- 1Institute of Marine and Fisheries, Zhejiang Ocean University, Zhoushan, China
- 2Zhejiang Provincial Key Lab of Mariculture and Enhancement, Zhejiang Marine Fisheries Research Institute, Zhoushan, China
The combined pollution of microplastics (MPs) and persistent organic pollutants (POPs) have attracted increasing attention from the international community in recent years. MPs can affect the toxicity, bioenrichment rate and bioavailability of POPs through adsorption and other interactions. Dechlorane Plus (DP) is a chlorinated flame retardant and a typical. DPs used mainly in various polymer materials, such as electrical wire and cable coating. The pollutions of MPs and DPs (syn and anti isomers, syn-DP [s-DP] and anti-DP [a-DP]) are ubiquitously present in the environment. However, the effect of MPs on the bioaccumulation of DP has not been reported. In this study, thick-shell mussels (Mytilus coruscus) were exposed to DPs (10 ng/L, DP10), DPs and polystyrene microplastics (PS) (10 ng/L DP +10 μg/L PS, DP10/PS10; 10 ng/LDP+20 μg/L PS, DP10/PS20) for 28 days to investigate the effect of MPs on DPs enrichment. Thick-shell mussels accumulated DPs in the adductor muscle, gill, and gonad showed an increasing trend with time, but the concentration of DPs in the visceral mass does not show a time-dependent manner. The concentration of DPs in the gonads and gills was significantly affected by the concentration of PS (p < 0.05), but there was no significant correlation between the concentration of DPs and the concentration of PS in the adductor muscle and visceral mass (p > 0.05). Moreover, DPs showed selective enrichment of syn-DP in thick-shell mussel tissues, and there was no significant correlation between this result and PS concentration (p > 0.05). These findings provide a new entry point for studying the interaction between microplastics and persistent organic pollutants in marine organisms.
1 Introduction
Dechlorane Plus (DP) is a highly chlorinated cycloaliphatic flame retardant that has been manufactured since the 1960s (Gagné et al., 2017). It was introduced into the market as an alternative to Mirex, but has been extensively used today as an important additive in plastics, textiles, electronic appliances, plastic building materials, and other combustible products to improve their fire resistance (Alaee et al., 2003; Wang et al., 2016; Wang et al., 2016). DP is a low production volume chemical in the European Union but a high production volume chemical in the United States due to an estimated annual production of more than 450 t (Gagné et al., 2017). The production of DP in China was carried out by Anpon Company in Jiangsu with an estimated production of 300 t per year (Ren et al., 2018). Although DP has been widely manufactured and used for nearly 50 years, it received little attention until it was first discovered in sediments from the Great Lakes of North America in 2006 (Hoh et al., 2006). Further researches have shown that DP is present not only in air, water, and sediment, but also in aquatic species, human blood and hair, indicating that DP is a universal pollutant (Gauthier and Letcher, 2009; Wang et al., 2010; Zhao et al., 2011; Abdel Malak et al., 2018; Chen et al., 2018). DP has been determined in surface sediments of the Pearl River Estuary and Daya Bay from South China, and the concentrations were 0.004–2.05 ng/g dry weight (dw) and 0.005–0.24 ng/g dw, respectively (Hu et al., 2022). It has also been reported in fish, shrimp and bivalves of the Yellow River Delta (YRD), China, with a concentration ranging from 0.5 to 29 ng/g of lipid weight (lw) (Zhang et al., 2020). Furthermore, DP has been reported in the Antarctic biota (Na et al., 2017), the natural mangrove ecosystems of South China (Qiu et al., 2019), and the marine benthos of Longyearbyen, Svalbard (Carlsson et al., 2018).
The commercial product of DP contains two stereoisomers (syn-DP [s-DP] and anti-DP [a-DP]), and the a-DP accounts for approximately 75% of total DP. Due to differences in water solubility, photodegradation efficiency and other properties, DP isomers exhibits a stereoselective bioaccumulation manner in the environment (Moller et al., 2010; Zhao et al., 2011). Most of the aquatic organisms showed a selective enrichment of s-DP (Hoh et al., 2006; Tang et al., 2018; Zhang et al., 2020), which may be due to its higher assimilation efficiency and lower degradation efficiency (Hoh et al., 2006; Zhang et al., 2020). In addition, the dominance of s-DP in aquatic biotas may affect by the DP composition in environment matrices (Mo et al., 2013). The severe effects of DP have been reported in many organisms (Wu et al., 2012; Li et al., 2013a; Li et al., 2013b). Subacute toxicological results indicated that prolonged skin exposure or inhalation of high concentrations of DP could cause lung and liver tissue lesions (Wu et al., 2012; Li et al., 2013a; Li et al., 2013b). A study of Cyprinus carpio has suggested that exposure of carp embryos to concentrations of 30, 60 and 120 μg/L of DP after 3 h of fertilization significantly affects the transcription of mRNA of genes related to neural and skeletal development. Moreover, research shows that DP is genotoxic to Mediterranean mussels, and could induce oxidative stress and decrease cyclooxygenase activity in the blue mussel (Gagné et al., 2017). Thus, it is critical to focus on the risk and environmental fate of this pollutant (Chen et al., 2018; Zhou et al., 2019).
The United Nations Environmental Programme (UNEP, 2014) describes plastic debris in the marine environment as a key emerging global environmental problem. Microplastics (≤5 mm) are ubiquitous in the environment and have attracted widespread attention in recent years (Farady, 2019; Tien et al., 2020). The characteristics of microplastics depend on the raw material and chemical composition, and low-trophic level fauna prefer to absorb small size, high transparency of the microplastics (Wright et al., 2013) and are transferred to higher trophic levels along the food chain, resulting in the enrichment of microplastics in many marine organisms. Existing studies have shown that in Mytilus edulis, smaller (3.0 µm) polystyrene (PS) microspheres retain a stronger ability to transfer from the gut cavity to the hemolymph than larger (9.6 µm) ones (Browne et al., 2008). The particles then migrate through the circulatory system to various tissues. Microplastics of 0.5 µm can also be transferred from M. edulis to Carcinus maenas through trophic transfer (Browne et al., 2008; Farrell and Nelson, 2013). In addition to directly affecting the physiological functions of marine organisms, MPs also have a highly hydrophobic surface that enable them to act a vector of chemical transport into marine organisms. They are highly compatible with and are capable of adsorbing hydrophobic organic pollutants, which makes them potential carriers of persistent organic pollutants, such as DP, polybrominated diphenyl ethers (PBDEs), and polychlorinated biphenyls (PCBs) in the marine environment (Cole et al., 2011; Besseling et al., 2013; Jeong et al., 2018; Turner, 2018). Microplastics are reported to account for more than 90% among marine plastic debris and have been identified in many environmental matrices globally. Their environmental presence and accumulation are increasing each year around the world due to the increase in the world plastic production that also usually contains increasing kinds of additives, such as the brominated flame retardants, chlorinated flame retardants, and phthalates, which added to the material to improve plastic characteristics. There is evidence that microplastics can affect bioaccumulation or change the toxic effects of organic pollutants by adsorption and interaction (Chen et al., 2017; Zhu et al., 2019). A reduction in feeding activity and increased PCBs bioaccumulation were observed at a low does of PS in Arenicola marina (Besseling et al., 2013). In addition, the presence of microplastic PS (micro-PS) amplified the negative effect of decabromodiphenyl ether (BDE-209) on hemocyte phagocytosis and histopathology of Chlamys farreri, and the carrier role of micro-PS greater than its scavenger role for BDE-209 (Bin et al., 2019). Gu et al. (2020) proposed that micro-PS aggravates the adverse effects of 2, 2′, 4, 4′-tetrabromodiphenyl ether (BDE-47) on physiological and defensive performance in Mytilus coruscus (Gu et al., 2020).
The thick-shell mussel, M. coruscus, is an economically important aquatic invertebrates that is widely distributed along the coast of the East China Sea, especially on the coast of Zhoushan, China (Wu et al., 2017). As a typical marine bivalve mollusc, M. coruscus easily accumulates environmental pollutants in the body due to its filter-feeding mode, offering an ideal indicator for the long-term assessment of environmental pollution in a certain area (Livingstone, 1991). In addition, their ability to accumulate and tolerate high concentrations of pollutants has made them useful biomonitoring tools for identifying temporal and geographical pollution trends (Booij et al., 2002).
Ecotoxicological studies on micro-PS and DP are well-represented in the literature. Unfortunately, there is little information on the combined exposure of micro-PS and DP as pollutants and their bioaccumulation in aquatic species, particularly in bivalves. Therefore, in the present study, thick-shell mussels were exposed to selected doses of DP and PS, and the accumulation and stereoselectivity of DP in the adductor muscle, gill, gonad and visceral mass were analyzed to investigate the effects of PS on the bioaccumulation of DP and to provide a basis for further research on the effects of interactions between PS and DP on the health of aquatic organisms.
2 Materials and methods
2.1 Chemicals and materials
Individual solutions of s-DP (purity ≥ 98.0%, CAS 135821-03-3), a-DP (purity ≥ 98.0%, CAS 135821-74-8) isomers (50 g/mL, in toluene), n-Hexane (water ≤ 0.01%, CAS 110-54-3) and acetone (water = 0.5%, CAS 67-64-1) were purchased from ANPEL Laboratory Technologies Inc. (Shanghai, China). Polystyrene beads (diameter 20 µm) were obtained from the BaseLine ChromTech Research Centre (Tianjin, China). S-DP and a-DP were dissolved in an acetone solution to obtain 5 g/mL stock solutions. The microplastics were diluted to 10 g/L with Milli-Q water and stored at 4°C away from light for later use. Silica gel (100–140 mesh) was supplied by ANPEL Laboratory Technologies Inc. and activated overnight at 180°C. The solvents and reagents used were of analytical grade. Anhydrous sodium sulfate (CAS 7757-82-6) was purchased from Maclin Biochemical Technology (Shanghai, China).
2.2 Experimental design
Adult mussels (n = 160, half female and half male) with shell lengths of 70–90 mm were collected from Zhoushan City, Zhejiang Province, China. Mussels were housed in a light- and temperature-controlled aquaculture facility with a light: dark period of 14: 10 h at a temperature of 19°C ± 1°C. Mussels were fed a phytoplankton (4–10 µm) suspension (PhytōGold-S; Brightwell Aquatics, PA, United States) twice daily. After 7 days of acclimatization in artificial seawater, all mussels were randomly assigned to eight tanks (20 L volume). Each closed-loop system provided 15 L of aerated artificial seawater (Tropic Marin, Wartenberg, Germany) with a salinity of 30 g/L for the duration of the experiment. Four experimental conditions were set up in duplicate: control, 10 ng/L DP (DP10), and DP + micro-PS (10 ng/L DP + 10 μg/L PS [DP10/PS10], 10 ng/L DP + 20 μg/L PS [DP10/PS20]). The selection of the concentration of DP is the same as in a previous study (Gagné et al., 2017) and on the basis of the reported concentration of DP of water samples from the coastal shore of northern China (up to 1.8 ng/L) (Jia et al., 2011). The entire volume of water in each experimental tank was replaced daily with artificial seawater containing the corresponding concentrations of PS and DP, and the water quality was checked daily. Then, four mussels sacrificed at the 3rd, 10th, 15th, 21st, and 28th day after changed the solution. The gonads, adductor muscles, gills, and visceral masses of the mussels were collected. All animals were treated in accordance with the criteria outlined in the Guide for the Care and Use of Laboratory Animals of the National Institute of Health. The Ethics Committee of Zhejiang Ocean University approved all animal experiments.
2.3 Sample preparation
The tissues were freeze-dried, weighed, and homogenized with anhydrous sodium sulfate and the target DP was extracted from the tissues using a 20 mL mixture of hexane and acetone (1:1, v/v). The samples were centrifuged at 3,000 rpm for 3 min (Centrifuge 5,810, Eppendorf, Hamburg, Germany), and the extraction procedure was repeated three times. The extract was concentrated to 1 mL using a rotary evaporator (R210, Buchi Laboratory Equipment Trading Ltd., Shanghai, China) and reconstituted to 10 mL with hexane. The lipid content of the tissues was analyzed using the gravimetric method (Kim et al., 2015). Briefly, 1 mL of lipid content was determined by gravimetry. The remaining 9 mL was transferred to a new glass centrifuge tube and sulfonated with 6 mL concentrated sulfuric acid (China National Pharmaceutical Group, Beijing, China), shaken, swirled, and separated at 5,000 rpm for 5 min. Subsequently, the lower layer of the colored phase was discarded, and sulfonation was repeated until the upper liquid level became clear. Milli-Q water was then added, and the sample was centrifuged at 3,000 rpm for 3 min. The supernatant was then concentrated at a reduced pressure at 35°C in a rotary evaporator to a volume of 1 mL.
The extracts were cleaned on multi-layer columns (1 cm internal diameter, i.d.) that had been wet-loaded sequentially with 8 cm deactivated silica gel (silica gel: Milli-Q water, w/w, 3%), 8 cm acidic silica gel (silica gel: sulfuric acid, w/w, 44%), and 2 cm anhydrous sodium sulfate from the bottom to the top. The column was activated with 4 mL of hexane solution. After the extract solution was added to the column, the rotary vial containing the sample solution was eluted four times with 40 mL of hexane/dichloromethane (1:1, v/v), and the eluent solution was then added to the column until the extract solution almost entered the filler, and the effluent was collected in a rotary flask. The purified solution was concentrated to 200 µL using rotary evaporation for Gas chromatography-mass spectrometry (GC-MS) analysis (Agilent 7890B-7000C, Agilent Technologies, Santa Clara, CA, United States). The collected mixed solvent was rotary evaporated to 1 mL, blown to near dryness under a gentle N2 stream (N-EVAP-112, Organomation, MI, United States), and redissolved in 50 µL of hexane for GC-MS analysis.
2.4 Instrumental analysis
S-DP and a-DP were determined by GC-MS with a DB-5HT capillary column (15 m × 0.25 mm × 0.1 μm, Agilent 122-5711, Agilent Technologies, Santa Clara, CA, United States), using an electron capture negative ionization (ECNI) source and in the selective ion-monitoring (SIM) mode. Samples (1 µL) were injected in the splitless mode at an injection port temperature of 280°C. The GC oven temperature started at 110°C for 1 min, increased to 290°C (and maintained there for 1 min) at 25°C/min, then ramped to 320°C at 30°C/min, and was held for 10 min. The monitoring ions were 653.8 and 655.8. The target analytes were quantified using an internal calibration method based on five-point calibration curves for the individual compounds.
2.5 Quality assurance/quality control
All analytical procedures were monitored using strict quality assurance and control measures. To avoid interference from potential contaminants, program blanks were analyzed simultaneously with samples, and the blank sample was tested below the quantitative limit. The linear coefficient (r2) of the correction curves for all targets was greater than 0.99. The method detection limits (MDLs) of s-DP and a-DP were three times the standard deviation of the blank target value. For compounds that were not detected in the blank, MDLs were defined as concentrations with a signal-to-noise ratio of 5:1. The average recovery of s-DP was 81.4% ± 5.1%, and that of a-DP was 85.6% ± 7.4%.
2.6 Statistical analysis
The results obtained were expressed as mean ± standard error. Comparisons of bioaccumulations between point of time and among different mixtures concentrations of polystyrene microplastics (PS) on Dechlorane Plus (DP), using a two-way analysis of variance (ANOVA) with interactions between the two factors (days and concentration), followed by the Tukey multiple comparison test. A p-value <0.05 was considered statistically significant.
3 Results
3.1 Dechlorane plus quantification in mussel tissues
Dechlorane Plus was not detected in any of the tissues tested from the thick-shell mussels of the control group. All concentrations are reported as means ± SE.
3.1.1 Visceral mass dechlorane plus concentrations
DP concentrations in the visceral mass of thick-shell mussels increased in the first 15 days of exposure in all three treatment groups and then decreased in the remaining exposure time in the DP10 and DP10/PS20 group (Figure 1A). The highest DP concentration in the DP10, DP10/PS10 and DP10/PS20 group appeared on the 15th day, with the value of 761.00 ± 81.53, 707.20 ± 153.30, 673.30 ± 100.20 ng/g dw, respectively (Table 1). The lowest concentration of DP in visceral mass has been detected in the DP10/PS20 group, except on the 3rd day of exposure.
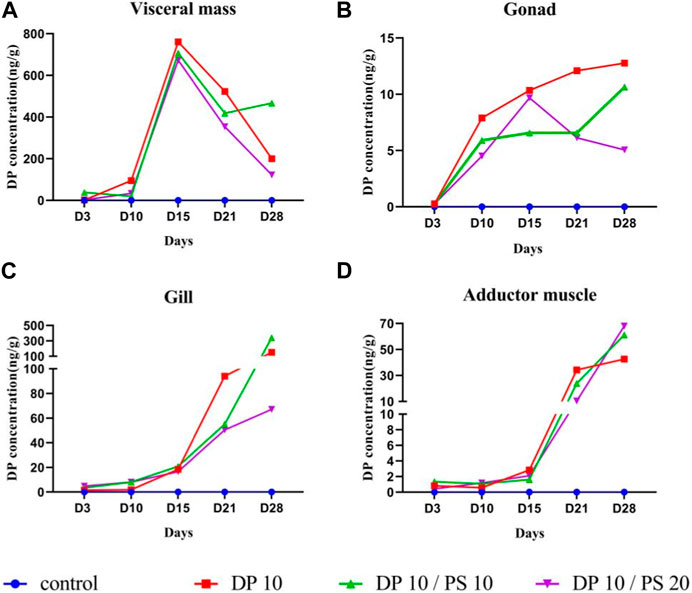
FIGURE 1. Concentrations (ng/g, dw) of Dechlorane Plus in different tissues of thick-shell mussels after exposure phases. Note: (A) Visceral mass; (B) Gonad; (C) Gill; (D) Adductor muscle; PS, Polystyrene; DP, Dechlorane Plus. Results are expressed as the mean concentration (n = 4).
A two-way ANOVA of the obtained data revealed that the bioaccumulation of DP in visceral mass was only significantly affected by exposure time (p < 0.01), but not by PS concentration (p = 0.5095). In addition, there was no significant difference in DP concentrations between the three groups on the same days of exposure.
3.1.2 Gonadal dechlorane plus concentrations
DP concentrations in the gonad of thick-shell mussels were increased throughout the exposure period in the DP10 and DP10/PS10 groups, while the DP level increases during the first 15 days and then decreases in the DP10/PS10 group (Figure 1B). The maximum value of DP concentrations in the gonad of thick-shell mussels of DP10, DP10/PS10 and DP10/PS20 were 12.76 ± 3.99, 15.64 ± 3.44 and 9.68 ± 3.42 ng/g dw, respectively (Table 1). DP concentrations in the gonad of the same number of exposure days decreased from DP10 to DP10/PS10 to DP10/PS20 expect the 15th day. Different from the situation in the visceral mass, bioaccumulation of DP in the gonads was significantly affected by both exposure time and PS concentration (p < 0.05).
3.1.3 Dechlorane plus concentrations in gill tissues
The addition of microplastics and longer exposure times significantly increased DP enrichment in the gills (two-way analysis of variance, p < 0.001). DP bioaccumulation in the thick-shell mussel gills of the three experimental groups showed a time-dependent increase during the 28 days of exposure (Figure 1C). DP concentrations in gills from DP10, DP10/PS10 and DP10/PS20 groups ranged from 1.52 ± 0.33 to 151.5 ± 11.56, 3.41 ± 1.64 to 341.40 ± 41.55 and 4.82 ± 1.10 to 67.11 ± 16.69 ng/g dw,respectively (Table 1). Specifically, concentrations of DP increased in the 28 days approximately 100 times in the DP10 and DP10/PS10 group and increased approximately 14 times in the DP10/PS20 group. Gills of the thick-shell mussel in DP10/PS10 group accumulated more DP than the other two groups Figure 2.
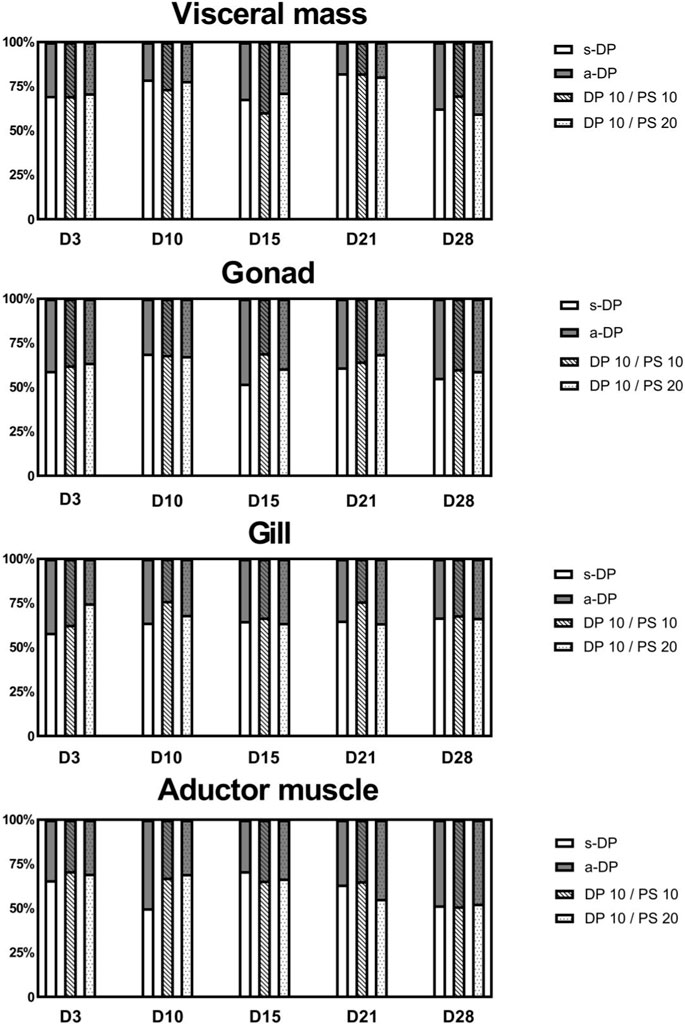
FIGURE 2. The proportion of syn-DP and anti-DP in different thick-shell mussel tissues of each experimental group after exposure phases.
3.1.4 Adductor muscle dechlorane plus concentrations
The DP enrichment in the adductor muscle tissues was similar to that in the gills, with a slow increase in concentration at the 3rd day to the 15th day and a dramatic increase in the following 13 days (Figure 1D). DP concentrations in the adductor muscle significantly affected by exposure time (p < 0.01). On day 28, maximum DP concentrations were observed in DP10, DP10/PS10 and DP10/PS20 group with the values of 42.51 ± 10.37, 61.2 ± 10.45 and 67.96 ± 17.43 ng/g dw, respectively (Table 1).
3.2 Comparison of s-DP and a-DP proportion in tissues
A selective enrichment of DP was observed in all tissues of thick-shell mussels throughout the period of the exposure experiment, with the fanti (fraction of a-DP to ΣDP) value ranging from 0.18 to 0.40, 0.31 to 0.48, 0.24 to 0.42, and 0.27 to 0.50 in visceral mass, gonads, gills, and adductor muscle, respectively. There were no significant differences in the fanti values between different tissues (p > 0.05).
Since the two individual DP isomers solution were used in the exposure experiments, the proportions of the two isomers are equal (fanti = 0.5). Almost all four tissues showed selective enrichment of s-DP. On the 3rd day of exposure, fanti showed a tendency to decrease with the increasing of PS concentration in the four tissues, but this trend disappeared with the duration of the exposure experiment.
4 Discussion
Microplastics are small, have a large specific surface area, and are strongly hydrophobic, making them ideal carriers for many pollutants in the environment. Various POPs, such as PCBs, dichlorobiphenyl trichloroethane (DDT), hexachlorocyclohexanes (HCHs), and polycyclic aromatic hydrocarbons (PAHs) have been detected on microplastics from beaches around the world (Ogata et al., 2009; Hirai et al., 2011; Heskett et al., 2012; Rochman et al., 2013). It is widely believed that microplastics can affect the environmental behavior of organic pollutants and have a significant influence on the key processes of migration, transformation, and bioenrichment (Lee et al., 2014). Studies have found substantial POP enrichment in polymers, where concentrations are often more than 100 fold their concentrations in solution (Lohmann, 2017). In addition, aquatic organisms are prone to ingesting microplastics carrying organic pollutants during the feeding process (Egbeocha et al., 2018).
Until now, the effects of PS on the bioaccumulation of frequently detected POPs, DPs in the thick-shell mussel tissues are still unknown. The result of the experiment indicated that no significant effects of PS on the distribution of DP among tissues of thick-shell mussel were observed.
A higher concentration of DP accumulated in the visceral mass of the three treatment groups after 10 days of exposure. This may be attributed to the fact that the visceral mass in this study contained the liver and the intestinal tract, which are usually the sites where organisms have higher concentrations of POPs due to the high amounts of fat and the transformation of MPs, e.g., after 7 days exposure, all three types of MPs (PE, PP, PVC) were identified in the liver and intestinal tract of zebrafish but none MPs were observed in the brain and gonad, also, the concentration of triclosan in the gut (191.56 μg/g) and liver (63.92 μg/g) was higher than that in the brain (21.32 μg/g) and gonad (23.19 μg/g) (p < 0.05) of the zebrafish (Sheng et al., 2021). Furthermore, the concentration in the visceral mass of DP10/PS10 group was higher than in the other two groups. Previous studies have reported similar results of polychlorinated biphenyls (PCBs), where a low concentration of microplastics (the PS concentration is equivalent to 0.074% DW PS in sediment) can improve the bioenrichment and toxicity of POPs, but at higher doses (7.4% DW PS in sediment), bioaccumulation decreased compared to the low dose (Besseling et al., 2013).
The DP concentration in the gills follows that of the visceral mass, indicating that gill has a stronger DP enrichment ability in this exposure experiment. This may be due to the filter feeders filtering and ingesting food and pollutants in water through their gills. Microplastics adsorb DP and are ingested during the feeding process, leading to an increase in DP content in the gills (Fossi et al., 2014). DP bioaccumulation in gill of three treatment groups is similar to that of visceral mass, briefly, a low concentration of PS facilitates the DP enrichment, while a higher concentration of PS reduced the enrichment in the gills.
DP concentrations in the gills and adductor muscles of the thick-shell mussel showed a time-dependent increased, especially from the 15th to 28th day. The result may suggest that co-exposure-treated mussels exhibited only a moderate increase during the exposure phases. The rapid uptake in the gills and adductor muscles may be explained by the direct contact of these tissues with chemicals dissolved in water (Banni et al., 2017; Lucia Pittura et al., 2018).
Of the four tissues, the gonad has the lowest DP concentration, possibly because it could not adsorb the pollutant directly from surrounding seawater like other tissues. The DP concentration in the DP10 group was higher than in the other two groups with a co-presence of PS, which may be due to the negative concentration gradient between the pollutants loaded onto microplastics and those in biological lipids (Magara et al., 2018). Additionally, the result may suggest a reduction in DP migration ability in vivo that caused by the interaction between DP and PS in other tissues. But further research is needed to supply evidence for that.
In this study, two DP monomers were used in the exposure experiments. Therefore, the DP composition in the mussels reflects the selective enrichment of the two DP isomers, regardless of the proportion of s-DP and a-DP in industrial DP products. The results showed that on day 3, some individuals in the mixed-exposure group of PS and DP had an fanti value of 1 in the adductor muscle, possibly because the concentration of s-DP was below the detection limit, a similar result was observed in exposure with low concentration DP (0.001 ng/L) to the blue mussel (Gagné et al., 2017). Because the response factor of a-DP on common GC-MS is higher than that of s-DP, when the total DP content is low, a-DP can be detected, while s-DP cannot (Ghelli et al., 2021). Additionally, on day 3, the proportion of a-DP in the total DP of each tissue in the experimental group treated with co-exposure was lower than in the group treated with DP alone. The reason for this phenomenon may be similar to the enrichment of s-DP in Oncorhynchus mykiss, and s-DP has higher assimilation efficiency and lower purification speed compared with a-DP (Tomy et al., 2008). In addition, selective enrichment of s-DP was found in all the tissue types, which suggests the selective enrichment of s-DP in aquatic organisms (Hoh et al., 2006; Klosterhaus et al., 2012) and the possible existence of selective metabolism or excretion of a-DP (Zeng et al., 2014; Tang et al., 2018). However, the fanti value increased slightly with increasing exposure time, and the concentration of a-DP in some individual tissues was even greater than that of s-DP on the end of the experiment. Continuous exposure to pollutants may damage the metabolic system of mussels, thus affecting the selective metabolism of a-DP. In terms of the overall DP composition, fanti values (in increasing order) in different tissues were visceral mass, gills, adductor muscles, and gonads, which might be caused by the differences in fat content and metabolic rate of DP in the different tissues. The selective enrichment of DP was affected by the DP concentration in organisms (Chen et al., 2013), tissue fat content (Peng et al., 2012), and other factors, but not by the addition of microplastics.
5 Conclusion
The co-exposure of DP and PS in thick-shell mussels resulted in a time-dependent bioaccumulation of DP. The concentration of DP in all tissues, except the visceral mass gradually increased with increasing exposure time. The concentration of DP in the visceral mass first increased and then decreased after 15 days of exposure, which may be due to liver detoxification. PS significantly affected DP bioaccumulation in the gonads and gills, but did not significantly affect the bioaccumulation of DP in the visceral mass and adductor muscles, which might be caused by the filter-feeding habit of mussels and the transfer of DP in vivo. The results of the composition of the DP isomer in thick-shell mussels showed that s-DP was selectively enriched in all mussel tissues regardless of the concentration of PS in the exposed group, which was similar to the results of the selective enrichment of DP in the environment. In view of the increased production and increasing detection of both DP and PS in aquatic environment around the world, the combined pollution of PS and DP should be a subject of long-term monitoring; furthermore, the toxicity of the combined pollution of PS and DP to aquatic organisms would also require further research.
Data availability statement
The raw data supporting the conclusion of this article will be made available by the authors, without undue reservation.
Author contributions
Conceptualization, QX and XP; methodology, QH and CY; software, XP; validation, QH and YG; formal analysis, QX and YH; investigation, QX; resources, QH; data curation, XP; writing—original draft preparation, QX; writing—review and editing, QH; visualization, YG; supervision, YG; project administration, YG All authors have read and agreed to the published version of the manuscript. All authors listed have made a substantial, direct, and intellectual contribution to the work and approved it for publication. All authors contributed to the article and approved the submitted version.
Funding
This work was supported by the Natural Science Foundation of Zhejiang Province, China under contract LQ20D030002 and the National Natural Science Foundation of China under contract 31800430.
Conflict of interest
The authors declare that the research was conducted in the absence of any commercial or financial relationships that could be construed as a potential conflict of interest.
Publisher’s note
All claims expressed in this article are solely those of the authors and do not necessarily represent those of their affiliated organizations, or those of the publisher, the editors and the reviewers. Any product that may be evaluated in this article, or claim that may be made by its manufacturer, is not guaranteed or endorsed by the publisher.
References
Abdel Malak, I., Cariou, R., Vénisseau, A., Dervilly-Pinel, G., Jaber, F., Babut, M., et al. (2018). Occurrence of dechlorane plus and related compounds in catfish (silurus spp) from rivers in France. Chemosphere 207, 413–420. doi:10.1016/j.chemosphere.2018.05.101
Alaee, M., Arias, P., Sjodin, A., and Bergman, A. (2003). An overview of commercially used brominated flame retardants, their applications, their use patterns in different countries/regions and possible modes of release. Environ. Int. 29, 683–689. doi:10.1016/S0160-4120(03)00121-1
Banni, M., Sforzini, S., Arlt, V. M., Barranger, A., Dallas, L. J., Oliveri, C., et al. (2017). Assessing the impact of benzo[a]pyrene on marine mussels: Application of a novel targeted low density microarray complementing classical biomarker responses. Plos One 12, e0178460. doi:10.1371/journal.pone.0178460
Besseling, E., Wegner, A., Foekema, E. M., van den Heuvel-Greve, M. J., and Koelmans, A. A. (2013). Effects of microplastic on fitness and pcb bioaccumulation by the lugworm arenicola marina (l). Environ. Sci. Technol. 47, 593–600. doi:10.1021/es302763x
Bin, J. Z., Xia, X. Z., Lin, X. S., and Zhu, K. Q. (2019). Polystyrene microplastics increase uptake, elimination and cytotoxicity of decabromodiphenyl ether (bde-209) in the marine scallop chlamys farreri. Environ. Pollut. 258, 113657. doi:10.1016/j.envpol.2019.113657
Booij, K., Zegers, B. N., and Boon, J. P. (2002). Levels of some polybrominated diphenyl ether (pbde) flame retardants along the Dutch coast as derived from their accumulation in spmds and blue mussels (mytilus edulis). Chemosphere 46, 683–688. doi:10.1016/s0045-6535(01)00232-6
Browne, M. A., Dissanayake, A., Galloway, T. S., Lowe, D. M., and Thompson, R. C. (2008). Ingested microscopic plastic translocates to the circulatory system of the mussel, mytilus edulis (l). Environ. Sci. Technol. 42, 5026–5031. doi:10.1021/es800249a
Carlsson, P., Vrana, B., Sobotka, J., Borgå, K., Nizzetto, P. B., and Varpe, Ø. (2018) New brominated flame retardants and dechlorane plus in the Arctic: Local sources and bioaccumulation potential in marine benthos. Chemosphere 211 (11), 1193–1202. doi:10.1016/j.chemosphere.2018.07.158
Chen, D., Wang, Y., Yu, L., Luo, X., Mai, B., and Li, S. (2013). Dechlorane plus flame retardant in terrestrial raptors from northern China. Environ. Pollut. 176, 80–86. doi:10.1016/j.envpol.2013.01.015
Chen, Q. Q., Gundlach, M., Yang, S. Y., Jiang, J., Velki, M., Yin, D., et al. (2017). Quantitative investigation of the mechanisms of microplastics and nanoplastics toward zebrafish larvae locomotor activity. Sci. total Environ. 584, 1022–1031. doi:10.1016/j.scitotenv.2017.01.156
Chen, X., Zhu, Y., Huang, Q., Liu, J., Liu, B., and Zhang, Y. (2018). Distributions, influencing factors, and risk assessment of dechlorane plus and related compounds in surficial water and sediment from the jiulong river estuary, southeast China. Environ. Sci. Pollut. Res. Int. 25, 30292–30300. doi:10.1007/s11356-018-2874-5
Cole, M., Lindeque, P., Halsband, C., and Galloway, T. S. (2011). Microplastics as contaminants in the marine environment: A review. Mar. Pollut. Bull. 62, 2588–2597. doi:10.1016/j.marpolbul.2011.09.025
Egbeocha, C. O., Malek, S., Emenike, C. U., and Milow, P. (2018). Feasting on microplastics: Ingestion by and effects on marine organisms. Aquat. Biol. 27, 93–106. doi:10.3354/ab00701
Farady, S. E. (2019). Microplastics as a new, ubiquitous pollutant: Strategies to anticipate management and advise seafood consumers. Mar. Policy 104, 103–107. doi:10.1016/j.marpol.2019.02.020
Farrell, P., and Nelson, K. (2013). Trophic level transfer of microplastic: mytilus edulis (l) to carcinus maenas (l). Environ. Pollut. 177, 1–3. doi:10.1016/j.envpol.2013.01.046
Fossi, M. C., Coppola, D., Baini, M., Giannetti, M., Guerranti, C., Marsili, L., et al. (2014). Large filter feeding marine organisms as indicators of microplastic in the pelagic environment: The case studies of the mediterranean basking shark (cetorhinus maximus) and fin whale (balaenoptera physalus). Mar. Environ. Res. 100, 17–24. doi:10.1016/j.marenvres.2014.02.002
Gagné, P. L., Fortier, M., Fraser, M., Parent, L., Vaillancourt, C., and Verreault, J. (2017). Dechlorane Plus induces oxidative stress and decreases cyclooxygenase activity in the blue mussel. Aquat. Toxicol. 188, 26–32. doi:10.1016/j.aquatox.2017.04.009
Gauthier, L. T., and Letcher, R. J. (2009). Isomers of dechlorane plus flame retardant in the eggs of herring gulls (larus argentatus) from the laurentian great lakes of North America: Temporal changes and spatial distribution. Chemosphere 75, 115–120. doi:10.1016/j.chemosphere.2008.11.030
Ghelli, E., Cariou, R., Dervilly, G., Pagliuca, G., and Gazzotti, T. (2021). Dechlorane plus and related compounds in food-a review. Int. J. Environ. Res. Public Health 18, 690. doi:10.3390/ijerph18020690
Gu, H., Wei, S., Hu, M., Wei, H., Wang, X., Shang, Y., et al. (2020). Microplastics aggravate the adverse effects of bde-47 on physiological and defense performance in mussels. J. Hazard Mater 398, 122909. doi:10.1016/j.jhazmat.2020.122909
Heskett, M., Takada, H., Yamashita, R., Yuyama, M., Ito, M., Geok, Y. B., et al. (2012). Measurement of persistent organic pollutants (pops) in plastic resin pellets from remote islands: Toward establishment of background concentrations for international pellet watch. Mar. Pollut. Bull. 64, 445–448. doi:10.1016/j.marpolbul.2011.11.004
Hirai, H., Takada, H., Ogata, Y., Yamashita, R., Mizukawa, K., Saha, M., et al. (2011). Organic micropollutants in marine plastics debris from the open ocean and remote and urban beaches. Mar. Pollut. Bull. 62, 1683–1692. doi:10.1016/j.marpolbul.2011.06.004
Hoh, E., Zhu, L., and Hites, R. A. (2006). Dechlorane plus, a chlorinated flame retardant, in the great lakes. Environ. Sci. Technol. 40, 1184–1189. doi:10.1021/es051911h
Hu, Y. X., Li, Z., Xiong, J., Zhang, Z., Yuan, J., Tang, Y., et al. (2022). Occurrence and ecological risks of brominated flame retardants and dechlorane plus in sediments from the pearl river estuary and daya bay, south China. Mar. Pollut. Bull. 185, 114182. doi:10.1016/j.marpolbul.2022.114182
Jeong, C. B., Kang, H. M., Lee, Y. H., Kim, M. S., Lee, J. S., Seo, J. S., et al. (2018). Nanoplastic ingestion enhances toxicity of persistent organic pollutants (pops) in the monogonont rotifer brachionus koreanus via multixenobiotic resistance (mxr) disruption. Environ. Sci. Technol. 52, 11411–11418. doi:10.1021/acs.est.8b03211
Jia, H., Sun, Y., Liu, X., Yang, M., Wang, D., Qi, H., et al. (2011). Concentration and bioaccumulation of dechlorane compounds in coastal environment of northern China. Environ. Sci. Technol. 45, 2613–2618. doi:10.1021/es103723h
Kim, U. J., Jo, H., Lee, I. S., Joo, G. J., and Oh, J. E. (2015). Investigation of bioaccumulation and biotransformation of polybrominated diphenyl ethers, hydroxylated and methoxylated derivatives in varying trophic level freshwater fishes. Chemosphere 137, 108–114. doi:10.1016/j.chemosphere.2015.05.104
Klosterhaus, S. L., Heather, M. S., Mark, J. L. G., and Denise, J. G. D. (2012). Brominated and chlorinated flame retardants in san francisco bay sediments and wildlife. Environ. Int. 47, 56–65. doi:10.1016/j.envint.2012.06.005
Lee, H., Shim, W. J., and Kwon, J. H. (2014). Sorption capacity of plastic debris for hydrophobic organic chemicals. Sci. Total Environ. 470-471, 1545–1552. doi:10.1016/j.scitotenv.2013.08.023
Li, Y., Yu, L., Wang, J., Wu, J., Mai, B., and Dai, J. (2013a). Accumulation pattern of dechlorane plus and associated biological effects on rats after 90 d of exposure. Chemosphere 90, 2149–2156. doi:10.1016/j.chemosphere.2012.10.106
Li, Y., Yu, L., Zhu, Z., Dai, J., Mai, B., Wu, J., et al. (2013b). Accumulation and effects of 90-day oral exposure to dechlorane plus in quail (coturnix coturnix). Environ. Toxicol. Chem. 32, 1649–1654. doi:10.1002/etc.2202
Livingstone, D. R. (1991). Towards a specific index of impact by organic pollution for marine invertebrates. Comp. Biochem. Physiology Part C Comp. Pharmacol. 100, 151–155. doi:10.1016/0742-8413(91)90143-H
Lohmann, R. (2017). Microplastics are not important for the cycling and bioaccumulation of organic pollutants in the oceans-but should microplastics be considered pops themselves? Integr. Environ. Assess. Manag. 13, 460–465. doi:10.1002/ieam.1914
Lucia Pittura, C. G. A. M., Giuseppe, D. E., Steffen, H. K., Bettie Cormier, S. G. A. F., Keiter, S. H., Cormier, B., et al. (2018). Microplastics as vehicles of environmental pahs to marine organisms: Combined chemical and physical hazards to the mediterranean mussels, mytilus galloprovincialis. Front. Mar. Sci. 5, 103. doi:10.3389/fmars.2018.00103
Magara, G., Elia, A. C., Syberg, K., and Khan, F. R. (2018). Single contaminant and combined exposures of polyethylene microplastics and fluoranthene: Accumulation and oxidative stress response in the blue mussel, mytilus edulis. J. Toxicol. Environ. Health a 81, 761–773. doi:10.1080/15287394.2018.1488639
Mo, L., Wu, J. P., Luo, X. J., Sun, Y. X., Zou, F. S., Mai, B. X., et al. (2013). Dechlorane Plus flame retardant in kingfishers (Alcedo atthis) from an electronic waste recycling site and a reference site, South China: Influence of residue levels on the isomeric composition. Environ. Pollut. 174, 57–62. doi:10.1016/j.envpol.2012.11.007
Moller, A., Xie, Z., Sturm, R., and Ebinghaus, R. (2010). Large-scale distribution of dechlorane plus in air and seawater from the arctic to Antarctica. Environ. Sci. Technol. 44, 8977–8982. doi:10.1021/es103047n
Na, G. S., Yao, Y., Gao, H., Li, R. J., Ge, L. K., Titaley, I. A., et al. (2017). Trophic magnification of dechlorane plus in the marine food webs of fildes peninsula in Antarctica. Mar. Pollut. Bull. 117 (1-2), 456–461. doi:10.1016/j.marpolbul.2017.01.049
Ogata, Y., Takada, H., Mizukawa, K., Hirai, H., Iwasa, S., Endo, S., et al. (2009). International pellet watch: Global monitoring of persistent organic pollutants (pops) in coastal waters. 1. Initial phase data on pcbs, ddts, and hchs. Mar. Pollut. Bull. 58, 1437–1446. doi:10.1016/j.marpolbul.2009.06.014
Peng, H., Zhang, K., Wan, Y., and Hu, J. (2012). Tissue distribution, maternal transfer, and age-related accumulation of dechloranes in Chinese sturgeon. Environ. Sci. Technol. 46, 9907–9913. doi:10.1021/es3025879
Qiu, Y. W., Qiu, H. L., Zhang, G., and Li, J. (2019). Bioaccumulation and cycling of polybrominated diphenyl ethers (PBDEs) and dechlorane plus (DP) in three natural mangrove ecosystems of South China. Sci. Total Environ. 651, 1788–1795. doi:10.1016/j.scitotenv.2018.10.055
Ren, G., Zhang, J., Yu, Z., Chen, L., and Zheng, K. (2018). Dechlorane plus (DP) in indoor and outdoor air of an urban city in south China: Implications for sources and human inhalation exposure. Environ. Forensic 19 (2), 155–163. doi:10.1080/15275922.2018.1448907
Rochman, C. M., Hoh, E., Hentschel, B. T., and Kaye, S. (2013). Long-term field measurement of sorption of organic contaminants to five types of plastic pellets: Implications for plastic marine debris. Environ. Sci. Technol. 47, 1646–1654. doi:10.1021/es303700s
Sheng, C., Zhang, S., and Zhang, Y. (2021). The influence of different polymer types of microplastics on adsorption, accumulation, and toxicity of triclosan in zebrafish. J. Haz Mat. 402, 123733. doi:10.1016/j.jhazmat.2020.123733
Tang, B., Luo, X. J., Huang, C. C., Sun, R. X., Wang, T., Zeng, Y. H., et al. (2018). Stereoselective bioaccumulation of syn- and anti-dechlorane plus isomers in different tissues of common carp (cyprinus carpio). Sci. Total Environ. 616-617, 1339–1346. doi:10.1016/j.scitotenv.2017.10.183
Tien, C. J. W. Z., Wang, Z. X., and Chen, C. S. (2020). Microplastics in water, sediment and fish from the fengshan river system: relationship to aquatic factors and accumulation of polycyclic aromatic hydrocarbons by fish. Environ. Pollut. 265, 114962. doi:10.1016/j.envpol.2020.114962
Tomy, G. T., Thomas, C. R., Zidane, T. M., Murison, K. E., Pleskach, K., Hare, J., et al. (2008). Examination of isomer specific bioaccumulation parameters and potential in vivo hepatic metabolites of syn- and anti-dechlorane plus isomers in juvenile rainbow trout (oncorhynchus mykiss). Environ. Sci. Technol. 42, 5562–5567. doi:10.1021/es800220y
Turner, A. (2018). Black plastics: Linear and circular economies, hazardous additives and marine pollution. Environ. Int. 117, 308–318. doi:10.1016/j.envint.2018.04.036
United Nation Environmental Programme (UNEP) (2014). UNEP Year Book 2014: Emerging Issues Update. Nairobi, Kenya: United Nations Environment Programme.
Wang, B., Iino, F., Huang, J., Lu, Y., Yu, G., and Morita, M. (2010). Dechlorane plus pollution and inventory in soil of huai'an city, China. Chemosphere 80, 1285–1290. doi:10.1016/j.chemosphere.2010.06.052
Wang, G. G., Peng, J. L., Hao, T., Liu, Y., Zhang, D. H., and Li, X. G. (2016a). Distribution and region-specific sources of dechloraneplus in marine sediments from the coastal east China sea. Sci. Total Environ. 573, 389–396. doi:10.1016/j.scitotenv.2016.08.090
Wang, P., Zhang, Q., Zhang, H., Wang, T., Sun, H., Zheng, S., et al. (2016b). Sources and environmental behaviors of dechlorane plus and related compounds - a review. Environ. Int. 88, 206–220. doi:10.1016/j.envint.2015.12.026
Wright, S. L., Thompson, R. C., and Galloway, T. S. (2013). The physical impacts of microplastics on marine organisms: A review. Environ. Pollut. 178, 483–492. doi:10.1016/j.envpol.2013.02.031
Wu, B., Liu, S., Guo, X., Zhang, Y., Zhang, X., Li, M., et al. (2012). Responses of mouse liver to dechlorane plus exposure by integrative transcriptomic and metabonomic studies. Environ. Sci. Technol. 46, 10758–10764. doi:10.1021/es301804t
Wu, J., Bao, M., Ge, D., Huo, L., Lv, Z., Chi, C., et al. (2017). The expression of superoxide dismutase in mytilus coruscus under various stressors. Fish. Shellfish Immunol. 70, 361–371. doi:10.1016/j.fsi.2017.08.018
Zeng, Y. H., Luo, X. J., Tang, B., Zheng, X. B., and Mai, B. X. (2014). Gastrointestinal absorption, dynamic tissue-specific accumulation, and isomer composition of dechlorane plus and related analogs in common carp by dietary exposure. Ecotoxicol. Environ. Saf. 100, 32–38. doi:10.1016/j.ecoenv.2013.11.021
Zhang, Z. W., Tong, X., Xing, Y., Ma, J. Y., Jiang, R. J., Sun, Y. X., et al. (2020). Polybrominated diphenyl ethers, decabromodiphenyl ethane and dechlorane plus in aquatic products from the Yellow River Delta, China. Mar. Pollut. Bull. 161, 111733. doi:10.1016/j.marpolbul.2020.111733
Zhao, Z., Zhong, G., Moller, A., Xie, Z., Sturm, R., Ebinghaus, R., et al. (2011). Levels and distribution of dechlorane plus in coastal sediments of the yellow sea, north China. Chemosphere 83, 984–990. doi:10.1016/j.chemosphere.2011.02.011
Zhou, H., Tam, N. F. Y., Cheung, S. G., Wei, P., Li, S., and Wu, Q. (2019). Contamination of polybrominated diphenyl ethers (pbdes) in watershed sediments and plants adjacent to e-waste sites. J. Hazard Mater 379, 120788. doi:10.1016/j.jhazmat.2019.120788
Keywords: dechlorane plus, bioaccumulation, tissues, polystyrene, Mytilus coruscus
Citation: Xu Q, Peng X, Guo Y, Hao Q, Hou Y and Yang C (2023) Effects of polystyrene microplastics on dechlorane plus bioaccumulation in the thick-shell mussel. Front. Environ. Sci. 11:1163075. doi: 10.3389/fenvs.2023.1163075
Received: 10 February 2023; Accepted: 02 May 2023;
Published: 11 May 2023.
Edited by:
Hai Ocean Xu, Jiangsu University, ChinaReviewed by:
Fernando Manuel Raposo Morgado, University of Aveiro, PortugalZhang Chunfang, Zhejiang University, China
Rebecca Von Hellfeld, University of Aberdeen, United Kingdom
Copyright © 2023 Xu, Peng, Guo, Hao, Hou and Yang. This is an open-access article distributed under the terms of the Creative Commons Attribution License (CC BY). The use, distribution or reproduction in other forums is permitted, provided the original author(s) and the copyright owner(s) are credited and that the original publication in this journal is cited, in accordance with accepted academic practice. No use, distribution or reproduction is permitted which does not comply with these terms.
*Correspondence: Qing Hao, aG9vcTAwMUAxNjMuY29t