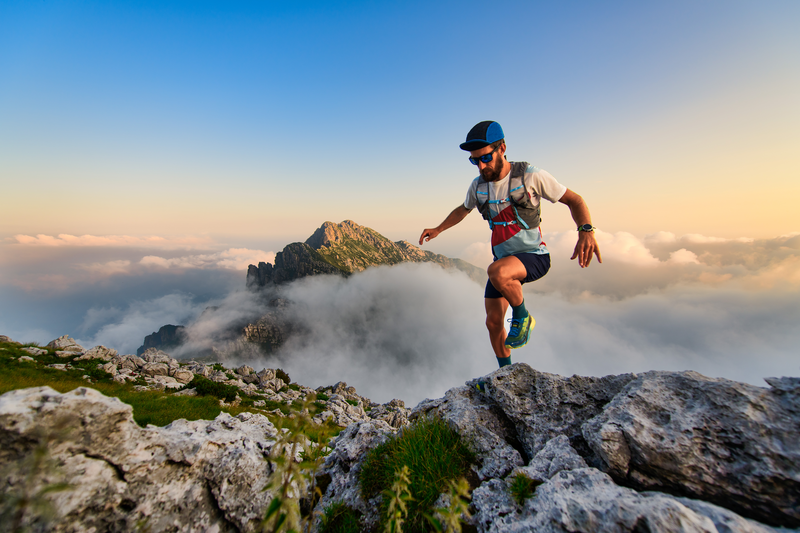
95% of researchers rate our articles as excellent or good
Learn more about the work of our research integrity team to safeguard the quality of each article we publish.
Find out more
REVIEW article
Front. Environ. Sci. , 18 September 2023
Sec. Land Use Dynamics
Volume 11 - 2023 | https://doi.org/10.3389/fenvs.2023.1175239
This article is part of the Research Topic Agricultural Land and Environmental Risks: Evidence, Assessment and Conservation Transition View all 15 articles
Cultivated land risk poses a critical threat to food security, and managing it is crucial for sustainable land use. To effectively manage this risk, it is essential to identify different types of cultivated land risk, understand their development trends, and research hotspots. This review constructs a comprehensive search strategy for subject terms in CiteSpace to analyze 12,581 literature sources related to cultivated land risk. Through tracking hot spots in cultivated land risk research, we have identified two main phases over the past 20 years. The first phase (2002–2015) focused on exploring various types of cultivated land risk, including soil, nitrogen, sewage sludge, organic matter, and carbon sequestration. Three keywords:soil, nitrogen, and sewage sludge were studied extensively during this period, with research on agricultural intensification, transport conservation, all aimed at enhancing the theoretical framework concerning cultivated land risk. The second phase (2015–2022) emphasized in-depth research into the mechanisms behind the generation of cultivated land risk. Key topics included methods and models for cultivated land risk research, source analysis, and source apportionment, as well as potentially toxic element and random forest analyses. This phase saw a shift towards a more comprehensive understanding of cultivated land risk, with a focus on uncovering underlying causes and developing effective mitigation strategies. Our research has identified three pivotal steps aimed at reducing cultivated land risk: 1) Rigorous Land Use Management: Implement stringent land use regulations to safeguard high-quality land resources. 2) Sustainable Agricultural Practices: Curtail the utilization of chemical fertilizers and pesticides, fostering improved soil fertility and minimizing environmental repercussions. 3) Robust Environmental Oversight: Establish a robust monitoring network to consistently track environmental concerns, concurrently encouraging the adoption of eco-friendly farming techniques. This comprehensive review holds substantial theoretical significance in advancing the agenda of sustainable cultivated land management and effectively alleviating the perils linked with land use alterations.
Cultivated land is an important strategic resource to ensure food security and social stability. Effective cultivated land production can make a significant contribution to solving the feeding problem of 7 billion people worldwide. However, the process of cultivated land use is inevitably accompanied by material and energy input. To obtain as much food as possible on a piece of land, producers often use a large number of pesticides and chemical fertilizers that generates serious ecological and environmental problems to both the cultivated land itself and the surrounding environment. Studies have found that global yield changes are largely controlled by fertilizer use, irrigation, and climate (Mueller et al., 2012). Cultivated land use systems are a key influence on global degradation (Foley et al., 2005; Foley et al., 2011). By 2050, land degradation and climate change are projected to combine to reduce global crop yields by an average of 10%, and in some regions by as much as 50%. It could force 50–700 million people to migrate, threatening the livelihoods of at least 3.2 billion people globally (IPBES, 2018). How to maintain sustainable high and stable food production and ensure food security while minimizing environmental impacts has been one of the century’s challenges for scholars and policymakers (Foley et al., 2011), so it is very meaningful to study the types, causes and control methods of cultivated land risk, which provides an important research basis for promoting sustainable use of cultivated land.
In an early study, Stevens et al. (1997) studied cultivation-wildlife conflicts in Western Europe, and most of its regions are characterized by agriculture as an important land use. For example, birds (Rolstad et al., 2000; Thiollay, 2006; Collard et al., 2009; Mzendah et al., 2015), wild boars (Amici et al., 2012; Hua et al., 2016; Liu et al., 2019), and insects (Forister, 2009; Cancela and Sarkar, 1996) have become important external risk factors that threaten the productive use of cultivated land. However, with the increase of urbanization and industrialization, such risks have been gradually replaced by risk types such as heavy metal pollution of soil, and a large number of studies have based on Multivariate statistical analysis, Positive Matrix Factorization receptor modeling techniques (Guan et al., 2018), Principal component analysis (Xiao et al., 2015), and Integrated Model (Marrugo-Negrete et al., 2017; Huang et al., 2018; Hu et al., 2017; Wang et al., 2019) to measure the heavy metal pollution status of cultivated land. The study of cultivated land risk gradually developed from qualitative analysis to the path of combining quantitative and qualitative analysis, but at this stage, the study of cultivated land risk still remained at the element level and failed to discover the uncoupled linear relationship between elements and systems, until Rockström et al. (2009) proposed a planetary boundary framework, and cultivated land risk studies began to focus on systematics-based studies with declining groundwater (Kong et al., 2016; Rodell et al., 2018), increasing nitrogen and phosphorus emissions (West et al., 2014; Schulte-Uebbing et al., 2022), and increasing greenhouse gas emissions (Carlson et al., 2017; Hu et al., 2020). Other external risks arising from cultivated land use systems are receiving more and more attention from scholars. In recent years, there has been a noticeable surge in the number of papers and reviews concerning cultivated land risk. However, the current research landscape on cultivated land risk exhibits fragmentation and a lack of integration with other systematic theories in land science. Additionally, there exists a deficiency in quantitative analyses of cultivated land risk, along with inadequate development of effective risk management strategies and control pathways. To address these shortcomings and advance the field, it is imperative to incorporate new models and methodologies into the study of cultivated land risk. By doing so, we can enhance the accuracy of research outcomes while also elucidating the various types and underlying causes of risk. This, in turn, will contribute to the promotion of sustainable cultivated land utilization.
Therefore, through the CiteSpace approach, this study attempts to systematically analyze the basic situation, management strategies, development trends, and hot issues of cultivated land risk research, focusing on the following issues.
(1) What are the general dynamics of cultivated land risk research?
(2) What are the salient phases and hot topics of cultivated land risk research?
(3) How has the methodology and management strategy for cultivated land risk research evolved?
(4) What are the challenges and future directions of the cultivated land risk?
The literature data in this article were obtained from the core collection of the Web of Science database (https://www.webofscience.com/, accessed on 25 November 2022). The Web of Science is an important database for accessing global academic information. It contains more than 13,000 authoritative and high-impact scholarly journals worldwide, covering a wide range of fields such as natural sciences, engineering and technology, biomedicine, social sciences, arts and humanities. The web of Science includes references cited in papers. Through the unique citation index, users can use an article, a patent number, a conference paper, a journal, or a book as search terms to retrieve their citations and easily retrace the origin and history of research literature or track its latest progress; the more extensive, newer and deeper the search, the more in-depth the search can be.
This paper analyzes the knowledge graph through CiteSpace, a data mining and visualization analysis software jointly developed by Professor Chaomei Chen of the School of Information Science and Technology at Drexel University and the WISE Lab of the Dalian University of Technology. By extracting and analyzing subjective information such as keywords, subject terms, authors, and institutions, the software can mine the underlying information and visualize the interrelationships between related information and information entities through visual knowledge graphs. The software can also reveal the development status of scientific knowledge in a certain field by showing the development trend of a certain science or knowledge field in a certain period through the convergence of related information. It is commonly used in information science, economics, sociology, and many other fields.
The accuracy and comprehensiveness of data retrieval guarantee the accuracy of CiteSpace’s operation. In practical literature retrieval, it is often relatively challenging to simultaneously ensure both accuracy and comprehensiveness. To maximize the accuracy of data analysis results, we can refer to the article published by Professor Chen Chaomei in 2017. Following his advice, the primary consideration in data retrieval should be comprehensiveness, as CiteSpace software automatically removes irrelevant or duplicate information during the data processing (to enhance accuracy). Therefore, in this paper, we established a “comprehensive search strategy for subject terms” to obtain the original data more scientifically and comprehensively, as shown in Table 1. We used the latest CiteSpace.6.1. R3, with a time slice of 1 year, and the node selection method of g-index, where k = 25/10; the choice of node type determines the purpose and main focus of our analysis using CiteSpace, in this study, to explore the regression changing characteristics of cultivated land risk development, we mainly used the keyword co-occurrence analysis method to conduct a macro visualization study of 12581 literature records to obtain the co-occurrence network, which can further discuss the development pulse of the literature.
TABLE 1. Comprehensive search rules for cultivated land risk subject terms based on Web of Science core dataset.
We assessed the number and trend of literature publications on cultivated land risk studies from 2002 to 2022 (Figure 1). Overall, the literature on cultivated land risk showed a fluctuating upward trend in the past 20 years, increasing from 122 in 2002 to 1401 in 2022. Based on the number of publications in different years, we broadly divided the research on cultivated land risk into two stages of change: 1) Steady development-steady increase stage (2002–2014), during which the literature on cultivated land risk research began to develop and show a more obvious trend of increase. 2) The rapid development-stabilization phase (2014–2022), during which the literature on cultivated land risk has increased rapidly and different countries, research institutions and scholars have started to focus on the impact of cultivated land risk from different research perspectives, and have conducted a series of research works. While there is a substantial disparity in the number of articles published in “Review” and “Article,” it is evident that the number of articles published in “Review” has been steadily increasing year by year. Starting with just 8 articles, this number has grown to 87 articles in 2022, representing an almost 91% increase. This trend suggests a significant rise in the quantity of reviews and articles addressing cultivated land risk.
Based on the Web of Science core dataset, we found that the top ten countries conducting the most research on cultivated land risk by the end of 2022 are China, the United States, the United Kingdom, Germany, Australia, Italy, Canada, Spain, France, and New Zealand (Table 2). Among them, China, the United States, the United Kingdom, and Germany all have more than 1000 publications, with China having the largest number of publications at about 3204, followed by the United States with about 2663, but the United States has the highest intermediary centrality at about 0.18, indicating that the United States collaborates more frequently with other countries in publishing environmental risk literature; although China has the largest number of publications, its intermediary centrality is the smallest at 0.02, indicating that Chinese researchers are more inclined to intra-national communication and cooperation when publishing relevant literature. The Sigma metric reveals substantial discrepancies between the UK and the US compared to other nations. Remarkably, the Sigma value for the UK surpasses that of all other countries, with a notable threefold disparity compared to the US, measuring around 24.36. The Sigma value for the US stands at approximately 8.21. In contrast, the remaining countries exhibit Sigma values ranging between 1.0 and 2.1. Despite the UK publishing only half the number of articles compared to the US, this discrepancy underscores the superior quality of the UK’s research contributions in the realm of cultivated land systemic risk. This observation underscores that the UK outperforms other nations not only in terms of publication quality but also in terms of innovation. This exceptional performance can potentially be attributed to the collaborative nature of research endeavors between the UK, the US, and international researchers. This emphasis on cross-border collaboration likely contributes to their capacity to attain innovative outcomes in their investigation of arable land risk, as evidenced by the centrality indicator.
The construction of the disciplinary analysis network is based on the dual map overlay of CiteSpace software, and the consequences of the dual map overlay show the position of the cultivated land risk relative to the main research disciplines. The dual-map overlay analysis provides readers with an overarching view of the fields encompassed by the research direction. It allows us to observe the disciplinary domains within which the citing and cited journals are situated. This approach is particularly useful in identifying potential interdisciplinary intersections within the research direction. The z-scores function highlights stronger and smoother trajectories, and higher index values are represented by thicker lines. It can be seen that publications in the field of “ecology, earth, ocean” (blue trajectory) are mainly dominated by “botany, ecology, zoology (z = 6.97, f = 17338)”, “environment, toxicology, nutrition (z = 2.97, f = 17338)”, “environmental science, toxicology, and the environment, Nutrition (z = 2.72, f = 7309)”, “Earth, Geology, Geophysics (z = 3.42, f = 8979)”, and “Economics, Policy (z = 2.05, f = 5712)”. In addition, publications in the field of “Veterinary medicine, animals, science (yellow trajectory)” were mainly influenced by “Botany, ecology, zoology (z = 4.45, f = 11383)”, “Environment, toxicology, nutrition (z = 3.57, f = 9308)”. It can be seen that cultivating land risk research involves several subject areas. On the whole, cultivated land risk research is based on ecology, geology, environmental science, botany and other disciplines, and applied to ecology, earth, ocean, animal and other disciplines, which has strong interdisciplinary nature and is conducive to the integration of multidisciplinary resources and easy to achieve original and major scientific breakthroughs (Figure 2).
FIGURE 2. Subject Analysis Network of environmental risk research literature based on the dual map overlay.
The core of the analysis is the condensation of keywords. CiteSpace can visualize the keywords and generate a keyword clustering map, based on which the cluster number is the y-axis and the year of citation publication is the x-axis, the timeline map of keyword clustering can be laid out (Figure 3). The timeline map can show the time span and the research process in the development and evolution of each cluster (i.e., subfield) over the period of 2002–2022. The larger the number of the cluster’s serial number, the fewer keywords are contained in that cluster, and conversely, the smaller the number, the more keywords are contained in that cluster. In order to assess the reliability of the bibliographic results. “Modularity value Q)" and “weighted average profile value S)" was set as the evaluation indicators in CiteSpace. It is generally considered that a modularity value Q) > 0.3 and a weighted average profile value S) > 0.7 indicate high reliability of the results (Chen, 2017; Chen et al., 2020), and based on the timeline mapping, it can be seen that the modularity value of the network is 0.4192 and the weighted average profile value is 0.7396, which can be considered as very high, indicating that the cultivated land risk study is well defined in terms of co-citation clustering. The overall complexity of the clustering network indicates that there are still more links between the subcategories of distinct clusters. The timeline diagram shows that the clusters related to the study of cultivated land risk mainly include eight categories: heavy metal, climate change, conservation, water quality, carbon sequestration, remote sensing, land degradation and resource use efficiency. The cluster “heavy metal” has the smallest number and contains the most keywords, which is the largest cluster in the study of cultivated land risk, while “resource use efficiency” is the smallest cluster. These clusters encompass not only the factors contributing to cultivated land risk but also encompass the various risk categories, research methodologies, and prevalent research focal points. Noteworthy within these are ecological risks, ecological risk assessment, greenhouse gas emissions, land expansion, sustainable intensification, and more—each of which holds a significant presence in prominent academic journals.
Remote sensing stands out as a prominent approach in investigating cultivated land risk, offering a plethora of tools. The utilization of remote sensing techniques initially focused on basin-scale assessments, employing classification methods to discern and delve into cultivated land risk. Since 2014, the application of sensitivity analysis, indices, time series analysis, and random forest methods has notably expanded, making substantial contributions to the study of cultivated land risk. These methodologies not only enhance our comprehension of the subject but also lay the groundwork for more refined and comprehensive research endeavors.
Keyword emergence refers to a significant increase in the frequency of keywords in a certain time span, expressed by strength, and the larger the strength value, the higher the emergence intensity, indicating that the keywords are more influential and the hot words in the field of research in that time span (Chen et al., 2009; Chen et al., 2010). The red line in the table indicates the year in which the mutation occurred in the keyword, and the blue line indicates the year in which there was no mutation (Table 3). Based on the CiteSpace hotspot analysis tool we detected the keywords with mutation intensity in the top 20 and sorted them by the year of mutation. We found that mutation words have more obvious phase characteristics. In the first phase (2002–2015), the main types of cultivated land risk explored in this phase were main elements: soil, nitrogen, sewage sludge, organic matter, carbon sequestration, etc. Among them, soil, nitrogen, and sewage sludge these three keywords have a long mutation time, spanning from 2002 to 2013. In the past 11 years, the research on the theme of cultivated land risk has been analyzed mainly from three aspects, and we can also see that the words agricultural intensification, transport conservation, and system also become strong mutations in this phase, indicating that the research on the theoretical framework related to cultivated land risk is also gradually strengthened and begins to focus on the drivers and driving factors of cultivated land risk. In the second phase (2015–2022), this phase focuses on cultivated land risk more on the mechanism behind the generation of cultivated land risk for in-depth research, involving methods and models for cultivated land risk research, source analysis, etc., and source apportionment, potentially toxic element, random forest, etc. have been the hot and key topics of cultivated land risk research in the past 5 years.
Mapping of institutional collaboration networks can tell us about the links between institutions and the contribution of each institution in the field of cultivated land risk research, which helps us to identify researchers and institutions that deserve attention. By doing a network analysis of the major research institutions and cultivated land risk research, we found that cultivated land risk research is widely followed in 39 countries and 47 research institutions worldwide (Figure 4). Universities and research institutions have relatively close ties and collaboration. There are ten institutions with more than 100 articles. The Chinese Academy of Sciences occupies a central position in the collaborative network in the field of arable land risk research, with Wageningen University, Beijing Normal University, China Agricultural University, Zhejiang University, University of California, Davis, Agriculture and Agri-Food Canada, Chinese Academy of Agricultural Sciences, and Northwest Agriculture and Forestry University as important links in the network of collaborating institutions. In addition, more fruitful work has been published by research institutions such as Harvard University, Michigan State University, University of Zurich, French National Institute of Agricultural Research, USDA Agricultural Research Service, Illinois State University, and Aarhus University.
As the study of cultivated land risk continues to increase, its connotation becomes richer and richer. In diverse agricultural production and ecosystems around the world, the research methods and control strategies also vary greatly in response to the multidimensional nature of the research scale and the diversity of risk types (Table 4). Current research on cultivated land risk focuses on large scales (world or regional scales), and the types of risks studied at these scales are mainly biodiversity loss, wildlife habitat degradation, etc. Green et al. (2005) used a data analysis approach to assess the risk to wildlife habitat arising from cultivated land use using the FAO bird database, and by examining studies in developing countries suggest that highly productive farming practices may allow for the presence of more species. Contrary to our perception, there does not necessarily represent a trade-off between high yield and maintaining biodiversity, and we can maintain species diversity while maintaining high yields. The study by Mueller et al. (2012) also suggested that sustainable intensification can be effective in achieving control of cultivated land risks. Except for heavy metal pollution (Jiang et al., 2017; Liang et al., 2017; Guan et al., 2018), more effective control measures exist for all other cultivated land risk types, because the problem of soil heavy metal pollution is influenced not only by the process of cultivated land use, but also by regional resource background conditions and industrial and other processes (Chen et al., 2023). Gradually, in order to better assess the impact of cultivated land risk, scholars have slowly focused on characterizing the degree of impact of cultivated land risk through elements such as soil degradation (Lal, 2004), greenhouse gas emissions (Searchinger et al., 2008; Godfray et al., 2010; Foley et al., 2011; Tilman and Clark, 2014; Steffen et al., 2015), freshwater use (Godfray et al., 2010; Steffen et al., 2015), and nitrogen and phosphorus emissions (Steffen et al., 2015). The control strategies for cultivated land risk are also diversified, and carbon sequestration technology can effectively deal with greenhouse gas emissions, while reducing food waste and changing dietary habits have also become effective ways to reduce the risk of cultivated land. The development of planetary boundary framework (Rockström et al., 2009) has shifted the study of cultivated land risk from elemental to systemic research, and scholars at home and abroad have gained a deep understanding and explored the coupled nonlinear relationship between cultivated land use system and elements.
TABLE 4. Global research on the topic of cultivated land risks, including risk types, causes and control strategies.
Since the industrial revolution, human activities have become a major driver of global environmental change, and human activities can lead to an Earth system outside the stable environmental state of the Anthropocene, with threatening and even catastrophic consequences for much of the world (Crutzen, 2002; FU et al., 2006). To meet the challenge, the planetary boundary framework was formally proposed by Rockström et al. (2009), Center for Resilience Research, Stockholm University, Sweden, and has elicited a strong response from scientists worldwide (Dearing et al., 2014; Mace et al., 2014). The framework focuses on key biophysical processes of the Earth, which have evolved and now identify nine processes such as climate change, biosphere integrity, stratospheric ozone depletion, ocean acidification, biogeochemical flows (nitrogen and phosphorus cycles), land system changes, freshwater use, atmospheric aerosol loading, and the introduction of new entities (Steffen et al., 2015), defining the relative boundaries for human operations and security within which humans can safely operate in relation to the Earth system. The framework argues that these boundaries, if crossed, have a high potential to trigger irreversible nonlinear changes in the state of the Earth system, with consequent adverse effects on human wellbeing. This provides an important methodological reference for quantifying the magnitude of the impact of external risks on cultivated land.
The planetary boundary framework is based on the theory of homeostasis and mutation of complex systems and is centered on maintaining the stability of the Earth’s ecosystem for human wellbeing. To maintain system homeostasis, the stable resource and environmental conditions during the Holocene geological period before the industrial revolution are used as a reference to analyze the variables of the Earth’s key biophysical processes, determine the critical thresholds of the variables, and provide early warnings based on the critical thresholds to ensure that changes in the system state receive early attention and preventive measures are taken. The planetary boundaries represent a relatively conservative estimate of critical thresholds, which are positioned at the lower end of the uncertainty range. The current threshold interval of planetary boundaries consists of three parts: first, the safe operating space, in which human activities have basically no impact on the stability of the Earth system; second, the uncertainty region, which has exceeded the planetary boundaries, is the region where the risks to the Earth system beyond this region have not yet been quantified and cannot be analyzed; third, in the high-risk region, human activities significantly exceed planetary boundaries and may cause irreversible effects on the Earth system environment (Figure 5A).
FIGURE 5. A cultivated land use security boundary system constructed based on the earth system boundaries framework.
Currently, Rockström et al. (2009) and Steffen et al. (2015) have proposed and refined the planetary boundary framework, offering the possibility to study the effects of different subjects on the Earth system at the global scale. Newbold et al. (2016) explored the impact of land use and related stressors on local biodiversity based on the planetary boundary framework, quantifying the extent of biodiversity destruction due to the effects of cultivated land use and beyond the safe boundary of the environment. Springmann et al. (2018) applied the framework to food production systems and analyzed the safety boundaries of climate change, land use system changes, freshwater use, and nitrogen and phosphorus loss in biogeochemical flows in food production systems. Lade et al. (2020) based on the planetary boundary framework, argued that there are interlinked relationships among planetary boundaries, i.e., planetary boundaries affect each other and interact with each other, and carefully analyzed the effects of land use changes on planetary boundaries such as nitrogen, phosphorus, water and greenhouse gases. Cultivated land use is an important component of land use, and changes in cultivated land use will also have significant effects on climate change, freshwater use, and nitrogen and phosphorus loss in biogeochemical flows.
In summary, the planetary boundary model allows for the multidimensional quantification of factors influencing cultivated land risk. Anchored in fundamental logical perspectives and coupled with downscaling methodologies, the global-scale model can be downscaled to encompass larger regions, nations, and even smaller scales. Drawing insights from the research achievements of relevant scholars (Rockström et al., 2009; Steffen et al., 2015; Hu et al., 2020; Lade et al., 2020), we comprehensively construct the planetary boundary framework (Figure 5B) for cultivated land use systems. This framework serves as a theoretical guide for future investigations into cultivated land risk, rooted deeply in the principles of planetary boundary theory. Looking ahead, we anticipate the significant theoretical guidance this comprehensive endeavor will offer to forthcoming risk assessments within cultivated land systems, grounded in the foundations of planetary boundary theory.
In the 1960s, in order to solve the problem of severe soil erosion and massive soil erosion and to avoid environmental degradation caused by land use, the United States was the first to propose the Evaluation of Land Resource Production Potential, to classify land use potential, to classify eight soil potential classes according to soil, slope, erosion type and erosion intensity, and to develop a more complete soil map for soil and water conservation purposes (Klingebiel and Montgomery, 1961; Zhu, 1997). Best Management Practices (BMPs) were introduced in the 1970s in the United States, and the core of the BMPs is the use of a combination of measures to control agricultural pollution from cultivated land use, which has achieved good results (Bracmort et al., 2006; Reimer et al., 2012). A system of pesticide business license, pest control consultation, and restricted level pesticide application license has been established (Guo et al., 2015). The BMPs includes three levels of nutrient management practices, tillage management practices, and landscape management practices, and each state government has developed detailed rules and practices and formed corresponding testing and management agencies. In 1985, the U.S. Congress passed the “Food Safety and Security Act,” and the following year initiated a nationwide 10–15-year ecological restoration program for cultivated land (Wu et al., 2019). This program aimed to systematically remediate cultivated land with severe ecological issues. The U.S. government also introduced fallow and land protection programs to safeguard environmentally vulnerable lands and promote ecological restoration through fallowing (Sullivan et al., 2004; Stubbs, 2013). Additionally, a series of conservation programs were implemented, effectively preserving high-quality cultivated land (Jiang et al., 2019; Ke, 2001).
The EU has devised policies addressing environmental risks in agriculture, demonstrating a profound concern for the environmental condition of the agricultural sector (Dobbs and Pretty, 2008; Vannini et al., 2008), which define the two pillars of the EU agricultural policy, including subsidies for ecologically fragile areas to reduce the use of harmful fertilizers and pesticides, as well as subsidies for afforestation, and encouraging the development of multifunctional roles of agriculture. In 2003, the EU reformed its agricultural policy, with a focus on the introduction of the decoupled “Single Farm Payment” (SFP). In 2003, the EU reformed its agricultural policy, and one of the highlights of the reform was the introduction of the decoupled “Single Farm Payment” (SFP) (Fraser, 2003; Wang, 2009), where agricultural land use must meet a series of criteria related to soil conservation, soil organic matter maintenance, habitat avoidance and water management in order to receive agricultural subsidies. This provides a solid basis for agricultural land risk control in the EU. In order to effectively monitor the presence of soil erosion, soil ecological degradation, soil heavy metal contamination, soil compaction and soil salinization in each country, the Soil Environmental Assessment Health Project was introduced in the EU in 2007 (Kibblewhite et al., 2007). The project identified nine major threats affecting soil quality in Europe as soil erosion, declining organic matter content, soil pollution, soil compaction, soil salinization, declining biodiversity, soil sealing, landslides, and desertification. Based on their threat level, a minimum data set of 27 key issues and 27 monitoring indicators that can encompass all threats were finally selected to form the monitoring indicators. The EU’s farm and soil environmental policy has, to a certain extent, effectively managed the environmental risks caused by the current agricultural production process and provided a guarantee for the sustainable development of EU agriculture.
The Japanese government attached great importance to agricultural land and arable land, and enacted many ordinances aimed at strengthening the management and protection of cultivated land. After the establishment of the peasant land ownership system in post-war Japan, a strict control policy on high-quality arable land was implemented, stipulating that all types of land could not be freely transferred. The rapid economic development of Japan in the 1960s and 1970s led to an increase in agricultural inputs, especially the extensive use of chemical fertilizers and pesticides. Consequently, this not only adversely affected the ecological environment but also had a negative impact on the quality of agricultural products (Qian et al., 2016). In 1992, the Ministry of Agriculture, Forestry and Fisheries of Japan passed the New Food/Agriculture/Rural Policy Act, which established the “Basic Policy for the Promotion of Environmentally Friendly Agriculture” to reduce the use of pesticides and chemical fertilizers by improving soil fertility and promoting organic farming and pesticide- and fertilizer-free cultivation to achieve sustainable agricultural production. The Basic Law for Food Agriculture and Rural Development, enacted in 1999, promotes environmentally friendly agriculture throughout the country, taking measures such as promoting the use of compost while reducing chemical fertilizers, promoting eco-farmer certification and giving interest-free loan support for agricultural improvement funds (Ma and Mao, 2019). In the 21st century, Japan has enacted policies and measures such as the Agricultural Environmental Code, the Organic Farming Promotion Act, and the Measures to Protect and Enhance Agricultural Land, Water, and the Environment, aiming to improve soil, reduce the intensity of chemical fertilizer and pesticide inputs, increase biodiversity, reduce environmental loads, achieve sustainable use of agricultural production, and ensure food safety.
The cultivated land risk control policies implemented in the United States, Japan, and Europe underscore several key recommendations for achieving sustainable development of cultivated land and mitigating associated risks. These recommendations can be summarized as follows.
(1) Rigorous Land Use Control: The first crucial recommendation involves the strict control of cultivated land, which includes prohibiting arbitrary changes in land use and giving priority to the protection of high-quality cultivated land.
(2) Enhancing Soil Fertility: The second recommendation centers on improving soil fertility. This entails the strict regulation of chemical fertilizers and pesticides use to reduce environmental impacts and prevent soil degradation.
(3) Regular Environmental Monitoring: The third recommendation underscores the importance of establishing regular environmental monitoring systems. This comprehensive network should be complemented by incentives for agricultural producers adopting eco-friendly practices.
(4) Positive International Impacts: Acknowledging the positive effects of the risk control policies of the United States, Japan, and Europe on cropland risk control worldwide.
(5) Global Research and Collaboration: The need for enhanced scientific research in cultivated land risk control, the development of unified and coordinated policies, the creation of risk control mechanisms and institutions, and the promotion of sustainable land resource management on a global scale is emphasized.
In summary, these recommendations emphasize the importance of taking proactive measures to protect cultivated land, improve soil quality, monitor environmental conditions, and glean valuable insights from successful international policies. This approach is aimed at promoting and ensuring sustainable land use practices worldwide.
The sustainable use of cultivated land is crucial for supporting future food production. However, risks to cultivated land pose a significant challenge to achieving sustainable use. Therefore, it is essential to scientifically quantify the various risks and identify ways to prevent or manage them. However, due to the wide variety and partial difficulty in quantifying the types of risks, it is challenging to guide the sustainable use of cultivated land. The planetary boundary framework provides a good paradigm for quantifying the impact of each element on the Earth system. However, finding the boundaries of the Earth system related to cultivated land is an important issue for future research. Scholars have made efforts to quantify the relevant boundaries of cultivated land use, but the cultivated land system is a complex human-natural complex, and further research is needed to quantify the relevant boundaries and the inter-boundary relationships, scale effects, and driving mechanisms. Future studies can refer to the planetary boundary framework to explore the effects of changes in cultivated land use on planetary boundary indicators such as climate change, freshwater use, and nitrogen and phosphorus loss in biogeochemical flows. By applying this framework to the cultivated land utilization system, we can quantify the environmental risk thresholds in a scientific way and have control objectives in the regulation of the cultivated land utilization system, thus realizing the sustainable utilization of cultivated land resources.
The implementation of cultivated land risk control mainly involves two mechanisms: the government mechanism and the social mechanism. The government mechanism plays a leading and supervisory role in risk management, while the social mechanism involves the owners and direct users of the cultivated land use system. To achieve effective cultivated land risk management, it is necessary to guide these two mechanisms to work together and build a main system of cultivated land risk management. Furthermore, there is a need for debate and discussion to develop pathways for sustainable cultivated land risk research and utilization. The objectives of cultivated land risk research in the new era should focus on transformative ecological governance, which involves stakeholder and institutional changes and methodological changes to proactively improve the degraded cultivated land use system. This will build a system of cultivated land risk research with multi-interest synergy, multiple resource integration, and full-cycle regulation to ensure social response matches the changing cultivated land use system. This will narrow the sustainable development gap of the cultivated land use system and regulate it back to a safe operating space, thus realizing the sustainable use of cultivated land resources and fair and just human social development.
Data curation: ZZ, ML, LW, and EX; formal analysis: ZZ, ML, and LW; methodology: ZZ, ML, LW, EX, and XK; writing—original draft: ZZ and ML; writing—review and editing: ZZ, ML, LW, EX, and XK. All authors contributed to the article and approved the submitted version.
This work was supported by the Chinese Social Science Foundation (19ZDA096), the Chinese National Natural Science Foundation (42171289 and 42201285). China Postdoctoral Science Foundation (2023M733781); Ministry of Science and Technology of the People’s Republic of China (2021FY100403).
The authors declare that the research was conducted in the absence of any commercial or financial relationships that could be construed as a potential conflict of interest.
All claims expressed in this article are solely those of the authors and do not necessarily represent those of their affiliated organizations, or those of the publisher, the editors and the reviewers. Any product that may be evaluated in this article, or claim that may be made by its manufacturer, is not guaranteed or endorsed by the publisher.
Amici, A., Serrani, F., Rossi, C. M., and Primi, R. (2012). Increase in crop damage caused by wild boar (sus scrofa l.): The "refuge effect. Agron. Sustain. Dev. 32, 683–692. doi:10.1007/s13593-011-0057-6
Benton, T. G., Vickery, J. A., and Wilson, J. D. (2003). Farmland biodiversity: Is habitat heterogeneity the key? Trends Ecol. Evol. 18, 182–188. doi:10.1016/s0169-5347(03)00011-9
Bracmort, K. S., Arabi, M., Frankenberger, J. R., Engel, B. A., and Arnold, J. G. (2006). Modeling long-term water quality impact of structural bmps. Trans. Asabe 49, 367–374. doi:10.13031/2013.20411
Cancela, J. P., and Sarkar, S. (1996). On the evaluation of spatial diversity of soil microarthropod communities. Eur. J. Soil Biol. 32, 131–140.
Carlson, K. M., Gerber, J. S., Mueller, N. D., Herrero, M., MacDonald, G. K., Brauman, K. A., et al. (2017). Greenhouse gas emissions intensity of global croplands. Nat. Clim. Change 7, 63–68. doi:10.1038/NCLIMATE3158
Chen, C. (2020). A glimpse of the first eight months of the covid-19 literature on microsoft academic graph: Themes, citation contexts, and uncertainties. Front. Res. Metrics Anal. 5, 607286. doi:10.3389/frma.2020.607286
Chen, C., Chen, Y., Hou, J., and Liang, Y. (2009). Citespace ⅱ:detecting and visualizing emerging trends and transient patterns in scientific literature. J. China Soc. Sci. Tech. Inf. 28, 401–421.
Chen, C., Ibekwe-SanJuan, F., and Hou, J. (2010). The structure and dynamics of co-citation clusters: A multiple-perspective co-citation analysis. JASIST 61 (7), 1386–1409. doi:10.1002/asi.21309
Chen, C. M. (2004). Searching for intellectual turning points: Progressive knowledge domain visualization. Proc. Natl. Acad. Sci. U. S. A. 101, 5303–5310. doi:10.1073/pnas.0307513100
Chen, C. M., and Song, M. (2019). Visualizing a field of research: A methodology of systematic scientometric reviews. Plos One 14, e0223994. doi:10.1371/journal.pone.0223994
Chen, C. (2017). Science mapping: A systematic review of the literature. J. Data Inf. Sci. 2, 1–40. doi:10.1515/jdis-2017-0006
Chen, Z., Zhao, Y., Chen, D., Huang, H., Zhao, Y., Wu, Y., et al. (2023). Ecological risk assessment and early warning of heavy metal cumulation in the soils near the luanchuan molybdenum polymetallic mine concentration area, henan province, central China. China Geol. 6, 15–26. doi:10.31035/cg2023003
Collard, S., Le Brocque, A., and Zammit, C. (2009). Bird assemblages in fragmented agricultural landscapes: The role of small brigalow remnants and adjoining land uses. Biodivers. Conservation 18, 1649–1670. doi:10.1007/s10531-008-9548-4
Dearing, J. A., Wang, R., Zhang, K., Dyke, J. G., Haberl, H., Hossain, M. S., et al. (2014). Safe and just operating spaces for regional social-ecological systems. Glob. Environ. Change-Human Policy Dimensions 28, 227–238. doi:10.1016/j.gloenvcha.2014.06.012
Dobbs, T. L., and Pretty, J. (2008). Case study of agri-environmental payments: The United Kingdom. Ecol. Econ. 65, 765–775. doi:10.1016/j.ecolecon.2007.07.030
Fader, M., Gerten, D., Krause, M., Lucht, W., and Cramer, W. (2013). Spatial decoupling of agricultural production and consumption: Quantifying dependences of countries on food imports due to domestic land and water constraints. Environ. Res. Lett. 8, 014046. doi:10.1088/1748-9326/8/1/014046
Foley, J. A., DeFries, R., Asner, G. P., Barford, C., Bonan, G., Carpenter, S. R., et al. (2005). Global consequences of land use. Science 309, 570–574. doi:10.1126/science.1111772
Foley, J. A., Ramankutty, N., Brauman, K. A., Cassidy, E. S., Gerber, J. S., Johnston, M., et al. (2011). Solutions for a cultivated planet. Nature 478, 337–342. doi:10.1038/nature10452
Forister, M. L. (2009). Anthropogenic islands in the arid west: comparing the richness and diversity of insect communities in cultivated fields and neighboring wildlands. Environ. Entomol. doi:10.1603/022.038.0410
Fraser, R. (2003). An evaluation of the compensation required by European Union cereal growers to accept the removal of price support. J. Agric. Econ. 54, 431–445. doi:10.1111/j.1477-9552.2003.tb00070.x
Fu, C., An, Z., Qiang, X., Song, Y., and Ghang, H. (2006). Strategy, challenge and progress of global change science. Arid Zone Res. 23, 1–7.
Godfray, H., Beddington, J. R., Crute, I. R., Haddad, L., Lawrence, D., Muir, J. F., et al. (2010). Food security: The challenge of feeding 9 billion people. Science 327, 812–818. doi:10.1126/science.1185383
Green, R. E., Cornell, S. J., Scharlemann, J., and Balmford, A. (2005). Farming and the fate of wild nature. Science 307, 550–555. doi:10.1126/science.1106049
Guan, Q. Y., Wang, F. F., Xu, C. Q., Pan, N. H., Lin, J. K., Zhao, R., et al. (2018). Source apportionment of heavy metals in agricultural soil based on pmf: A case study in hexi corridor, northwest China. Chemosphere 193, 189–197. doi:10.1016/j.chemosphere.2017.10.151
Guo, H, Xu, B, Liu, C, and Shu, K (2015). Pesticide and fertilizer regulation: American experiences and enlightenment. Environ. Prot. 43, 64–69. doi:10.14026/j.cnki.0253-9705.2015.21.016
Hu, W. Y., Wang, H. F., Dong, L. R., Huang, B. A., Borggaard, O. K., Bruun Hansen, H. C., et al. (2018). Source identification of heavy metals in peri-urban agricultural soils of southeast China: An integrated approach. Environ. Pollut. 237, 650–661. doi:10.1016/j.envpol.2018.02.070
Hu, Y. C., Su, M. R., Wang, Y. F., Cui, S. H., Meng, F. X., Yue, W., et al. (2020). Food production in China requires intensified measures to be consistent with national and provincial environmental boundaries. Nat. Food 1, 572–582. doi:10.1038/s43016-020-00143-2
Hua, X. B., Yan, J. Z., Li, H. L., He, W. F., and Li, X. B. (2016). Wildlife damage and cultivated land abandonment: findings from the mountainous areas of Chongqing, China. Crop Prot. 84, 141–149. doi:10.1016/j.cropro.2016.03.005
Huang, J. H., Guo, S. T., Zeng, G. M., Li, F., Gu, Y. L., Shi, Y., et al. (2018). A new exploration of health risk assessment quantification from sources of soil heavy metals under different land use. Environ. Pollut. 243, 49–58. doi:10.1016/j.envpol.2018.08.038
IPBES (2018). Media release: Worsening worldwide land degradation now ‘critical’, undermining well-being of 3.2 billion people. Available at: https://ipbes.net/news/media-release-worsening-worldwide-land-degradation-now-%E2%80%98critical%E2%80%99-undermining-well-being-32.
Jiang, Y. X., Chao, S. H., Liu, J. W., Yang, Y., Chen, Y. J., Zhang, A., et al. (2017). Source apportionment and health risk assessment of heavy metals in soil for a township in jiangsu province, China. Chemosphere 168, 1658–1668. doi:10.1016/j.chemosphere.2016.11.088
Jiang, Z, Zhang, H, and Duan, Z (2019). The establishment of U. S. Risk management system and its impact: With observations on the new 2018 U. S. Agriculture Act development. Issues Agric. Econ. 475, 134–144. doi:10.13246/j.cnki.iae.2019.07.014
Jiang, Z., Huijie, Z., and Zhihuang, D. (2019). The establishment of U. S. risk management system and its impact: with observations on the new 2018 U. S. Agriculture Act Development. Issues in Agricultural Economy 475, 134–144. doi:10.13246/j.cnki.iae.2019.07.014
Ke, B. (2001). U.S. Agricultural risk management policy and implications. Rome, Italy: World Agriculture, 11–13.
Kibblewhite, M., Rubio, J. L., Kosmas, C., Jones, R., and Verheijen, F. (2007). Environmental assessment of soil for monitoring desertification in europe.
Klingebiel, A. A., and Montgomery, P. H. (1961). Land-capability classification. State of New Jersey: Soil Conservation Service.
Kong, X., Zhang, X., Lal, R., Zhang, F., Chen, X., Niu, Z., et al. (2016). “Chapter Two - Groundwater Depletion by Agricultural Intensification in China's HHH Plains, Since 1980s,” in Advances in Agronomy. Editors D. L. Sparks: (Academic Press), 59–106. doi:10.1016/bs.agron.2015.09.003
Krebs, J. R., Wilson, J. D., Bradbury, R. B., and Siriwardena, G. M. (1999). The second silent spring? Nature 400, 611–612. doi:10.1038/23127
Lade, S. J., Steffen, W., de Vries, W., Carpenter, S. R., Donges, J. F., Gerten, D., et al. (2020). Human impacts on planetary boundaries amplified by earth system interactions. Nat. Sustain. 3, 119–128. doi:10.1038/s41893-019-0454-4
Lal, R. (2004). Soil carbon sequestration impacts on global climate change and food security. Science 304, 1623–1627. doi:10.1126/science.1097396
Lambin, E. F., and Meyfroidt, P. (2011). Global land use change, economic globalization, and the looming land scarcity. Proc. Natl. Acad. Sci. U. S. A. 108, 3465–3472. doi:10.1073/pnas.1100480108
Liang, J., Feng, C. T., Zeng, G. M., Gao, X., Zhong, M. Z., Li, X., et al. (2017). Spatial distribution and source identification of heavy metals in surface soils in a typical coal mine city, lianyuan, China. Environ. Pollut. 225, 681–690. doi:10.1016/j.envpol.2017.03.057
Liu, Q., Yan, K., Lu, Y. F., Li, M., and Yan, Y. Y. (2019). Conflict between wild boars (sus scrofa) and farmers: Distribution, impacts, and suggestions for management of wild boars in the three gorges reservoir area. J. Mt. Sci. 16, 2404–2416. doi:10.1007/s11629-019-5453-4
Ma, H, and Mao, S (2019). Comparison of the transformation paths of agricultural support policies in Japan and the EU and insights. J. Huazhong Agric. Univ. Sci. Ed. 143, 46–53. doi:10.13300/j.cnki.hnwkxb.2019.05.006
Mace, G. M., Reyers, B., Alkemade, R., Biggs, R., Chapin, F. S., Cornell, S. E., et al. (2014). Approaches to defining a planetary boundary for biodiversity. Glob. Environ. Change-Human Policy Dimensions 28, 289–297. doi:10.1016/j.gloenvcha.2014.07.009
Marrugo-Negrete, J., Pinedo-Hernandez, J., and Diez, S. (2017). Assessment of heavy metal pollution, spatial distribution and origin in agricultural soils along the sinu river basin, Colombia. Environ. Res. 154, 380–388. doi:10.1016/j.envres.2017.01.021
Mueller, N. D., Gerber, J. S., Johnston, M., Ray, D. K., Ramankutty, N., and Foley, J. A. (2012). Closing yield gaps through nutrient and water management. Nature 490, 254–257. doi:10.1038/nature11420
Mzendah, C. M., Mashapa, C., Magadza, C., Gandiwa, E., and Kativu, S. (2015). Bird species diversity across a gradient of land use in southern gonarezhou national park and adjacent areas, Zimbabwe. J. Animal Plant Sci. 25, 1322–1328.
Newbold, T., Hudson, L. N., Arnell, A. P., Contu, S., De Palma, A., Ferrier, S., et al. (2016). Has land use pushed terrestrial biodiversity beyond the planetary boundary? A global assessment. Science 353, 288–291. doi:10.1126/science.aaf2201
Newbold, T., Hudson, L. N., Hill, S., Contu, S., Lysenko, I., Senior, R. A., et al. (2015). Global effects of land use on local terrestrial biodiversity. Nature 520, 45–50. doi:10.1038/nature14324
Phalan, B., Onial, M., Balmford, A., and Green, R. E. (2011). Reconciling food production and biodiversity conservation: Land sharing and land sparing compared. Science 333, 1289–1291. doi:10.1126/science.1208742
Qian, X., Yin, C., and Fang, L. (2016). Inspiration of agricultural environment support policies of Japan, EU and America to China. Chin. J. Agric. Resour. Regional Plan. 37, 35–44. doi:10.7621/cjarrp.1005-9121.20160706
Reimer, A. P., Weinkauf, D. K., and Prokopy, L. S. (2012). The influence of perceptions of practice characteristics: An examination of agricultural best management practice adoption in two Indiana watersheds. J. Rural Stud. 28, 118–128. doi:10.1016/j.jrurstud.2011.09.005
Robinson, R. A., and Sutherland, W. J. (2002). Post-war changes in arable farming and biodiversity in great britain. J. Appl. Ecol. 39, 157–176. doi:10.1046/j.1365-2664.2002.00695.x
Rockstrom, J., Steffen, W., Noone, K., Persson, A., Chapin, F. S., Lambin, E. F., et al. (2009). A safe operating space for humanity. Nature 461, 472–475. doi:10.1038/461472a
Rodell, M., Famiglietti, J. S., Wiese, D. N., Reager, J. T., Beaudoing, H. K., Landerer, F. W., et al. (2018). Emerging trends in global freshwater availability. Nature 557, 651–659. doi:10.1038/s41586-018-0123-1
Rolstad, J., Loken, B., and Rolstad, E. (2000). Habitat selection as a hierarchical spatial process: The green woodpecker at the northern edge of its distribution range. Oecologia 124, 116–129. doi:10.1007/s004420050031
Schulte-Uebbing, L. F., Beusen, A. H. W., Bouwman, A. F., and de Vries, W. (2022). From planetary to regional boundaries for agricultural nitrogen pollution. Nature 610, 507–512. doi:10.1038/s41586-022-05158-2
Searchinger, T., Heimlich, R., Houghton, R. A., Dong, F. X., Elobeid, A., Fabiosa, J., et al. (2008). Use of us croplands for biofuels increases greenhouse gases through emissions from land-use change. Science 319, 1238–1240. doi:10.1126/science.1151861
Springmann, M., Clark, M., Mason-D’Croz, D., Wiebe, K., Bodirsky, B. L., Lassaletta, L., et al. (2018). Options for keeping the food system within environmental limits. Nature, 562–519. doi:10.1038/s41586-018-0594-0
Steffen, W., Richardson, K., Rockstrom, J., Cornell, S. E., Fetzer, I., Bennett, E. M., et al. (2015). Sustainability Planetary boundaries: Guiding human development on a changing planet. Science 347, 1259855. doi:10.1126/science.1259855
Stevens, M., Pain, D. J., and Pienkowski, M. W. (1997). Farming and birds in europe: the common agricultural policy and its implications for bird conservation. J. Animal Ecol. 66, 917. doi:10.2307/6012
Stubbs, M. (2013). Conservation reserve program (crp): Status and issues. Washington, D.C.: Congressional Research Service
Sullivan, P., Hellerstein, D., Hansen, L., Johansson, R., Koenig, S., Lubowski, R. N., et al. (2004). The conservation reserve program: economic implications for rural America. USDA-ERS Agricultural Economic Report. doi:10.2139/ssrn.614511
Thiollay, J. M. (2006). Large bird declines with increasing human pressure in savanna woodlands (Burkina Faso). Biodivers. Conservation 15, 2085–2108. doi:10.1007/s10531-004-6684-3
Tilman, D., and Clark, M. (2014). Global diets link environmental sustainability and human health. Nature 515, 518–522. doi:10.1038/nature13959
Vannini, L., Gentile, E., Bruni, M., Loi, A., and Bernini, C. (2008). Evaluation of the set-aside measure 2000 to 2006.
Wang, S., Cai, L. M., Wen, H. H., Luo, J., Wang, Q. S., and Liu, X. (2019). Spatial distribution and source apportionment of heavy metals in soil from a typical county-level city of guangdong province, China. Sci. Total Environ. 655, 92–101. doi:10.1016/j.scitotenv.2018.11.244
Wang, Y. (2009). Exploring the shift from the EU common agricultural policy to the common agricultural and rural development policy. Rural. Econ. No 319, 118–120.
West, P. C., Gerber, J. S., Engstrom, P. M., Mueller, N. D., Brauman, K. A., Carlson, K. M., et al. (2014). Leverage points for improving global food security and the environment. Science 345, 325–328. doi:10.1126/science.1246067
Wu, Y. Z., and Xu, Z. Y. (2019). Study on the transformation of cropland protection under the background of rehabilitation system. Resour. Sci. 41 (1), 9–22. doi:10.18402/resci.2019.01.02
Xiao, Q., Zong, Y. T., and Lu, S. G. (2015). Assessment of heavy metal pollution and human health risk in urban soils of steel industrial city (anshan), liaoning, northeast China. Ecotoxicol. Environ. Saf. 120, 377–385. doi:10.1016/j.ecoenv.2015.06.019
Keywords: cultivated land risk, literature review, CiteSpace, planetary boundaries, cultivated land use system
Citation: Zhao Z, Lei M, Wen L, Xie E and Kong X (2023) Research status, development trends, and the prospects of cultivated land risk. Front. Environ. Sci. 11:1175239. doi: 10.3389/fenvs.2023.1175239
Received: 27 February 2023; Accepted: 06 September 2023;
Published: 18 September 2023.
Edited by:
Christopher Lant, Utah State University, United StatesReviewed by:
Peipei Tian, Shandong University, ChinaCopyright © 2023 Zhao, Lei, Wen, Xie and Kong. This is an open-access article distributed under the terms of the Creative Commons Attribution License (CC BY). The use, distribution or reproduction in other forums is permitted, provided the original author(s) and the copyright owner(s) are credited and that the original publication in this journal is cited, in accordance with accepted academic practice. No use, distribution or reproduction is permitted which does not comply with these terms.
*Correspondence: Xiangbin Kong, a3hiQGNhdS5lZHUuY24=
†These authors have contributed equally to this work
Disclaimer: All claims expressed in this article are solely those of the authors and do not necessarily represent those of their affiliated organizations, or those of the publisher, the editors and the reviewers. Any product that may be evaluated in this article or claim that may be made by its manufacturer is not guaranteed or endorsed by the publisher.
Research integrity at Frontiers
Learn more about the work of our research integrity team to safeguard the quality of each article we publish.