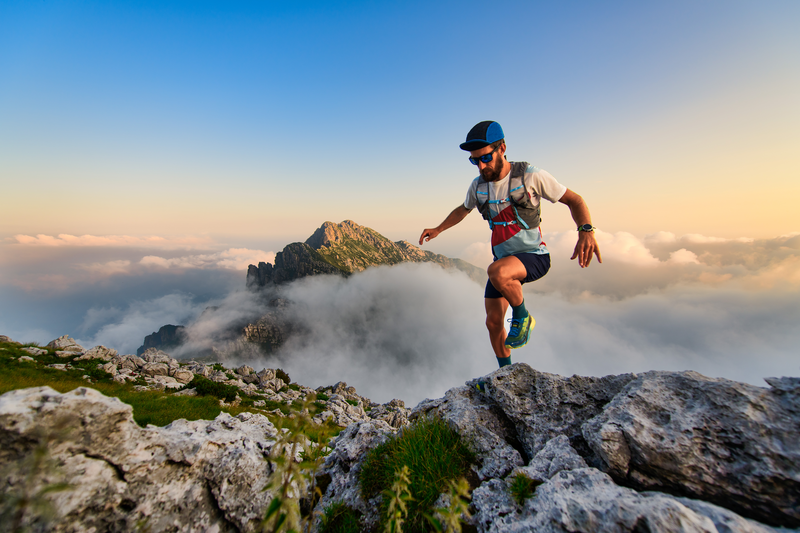
95% of researchers rate our articles as excellent or good
Learn more about the work of our research integrity team to safeguard the quality of each article we publish.
Find out more
ORIGINAL RESEARCH article
Front. Environ. Sci. , 03 August 2023
Sec. Soil Processes
Volume 11 - 2023 | https://doi.org/10.3389/fenvs.2023.1170507
Potentilla fruticosa, a major alpine shrubland type, is widely distributed across the Tibetan Plateau, and grazing is the most common disturbance in the shrublands of P. fruticosa. However, soil organic carbon (SOC), soil total nitrogen (STN), soil total phosphorus (STP), and their stoichiometry under different grazing intensities were unclear. In our study, we explored SOC, STN, STP, their stoichiometry, and their controlling factors in the grazing disturbance of heavy grazing (HG), moderate grazing (MG), light grazing (LG), and no grazing (NG) conditions in the Tibetan Plateau P. fruticosa shrublands. The grazing intensities were mainly assessed by considering the shrublands’ ground cover, the indicators of the road density, the distance between sampling sites and cowshed or sheep shed, the amounts of cow and sheep dung, and vegetation that had been gnawed and stampeded. Our results indicated that soil physical properties of soil temperature and bulk density have decreasing trends with decreasing grazing intensities from HG to NG. The SOC, STN, STP, and soil C:N and C:P ratios have increasing trends with decreasing grazing intensities from HG to NG, while the changes in soil N:P ratio were relatively stable along grazing intensities. Our results indicated that HG generally had stronger effects on SOC, STN, and soil C:N and C:P ratios than NG, indicating substantial effects of grazing disturbance on biogeochemical cycles of SOC and STN in the shrubland ecosystems. Therefore, for the alpine shrubland of P. fruticosa, the HG should be avoided for sustainable cycling of soil nutrients and the balance of soil nutrient stoichiometry. The grazing types can directly affect plant conditions, and plant conditions can directly affect soil physical and chemical properties and litter standing crops. Finally, soil physicochemical properties and litter standing crop resulting from different grazing intensities directly control SOC, STN, and STP. For the soil stoichiometry, the soil’s physical and chemical properties resulting from different grazing intensities have direct impacts on soil C:P and N:P ratios.
Carbon (C) can provide the structural basis for plants, and soil organic carbon (SOC), a major C pool for terrestrial C cycling, significantly regulates atmospheric CO2 (Wang et al., 2015). Nitrogen (N), a bio-element, is responsible for litter decomposition, photosynthesis, and plant growth (Klausmeier et al., 2004), and soil N is derived from soil organic matter (Nie et al., 2022). Phosphorous (P), a component of DNA, RNA, and cell structure, has a significant coupled relationship with C and N for plant photosynthesis, SOC decomposition, N fixation, and assimilation of C:N (Bai et al., 2012). Ecological stoichiometry mainly includes the balance of C and nutrient elements, e.g., C:N, C:P, and N:P, in terrestrial ecosystems (Bai et al., 2019). Soil C:N, C:P, and N:P ratios were different in various plant communities because of different substrate inputs (Zechmeister-Boltenstern et al., 2015). Soil C:N:P ratio not only has been used to explore the intrinsic relationships between belowground and aboveground components in ecosystems but also can affect plant nutrient state, regulate plant growth, and reflect soil fertility (Zechmeister-Boltenstern et al., 2015).
Grazing occupies more than one-third of terrestrial land regions (Xun et al., 2018). Overgrazing, however, may be detrimental to habitat conservation status, and it is thus important to assess potential trade-offs between grazing and ecosystem systems. Grazing is an important anthropogenic disturbance and strongly affects the cycles of C, N, and P, and their stoichiometry (He et al., 2020). The disturbance of grazing has been demonstrated to induce changes in plant diversity in the steppe (Zhang et al., 2018), and the changes of plant can affect SOC (Nie et al., 2021), thus inevitably affecting soil stoichiometry. Overgrazing has caused considerable losses of soil C in the form of CO2 from grasslands (Zhou et al., 2017). Grazing has had negative impacts on SOC, STN, and STP in the Tibetan Plateau alpine grasslands (Sun et al., 2019). Heavy grazing (HG) can negatively affect the accumulation of litter and soil coverage, and soil fertility and quality significantly decrease and substantially reduce production in ecosystems (Zhang et al., 2018). However, it was also demonstrated that grazing exclusion was not an effective option for SOC and STN sequestration, and the SOC and STN were relatively stable in the mountain meadow and typical steppe habitats in a mountain-basin ecosystem in an arid region of central China (Bi et al., 2018). On the Tibetan Plateau, grazing could reduce C emissions when alpine meadows suffer from droughts (Zhang Z. C et al., 2019). The rates of soil C changes increased linearly with those of N change, and soil C:N ratio was demonstrated to remain stable over the years of grazing exclusion (Yu et al., 2019). Among the 96 observations after grazing exclusion, 73% and 27% of the observations indicated an increase and decrease in soil C stock, respectively (Yu et al., 2019). Furthermore, the SOC has a significantly decreased trend with increasing grazing intensities in the Tibetan Plateau alpine meadow (Li et al., 2019). Grazing exclusion increased SOC, STN, and STP by 395 g·m−2 in the 0–15 cm soil depth, and 239 and 196 g·cm−2 in the 0–40 cm soil depth, respectively, for naturally restoring degraded grasslands in northern China (Wang N et al., 2019). In the grasslands of northern China, the enhancement of SOC with grazing exclusion is greater than the increase in STN, leading to an increase in the soil C:N ratio of 0.82 (Wang L et al., 2019). The deep understanding of soil C, N, P, and their stoichiometry variations to different grazing intensities is important for developing sustainable strategies for management and human-induced impacts on ecosystem functions.
Grazing has been demonstrated to affect C, N, and P, and their stoichiometry substantially varies among ecosystems and regions (Li et al., 2017; Zhou et al., 2017; He et al., 2020). Generally, no grazing (NG) fencing can sustain high SOC (Deng et al., 2017). However, other studies demonstrated that SOC was stable under the treatment of NG, light grazing (LG), moderate grazing (MG), and HG (Yang et al., 2018). Not only elements showed different responses to grazing treatments, but also the stoichiometry indicated various changes in grazing intensities. For example, HG can increase the soil C:N ratio, and LG decreases the soil C:N ratio in the alpine meadow (Yang et al., 2018). Different from the results in the alpine meadow, the soil C:N ratio was stable in the condition of LG in a sagebrush steppe (Shrestha and Stahl, 2008). In the desert steppe, typical steppe, and meadow steppe, both soil C:P and N:P ratios increased in HG (Bai et al., 2012), but in upland grasslands, the soil C:P and N:P ratios decreased in HG (Medina-Roldán et al., 2012). Therefore, the magnitude of grazing impacts on C, N, P, and their stoichiometry patterns depends on not only climatic conditions but also plant types and grazing intensity (McSherry and Ritchie, 2013). It was unclear how SOC, STN, STP, and their stoichiometry change in the different grazing intensities in the Tibetan Plateau alpine shrublands.
Soil stoichiometry, e.g., soil N:P ratio, has been explored in alpine shrublands, alpine wetlands, and alpine grasslands on the Tibetan Plateau (Yang et al., 2019; Nie et al., 2022; Nie et al., 2023). Grazing can stimulate soil C and N loss in the abiotic pathways (He et al., 2020). Specifically, livestock intensity can change soil structure through the surface crust and disrupt aggregates, resulting in increased soil susceptibility to wind and water erosions, finally leading to soil C and N losses (Neff et al., 2005). Climate, soil, and vegetation, including litter, characteristics can impact the biogeochemical cycles of SOC, STN, and STP, and thus control their stoichiometry. Microbes generally control the soil and plant feedback through nutrient mobilization and immobilization (Bai et al., 2012). Understanding the impacts of grazing intensity on the C:N:P stoichiometry in terrestrial ecosystems could contribute to predicting climate-biosphere feedback and improving management (Zhou et al., 2019a).
The alpine shrublands are important ecosystems across the Tibetan Plateau, and the Potentilla fruticosa, a major species in the alpine shrublands, is widely distributed and plays an important role in ecosystem security and C sequestration (Yashiro et al., 2010; Nie et al., 2022). Grazing animals in shrublands of P. fruticosa was the traditional way of life for the original residents of the Tibetan Plateau However, the unique conditions of high altitude and low temperature create a fragile alpine ecosystem, especially under the stress of overgrazing (Zhang T et al., 2019). A long history of grazing has existed across the Tibetan Plateau, and the degradation of shrublands can not only threaten herders’ livelihoods but also damage the sustainability of ecosystem services. In this study, we aimed to explore SOC, STN, STP, and their stoichiometry under different grazing intensities. We hypothesized that 1) grazing intensities can significantly affect SOC, STN, and STP, thus controlling soil C:N, C:P, and N:P ratios; 2) grazing intensities can significantly affect soil physical properties; and 3) the changing soil physicochemical properties and reduced litter resulting from different grazing intensities can change SOC, STN, STP, and their stoichiometry.
The study areas were mainly distributed in eastern Tibetan Plateau alpine shrublands, with latitude from 33°02′10.05″ to 38°09′39.09″ N, and longitude from 96°50′42.43″ to 102°13′28.55″ E. P. fruticosa, with a height of 25–150 cm, is an important shrubland type across the Tibetan Plateau. P. fruticosa is mainly located in the range of 2,300–4,000 m and can bear poor environments, e.g., snowy, cold, or windy conditions (Yashiro et al., 2010; Xu et al., 2019). The area of P. fruticosa is more than 100 km2, and it plays an important role in water and heat balance for the Tibetan Plateau and its surrounding locations (Peng et al., 2020). Mesophytes are commonly distributed in the shrublands of P. fruticosa, e.g., P. anserine, Dracocephalum heterophyllum, and Poa pratensis. According to the soil classification system by the Food and Agriculture Organization (FAO) of the United Nations, the main soil types in the study area are mainly Histosols and Kastanozems (Nachtergaele et al., 2012).
To divide different grazing intensities, we referred to the methods employed in grasslands, which divide the grassland degradation as HG, MG, LG, and NG, with a ground cover of 0%–20%, 20%–70%, 70%–90%, and >90%, respectively. Therefore, we divided the grazing intensities as HG, MG, LG, and NG as having ground cover of <20%, 20%–70%, 70%–90%, and >90%, respectively. The distance of any two grazing intensities was less than 30 km to reduce the interference from the spatial heterogeneity (Dai et al., 2019). Besides the shrublands’ ground cover, the road density, the distance between sampling sites and cowshed or sheep shed, the amount of cow and sheep dung, and vegetation that had been gnawed and stampeded were also assessed to decide the grazing intensity (Chen et al., 2018; Wang N et al., 2019; Huang et al., 2022). A total of 11 areas were chosen, and each area included HG, MG, LG, and NG; thus a total of 44 grazing sites (4 grazing sites/area × 11 areas) were found.
Field sampling was carried out during the growing season in 2021. In each site, three plots of 5 m × 5 m were created, and the distance between any two plots for the same grazing intensity was less than 50 m and larger than 5 m (Technical manual writing group of ecosystem carbon sequestion project, 2015). An ecological investigation was conducted in each plot: specifically, the aboveground biomass of shrublands, the ground cover of shrublands, belowground biomass, litter of foliage, and litter of stem. Soil samples were collected at a soil depth of 0–20 cm, and three replicate soil samples in three plots were mixed. Gentle homogenization then took place, where the plant and stones were removed, and they were sieved using a mesh of 2 mm (Nie et al., 2022). In each site, the mixed soil samples were divided into two parts; one was air-dried to measure physical and chemical factors, and the other was stored at −20°C to measure soil microbial diversity.
Soil-state 13C nuclear magnetic resonance (NMR) spectroscopy was used to estimate organic C functional groups, combing with the cross-polarization/total sideband suppression (CP/TOSS) technique (Zhang et al., 2015). Improving the signal-to-noise is necessary before NMR spectral analysis (Schmidt et al., 1997). The 13C NMR ranges of chemical compositions of alkyl C, O-alkyl C, aromatic C, and carbonyl C, which were determined by chemical shift areas, were 0–45 ppm, 45–110 ppm, 110–165 ppm, and 165–210 ppm, respectively (Kögel-Knabner, 2002). The soil total C and STN were measured by an element analyzer (2400 Ⅱ CHNS/O, Perkin, Waltham, United States). Soil inorganic C was equivalent to carbonate values estimated by CO2 evolution with a strong acid (Nelson and Sommers, 1982). SOC was determined by measuring the difference between soil total C and soil inorganic C. The STP was determined by the molybdate/ascorbic acid method with H2SO4-H2O2 digestion (Jones, 2001). Soil moisture and temperature were determined by a portable soil temperature and moisture probe (Testo 175H1, Huey Analytical Instrument, Qingdao, China). Soil pH was determined with a 2.5:1 ratio of water to soil. Soil sample of bulk density was collected in a container of 100 cm3 (50 mm height and 50.46 mm diameter), then dried in an oven (105°C). The bulk density was measured using soil dry mass by volume. The litter of foliage, litter of branches, belowground biomass, and aboveground biomass were dried at 65°C for 48 h to obtain their dry weight and weighed until they reached a constant value.
To explore microbial factors in different grazing intensities, molecular analyses were conducted to extract DNA from soil samples. Specifically, frozen soil (10 g) was freeze-dried for 72 h (ALPHA 1-2 LD plus, Christ, Germany). Then, DNA from the freeze-dried soils (0.5 g) was extracted using a Power Soil DNA Isolation Kit (Qiagen, Hilden, Germany). The DNA was tested for its content and quality by 1% agarose gel electrophoresis and a spectrophotometer (ND-1000, NanoDrop Technologies, Wilmington, United States), respectively. The V4-V5 hypervariable regions of 907R (5′-CCGTCAATTCCTTTGAGT TT-3′) and 515F (5′-GTGCCAGCMGCCGCGTAA-3′) were amplified for 16S rRNA gene. The amplified fungal ITS 1 regions were ITS2-2043R (5′-GCTGCGTTCTTCATCGATGC-3′) and ITS5-1737F (5′-GGAAGTAAAAGTCGTAACAAGG-3′). The paired-end reads were merged by using FLASH (http://ccb.jhu.edu/software/FLASH/). The bacteria and fungi were determined by the silva reference database (http://www.arb-silva.de) and the BLAST tool in QIIME (http://qiime.org/index.html), respectively. Community diversity indicators, Shannon, and PD whole tree estimator for bacteria and fungi were determined by 40,800 and 73,250 reads per sample, respectively.
One-way analysis of variance was conducted to determine the effects of the grazing intensity on SOC, STN, STP, and soil C:N, C:P, and N:P ratios, followed by the Games-Howell test. The significance was determined by SPSS 22.0 (SPSS Incorporation, United States). The ordinary least squares method was employed to explore the relationships among SOC, STN, STP, their stoichiometry on soil (bulk density, soil pH, soil temperature, soil moisture), plant (aboveground biomass, belowground biomass, ground cover of shrublands), litter (litter foliage, litter stem, total litter), and microbes (16s Shannon, 16s PD whole tree, IT Shannon, and IT PD whole tree).
The relative importance of the direct and indirect effects of soil, plant, litter, and microbe factors affecting SOC, STN, STP, and soil C:N, C:P, and N:P ratios was assessed using structural equation modeling (SEM). A prior conceptual model of hypothetical relationships was established before SEM analysis (Supplementary Figure S1). If Fisher’s C is statistically non-significant, a good model fit is necessary (p > 0.05). The package of “PIECEWISE” was used to estimate SEM analysis in the statistical R software (R Development Core Team, 2012).
The SOC, STN, and STP have increasing trends with decreasing grazing intensities from HG to NG. Specifically, SOC and STN in the HG, MG, LG, and NG were 64.57 ± 14.03 and 5.83 ± 1.13, 75.13 ± 14.55, and 6.62 ± 1.14, 84.92 ± 25.06 and 7.29 ± 2.26, and 103.03 ± 33.82 and 8.57 ± 2.72 g·kg−1, respectively. In addition, both SOC and STN were significantly lower in HG than in NG (p < 0.05) (Figure 1). STP in the HG, MG, LG, and NG were 1.23 ± 0.15, 1.32 ± 0.26, 1.46 ± 0.34, and 1.50 ± 0.34 g·kg−1, respectively. There was no significant difference in STP among different grazing intensities (Supplementary Figure S2).
FIGURE 1. Soil organic carbon (SOC), soil total nitrogen (STN), and soil C:N and C:P ratios in the grazing intensity of heavy grazing (HG), moderate grazing (MG), light grazing (LG) and no grazing (NG) in Potentilla fruticosa shrublands on the Tibetan Plateau. Different low letters indicate a significant difference among the grazing intensities.
Soil C:N and C:P ratios have increasing trends with decreasing grazing intensities from HG to NG, while the change in soil N:P ratio was relatively stable along grazing intensities. Soil C:N and C:P ratios in HG, MG, LG, and NG were 11.04 ± 0.62 and 53.41 ± 13.45, 11.31 ± 0.53 and 57.61 ± 9.31, 11.73 ± 1.09 and 58.19 ± 11.61, and 12.00 ± 0.54 and 67.66 ± 10.16, respectively (Figure 1). Soil N:P ratios in HG, MG, LG, and NG were 4.83 ± 1.15, 5.09 ± 0.76, 4.99 ± 1.05, and 5.64 ± 0.82, respectively (Supplementary Figure S2). Soil C:N ratio and N:P ratio in the NG were significantly larger than that in the HG, while the soil N:P ratio was not significantly different among different grazing intensities. The grazing intensities can significantly affect SOC and STN, thus controlling soil C:N and C:P ratios, and the significantly lower SOC, STN, soil C:N, and C:P ratios in HG than in NG partly supported the first hypothesis.
Furthermore, soil temperature has a decreasing trend with decreasing grazing intensities from HG to NG. The soil temperature of 18.10°C ± 4.09°C in HG is significantly larger than that in LG (13.46°C ± 4.01°C) and NG (10.46°C ± 2.76°C) (Figure 2). Although the bulk density also has a decreasing trend with grazing intensities from HG to NG, differences were not significant. The soil moisture was relatively stable in different grazing intensities (Supplementary Figure S3). Thus, these results supported the second hypothesis.
FIGURE 2. Soil temperature in the grazing intensity of heavy grazing (HG), moderate grazing (MG), light grazing (LG), and no grazing (NG) in P. fruticosa shrublands on the Tibetan Plateau. Different low letters indicate a significant difference among the grazing intensities.
Soil physical and chemical properties have significant effects on SOC, STN, STP, and stoichiometry. Specifically, with increasing bulk density, soil pH, and soil temperature, SOC, STN, and STP have significantly decreasing trends. However, with increasing soil moisture, SOC has a significantly increasing trend, and both STN and STP have weak increasing trends with increasing soil moisture (p = 0.09 for STN, and p = 0.15 for STP). Soil C:N ratio has an increasing trend with increasing soil moisture, and soil C:P and N:P ratios have decreasing trends with increasing bulk density (Figure 3).
FIGURE 3. Effects of bulk density, soil pH, soil temperature, and soil moisture on soil organic carbon (SOC), soil total nitrogen (STN), soil total phosphorus (STP), and soil C:N, C:P, and N:P ratios in P. fruticosa shrublands on the Tibetan Plateau. The gray areas correspond to 95% confidence intervals.
Plant factors have significant effects on SOC, STN, STP, and their stoichiometry. Specifically, with increasing aboveground biomass, belowground biomass, and ground cover of shrublands, SOC, STN, and STP have increasing trends (p < 0.05), while the increasing trend of STP along the ground cover of shrublands was not significant (p = 0.065). Soil C:N ratio and C:P ratio have increasing trends along increasing aboveground biomass and ground cover of shrublands (p < 0.05), but the increasing trends of soil N:P ratio along soil aboveground biomass and ground cover of shrublands were not significant (p = 0.137 for aboveground biomass, p = 0.131 for ground cover of shrublands). Soil C:N, C:P, and N:P ratios have increasing trends along increasing belowground biomass, but their relationships were not significant (p > 0.05) (Figure 4).
FIGURE 4. Effects of shrubland aboveground biomass, belowground biomass, and ground cover of shrublands on soil organic carbon (SOC), soil total nitrogen (STN), soil total phosphorus (STP), and soil C:N, C:P, and N:P ratios in P. fruticosa shrublands on the Tibetan Plateau. The gray areas correspond to 95% confidence intervals.
Litter standing crop has significant effects on SOC, STN, STP, and their stoichiometry. Specifically, with increasing litter standing crop foliage, litter standing crop stem and total litter standing crop, SOC, STN, and STP have increasing trends (p < 0.05). Although soil C:N, C:P, and N:P ratios have increasing trends with litter factors, only soil C:P ratio has increasing trends with increasing both litter standing crop stem and total litter standing crop (p > 0.05) (Figure 5).
FIGURE 5. Effects of litter foliage, litter stem, total litter on soil organic carbon (SOC), soil total nitrogen (STN), soil total phosphorus (STP), and soil C:N, C:P, and N:P ratios in P. fruticosa shrublands on the Tibetan Plateau. The gray areas correspond to 95% confidence intervals.
Microbes have significant effects on SOC, STN, STP, and their stoichiometry. The 16s Shannon, rather than IT Shannon, has significant effects on SOC, STN, and STP (p < 0.05). The SOC and STN have decreasing trends with increasing 16s PD whole tree (p < 0.05), while the decreasing trend of STP was not significant along the 16s PD whole tree (p = 0.248). The SOC, STN, and STP have significant and increasing trends along IT PD whole tree (p < 0.05). Soil C:P and N:P ratios have significant decreasing trends with increasing 16s PD whole tree (p < 0.05). In addition, soil C:P ratio has an increasing trend with IT PD whole tree (p < 0.05) (Figure 6).
FIGURE 6. Effects of 16s Shannon, 16s PD whole tree, IT Shannon, and IT PD whole tree on soil organic carbon (SOC), soil total nitrogen (STN), soil total phosphorus (STP), and soil C:N, C:P, and N:P ratios in P. fruticosa shrublands on the Tibetan Plateau. The gray areas correspond to 95% confidence intervals.
The SOC chemical compositions have significant effects on SOC and STP, and their C:N ratio. Specifically, with increasing carbonyl C, SOC and STP have decreasing trends. STP has an increasing trend with increasing alkyl C and O-alkyl C, and a decreasing trend with aromatic C (p < 0.05). Soil C:N ratio has a decreasing trend with increasing aromatic C, while it has an increasing trend with increasing carbonyl C (p < 0.05) (Figure 7).
FIGURE 7. Effects of alkyl C, O-alkyl C, aromatic C, and carbonyl C on soil organic carbon (SOC), soil total nitrogen (STN), soil total phosphorus (STP), and soil C:N, C:P, and N:P ratios in P. fruticosa shrublands on the Tibetan Plateau. The gray areas correspond to 95% confidence intervals.
Results from SEM indicated that grazing intensity can directly affect plant conditions, and plant conditions can directly affect soil physical and chemical properties and litter standing crops. Finally, soil physicochemical properties and litter-standing crop resulting from different grazing intensities also directly control SOC, STN, and STP. For the soil stoichiometry, the soil’s physical and chemical properties resulting from different grazing intensities have direct effects on soil C:P and N:P ratios (Figure 8). Thus, these results supported the third hypothesis.
FIGURE 8. Structural equation models depicting direct and indirect drivers of soil organic carbon (SOC), soil total nitrogen (STN), soil total phosphorus (STP), and soil C:N, C:P, and N:P ratios in P. fruticosa shrublands on the Tibetan Plateau. The dashed arrows show non-significant effects. The solid lines represent significant effects. HG, heavy grazing; MG, moderate grazing; LG, light grazing; NG, no grazing; AGB, aboveground biomass of shrublands; BGB, belowground biomass of shrublands.
Grazing, a significant anthropogenic activity, affects the biogeochemical cycles of C, N, and P and their stoichiometry in the terrestrial ecosystem (Knops et al., 2002; He et al., 2020). The SOC in different grazing intensities was from 64.57 g·kg−1 to 103.03 g·kg−1, which was in the range of SOC (0.04–126.12 g·kg−1) across the Tibetan Plateau (Nie et al., 2021). The aboveground biomass and litter are principal C inputs (Ding et al., 2017; Leal et al., 2019). The aboveground biomass and total litter in NG were significantly higher than that in HG, which results in a significantly lower SOC in HG than that in NG. It has also been shown that the disturbed soil surface crust and structure by grazing contribute to accelerate soil C loss because of enhanced soil susceptibility to water and wind erosion (McSherry & Ritchie, 2013). At a global scale, grazing can decrease soil C pool by 3.1% in grasslands (Zhou et al., 2017). Soil organic matter is the principal soil N resource (Nie et al., 2022), and the STN shift in different grazing intensities is similar to SOC, and STN was higher in NG than that in HG. Similarly, a meta-analysis at a global scale showed that soil N losses under HG and HG significantly decreased soil N by 13.04% (Zhou et al., 2017). However, STP was stable in different grazing intensities. This is because the parent materials instead of plant conditions can play a significant role in regulating STP (Lin et al., 2009; Zhang Z. C et al., 2019).
In our studies, soil C:N and C:P ratios, rather than soil N:P ratio, were affected by grazing intensities. This is because the decreasing level was larger in SOC (37%) than in STN (31%), while STP was relatively stable from HG to NG. Similarly, the enhancement of soil C sequestration with grazing exclusion is greater than the increase in soil N, leading to an increase in the soil C:N ratio of 0.82 units across the grasslands in northern China (Wang L et al., 2019). Other results were different from our results, and grazing can reduce about 22.4% of the SOC and 30.9% of STN than that in un-grazed regions (Zhang et al., 2018). Their difference may result from various ecological environments, types of grazing animals or plants, and grazing time of year (Li et al., 2017; Zhang et al., 2018; Yu et al., 2019). The increased SOC, on the one hand, might be attributed to the increased biomass induced by reducing livestock grazing intensities (Zhou et al., 2019b). On the other hand, increasing vegetation recovery, resulting from decreasing grazing intensities, could prevent soil C loss caused by wind erosion by improving vegetation cover, which mitigates the exposure of the bare soil surface to wind erosion (Mekuria et al., 2007). The increasing SOC along with decreasing grazing intensities indicated soil C accumulation after decreasing grazing intensities in the Tibetan Plateau alpine shrublands of P. fruticosa. In grasslands, grazing was also demonstrated to change the soil C:N and C:P ratios (Zhou et al., 2007; Yang et al., 2019), while grazing did not affect the soil N:P ratio in a semi-arid pasture in Brazil (Filho et al., 2019; Yang et al., 2019). The responses of SOC, STN, and STP and their stoichiometry to grazing intensities have significant applications for the strategy of sustainable development in terrestrial management (He et al., 2020). Therefore, for the alpine shrubland of P. fruticosa, HG should be avoided for sustainable cycling of soil nutrients and the balance of soil nutrient stoichiometry.
Grazing can modify the distribution of significant environmental predictors of soil pH (Fierer et al., 2007). Soil pH has negative relationships with SOC, STN, and STP. It has been demonstrated that soil pH can indicate various gradients of soil characteristics and is closely linked to biological processes (Hong et al., 2018; Rasmussen et al., 2018). With increasing soil pH, the available minerals, e.g., iron, manganese, and zinc, can decrease, limiting the growth of the plant. Thus, in the condition of increasing soil alkalinity, the SOC accumulation is limited, due to decreasing plant biomass input (Davidson and Janssens, 2006). Soil pH was found to be important in microbial activities and dynamics of soil C and N (Aciego Pietri and Brookes, 2008). In acidic soils, bacteria diversity was less, consequently limiting soil C and N decomposition (Shen et al., 2013; Tashi et al., 2016).
Grazing reduces leaf areas and exposes more soil surfaces. Lower plant cover caused by grazing would also increase soil temperature, which can stimulate microbe activity, limit SOC accumulation (Davidson and Janssens, 2006), and create a negative relationship with SOC. Soil temperature in HG was significantly higher than that in LG across the Tibetan Plateau alpine shrublands of P. fruticosa. Similarly, soil temperature was demonstrated to be increased exponentially with increasing grazing intensity in the warm season, and the increased temperature was 2.6°C in a Eurasian Steppe, due to the removal of aboveground biomass (Yan et al., 2018). However, increasing soil moisture can contribute to plant growth and lead to a high production of plants and more plant litter, which contribute to soil organic matter input (Wynn et al., 2006) and, eventually, shape its positive relationship with SOC. Similarly, precipitation also played a major role in the soil C change following grazing exclusion, with faster accumulation under wetter conditions (Yu et al., 2019). The positive relationship between soil C:N ratio and soil moisture existed in our study, which indicated that soil moisture can contribute to SOC accumulation.
Grazing is a major driver of the composition of microbial communities, which play significant roles in soil functioning (Eldridge et al., 2020). Grazing has been demonstrated to shift microbial communities from fungi dominated and slow growing to bacteria-dominated and fast-growing (Xun et al., 2018). Microbes have significant effects on SOC decomposition (Yang et al., 2008). In our study, with increasing 16s Shannon instead of IT Shannon, SOC, STN, and STP have decreasing trends, which indicated that bacteria rather than fungi have more significant effects on controlling soil nutrient element decomposition. Plant removal by grazing reduces leaves, leading to reduce litter input and shifts in soil bacterial communities and soil C processes (Zhou et al., 2017). Trampling of vegetation can lead to reductions in plant cover, changes in litter distribution, and reductions in resource connectivity (Eldridge et al., 2017), which might also alter microbial community composition (Eldridge et al., 2020). It should be noted that 16s PD whole tree and IT PD whole tree also can significantly affect SOC and STN. Our results were consistent with the previous result that microbial robustness can significantly affect soil stability (Griffiths and Philippot, 2013). Bacteria rather than fungi are more important in labile-SOC turnover (Xun et al., 2018), and with increasing 16s Shannon and 16s PD whole tree, the more labile-soil organic matter was decomposed, thus creating a negative relationship between 16s Shannon and 16s PD whole tree and SOC, STN, and STP.
Results from SEM indicated that SOC, STN, and STP in different grazing intensities can be directly controlled by soil physicochemical properties and litter standing crops. The effects of grazing types on soil nutrient elements were through their effects on plant conditions, and plant conditions can directly affect soil physical and chemical properties and litter standing crops. Livestock grazing usually inhibits leaf photosynthesis and primary production (Zhou et al., 2017). For the soil stoichiometry, the soil’s physical and chemical properties have direct effects on soil C:P and N:P ratios. Therefore, a certain amount of litter standing crop and healthy soil physicochemical properties should exist to avoid unbalanced nutrition in the Tibetan Plateau P. fruticosa shrublands.
In our study, we explored SOC, STN, STP, their stoichiometry, and their controlling factors in the grazing disturbance of HG, MG, LG, and NG in the Tibetan Plateau P. fruticosa shrublands. Our results indicated that SOC, STN, STP, and soil C:N, C:P, and N:P ratios were quantified, respectively. The SOC, STN, and C:N and C:P ratios were significantly higher in NG than that in HG; hence, for the alpine shrubland of P. fruticosa, HG should be avoided for sustainable cycling of soil nutrients and the balance of soil nutrient stoichiometry. The grazing types can directly affect plant conditions, and plant conditions can directly affect soil physical and chemical properties and litter standing crops. In addition, healthy soil physicochemical properties and a certain amount of litter standing crop are necessary to allow C sequestrations and soil nutrient balance in the P. fruticosa shrublands across the Tibetan Plateau. Although the effects of grazing intensities on SOC, STN, STP, and their stoichiometry have been explored, the grazing types of NG, LG, MG, and HG are only roughly defined. More specific grazing conditions quantified in the grazing regions are necessary to provide a more accurate estimation of soil element stoichiometry and nutrient balance for sustainable development across the Tibetan Plateau.
The original contributions presented in the study are included in the article/Supplementary Material, further inquiries can be directed to the corresponding author.
XN, YD, and GZ conceived the study and designed the methodology; XN, YD, GZ, LR, DW, CL, YC, and XL collected the data, XN analyzed the data; XN led the writing of the manuscript. All authors contributed to the article and approved the submitted version.
This work was supported by the National Natural Science Foundation of China (Grant No. 32001216), Qinghai Province International Exchange and Cooperation Project (Grant No. 2022-HZ-804), the National Nonprofit Institute Research Grant of Chinese Academy of Forestry (Grant No. CAFYBB2021MA015), the Second Tibetan Plateau Scientific Expedition and Research (STEP) program (Grant No. 2019QZKK0302), the National Natural Science Foundation of China (Grant No. U21A20191), and Qinghai University Youth Research Funding (Grant No. 2021-QNY-11).
The authors declare that the research was conducted in the absence of any commercial or financial relationships that could be construed as a potential conflict of interest.
All claims expressed in this article are solely those of the authors and do not necessarily represent those of their affiliated organizations, or those of the publisher, the editors and the reviewers. Any product that may be evaluated in this article, or claim that may be made by its manufacturer, is not guaranteed or endorsed by the publisher.
The Supplementary Material for this article can be found online at: https://www.frontiersin.org/articles/10.3389/fenvs.2023.1170507/full#supplementary-material
Aciego Pietri, J. C., and Brookes, P. C. (2008). Relationships between soil pH and microbial properties in a UK arable soil. Soil Biol. Biochem. 40, 1856–1861. doi:10.1016/j.soilbio.2008.03.020
Bai, X., Wang, B. R., An, S., Zeng, Q., and Zhang, H. J. C. (2019). Response of forest species to C: N:P in the plant-litter-soil system and stoichiometric homeostasis of plant tissues during afforestation on the loess plateau, China. Catena 183, 104186. doi:10.1016/j.catena.2019.104186
Bai, Y., Wu, J., Clark, C., Pan, Q., Zhang, L., Chen, S., et al. (2012). Grazing alters ecosystem functioning and C: N:P stoichiometry of grasslands along a regional precipitation gradient. J. Appl. Ecol. 49, 1204–1215. doi:10.1111/j.1365-2664.2012.02205.x
Bi, X., Li, B., Fu, Q., Fan, Y. L., Ma, Z., Yang, B., et al. (2018). Effects of grazing exclusion on the grassland ecosystems of mountain meadows and temperate typical steppe in a mountain-basin system in Central Asia's arid regions, China. Sci. Total Environ. 630, 254–263. doi:10.1016/j.scitotenv.2018.02.055
Chen, S., Wang, W., Xu, W., Wang, Y., Wan, H., Chen, D., et al. (2018). Plant diversity enhances productivity and soil carbon storage. Proceeding Natl. Acad. Sci. 115, 4027–4032. doi:10.1073/pnas.1700298114
Dai, L., Guo, X., Ke, X., Du, Y., Zhang, F., Li, Y., et al. (2019). The response of Potentilla fruticosa communities to degradation succession in qinghai-tibet plateau. Ecol. Environ. Sci. 28, 732–740.
Davidson, E. A., and Janssens, I. A. (2006). Temperature sensitivity of soil carbon decomposition and feedbacks to climate change. Nature 440, 165–173. doi:10.1038/nature04514
Deng, L., Shangguan, Z. P., Wu, G. L., and Chang, X. F. (2017). Effects of grazing exclusion on carbon sequestration in China's grassland. Earth-Science Rev. 173, 84–95. doi:10.1016/j.earscirev.2017.08.008
Ding, J., Chen, L., Ji, C., Hugelius, G., Li, Y., Liu, L., et al. (2017). Decadal soil carbon accumulation across Tibetan permafrost regions. Nat. Geosci. 10, 420–424. doi:10.1038/ngeo2945
Eldridge, D. J., Delgado-Baquerizo, M., Travers, S. K., Val, J., Oliver, I., Hamonts, K., et al. (2017). Competition drives the response of soil microbial diversity to increased grazing by vertebrate herbivores. Ecology 98, 1922–1931. doi:10.1002/ecy.1879
Eldridge, D. J., Travers, S. K., Val, J., Wang, J., Liu, H., Manuel Delgado-Baquerizo, B. K. S., et al. (2020). Grazing regulates the spatial heterogeneity of soil microbial communities within ecological networks. Ecosystems 23, 932–942. doi:10.1007/s10021-019-00448-9
Fierer, N., Bradford, M. A., and Jackson, R. B. (2007). Toward an ecological classification of soil bacteria. Ecology 88, 1354–1364. doi:10.1890/05-1839
Filho, J., Vieira, J., Silva, E., Oliveira, J., Pereira, M., and Brasileiro, F. (2019). Assessing the effects of 17 years of grazing exclusion in degraded semi-arid soils: Evaluation of soil fertility, nutrients pools and stoichiometry. J. Arid Environ. 166, 1–10. doi:10.1016/j.jaridenv.2019.03.006
Griffiths, B. S., and Philippot, L. (2013). Insights into the resistance and resilience of the soil microbial community. FEMS Microbiol. Rev. 37, 112–129. doi:10.1111/j.1574-6976.2012.00343.x
He, M., Zhou, G., Yuan, T., Groenigen, K., Shao, J. J., and Zhou, X. H. (2020). Grazing intensity significantly changes the C:N:P stoichiometry in grassland ecosystems. Glob. Ecol. Biogeogr. 29, 355–369. doi:10.1111/geb.13028
Hong, S., Piao, S., Chen, A., Liu, Y., Liu, L., Peng, S., et al. (2018). Afforestation neutralizes soil pH. Nat. Commun. 9, 520. doi:10.1038/s41467-018-02970-1
Huang, K., Hu, G., Pang, Q., Zhang, B., He, Y., Hu, C., et al. (2022). Effects of grazing on species composition and community structure of shrub tussock in subtropical karst mountains, southwest China. Chin. J. Plant Ecol. 46, 1350–1363. doi:10.17521/cjpe.2022.0069
Jones, J. B. (2001). Laboratory guide for conductiong soil tests and plant analysis. Florida, United States: CRC Press.
Klausmeier, C. A., Litchman, E., Daufresne, T., and Levin, S. A. (2004). Optimal nitrogen-to-phosphorus stoichiometry of phytoplankton. Nature 429, 171–174. doi:10.1038/nature02454
Knops, J. M. H., Bradley, K. L., and Wedin, D. A. (2002). Mechanisms of plant species impacts on ecosystem nitrogen cycling. Ecol. Lett. 5, 454–466. doi:10.1046/j.1461-0248.2002.00332.x
Kögel-Knabner, I. (2002). The macromolecular organic composition of plant and microbial residues as inputs to soil organic matter. Soil Biol. Biochem. 34, 139–162. doi:10.1016/s0038-0717(01)00158-4
Leal, O. A., Dick, D. P., Costa, F. S., Knicker, H., Carvalho Júnior, J. A. d., and Santos, J. C. (2019). Carbon in physical fractions and organic matter chemical composition of an acrisol after amazon forest burning and conversion into pasture. J. Braz. Chem. Soc. 30, 413–424.
Li, G., Shi, L., Zhang, Z., Grace, J., Yang, M., Wu, S., et al. (2019). Distribution of soil organic carbon and potential carbon sequestration in an alpine meadow grazed by domesticated yak in Zeku, Qinghai-Tibetan Plateau, China. Carbon Manag. 10, 135–147. doi:10.1080/17583004.2018.1560195
Li, W., Cao, W., Wang, J., Xiaolong, L., Xu, C., and Shi, S. (2017). Effects of grazing regime on vegetation structure, productivity, soil quality, carbon and nitrogen storage of alpine meadow on the Qinghai-Tibetan Plateau. Ecol. Eng. 98, 123–133. doi:10.1016/j.ecoleng.2016.10.026
Lin, J., Shi, X., Lu, X., Yu, D., Wang, H., Zhao, Y., et al. (2009). Storage and spatial variation of phosphorus in paddy soils of China. Pedosphere 19, 790–798. doi:10.1016/s1002-0160(09)60174-0
McSherry, M. E., and Ritchie, M. E. (2013). Effects of grazing on grassland soil carbon: A global review. Glob. Change Biol. 19, 1347–1357. doi:10.1111/gcb.12144
Medina-Roldán, E., Paz-Ferreiro, J., and Bardgett, R. (2012). Grazing exclusion affects soil and plant communities, but has no impact on soil carbon storage in an upland grassland. Agric. Ecosyst. Environ. 149, 118–123. doi:10.1016/j.agee.2011.12.012
Mekuria, W., Veldkamp, E., Haile, M., Nyssen, J., Muys, B., and Gebrehiwot, K. (2007). Effectiveness of exclosures to restore degraded soils as a result of overgrazing in Tigray, Ethiopia. J. Arid Environ. 69, 270–284. doi:10.1016/j.jaridenv.2006.10.009
Nachtergaele, F., Velthuizen, H., Verelst, L., Wiberg, D., Batjes, N., Dijkshoorn, K., et al. (2012). Harmonized world soil database. Rome: Italy and IIASA. FAO, Laxenburg, Austria.
Neff, J. C., Reynolds, R. L., Belnap, J., and Lamothe, P. (2005). Multi-decadal impacts of grazing on soil physical and biogeochemical properties in southeast Utah. Ecol. Appl. 15, 87–95. doi:10.1890/04-0268
Nelson, D., and Sommers, L. (1982). “Total carbon, organic carbon, and oganic matte,” in Methods of soil analysis (Madison: American Society of Agronomy), 539–579.
Nie, X., Wang, D., Ren, L., Ma, K., Chen, Y., Yang, L., et al. (2022). Distribution characteristics and controlling factors of soil total nitrogen: Phosphorus ratio across the northeast Tibetan plateau shrublands. Front. Plant Sci. 13, 825817. doi:10.3389/fpls.2022.825817
Nie, X., Wang, D., Ren, L., Zhou, G., and Du, Y. (2023). Soil N:P ratio and its regulation factors in alpine wetlands across the Three Rivers Source Region. J. Soil Sci. Plant Nutr. 23, 1138–1148. doi:10.1007/s42729-022-01109-y
Nie, X., Wang, D., Yang, L., and Zhou, G. (2021). Controls on variation of soil organic carbon concentration in the shrublands of the north-eastern Tibetan Plateau. Eur. J. Soil Sci. 72, 13084. doi:10.1111/ejss.13084
Peng, C., Song, M., Zhou, C., Li, Y., Li, X., and Cao, G. (2020). Relationship between leaf functional traits of herbaceous plants and soil factors in different coverage gradients of Potentilla fruticose shrub under grazing. Acta bot. boreali - Occident. Sin. 40, 870–881.
Rasmussen, C., Heckman, K., Wieder, W. R., Keiluweit, M., Lawrence, C. R., Berhe, A. A., et al. (2018). Beyond clay: Towards an improved set of variables for predicting soil organic matter content. Biogeochemistry 137, 297–306. doi:10.1007/s10533-018-0424-3
Schmidt, M. W. I., Knicker, H., Hatcher, P. G., and Kogel-Knabner, I. (1997). Improvement of 13C and 15N CPMAS NMR spectra of bulk soils, particle size fractions and organic material by treatment with 10% hydrofluoric acid. Eur. J. Soil Sci. 48, 319–328. doi:10.1111/j.1365-2389.1997.tb00552.x
Shen, C., Xiong, J., Zhang, H., Feng, Y., Lin, X., Li, X., et al. (2013). Soil pH drives the spatial distribution of bacterial communities along elevation on Changbai Mountain. Soil Biol. Biochem. 57, 204–211. doi:10.1016/j.soilbio.2012.07.013
Shrestha, G., and Stahl, P. D. (2008). Carbon accumulation and storage in semi-arid sagebrush steppe: Effects of long-term grazing exclusion. Agric. Ecosyst. Environ. 125, 173–181. doi:10.1016/j.agee.2007.12.007
Sun, J., Zhan, T., Liu, M., Zhang, Z., Wang, Y., Liu, S., et al. (2019). Verification of the biomass transfer hypothesis under moderate grazing across the Tibetan plateau: A meta-analysis. Plant Soil 458, 139–150. doi:10.1007/s11104-019-04380-8
Tashi, S., Singh, B., Keitel, C., and Adams, M. (2016). Soil carbon and nitrogen stocks in forests along an altitudinal gradient in the eastern Himalayas and a meta-analysis of global data. Glob. Change Biol. 22, 2255–2268. doi:10.1111/gcb.13234
Technical manual writing group of ecosystem carbon sequestion project (2015). Observation and Investigation for carbon sequestion in terrestrial ecosystems. Beijing: Science Press.
Wang, J., Wang, X., Zhang, J., and Zhao, C. (2015). Soil organic and inorganic carbon and stable carbon isotopes in the Yanqi Basin of northwestern China. Eur. J. Soil Sci. 66, 95–103. doi:10.1111/ejss.12188
Wang, L., Gan, Y., Wiesmeier, M., Zhao, G., Zhang, R., Hou, F., et al. (2019). Grazing exclusion – An effective approach for naturally restoring degraded grasslands in northern China. Land Degrad. Dev. 29, 4439–4456. doi:10.1002/ldr.3191
Wang, N., Xiong, H., Ye, H., Ma, L., and Zhang, F. (2019). Soil salinity and ion characteristics under different degrees of human disturbance in Junggar Basin. Soil Fertilizer Sci. China 61, 71–77.
Wynn, J. G., Bird, M. I., Vellen, L., Grand-Clement, E., Carter, J., and Berry, S. L. (2006). Continental-scale measurement of the soil organic carbon pool with climatic, edaphic, and biotic controls. Glob. Biogeochem. Cycles 20, 1–12. doi:10.1029/2005gb002576
Xu, Y., Huang, J., Lu, X., Ding, Y., and Zang, R. (2019). Priorities and conservation gaps across three biodiversity dimensions of rare and endangered plant species in China. Biol. Conserv. 229, 30–37. doi:10.1016/j.biocon.2018.11.010
Xun, W., Yan, R., Ren, Y., Jin, D., Xiong, W., Zhang, G., et al. (2018). Grazing-induced microbiome alterations drive soil organic carbon turnover and productivity in meadow steppe. Microbiome 6, 170. doi:10.1186/s40168-018-0544-y
Yan, Y., Yan, R., Chen, J., Xin, X., Eldridge, D. J., Shao, C., et al. (2018). Grazing modulates soil temperature and moisture in a Eurasian steppe. Agric. For. Meteorolgy 262, 157–165. doi:10.1016/j.agrformet.2018.07.011
Yang, X., Dong, Q., Chu, H., Ding, C., Yu, Y., Zhang, C., et al. (2019). Different responses of soil element contents and their stoichiometry (C:N:P) to yak grazing and Tibetan sheep grazing in an alpine grassland on the eastern Qinghai – Tibetan Plateau. Agric. Ecosyst. Environ. 285, 106628. doi:10.1016/j.agee.2019.106628
Yang, Y., Fang, J., Tang, Y., Ji, C., Zheng, C., He, J., et al. (2008). Storage, patterns and controls of soil organic carbon in the Tibetan grasslands. Glob. Change Biol. 14, 1592–1599. doi:10.1111/j.1365-2486.2008.01591.x
Yang, Y., Liu, B. R., and An, S. (2018). Ecological stoichiometry in leaves, roots, litters and soil among different plant communities in a desertified region of Northern China. Catena 166, 328–338. doi:10.1016/j.catena.2018.04.018
Yashiro, Y., Shizu, Y., Hirota, M., Shimono, A., and Ohtsuka, T. (2010). The role of shrub (Potentilla fruticosa) on ecosystem CO2 fluxes in an alpine shrub meadow. J. Plant Ecol. 3, 89–97. doi:10.1093/jpe/rtq011
Yu, L., Chen, Y., Sun, W., and Huang, Y. (2019). Effects of grazing exclusion on soil carbon dynamics in alpine grasslands of the Tibetan Plateau. Geoderma 353, 133–143. doi:10.1016/j.geoderma.2019.06.036
Zechmeister-Boltenstern, S., Keiblinger, K., Mooshammer, M., Penuelas, J., Richter, A., Sardans, J., et al. (2015). The application of ecological stoichiometry to plant–microbial–soil organic matter transformations. Ecol. Monogr. 85, 133–155. doi:10.1890/14-0777.1
Zhang, R., Wang, Z., Han, G., Schellenberg, M. P., Wu, Q., and Gu, C. (2018). Grazing induced changes in plant diversity is a critical factor controlling grassland productivity in the Desert Steppe, Northern China. Agric. Ecosyst. Environ. 265, 73–83. doi:10.1016/j.agee.2018.05.014
Zhang, T., Xu, M., Zhang, Y., Zhao, T., and An, T. (2019). Grazing-induced increases in soil moisture maintain higher productivity during droughts in alpine meadows on the Tibetan Plateau. Agric. For. Meteorology 270, 249–256. doi:10.1016/j.agrformet.2019.02.022
Zhang, Y., Yao, S., Mao, J., Olk, D. C., Cao, X., and Zhang, B. (2015). Chemical composition of organic matter in a deep soil changed with a positive priming effect due to glucose addition as investigated by 13C NMR spectroscopy. Soil Biol. Biochem. 85, 137–144. doi:10.1016/j.soilbio.2015.03.013
Zhang, Z. C., Liu, M., Wei, T. X., Sun, J., and Ge, H. (2019). Degradation induces changes in the soil C:N:P stoichiometry of alpine steppe on the Tibetan Plateau. J. Mt. Sci. 16, 2348–2360. doi:10.1007/s11629-018-5346-y
Zhou, G., Luo, Q., Chen, Y., He, M., Zhou, L., Frank, D., et al. (2019b). Effects of livestock grazing on grassland carbon storage and release override impacts associated with global climate change. Glob. Change Biol. 25, 1119–1132. doi:10.1111/gcb.14533
Zhou, G., Luo, Q., Chen, Y., Hu, J., He, M., Gao, J., et al. (2019a). Interactive effects of grazing and global change factors on soil and ecosystem respiration in grassland ecosystems: A global synthesis. J. Appl. Ecol. 22, 13443–23169. doi:10.1111/1365-2664.13443
Zhou, G., Zhou, X., He, Y., Shao, J., Zhenhong, H., Liu, R., et al. (2017). Grazing intensity significantly affects belowground carbon and nitrogen cycling in grassland ecosystems: A meta-analysis. Glob. Change Biol. 23, 1167–1179. doi:10.1111/gcb.13431
Zhou, X., Wang, Z. B., and Du., Q. (1987). Vegetation of Qinghai. China. Xining: Qinghai people's Publishing house, 53–72.
Keywords: soil element, soil stoichiometry, controlling factors, Tibetan plateau, alpine shrublands, grazing
Citation: Nie X, Li C, Ren L, Chen Y, Du Y, Li X, Wang D and Zhou G (2023) Different responses of soil element contents and their stoichiometry (C: N: P) to different grazing intensity on the Tibetan Plateau shrublands. Front. Environ. Sci. 11:1170507. doi: 10.3389/fenvs.2023.1170507
Received: 21 February 2023; Accepted: 30 May 2023;
Published: 03 August 2023.
Edited by:
Anoop Kumar Srivastava, Central Citrus Research Institute (ICAR), IndiaReviewed by:
Suresh Kumar Malhotra, ICAR-Directorate of Knowledge Management in Agriculture, IndiaCopyright © 2023 Nie, Li, Ren, Chen, Du, Li, Wang and Zhou. This is an open-access article distributed under the terms of the Creative Commons Attribution License (CC BY). The use, distribution or reproduction in other forums is permitted, provided the original author(s) and the copyright owner(s) are credited and that the original publication in this journal is cited, in accordance with accepted academic practice. No use, distribution or reproduction is permitted which does not comply with these terms.
*Correspondence: Xiuqing Nie, bmlleGl1cWluZzEyM0AxNjMuY29t; Guoying Zhou, emhvdWd5QG53aXBiLmNhcy5jbg==
Disclaimer: All claims expressed in this article are solely those of the authors and do not necessarily represent those of their affiliated organizations, or those of the publisher, the editors and the reviewers. Any product that may be evaluated in this article or claim that may be made by its manufacturer is not guaranteed or endorsed by the publisher.
Research integrity at Frontiers
Learn more about the work of our research integrity team to safeguard the quality of each article we publish.