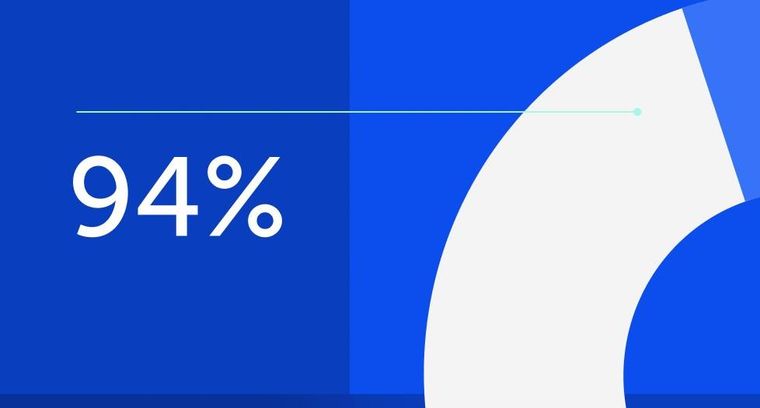
94% of researchers rate our articles as excellent or good
Learn more about the work of our research integrity team to safeguard the quality of each article we publish.
Find out more
ORIGINAL RESEARCH article
Front. Environ. Sci., 30 May 2023
Sec. Soil Processes
Volume 11 - 2023 | https://doi.org/10.3389/fenvs.2023.1152439
This article is part of the Research TopicSoil Health, Functions, and Ecosystem Services: Insights into Soil Parameters and Methods of IntegrationView all 7 articles
Enhancing soil organic carbon (SOC) stocks through fertilization and crop rotation will contribute to sustaining crop productivity and mitigating global warming. In this study, we analyzed the differences in total SOC stocks and their driving factors in the topsoil (0–20 cm) with various fertilization measures in two puddled lowland rice-based cropping systems (i.e., rice-wheat rotation and double rice rotation systems) over the last four decades from seven long-term experiments in the Yangtze River catchment. The soil types include Cambisol, Luvisol, and Anthrosol. The treatments include no fertilizer application (CK), application of chemical nitrogen, phosphorus and potassium fertilizers (NPK) and a combination of NPK and manure applications (NPKM). Every year, field was ploughed to a depth of 15–20 cm before wheat sowing and rice transplanting. Residue was removed after plant harvesting. Results showed that during the last four decades, the average crop grain yield ranged from 1,151 ± 504 kg ha−1 yr−1 under CK treatment to 7,553 ± 1,373 kg ha−1 yr−1 under NPKM treatment. The topsoil SOC stock significantly increased by 8.6 t ha−1 on average under NPKM treatment in rice-wheat system and by 2.5–6.4 t ha−1 on average under NPK and NPKM treatments in double rice system as compared with CK. A higher SOC sequestration rate and a longer SOC sequestration duration were found in NPKM treatment than that in NPK treatment in both cropping systems. The highest SOC stock ratio (SOC stock in fertilizer treatments to CK) was observed under the NPKM treatment in both cropping systems, though no significant difference was found between these two cropping systems. However, the fertilization-induced relative increase of the SOC stock was 109.5% and 45.8% under the NPK and NPKM treatments, respectively in the rice-wheat system than that in the double rice system. This indicates that the rice-wheat system is more conducive for SOC sequestration. RF and SEM analyses revealed that the magnitude and influencing factors driving SOC sequestration varied between two systems. In the double rice system, continuous flooding weakens the influence of precipitation on SOC sequestration and highlights the importance of soil properties and C input. In contrast, soil properties, C input and climate factors all have important impacts on SOC sequestration in rice-wheat system. This study reveals that the rice-wheat system is more favorable for SOC sequestration despite its lower C input compared to the double rice system in China’s paddies.
Soil constitutes the largest terrestrial soil organic carbon (SOC) pool in the world and plays a key role in ensuring food security and mitigating climate change. Paddy soils are more favorable to the accumulation of organic carbon (OC) than upland soils due to the presence of floodwater causing anaerobic conditions (Pan and Zhao, 2005). All over the world, paddy soils (0–100 cm) contain a considerable SOC pool (i.e. 18 Pg C), of which China, India and Indonesia together contribute to more than half of the global SOC pool of paddies (Liu et al., 2021). More precisely, the surface SOC pool (the plow layer and the plowpan) of paddy soils in China has been estimated at ∼1.31 Pg (Pan et al., 2003). Hence, the management of paddy soils may have a great impact on the net soil–atmosphere C exchange at the national scale, and therefore, is of great significance for the delivery of key ecosystem services such as climate regulation.
Soil organic carbon can be sequestrated in soils as the result of C input and mineralization rates which are on its turn affected by climatic conditions, soil properties and agronomic practices (e.g., fertilization, residue retention, irrigation, crop rotation, tillage, etc.) (Follett, 2001; Wiesmeier et al., 2019). Consequently, any factors that influence the C input and mineralization will influence the SOC stock. Improved agronomic practices, such as chemical fertilizers application, manure amendment or diversified cropping, have been proved to enhance SOC sequestration in agricultural soils (Chen et al., 2014). For example, a global synthesis with 4,803 paired observations from 369 experimental sites indicated that nitrogen fertilization significantly promoted SOC content by 4.2% on average (Xu et al., 2021). In addition, based on a global synthesis, Li et al. (2021) showed that manure amendment could increase SOC stock by 8.96 t C ha−1 on average as compared to unfertilizer treatment.
Over the last two decades, mounting evidence has demonstrated that the average SOC stocks in paddy soils are higher than those in upland soils at both the regional (Sun et al., 2015) and global (Wei et al., 2021) scale. This is mainly attributed to the following reasons: 1) the higher residue input in paddy than upland soils (Liu et al., 2019); 2) the lower decomposition rate and turnover rate due to the lower microbial activity under anaerobic conditions (Qiu et al., 2017); 3) the higher content of recalcitrant C fractions in paddy soils such as cell walls derived components like lignin (Chen et al., 2021); 4) some specific soil organic matter (SOM) protection mechanisms in paddy soils like “enzyme latch” mechanism or the adsorption of SOM to iron (Fe) oxides (Freeman et al., 2001; Wissing et al., 2013).
In 2021, rice yield accounts for 40% of China’s total grain yield (NBSC, https://data.stats.gov.cn/). Rice is typically planted in three cropping systems in China: single rice cropping, mainly located in North China, rice-upland cropping, mainly located in Central China and double rice cropping, mainly located in South China (Huang et al., 2012). The rice production in the three cropping systems accounts for 15.5, 43.4, and 41.1%, respectively of China’s total rice production (Guo et al., 2017). Various cropping systems are characterized by different climatic and soil conditions as well as different agronomic practices (e.g., crop varieties, residue retention rate and duration of flooding). For example, the average soil temperature in the three cropping systems are 15, 26 and 25°C, respectively (Cai et al., 2003; Yue et al., 2005; Yang et al., 2010). Among the three cropping systems, the residue retention rate in the single rice cropping is the lowest and the duration of flooding in the double rice cropping is the longest (Feng et al., 2013). In the context of climate change, many factors constrain the rice planting and production in China, such as the shortage of irrigation water resources (Piao et al., 2010), the shortened crop growth duration (Zhang et al., 2013) and the changes in the planting structure (Chen et al., 2020). As such, the adoption of new cultivars, shifted sowing date and the optimized usage of water and fertilizers is proposed to mitigate the negative effects of global warming (Hu et al., 2017; Hussain et al., 2020).
Cropping systems may affect SOC stocks by influencing the balance between C input and C decomposition (Toriyama, K et al., 2005). Previous studies have shown that the SOC dynamics in paddy soils are influenced to a large extent by the different C input levels depending on the crop rotation (Cha-un et al., 2017). In addition, the different rice cropping system specific agro-management practices are other important drivers of the long-term SOC trends in paddy soils. It is generally considered that continuous flooded paddy soils have the greatest potential for sequestering SOC as compared to other cropping systems, as continuous flooding can retard the microbial decomposition and thus stimulate long-term SOC accumulation (Marschner, 2021). For example, Huang et al. (2012) indicated that the double rice cropping system leads to a higher increase of the SOC stock than rice-wheat and single rice cropping systems under the application of chemical N, P, and K fertilizers when no SOC saturation trend was found. In addition, the periodic wetting-drying cycles in rice-wheat system may affect the aggregate stability and thus decrease the physical protection of SOC (Jastrow et al., 2007). However, some studies come to the opposite conclusion. For example, Mehmood et al. (2020) showed that the maize-rice cropping system enhanced aggregate stability through increasing of small macro-aggregates (0.25–2 mm), resulting in more SOC sequestration in comparison with adjacent continuous flooded rice following biochar addition. Moreover, the increased diversity of soil microbes (Zhou et al., 2014) and the high efficiency of microbial assimilation of organic C (Wei et al., 2022) in paddy-upland system than continuous flooded paddies may also contributed to the accumulation of SOC. This inconsistency between different studies results from the various conditions of the climate, soil and agronomic management (Yu et al., 2009; Huang et al., 2012; Tian et al., 2015; Mehmood et al., 2020). Therefore, an improved understanding of the SOC dynamics in paddy soils in response to different cropping systems as well as various fertilization strategies, is critical to improve SOC sequestration in paddy soils and make a contribution to mitigating climate change.
The middle and lower catchments of the Yangtze River is a major rice production area in China, which accounts for 44.2% of the total rice planting area and 44.4% of the total rice production in China (Liu et al., 2011). The long-term experiments established in 1980s/1990s in paddies in the middle and lower catchments of the Yangtze River provide a good opportunity to explore the SOC sequestration and its influencing factors under different fertilization measures and rice-based cropping systems. Therefore, our study uses this particular data resource in order to address following objectives: 1) explore the impacts of different fertilization measures and rice-based cropping systems (rice-wheat system and double rice system) on SOC sequestration; and 2) assess the main influencing factors of SOC sequestration for the two cropping systems under fertilization treatment.
Datasets from long-term experiment (i.e. 1980s/1990s until 2010s) from puddled lowland paddy fields located along seven locations along the middle and lower reaches of the Yangtze River were used for this study (Figure 1). The study area is characterized by a subtropical climate with typical cropping systems of rice-wheat and double rice rotation. Information about the location, weather condition and soil properties at the beginning of the experiments are presented in Table 1.
FIGURE 1. Geographic locations of seven long-term experimental sites (A) and distributions of soil organic carbon stock in the topsoil (0–20 cm) under different fertilization treatments over the last four decades with the rice-wheat system (B) and double rice system (C). Rice-wheat system: WH, Wuhan; SN, Suining; CQ, Chongqing; SZ, Suzhou; Double rice system: NC, Nanchang; JX, Jinxian; QY, Qiyang.
TABLE 1. Location, weather condition and soil properties at the beginning of the seven long-term experiments in the Yangtze River catchment.
The rice-wheat rotation was established at sites located in Wuhan (WH), Suining (SN), Chongqing (CQ) and Suzhou (SZ), while the rotation of double rice at sites in Nanchang (NC), Jinxian (JX) and Qiyang (QY) (Figure 1). The treatments include i) no fertilizer application (CK), ii) application of chemical nitrogen, phosphorus and potassium fertilizers (NPK) and iii) a combination of NPK and manure applications (NPKM). Table 2 shows the application rates of urea derived inorganic N, P (Calcium superphosphate or monoammonium phosphate), K (Potassium Chloride or potassium sulphate) fertilizers as well as manure at the sites.
TABLE 2. Inorganic nitrogen (N), phosphorus (P), potassium (K) fertilizers (kg ha-1) and manure application rates in each cropping season under different fertilization treatments at 7 long-term experimental sites in the Yangtze River catchment.
Each year, plots were ploughed to a depth of between 15 and 20 cm before wheat sowing and rice transplanting. In the rice-wheat system, rice transplanting took place somewhere between late May and early June, whereas harvest occurred between late September and early October. Subsequently, wheat sowing and harvesting took place the following year in late October—early November and late May, respectively. In the double rice system, early rice transplanting and harvesting occurred in late April—early May and mid to late July, respectively, whereas late rice transplanting and harvesting were at late July and late October—early November, respectively. Irrigation was implemented in rice season, while no irrigation in wheat season. Pesticide was used to control pests and weeds when necessary. Crop plants were harvested manually leaving stubbles with a height of 6–15 cm. Harvested plants were collected, air-dried and oven-dried for 30 min at 105°C, then heated at 70°C to a constant weight for determination of nutrient contents. Soils were sampled until a depth of 20 cm every year. Air-dried soils were sieved through a 2 mm mesh to measure soil pH. Subset soil samples were passed through a 0.25 mm sieve for the determination of total SOC with a modified Walkley & Black method (Heating solution for 12 min under 220°C, and a correction factor of 1.1 was applied) (Walkley and Black, 1934; Meersmans et al., 2009) and total N (TN) content (Black, 1965). Soil bulk density (BD) was measured with steel ring (inner diameter, 5 cm) (Vomocil, 1957).
C input include crop residues (roots and stubble) and animal manure. A ratio of belowground biomass to aboveground biomass was used to estimate the C input of roots (Jiang et al., 2014). Root biomass was assumed as 30% of the total plant biomass for wheat with the assumption that 75.3% of roots are located in the top 20 cm of soil (Jiang et al., 2014). Belowground biomass to aboveground biomass ratio was assumed as 30% for rice (Kundu et al., 2007). For wheat, the stubble quantity was estimated as 13.1% (fertilizer treatments) and 18.3% (control) of the straw yield (t ha−1 yr−1) (Xu et al., 2006; Jiang et al., 2014). For rice, the stubble quantity was estimated as 5.6% of the straw yield (t ha−1 yr−1) considering all treatments (Huang et al., 2015). We applied the national average carbon contents for wheat residues, rice roots and rice straw (i.e., 399, 418 and 444 g C kg−1, respectively) on oven-dried basis (NCATS, 1994).
The total soil organic carbon stock (SOC, t C ha−1) in the top 20 cm soil is estimated as:
where SOCC is soil C content (g kg−1), BD is soil bulk density (g cm−3), H is the thickness (cm), and 0.1 is a conversion coefficient.
The SOC stock change (∆SOC) and its change rate (SOCR) are calculated by:
where SOCn (t C ha−1) is SOC stock in different treatment (CK, NPK and NPKM) of the year n, SOCi is the initial SOC stock, n is the experimental duration (year).
Following Li et al. (2021), SOC stock ratio (%SOC) between a fertilizer treatment and CK is defined as:
where SOCTn and SOCCn (t C ha−1) are SOC stocks in fertilizer (NPK, NPKM) and CK treatments in the year n, respectively.
According to Berhane et al. (2019), the fertilization-induced changes of the SOC stock (%∆SOC) in fertilizer treatments relative to CK are calculated by the following formula, in which a positive value indicate fertilization-induced increase of the SOC stock and a negative value indicate fertilization-induced decrease as compared to its initial level.
The statistical software R 4.1.1 have been used for all the analyses. The statistically significant differences in SOC stocks among fertilization treatments and the statistically significant differences in SOC stock ratio or the fertilization-induced changes of the SOC stock between the two cropping systems were analyzed by one-way ANOVA followed by the Fisher’s LSD test (p = 0.05). The relationships of SOC stock changes and its change rate with cumulative C input and experimental duration were fitted by logarithmic regression. According to Six et al. (2002), soil C saturation occurs when the soil is incapable of accumulating additional C following changes in cropland management, and as such a new equilibrium has been reach. SOC sequestration duration refers to the time during which sequestration occurs (West and Six, 2007). With the assumption that a new SOC steady state occurs when the rate of SOC stock changes is lower than 0.01 t C ha−1 yr−1, we estimated the SOC sequestration duration.
Random forest (RF) with percentage increases in the MSE (mean squared error) was applied to quantify the relative importance of different environmental variables (MAT, MAP, C input, TN, initial SOC content and soil pH) (Breiman, 2001). With the “A3” package, we obtained the significance of the model and the cross-validated R2 value by considering 5,000 permutations of the response variable. Using the “rfPermute” package, we determined the significance of each driving factor on the response variable (Jiao et al., 2018). Based on the identified factors regulating SOC sequestration, a structural equation modeling (SEM) (IBM SPSS Amos 21.0 software) was used to clarify the direct and indirect effects of climatic condition (MAT and MAP), soil properties (TN, initial SOC content and soil pH) and C input on the fertilization-induced changes of the SOC stock in the two cropping systems. The following criteria were used to evaluate the overall performance of the model: The chi-square to degrees of freedom ratio (χ2/df < 2), probability level (p > 0.05), comparative fit index (CFI >0.9), root mean square error of approximation (RMSEA <0.08) and the Akaike information criterion (AIC: the model has a good fit when AIC is low) (Wen et al., 2004) (Figure 2).
The average and dynamic changes of grain and straw yield of wheat and rice in two cropping systems are shown in Tables 3, 4 and Supplementary Figure S2. Overall, NPKM treatment produced the highest grain and straw yield for both rice and wheat in two systems. In rice-wheat system, the C inputs under NPKM treatment are double that of NPK treatment and four times that of CK treatment (Figure 3A). In double rice system, the C inputs under NPKM treatment are threefold that of NPK treatment and five times that of CK treatment (Figure 3B).
TABLE 3. Average grain yield of wheat and rice in two cropping systems from the seven long-term experiments in the Yangtze River catchment.
TABLE 4. Average straw yield of wheat and rice in two cropping systems from the seven long-term experiments in the Yangtze River catchment.
FIGURE 3. C input under different fertilization treatments in rice-wheat system (A) and double rice system (B) during the last four decades. Small letters indicate significant differences between treatments at p < 0.05. CK, no fertilizer application; NPK, application of chemical nitrogen, phosphorus and potassium fertilizers; NPKM, a combination of NPK and manure applications. IQR: interquartile range.
The topsoil SOC stocks (0–20 cm) were 36.2, 39.5 and 44.8 t C ha−1 (Figure 4A) on average under CK, NPK and NPKM treatments, respectively in the rice-wheat system, and were 36.0, 38.5 and 42.4 t C ha−1 (Figure 4B) on average under CK, NPK and NPKM treatments, respectively in the double rice system. During the last four decades, the topsoil SOC stocks significantly increased by 8.6 t ha−1 on average under NPKM treatment in the rice-wheat system and by 2.5–6.4 t ha−1 on average under NPK and NPKM treatments in the double rice system compared with CK.
FIGURE 4. The total soil organic carbon (SOC) stock (0–20 cm) under different fertilization treatments in rice-wheat system (A) and double rice system (B) during the last four decades. Small letters indicate significant differences between treatments at p < 0.05. CK, no fertilizer application; NPK, application of chemical nitrogen, phosphorus and potassium fertilizers; NPKM, a combination of NPK and manure applications. IQR: interquartile range.
The SOC stock changes in rice paddies showed a significant logarithmic correlation with cumulative C input under all fertilization treatments (Figure 5A). According to the regressions of different treatments, the increase in cumulative C input caused significant SOC stock increases in the initial stage and then showed non-significant SOC stock increases over a longer period of time. And the potential SOC stock increases in NPK treatment are much lower than that in NPKM treatment (Figure 5A). The rate of SOC stock changes decreased significantly with increasing fertilization duration and gradually approached zero in NPK and NPKM treatments (Figure 5B). Moreover, a higher SOC sequestration rate and a longer SOC sequestration duration were found in NPKM treatment compared to NPK treatment (Figure 5B).
FIGURE 5. Relationships of SOC stock changes (∆SOC) (0–20 cm) (A) and its change rate (SOCR) (B) with cumulative C input and experimental duration under different fertilization treatments (CK, no fertilizer application; NPK, application of chemical nitrogen, phosphorus and potassium fertilizers; NPKM, a combination of NPK and manure applications) in paddies during the last four decades.
The SOC stock ratio of NPK and NPKM treatments were 109.3% ± 1.4% (mean ± standard error) and 120.4% ± 1.8%, respectively, in rice-wheat system, and were 108.6% ± 2.2% and 123.6% ± 2.3%, respectively, in double rice system (Figure 6A). The highest SOC stock ratio was observed under NPKM treatment in both cropping systems, and no significant difference was found between these two cropping systems both in NPK and NPKM treatments (Figure 6A). However, the fertilization-induced changes of the SOC stock from its initial level in fertilizer treatments relative to CK was higher in rice-wheat system (i.e., NPK: 15.5%, NPKM: 31.5%) than that in double rice system (i.e., NPK: 7.4%, NPKM: 21.6%) (Figure 6B). This indicates a greater fertilization-induced increase of the SOC stock under rice-wheat system as compared to its initial SOC stock during the 30–40 years of fertilization.
FIGURE 6. Differences in SOC stock ratio (%SOC) (A) and the fertilization-induced changes of the SOC stock (%∆SOC) (B) between the two systems (rice-wheat system and double rice system) under NPK and NPKM treatments. Symbols above the paired boxplots indicate statistic differences between the systems (one-way ANOVA, ns: non significance, *p < 0.05). NPK, application of chemical nitrogen, phosphorus and potassium fertilizers; NPKM, a combination of NPK and manure applications. IQR: interquartile range.
RF and SEM analyses showed that the dominant factors regulating the SOC sequestration differed between the two cropping systems (Figure 7). RF analysis indicated that soil properties (TN, initial SOC content and pH) and climate factors (MAT and MAP) together impacts on SOC sequestration in the rice-wheat system (Figure 7C). Whereas soil properties (initial SOC and TN content), C input and MAT play an important role in controlling SOC sequestration in the double rice system (Figure 7D). SEM confirmed the importance of soil properties with a total standardized direct effect of 1.09 in the rice-wheat system. And meanwhile highlighted the importance of C input in regulating SOC sequestration, with a standardized direct effect of 0.22 in the rice-wheat system (Figure 7A; Supplementary Figure S1). While SOC sequestration was dominantly and directly affected by initial SOC content, TN content and C input, with standardized direct effects of −0.89, 0.44 and 0.28, respectively, in the double rice system (Figure 7B; Supplementary Figure S1).
FIGURE 7. Structural equation modeling (SEM) (A,B) and random forest (RF) (C,D) analyses of the fertilization-induced changes of the SOC stock (%∆SOC) with explanatory variables in rice-wheat system (A,C) and double rice system (B,D). The red and blue lines in (A,B) represent positive and negative effects, respectively. The line width and the numbers above the lines (standardized path coefficient) corresponding to the strength of the path. Solid and dashed lines represent significant and nonsignificant paths, respectively (*p < 0.05, **p < 0.01, ***p < 0.001). The R2 value indicates the proportion of variance explained by all variables. MAT, mean annual temperature; MAP, mean annual precipitation; Initial SOC, soil organic carbon content at the beginning of the experiment; TN, soil total nitrogen content; pH, soil pH.
Many studies have shown the benefits of fertilizer application, manure amendments, straw retention, crop rotation and conservation tillage for SOC sequestration (West and Post, 2002; Lu et al., 2009). Based on the long-term experimental dataset, our study re-quantified the magnitude of SOC stock changes induced by chemical fertilizers and manure application in China’s paddies. Our result of SOC increment in rice-wheat system (Figure 4) is close to the value of 8.96 t C ha−1 reported by Li et al. (2021), and the result in double rice system is within the range of 2.5–13.5 t C ha−1 yr−1 as being reported by Maillard and Angers (2014). Similarly, Rui and Zhang (2010) synthesized results from 26 long-term field experiments and concluded that the application of crop residue and manure enhanced SOC stock by 0.41 and 0.34 t C ha−1 yr−1 over approximately four decades in paddy soils as compared to fields where no fertilizer were applied, respectively. Among fertilization practices, chemical fertilizers combined with manure (NPKM) showed the highest SOC stock (Figure 4; Supplementary Table S1), which seems to be in agreement with the results obtained in previous studies (e.g., Brar et al., 2013; Zhu et al., 2015; Atere et al., 2020). This result might be explained by the following factors: firstly, in contrary to chemical fertilizers, manure applications are causing a direct C input into soil; Secondly, manure amendments can increase C input indirectly through enhancing plant productivity; Thirdly, the manure amendment derived fresh organic matter input can accelerate the formation of microaggregates within macroaggregates, and therefore results in an improved sequestration of more stable intra-aggregate particulate organic matter (iPOM) (Six et al., 1998; Six et al., 1999; Six et al., 2000).
Our results indicated that fertilization-induced increase of the SOC stock was higher in rice-wheat system than that in double rice system (Figure 6B). This may be caused by the following reasons: firstly, in rice-wheat system, the aerobic and anaerobic cycles caused by flooding and drainage will accelerate the process of iron (Fe) or aluminum (Al) oxides being adsorbed by organic compounds (Wei et al., 2022), and meanwhile more amorphous Fe oxides are formed with greater reactive mineral surface area for C accumulation than crystalline Fe oxides (Wissing et al., 2014); Secondly, the frequent drying-rewetting cycles can increase the quantity and diversity of soil organisms (Zhou et al., 2014), and as such the higher ratio of microbial biomass C to SOC lead to a high microbial C use efficiency (Wei et al., 2022); Thirdly, in comparison with continuous waterlogging, drying-rewetting cycles combined with the application of manure can improve soil water stable aggregation (Yang et al., 2005).
The logarithmic correlation between the SOC stock changes and cumulative C input under fertilizer treatments indicates that C input act as the main driving factor of SOC stock increases (Figure 5A). Furthermore, these results are showing that China’s paddy soils are being characterized by a steady state C trend under the current land management, indicating C saturation. Based on the equations, we estimated that the SOC sequestration duration is between 50 and 85 years for NPK and NPKM treatments in China’s paddy soils (Figure 5B). This means that the paddy soils in China can continue sequestering C for 11 and 46 years under NPK and NPKM treatments, respectively. This result is higher than the estimations from previous studies. For example, Tian et al. (2015) reported that the SOC sequestration duration are 28–34 and 46–51 years for NPK and NPKM treatments, respectively. Similarly, Zhu et al. (2015) estimated that the SOC sequestration duration is 46–55 years under NPK and NPKM treatments. The difference between our results and others is probably the result of the differences in site-specific conditions, including soil texture, climate condition and field management (West and Six, 2007). Specifically, many studies have shown that SOC sequestration rates are higher in soils characterized by higher clay content (Hassink, J, 1997; Liu et al., 2014; Gross, A and Glaser, B, 2021). In addition, differences in field management and land use may result in different C input rates (e.g., crop residues and manure) or C losses through decomposition (Ma et al., 2021). Hence, in essence, our results seem to confirm the advantage in SOC sequestration in paddy soils as compared to other land uses as being highlighted by numerous other studies (e.g., Huang et al., 2014; Wissing et al., 2014; Hou et al., 2018).
Consistent with previous researches (Mandal et al., 2008; Tian et al., 2015; Fujisaki et al., 2018), in this study, greater SOC sequestration rate and longer SOC sequestration duration were found in the NPKM treatment as compared to the NPK treatment (Figure 5). This is probably the consequence of a higher direct C input rate from manure addition as well as enhanced indirect C input rates (e.g., crop residue and root derived C) due to higher crop productivity in the NPKM treatment. In essence, our study clearly indicates that the application of chemical fertilizers combined with manure (NPKM) can be considered as an effective measure to enhance SOC sequestration in paddy soils.
Our results showed that the magnitude and influencing factors driving SOC sequestration varied between rice-based cropping systems (Figure 7; Supplementary Figure S1). In double rice system, continues flooding weakens the impact of precipitation on SOC sequestration and then highlighted the importance of soil properties (initial SOC and TN content) and field management practice (C input), which control SOC sequestration. In contrast, soil properties (TN, initial SOC content and pH), field management practice (C input) and climate factors (temperature and precipitation) all have important impacts on SOC sequestration in rice-wheat system. Similar result has been found in previous studies. For example, Wei et al. (2021) identified a smaller impact of climate on SOC stock dynamics in paddy soils as compared to corresponding upland soils. Furthermore, a global synthesis indicated that mean annual temperature and soil pH are having an important effect on SOC stock changes in paddy soils, but the impact of mean annual total precipitation amount and soil clay content is rather limited (Liu et al., 2021).
Initial SOC content was an important explanatory variable for SOC sequestration, which was similar to the findings of Zhao et al. (2018), who found that the initial SOC content explained ∼30% of the variation in SOC stock changes in Chinese croplands. This can be explained by the fact that, according to the concept of soil C saturation theory, soils with a lower initial SOC content level have a larger potential and a longer total duration of C sequestration (Stewart et al., 2007; Stewart et al., 2008). In this study, the impacts of initial SOC content on SOC sequestration is opposite in two systems (Figure 7), this may be because of the difference in initial SOC content. In rice-wheat system, the initial SOC content is relatively lower than that in double rice system (Table 1), which may have contributed to the higher SOC sequestration.
Furthermore, our results are line with previous studies, such as Virto et al. (2012), who indicating that C input was another important factor driving SOC sequestration in rice-based cropping systems. In this regard, Fujisaki et al. (2018) concluded that the SOC stock increases in tropical croplands are mainly driven by C input. Furthermore, Zhao et al. (2018) reported that the SOC sequestration in China’s croplands was primarily caused by increased OC input (crop residues) due to economic and policy changes during 1980–2010. More specifically, according to global synthesis, the C retention coefficient of added manure and straw are 12% and 16% in cropland, respectively (Liu et al., 2014; Maillard and Angers, 2014). The relatively higher total effect of C input on SOC sequestration in double rice system (0.43) as compared to rice-wheat system (0.32) (Figure 7; Supplementary Figure S1) may be attributed to its higher C input associated with its aerobic conditions. In the double rice system, the soil was submerged for about 7–8 months throughout the year, which hampered the decomposition rate of freshly added organic materials as well as the native SOM as compared to the aerobic conditions (Mandal et al., 2008).
The results of our study identified the advantage of SOC sequestration under long-term fertilization in two puddled lowland rice-based cropping systems, which may help to make adaptations of cropping systems in a changing climate, such as a conversion from continuous paddies to alternative paddy-upland systems, especially in a future context of irrigation water shortages (Schewe et al., 2014). However, the climate benefit of SOC sequestration in paddy soils is generally offset by inducing greenhouse gas (GHG) emissions (e.g., CH4, N2O). As reported, rice accounts for about half of the total crop emissions due to high CH4 emissions (Carlson et al., 2017). Specifically, CH4 and N2O emissions in paddies have been estimated as 6,300 kg CO2-eq ha−1 yr−1 and 280 kg CO2-eq ha−1 yr−1 globally (Carlson et al., 2017; FAO, 2018). In which the yield-scaled global warming potential (GWP) of the double rice system is higher than that of the paddy-upland system (Feng et al., 2013; Zhang et al., 2019). As such, a more comprehensive assessment of SOC sequestration, crop production and GHG emissions in terms of CO2 equivalents in paddy soils is needed in further research to achieve sustainable rice cultivation.
This study explored the difference in total SOC stocks (0–20 cm) and their driving factors under different fertilization treatments in two puddled lowland rice-based cropping systems over the last four decades. Results showed that the SOC stock significantly increased by 2.5–8.6 t ha−1 on average under fertilization in two cropping systems as compared to CK over the last four decades. The relationship between the rate of SOC stock changes and duration suggests that China’s paddy soils are having the potential to continue sequestering carbon for approximately 11–46 years under fertilization. The relatively higher fertilization-induced increase of the SOC stock from its initial level in rice-wheat system than that in double rice system suggesting that rice-wheat system is more beneficial to SOC sequestration. Soil properties, C input and MAT driving SOC sequestration in double rice system, in contrast, soil properties, C input and climate factors all have important impacts on SOC sequestration in rice-wheat system.
The data that supports the findings of this study are available from the corresponding author NS and co-author MX, upon reasonable request.
SW, NS, and MX contributed to conception and design of the study, SL and SZ contributed to analysis, SW performed the data analyses and wrote the first draft of the manuscript. SW, JM, GC, and LW contributed to the revision of the manuscript. All authors contributed to the article and approved the submitted version.
This study was supported by the National Key Research and Development Program of China (2021YFD1901205), the National Natural Science Foundation of China (42177341), SW was supported by the China Scholarship Council (No. 202103250053).
We acknowledge the Chinese Academy of Agricultural Sciences—Gembloux Agro-Bio Tech joint PhD program and all the colleagues from the long-term fertilization experimental sites for their unremitting assistance.
The authors declare that the research was conducted in the absence of any commercial or financial relationships that could be construed as a potential conflict of interest.
All claims expressed in this article are solely those of the authors and do not necessarily represent those of their affiliated organizations, or those of the publisher, the editors and the reviewers. Any product that may be evaluated in this article, or claim that may be made by its manufacturer, is not guaranteed or endorsed by the publisher.
The Supplementary Material for this article can be found online at: https://www.frontiersin.org/articles/10.3389/fenvs.2023.1152439/full#supplementary-material
Atere, C. T., Gunina, A., Zhu, Z. K., Xiao, M. L., Liu, S. L., Kuzyakov, Y., et al. (2020). Organic matter stabilization in aggregates and density fractions in paddy soil depending on long-term fertilization: Tracing of pathways by 13C natural abundance. Soil Biol. biochem. 149, 107931. doi:10.1016/j.soilbio.2020.107931
Black, C. A. (1965). Methods of soil analysis, part 2. Chemical and microbiological properties. Madison, Wisconsin, USA: American Society of Agronomy.
Brar, B. S., Singh, K., Dheri, G. S., and Kumar, B. (2013). Carbon sequestration and soil carbon pools in a rice-wheat cropping system: Effect of long-term use of inorganic fertilizers and organic manure. Soil till. Res. 128, 30–36. doi:10.1016/j.still.2012.10.001
Cai, Z., Tsuruta, H., Gao, M., Xu, H., and Wei, C. (2003). Options for mitigating methane emission from a permanently flooded rice field. Glob. Chang. Biol. 9, 37–45. doi:10.1046/j.1365-2486.2003.00562.x
Carlson, K., Gerber, J., Mueller, N., Herrero, M., MacDonald, G., Brauman, K., et al. (2017). Greenhouse gas emissions intensity of global croplands. Nat. Clim. Change 7, 63–68. doi:10.1038/nclimate3158
Cha-un, N., Chidthaisong, A., Yagi, K., Sudo, S., and Towprayoon, S. (2017). Greenhouse gas emissions, soil carbon sequestration and crop yields in a rain-fed rice field with crop rotation management. Agric. Ecosyst. Environ. 237, 109–120. doi:10.1016/j.agee.2016.12.025
Chen, C. Q., Groenigen, K. J. V., Yang, H. Y., Hungate, B. A., Yang, B., Tian, Y. L., et al. (2020). Global warming and shifts in cropping systems together reduce China's rice production. Glob. Food Secur. 24, 100359. doi:10.1016/j.gfs.2020.100359
Chen, X. B., Hu, Y. J., Xia, Y. H., Zheng, S. M., Ma, C., Rui, Y. C., et al. (2021). Contrasting pathways of carbon sequestration in paddy and upland soils. Glob. Chang. Biol. 27, 2478–2490. doi:10.1111/gcb.15595
Chen, X. P., Cui, Z. L., Fan, M. S., Vitousek, P., Zhao, M., Ma, W. Q., et al. (2014). Producing more grain with lower environmental costs. Nature 514, 486–489. doi:10.1038/nature13609
FAO (Food and Agriculture Organization of the United Nations) (2018). Faostat: FAO statistical databases. Available at: http://faostat.fao.org/default.aspx.
Feng, J. F., Chen, C. Q., Zhang, Y., Song, Z. W., Deng, A. X., Zheng, C. Y., et al. (2013). Impacts of cropping practices on yield-scaled greenhouse gas emissions from rice fields in China: A meta-analysis. Agric. Ecosyst. Environ. 164, 220–228. doi:10.1016/j.agee.2012.10.009
Follett, R. F. (2001). Soil management concepts and carbon sequestration in cropland soils. Soil till. Res. 61, 77–92. doi:10.1016/S0167-1987(01)00180-5
Freeman, C., Ostle, N., and Kang, H. (2001). An enzymic 'latch' on a global carbon store. Nature 409, 149. doi:10.1038/35051650
Fujisaki, K., Chevallier, T., Lardy, L. C., Albrecht, A., Razafimbelo, T., Masse, D., et al. (2018). Soil carbon stock changes in tropical croplands are mainly driven by carbon inputs: A synthesis. Agric. Ecosyst. Environ. 259, 147–158. doi:10.1016/j.agee.2017.12.008
Gross, A., and Glaser, B. (2021). Meta-analysis on how manure application changes soil organic carbon storage. Sci. Rep. 11, 5516. doi:10.1038/s41598-021-82739-7
Guo, J., Song, Z. F., Zhu, Y. J., Wei, W., Li, S. N., and Yu, Y. L. (2017). The characteristics of yield-scaled methane emission from paddy field in recent 35-year in China: A meta-analysis. J. Clean. Prod. 161, 1044–1050. doi:10.1016/j.jclepro.2017.06.073
Hassink, J. (1997). The capacity of soils to preserve organic C and N by their association with clay and silt particles. Plant Soil 191, 77–87. doi:10.1023/A:1004213929699
Hou, P. F., Chien, C. H., Chiang-Hsieh, Y. F., Tseng, K. C., Chow, C. N., Huang, H. J., et al. (2018). Paddy-upland rotation for sustainable agriculture with regards to diverse soil microbial community. Sci. Rep. 8, 7966. doi:10.1038/s41598-018-26181-2
Hu, X. Y., Huang, Y., Sun, W. J., and Yu, L. F. (2017). Shifts in cultivar and planting date have regulated rice growth duration under climate warming in China since the early 1980s. Agric. For. Meteorol. 247, 34–41. doi:10.1016/j.agrformet.2017.07.014
Huang, J., Zhang, Y. Z., Gao, J. S., Zhang, W. J., and Liu, S. J. (2015). Variation characteristics of soil carbon sequestration under long-term different fertilization in red paddy soil. J. Appl. Ecol. 26, 3373–3380. (in Chinese).
Huang, S., Pan, X. H., Guo, J., Qian, C. R., and Zhang, W. J. (2014). Differences in soil organic carbon stocks and fraction distributions between rice paddies and upland cropping systems in China. J. Soils Sediment. 14, 89–98. doi:10.1007/s11368-013-0789-9
Huang, S., Sun, Y. N., and Zhang, W. J. (2012). Changes in soil organic carbon stocks as affected by cropping systems and cropping duration in China’s paddy fields: A meta-analysis. Clim. Change 112, 847–858. doi:10.1007/s10584-011-0255-x
Hussain, S., Huang, J., Huang, J., Ahmad, S., Nanda, S., Anwar, S., et al. (2020). “Rice production under climate change: Adaptations and mitigating strategies,” in Environment, climate, plant and vegetation growth (Cham: Springer), 659–686. doi:10.1007/978-3-030-49732-3_26
Jastrow, J. D., Amonette, J. E., and Bailey, V. L. (2007). Mechanisms controlling soil carbon turnover and their potential application for enhancing carbon sequestration. Clim. Change 80, 5–23. doi:10.1007/s10584-006-9178-3
Jiang, G. Y., Xu, M., He, X. H., Zhang, W. J., Huang, S. M., Yang, X., et al. (2014). Soil organic carbon sequestration in upland soils of northern China under variable fertilizer management and climate change scenarios. Glob. Biogeochem. Cy. 28, 319–333. doi:10.1002/2013GB004746
Jiao, S., Chen, W. M., Wang, J. L., Du, N. N., Li, Q. P., and Wei, G. H. (2018). Soil microbiomes with distinct assemblies through vertical soil profiles drive the cycling of multiple nutrients in reforested ecosystems. Microbiome 6, 146. doi:10.1186/s40168-018-0526-0
Kundu, S., Bhattacharyya, R., Prakash, V., Ghosh, B. N., and Gupta, H. S. (2007). Carbon sequestration and relationship between carbon addition and storage under rainfed soybean–wheat rotation in a sandy loam soil of the Indian Himalayas. Soil till. Res. 92, 87–95. doi:10.1016/j.still.2006.01.009
Li, B. Z., Song, H., Cao, W. C., Wang, Y. J., Chen, J. S., and Guo, J. H. (2021). Responses of soil organic carbon stock to animal manure application: A new global synthesis integrating the impacts of agricultural managements and environmental conditions. Glob. Chang. Biol. 27, 5356–5367. doi:10.1111/gcb.15731
Liu, C., Lu, M., Cui, J., Li, B., and Fang, C. M. (2014). Effects of straw carbon input on carbon dynamics in agricultural soils: A meta-analysis. Glob. Chang. Biol. 20, 1366–1381. doi:10.1111/gcb.12517
Liu, W., Zhang, Q., and Liu, G. (2011). Effects of watershed land use and lake morphometry on the trophic state of Chinese lakes: Implications for eutrophication control. Clean. Soil Air Water 39, 35–42. doi:10.1002/clen.201000052
Liu, Y. L., Ge, T. D., van Groenigen, K. J., Yang, Y. H., Wang, P., Cheng, K., et al. (2021). Rice paddy soils are a quantitatively important carbon store according to a global synthesis. Commun. Earth Environ 2, 154. doi:10.1038/s43247-021-00229-0
Liu, Y. L., Ge, T. D., Zhu, Z. K., Liu, S. L., Luo, Y., Li, Y., et al. (2019). Carbon input and allocation by rice into paddy soils: A review. Soil Biol. biochem. 133, 97–107. doi:10.1016/j.soilbio.2019.02.019
Lu, F., Wang, X. K., Han, B., Ouyang, Z. Y., Duan, X. N., Zheng, H., et al. (2009). Soil carbon sequestrations by nitrogen fertilizer application, straw return and no-tillage in China's cropland. Glob. Chang. Biol. 15, 281–305. doi:10.1111/j.1365-2486.2008.01743.x
Ma, W. Z., Zhan, Y., Chen, S. C., Ren, Z. Q., Chen, X. J., Qin, F. J., et al. (2021). Organic carbon storage potential of cropland topsoils in East China: Indispensable roles of cropping systems and soil managements. Soil till. Res. 211, 105052. doi:10.1016/j.still.2021.105052
Maillard, É., and Angers, D. A. (2014). Animal manure application and soil organic carbon stocks: A meta-analysis. Glob. Chang. Biol. 20, 666–679. doi:10.1111/gcb.12438
Mandal, B., Majumder, B., Adhya, T. K., Bandyopadhyay, P. K., Gangopadhyay, A., Sarkar, D., et al. (2008). Potential of double-cropped rice ecology to conserve organic carbon under subtropical climate. Glob. Chang. Biol. 14, 2139–2151. doi:10.1111/j.1365-2486.2008.01627.x
Marschner, P. (2021). Processes in submerged soils-linking redox potential, soil organic matter turnover and plants to nutrient cycling. Plant Soil 464, 1–12. doi:10.1007/s11104-021-05040-6
Meersmans, J., Van Wesemael, B., and Van Molle, M. (2009). Determining soil organic carbon for agricultural soils: A comparison between the Walkley and black and the dry combustion methods (north Belgium). Soil Use Manage 25, 346–353. doi:10.1111/j.1475-2743.2009.00242.x
Mehmood, I., Qiao, L., Chen, H. Q., Tang, Q. Y., Woolf, D., and Fan, M. S. (2020). Biochar addition leads to more soil organic carbon sequestration under a maize-rice cropping system than continuous flooded rice. Agric. Ecosyst. Environ. 298, 106965. doi:10.1016/j.agee.2020.106965
NCATS (1994). Chinese organic fertilizer handbook. Beijing, China: National Center for Agricultural Technology Service.
Pan, G. X., Li, L. Q., Wu, L. S., and Zhang, X. H. (2003). Storage and sequestration potential of topsoil organic carbon in China’s paddy soils. Glob. Chang. Biol. 10, 79–92. doi:10.1111/j.1365-2486.2003.00717.x
Pan, G. X., and Zhao, Q. G. (2005). Study on evolution of organic carbon stock in agricultural soils of China: Facing the challenge of global change and food security. Adv. earth Sci. 20, 384–393. doi:10.11867/j.issn.1001-8166.2005.04.0384
Piao, S., Ciais, P., Huang, Y., Shen, Z. H., Peng, S. S., Li, J. S., et al. (2010). The impacts of climate change on water resources and agriculture in China. Nature 467, 43–51. doi:10.1038/nature09364
Qiu, H. S., Zheng, X. D., Ge, T. D., Dorodnikov, M., Chen, X. B., Hu, Y. J., et al. (2017). Weaker priming and mineralisation of low molecular weight organic substances in paddy than in upland soil. Eur. J. Soil Biol. 83, 9–17. doi:10.1016/j.ejsobi.2017.09.008
Rui, W. Y., and Zhang, W. J. (2010). Effect size and duration of recommended management practices on carbon sequestration in paddy field in Yangtze delta plain of China: A meta-analysis. Agric. Ecosyst. Environ. 135, 199–205. doi:10.1016/j.agee.2009.09.010
Schewe, J., Heinke, J., Gerten, D., Haddeland, I., Arnell, N. W., Clark, D. B., et al. (2014). Multimodel assessment of water scarcity under climate change. PNAS 111 (9), 3245–3250. doi:10.1073/pnas.1222460110
Six, J., Conant, R. T., Paul, E. A., and Paustian, K. (2002). Stabilization mechanisms of soil organic matter: Implications for C-saturation of soils. Plant Soil 241, 155–176. doi:10.1023/A:1016125726789
Six, J., Elliott, E. T., and Paustian, K. (2000). Soil macroaggregate turnover and microaggregate formation: A mechanism for C sequestration under no-tillage agriculture. Soil Biol. biochem. 32, 2099–2103. doi:10.1016/S0038-0717(00)00179-6
Six, J., Elliott, E. T., Paustian, K., and Doran, J. W. (1998). Aggregation and soil organic matter accumulation in cultivated and native grassland soils. Soil Sci. Soc. Am. J. 62, 1367–1377. doi:10.2136/sssaj1998.03615995006200050032x
Six, J., Elliott, T., and Paustian, K. (1999). Aggregate and soil organic matter dynamics under conventional and No-tillage systems. Soil Sci. Soc. Am. J. 63, 1350–1358. doi:10.2136/sssaj1999.6351350x
Stewart, C. E., Paustian, K., Conant, R. T., Plante, A. F., and Six, J. (2007). Soil carbon saturation: Concept, evidence and evaluation. Biogeochemistry 86, 19–31. doi:10.1007/s10533-007-9140-0
Stewart, C. E., Paustian, K., Conant, R. T., Plante, A. F., and Six, J. (2008). Soil carbon saturation: Evaluation and corroboration by long-term incubations. Soil Biol. biochem. 40 (7), 1741–1750. doi:10.1016/j.soilbio.2008.02.014
Sun, Y. N., Huang, S., Yu, X. C., and Zhang, W. J. (2015). Differences in fertilization impacts on organic carbon content and stability in a paddy and an upland soil in subtropical China. Plant Soil 397, 189–200. doi:10.1007/s11104-015-2611-5
Tian, K., Zhao, Y. C., Xu, X. H., Hai, N., Huang, B., and Deng, W. J. (2015). Effects of long-term fertilization and residue management on soil organic carbon changes in paddy soils of China: A meta-analysis. Agric. Ecosyst. Environ. 204, 40–50. doi:10.1016/j.agee.2015.02.008
Toriyama, K., Heong, K. L., and Hardy, B. (2005). “Rice is life: Scientific perspectives for the 21 st century,” in Proceedings of the World Rice Research Conference, Tsukuba, Japan, November 4-7, 2004, 377–379.
Virto, I., Barré, P., Burlot, A., and Chenu, C. (2012). Carbon input differences as the main factor explaining the variability in soil organic C storage in no-tilled compared to inversion tilled agrosystems. Biogeochemistry 108, 17–26. doi:10.1007/s10533-011-9600-4
Vomocil, J. A. (1957). Measurement of soil bulk density and penetrability: A review of methods. Adv. Agron. 9, 159–175. doi:10.1016/S0065-2113(08)60112-1
Walkley, A., and Black, I. A. (1934). An examination of the degtjareff method for determining soil organic matter, and a proposed modification of the chromic acid titration method. Soil Sci. 37, 29–38. doi:10.1097/00010694-193401000-00003
Wei, L., Ge, T. D., Zhu, Z. K., Luo, Y., Yang, Y. H., Xiao, M. L., et al. (2021). Comparing carbon and nitrogen stocks in paddy and upland soils: Accumulation, stabilization mechanisms, and environmental drivers. Geoderma 398, 115121. doi:10.1016/j.geoderma.2021.115121
Wei, L., Ge, T. D., Zhu, Z. K., Ye, R. Z., Peñuelas, J., Li, Y. H., et al. (2022). Paddy soils have a much higher microbial biomass content than upland soils: A review of the origin, mechanisms, and drivers. Agric. Ecosyst. Environ. 326, 107798. doi:10.1016/j.agee.2021.107798
Wen, Z. L., Hau, K. T., and Marsh, H. W. (2004). Structure equation model testing: Cutoff criteria for goodness of fit indices and chi-square test. Acta Psychol. Sin. 36, 186–194.
West, T. O., and Post, W. M. (2002). Soil organic carbon sequestration rates by tillage and crop rotation a global data analysis. Soil Sci. Soc. Am. J. 66, 1930–1946. doi:10.2136/sssaj2002.1930
West, T. O., and Six, J. (2007). Considering the influence of sequestration duration and carbon saturation on estimates of soil carbon capacity. Clim. Change. 80, 25–41. doi:10.1007/s10584-006-9173-8
Wiesmeier, M., Urbanski, L., Hobley, E., Lang, B., von Lützow, M., Marin-Spiotta, E., et al. (2019). Soil organic carbon storage as a key function of soils-A review of drivers and indicators at various scales. Geoderma 333, 149–162. doi:10.1016/j.geoderma.2018.07.026
Wissing, L., Kölbl, A., Häusler, W., Schad, P., Cao, Z. H., and Kögel-Knabner, I. (2013). Management-induced organic carbon accumulation in paddy soils: The role of organo-mineral associations. Soil till. Res. 126, 60–71. doi:10.1016/j.still.2012.08.004
Wissing, L., Kölbl, A., Schad, P., Bräuer, T., Cao, Z. H., and Kögel-Knabner, I. (2014). Organic carbon accumulation on soil mineral surfaces in paddy soils derived from tidal wetlands. Geoderma 228-229, 90–103. doi:10.1016/j.geoderma.2013.12.012
Xu, C. H., Xu, X., Ju, C. H., Chen, H. Y. H., Wilsey, B. J., Luo, Y. Q., et al. (2021). Long-term, amplified responses of soil organic carbon to nitrogen addition worldwide. Glob. Chang. Biol. 27, 1170–1180. doi:10.1111/gcb.15489
Xu, M. G., Liang, G. Q., and Zhang, F. D. (2006). Soil fertility evolution in China. Beijng, China: China Agricultural Science and Technology Press.
Yang, C. M., Yang, L. Z., and Ouyang, Z. (2005). Organic carbon and its fractions in paddy soil as affected by different nutrient and water regimes. Geoderma 124, 133–142. doi:10.1016/j.geoderma.2004.04.008
Yang, X., Shang, Q., Wu, P., Liu, J., Shen, Q., Guo, S., et al. (2010). Methane emissions from double rice agriculture under long-term fertilizing systems in Hunan, China. Agric. Ecosyst. Environ. 137, 308–316. doi:10.1016/j.agee.2010.03.001
Yu, Y., Guo, Z., Wu, H., Kahmann, J. A., and Oldfield, F. (2009). Spatial changes in soil organic carbon density and storage of cultivated soils in China from 1980 to 2000. Glob. Biogeochem. Cy. 23, GB2021. doi:10.1029/2008GB003428
Yue, J., Shi, Y., Liang, W., Wu, J., Wang, C. R., and Huang, G. H. (2005). Methane and nitrous oxide emissions from rice field and related microorganism in black soil, northeastern China. Nutr. Cycl. Agroecosyst. 73, 293–301. doi:10.1007/s10705-005-3815-5
Zhang, T., Huang, Y., and Yang, X. (2013). Climate warming over the past three decades has shortened rice growth duration in China and cultivar shifts have further accelerated the process for late rice. Glob. Chang. Biol. 19, 563–570. doi:10.1111/gcb.12057
Zhang, Y., Jiang, Y., Tai, A., Feng, J. F., Li, Z. J., Zhu, X. C., et al. (2019). Contribution of rice variety renewal and agronomic innovations to yield improvement and greenhouse gas mitigation in China. Environ. Res. Lett. 14, 114020. doi:10.1088/1748-9326/ab488d
Zhao, Y. C., Wang, M. Y., Hu, S. J., Zhang, X. D., Ouyang, Z., Zhang, G. L., et al. (2018). Economics- and policy-driven organic carbon input enhancement dominates soil organic carbon accumulation in Chinese croplands. PNAS 115, 4045–4050. doi:10.1073/pnas.1700292114
Zhou, W., Lv, T. F., Chen, Y., Westby, A. P., and Ren, W. J. (2014). Soil physicochemical and biological properties of paddy-upland rotation: A review. Sci. World J. 2014, 1–8. doi:10.1155/2014/856352
Keywords: crop rotation, fertilization, soil organic carbon, paddy soils, the Yangtze River catchment
Citation: Wang S, Sun N, Liang S, Zhang S, Meersmans J, Colinet G, Xu M and Wu L (2023) SOC sequestration affected by fertilization in rice-based cropping systems over the last four decades. Front. Environ. Sci. 11:1152439. doi: 10.3389/fenvs.2023.1152439
Received: 27 January 2023; Accepted: 18 May 2023;
Published: 30 May 2023.
Edited by:
Jehangir Bhadha, University of Florida, United StatesReviewed by:
Amit Anil Shahane, Central Agricultural University, IndiaCopyright © 2023 Wang, Sun, Liang, Zhang, Meersmans, Colinet, Xu and Wu. This is an open-access article distributed under the terms of the Creative Commons Attribution License (CC BY). The use, distribution or reproduction in other forums is permitted, provided the original author(s) and the copyright owner(s) are credited and that the original publication in this journal is cited, in accordance with accepted academic practice. No use, distribution or reproduction is permitted which does not comply with these terms.
*Correspondence: Nan Sun, c3VubmFuQGNhYXMuY24=; Shuxiang Zhang, emhhbmdzaHV4aWFuZ0BjYWFzLmNu
Disclaimer: All claims expressed in this article are solely those of the authors and do not necessarily represent those of their affiliated organizations, or those of the publisher, the editors and the reviewers. Any product that may be evaluated in this article or claim that may be made by its manufacturer is not guaranteed or endorsed by the publisher.
Research integrity at Frontiers
Learn more about the work of our research integrity team to safeguard the quality of each article we publish.