statement (ES), after the validation by an independent verifier. Another significant difference between EMAS and ISO 14001 is that the former foresees also a formal institutional assessment by the National Competent Authority, which includes a check by the Environment Agency on the effective legal compliance to all applicable environmental requirements, thus enforcing the accuracy and robustness of the environmental information disclosed in the ESs.
The Italian HP sector was selected as a case study because it accounts for 15% of the total installed capacity in the EU in 2020 (IRENA, 2022), and 148 out of the 241 EMAS registered sites producing energy are located in Italy (European Commission, 2022).
This study was aimed at: i) analyzing how the companies managing existing HP plants describe their environmental performance; ii) identifying the metrics and indicators used to describe the environmental impacts; iii) assessing on which aspects, how, and to what extent are set the improvement objectives, if any; iv) analyzing how the companies describe their impacts on the hosting site and on biodiversity, with a specific focus on aquatic ecosystems, and what measures have been implemented or planned to mitigate these impacts.
The remainder of this paper is structured as follows: Section 2 presents the methodology adopted in this study, while Section 3 describes the results of the analysis, including the key significant aspects and related performance, the improvement objectives, and how the impacts on biodiversity are reported. The discussion in Section 4 summarizes the study’s key findings and managerial implications and recommendations.
2 Methodology
The methodology applied in this study included three consequent phases: an inventory of the HP plants registered to EMAS in 2022 in Italy, the analysis of the information retrieved in the ESs on the technical, environmental, and social aspects disclosed by the companies managing the inventoried plants, and finally a sensitivity analysis of the analyzed data.
An inventory of the HP plants registered to EMAS in 2022 in Italy was created as an Excel database by analyzing the National Register of EMAS-certified sites (ISPRA, 2022) and cross-checking the sites with the NACE code “35.11” (i.e., production of electricity). The environmental statements (ESs) of the pre-selected plants were then screened to exclude sites referring to large combustion plants, photovoltaic power stations, and wind farms. Finally, the selected HP plants were individually assessed to confirm that it was possible to separate the data regarding HP plants from any other activities included in the ES.
Once the final sample of HP plants was delineated, the ESs were analyzed in detail focusing on the following 4 key elements:
• Technical features of the plants and production data
• Reporting on the environmental and socio-economic context
• Reporting on the key environmental, technical, and social aspects (significance, indicators, and improvement objectives)
• Reporting on the impacts on biodiversity and their mitigation
The technical characteristics of each HP plant were analyzed to identify the key features of the sample in relation to the water abstraction typology (dam, RoR, pumped storage), the HP plants characteristics (installed capacity, turbine type, available drop, average allowed outflow), and the energy production (gross and net electricity production, water volume fed to the turbines).
The ESs also allowed to retrieve the main characteristics and the related quantitative indicators used by the companies to describe the environmental and socio-economic context hosting the HP plants through 9 domains (or matrices): ecosystems, watercourses, fauna, aquifers, soil and subsoil, flora, geological and hydrogeological risk, population and socio-economic aspects, and landscape.
The ESs were finally scrutinized with regard to the following 18 key environmental, technical and social aspects: effects on biodiversity, soil contamination, emissions to air, water pollution and flow management, raw materials/energy/water consumption, odor/noise emissions, waste production, risks of environmental accidents, energy production, visual impact, radiations, stakeholder engagement, light pollution, transport, and process management. Specifically, data regarding those aspects were retrieved from the ESs, considering in sequence: the significance assigned by the companies to each aspect; then the metrics and indicators used to quantify each aspect; and finally, the objectives set to improve the environmental performances of the plant along the period 2017–2026, collecting the related actions, allocated budget, and metrics. EMAS requires organizations to evaluate the significance of each aspect based on its potential impact on the environment, considering the nature, scope, frequency, and severity of the impact, the sensitivity and vulnerability of the affected environment, regulatory requirements and public expectations, and the organization’s objectives, targets, and environmental policy.
A specific focus was devoted to analyzing how companies reported on their impacts on biodiversity, with a particular attention on fluvial ecosystems, and the related mitigation measures taken or planned. Firstly, the selected ESs were examined to assess whether the impacts of hydropeaking and sediment deposition in reservoirs were described and if mitigation measures were implemented. Then, the mitigation measures for habitat fragmentation were considered by collecting information on fish passages and barriers for fish safeguard. Finally, data on the definition and quantification of the ecological flow were collected.
The presence of correlations among the significance of the aspects, the number of indicators used to describe each aspect, and the number of improvement objectives was then investigated with the Spearman and Kendall-Tau correlation tests.
3 Results
148 energy production sites were registered to EMAS in 2022, and 17 included HP plants. All 17 sites were selected after the screening, as it was possible to separate the data regarding HP plants from other activities (e.g., photovoltaic installations, wind farms). Each site included between 1 and 39 HP plants for a total of 206 installations producing in overall 14.1 TWh of electricity in 2019, corresponding to 29.3% of the total electricity produced by HP plants in Italy.
The most common plant type was run-of-the-river (58%), followed by dams (41%) and pumped storage (1%) (Figure 1). Although a large variability was observed among plants’ installed capacity, produced electricity, available drop, and amount of water used, most of the plants were small-sized (60% below 10 MW), and the majority was characterized by high drops (53% above 90 m) and low average flow (60% below 5 m3/s). As expected, Pelton turbines were implemented more frequently (35%) than Kaplan turbines (23%), as Pelton turbines are used for high drops while Kaplan for small drops. The most applied turbine type was the Francis (42% of the total), used for average drops and a wide range of flows and capacities.
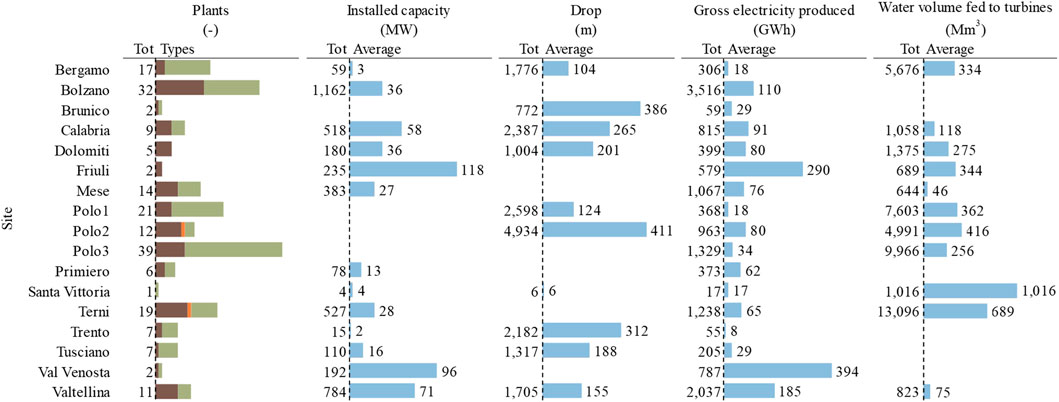
FIGURE 1. Technical features and production data of the analyzed sites. Tot refers to the entire site and average to the plants included in each site. Gross electricity produced and water volume used data are referred to 2019 (Brown = Dam; Orange = Pumped storage; Green = Run-of-the-river).
On average, each plant produced 68.5 GWh of gross electricity using 313 Mm3 of water in 2019.
3.1 Environmental and socio-economic context
The ESs rarely described the environmental and socio-economic context hosting the HP plants (Table 1). The state of the matrix “ecosystems” was addressed 11 times, but just declaring the presence of natural protected areas of interest (e.g., Natura 2000 or UNESCO sites, Parks, etc.) in the watershed. Only one indicator was used to quantify the state of the ecosystems, and only in relation to macrobenthic communities. Information about the other environmental and socio-economic matrices was disclosed in less than half of the ESs, and only 10 indicators were used to describe their state. Population characteristics and landscape resulted more reported than watercourses, flora, and fauna, also related to the aquatic ecosystems impacted by the HP plants.
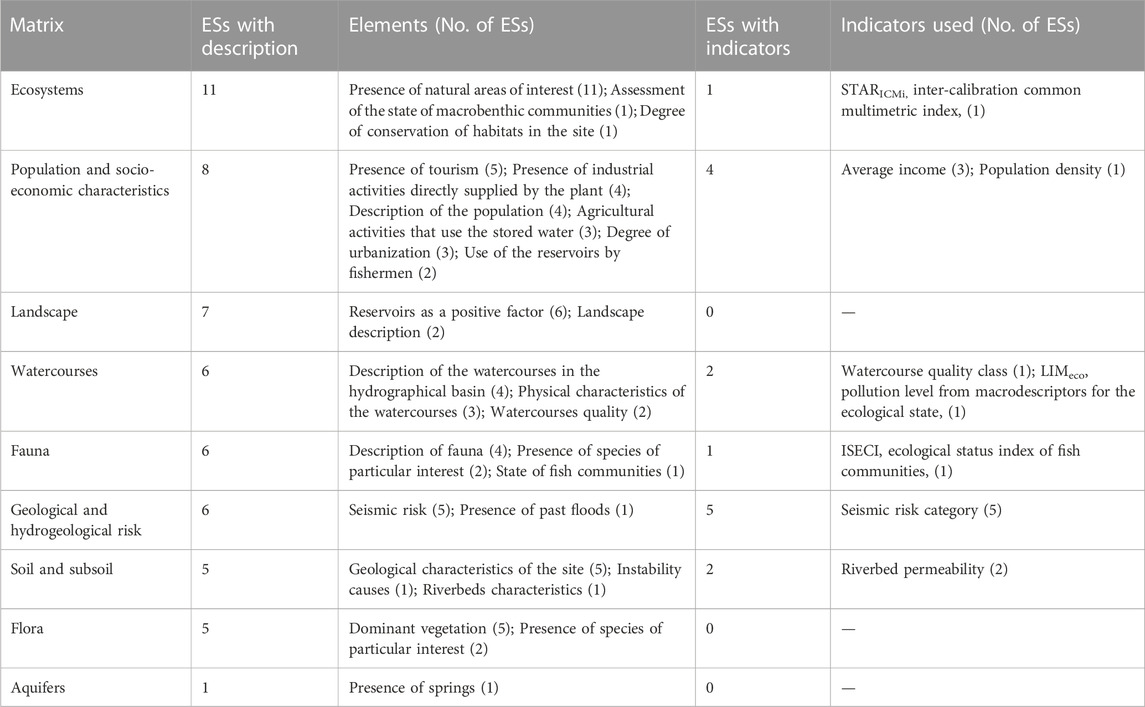
TABLE 1. ESs reporting on the environmental and socio-economic context. From left to right, the table reports: matrices described in the ESs; number of ESs that described the matrices; elements of the matrices described in the ESs and number of ESs that described such elements; number of ESs that used at least one indicator to quantify the matrix state; indicators used in the ESs and number of ESs that used the indicator.
3.2 Reporting on the key environmental, technical, and social aspects (significance, indicators, and improvement objectives)
3.2.1 Key significant aspects and related performance indicators
Table 2 reports the data related to the 18 key environmental, technical and social aspects disclosed in the ESs. Although 11 aspects were listed in over 80% of the ESs, only 6 were considered significant in more than half of the ESs (soil contamination, biodiversity, waste production, risk of environmental accidents, water pollution and flow management, and noise emissions). All those aspects received attention in the literature, with a particular focus on “biodiversity” and “water quality” (Parish et al., 2019; Nautiyal and Goel, 2020; Roy and Roy, 2022). On the other hand, the technical aspects were rarely considered significant (only by less than 20% of the ESs), as well as “stakeholder engagement”, “light pollution”, “transport”, and “odor emissions”.
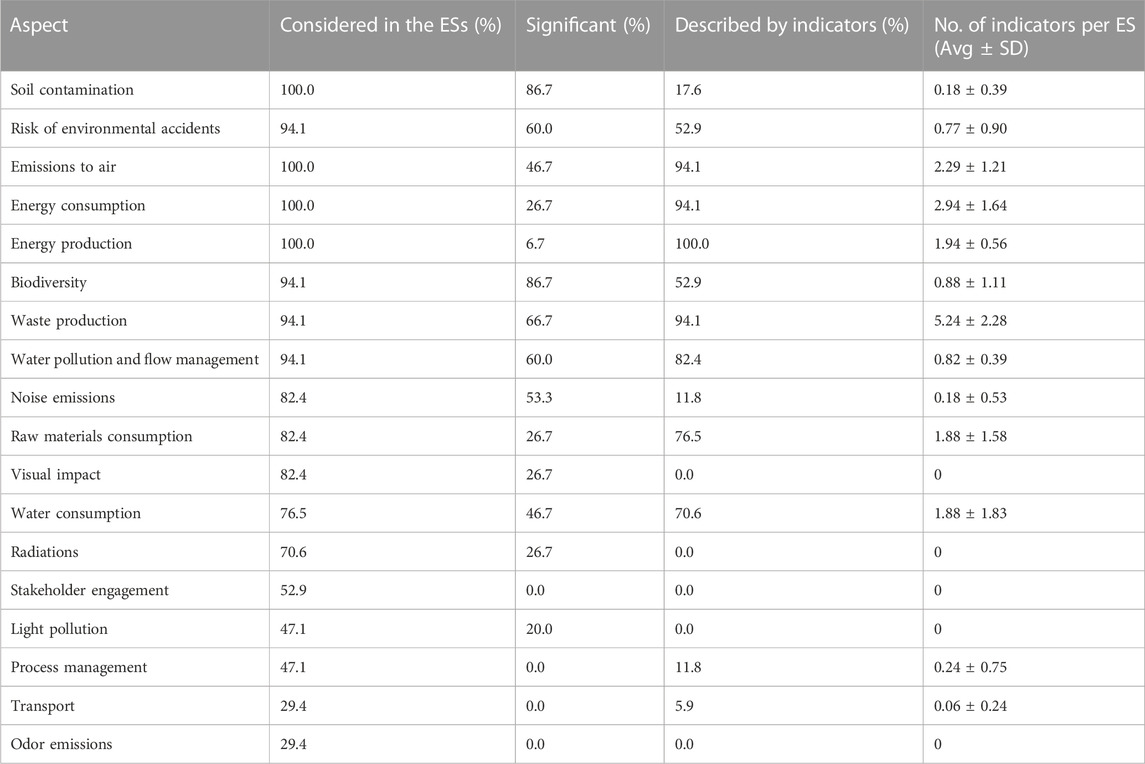
TABLE 2. Summary of the key aspects considered in the ESs, their significance assessment, and indicators used.
Four aspects (energy production, emissions to air, energy consumption, and waste production) were described by quantitative indicators in over 90% of the ESs, quantified by the highest number of indicators on average (1.9, 2.3, 2.9, and 5.2, respectively), and accounted for 65.3% of the 323 total environmental performance indicators. However, none of those aspects was considered significant and the detailed quantification found in the ESs might be related to other reasons. In line with a previous study on the waste-to-energy sector (Comoglio et al., 2022), “energy consumption”, “waste production”, and especially “energy production”, could have been quantified with more indicators because of their direct relationships with operational costs. Monitoring waste production is mandatory by law, leading to a consistent data availability, while emissions to air was influenced by the reporting on avoided GHG emissions due to electricity production by the HP sector, reported in 16 out of 17 of the ESs.
On the contrary, the aspects “soil contamination” and “noise emissions” were described by indicators in less than 20% of ESs and quantified by only 0.2 indicators on average, despite being considered significant in 86.7% and 53.3% of the ESs, respectively. “Biodiversity” was also quantified in a limited number of ESs (53%) and by few indicators (0.9 on average), despite being considered the most significant aspect together with soil contamination. No correlation was found between the significance of an aspect and the percentage of ESs describing them with indicators or the number of indicators used.
Table 3 lists the environmental indicators most frequently found (at least in two different ESs) for each aspect and represents an initial reference set of metrics for the organizations that manage HP plants to describe their environmental performance. The complete list is reported in Table 1 of the Supplementary Materials. “Total annual gross electricity” was the only indicator reported in all ESs, followed by “total annual mass of waste produced”, “total annual mass of non-hazardous waste produced”, and “total annual mass of hazardous waste produced” found in 15 ESs. Notably, 14 of the 17 ESs reported data regarding the minimum in-stream flow release. On the contrary, 12 of the 15 indicators reported for “biodiversity” were not related to relevant impacts of HP plants on the watercourse and other environmental matrices but rather to quantifying the total site area (7 indicators) and the amount of that area allocated to different uses, e.g., “total built-up area”, “total non-permeable area”, “total nature-oriented area” (5 indicators) (Table 3). Regarding “waste production”, 7 ESs disclosed data regarding waste production from materials substitution and maintenance, 2 ESs from screening residues, and 2 ESs from biological sludges.
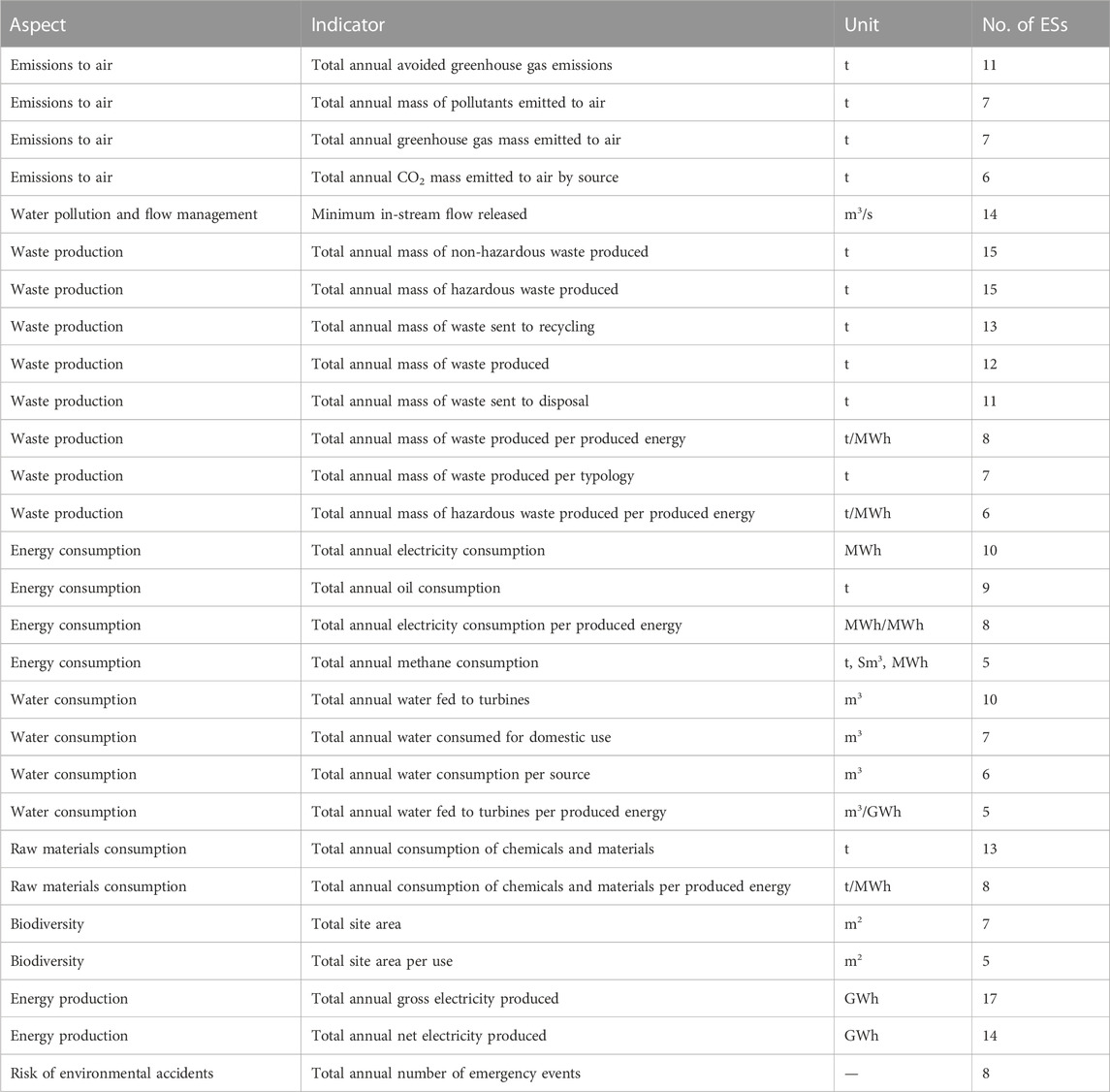
TABLE 3. List of the environmental indicators reported most frequently in the ESs (at least two different ESs).
3.2.2 Improvement objectives
“Visual impact” resulted the aspect with the highest number of improvement objectives set (30), followed by “energy production” (24), “energy consumption” (21), and “soil contamination” (19) (Table 4). The complete list is reported in Table 2 of the Supplementary Materials. Nevertheless, compared to the performance indicators, the improvement objectives were more spread across different aspects. Ten aspects had at least ten related improvement objectives, and nine had related improvement objectives in 40% of the ESs or more. The allocated budget was specified only for 41.4% of the 186 declared improvement objectives and accounted for 22.2 M€. “Energy production” was the aspect with the highest allocated budget (13.5 M€ and 60.8% of the total), followed by “process management” (2.5 M€) and “energy consumption” (1.2 M€). Again, no correlation was found between the significance of an aspect and the number of improvement objectives set or the total budget allocated for its improvement.
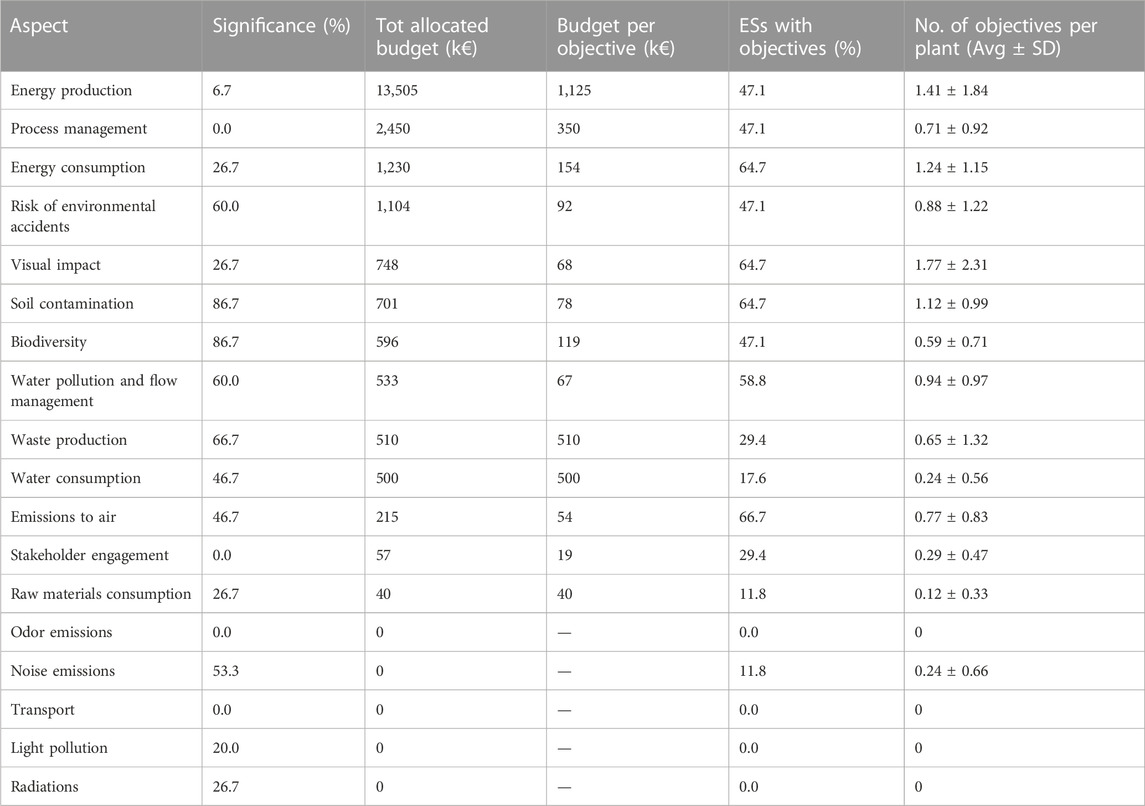
TABLE 4. Summary of the environmental improvement objectives set by the organizations and of budget allocation (cross: average; horizontal line: median).
Despite their low significance, the technical aspects of “process management” and especially “energy production” had a high objective occurrence rate in the ESs. Those objectives were also characterized by a high average budget per objective (1.11 M€ for “energy production” and 0.35 M€ for “process management”) due to expensive technical interventions such as turbines or generators substitution, refurbishments of plants, and the construction of a control center, leading to a combined allocated budget of 16.0 M€ (72.1% of the total) for the two aspects. “Visual impact”, “energy consumption”, and “stakeholder engagement” also received a high number of improvement objectives in several ESs despite their low significance. However, those aspects were characterized by a low budget per objective (0.07, 0.15, and 0.02 M€, respectively) related to inexpensive actions such as organizing plant tours, repainting buildings, and installing LED lights, accounting for a low total allocated budget (0.75, 1.23, and 0.06 M€, respectively).
On the contrary, either a few improvement objectives were set or a limited budget was allocated for the aspects defined with more frequency as significant in the ESs. For instance, a relatively high number of objectives were set to improve “risk of environmental accidents”, “soil contamination”, and “water pollution and flow management” (16, 19, and 16, respectively), but the average budget per objective was very low (0.08, 0.08, and 0.07 M€, respectively). This is because of relatively inexpensive actions such as installing leakage sensors, removing asbestos materials, and employing epoxy resin for transformers, leading to a limited total allocated budget (0.8, 0.7, and 0.5 M€, respectively). “Waste production”, “biodiversity”, and especially “noise emissions” had instead a lower number of objectives set for their improvement (11, 10, and 4), generally with a low total allocated budget, although the availability of budget data in the ESs was limited for those aspects.
Table 5 lists the objectives set in at least two of the analyzed ESs. Renovation of buildings to lower their visual impact was the one with the highest occurrence (16 ESs), followed by installing LED lights (14 ESs), purchase of lower emission vehicles (8 ESs), and removal of asbestos materials (8 ESs). It is notable that only 5 of the objectives set by the companies (i.e., “determination of the ecological flow”, “modification of the water release procedures”, “construction of a fishway”, “flushing of sediments”) might have a positive impact on biodiversity while, on the other hand, 12 objectives could have a negative effect. “Reducing waste production discharging water extracted from wells into the watercourse” could modify the water quality, while the “release of fish upstream of the plants” could represent an impact if the released fishes come from not accurately genetically selected hatcheries. The “construction of gabions to reduce the erosion of the banks” could alter the habitat diversity and availability, and “decreasing the released flow to increase electricity production” determines a clear alteration in habitat availability in the downstream river reaches and decreases the environmental sustainability of the plant. Lastly, “constructing a new HP plant” and “construction of a new weir”, represent a new anthropic element causing an environmental impact upon the river ecosystems. With regard to “water pollution and flow management”, most objectives were set to limit the risks of water contamination, but only one was associated with the “determination of the ecological flow” to be released downstream the dam. The lack of objectives associated with the determination of the EF can be related to its current limited and fragmented implementation in the regional water resources management policies in Italy (Moccia et al., 2020).
3.2.3 Reporting of the impacts on biodiversity and their mitigation
The impacts on biodiversity were scarcely reported, as summarized in Table 6.
Sediment management was mentioned only in 8 ESs, and 7 described its impacts. Furthermore, while all those 7 ESs stated operational problems (e.g., reduction of reservoir volume) and 2 ESs acknowledged issues related to security and efficiency decrease, only 1 ES described sediment management as an impact on biodiversity. It acknowledged potential fauna disturbance due to increased turbidity (assessed as comparable to flood events), and evaluated decreases in the macrobenthic community after flushing operations as temporary (2–3 months) without providing details about monitoring campaigns. Six ESs mentioned the reservoir management project, a document requested by Italian legislation for large reservoirs that describes the planned procedures for the removal of sediments from the reservoir in compliance with the quality objectives of the concerned water bodies. However, only 3 ESs described the sediment removal operations adopted. One ES reported that for smaller reservoirs operations were mechanical and that flushing was performed for larger reservoirs (Val Venosta). Another ES mentioned that flushing operations were conducted for one reservoir (Polo 3), and the last ES reported flushing of sediments scheduled every 3 years (Bolzano). None of the 3 ESs disclosed information on characterizations of the sediments. Although one ES stated that competent authorities authorized maximum turbidity and flow values during flushing operations (Bolzano), none of the ESs described any measure implemented to mitigate the impacts of sediments management. Two ESs defined improvement objectives to mitigate the impact from sediments management (Table 2 of the Supplementary Materials). The first ES (Bolzano) reported a dredging operation in 2020 aimed at reducing the impact on fish fauna. This operation was defined as “experimental” as sediments are currently flushed through the turbines. However, no information was provided regarding the cost or outcome of the experiment. The second ES (Polo3) reported an objective of increasing solid transport downstream of the plant by implementing sediments flushing through the turbines. Again, the ES lacked information on the effect of this action on the aquatic ecosystem as well as any financial data.
Only two ESs mentioned hydropeaking. Both ESs underlined the importance of hydropeaking to match the electricity demand peaks but recognized its impact on aquatic fauna due to rapid changes in the wetted perimeter of the river, especially during fish breeding seasons. Furthermore, none of the ESs described any measure implemented to mitigate hydropeaking impacts, nor did they report any improvement objectives for the implementation of such measures.
Regarding habitat fragmentation, fish passages for upstream migration were reported only in 4 ESs and related to 10 HP plants (5% of the total, all fish ladders). Moreover, none of the mentioned fishways was described in terms of technical characteristics (e.g., specific typology, drop between pools, total drop, etc.) or passage efficiency. The presence of fish bypasses for downstream migration was reported for 3 HP plants (1.5% of the total). The use of screens at water intake structures was mentioned for 43 plants (21% of the total), but just as a mean for protecting intake structures and turbines from debris or logs rather than for preventing fish from accessing the turbines (Calles et al., 2013). Only one improvement objective was directed towards mitigating habitat fragmentation, consisting in the construction of a fishway for upstream migration to reduce the impact on the fish fauna (Val Venosta). The construction was expected to be concluded during 2021, with a total cost of 0.54 M€. No information on the design process and typology of this fishway was disclosed in the ES. The limited number of fish passage solutions mentioned in the analyzed ESs basically reflects the fact that in Italy the related legal framework is still limited and fragmented and the implementation of these mitigation measures can be a mandatory requirement only for new dams or a retrofit requested by the competent Authorities at the renewal of the water withdrawal license of existing sites.
Finally, 16 ESs mentioned and 14 quantified the minimum in-stream flows. Nevertheless, only 2 ESs mentioned the stricter ecological flow, and none of the ESs reported its value. Two ESs defined improvement objectives concerning flow regulation. One ES (Polo 3) reported a modification of the calculated minimum in-stream flow to reduce the environmental impact of the affected HP plant, but without quantifying such flow release modification nor the criteria used for its definition. Another ES (Calabria) aimed at calculating the ecological flow to ensure the health of water bodies, but this was considered experimental due to ongoing discussions with local regulatory bodies regarding the definition of a new regional regulation. Moreover, both improvement objectives lacked further details on associated investments or implementation plans.
In summary, the reporting of impacts on biodiversity and their mitigation was found to be surprisingly lacking in the analyzed ESs, with sediment management, hydropeaking, and habitat fragmentation being poorly described. Only a few ESs mentioned mitigation measures, and improvement objectives were scarce, highlighting the need for specific guidelines to improve reporting in the hydropower sector especially on the crucially important environmental impacts of the hydropower plants. Furthermore, the only significant investment for the mitigation of the impacts on biodiversity was the construction of a fishway for the upstream migration of fish fauna (0.54 M€). This investment accounted for 91% of the overall budget allocated to biodiversity by all the plants of the sample, but still represented only 2% of the total budget allocated by plant operators, which amounted to 22.2 M€.
4 Discussion
4.1 Key findings of the study
This work analyzed the environmental reports (the ESs) of 206 Italian HP plants managed by organizations registered to EMAS in 2020, discussing how the managing companies evaluated their sustainability. The key findings of the study are as follows. Firstly, the ESs rarely described the environmental state of the site hosting the HP plant. Specifically, only 1 company described the ecosystems using a relevant indicator, and the watercourses, flora and fauna were considered less than population characteristics and landscape. The impacts on biodiversity were scarcely reported and 12 of the 15 indicators found in ESs were not related to relevant impacts of the HP plants. In details, of the 17 ESs, only one described the impacts of sediment management on biodiversity, 2 mentioned hydropeaking, 4 reported fish passages, and only 2 defined the ecological flow, although 14 defined the minimum in-stream flow. A key finding of our study is that there is a significant gap between what the HP plant operators report in their ESs and the information necessary for an effective assessment of the environmental impacts of the HP plants upon the aquatic ecosystems. This gap can be attributed to the fact that the organizations primarily focused on the reporting of environmental aspects with legally binding targets, such as waste management, and directly related with operational costs, for instance energy production and consumption. Therefore, those aspects were addressed in the ESs with more detail than more significant aspects such as “biodiversity”, for which the legal framework in Italy is still limited and fragmented.
No correlation was found between the significance of an aspect, the number of indicators used to quantify the impacts, and the budget that the companies invested in reducing them. This is in contrast with previous studies based on the analysis of the environmental reporting of companies registered to EMAS managing biodegradable waste treatment plants (Castelluccio et al., 2022) and waste-to-energy plants (Castelluccio et al., 2022; Comoglio et al., 2022), where a strong positive correlation between the significance of a single aspect and the total number of indicators used was found.
Considering the economic efforts, the companies managing the analyzed HP plants dedicated 76% of total budget to the improvement of technical aspects (“process management” and “energy production”) due to expensive technical interventions lacking a clear implication towards any environmental improvement. On the contrary, few improvement objectives were set for the aspects defined more significant, or a limited budget per objective was allocated. This is substantially consistent with the findings of the mentioned studies related to the biodegradable waste management (Castelluccio et al., 2022) and waste-to-energy (Castelluccio et al., 2022; Comoglio et al., 2022) sectors.
The findings of this study have important implications for the management of HP plants. Firstly, the unexpectedly low level of reporting on the environmental state of the sites hosting the HP plants suggests that more attention needs to be paid to environmental monitoring and assessment. This could include the development of standardized metrics that are relevant to the impacts of the sector, particularly in relation to the physical and ecological characteristics of the impacted watercourse.
Secondly, the lack of correlation between the significance of an aspect and the budget that the companies invested in those aspects highlights the need for more targeted investments. HP plant managers should prioritize environmental performance indicators that are most relevant to the sector and allocate sufficient budget to meet those objectives.
This study suggests that organizations managing HP plants could benefit from adopting environmental management systems such as EMAS. However, to be effective, these systems need to be supplemented with specific reporting guidelines for the HP sector. The implementation of these guidelines would have practical implications for policymakers and industry stakeholders, as it would enable more informed decisions and more appropriate measures to mitigate the environmental impacts of the sector, with a specific focus on the biodiversity of aquatic ecosystems. Furthermore, our findings have significant value for future research. The definition of environmental reporting guidelines specifically for the HP sector would address the focus on the most significant environmental aspects and allow researchers to better quantify its environmental impacts.
4.2 Novelty compared to prior research
The available literature has considered the sustainability assessment of the HP sector since about 25–30 years ago (Goodland, 1994; Kaygusuz, 2002), and it is mostly devoted to the sustainability assessment of new HP installations through site-specific indicators (i.e., “soil contamination”, “biodiversity”, “waste production”, “risk of environmental accidents”, “water pollution and flow management”, and “noise emissions”). The applied approach usually compared the status of the site before and after the construction of the HP plant (Lopes et al., 2022; Roy and Roy, 2022), while much less focus is dedicated to the impacts associated to existing plants. A recent study that assessed the ecological impacts of HP plants in operation proposed an ecological impact scorecard based on four criteria: environmental flow, hydropeaking, fish protection and passage performance, and sediment management (Alp et al., 2020). This study is particularly relevant because it suggests an integrated approach to evaluate the sustainability of existing HP plants in relation to aquatic ecosystems using an internationally recognized regulatory context. Other authors reviewed the indicators used in previous studies (Nautiyal and Goel, 2020; Pimentel Da Silva, 2021), and established environmental assessment frameworks. Some researchers also suggested subsets of indicators (Kumar and Katoch, 2014; Tahseen and Karney, 2017; Parish et al., 2019) to improve the efficiency of the assessment. Additionally, various frameworks relevant to the sustainability assessment of the HP sector have been developed. Richter et al. (1996) established a method for assessing the degree of hydrologic alteration attributable to human influence within an ecosystem. Bratrich et al. (2004) designed a concept for evaluating environmentally compatible hydropower production, while McManamay et al. (2020) proposed a toolkit for assessing the most relevant impacts of hydropower on river ecosystems. However, various authors documented shortcomings of the existing studies on the topic, including the lack of standardization (Tahseen and Karney, 2017), the bias towards global aspects due to limited use of local metrics (Mortey et al., 2019), the unavailability of data (Nautiyal and Goel, 2020), and the inadequate description of some impacts (Nautiyal and Goel, 2020). The established frameworks for the sustainability assessment of the HP sector also present limitations. For instance, some methods necessitate data spanning several decades before and after a particular impact to accurately determine changes in flow variability (Ma et al., 2014; Timpe and Kaplan, 2017). Other frameworks have been criticized due to the exclusion of important criteria that play critical roles in river ecosystem functions (Kunz et al., 2013).
In summary, our literature analysis highlighted three knowledge gaps: i) the limited standardization in the evaluation of the environmental performance of existing HP plants; ii) the limitations of some of the established frameworks, such as the scarce use of metrics relevant to the impacts of the HP sector and data availability; and iii) the lack of emphasis on how HP organizations evaluate their sustainability. Compared to the existing literature, this study is characterized by two main novelties. Firstly, it focused on the environmental performance of existing HP plants disclosed by the managing companies. Our first research question was related to how the environmental performances are quantitatively assessed, and if the companies planned to contain the environmental impacts through improvement objectives and mitigation measures. Secondly, this study explored the role of the adoption of an environmental management system (EMAS in our case) in supplying data useful for an environmental assessment of the HP sector. Therefore, our second research question investigated the reliability and consistency of the environmental reports associated to an environmental management system (EMAS) in providing a useful base of data to evaluate the sustainability of the HP sector.
4.3 Future directions of the research
The results of this study showed that the ESs of EMAS-registered organizations are not sufficient to evaluate the sustainability of the managed HP plants. There is an urgent need of specific environmental reporting guidelines for the HP sector, addressing the focus on the most significant environmental aspects, and particularly on the physical and ecological characteristics of the impacted watercourse, also defining a minimum set of performance indicators relevant to the HP sector to quantify its environmental impacts. To establish the reporting guidelines for the HP sector, it is recommended to reference the already developed frameworks for the assessment of the environmental impacts of HP plants on the aquatic ecosystem (Bratrich et al., 2004; Alp et al., 2020; McManamay et al., 2020). This could allow to use ESs, and environmental reporting in general, as tools for the evaluation of the sustainability performance of organizations (Barón Dorado et al., 2022).
Data availability statement
Publicly available datasets were analyzed in this study. This data can be found in the Environmental Statements concerning the analyzed plants, which can be obtained by contacting the managing companies or visiting their websites. For any further information, please contact the corresponding author.
Author contributions
SC: investigation, data curation, visualization, writing—original draft; CC: conceptualization, methodology, supervision, writing—original draft, writing-review and editing; SF: conceptualization, methodology, writing-review and editing. All authors contributed to the article and approved the submitted version.
Conflict of interest
The authors declare that the research was conducted in the absence of any commercial or financial relationships that could be construed as a potential conflict of interest.
Publisher’s note
All claims expressed in this article are solely those of the authors and do not necessarily represent those of their affiliated organizations, or those of the publisher, the editors and the reviewers. Any product that may be evaluated in this article, or claim that may be made by its manufacturer, is not guaranteed or endorsed by the publisher.
Supplementary material
The Supplementary Material for this article can be found online at: https://www.frontiersin.org/articles/10.3389/fenvs.2023.1178037/full#supplementary-material
References
Alecci, S., and Rossi, G. (2020). “Ecological in-stream flows,” in Water resources of Italy: Protection, use and control, world water resources. Editors G. Rossi, and M. Benedini (Cham: Springer International Publishing), 231–247. doi:10.1007/978-3-030-36460-1_10
Algieri, A., Zema, D. A., Nicotra, A., and Zimbone, S. M. (2020). Potential energy exploitation in collective irrigation systems using pumps as turbines: A case study in calabria (southern Italy). J. Clean. Prod. 257, 120538. doi:10.1016/j.jclepro.2020.120538
Almeida, R. M., Shi, Q., Gomes-Selman, J. M., Wu, X., Xue, Y., Angarita, H., et al. (2019). Reducing greenhouse gas emissions of Amazon hydropower with strategic dam planning. Nat. Commun. 10, 4281. doi:10.1038/s41467-019-12179-5
Alp, A., Akyüz, A., and Kucukali, S. (2020). Ecological impact scorecard of small hydropower plants in operation: An integrated approach. Renew. Energy 162, 1605–1617. doi:10.1016/j.renene.2020.09.127
Alpine Convention (2009). “Water and water management issues - report on the state of the Alps,” in Alpine signals - special edition 2 (Innsbruck: Ständiges Sekretariat der Alpenkonvention).
AlQattan, N., Acheampong, M., Jaward, F. M., Ertem, F. C., Vijayakumar, N., and Bello, T. (2018). Reviewing the potential of Waste-to-Energy (WTE) technologies for Sustainable Development Goal (SDG) numbers seven and eleven. Renew. Energy Focus 27, 97–110. doi:10.1016/j.ref.2018.09.005
Anderson, D., Moggridge, H., Shucksmith, J. D., and Warren, P. H. (2017). Quantifying the impact of water abstraction for low head ‘run of the river’ hydropower on localized river channel hydraulics and benthic macroinvertebrates. River Res. Appl. 33, 202–213. doi:10.1002/rra.2992
Barón Dorado, A., Giménez Leal, G., and de Castro Vila, R. (2022). EMAS environmental statements as a measuring tool in the transition of industry towards a circular economy. J. Clean. Prod. 369, 133213. doi:10.1016/j.jclepro.2022.133213
Bejarano, M. D., Jansson, R., and Nilsson, C. (2018). The effects of hydropeaking on riverine plants: A review. Biol. Rev. 93, 658–673. doi:10.1111/brv.12362
Bratrich, C., Truffer, B., Jorde, K., Markard, J., Meier, W., Peter, A., et al. (2004). Green hydropower: A new assessment procedure for river management. River Res. Appl. 20, 865–882. doi:10.1002/rra.788
Bruder, A., Tonolla, D., Schweizer, S. P., Vollenweider, S., Langhans, S. D., and Wüest, A. (2016). A conceptual framework for hydropeaking mitigation. Sci. Total Environ. 568, 1204–1212. doi:10.1016/j.scitotenv.2016.05.032
Calles, O., Karlsson, S., Hebrand, M., and Comoglio, C. (2012). Evaluating technical improvements for downstream migrating diadromous fish at a hydroelectric plant. Ecol. Eng., Ecohydraulic Approaches Restoring Habitat Connectivity Suitabil. 48, 30–37. doi:10.1016/j.ecoleng.2011.05.002
Calles, O., Karlsson, S., Vezza, P., Comoglio, C., and Tielman, J. (2013). Success of a low-sloping rack for improving downstream passage of silver eels at a hydroelectric plant. Freshw. Biol. 58, 2168–2179. doi:10.1111/fwb.12199
Castelluccio, S., Comoglio, C., and Fiore, S. (2022). Environmental performance reporting and assessment of the biodegradable waste treatment plants registered to EMAS in Italy. Sustainability 14, 7438. doi:10.3390/su14127438
Climate Watch (2019). Greenhouse gas (GHG) emissions. [WWW Document]. Available at: https://www.climatewatchdata.org/ghg-emissions (Accessed September 21, 2022).
Comoglio, C., Castelluccio, S., Scarrone, A., and Fiore, S. (2022). Analysis of environmental sustainability reporting in the waste-to-energy sector: Performance indicators and improvement targets of the EMAS-registered waste incineration plants in Italy. J. Clean. Prod. 2022, 134546. doi:10.1016/j.jclepro.2022.134546
Couto, T. B., and Olden, J. D. (2018). Global proliferation of small hydropower plants – science and policy. Front. Ecol. Environ. 16, 91–100. doi:10.1002/fee.1746
Danso, D. K., François, B., Hingray, B., and Diedhiou, A. (2021). Assessing hydropower flexibility for integrating solar and wind energy in West Africa using dynamic programming and sensitivity analysis. Illustration with the Akosombo reservoir, Ghana. J. Clean. Prod. 287, 125559. doi:10.1016/j.jclepro.2020.125559
Doretto, A., Piano, E., Fenoglio, S., Bona, F., Crosa, G., Espa, P., et al. (2021). Beta-diversity and stressor specific index reveal patterns of macroinvertebrate community response to sediment flushing. Ecol. Indic. 122, 107256. doi:10.1016/j.ecolind.2020.107256
Espa, P., Batalla, R. J., Brignoli, M. L., Crosa, G., Gentili, G., and Quadroni, S. (2019). Tackling reservoir siltation by controlled sediment flushing: Impact on downstream fauna and related management issues. PLoS ONE 14, e0218822. doi:10.1371/journal.pone.0218822
European Commission and Council of the EU (2009). Regulation (EC) No 1221/2009 of the European Parliament and of the Council of 25 November 2009 on the voluntary participation by organisations in a Community eco-management and audit scheme (EMAS). OJ L 342.
European Commission and Directorate-General for Environment (2016). Ecological flows in the implementation of the water framework directive. Luxembourg (Luxembourg): Publications Office. Guidance document No 31. doi:10.2779/775712
European Commission (2000). Directive 2000/60/EC of the European Parliament and of the Council of 23 October 2000 establishing a framework for Community action in the field of water policy. OJ L 327.
European Commission (2022). EMAS register [WWW Document]. Eur. Comm. - EMAS Regist. Available at: https://webgate.ec.europa.eu/emas2/public/registration/list (Accessed September 28, 2022).
European Commission (2019). European green deal [WWW Document]. Eur. Comm. - Eur. Green Deal. Available at: https://ec.europa.eu/info/strategy/priorities-2019-2024/european-green-deal_en (Accessed September 22, 2022).
Goodland, R. (1994). Environmental sustainability and the power sector. Impact Assess. 12, 409–470. doi:10.1080/07349165.1994.9725877
He, F., Thieme, M., Zarfl, C., Grill, G., Lehner, B., Hogan, Z., et al. (2021). Impacts of loss of free-flowing rivers on global freshwater megafauna. Biol. Conserv. 263, 109335. doi:10.1016/j.biocon.2021.109335
Hecht, J. S., Lacombe, G., Arias, M. E., Dang, T. D., and Piman, T. (2019). Hydropower dams of the mekong river basin: A review of their hydrological impacts. J. Hydrol. 568, 285–300. doi:10.1016/j.jhydrol.2018.10.045
Hershey, H. (2021). Updating the consensus on fishway efficiency: A meta-analysis. Fish. Fish. 22, 735–748. doi:10.1111/faf.12547
Hogeboom, R. J., Knook, L., and Hoekstra, A. Y. (2018). The blue water footprint of the world’s artificial reservoirs for hydroelectricity, irrigation, residential and industrial water supply, flood protection, fishing and recreation. Adv. Water Resour. 113, 285–294. doi:10.1016/j.advwatres.2018.01.028
Hunt, J. D., Byers, E., Wada, Y., Parkinson, S., Gernaat, D. E. H. J., Langan, S., et al. (2020). Global resource potential of seasonal pumped hydropower storage for energy and water storage. Nat. Commun. 11, 947. doi:10.1038/s41467-020-14555-y
IRENA (2022). Renewable energy statistics 2022. Masdar, United Arab Emirates: International Renewable Energy Agency.
ISO (2015). ISO 14001:2015. Environmental management systems. 3rd ed. Geneva, Switzerland: International Organization For Standardization. Requirements with guidance for use.
ISPRA (2022). List of organizations registered to EMAS. [WWW Document]. Ist. Super. Prot. E Ric. Ambient. Available at: https://www.isprambiente.gov.it/it/attivita/certificazioni/emas/elenco-organizzazioni-registrate-emas (Accessed October 6, 2022).
Kaygusuz, K. (2002). Sustainable development of hydroelectric power. Energy sources. 24, 803–815. doi:10.1080/00908310290086725
Koutrakis, E. T., Triantafillidis, S., Sapounidis, A. S., Vezza, P., Kamidis, N., Sylaios, G., et al. (2019). Evaluation of ecological flows in highly regulated rivers using the mesohabitat approach: A case study on the nestos river, N. Greece. Ecohydrol. Hydrobiol. 19, 598–609. doi:10.1016/j.ecohyd.2018.01.002
Kumar, A., Yu, Z.-G., Klemeš, J. J., and Bokhari, A. (2021). A state-of-the-art review of greenhouse gas emissions from Indian hydropower reservoirs. J. Clean. Prod. 320, 128806. doi:10.1016/j.jclepro.2021.128806
Kumar, D., and Katoch, S. S. (2014). Sustainability indicators for run of the river (RoR) hydropower projects in hydro rich regions of India. Renew. Sustain. Energy Rev. 35, 101–108. doi:10.1016/j.rser.2014.03.048
Kunz, M. J., Senn, D. B., Wehrli, B., Mwelwa, E. M., and Wüest, A. (2013). Optimizing turbine withdrawal from a tropical reservoir for improved water quality in downstream wetlands. Water Resour. Res. 49, 5570–5584. doi:10.1002/wrcr.20358
Kuriqi, A., Pinheiro, A. N., Sordo-Ward, A., Bejarano, M. D., and Garrote, L. (2021). Ecological impacts of run-of-river hydropower plants—current status and future prospects on the brink of energy transition. Renew. Sustain. Energy Rev. 142, 110833. doi:10.1016/j.rser.2021.110833
Lopes, P. V. F., Costa, C. M., da, S. B., Almeida, A. K., and de Almeida, I. K. (2022). Sustainability assessment model for Brazilian hydroelectric projects using multicriteria analysis. Sustain. Energy Technol. Assess. 51, 101851. doi:10.1016/j.seta.2021.101851
Ma, S., Xu, X., Li, C., Zhang, L., and Sun, M. (2021). Energy consumption inequality decrease with energy consumption increase: Evidence from rural China at micro scale. Energy Policy 159, 112638. doi:10.1016/j.enpol.2021.112638
Ma, Z. Z., Wang, Z. J., Xia, T., Gippel, C. J., and Speed, R. (2014). Hydrograph-based hydrologic alteration assessment and its application to the yellow river. J. Environ. Inf. 23, 1–13. doi:10.3808/jei.201400252
Majumder, P., Majumder, M., Saha, A. K., and Nath, S. (2020). Selection of features for analysis of reliability of performance in hydropower plants: A multi-criteria decision making approach. Environ. Dev. Sustain. 22, 3239–3265. doi:10.1007/s10668-019-00343-2
McManamay, R. A., Parish, E. S., DeRolph, C. R., Witt, A. M., Graf, W. L., and Burtner, A. (2020). Evidence-based indicator approach to guide preliminary environmental impact assessments of hydropower development. J. Environ. Manage. 265, 110489. doi:10.1016/j.jenvman.2020.110489
Moccia, D., Salvadori, L., Ferrari, S., Carucci, A., and Pusceddu, A. (2020). Implementation of the EU ecological flow policy in Italy with a focus on Sardinia. Adv. Oceanogr. Limnol. 11. doi:10.4081/aiol.2020.8781
Mortey, E. M., Kouassi, K. L., Diedhiou, A., Anquetin, S., Genoud, M., Hingray, B., et al. (2019). Sustainable hydroelectric dam management in the context of climate change: Case of the taabo dam in côte D’ivoire, west africa. Sustainability 11, 4846. doi:10.3390/su11184846
Nautiyal, H., and Goel, V. (2020). Sustainability assessment of hydropower projects. J. Clean. Prod. 265, 121661. doi:10.1016/j.jclepro.2020.121661
Noonan, M. J., Grant, J. W. A., and Jackson, C. D. (2012). A quantitative assessment of fish passage efficiency. Fish. Fish. 13, 450–464. doi:10.1111/j.1467-2979.2011.00445.x
Nukazawa, K., Kajiwara, S., Saito, T., and Suzuki, Y. (2020). Preliminary assessment of the impacts of sediment sluicing events on stream insects in the Mimi River, Japan. Ecol. Eng. 145, 105726. doi:10.1016/j.ecoleng.2020.105726
Ouda, O. K. M., Raza, S. A., Nizami, A. S., Rehan, M., Al-Waked, R., and Korres, N. E. (2016). Waste to energy potential: A case study of Saudi arabia. Renew. Sustain. Energy Rev. 61, 328–340. doi:10.1016/j.rser.2016.04.005
Parish, E. S., Pracheil, B. M., McManamay, R. A., Curd, S. L., DeRolph, C. R., and Smith, B. T. (2019). Review of environmental metrics used across multiple sectors and geographies to evaluate the effects of hydropower development. Appl. Energy 238, 101–118. doi:10.1016/j.apenergy.2019.01.038
Pimentel Da Silva, G. D. (2021). Powering corporate citizenship: Assessing corporate social responsibility of hydroelectric companies in Canada. Impact Assess. Proj. apprais. 39, 127–137. doi:10.1080/14615517.2021.1893927
Pini Prato, E., Comoglio, C., and Calles, O. (2011). A simple management tool for planning the restoration of river longitudinal connectivity at watershed level: Priority indices for fish passes. J. Appl. Ichthyol. 27, 73–79. doi:10.1111/j.1439-0426.2011.01856.x
Pracheil, B. M., McManamay, R. A., Parish, E. S., Curd, S. L., Smith, B. T., DeRolph, C. R., et al. (2019). A checklist of river function indicators for hydropower ecological assessment. Sci. Total Environ. 687, 1245–1260. doi:10.1016/j.scitotenv.2019.06.049
Quaranta, E., Aggidis, G., Boes, R. M., Comoglio, C., De Michele, C., Ritesh Patro, E., et al. (2021). Assessing the energy potential of modernizing the European hydropower fleet. Energy Convers. Manag. 246, 114655. doi:10.1016/j.enconman.2021.114655
Richter, B. D., Baumgartner, J. V., Powell, J., and Braun, D. P. (1996). A method for assessing hydrologic alteration within ecosystems. Conserv. Biol. 10, 1163–1174. doi:10.1046/j.1523-1739.1996.10041163.x
Roy, N. C., and Roy, N. G. (2022). Sustainable hydroelectric power project planning under socio-economic and environmental concerns using Index based approach. Built Environ. Proj. Asset Manag. 12, 401–432. doi:10.1108/BEPAM-06-2021-0082
Sánchez-Pérez, A., Torralva, M., Zamora-Marín, J. M., Bravo-Córdoba, F. J., Sanz-Ronda, F. J., and Oliva-Paterna, F. J. (2022). Multispecies fishways in a Mediterranean river: Contributions as migration corridors and compensatory habitat for fish. Sci. Total Environ. 830, 154613. doi:10.1016/j.scitotenv.2022.154613
Saw, M. M. M., and Ji-Qing, L. (2019). Review on hydropower in Myanmar. Appl. Water Sci. 9, 118. doi:10.1007/s13201-019-0984-y
Tahseen, S., and Karney, B. W. (2017). Reviewing and critiquing published approaches to the sustainability assessment of hydropower. Renew. Sustain. Energy Rev. 67, 225–234. doi:10.1016/j.rser.2016.09.031
Timpe, K., and Kaplan, D. (2017). The changing hydrology of a dammed Amazon. Sci. Adv. 3, e1700611. doi:10.1126/sciadv.1700611
United Nations (2020). Carbon neutrality by 2050: The world’s most urgent mission. [WWW Document]. Available at: https://www.un.org/sg/en/content/sg/articles/2020-12-11/carbon-neutrality-2050-the-world%E2%80%99s-most-urgent-mission (Accessed September 22, 2022).
United Nations (2015). Transforming our world: The 2030 Agenda for sustainable development. New York, United States: United Nations.
van Puijenbroek, P. J. T. M., Buijse, A. D., Kraak, M. H. S., and Verdonschot, P. F. M. (2019). Species and river specific effects of river fragmentation on European anadromous fish species. River Res. Appl. 35, 68–77. doi:10.1002/rra.3386
Vassoney, E., Mammoliti Mochet, A., and Comoglio, C. (2020). Multicriteria analysis for the assessment of flow release scenarios from a hydropower plant in the alpine region. Water Resour. Manag. 34, 637–651. doi:10.1007/s11269-019-02459-6
Vassoney, E., Mammoliti Mochet, A., and Comoglio, C. (2017). Use of multicriteria analysis (MCA) for sustainable hydropower planning and management. J. Environ. Manage. 196, 48–55. doi:10.1016/j.jenvman.2017.02.067
Vassoney, E., Mammoliti Mochet, A., Rocco, R., Maddalena, R., Vezza, P., and Comoglio, C. (2019). Integrating meso-scale habitat modelling in the multicriteria analysis (MCA) process for the assessment of hydropower sustainability. Water 11, 640. doi:10.3390/w11040640
Vera, I., and Langlois, L. (2007). Energy indicators for sustainable development. Energy 32, 875–882. Third Dubrovnik Conference on Sustainable Development of Energy, Water and Environment Systems. doi:10.1016/j.energy.2006.08.006
Vezza, P., Parasiewicz, P., Calles, O., Spairani, M., and Comoglio, C. (2014). Modelling habitat requirements of bullhead (Cottus gobio) in Alpine streams. Aquat. Sci. 76, 1–15. doi:10.1007/s00027-013-0306-7
Wagner, B., Hauer, C., and Habersack, H. (2019). Current hydropower developments in Europe. Curr. Opin. Environ. Sustain., Sustain. challenges 37, 41–49. doi:10.1016/j.cosust.2019.06.002
Wilkes, M. A., Webb, J. A., Pompeu, P. S., Silva, L. G. M., Vowles, A. S., Baker, C. F., et al. (2019). Not just a migration problem: Metapopulations, habitat shifts, and gene flow are also important for fishway science and management. River Res. Appl. 35, 1688–1696. doi:10.1002/rra.3320
Keywords: biodiversity, EMAS, environmental management system, environmental assessment, hydropower, renewable energy, sustainability
Citation: Comoglio C, Castelluccio S and Fiore S (2023) Environmental reporting in the hydropower sector: analysis of EMAS registered hydropower companies in Italy. Front. Environ. Sci. 11:1178037. doi: 10.3389/fenvs.2023.1178037
Received: 02 March 2023; Accepted: 30 May 2023;
Published: 21 June 2023.
Edited by:
Monica Rivas Casado, Cranfield University, United KingdomReviewed by:
Francisco Godinho, Hidroerg, PortugalFederica Murmura, University of Urbino Carlo Bo, Italy
Serhat Kucukali, Hacettepe University, Türkiye
Copyright © 2023 Comoglio, Castelluccio and Fiore. This is an open-access article distributed under the terms of the Creative Commons Attribution License (CC BY). The use, distribution or reproduction in other forums is permitted, provided the original author(s) and the copyright owner(s) are credited and that the original publication in this journal is cited, in accordance with accepted academic practice. No use, distribution or reproduction is permitted which does not comply with these terms.
*Correspondence: Claudio Comoglio, Y2xhdWRpby5jb21vZ2xpb0Bwb2xpdG8uaXQ=