- 1Department of Soil Science, Faculty of Organic Agricultural Sciences, University of Kassel, Witzenhausen, Germany
- 2Department of Agricultural and Biosystems Engineering, Faculty of Organic Agricultural Sciences, University of Kassel, Witzenhausen, Germany
Technosols are characterized by a substantial quantity of artifacts or industrial wastes in the upper 100 cm. They can be used as cover caps in mining rehabilitation, in which vegetation cover is subsequently established. However, prolific vegetation growth can be limited by the chemical and physical properties of technogenic substrates. As further studies need to be conducted to evaluate the suitability of municipal solid waste incineration bottom ash and coal combustion residues as cover caps, the pH, electrical conductivity, total porosity, and bulk density of these substrates were measured. Furthermore, the seepage pH and electrical conductivity of the covered and uncovered technosols after saturation were determined. The municipal solid waste incineration bottom ash, 4–10 mm in diameter, showed the highest bulk density (1.16 g cm-3) and lowest total porosity by saturation (24.7%). In contrast, the coal combustion residues registered the highest total porosity (57.2%). The coal combustion residues also revealed a higher pH of 12.5 and electrical conductivity values of 7.8 mS cm-1. Furthermore, no differences were observed between the treatments for pH seepage (8.2–8.3) and its electrical conductivity (14.3–16.0 mS cm-1) in the covered and uncovered columns, when using a technosol. This study provides information on the use of municipal solid waste incineration bottom ash and coal combustion residues as evapotranspiration covers during mining rehabilitation. The management of municipal solid waste residues is a global challenge and the use of this resource is valuable not only for the mining industry but potentially also in other fields, such as construction. The heavy metal content of the bottom ash from municipal solid waste incineration and coal combustion residues should be studied before implementing these waste residues on a large scale.
Highlights
- The seepage pH and electrical conductivity were evaluated from a technosol.
- The pH ranged from 8.2 to 8.3, and salt solubility ranged from 14.3 to 16.0 mS cm-1.
- Coal combustion residues increased the total porosity of the technogenic substrates.
- Heavy metal content should be evaluated before using technosols.
1 Introduction
Mining was one of the first economic activities and, to the present day, continues to supply many basic resources used to sustain human life (Hartman and Mutmansky, 2002). Mining is defined as the extraction of any solid, liquid, or gaseous substance from the earth for utilitarian purposes (Hartman and Mutmansky, 2002). Minerals are used in construction, as well as automobile, airplane, and electronic manufacturing (Hartman and Mutmansky, 2002). Mining is also closely related to agriculture, providing materials for the fertilizers used to support crop growth and, animal feeding, as well as the metals and fuel required for machinery. Mining activities contribute to economic development and the creation of wealth (Paredes, 2016), jobs (Wessman et al., 2014), and infrastructure (Hartman and Mutmansky, 2002).
However, several significant side effects of the mining industry have been documented, including soil and water pollution (Kwon et al., 2017), soil nutrient depletion (Arshi, 2017), changes in the hydrological cycle (Lima et al., 2016), competition for land (Hilson and Laing, 2017), and loss of productive areas (Moomen, 2017).
Mining activities are also associated with the generation of millions of tons of waste and overburden materials, which are often landfilled around processing facilities and subsequently exposed to environmental conditions such as precipitation and wind (Lei et al., 2016; Arshi, 2017; Espinoza and Morris, 2017). Overburden materials originate from the extraction of the surface soil layer to gain access to the mineral deposits beneath (Arshi, 2017), and waste residues are created as by-products due to the low concentrations of target minerals in ore deposits (Espinoza and Morris, 2017). For example, the mine residues from potash mining can reach 75% of the mined volume (Podlacha, 1999). Copper is mined from ores containing a maximum of 0.8% copper (8,000 g/Mg), and gold is mined from ores with gold concentrations of only 0.0001% (1 g/Mg) (Espinoza and Morris, 2017). Overburden materials and waste residues are the most notably barriers to the sustainability of mining sites (Cooke and Johnson, 2002; Espinoza and Morris, 2017; Kwon et al., 2017). Furthermore, pollutants can be transferred from mining residues to nearby soils and waters through mine drainage and windblown dust (Kwon et al., 2017).
Since the 1980s, the University of Kassel has been working on the greening of potash tailings piles (Schmeisky and Hofmann, 2000), which are piles of solid residues from potash mining (Rauche, 2015). The volume of solid and liquid waste also depends on the potash rock. Sylvinite rock, a mixture of sylvite and halite, is the most concentrated potash ore, with 10%–35% potassium oxide and carnallite, 10%–16% potassium oxide (Warren, 2006).
Solid wastes, i.e., tailings, are deposited around mining facilities and exposed to precipitation erosion, whereas liquid residues are injected underground or released into surface water courses (Rauche, 2015). Several studies have been conducted to reduce brine production from potash tailings piles (Podlacha, 1999; Rauche and Fulda, 2001; Scheer, 2001; Niessing, 2005), and included the use of static layers such as synthetic mats and concrete (Schmeisky and Hofmann, 2000) and non-static covers, such as residue of aluminium recycling (Niessing, 2005). Currently, the focus has been on revegetation through an evapotranspiration cover made of municipal solid waste incineration bottom ash and coal combustion residues (CCRs), due to their high availability and affordability (Bilibio et al., 2017a; 2021a; 2021b; 2022; Bilibio and Hensel, 2021). Germany produces 49.570 million tons of municipal solid waste annually (Nelles et al., 2016), which must be recycled, composted, or incinerated. Thermal treatment reduces 90% of its waste volume (Sabbas et al., 2003). The main product of municipal solid waste (MSW) incineration is bottom ash residue, 80% (Inkaew et al., 2016), which is used in construction, roads, and landfills (Holm and Simon, 2017). The availability of CCRs in Germany is also high, comprising 22 million tons annually, which is primarily used in construction and refilling mining voids (Feuerborn et al., 2012). In Germany, coal combustion is performed to produce electricity (Heinrichs et al., 2017).
An evapotranspiration cover includes the use of a soil layer covered with native grasses (Hauser, 2009). The soil works as a water reservoir and the evaporation from it and transpiration of the vegetation empties this reservoir, effectively decreasing water infiltration into the waste (Hauser, 2009). As further studies are needed to evaluate the properties of cover caps for potash tailings, the objective of this study was to measure the physical and chemical properties of technogenic substrates made of MSW incineration bottom ash and CCRs. Moreover, the seepage properties of the technogenic substrates were assessed during 25 weeks using a column leaching experiment. This study provides information on the use of MSW incineration bottom ash and CCRs as evapotranspiration covers during mining rehabilitation. The management of MSW residues is a challenge globally and the use of this resource carries value not only for the mining industry but potentially in other fields such as construction too.
2 Materials and methods
2.1 Experimental site
The technogenic substrates were evaluated in the laboratory of the Department of Agricultural and Biosystems Engineering, and the column leaching experiment was conducted in a greenhouse at the University of Kassel, Witzenhausen. The greenhouse is located at 51° 20′59.5428″North and 9° 51′21.888″East.
According to the Köppen-Geiger classification, the climate in Witzenhausen is classified as Cfb (temperate, no dry season, warm summer, and 4 months over 10 C) (Peel et al., 2007), with a mean annual air temperature of 9.0°C and an annual precipitation of 810 mm (Merkel, 2023). The lowest mean air temperature in Witzenhausen was registered in January (0.5°C), and the mean maximum temperature was recorded in July, 17.9°C (Merkel, 2023).
2.2 Water content, total porosity and bulk density of different technogenic substrates
The water content, total porosity and bulk density of different mixtures of municipal solid waste incineration bottom ash (from the FES Frankfurter Entsorgungs - und Service GmbH–FES, Frankfurt, Germany) and CCR (from the Gesellschaft für die Aufbereitung und Verwertung von Reststoffen mbH–GFR, Würzburg, Germany) were determined.
The water content was evaluated by drying 20 g of each substrate at 105 C for 24 h. Three repetitions were performed for each substrate, for 30 samples. The water content was then determined using the following formula (Lal and Shukla, 2004; Blume et al., 2016; Hartge and Horn, 2016):
where θm is the water content (g g-1), Mw is the mass of water (g), and Ms is the mass of the dry substrate (g).
The total porosity of the substrates was assessed by placing them in stainless steel rings (approximately 100 cm3) under 4 kg cm-2 of pressure at the beginning, middle, and end of the filling (Figure 1). The samples were then saturated for 48 h and placed in an oven for drying at 105 C for 24 h. Five repetitions per substrate were performed for 50 samples.
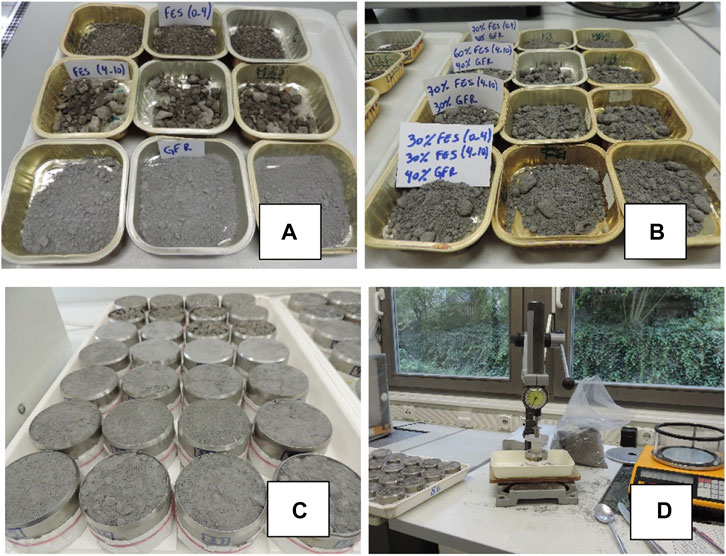
FIGURE 1. (A) and (B) Aluminum containers with substrates to determine water content; (C) equipment used for packing the substrates with metal rings; (D) undisturbed samples to measure total porosity and bulk density.
Total porosity (f) was determined using the following formula (Lal and Shukla, 2004; Blume et al., 2016; Hartge and Horn, 2016):
where Vf is the pore volume, Vt is the total volume, Va is the air volume, Vw is the water volume, and Vs is the solid volume.
The bulk densities of the technogenic substrates were calculated using Equation 3 (Lal and Shukla, 2004; Blume et al., 2016; Hartge and Horn, 2016):
where ρb is the bulk density (g cm-3), Ms is the dry sample weight (g), and Vt is the cylinder volume (cm3).
Table 1 shows the single technogenic substrates and substrates mixtures used to determine the total porosity, bulk density and water content. The municipal solid waste incineration bottom ash considered two diameters (0–4 mm; 4–10 mm) to verify if the different particle sizes affects the total porosity and bulk density of the constructed technosol. The different portions of substrates were measured by volume in a 1 L measuring jug.
2.3 Column leaching experiment
A column leaching experiment was installed in a greenhouse at the University of Kassel, Witzenhausen, to evaluate the seepage pH and electrical conductivity of a technosol made of MSW incineration bottom ash and coal combustion residue. The experiment was conducted in November 2014, and seepage measurements were conducted weekly up to May 2015 (25 weeks in total). In addition, the volume reduction, pH, and electrical conductivity of the substrates used to fill the columns were evaluated in November 2014.
2.3.1 Experimental design
A mixture of different single substrates was used: 32.5% MSW incineration bottom ash, 0–4 mm diameter; 32.5% MSW incineration bottom ash, 4–10 mm diameter; and 35% CCR. This mixture was chosen to include so much MSW incineration bottom ashes as possible due to its large availability in Germany. Furthermore, 628 g of compost column−1 (approximately 200 t ha-1) was mixed by hand with the substrate on the column surface (from 0.0 to 20 cm depth). The use of compost of soil surface increases the availably of nutrients to crops and improve the physical properties of the substrates, such as aggregate stability.
Two treatments were considered in a randomized design: covered columns (1a, 1b, 1c) and uncovered columns (2a, 2b, 2c) (Figure 2A). These treatments were considered to verify whether the application of compost and the coverage of the columns interferes with the pH levels of the seepage. Bilibio et al. (2017b) measured pH values lower than 5 after saturation of lysimeters filled with technogenic substrates made of MSW incineration bottom ash and CCRs from 26 July to 27 December 2013 in Germany. The coverage of lysimeters in lysimeter experiments is usually performed after saturation to reach field capacity and to start water balance assessments. The experiment was repeated three times for six experimental units.
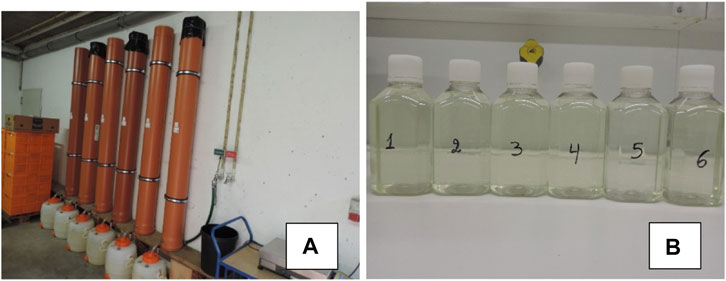
FIGURE 2. (A) Covered and uncovered columns; (B) Sampling of columns’ drainage to determine their pH value and electrical conductivity.
2.3.2 Column layers
Polyvinyl chloride columns were 200 cm high and 20 cm wide, for a volume of 62,831.8 cm3. The layers of the columns were divided as stated in Table 2.
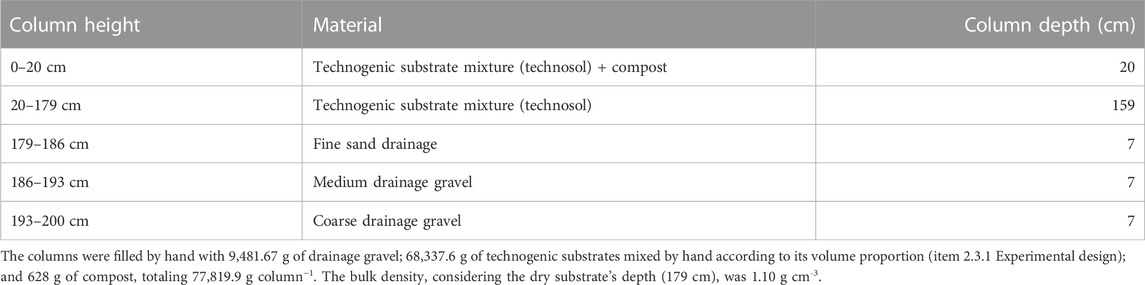
TABLE 2. Composition of the materials used in the column leaching experiment, with layers arranged from top to bottom.
2.3.3 Water content, pH, electrical conductivity, and volume reduction of the substrates
The water content of the substrates was determined using 50 g of each substrate, which was weighed, placed in aluminum containers (Figure 3D), and dried at 105 C for 24 h. Five replicates were performed for each substrate, comprising 25 samples. The water content was determined using Equation 1.
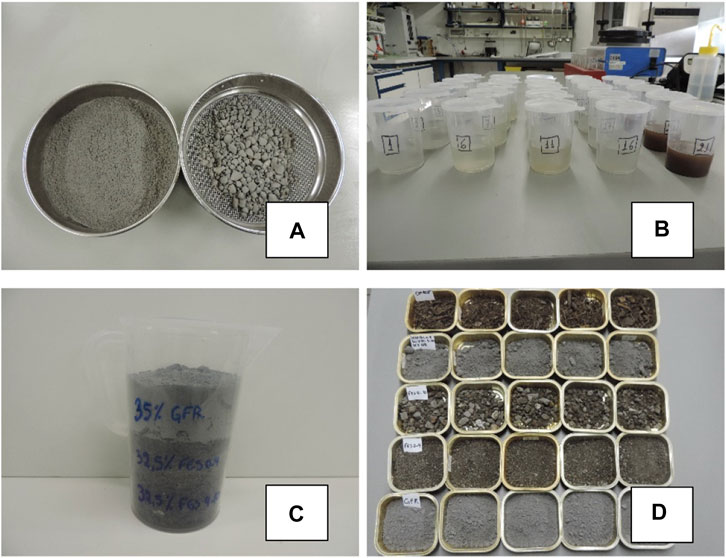
FIGURE 3. (A) Substrates sieved with a 2 mm diameter steel sieve; (B) Substrate solutions to determine electrical conductivity; (C) Measuring jug to study volume reduction of substrates; (D) Aluminum containers to determine the substrates’ moisture content.
Electrical conductivity and pH levels were verified according to the German Institute for Standardization (DIN ISO 10390 (2005); DIN ISO 11265 (1997)). For pH measurements, 20 g of each sieved substrate (2 mm in diameter; Figure 3A) was weighed and placed in plastic containers. Subsequently, 100 mL of calcium chloride solution (0.01 mol L-1) was added to each numbered container to obtain a homogenized suspension. The containers were then stirred for 60 min using a Köttermann electric stirrer (Uetze, Germany). Finally, a pH electrode (BlueLine 26 pH NTC 10, SI Analytics, Munich, Germany; Sartorius portable meter PT-10, Goettingen, Germany) was dipped in the homogenized suspension for pH reading.
For electrical conductivity, 100 mL of distilled water was added to each plastic container with 20 g of sieved substrate to obtain a homogenized suspension. The containers were then stirred for 30 min using a Köttermann electric stirrer. Finally, the suspension was filtered through a folded filter paper (Schleicher and Schüll 595 1/2; retention range of 4–7 µm), as in Figure 3B, and electrical conductivity was evaluated using a conductivity meter (WTW Pocket Meter Multi 340i).
Volume reduction was studied by shaking each substrate 100 times in a 1 L measuring jug (Figure 3C). The volume reduction of substrates in the field as potash tailings piles can be because of transport, mixing, application, and precipitation. This information is important to determine the potential decrease in depth of technogenic substrates over time in potash tailings piles.
2.3.4 Saturation
The saturation of the columns was conducted to reach the field capacity and start the soil water balance. The saturation volume of the substrates was estimated using the measured (Eq. 4) and estimated total porosities of the substrates (Equations 5 and 6) (Lal and Shukla, 2004).
where θS is the saturation water content,
After initial saturation, the columns were covered with plastic sheeting for 7 weeks (51 days). The pH and electrical conductivity (µS/cm) of the precipitation water used to saturate the columns were assessed before irrigation.
2.3.5 Irrigation
Columns were manually irrigated with a graduated cylinder from January to May 2015 (18 weeks), according to the weekly precipitation recorded by 1-m height gauges located at an experimental site in Heringen, Germany (Bilibio et al., 2017a). The Heringen experimental site also evaluated the use of municipal solid waste incineration bottom ash and CCRs (Bilibio et al., 2017a).
2.3.6 Drainage
The drainage volume was collected in 20-L barrels and measured weekly on Thursdays, around 17:00. After measuring the drainage volume using a graduated cylinder, the pH, and EC of the seepage were assessed. The pH values were measured using a Sartorius portable meter and BlueLine 26 pH Electrode (SI Analytics). Electrical conductivity was measured using a conductivity meter (WTW Pocket Meter Multi 340i).
2.3.7 Water balance
To calculate the column water balance, irrigation was used as the incoming water flux and column drainage as the outgoing water flux. Evaporation was determined by a simplified water balance expression (Aboukhaled et al., 1982):
where E is evaporation (mm), I is irrigation (mm), and D is drainage (mm).
Water balance was evaluated from January 2015, when irrigation started, to May 2015, when the experiment was completed (18 weeks).
2.3.8 Meteorological data and statistical analysis
Environmental conditions such as temperature and relative humidity were recorded using a mini data logger located inside the greenhouse from November 2014 to May 2015. The statistical analysis concerning the technogenic substrates, was performed by analysis of variance and Tukey’s Honest Significant Difference (HSD), where population and residuals had normal distribution and variances were considered homogeneous. We used Levene’s test to evaluate the homogeneity of variance (p > 0.05), while normality of data and residuals were assessed using the Shapiro Wilk test (p > 0.05). Moreover, the Welch-Anova test was utilized when groups showed normal distribution but unequal variances, and the Kruskall-Wallis test when population and residuals were non-normally distributed. For the column leaching experiment, statistical analysis of the drainage amount, drainage pH and drainage EC between the two treatments (covered and uncovered columns) was performed using the paired t-test (t), where differences of data pairs showed normal distribution. Normality of paired differences was assessed through the Shapiro-Wilk test (p > 0.05) and by graphic visualization using the Quantile-Comparison Plot. When differences between data were non-normally distributed, we used the Wilcoxon signed-rank test. Descriptive statistics were used to summarize the data. The central tendency of the data was studied using mean values, whereas the variability of the mean was determined based on the standard deviation and the coefficient of variation (Field et al., 2012; Couto et al., 2013; Crawley, 2014). Statistical analysis was performed with Rstudio, version 1.3.959 (RStudio Team, 2020).
3 Results
3.1 Water content, total porosity and bulk density of different technogenic substrates
The substrate analysis included measurements of the initial water content, total porosity by saturation, and bulk density of the 10 technogenic substrates (Table 1). The initial water content of the substrates ranged from 0.41% in the CCRs to 21.6% in the MSW incineration bottom ash (0–4 mm diameter). The initial water content of the substrate mixtures ranged from 6.3% in treatment 10 [25% MSW (0–4 mm) + 25% MSW (4–10 mm) + 50% CCR] to 14.2% in treatment 5 [70% MSW (0–4 mm) + 30% CCR], which had the highest MSW incineration bottom ash proportion. The analysis of variance showed significant differences among the treatments for the water content (p < 0.001), data not shown.
Regarding the total porosity, the minimum value was found in the MSW (4–10 mm diameter) at 24.7%, whereas the highest was registered in the CCRs (57.2%) (Figure 4). Considering the substrate mixtures, lower values were verified for the substrates made of MSW (4–10 mm diameter) in treatments 6 (38.9%) and 7 (32.8%), and higher total porosity was found for the substrates made of MSW (0–4 mm diameter) and CCRs (treatment 4: 48.4%; treatment 5: 46.4%).
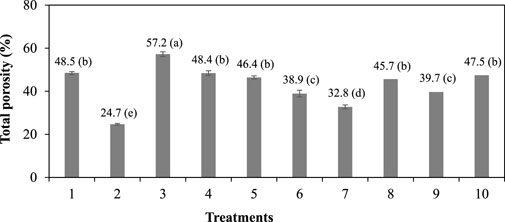
FIGURE 4. Total porosity (n = 5) of each of the tested substrates. Values are presented as means ± standard deviation. For the treatments refer to Table 1. (A), (B), (C), (D), and (E) significant differences according to the ANOVA and Tukey-test (p < 0.001).
Further analysis about the bulk density revealed values ranging from 0.96 g cm-3 in the MSW (0–4 mm diameter) (treatment 1) to 1.16 g cm-3 in the MSW (4–10 mm diameter) (treatment 2), Figure 5. Higher values were found for substrates with a higher portion of MSW (4–10 mm diameter), that is, treatment 6 (60% MSW (4–10 mm) + 40% CCR) with 1.14 g cm-3 and treatment 7 (70% MSW (4–10 mm) + 30% CCR) with 1.12 g cm-3, and those with an equal distribution of the single substrates, such as treatment 9 (35% MSW (0–4 mm) + 35% MSW (4–10 mm) + 30% CCR), with 1.13 g cm-3.
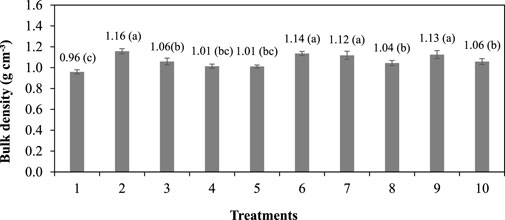
FIGURE 5. Bulk density (n = 5) of each of the tested substrates. Values are presented as means ± standard deviation. For the treatments refer to Table 1. (A), (B), and (C) significant differences according to the ANOVA and Tukey-test (p < 0.001).
3.2 Column leaching experiment
3.2.1 Substrate analysis
The water content of the substrates evaluated before filling the columns was like that previously evaluated. The lowest water content was found in the CCRs (0.5%) and the highest in the municipal solid waste incineration bottom ash (0–4 mm diameter) (19.4%). According to the analysis of variance there were significant differences among treatments for the water content (p < 0.001).
pH of the substrates ranged from 7.9 at the MSW (4–10 mm diameter) to 12.5 in the CCR. The pH of the technogenic substrate mixture (technosol) was 11.3, and that of the compost was 8.0. Soluble salts (EC) were higher in CCRs (7.8 mS cm-1) and lower in the technogenic substrate mixture, technosol, and compost (2.5 mS cm-1). According to the analysis of variance there were significant differences among treatments for the pH and electrical conductivity (p < 0.001), Figure 6. The highest volume reduction was found in the substrate mixture (31.2%) and the lowest in the MSW (4–10 mm diameter) (13.3%) (Figure 6). According to the Kruskal-Wallis test there were significant differences among treatments for the volume reduction (p < 0.001), where the volume reduction of CCRs (19.9%) was significantly higher than the MSW (4–10 mm diameter), 13.3%. Additionally, the volume reduction MSW (0–4 mm diameter), 18.5%, was significantly higher than the MSW (4–10 mm diameter), and significantly lower than the compost (20.1%). At last, the volume reduction of the MSW (4–10 mm diameter), was significantly lower than the technosol (31.2%) and the compost.
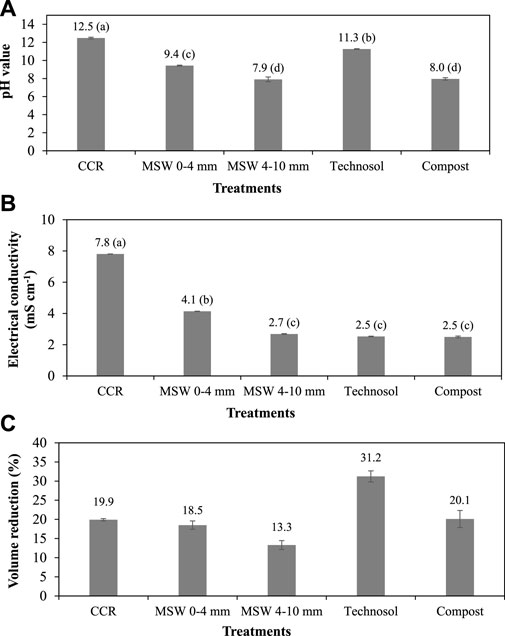
FIGURE 6. pH values (pH; n = 5) (A), electrical conductivity (EC; n = 5) (B), and volume reduction (VR; n = 10) (C) of the tested substrates. Values are presented as means ± standard deviation. (A), (B), (C) and (D) significant differences according to the ANOVA and Tukey-test (p < 0.001).
3.2.2 Environment
Table 3 presents the minimum, mean, and maximum air temperatures and relative humidity monitored within the greenhouse with a mini data logger. Lower temperatures were observed during the winter months, from December to February. The temperature ranged from 15.7°C to 16.7°C. The relative humidity ranged from 62.4% to 68.5%, with a mean value of 65.5% (Table 4).
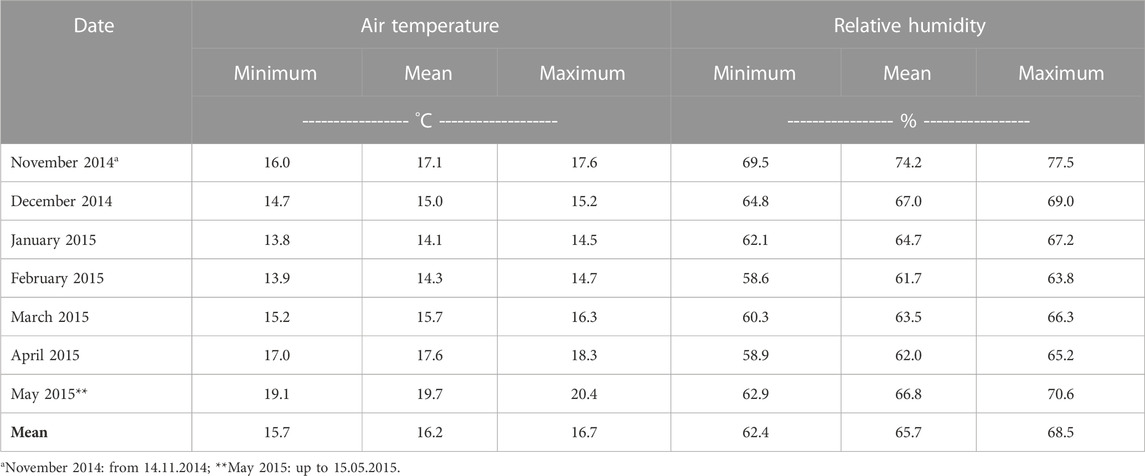
TABLE 3. Monthly air temperature and relative humidity within the greenhouse from 14.11.2014 to 15.05.2015.
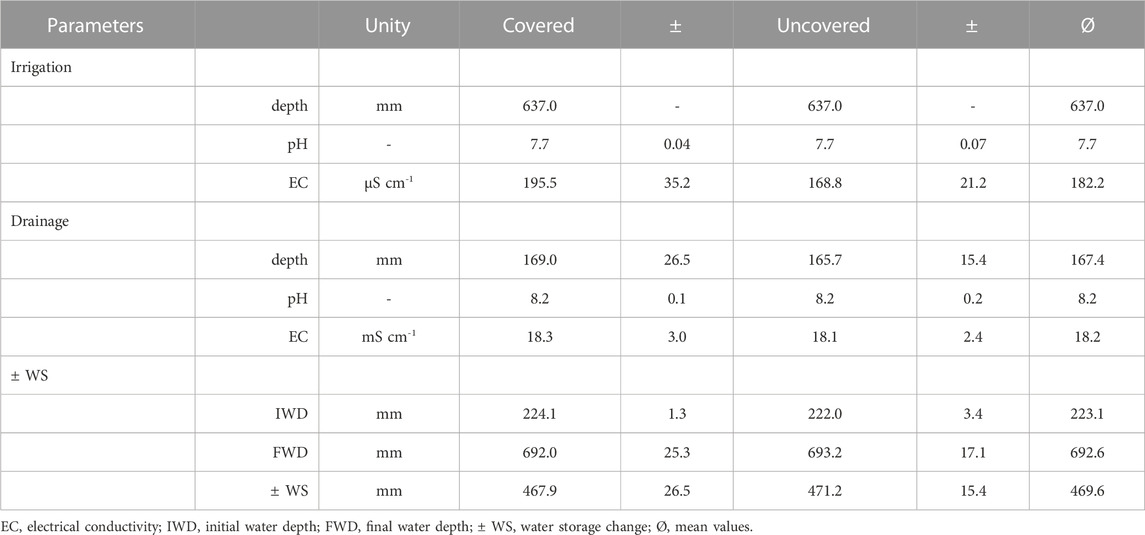
TABLE 4. Saturation of the covered and uncovered columns from 14.11.2015 to 14.01.2015. Values are presented as means ± standard deviation.
3.2.3 Water balance
The saturation of the columns was completed by applying 20 L, 637 mm, of precipitation water to each column. The mean pH of the precipitation was 7.7, and its average electrical conductivity (EC) was 182.2 μS cm-1.
After saturation, 167.4 mm of drainage was observed, on average. The pH of the drainage was similar between the covered and uncovered columns (8.2). Similarly, the EC of the seepage ranged from 18.1 mS cm-1 in the uncovered columns to 18.3 mS cm-1 in the covered columns. Overall, the substrates moved from an initial water depth of 223.1 mm to a final water depth of 692.6 mm, increasing the storage moisture by 469.6 mm (Table 4).
The irrigation applied after the columns were opened from 15.01.2015 to 14.05.2015 totaled 139.4 mm. The pH of the precipitate was on average 8.2 and the EC 290.4 μS cm-1.
Drainage from 15.01.2015 to 14.05.2015 ranged from 71.0 mm in the uncovered columns to 89.9 mm in the covered columns. A low variation between treatments was found for the pH (mean 8.3) and EC (mean 15.2 mS cm-1) (Table 5). The evaporation of the covered columns (49.4 mm) was lower than the uncovered columns (68.4 mm), which may be due to surface exposure of the substrates to the environment during the initial 7 experimental weeks (51 days). According to the paired t-test there were significant differences between the covered and uncovered columns only for drainage amount and evaporation (amount and rate), p < 0.05.
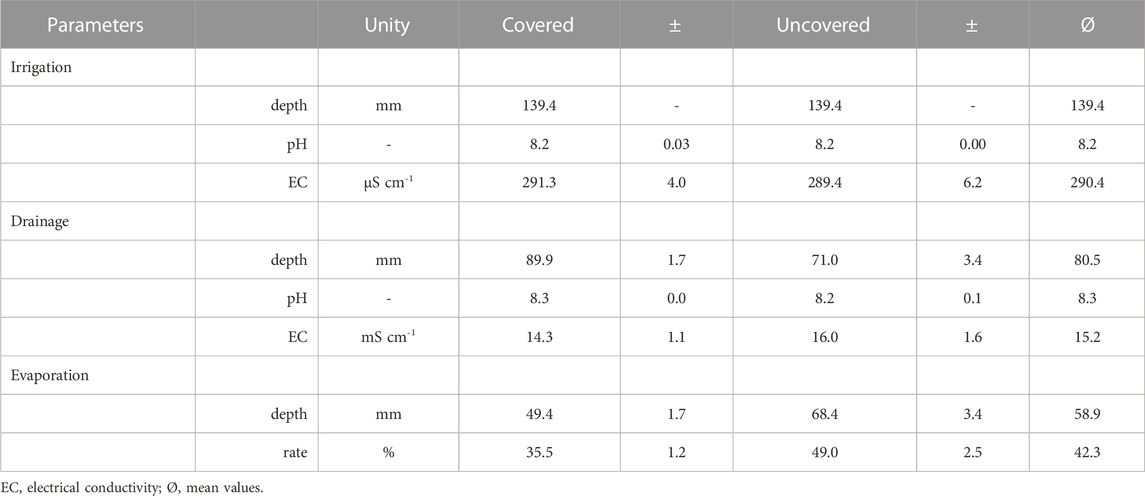
TABLE 5 . Water balance and drainage properties of the covered and uncovered columns from 15.01.2015 to 14.05.2015. Values are presented as means ± standard deviation.
4 Discussion
4.1 Substrate analyses
Total porosity represents the soil volume available for the movement of water, air, and nutrients (Indoria et al., 2017). Total porosity values lower than 30% are considered very low, and those higher than 54% are considered very high (AG Boden, 2005). In the current study, the highest total porosity was found for CCR (57.2%) and the lowest for the MSW incineration bottom ash (4–10 mm) (24.7%). In addition, the total porosity measured in technogenic substrate mixtures (from 32.8% to 48.4%) are between mineral soils (from 30% to 56%, Blume et al., 2016), and total porosity increased with increasing proportion of CCR, owing to its low silt-sand particle sizes (Niessing, 2005). The results of the current study agree with the findings of Bilibio et al. (2022), who estimated 52.8% of the total porosity using bulk and particle density, and measured 48.6% of the total porosity by saturating the undisturbed samples for different technosols in a lysimeter experiment in Germany. Higher total porosities for technogenic substrates made of fine granular saline aluminum recycling by-products and CCRs were estimated by Hermsmeyer (2001), Hermsmeyer et al. (2002) using particle and bulk density values. The authors calculated a total porosity of 63%–75.7%. Kaczmarek et al. (2021) calculated a total porosity ranging from 62.20% to 63.68% in the surface of technosols made of fly ash from lignite power plant in Poland. The total porosity of technosols for evapotranspiration cover is important because it represents the volume available for water infiltration and storage (Hermsmeyer, 2001).
The bulk density shows soil association between the mass and volume and affects several soil measurements, such as infiltration, available water capacity, soil porosity, and soil root restrictions of soils (Indoria et al., 2020). The highest bulk density was observed for MSW incineration bottom ash (4–10 mm), 1.16 g cm-3, and a lower value for MSW incineration bottom ash (0–4 mm), (0.96 g cm-3). Bulk densities of mineral soils range from very low (<1.2 g cm-3) to very high (>1.8 g cm-3) (AG Boden, 2005). In mineral soils, the bulk density threshold limits for root and plant growth are 1.4 g cm-3 for clayey soils, 1.6 g cm-3 for silty soils, and 1.8 g cm-3 for sandy soils (Blanco-Canqui and Ruis, 2018). The bulk densities measured in this study complement the findings of Bilibio et al. (2022), who verified a bulk density ranging from 1.17 to 1.25 g cm-3 in four technogenic substrates for evapotranspiration covers in Germany. In contrast, Hermsmeyer (2001), Hermsmeyer et al. (2002) measured considerably lower bulk densities ranging from 0.56 to 0.93 g cm-3 for technogenic substrates for potash tailings covers, also in Germany. Low bulk densities were also measured in technosols made of fly ashes by Kaczmarek et al. (2021), which varied from 0.76 to 0.80 g cm−³ in the upper horizons of soil profiles.
4.2 Column leaching experiment
The pH is an important parameter for evaluating the soil’s suitability for vegetation growth, as a habitat for soil organisms, and as a filter of pollutants (Blume et al., 2016). Furthermore, EC indirectly evaluates dissolved salts within the solution (Howard, 2017). All pH values for the technogenic substrates used in the column leaching were in the alkaline range. The pH of the substrates ranged from 7.9 for the MSW incineration bottom ash (4–10 mm) to 12.5 for the CCR, with EC ranging from 2.7 to 7.8 mS cm-1, respectively. However, when technogenic substrates were mixed (35% MSW (0–4 mm) + 35% MSW (4–10 mm) + 30% CCR), the pH was 11.3, and the EC decreased to 2.5 mS cm-1. The pH values were higher than those suggested for cultivated soils, which ranged from 5 to 6.5 (Blume et al., 2016). The pH values of the cultivated soils should be higher than 5 to avoid Al and Mn toxicity (Blume et al., 2016). EC values for non-saline soils range from to 0–2 mS cm-1, whereas it is greater than 32 mS cm-1 for extremely saline soils (Simons and Bennett, 2022). A high EC may indicate problems associated with water absorption and toxicity to plants. Bilibio et al. (2022) measured a pH ranging from 8.4 to 8.7 and EC ranging from 2.6 to 3.5 mS cm-1 in technosols installed in a lysimeter experiment in Heringen, Germany.
The high pH and EC of the technogenic substrates were reflected in the respective values of the drainage water after saturation (8.2 and 18.2 mS cm-1, respectively) and during the irrigation treatments (8.3 and 15.2 mS cm-1, respectively). The pH of the drainage water was within the range of the recommended values for drinking water in Germany, i.e., 6.5 to 9.5 (Deutsche Verein des Gas- und Wasserfaches, 2023). However, its EC was higher than that recommended for drinking water in Germany, 2.79 mS cm-1 (Deutsche Verein des Gas- und Wasserfaches, 2023). For comparison, the EC of oceans is 56 mS cm-1, and that of the rainwater used to irrigate the column leaching experiment was 290.4 μS cm-1. The pH values found in this study approximated the values measured by Bilibio et al. (2021b) for the drainage water of different technogenic substrates after three experimental years in a lysimeter experiment (7.2). However, the low pH measured in the same study (Bilibio et al., 2021b) in the first experimental year after saturation (average 5.3) was not confirmed in the current column leaching experiment. The EC measured in the column leaching experiment was lower than that measured by Bilibio et al. (2021b) in 2014 (39.5 mS cm-1), 2015 (25.1 mS cm-1), and 2016 (21.8 mS cm-1). The reasons for these differences may include the size of the lysimeters used in the field experiment by Bilibio et al. (2021b) (6 m3) and the size of the column leaching experiment here, 0.06 m3. Further reasons for these differences are unknown.
Regarding the water balance, a high soil water storage of the substrates was found, 435 mm m-1 (AG Boden, 2005) and an evaporation rate of 42.3%, which is higher than that suggested for potash tailings (10%). However, the evaporation rate was lower than the evapotranspiration rate measured in an experiment conducted in Germany, which ranged from 66.4% to 68% (Bilibio et al., 2021b). Bilibio et al. (2021a) also simulated an actual evapotranspiration of 76.3% for a potash tailings cover, considering 27 hydrological years for Bad Hersfeld in Germany. Scheer (2001) measured drainage water lower than 50% of the precipitation in a lysimeter experiment near to Hannover, Germany, using slags from secondary aluminium industry and coal combustion residues. Hermsmeyer (2001) measured also drainage rates lower than 50% of the precipitation in a lysimeter experiment at the Sigmundshall potash mine area near to Hannover. This is because the greenhouse experiment was conducted without vegetation. Vegetation increases the evapotranspiration rate, and exudates of the roots could interfere with the seepage pH levels. Moreover, vegetation absorbs nutrients that may change the chemical composition of the seepage. It must be considered that different environments than that registered in the greenhouse column leaching experiment (Table 3) with distinct precipitation characteristics, temperature, solar radiation, and relative humidity may contribute to distinct evaporation rates and seepage properties. Higher activities of microorganisms is expected at soil temperatures higher than 6 C (Näser and Niessing, 2020). The optimum air temperature varies according to each crop, for example, the optimum air temperature for wheat at the grain filling is 26 C (Khan et al., 2020) and for rice it is 25–35 C (Biswas et al., 2019).
5 Conclusion
The objective of this study was to verify the suitability of using technogenic substrates made of MSW incineration bottom ash and CCRs as cover caps in potash tailings piles by means of physical and chemical parameters. Moreover, the seepage properties of the technogenic substrates were assessed during 25 weeks using a column leaching experiment. No differences between the covered and uncovered columns for seepage pH and EC were verified, which indicates that covering the field lysimeter after saturation and the use of compost do not affect the pH and EC of the drainage water, as assumed at the beginning of the study. Furthermore, higher pH (12.5) and EC (7.8 mS cm-1) values were found for the CCR, which are higher than the recommended values for cultivated soils. However, this artifact may enhance the water holding capacity of the substrates, owing to its smaller particle size and larger surface area and total porosity. For the technogenic substrate mixtures such as 35% MSW (0–4 mm) + 35% MSW (4–10 mm) + 30% CCR, the EC decreased to non-saline soil levels, demonstrating the suitability of the technogenic substrate for use as an evapotranspiration cover in potash tailings piles. Further studies should consider the heavy metal content of the CCR and MSW incineration bottom ash, although previous measurements showed low levels of these components in the drainage water.
Data availability statement
The original contributions presented in the study are included in the article/supplementary material, further inquiries can be directed to the corresponding author.
Author contributions
All authors listed have made a substantial, direct, and intellectual contribution to the work and approved it for publication.
Funding
This research was supported by K + S KALI GmbH (project number: 6525103).
Acknowledgments
The authors thank Professor Helge Schmeisky for the support in conducting the column leaching experiment.
Conflict of interest
The authors declare that this study received funding from K + S KALI GmbH. The funder was not involved in the study design, collection, analysis, interpretation of data, the writing of this article, or the decision to submit it for publication.
Publisher’s note
All claims expressed in this article are solely those of the authors and do not necessarily represent those of their affiliated organizations, or those of the publisher, the editors and the reviewers. Any product that may be evaluated in this article, or claim that may be made by its manufacturer, is not guaranteed or endorsed by the publisher.
References
Aboukhaled, A., Alfaro, A., and Smith, M. (1982). Lysimeters. FAO irrigation and drainage paper 39. Rome.
AG Boden, (2005). Bodenkundliche Kartieranleitung. 5. Auflage, Bundesanstalt für Geowissenschaften und Rohstoffe, Hannover.
Arshi, A. (2017). Reclamation of coalmine overburden dump through environmental friendly method. Saudi J. Biol. Sci. 24, 371–378. doi:10.1016/j.sjbs.2015.09.009
Bilibio, C., and Hensel, O. (2021). The water deficit of evapotranspiration covers on potash tailing piles using CropWat. Agric. Eng. Int. CIGR J. 23, 75–91. https://cigrjournal.org/index.php/Ejounral/article/view/6327.
Bilibio, C., Hensel, O., Uteau, D., and Peth, S. (2021a). Simulation of evapotranspiration and drainage from potash tailings covers using Hydrus-1D. Agric. Eng. Int. CIGR J. 23, 85–98. https://cigrjournal.org/index.php/Ejounral/article/view/6649.
Bilibio, C., Retz, S., Schellert, C., and Hensel, O. (2021b). Drainage properties of technosols made of municipal solid waste incineration bottom ash and coal combustion residues on potash-tailings piles: A lysimeter study. J. Clean. Prod. 279, 123442. doi:10.1016/j.jclepro.2020.123442
Bilibio, C., Schellert, C., Retz, S., Hensel, O., Schmeisky, H., Uteau, D., et al. (2017a). Water balance assessment of different substrates on potash tailings piles using non-weighable lysimeters. J. Environ. Manage. 196, 633–643. doi:10.1016/j.jenvman.2017.01.024
Bilibio, C., Schellert, C., Retz, S., and Hensel, O. (2017b). “Teilbericht B (Sickerwasser/Klima),” in Umweltsicherung Schmeisky, Universität Kassel, FB 11, Fachgebiet Agrartechnik im Auftrag von K+S KALI GmbH, unveröffentlicht (Hrsg.): Begrünungskonzept für Kalirückstandshalden im Werra-Gebiet. Stufe 2 -Feldversuch auf der Halde IV in Heringen: Endbericht (Witzenhausen: University of Kassel), 1–83.
Bilibio, C., Uteau, D., Peth, S., Retz, S., Schellert, C., and Hensel, O. (2022). Physical properties of technosols as evapotranspiration covers on potash tailings piles. J. Environ. Manage. 310, 114654. doi:10.1016/j.jenvman.2022.114654
Biswas, P. S., Rashid, M. M., Khatun, H., Yasmeen, R., and Biswas, J. K. (2019). “Scope and progress of rice research harnessing cold tolerance,” in Advances in rice research for abiotic stress tolerance. Editors M. M. Hasanuzzaman, N. K. Fujita, and J. K. Biswas (Amsterdam, Netherlands: Elsevier), 225–264. doi:10.1016/B978-0-12-814332-2.00011-3
Blanco-Canqui, H., and Ruis, S. J. (2018). No-tillage and soil physical environment. Geoderma 326, 164–200. doi:10.1016/j.geoderma.2018.03.011
Blume, H. P., Brümmer, G. W., Fleige, H., Horn, R., Kandeler, E., Kögel-Knabner, I., et al. (2016). Scheffer/schachtschabel soil science. 1 ed. Berlin, Germany: Springer.
Cooke, J. A., and Johnson, M. S. (2002). Ecological restoration of land with particular reference to the mining of metals and industrial minerals: A review of theory and practice. Environ. Rev. 10, 41–71. doi:10.1139/a01-014
Couto, M. F., Peternelli, L. A., and Barbosa, M. H. P. (2013). Classification of the coefficients of variation for sugarcane crops. Cienc. Rural. 43, 957–961. doi:10.1590/S0103-84782013000600003
Deutsche Verein des Gas- und Wasserfaches (2023). Trinkwasserverordnung 2022/2023 https://www.dvgw.de/themen/wasser/trinkwasserverordnung (accessed 01 07, 2023).
DIN ISO 11265 (1997). Soil quality. Determination of the specific electrical conductivity. Berlin: Beuth Verlag GmbH.
Espinoza, R. D., and Morris, J. W. F. (2017). Towards sustainable mining (part II). Accounting for mine reclamation and post reclamation care liabilities. Resour. Policy 52, 29–38. doi:10.1016/j.resourpol.2017.01.010
Feuerborn, H., Müller, B., and Walter, E. Use of calcareous fly ash in Germany,” in Proceedings of the EUROCOALASH 2012 Conference September 2012. (Thessaloniki, Greece.
Hartge, K.-H., and Horn, R. (2016). in Essential Soil Physics. An introduction to soil processes, functions, structure and mechanics. Editors R. Horten, J. Bachmann, R. Horn, and S. Peth (Stuttgart, Germany: Schweizerbart’sche Verlagsbuchhandlung). Available at: http://www.schweizerbart.de//publications/detail/isbn/9783510652808/Einfuhrung_in_die_Bodenphysik).
Hartman, H. L., and Mutmansky, J. M. (2002). Introductory mining engineering. 2nd ed. Hoboken, N.J., Chichester: J. Wiley.
Hauser, V. L. (2009). Evapotranspiration covers for landfills and waste sites. Boca Raton. Florida, USA: CRC Press.
Heinrichs, H. U., Schumann, D., Vögele, S., Biß, K. H., Shamon, H., Markewitz, P., et al. (2017). Integrated assessment of a phase-out of coal-fired power plants in Germany. Energy 126, 285–305. doi:10.1016/j.energy.2017.03.017
Hermsmeyer, D. (2001). Soil physical and hydrological evaluation of aluminum recycling by-product as an infiltration barrier for potash mine tailings (Doctoral Dissertation). Welfengarten, Germany: Hanover University.
Hermsmeyer, D., Diekmann, R., Van Der Ploeg, R. R., and Horton, R. (2002). Physical properties of a soil substitute derived from an aluminum recycling by-product. J. Hazard. Mater. 95, 107–124. doi:10.1016/S0304-3894(02)00087-0
Hilson, G., and Laing, T. (2017). Gold mining, indigenous land claims and conflict in Guyana's hinterland. J. Rural. Stud. 50, 172–187. doi:10.1016/j.jrurstud.2017.01.004
Holm, O., and Simon, F. (2017). Innovative treatment trains of bottom ash (BA) from municipal solid waste incineration (MSWI) in Germany. Waste Manage 59, 229–236. doi:10.1016/j.wasman.2016.09.004
Indoria, A. K., Rao, S., Sharma, K. L., and Sammi Reddy, K. (2017). Conservation agriculture—A panacea to improve soil physical health. Curr. Sci. 112, 52. doi:10.18520/cs/v112/i01/52-61
Indoria, A. K., Sharma, K. L., and Reddy, K. S. (2020). “Hydraulic properties of soil under warming climate,” in Majeti narasimha vara prasad (Hg.): Climate change and soil interactions (San Diego. Amsterdam, Netherlands: Elsevier), 473–508.
Inkaew, K., Saffarzadeh, A., and Shimaoka, T. (2016). Modeling the formation of the quench product in municipal solid waste incineration (MSWI) bottom ash. Waste manage. 52, 159–168. doi:10.1016/j.wasman.2016.03.019
Kaczmarek, Z., Mocek-Płóciniak, A., Gajewski, P., Mendyk, Ł., and Bocianowski, J. (2021). Physical and soil water properties of technosols developed from lignite fly ash. Arch. Environ. Prot. 47, 95–102. doi:10.24425/AEP.2021.137281
Khan, A., Ahmad, M., Ahmed, M., and Iftikhar Hussain, M. (2020). Rising atmospheric temperature impact on wheat and thermotolerance strategies. Plants (Basel, Switz. 10 (1), 43. doi:10.3390/plants10010043
Kwon, J. C., Nejad, Z. D., and Jung, M. C. (2017). Arsenic and heavy metals in paddy soil and polished rice contaminated by mining activities in Korea. CATENA 148, 92–100. doi:10.1016/j.catena.2016.01.005
Lei, K., Pan, H., and Lin, C. (2016). A landscape approach towards ecological restoration and sustainable development of mining areas. Ecol. Eng. 90, 320–325. doi:10.1016/j.ecoleng.2016.01.080
Lima, A. T., Mitchell, K., O’Connell, D. W., Verhoeven, J., and van Cappellen, P. (2016). The legacy of surface mining: Remediation, restoration, reclamation and rehabilitation. Environ. Sci. Policy 66, 227–233. doi:10.1016/j.envsci.2016.07.011
Merkel, A. (2023). Climate witzenhausen: Temperature, climate graph, climate table for witzenhausen - climate-data.org. Available at: https://en.climate-data.org/europe/germany/hesse/witzenhausen-19590/(accessed 01 12, 23).
Moomen, A. W. (2017). Strategies for managing large-scale mining sector land use conflicts in the global south. Resour. Policy 51, 85–93. doi:10.1016/j.resourpol.2016.11.010
Näser, D. (2020). Regenerative landwirtschaft. Stuttgart: Ulmer Verlag. 190 pp. Niessing, S. (2005). Begrünungsmaßnahmen auf der Rückstandshalde des Kaliwerkes - Sigmundshall in Bokeloh (Doctoral Dissertation). Ökologie und Umweltsicherung, Bd. 25/2005, Universität Kassel, Witzenhausen, Germany.
Nelles, M., Grünes, J., and Morscheck, G. (2016). Waste management in Germany - development to a sustainable circular economy? Procedia Environ. Sci. 35, 6–14. doi:10.1016/j.proenv.2016.07.001
Niessing, S. (2005). Begrünungsmaßnahmen auf der Rückstandshalde des Kaliwerkes - Sigmundshall in Bokeloh (Doctoral Dissertation) Ökologie und Umweltsicherung, Bd. 25/2005. Witzenhausen: Universität Kassel.
Paredes, M. (2016). The glocalization of mining conflict: Cases from Peru. Extr. Industries Soc. 3, 1046–1057. doi:10.1016/j.exis.2016.08.007
Peel, M. C., Finlayson, B. L., and Mcmahon, T. A. (2007). Updated world map of the Köppen-Geiger climate classification. Hydrol. Earth Syst. Sci. 11, 1633–1644. doi:10.5194/hess-11-1633-2007
Podlacha, G. (1999). Untersuchungen zur Substratandeckung mit geringen Schichtstärken aus Bodenaushub-Wirbelschichtasche-Gemischen und ihrer Begrünung (Doctoral Dissertation). Ökologie und Umweltsicherung, Bd. 16/1999, Universität Kassel, Witzenhausen, Germany.
Rauche, H. A. M., and Fulda, D. (2001). Tailings and disposal brine reduction – design criteria for potash production in the 21st century. Proceedings of the Eighth International Conference on Tailings and Mine Waste `01, Colorado, USA, January 16-19, 2001 (Fort Collins: International Conference on Tailings and Mine Waste).
Rauche, H. (2015). Die Kaliindustrie im 21. Jahrhundert. Stand der Technik bei der Rohstoffgewinnung und der Rohstoffaufbereitung sowie bei der Entsorgung der dabei anfallenden Rückstände. 1. Aufl. 2015 Edition. Berlin, Heidelberg: Springer Vieweg.
Sabbas, T., Polettini, A., Pomi, R., Astrup, T., Hjelmar, O., Mostbauer, P., et al. (2003). Management of municipal solid waste incineration residues. Waste Manage 23, 61–88. doi:10.1016/S0956-053X(02)00161-7
Scheer, T. (2001). Rekultivierung von Rueckstandshalden der Kaliindustrie. Untersuchungen zur Nutzbarkeit aufbereiteter Salzschlacke der Sekundaeraluminium-Industrie als Rekultivierungsmaterial einer Kali-Rückstandshalde (Doctoral Dissertation). Ökologie und Umweltsicherung, Bd. 20/2001. Witzenhausen, Germany: Universität Kassel.
Schmeisky, H., and Hofmann, H. (2000). Rekultivierung von Rückstandshalden der Kaliindustrie - untersuchungen zum Salzaustrag, zur Sukzession sowie Maßnahmen und Erkenntnisse zur Begrünung. Ökologie und Umweltsicherung, 19/2000,. Witzenhausen, Germany: Universität Kassel.
Simons, J., and Bennett, D. (2022). Measuring soil salinity. Dep. Prim. Industries Regional Dev. Australia 913. Available at: https://www.agric.wa.gov.au/soil-salinity/measuring-soil-salinity#ECeflag (accessed on 01 07, 2023).doi:10.1007/978-1-61779-986-0_28
Warren, J. K. (2006). Evaporites: Sediments, resources and hydrocarbons. Berlin, Heidelberg: Springer-Verlag Berlin Heidelberg. doi:10.1007/3-540-32344-9
Keywords: evapotranspiration covers, mining, technogenic substrates, physical properties, evaporation, drainage
Citation: Bilibio C and Hensel O (2023) Pile covering of waste disposal using technosols made of municipal solid waste incineration bottom ash and coal combustion residues: A column leaching experiment. Front. Environ. Sci. 11:1144043. doi: 10.3389/fenvs.2023.1144043
Received: 13 January 2023; Accepted: 27 February 2023;
Published: 10 March 2023.
Edited by:
Jose Navarro Pedreño, Miguel Hernández University of Elche, SpainReviewed by:
Gregorio Garcia, Polytechnic University of Cartagena, SpainIrene Voukkali, Open University of Cyprus, Cyprus
Copyright © 2023 Bilibio and Hensel. This is an open-access article distributed under the terms of the Creative Commons Attribution License (CC BY). The use, distribution or reproduction in other forums is permitted, provided the original author(s) and the copyright owner(s) are credited and that the original publication in this journal is cited, in accordance with accepted academic practice. No use, distribution or reproduction is permitted which does not comply with these terms.
*Correspondence: Carolina Bilibio, Y2Fyb2xpbmEuYmlsaWJpb0B1bmkta2Fzc2VsLmRl