- 1Department of Geoinformatics, Cartography and Remote Sensing, Faculty of Geography and Regional Studies, University of Warsaw, Warsaw, Poland
- 2NORCE Norwegian Research Centre AS, Tromsø, Norway
- 3Department of Antarctic Biology, Institute of Biochemistry and Biophysics, Polish Academy of Sciences, Warsaw, Poland
- 4Department of Botany, Institute of Biology, Warsaw University of Life Sciences-SGGW, Warsaw, Poland
Polar areas are among the regions where climate change occurs faster than on most of the other areas on Earth. To study the effects of climate change on vegetation, there is a need for knowledge on its current status and properties. Both classic field observation methods and remote sensing methods based on manned aircraft or satellite image analysis have limitations. These include high logistic operation costs, limited research areas, high safety risks, direct human impact, and insufficient resolution of satellite images. Fixed-wing unmanned aerial vehicle beyond the visual line of sight (UAV BVLOS) missions can bridge the scale gap between field-based observations and full-scale airborne or satellite surveys. In this study the two operations of the UAV BVLOS, at an altitude of 350 m ASL, have been successfully performed in Antarctic conditions. Maps of the vegetation of the western shore of Admiralty Bay (King George Island, South Shetlands, Western Antarctic) that included the Antarctic Specially Protected Area No. 128 (ASPA 128) were designed. The vegetation in the 7.5 km2 area was mapped in ultra-high resolution (<5 cm and DEM of 0.25 m GSD), and from the Normalized Difference Vegetation Index (NDVI), four broad vegetation units were extracted: “dense moss carpets” (covering 0.14 km2, 0.8% of ASPA 128), “Sanionia uncinata moss bed” (0.31 km2, 1.7% of ASPA 128), “Deschampsia antarctica grass meadow” (0.24 km2, 1.3% of ASPA 128), and “Deschampsia antarctica–Usnea antarctica heath” (1.66 km2, 9.4% of ASPA 128). Our results demonstrate that the presented UAV BVLOS–based surveys are time-effective (single flight lasting 2.5 h on a distance of 300 km) and cost-effective when compared to classical field-based observations and are less invasive for the ecosystem. Moreover, unmanned airborne vehicles significantly improve security, which is of particular interest in polar region research. Therefore, their development is highly recommended for monitoring areas in remote and fragile environments.
1 Introduction
Maritime Antarctic is a region where recent dynamic climate changes are taking place (Siegert et al., 2019), and projections forecast a strong impact of future climate change on Antarctic biodiversity (Koerich et al., 2022). The development of vegetation in maritime Antarctica is limited to scatter ice-free areas located mainly in the coastal zone, which account for only a few percent (2%–5%) of the total area. The productivity and growth of the terrestrial tundra communities in the harsh Antarctic environment are controlled by a set of extreme environmental factors, like sub-zero temperatures, limited liquid water availability, a specific light regime, elevated ultraviolet-B radiation levels, desiccating and destructively strong winds, poorly developed soils with low organic matter and nutrient content, irregular nutrient distribution (from nutrient-deficient habitats to the ones extremely enriched in nutrients by, e.g., breeding colonies of seabirds), high salinity in many locations, and cryogenic processes (e.g., Convey, 1996; Znój et al., 2017; Łachacz et al., 2018). The presence of so many stress factors makes the Antarctic terrestrial ecosystems a mosaic of microhabitats colonized by highly heterogeneous and discontinuous communities (Smith, 1984; Block et al., 2009), currently threatened by biological invasions of alien species (Galera et al., 2018). Although so urgent, the detection and mapping of vegetation remain limited in the Antarctic environment. The mapping of the tundra communities in Antarctica has a short history, and usually small vegetation areas around research stations have been investigated (e.g., Lindsay, 1971; Smith, 1972). The classical field observation methods are laborious, generate a high cost of expeditions, high levels of human impact on the environment, and require specialized botanical knowledge. Also, vegetation mapping by image classification using remote sensing techniques remains limited in the Antarctic environment (Casanovas et al., 2015). Mainly due to the patchiness of terrestrial communities, its surface coverage can be sparse, with isolated individuals interspersed with bare ground and rocks, in small communities forming biocrusts on soil or rocks, or sometimes communities forming more extensive dense patches (Sotille et al., 2020). Obtaining satellite imagery material at the appropriate time of a vegetation stage and in sufficient qualities is still problematic, especially in the Antarctic Peninsula region, due to frequent occurrences of dense cloud cover and mist (Mustafa et al., 2012). Therefore, cost-effective and reliable wide-scale survey methods are required to accelerate assessments of Antarctic biodiversity (Wall et al., 2011; Casanovas et al., 2015).
A high spatial resolution is required to map most of the plant communities, and spectral bands in the short-wave infrared parts are crucial when distinguishing between bryophyte communities. This limits the use of the available satellite-based sensors. For example, Casanovas et al. (2015) used the Normalized Difference Vegetation Index (NDVI) and the matched filtering (MF) approach from Landsat data to study the vegetation distribution, however, they were unable to identify a pattern between the NDVI values and vegetation types. The NDVI is a standard tool in remote sensing monitoring of green biomass (Pettorelli et al., 2005). It takes values ranging from −1 to 1. The higher the value of this indicator, the higher the biomass content and better the condition of the vegetation (Rouse et al., 1974). The NDVI has gained popularity due to its ease of calculation based on two spectral ranges—a standardized range and high correlation with plant properties. The NDVI has found widespread use for assessing chlorophyll content, assessing plant stress, or identifying vegetation types (Geerken et al., 2005; Ozyavuz et al., 2015), and classifying land cover (Defries and Townshend, 1994) and detecting change for vegetation cover (Gandhi et al., 2015; Ju and Bohrer, 2022). It can be also used to estimate the density of green on an area of land (Weier and Herring, 2000).
Fretwell et al. (2011) used data covering Graham Land in the northern part of the Antarctic Peninsula and ground truthing in a test that found that 0.086% of the study area (74,468 km2) showed a probability of vegetation presence of over 50%. In this study, the authors could not determine whether low NDVI values referred to partial coverage of mosses or a continuous coverage of lichens or algae, or if the pixel contained a significant area of vegetation-free soil. They found out that olivine-rich sub-aerial palagonite tuffs (Middle to Upper Miocene, James Ross Island Volcanic Group) return NDVI ratios that are within the range of values given by sparse vegetation. Detailed information from these studies is not available due to the images’ limited spatial and spectral resolution and ground-truthing efforts. In such an environment, where terrestrial communities are dominated by species of low biomass and are characterized by irregular distribution, mid- or low-resolution images have limited use in the analysis of vegetation spatial coverage. Better results were obtained by Murray et al. (2010) and Andrade et al. (2018) who used, respectively, high-resolution IKONOS and QuickBird (four bands) imagery to map Antarctic vegetation, but they could not discriminate between lichens and bare ground and rocks. Whereas, Shin et al. (2014) successfully used linear unmixing of three endmember spectra (snow, rock/soil, and vegetation) extracted from QuickBird and KOMPSAT-2 imagery to map vegetation in Barton Peninsula. Furthermore, multispectral WorldView-2 satellite data having eight bands could capture most of the spectral characteristics of the cryptogam-dominating vegetation. These data have been applied by Power et al. (2020) to estimate microbial mat biomass based on the NDVI in Dry Valleys, Eastern Antarctic, and by Jawak et al. (2019) to map the vegetation of the Larsemann Hills and Schirmacher Oasis, by ensemble merging of the five top-performing methods (mixture-tuned matched filtering, matched filtering, matched filtering/Spectral Angle Mapper ratio, NDVI-2, and NDVI-4).
Sun et al. (2021) using WorldView-2 images and seven SMA models (FCLS, NM, FM, GBM, and three MNM-AVs) extracted vegetation data in the Fildes Peninsula, a part of the Nelson Peninsula (the King George Island) and Ardley Island. The newly proposed models achieved the best performance in abundance estimation of both mosses and lichens when compare to the previous study.
But, all space-borne methodological approaches share a common feature: the classifiers of vegetation communities perform well only when the spectral mixing in each image pixel is low (Calviño-Cancela and Martín-Herrero, 2016; Miranda et al., 2020). Therefore, these techniques do not work well with plant communities in polar regions where tundra patches are scattered and discontinuous and may provide imagery with undesired degrees of spectral mixing with other classes.
More reliable specific vegetation maps may be provided through the incorporation of the classification procedure of intermediate scales of observation between ground and satellite levels. Obtaining data by the traditional aerial surveys with airplanes or helicopters (Furmańczyk and Ochyra, 1982) and currently unmanned aerial vehicles (UAVs) (e.g., Lucieer et al., 2014; Miranda et al., 2020; Váczi et al., 2020) is the solution. Although, UAV data can cover a much smaller area than do satellite images, their high resolution permits distinguishing many details related to the vegetation that are not evident in satellite images. Moreover, using the far-range UAVs and beyond the visual line of sight method allows exploring sites located away from the operation center. Therefore, the use of small UAVs in the terrestrial regions of Antarctica is increasing steadily. The successful application of the UAV methodology was already implemented in a multitude of objectives (Zmarz et al., 2018), like wildlife inventorying and monitoring (e.g.,: Goebel et al., 2015; Mustafa et al., 2018; Korczak-Abshire et al., 2019), periglacial landform mapping (Dąbski et al., 2017; Pina et al., 2019), glacier forelands (Dąbski et al., 2020; Kreczmer et al., 2021), and monitoring sea ice surface features (Li et al., 2019), among others (Pina and Vieira, 2022). The UAVs based vegetation studies in Antarctica are extremely helpful in providing additional details for mapping procedures (Turner et al., 2014), obtaining the micro-topography of moss beds (Lucieer et al., 2014), assessing the stress (Malenovský et al., 2017) and health status (Turner et al., 2019) of plants, or monitoring the changes taking place in vegetation over time (Jawak et al., 2019; Bollard et al., 2022).
The entire of Antarctica is governed internationally by the decisions of the Antarctic Treaty countries and has the status of a natural reserve (www.ats.aq/e/ep.htm). To protect this unique ecosystem, the Antarctic Specially Protected Area system was established (http://www.ats.aq/documents/recatt/Att004_e.pdf). Unfortunately, there has been no proper long-term monitoring system for these areas (Convey and Peck, 2019). Any conservation actions in Antarctica would depend on robust and reliable baseline information, which is still sparse for most regions. Therefore, the development of an effective and efficient vegetation monitoring system is currently a challenge.
The main aim of this study is to develop a UAV BVLOS–based NDVI map of the Antarctic Specially Protected Area No. 128, located on the west coast of Admiralty Bay on King George Island. A second aim is to relate the NDVI values to broad vegetation units. These maps will become important baseline maps for future studies on changes in vegetation cover.
2 Materials and methods
2.1 Study area
The study area is located on King George Island (62°10′S, 58°28′W), the largest volcanic island in the South Shetlands archipelago, with a surface area of around 1,310 km2, of which more than 92% is covered by glaciers (Lim et al., 2014). The area covering the west coast of Admiralty Bay: the main body of Point Thomas Oasis with Henryk Arctowski Polish Antarctic Station (Arctowski) and the Antarctic Specially Protected Area No. 128 (ASPA 128) (Figure 1). The Point Thomas Oasis is one of the largest seasonally ice-free areas (approx. 25 km2) in the maritime Antarctic region with relatively high temperatures during the austral summer (Kejna et al., 2013; Galera et al., 2015) and a constant flow of freshwater (Nędzarek et al., 2014) throughout most of the summer season (Kejna et al., 2013; Sancho et al., 2017). The area is supplied with nutrients by large animal colonies, especially penguins and pinnipeds (Sierakowski et al., 2017), and is under the constant influence of sea aerosols (Łachacz et al., 2018). Apart from temperature (mean annual air temperature is −1.8°C with a minimum of −32.3°C and a maximum of 16.7°C; Kejna, 1999; Kejna et al., 2013) and humidity (average relative humidity is 86.2%, with a monthly average precipitation of 33.0 mm; Kejna, 1999), the driving force that shapes tundra communities is the constantly blowing strong wind (average annual wind speed equals 6.6°ms−1; Marsz and Styszyńska, 2000, with a maximum exceeding 65.0°ms−1; Zwoliński, 2007). Winds cause desiccation stress and act as an abrasion factor. All these conditions favor the development of one of the most diverse tundra communities in maritime Antarctic (Furmańczyk and Ochyra, 1982; Ferrari et al., 2021). The tundra in this region is mainly made up of cryptogams: lichens—ca. 380 species (Øvstedal and Smith, 2001; Olech, 2004), fungi—over 100 species (Malosso et al., 2006), bryophytes—ca. 140 species (Ochyra et al., 2008), and 22 species of liverworts (Bednarek-Ochyra et al., 2000) and algae (Broady, 1996). These terrestrial ecosystems are extremely deficient in Magnoliophyta, of which only two native flowering plant species are found, and these are limited to the coastal parts of the west Antarctic Peninsula and its associating islands and archipelagoes: the Antarctic hairgrass Deschampsia antarctica Desv. (Poaceae) and pearlwort Colobanthus quitensis (Kunth) Bartl. (Caryophyllaceae) (Chwedorzewska et al., 2004; Androsiuk et al., 2015; Koc et al., 2018). In the study area, some of the plant communities had been described by Zarzycki (1993), Victoria et al. (2006, 2009), and Pereira et al. (2010), showing the spatial distribution of selected vegetation communities at Demay Point, in the southern parts of ASPA 128.
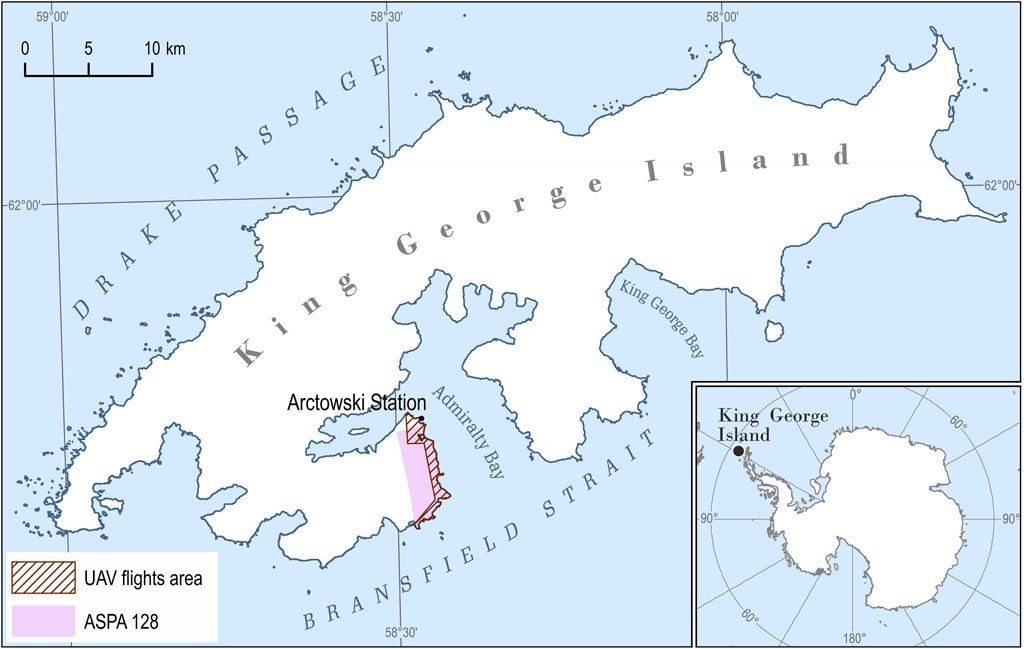
FIGURE 1. The study area located on King George Island, South Shetlands Archipelago, the Western Antarctic; UAV flights area (7.5 km2) marked in the hatch; and Antarctic Specially Protected Area No. 128 marked in purple [The coastline of the island on the basis of the SCAR King George Island geographic information system project (http://www.kgis.scar.org/)].
2.2 Data acquisition
BVLOS flights at an altitude of 350 m ASL were implemented by the PW-ZOOM fixed-wing UAV (Goetzendorf-Grabowski and Rodzewicz, 2016; Rodzewicz et al., 2017; Zmarz et al., 2018), which was equipped with an MP autopilot: MicroPilot (Stony Mountain, Canada). The operation was performed by a three-member team: remote control operator (RC), ground control station operator (GCS), and maintenance operator (all had BVLOS licenses issued by national authorities). Two separate flights were implemented to obtain the data that were required to calculate the NDVI: one with the R-G-B camera (Canon EOS 700D + 35 mm lens) and another with the B-G-NIR camera (blue channel, visible blue light—green channel, visible green light—NIR from 680 nm to 800 nm; Canon EOS REBEL T5i + 35 mm lens). The B-G-NIR camera uses blue as the absorption channel and NIR as the reflection channel. The two flights were undertaken on 10–11 November 2016 at an altitude of 350 m ASL and obtained data at a spatial resolution of <5 cm GSD and DEM of 0.25 m. The area for which the data was obtained amounted to 7.5 km2 (Figure 1). The time that each flight took was 2 h and 2.5 h, respectively. All images that were obtained had georeferences (X, Y, and Z) registered by the autopilot logger that was mounted on the UAV. The GPS Receiver GP-E2 was used for the geolocation of images allowing for horizontal accuracy of measurement <5 m. The data in the form of single images were processed into an orthophoto map in the Universal Transverse Mercator system, zone 21 S (EPSG: 32721). The two sensors were matched into a single four-bands file (R-G-B-NIR) using the layer stacking method in the ENVI software. Then, the normalized difference vegetation index (NDVI), which reflects the vegetation coverage and biomass, was calculated.
2.3 Dividing NDVI values to vegetation units
After processing the NDVI map, we aimed to divide the NDVI values into broad vegetation units. The vegetation was studied in January and February 2016, the austral summer before the acquisition of the NDVI image. The percent cover of each species and for impediment was estimated in altogether 99 1 × 1 m2 phytosociological relevés. The relevés were placed systematically every 10 m in the north–south direction along three transects (Figure 4). Then, to reveal the main vegetation units in the area, the relevés were clustered by Euclidean distances based on the species cover of each relevé and a dendrogram was produced (Figure 2). For the groups separated by the clustering, the number of relevés (n) was taken, and the percentage cover (c) and frequency (f) of the main species were described for each group. We also calculated the mean NDVI value for each relevé and thereby found the NDVI range for each group. This range was used in the main division of the NDVI values into vegetation units.
However, the transects with relevés did not cover a penguin colony and protected wetland, these areas have some differences in vegetation composition when compared with the areas that the transects cover, and validation data for these areas were obtained separately.
In the Point Thomas penguin colony area, east of the Arctowski Station (Rakusa Point), the cliffs and rocks close to the sea are enriched by guano and are dominated by epilithic lichen with orange thallus (mainly, Caloplaca regalis, C. sublobulata, and Xanthoria elegans). A total of 50 polygons of these lichens were identified on the RGB image and the NDVI values were calculated. In this penguin colony, the green algae Prasiola crispa locally dominates the ground. Also for these algae, 50 polygons were identified on the RGB image and the NDVI values were calculated.
Furthermore, just east of the Arctowski Station, there is a small (<0.08 km2) very vulnerable protected wetland (Jasnorzewski Gardens), with a dense vegetation cover in mainly standing waters (Figure 4). To avoid walking in this area, we instead georeferenced a previous vegetation map interpreted from an ordinary paper of a photo captured from a helicopter back in 1979 (Furmańczyk and Ochyra, 1982). Then, the NDVI values from our flight were extracted for the vegetation types and species composition of the communities in the old map.
3 Results
3.1 NDVI map
Altogether ASPA 128 covers 17.5 km2, and 9.2 km2 (53%) is covered by glaciers (Pudełko et al., 2018). The ice-free area of ASPA 128 is 8.2 km2 (47%) and most of this was mapped with the NDVI (Figure 3). Altogether 7.5 km2 was mapped with 4 × 4 cm2 pixel resolution that covered the entire coastline and most of the ice-free areas of the ASPA 128. The map shows that 2.35 km2 have NDVI values above 0.12, which we use as a threshold for vegetation cover (for details, please refer Section 3.2). Continuous areas with NDVI above 0.12 were mainly found in the southern parts at Demay Point and northern parts closer to the Arctowski.
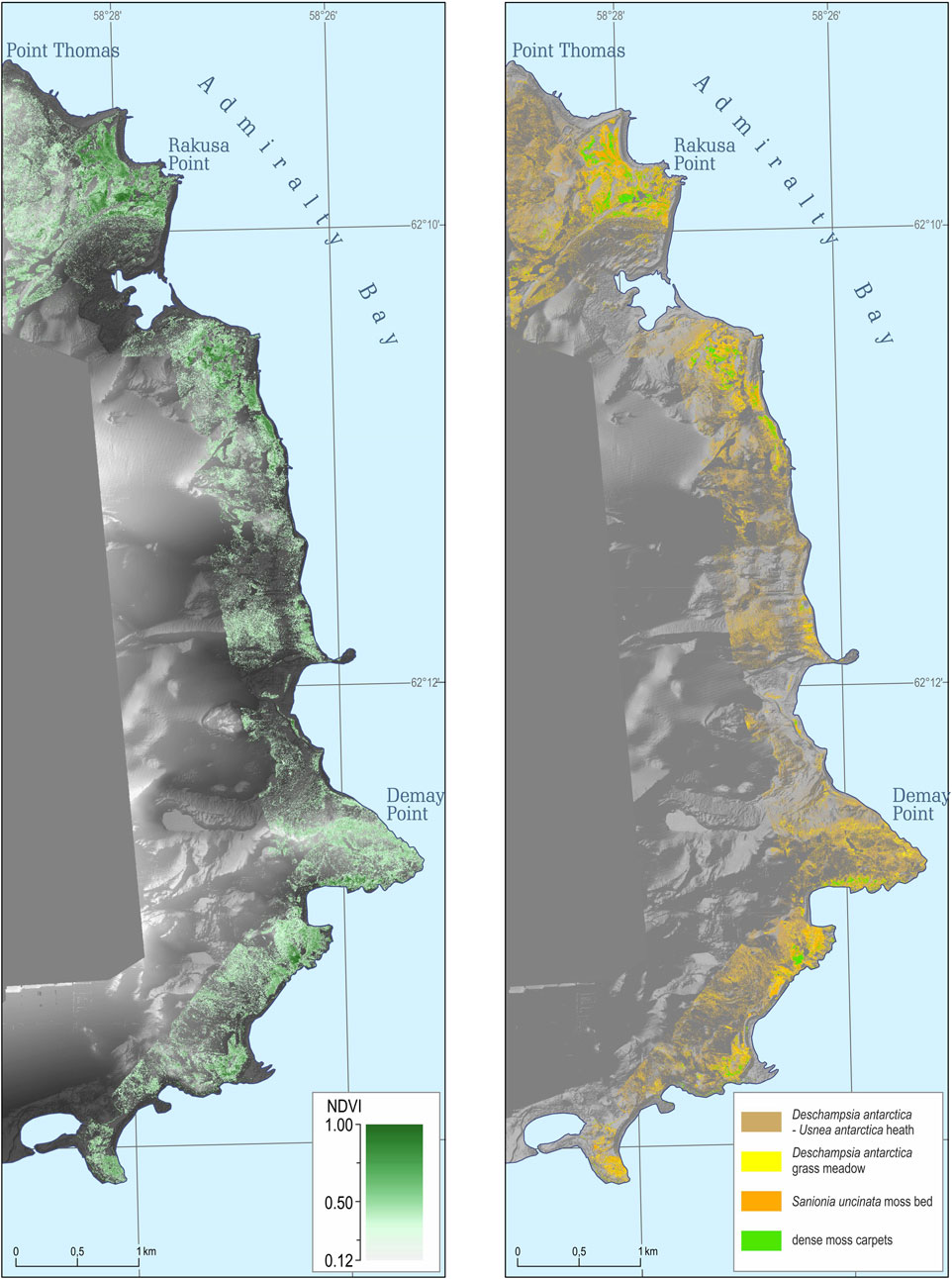
FIGURE 3. NDVI map (left) of the western shore of Admiralty Bay, King George Island, South Shetlands Archipelago, and ultra-high resolution vegetation map (right) covering an area of 7.5 km2 (Source data: DEM and orthophoto based on the original UAV-derived data).
3.2 Vegetation units
The clustering of the 99 relevés separated three main groups on Euclidian distance of 100 (Figure 4). In the left group (Deschampsia antarctica—Usnea antarctica heath) 48 of the 51 relevés had NDVI values in the range of 0.05–0.154. The remaining three relevés had NDVI values of 0.030, 0.234, and 0.286. However, relevés with <5% vegetation cover mainly had NDVI values below 0.120. For the ‘Sanionia uncinata moss bed’ group, the 18 relevés had NDVI values in the range of 0.235–0.384, but 14 of these were in the NDVI range of 0.252–0.370. For the ‘Deschampsia antarctica grass meadow’ group, 26 of the 30 relevés had NDVI values in the range of 0.163–0.298. The relevés did not cover the penguin colony. A total of 50 polygons from RGB images of the epilithic lichen with orange thallus show NDVI values mainly in the range of 0.25–0.30. In addition, 50 polygons of the green algae (Prasiola crispa) had NDVI values in the range of 0.17–0.30.
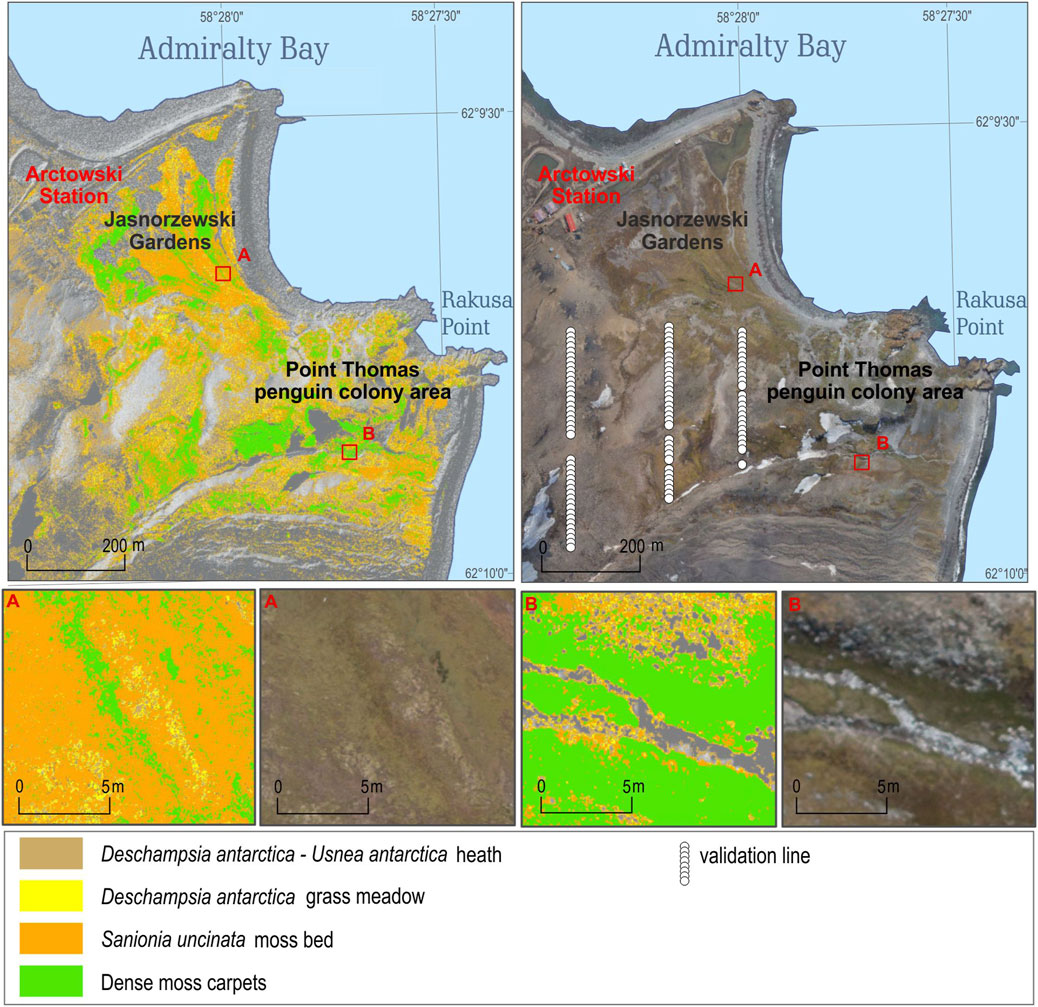
FIGURE 4. Jasnorzewski Gardens and Point Thomas penguin colony vegetation map based on UAV-derived images. The map also shows the location of the 99 relevés (white dots) which were placed systematically every 10 m along three transects.
We also analyzed the NDVI values of the plant communities described in the old vegetation map of the protected wetland (Furmańczyk and Ochyra, 1982), and most of these dense moss carpets that were found had high NDVI values of mainly above 0.37.
Based on the data set mentioned above, we divided the NDVI map into four broad vegetation units, where the exact threshold in NDVI values between the units was subjectively chosen due to some overlaps in relevés NDVI values. The ‘dense moss carpet’ unit had NDVI values >0.37. However, the NDVI threshold of 0.37 was subjectively chosen, and the group had some overlap with the related unit below. The ‘Sanionia uncinata moss bed’ unit occurs in the NDVI range of 0.27–0.37. This unit also has a gradual transition in NDVI values to the group below. The ‘Deschampsia antarctica grass meadow’ has NDVI values in the range of 0.22–0.26. The ‘Deschampsia antarctica–Usnea antarctica heath’ has NDVI values in the range of 0.12–0.22. The lower values were classified as those without vegetation.
3.2.1 Dense moss carpets
This unit displays different bryophyte plant communities, which make thick, dense moss carpets in moist to wet areas, partly protected from the wind. In more permanently wet (standing water) parts, hydrophilous bryophytes such as Warnstorfia sarmentosa and W. fontinaliopsis locally dominate. Bryum pseudotriquetrum is widespread in the study area, particularly along creeks and in concave parts. Some of the thickest carpets of Sanionia uncinate are also included in this class. This unit covered 0.14 km2 (0.8 % ASPA 128) and is mainly found at lower altitudes (<30 m. ASL), and 89% of the unit occurs in flat areas.
3.2.2 Sanionia uncinata moss bed
This unit is dominated by the widespread bryophyte Sanionia uncinata. From the Euclidean clustering, 18 relevés (n = 18) show that Sanionia uncinata occurs in every relevé (frequency, f = 100) used to describe this unit and has an average percentage cover (c) of 64%. This bryophyte often forms dense homogeneous carpets, but with a gradual transition to dominants of Deschampsia antarctica (f = 80, c = 7%). Also frequently found are the bryophytes Polytrichastrum alpinum (f = 50) and Polytrichum juniperinum (f = 28), but these cover less than 2% each. The relevés show that some of the moss were classified as dead (c = 14%). The unit is widespread in ASPA 128 and covers 0.31 km2 (1.7% of ASPA 128, and is often dominated in less wind-exposed areas.
3.2.3 Deschampsia antarctica grass meadow
Together with S. uncinata, D. antarctica is the most widespread species in the study area, and these are often associated with each other. The 30 relevés (n = 30) show a mean cover (c) of 30% of D. antarctica in this unit, in addition to dead moss and grass cover altogether covering 15%. The unit is rather homogeneous, but some other species are also found, such as the lichens Ochrolechia frigida (f = 63, c = 6%) and Usnea antarctica (f = 43, c = 6%), Antarctic pearlwort (Colobanthus quitensis) (f = 37, c = 2%), Sanionia uncinata (f = 47, c = 6%), the brownish bryophytes Polytrichastrum alpinum (f = 20), Polytrichum juniperinum (f = 23), and P. piliferum (f = 23), but the latter three cover less than 2% each. The unit dominates often in slightly convex, north-facing slopes. In addition, since D. antarctica tolerates high salinity, the unit occurs in the littoral zone, that is, areas directly influenced by marine aerosols and/or over-manured by birds’ feces. In the penguin colony area, some of the algae Prasiola crispa and epilithic lichen with orange thallus are also included in this unit. The unit covers 0.24 km2 (1.3% of ASPA 128).
3.2.4 Deschampsia antarctica–Usnea antarctica heath
This unit is characterized by high species diversity, sparse vegetation cover, and the visually dominated lichen Usnea antarctica. The unit has a discontinued vegetation cover, and gravel and open soil cover altogether of 85%. The unit has a high species diversity of lichens, but only U. antarctica (f = 35, c = 5%) and Ochrolechia frigida (f = 20, c = 1%) occur frequently. D. antarctica is the most frequent species (f = 58, c = 1%), but it covers far less than U. antarctica does. C. quitensis occurs frequently (f = 24) but has an insignificant cover (c = 0.2%). The unit also has a high diversity of bryophytes, which occurs in all of the 51 (n = 51) relevés, and as in the previous units, the Sanionia uncinata, Polytrichastrum alpinum, P. juniperinum, and P. piliferum are among the most frequent bryophytes. This unit is widespread at altitudes above the previously described units (mean height 58 m H ellipse), and mainly occurs in areas characterized by less snow cover which is often slightly convex, dry, and exposed. These areas are rarely influenced by pinnipeds and penguin disturbances. In addition, the unit includes a variety of plant communities at lower altitudes, all with only scattered vegetation cover, and some of the epilithic lichens with orange thallus in the penguin colony. This is the most commonly mapped unit and covers 1.66 km2 (9.4% of ASPA 128).
4 Discussion
In our work, we laid the foundations for BVLOS UAV–based monitoring vegetation on the western shore of Admiralty Bay of King George Island (South Shetlands). The vegetation in the 7.5 km2 area was mapped with ultra-high resolution and is to our knowledge among the largest UAV-based vegetation maps in maritime Antarctic.
The 99 relevés used in this study were originally meant for accuracy assessment for a vegetation map based on a UAV-mounted multispectral camera. However, during the 1 month from 23 January to 24 February 2016, not a single day had usable flight conditions (too strong wind), or there was too much fresh snow on the ground. This illustrates the challenges in vegetation mapping in maritime Antarctic. Instead, the relevés were used to interpret the later obtained NDVI map to identify some broad vegetation units, but then without the possibilities for accuracy assessment. However, three of the four mapped units were based on the clustering of relevés, and with the separation of the units at an Euclidean distance of 100, some very distinct units appear. Based on this division, the cover and frequency of the species in these three units were taken. Hence, it is reasonable to believe that in most cases, the four mapped units described in this study are reasonably accurate. However, with some exceptions, within the penguin colony, rocks are covered with ornithocoprophilous, epilithic lichen with orange thallus, and on the ground, the green algae Prasiola crispa often dominates. These unusual cases mainly occur within the mapped units ‘Deschampsia antarctica grass meadow’ and ‘Deschampsia antarctica–Usnea antarctica heath,’ respectively. This is due to the spectral similarities between P. crispa and D. antarctica in both the red and NIR bands (Calviño-Cancela and Martín-Herrero, 2016) and thereby similarities in the NDVI values, and the relatively high reflectance in the red band to the epilithic lichen with orange thallus Caloplaca sp. and Xanthoria sp. (Calviño-Cancela and Martín-Herrero, 2016). Furthermore, scattered occurrences of the lichen Usnea antarctica have been noted at higher altitudes that did not give any significant signal in the NDVI value and were not mapped, as the NDVI values were below 0.12. This suggests that the NDVI is not useful in mapping spots of brightly colored lichens like Usnea antarctica, probably due to the high reflectance in both the visible and NIR bands of such lichens (Calviño-Cancela and Martín-Herrero, 2016; da Rosa et al., 2022).
Moreover, since we used an ordinary GPS for determining the location of the relevés, there is an accuracy issue, and by adding the accuracy error in the NDVI map, the NDVI values extracted from the 1 × 1 m2 relevés would not fit perfectly with the NDVI map. However, due to the gradual changes in vegetation/NDVI in the rather flat landscape, this inaccuracy does not influence the main results when dividing the NDVI values into vegetation units. The extracted NDVI values from the 6 × 6 m2 plots correlate significantly with the NDVI values from the 1 × 1 m2 relevés (r2 = 0.95, n = 99, and p = 5.34–65), indicating that the inaccuracy in location/NDVI map does not influence the results. Since no other maps of the vegetation in ASPA 128 exist, except for the very small and old vegetation map of the protected wetland (Furmańczyk and Ochyra, 1982) and a coarse vegetation map of Demay Point (Pereira et al., 2010), this is a huge step forward. The presented NDVI map and vegetation map show the current status of the vegetation and will be highly useful in the study of the ongoing environmental change, particularly the changes in vegetation cover, and for the conservation of the ASPA 128. The presented map could also be used as validation, giving a potential baseline for satellite-based vegetation mapping (Miranda et al., 2020), monitoring, and assessment of even larger areas. Future UAV-based vegetation mapping of the area has to use cameras with more spectral bands in order to better separate plant communities at a more detailed level when compared with this level of mapping. Alternatively, a recent geographic object-based image analysis of UAV data in a comparable area in maritime Antarctic has shown promising results (Sotille et al., 2022), although they have not separated the different bryophyte species, which dominate in our study area.
Under the strongest forcing scenario, projections predict not only a tripling (300% increase) of ice-free areas over parts of the Antarctic Peninsula as a result of more than 50 additional-degree days above freezing by the end of the 21st century (Lee et al., 2017) but also the increases in precipitation (Turner et al., 2019), permafrost warming, and active layer thickening (Guglielmin et al., 2014; Hrbăcek et al., 2021). This means that groundwater will remain available in the upper parts of the soil throughout the season. In maritime Antarctic, the development of terrestrial communities is controlled by extreme environmental conditions, with the crucial role of liquid water availability, rather than biotic interactions (Convey & Peck, 2019). Such communities are expected to be very sensitive to changes in climate and consequential processes (Bargagli, 2005; Frenot et al., 2005). Since more cumulative energy is available to terrestrial biota (both in terms of the absolute positive temperature achieved and cumulative degree days) the consequences are thought to include increased diversity (also by migrating some species from temperate zones to maritime Antarctic), biomass, trophic complexity, and rapid colonization of newly deglaciated terrains, all of which enable the development of a more complex ecosystem (Siegert et al., 2019; Weisleitner et al., 2020). Thus, most likely, further complications will arise from the complexity of those species interactions (Molina-Montenegro et al., 2019). While the contemporary Antarctic biota shows the ability to survive abiotic environmental extremes, its competitive abilities are very poorly developed, and even whole communities are vulnerable to increased competition from opportunistic invaders (Galera et al., 2019; Colesie et al., 2022). These observed changes are an area of concern for the Antarctic Treaty System, whose decisions to protect the Antarctic ecosystem are based on the best available reliable scientific data. As described here, the UAV BVLOS operations proved to be very robust in gathering valuable qualitative and quantitative data that are necessary for monitoring distant and isolated polar environments. It is important to notice the differences between the multi-rotor and fixed-wing platforms, and between the visual line of sight and beyond visual line of sight operations. Multi-rotor and fixed-wing platforms are completely different solutions with extremely different performances, but also with different functional and operational requirements. That is why, it is so important to precisely define tasks in order to choose the optimal system. The factors which significantly affect the efficiency of UAV operations are flight time, flight speed, type of drive (combustion or electric engine), possible distance to be covered by one flight, telemetric range, and payload. Unmanned multi-rotor platforms are mainly used for small, even very small areas located close to the take-off/landing point and in favorable calm weather conditions. For tasks requiring longer flight time and range, fixed-wing platforms turn out to be much more effective especially in the BVLOS operation, as was proved here, as in previous studies (see Zmarz et al., 2018; Korczak-Abshire et al., 2019; Dąbski et al., 2020). Data described here can be applied in the conservation of the protected areas and further studies of environmental changes.
5 Conclusion
The BVLOS operation of the fixed-wing PW-ZOOM UAV, equipped with an R-G-B camera and a B-G-NIR camera allowed obtaining data for the development of a vegetation map in the Antarctic Specially Protected Area No. 128. The flights were made for an area of 7.5 km2 in a relatively short time (one flight lasted for 2 h and the second flight for 2.5 h). Using BVLOS operations in Antarctic conditions proved to be an effective method of obtaining data in hard-to-reach areas. Advantages include short data acquisition time, long range of BVLOS flights, and few crew members involved in servicing the BVLOS flights. Furthermore, the proposed method of acquiring high-resolution data fills the gap between satellite data and data obtained by traditional field-based methods.
Data availability statement
The data: reléve coordinates, NDVI reléve values, NDVI map as pdf, supporting the conclusion of this article will be made available by the authors, without undue reservation.
Author contributions
AZ and SK conceived the ideas and designed the methodology; AZ, SK, MK-A, and KC collected the data; AZ, SK, MK, MK-A, IG, IK, and KC analyzed the data; AZ, SK, MK, MK-A, and KC led the writing of the manuscript. All authors contributed critically to the drafts and gave final approval for publication.
Funding
The research was conducted as part of the project “A novel approach to monitoring the impact of climate change on Antarctic ecosystems (MONICA)” funded by the Polish–Norwegian Research Programme operated by the National Centre for Research and Development under the Norwegian Financial Mechanism 2009–2014 in the frame of Project Contract No. 197810. The data used in the study were collected at the Henryk Arctowski Polish Antarctic Station.
Conflict of interest
The authors declare that the research was conducted in the absence of any commercial or financial relationships that could be construed as a potential conflict of interest.
Publisher’s note
All claims expressed in this article are solely those of the authors and do not necessarily represent those of their affiliated organizations, or those of the publisher, editors, and reviewers. Any product that may be evaluated in this article, or claim that may be made by its manufacturer, is not guaranteed or endorsed by the publisher.
References
Andrade, A. M. De, Michel, R. F. M., Bremer, U. F., Schaefer, C. E. G. R., and Simões, J. C. (2018). Relationship between solar radiation and surface distribution of vegetation in Fildes Peninsula and Ardley Island, maritime Antarctica. Int. J. Remote Sens. 39 (8), 2238–2254. doi:10.1080/01431161.2017.1420937
Androsiuk, P., Chwedorzewska, K., Szandar, K., and Giełwanowska, I. (2015). Genetic variability of Colobanthus quitensis from King George Island (Antarctica). Pol. Polar Res. 36 (3), 281–295. doi:10.1515/popore-2015-0017
Bargagli, R. (2005). Antarctic ecosystems, environmental contamination, climate change, and human impact. Ecological studies, 175 M. M. Caldwell, G. Heldmaier, R. B. Jackson, O. L. Lange, H. A. Mooney, E. D. Schulzeet al. (Heidelberg, Germany: U., Friedmut Kröner), 393.
Bednarek-Ochyra, H., Váňa, J., Ochyra, R., and Lewis Smith, R. I. (2000). The liverwort flora of Antarctica. Cracow: Polish Academy of Sciences, Institute of Botany, 236.
Block, W., Lewis Smith, R. I., and Kennedy, A. D. (2009). Strategies of survival and resource exploitation in the Antarctic fellfield ecosystem. Biol. Rev. 84 (3), 449–484. doi:10.1111/j.1469-185X.2009.00084.x
Bollard, B., Doshi, A., Gilbert, N., Poirot, C., and Gillman, L. (2022). Drone technology for monitoring protected areas in remote and fragile environments. Drones 6 (2), 42. doi:10.3390/drones6020042
Broady, P. A. (1996). Diversity, distribution and dispersal of Antarctic terrestrial algae. Biodivers. Conservation 5 (11), 1307–1335. doi:10.1007/BF00051981
Calviño-Cancela, M., and Martín-Herrero, J. (2016). Spectral discrimination of vegetation classes in ice-free areas of Antarctica. Remote Sens. 8 (10), 856–915. doi:10.3390/rs8100856
Casanovas, P., Black, M., Fretwell, P., and Convey, P. (2015). Mapping lichen distribution on the Antarctic Peninsula using remote sensing, lichen spectra and photographic documentation by citizen scientists. Polar Res. 34 (1), 25633. doi:10.3402/polar.v34.25633
Chwedorzewska, K. J., Bednarek, P. T., and Puchalski, J. (2004). Molecular variation of antarctic grass Deschampsia Antarctica Desv. From King George Island (Antarctica). Acta Soc. Bot. Pol. 73 (1), 23–29. doi:10.5586/asbp.2004.004
Colesie, C., Walshaw, C. V., Sancho, L. G., Davey, M. P., and Gray, A. (2022). Antarctica's vegetation in a changing climate. WIREs Clim. Change 14, e810. doi:10.1002/wcc.810
Convey, P., and Peck, L. S. (2019). Antarctic environmental change and biological responses. Sci. Adv. 5 (11), eaaz0888. doi:10.1126/sciadv.aaz0888
Convey, P. (1996). The influence of environmental characteristics on life history attributes of Antarctic terrestrial biota. Biol. Rev. 71, 191–225. doi:10.1111/j.1469-185x.1996.tb00747.x
da Rosa, C. N., Pereira Filho, W., Bremer, U. F., Putzke, J., de Andrade, A. M., Kramer, G., et al. (2022). Spectral behavior of vegetation in Harmony Point, Nelson Island, Antarctica. Biodivers. Conservation 31, 1867–1885. doi:10.1007/s10531-022-02408-7
Dąbski, M., Zmarz, A., Pabjanek, P., Korczak-Abshire, M., Karsznia, I., and Chwedorzewska, K. J. (2017). UAV-Based detection and spatial analyses of periglacial landforms on Demay Point (King George Island, South Shetland Islands, Antarctica). Geomorphology 290, 29–38. doi:10.1016/j.geomorph.2017.03.033
Dąbski, M., Zmarz, A., Rodzewicz, M., Korczak-Abshire, M., Karsznia, I., Lach, K., et al. (2020). Mapping glacier forelands based on UAV BVLOS operation in Antarctica. Remote Sens. 12 (4), 630. doi:10.3390/rs12040630
Defries, R. S., and Townshend, J. R. (1994). NDVI-derived land cover classifications at a global scale. Int. J. Remote Sens. 15 (17), 3567–3586. doi:10.1080/01431169408954345
Ferrari, F. R., Schaefer, C. E. G. R., Pereira, A. B., Thomazini, A., Schmitz, D., and Francelino, M. R. (2021). Coupled soil-vegetation changes along a topographic gradient on King George Island, maritime Antarctica. Catena 198, 105038. doi:10.1016/j.catena.2020.105038
Frenot, Y., Chown, S. L., Whinam, J., Selkirk, P. M., Convey, P., Skotnicki, M., et al. (2005). Biological invasions in the Antarctic: Extent, impacts and implications. Biol. Rev. 80, 45–72. doi:10.1017/s1464793104006542
Fretwell, P. T., Convey, P., Fleming, A. H., Peat, H. J., and Hughes, K. A. (2011). Detecting and mapping vegetation distribution on the Antarctic Peninsula from remote sensing data. Polar Biol. 34 (2), 273–281. doi:10.1007/s00300-010-0880-2
Furmańczyk, K., and Ochyra, R. (1982). Plant communities of the Admiralty Bay region (King George Island, South Shetland Islands, Antarctic) I. Jasnorzewski Gardens. Pol. Polar Res. 3 (1–2), 25–39.
Galera, H., Chwedorzewska, K. J., Korczak-Abshire, M., and Wódkiewicz, M. (2018). What affects the probability of biological invasions in Antarctica? Using an expanded conceptual framework to anticipate the risk of alien species expansion. Biodivers. Conservation 27, 1789–1809. doi:10.1007/s10531-018-1547-5
Galera, H., Chwedorzewska, K. J., and Wódkiewicz, M. (2015). Response of Poa annua to extreme conditions: Comparison of morphological traits between populations from cold and temperate climate conditions. Polar Biol. 38 (10), 1657–1666. doi:10.1007/s00300-015-1731-y
Galera, H., Rudak, A., Czyż, E. A., Chwedorzewska, K. J., Znój, A., and Wódkiewicz, M. (2019). The role of the soil seed store in the survival of an invasive population of Poa annua at Point Thomas Oasis, King George Island, maritime Antarctica. Glob. Ecol. Conservation 19, e00679. doi:10.1016/j.gecco.2019.e00679
Gandhi, G. M., Parthiban, S., Thummalu, N., and Christy, A. (2015). NDVI: Vegetation change detection using remote sensing and GIS — a case study of vellore district. Procedia Comput. Sci. 57, 1199–1210. doi:10.1016/j.procs.2015.07.415
Geerken, R., Zaitchik, B., and Evans, J. P. (2005). Classifying rangeland vegetation type and coverage from NDVI time series using Fourier Filtered Cycle Similarity. Int. J. Remote Sens. 26 (24), 5535–5554. doi:10.1080/01431160500300297
Goebel, M. E., Perryman, W. L., Hinke, J. T., Krause, D. J., Hann, N. A., Gardner, S., et al. (2015). A small unmanned aerial system for estimating abundance and size of Antarctic predators. Polar Biol. 38 (5), 619–630. doi:10.1007/s00300-014-1625-4
Goetzendorf-Grabowski, T., and Rodzewicz, M. (2016). Design of UAV for photogrammetric mission in Antarctic area. Proc. Institution Mech. Eng. Part G J. Aerosp. Eng. 231 (9), 1660–1675. doi:10.1177/0954410016656881
Guglielmin, M., Dalle Fratte, M., and Cannone, N. (2014). Permafrost warming and vegetation changes in continental Antarctica. Environ. Res. Lett. 9 (4), 045001. doi:10.1088/1748-9326/9/4/045001
Hrbăcek, F., Vieira, G., Oliva, M., Balks, M., Guglielmin, M., Pablo, M. A., et al. (2021). Active layer monitoring in Antarctica: An overview of results from 2006 to 2015. Polar Geogr. 44 (3), 217–231. doi:10.1080/1088937X.2017.1420105
Jawak, S. D., Luis, A. J., Fretwell, P. T., Convey, P., and Durairajan, U. A. (2019). Semiautomated detection and mapping of vegetation distribution in the Antarctic environment using spatial-spectral characteristics of WorldView-2 imagery. Remote Sens. 11 (16), 1909. doi:10.3390/rs11161909
Ju, Y., and Bohrer, G. (2022). Classification of wetland vegetation based on NDVI time series from the HLS dataset. Remote Sens. 14 (9), 2107. doi:10.3390/rs14092107
Kejna, M. (1999). Air temperature on King George Island, South Shetland Islands, Antarctica. Pol. Polar Res. 20 (3), 183–201.
Kejna, M., Araźny, A., and Sobota, I. (2013). Climatic change on King George Island in the years 1948 – 2011. Pol. Polar Res. 34 (2), 213–235. doi:10.2478/popore-2013-0004
Koc, J., Androsiuk, P., Chwedorzewska, K. J., Cuba-Díaz, M., Górecki, R., and Giełwanowska, I. (2018). Range-wide pattern of genetic variation in Colobanthus quitensis. Polar Biol. 41 (12), 2467–2479. doi:10.1007/s00300-018-2383-5
Koerich, G., Fraser, C. I., Lee, C. K., Morgan, F. J., and Tonkin, J. D. (2022). Forecasting the future of life in Antarctica. Trends Ecol. Evol. 38, 24–34. doi:10.1016/j.tree.2022.07.009
Korczak-Abshire, M., Zmarz, A., Rodzewicz, M., Kycko, M., Karsznia, I., and Chwedorzewska, K. J. (2019). Study of fauna population changes on Penguin Island and Turret Point Oasis (King George Island, Antarctica) using an unmanned aerial vehicle. Polar Biol. 42 (1), 217–224. doi:10.1007/s00300-018-2379-1
Kreczmer, K., Dąbski, M., and Zmarz, A. (2021). Terrestrial signature of a recently-tidewater glacier and adjacent periglaciation, Windy Glacier (South Shetland Islands, Antarctic). Front. Earth Sci. 9, 671985. doi:10.3389/feart.2021.671985
Łachacz, A., Kalisz, B., Giełwanowska, I., Olech, M., Chwedorzewska, K. J., and Kellmann-Sopyła, W. (2018). Nutrient abundance and variability from soils in the coast of King George Island. J. Soil Sci. Plant Nutr. 18 (2), 294–311. doi:10.4067/S0718-95162018005001101
Lee, J. R., Raymond, B., Bracegirdle, T. J., Chades, I., Fuller, R. A., Shaw, J. D., et al. (2017). Climate change drives expansion of Antarctic ice-free habitat. Nature 547 (7661), 49–54. doi:10.1038/nature22996
Li, T., Zhang, B., Cheng, X., Westoby, M. J., Li, Z., Ma, C., et al. (2019). Resolving fine-scale surface features on polar sea ice: A first assessment of uas photogrammetry without ground control. Remote Sens. 11 (7), 784. doi:10.3390/rs11070784
Lim, H. S., Park, Y., Lee, J. Y., and Yoon, H. Il. (2014). Geochemical characteristics of meltwater and pondwater on Barton and Weaver Peninsulas of King George Island, west Antarctica. Geochem. J. 48 (4), 409–422. doi:10.2343/geochemj.2.0316
Lindsay, D. C. (1971). Vegetation of the South Shetland Islands. Br. Antarct. Surv. Bull. 25, 59–83.
Lucieer, A., Turner, D., King, D. H., and Robinson, S. A. (2014). Using an unmanned aerial vehicle (UAV) to capture micro-topography of Antarctic moss beds. Int. J. Appl. Earth Observation Geoinformation 27, 53–62. doi:10.1016/j.jag.2013.05.011
Malenovský, Z., Robinson, S. A., Lucieer, A., King, D. H., Turnbull, J. D., and Robinson, S. A. (2017). Unmanned aircraft system advances health mapping of fragile polar vegetation. Methods Ecol. Evol. 8, 1842–1857. doi:10.1111/2041-210X.12833
Malosso, E., Waite, I. S., English, L., Hopkins, D. W., and O’Donnell, A. G. (2006). Fungal diversity in maritime Antarctic soils determined using a combination of culture isolation, molecular fingerprinting and cloning techniques. Polar Biol. 29 (7), 552–561. doi:10.1007/s00300-005-0088-z
Marsz, A. A., and Styszyńska, A. (2000). Główne cechy klimatu rejonu Polskiej Stacji Antarktycznej im. H. Arctowskiego (Antarktyka Zachodnia, Szetlandy Południowe, Wyspa Króla Jerzego. [Main climate characteristics of the region of the Polish Antarctic Station H. Arctowski (West Antarctica, South Shetland Islands, King George Island) - in Polish], WSM, Gdynia, pp. 264.
Miranda, V., Pina, P., Heleno, S., Vieira, G., Mora, C., and Schaefer, E. G. R. C. (2020). Monitoring recent changes of vegetation in Fildes Peninsula (King George Island, Antarctica) through satellite imagery guided by UAV surveys. Sci. Total Environ. 704, 135295. doi:10.1016/j.scitotenv.2019.135295
Molina-Montenegro, M. A., Bergstrom, D. M., Chwedorzewska, K. J., Convey, P., and Chown, S. L. (2019). Increasing impacts by Antarctica's most widespread invasive plant species as result of direct competition with native vascular plants. Neobiota 51, 19–40. doi:10.3897/neobiota.51.37250
Murray, H., Lucieer, A., and Williams, R. (2010). Texture-based classification of sub-Antarctic vegetation communities on Heard Island. Int. J. Appl. Earth Observation Geoinformation 12 (3), 138–149. doi:10.1016/j.jag.2010.01.006
Mustafa, O., Barbosa, A., Krause, D. J., Peter, H. U., Vieira, G., and Rümmler, M. C. (2018). State of knowledge: Antarctic wildlife response to unmanned aerial systems. Polar Biol. 41 (11), 2387–2398. doi:10.1007/s00300-018-2363-9
Mustafa, O., Pfeifer, C., Peter, H.-U., Kopp, M., and Metzig, R. (2012). Pilot study on monitoring climate-induced changes in penguin colonies in the Antarctic using satellite images. On behalf of the German Environment Agency. Texte 19/2012. Dessau-Roßlau. Available at: http://www.umweltbundesamt.de/publikationen/pilot-study-on-monitoring-climate-induced
Nędzarek, A., Tórz, A., and Drost, A. (2014). Selected elements in surface waters of Antarctica and their relations with the natural environment. Polar Res. 33, 21417. doi:10.3402/polar.v33.21417
Ochyra, R., Lewis Smith, R. I., and Bednarek-Ochyra, H. (2008). The illustrated moss flora of Antarctica. Cambridge: Cambridge University Press, 704. doi:10.1093/aob/mcp111
Olech, M. (2004). Lichens of King George Island, Antarctica. Kraków: Institute of Botany of The Jagiellonian University, 391.
Øvstedal, D. O., and Lewis Smith, R. I. (2001). Lichens of Antarctica and South Georgia. A guide to their identification and ecology. Cambridge: Cambridge University Press, 424. doi:10.1177/1748895811401979
Ozyavuz, M., Bilgili, B. C., and Salici, A. (2015). Determination of vegetation changes with NDVI method. J. Environ. Prot. Ecol. 16 (1), 264–273.
Pereira, A. B., Francelino, M. R., Stefenon, V. M., Schünemann, A. L., and Roesch, L. F. W. (2010). Plant communities from ice-free areas of Demay Point, King George Island, Antarctica. INCT-APA Annu. Act. Rep. 2, 58–62. doi:10.4322/apa.2014.024
Pettorelli, N., Vik, J. O., Mysterud, A., Gaillard, J.-M., Tucker, C. J., and Stenseth, N. Chr. (2005). Using the satellite-derived NDVI to assess ecological responses to environmental change. Trends Ecol. Evol. 20 (9), 503–510. doi:10.1016/j.tree.2005.05.011
Pina, P., Pereira, F., Marques, J. S., and Heleno, S. (2019). “Detection of stone circles in periglacial regions of Antarctica in UAV datasets,” in Pattern recognition and image analysis, lecture notes in computer science A. Morales, J. Fierrez, J. S. Sánchez, and B. Ribeiro (Germany: Springer Nature Switzerland AG), 279–288. doi:10.1016/0165-1684(94)90196-1
Pina, P., and Vieira, G. (2022). UAVs for science in Antarctica. Remote Sens. 14 (7), 1610–1639. doi:10.3390/rs14071610
Power, S. N., Salvatore, M. R., Sokol, E. R., Stanish, L. F., and Barrett, J. E. (2020). Estimating microbial mat biomass in the McMurdo Dry Valleys, Antarctica using satellite imagery and ground surveys. Polar Biol. 43 (11), 1753–1767. doi:10.1007/s00300-020-02742-y
Pudełko, R., Angiel, P. J., Potocki, M., Jędrejek, A., and Kozak, M. (2018). Fluctuation of glacial retreat rates in the eastern part of Warszawa Icefield, King George Island, Antarctica, 1979-2018. Remote Sens. 10, 892. doi:10.3390/rs10060892
Rodzewicz, M., Głowacki, D., and Hajduk, J. (2017). Some dynamic aspects of photogrammetry missions performed by “PW-ZOOM”-the UAV of Warsaw University of Technology. Archive Mech. Eng. 64 (1), 37–55. doi:10.1515/meceng-2017-0003
Rouse, J. W., Haas, R. H., Schell, J. A., and Deering, D. W. (1974). “Monitoring vegetation systems in the great plains with ERTS,” in Third earth resources technology satellite–1 syposium. Volume I: Technical presentations, NASA SP-351 S. C. Freden, E. P. Mercanti, and M. Becker (Washington, D.C.: NASA), 309–317.
Sancho, L. G., Pintado, A., Navarro, F., Ramos, M., De Pablo, M. A., Blanquer, J. M., et al. (2017). Recent warming and cooling in the Antarctic Peninsula region has rapid and large effects on lichen vegetation. Sci. Rep. 7, 5689. doi:10.1038/s41598-017-05989-4
Shin, J. Il, Kim, H. C., Kim, S. Il, and Hong, S. G. (2014). Vegetation abundance on the Barton Peninsula, Antarctica: Estimation from high-resolution satellite images. Polar Biol. 37 (11), 1579–1588. doi:10.1007/s00300-014-1543-5
Siegert, M., Atkinson, A., Banwell, A., Brandon, M., Convey, P., Davies, B., et al. (2019). The Antarctic Peninsula under a 1.5C global warming scenario. Front. Environ. Sci. 1 (102), 1–7. doi:10.3389/fenvs.2019.00102
Sierakowski, K., Korczak-Abshire, M., and Jadwiszczak, P. (2017). Changes in bird communities of Admiralty Bay, King George Island (West Antarctic): Insights from monitoring data (1977-1996). Pol. Polar Res. 38 (2), 231–262. doi:10.1515/popore-2017-0010
Smith, R. I. L. (1984). “Terrestrial biology of the Antarctic and sub-Antarctic,” in Antarctic ecology R. M. Laws (London: Academic Press), 61–162.
Smith, R. I. L. (1972). Vegetation of the South Orkney Islands with particular reference to Signy Island Scientific Report of the British Antarctic Survey, 68. London: British Antarctic Survey Scientific Reports.
Sotille, M. E., Bremer, U. F., Vieira, G., Velho, L. F., Petsch, C., Auger, J. D., et al. (2022). UAV-based classification of maritime Antarctic vegetation types using GEOBIA and random forest. Ecol. Inf. 71, 101768. doi:10.1016/j.ecoinf.2022.101768
Sotille, M. E., Bremer, U. F., Vieira, G., Velho, L. F., Petsch, C., and Simões, J. C. (2020). Evaluation of UAV and satellite-derived NDVI to map maritime Antarctic vegetation. Appl. Geogr. 125, 102322. doi:10.1016/j.apgeog.2020.102322
Sun, X., Wu, W., Li, X., Xu, X., and Li, J. (2021). Vegetation abundance and health mapping over southwestern Antarctica based on WorldView-2 data and a Modified Spectral Mixture Analysis. Remote Sens. 13 (2), 166. doi:10.3390/rs13020166
Turner, D. J., Malenovsky, Z., Lucieer, A., Turnbull, J. D., and Robinson, S. A. (2019). Optimizing spectral and spatial resolutions of Unmanned Aerial System imaging sensors for monitoring Antarctic vegetation. IEEE J. Sel. Top. Appl. Earth Observations Remote Sens. 12 (10), 3813–3825. doi:10.1109/JSTARS.2019.2938544
Turner, D., Lucieer, A., Malenovský, Z., King, D. H., and Robinson, S. A. (2014). Spatial co-registration of ultra-high resolution visible, multispectral and thermal images acquired with a micro-UAV over Antarctic moss beds. Remote Sens. 6 (5), 4003–4024. doi:10.3390/rs6054003
Váczi, P., Barták, M., Bednaříková, M., Hrbáček, F., and Hájek, J. (2020). Spectral properties of Antarctic and Alpine vegetation monitored by multispectral camera: Case studies from James Ross Island and Jeseníky Mts. Czech Polar Rep. 10 (2), 297–312. doi:10.5817/CPR2020-2-22
Victoria, F. C., Albuquerque, M. P., and Pereira, A. B. (2006). Lichen-moss associations in plant communities of the southwest Admiralty Bay, King George Island, Antarctica. Neotropical Biol. Conservation 1 (2), 84–89.
Victoria, F. C., Pereira, A. B., and Pinheiro-da-Costa, D. (2009). Composition and distribution of moss formations in the ice-free areas adjoining the Arctowski region, Admiralty Bay, King George Island, Antarctica. Iheringia, Série Botânica 64 (1), 81–91.
Wall, D. H., Lyons, B. W., Chown, S. L., Convey, P., Howard-Williams, C., Quesada, A., et al. (2011). Long-term ecosystem networks to record change: An international imperative. Antarct. Sci. 23 (3), 209. doi:10.1017/S0954102011000319
Weier, J., and Herring, D. (2000). Measuring vegetation (NDVI and EVI). Washington DC: NASA Earth Observatory.
Weisleitner, K., Perras, A. K., Unterberger, S. H., Moissl-Eichinger, C., Andersen, D. T., and Sattler, B. (2020). Cryoconite hole location in East Antarctic Untersee oasis shapes physical and biological diversity. Front. Microbiol. 11, 1165. doi:10.3389/fmicb.2020.01165
Zarzycki, K. (1993). “Vascular plants and terrestrial biotopes,” in The maritime antarctic coastal ecosystem of Admiralty Bay S. Rakusa-Suszczewski (Warsaw: Department of Antarctic Biology, Polish Academy of Sciences), 181–187.
Zmarz, A., Rodzewicz, M., Dąbski, M., Karsznia, I., Korczak-Abshire, M., and Chwedorzewska, K. J. (2018). Application of UAV BVLOS remote sensing data for multi-faceted analysis of Antarctic ecosystem. Remote Sens. Environ. 217, 375–388. doi:10.1016/j.rse.2018.08.031
Znój, A., Chwedorzewska, K. J., Androsiuk, P., Cuba-Diaz, M., Giełwanowska, I., Koc, J., et al. (2017). Rapid environmental changes in the Western Antarctic Peninsula region due to climate change and human activity. Appl. Ecol. Environ. Res. 15 (4), 525–539. doi:10.15666/aeer/1504_525539
Zwoliński, Z. (2007). “Mobilność materii mineralnej na obszarach paraglacjalnych, Wyspa Króla Jerzego, Antarktyka Zachodnia [the mobility of mineral matter in paraglacial area, King George Island, western Antarctica - in polish,” in Seria geograficzna 74 (Poznań: Adam Mickiewicz University Press), 266.
Keywords: UAV, King George Island, the Antarctic, NDVI, vegetation
Citation: Zmarz A, Karlsen SR, Kycko M, Korczak-Abshire M, Gołębiowska I, Karsznia I and Chwedorzewska K (2023) BVLOS UAV missions for vegetation mapping in maritime Antarctic. Front. Environ. Sci. 11:1154115. doi: 10.3389/fenvs.2023.1154115
Received: 30 January 2023; Accepted: 17 April 2023;
Published: 09 May 2023.
Edited by:
Meenu Rani, Kumaun University, IndiaCopyright © 2023 Zmarz, Karlsen, Kycko, Korczak-Abshire, Gołębiowska, Karsznia and Chwedorzewska. This is an open-access article distributed under the terms of the Creative Commons Attribution License (CC BY). The use, distribution or reproduction in other forums is permitted, provided the original author(s) and the copyright owner(s) are credited and that the original publication in this journal is cited, in accordance with accepted academic practice. No use, distribution or reproduction is permitted which does not comply with these terms.
*Correspondence: Anna Zmarz, YXptYXJ6QHV3LmVkdS5wbA==
†ORCID ID: Anna Zmarz, orcid.org/0000-0001-5846-4017; Stein Rune Karlsen, orcid.org/0000-0002-9456-6990; Marlena Kycko, orcid.org/0000-0001-5133-3727; Małgorzata Korczak-Abshire, orcid.org/0000-0001-7695-0588; Izabela Gołębiowska, orcid.org/0000-0002-4307-7054; Izabela Karsznia, orcid.org/0000-0001-5510-8770; Katarzyna Chwedorzewska, orcid.org/0000-0001-6860-3666