- 1Queensland Herbarium, Department of Environment and Sciencey, Toowong, QLD, Australia
- 2School of Biological Sciences, University of Queensland, Brisbane, QLD, Australia
- 3Departement Fysische Geografie, Universiteit Utrecht, Utrecht, Netherlands
- 4Australian Museum Research Institute, Sydney, NSW, Australia
- 5Departamento de Ecología Evolutiva, Instituto de Ecología, Universidad Nacional Autónoma de México, Mexico City, Mexico
- 6Springs Stewardship Institute, Flagstaff, AZ, United States
Introduction: While the biodiversity value of springs is recognised, it has not been systematically compiled. The aim of the current study is to highlight the extraordinary endemism associated with the isolated habitat of arid-land springs at three locations in two continents.
Methods: The habitat endemism of the eukaryote species associated with the aquatic and terrestrial habitats at Ash Meadows in the USA, Byarri in Australia and Cuatro Ciénegas in Mexico was assembled based on their geographic distribution.
Results: The currently-known aquatic and semi-aquatic endemic species number 27 at Ash Meadows, 31 at Byarri and 34 at Cuatro Ciénegas. Terrestrial endemic species are represented by two species at Ash Meadows, five at Byarri and 26 at Cuatro Ciénegas. The terrestrial endemics are associated with the scalded areas surrounding the springs impregnated with soda and gypsum. The persistence of the endemics is astonishing given that the wetlands represent tiny islands of habitat (216 small wetlands over 40 km2 in the case of Byarri).
Discussion: A key factor for the persistence and radiation of endemic species is the stability and permanence of the wetlands over evolutionary time-scales. Genetic evidence indicates the presence of both paleo-endemics, species that persisted in spring wetlands as relics of previous mesic climates; and neo-endemics that have dispersed from more mesic environments and subsequently radiated in the spring wetlands as distinct forms. The former evolved from their relatives greater than 106 ya and the latter less than 106 ya. The concentration of endemic species in and around arid-land springs is among the highest concentrations of endemic organisms specialised to a particular habitat and substantiates the paramount conservation significance of desert springs.
Introduction
Springs can provide a perennial water-source for wetlands, and when they occur in arid landscapes represent tiny islands of habitat for aquatic species (Davis et al., 2017). It has already been recognised that spring wetlands can provide habitat for endemic species across the globe (Stevens and Meretsky, 2008; Rossini et al., 2018; Souza and Eguiarte, 2022). Endemism in this context refers to species that are only known from habitats associated with springs, and in many cases are only known from single locations. Wetlands with a source from artesian springs have greater biodiversity values than other wetlands in arid-lands. The diversity and habitat specialisation of plants, molluscs and fish in four permanent wetland types: riverine waterholes, rockholes, outcrop springs, and discharge (or artesian) springs from the arid-lands of Australia were compared (Fensham et al., 2011). Outcrop springs are fed by gravity and occur in unconfined geological substrata, generally close to where their water source is recharged. They contrast with artesian springs, which occur in confined aquifers and are fed by pressurised groundwater with sufficient residence time to be affected by the aquifer substrate and are often in areas remote from where recharge is occurring. The riverine waterholes, rockholes, outcrop springs had low levels of endemism compared to artesian springs, which were shown to have a high diversity of specialised endemics across all three taxonomic groups. This study identified three factors that may be important determinants of endemism in artesian spring wetlands: isolation, distinctive habitat, and long-term continuity of habitat.
Aridity (a surrogate of isolation) was a predictor of increasing endemic diversity in the artesian springs of the Great Artesian Basin (GAB) in Australia (Rossini et al., 2018). In contrast riverine waterholes have low concentrations of endemics because the perpetuation of unique genotypes is precluded by flooding disturbance and connection to more mesic environments during floods (Fensham et al., 2011; Stevens et al., 2020).
Specialisation to spring habitats relates to environmental constancy, for example, in water level, low turbidity, and geochemistry (Fensham et al., 2011). Habitat permanency is an essential pre-requisite for endemism in spring wetlands by providing sufficient time for occurrence and fixation of unique genotypes. Supporting this contention, permanent wetlands support more distinctive biota compared to ephemeral wetlands (Erman and Erman, 1995; Wood et al., 2005). The lack of endemism in the outcrop springs and rockholes of Australia may relate to the impermanence of their aquatic environments over evolutionary timescales (e.g., Ponder and Slatyer, 2007).
The occurrence of endemic species is evidence that some springs have fed permanent wetlands over very long timescales. They may function as paleo-refugia (Nekola, 1999), where lineages that evolved in ancestral mesic conditions survive despite aridification of environment. Species that have persisted in spring wetlands as relics of previous climate regimes have been referred to as “paleo-endemic” species (Stebbins and Major, 1965). Alternatively, organisms may have colonized springs recently from more mesic environments and then evolved in these habitats as “neo-endemic” species. Paleo-endemic species are relics of a formerly more widespread matrix community, while neo-endemic species occur in habitats that are younger than the matrix community (Nekola, 1999).
Around the periphery of spring wetlands where the water chemistry is affected by the aquifer substrate there is a distinctive ‘scalded’ habitat. These habitats are not wetlands despite being characterised by salts which are concentrated by the evaporation of groundwater. In Australia, the soils in these habitats typically have pH values greater than 9 supporting a low cover of succulent plants (Fensham et al., 2021). These habitats also support endemic species with very local distributions compared to other species specialised to non-alkaline saline environments.
Three complexes of spring wetlands on two continents have reasonably comprehensive biological surveys and reveal high concentrations of both aquatic and terrestrial endemic species: Ash Meadows, Nevada, United States (Stevens and Meretsky, 2008), Byarri (also known as Edgbaston Springs) in Queensland (Rossini et al., 2018), Australia, and Cuatro Ciénegas Basin, Coahuila, Mexico (Souza and Eguiarte, 2022). While the macro-biota of these three sites is relatively well known, not all species have been formally described and collation of comprehensive, comparative species lists has not previously been attempted.
Here we present lists of the endemic species occurring in the three spring complexes and use the data to 1) highlight their overall extraordinary unique biodiversity; 2) compare their endemic diversity across taxonomic groups; 3) compare common features of the three regions to further understand the critical features of arid-land springs related to endemic diversity; and 4) and establish that both ‘paleo-endemics’ and ‘neo endemics’ occur in these habitats. We also comment on the possibilities for new discoveries of unique life in these specialised habitats.
Methods
Geography of spring complexes
We compiled the locations of individual spring wetlands at Ash Meadows (Scoppettone et al., 2012), Byarri (Fensham and Laffineur, 2022)and at Cuatro Ciénegas (Wolaver et al., 2013). Discharge at Byarri was determined using a method relating wetland area to flow rates (Fensham and Laffineur, 2022) but including previously unmapped wetlands and another large wetland that had been excluded from previous discharge estimates because it had been artificially drained (Fensham and Laffineur, 2022). Discharge data for Ash Meadows were obtained from Laczniak et al. (1999)and for Cuatro Ciénegas from Wolaver et al. (2008). Other information on the geography of these spring complexes was obtained from global datasets of rainfall (Fick and Hijmans, 2017), a digital elevation model (Japan Aerospace Exploration Agency, 2021), the literature, and the knowledge of the co-authors.
Endemic species
We define the term ‘spring endemic’ as meaning any species confined to a spring-dependent ecosystem, including the aquatic species confined to groundwater-fed wetlands, as well as terrestrial species confined to the specialised (often saline) habitats on the periphery of springs (Fensham et al., 2021). Endemic terrestrial plant species associated with spring-related terrestrial environments at Cuatro Ciénegas were identified as those occurring in chaparral; desert scrub, bajadas, and flats, as well as gypsum dunes and flats (Pinkava, 1984). Many endemic species are confined to one of the three springs complexes, and these species are distinguished from the more general endemics that occur in spring environments elsewhere. Although eDNA research has revealed an enormous biodiversity of microbial life at springs (e.g., Paulson and Martin, 2019; Espinosa-Asuar et al., 2022), we only include eukaryotes in this study. Species lists for the three spring complexes were compiled from available literature and consultation with taxonomic experts to confirm the species rank of undescribed species (See Supplementary Material). Only taxa at the species level were included for comparison. Our lists were sorted by taxonomic groupings and required habitat(s).
The genera of endemic spring-dependent species occurring within these three spring complexes but not otherwise known from non-spring wetland habitats in the major catchment must have either become isolated in the springs, evolved within the springs, or accomplished long-distance dispersal to colonise the springs. Such genera were identified using the Great Basin (0.54 M km2), Lake Eyre Basin (1.20 M km2), the Rio-Grande Basin (0.47 M km2) as the major catchments for Ash Meadows, Byarri, and Cuatro Ciénegas, respectively.
Results
Geography of spring complexes
Ash Meadows springs are situated in a Mojave Desert valley in south-western Nevada, with a mean annual rainfall of about 100 mm (Figures 1, 2). The springs are supplied by a carbonate aquifer of Cambrian limestone, which is exposed adjacent to the springs forming Devils Hole Ridge to the east (Dublyansky and Spötl, 2015). These limestones have undergone major deformation, including block faulting in the mid-Tertiary that caused north-south orientated fractures, allowing discharge at the springs (Schweickert and Lahren, 1997). A groundwater flow corridor of about 120 km also extends northward from a recharge area in the Spring Mountains, where mean annual rainfall is approximately 600 mm (Winograd et al., 1998) and is possibly supplemented by other flow corridors (Dublyansky and Spötl, 2015).
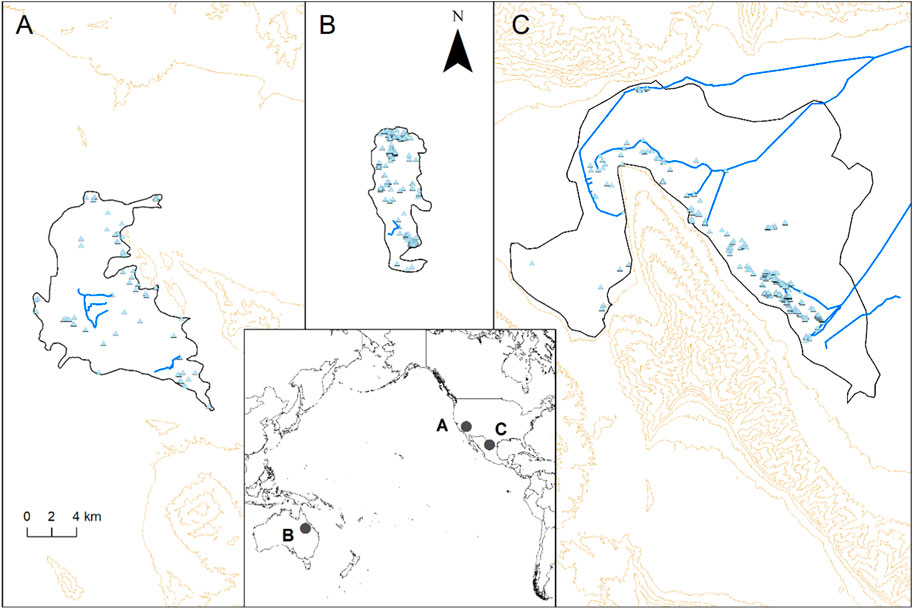
FIGURE 1. Ash Meadows (A), Byarri (B) and Cuatro Ciénegas (C) with spring vents (triangles), the approximate area of the surrounding scalded habitat (black line), artificial canals (blue lines), and the topography (100 m contours) in the inset map indicating their location.
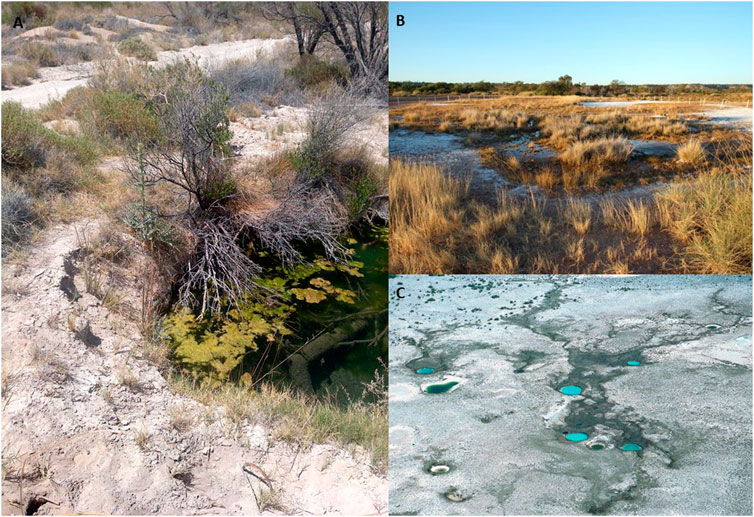
FIGURE 2. (A) Five Springs; a typical example of the springs at Ash Meadows depicting the deep water vent and the scalded environment surrounding the springs (Photo: Lawrence Stevens); (B) Type Locality spring at Byarri depicting the typical shallow water vegetated wetlands dominated by endemic plant species (Photo: Roderick Fensham); (C) Aerial view of part of wetlands and scalded surrounding environment at Cuatro Ciénegas (Photo: Italo Giulivo) where a rim of stromatolite reef can be observed.
Lying within the Amargosa River basin, Ash Meadows Valley includes twelve large springs with associated aquatic and peripheral riparian vegetation, as well as several dozen smaller hillslope springs and Devils Hole. The latter is a 22 m long by 3.5 m sinkhole of non-flowing groundwater in a sheltered bedrock crevice. The large springs in Ash Meadows form open water pools several tens of meters in diameter and reaching a depth of 10 m. Collectively, northern Ash Meadows springs coalesce to form a large, vegetated wetland called the Carson Slough, which flows south and is impounded in two reservoirs. The Carson Slough discharges into the Amargosa River basin. This endorheic catchment terminates at an elevation of 80 m below sea level on the south side of Death Valley. Prior to 1984, parts of Ash Meadows were drained by irrigation canals, and the wetlands sustained peat-cutting and alfalfa farming. Most of this springs complex is now managed as a conservation reserve.
Water quality among Ash Meadows springs varies but is generally moderately low in dissolved oxygen, moderately mineralized, slightly above normal pH, and slightly warmer than mean air temperature (Table 1), with Devils Hole maintaining a constant temperature of 33°C.
Byarri is a complex of springs occurring over an area of 40 km2 of scalded habitat within a semi-arid landscape (mean annual rainfall 461 mm) (Figures 1, 2). The springs are fed by a confined aquifer composed of Jurassic sandstone where the fine-grained sediments of the overlying aquitard have been thinned by erosion (Fensham and Laffineur, 2022). The aquifer is within the subterranean Eromanga Basin, a major component of the Great Artesian Basin. The recharge for the springs occurs where the sediments outcrop in a flat landscape approximately 30 km to the east and the potentiometric surface of the aquifer is less than a metre above the ground surface in the spring complex (Fensham and Laffineur, 2022). The springs are located at the head of the catchment of Pelican Creek beneath a low scarp rising 40 m above a floodplain associated with ephemeral drainage, and including two ephemeral lakes (Fensham and Laffineur, 2022). The area where the springs occur is interspersed by clay scalds, calcrete and aeolian sand dominated by spinifex (Triodia longiceps) hummock grassland. The dominant land use has been sheep and cattle grazing, although more than half of the area is now a conservation reserve.
The Byarri springs support vegetated wetlands with occasional open water pools, varying in size up to 5.1 ha, although only 21 of the 216 springs are larger than 1000 m2. The water in these wetlands is generally less than 1 cm deep, but up to 10 cm deep near the discharge vents. The pH of these springs is relatively neutral but the CaHCO3–type water leaves evaporites of alkaline salts accumulating around the spring margins, creating scalded habitat where pH reaches 10.3 (Fensham et al., 2021). Discharging groundwater is not geothermal, although temperatures in the pools vary between about 5 °C and 40 °C, depending on distance from the discharge point, and seasonal and diurnal conditions (Rossini, et al., 2017a). Conductivity is 300 μS cm-1 within the wetlands but can be higher than 100,000 μS cm−1 in the scalded terrestrial habitats surrounding the spring pools (Fensham et al., 2021). Total discharge from the springs has been estimated as 22 L s−1, has been substantially lower in the recent past but was almost certainly higher prior to extraction by free-flowing bores for pastoralism (Fensham and Laffineur, 2022).
Cuatro Ciénegas is a spring complex in the Mexican state of Coahuila, with a mean annual rainfall of 241 mm (Figures 1, 2). The springs occur at the base of the Sierra San Marcos Mountains, which rise 700 m above the surrounding plains. The springs are fed by a regional aquifer of folded Cretaceous limestone discharging through faults but is also supplemented in places by locally recharged water (Wolaver and Diehl, 2011; Wolaver et al., 2013). Hundreds of springs feed a mosaic of vegetated wetlands connected by channels, with individual wetlands up to 100 ha. Channels drain the springs and wetlands and there are open water pools up to 4 ha in size and 15 m deep. The pH of Cuatro Ciénegas waters ranges from 9 in shallow ponds to neutral in deeper springs, and both Ca HCO3-and Ca SO4–type waters occur, resulting in extensive travertine and gypsum deposits that form dunes and scalded habitat (Wolaver et al., 2013). Most of the groundwater is moderately geothermal, ranging up to 35 °C (Table 1). Discharge from the springs has been estimated between 1100 and 1700 L s−1 (Wolaver et al., 2008). Even though the biota of the springs are protected in a conservation reserve, the water is subject to the national water distribution program, Ley Nacional de Agua. The wetlands continue to be dewatered since the outflow from the springs is drained by canals that divert the flow for intensive agriculture downstream (Leal Nares et al., 2022). These impacts have lowered the water table and diminished the wetland habitat area (Hendrickson and Minckley, 1985).
Endemic species
The currently-known aquatic and semi-aquatic endemic species number 34 at Cuatro Ciénegas, 31 at Byarri, and 27 at Ash Meadows (Table 2). Endemic aquatic and semi-aquatic plant species, represented by 12 eudicot and monocot families, number 11 at Byarri, six at Ash Meadows and none at Cuatro Ciénegas. There are nine aquatic endemic plant species at Byarri and only one at Ash Meadows. The animal phyla Platyhelminthes and Annelida are represented by single endemic species at Byarri but are not known from the other two locations. Endemic gastropods from seven families are represented at all three sites, with twelve extant and one extinct species at Ash Meadows, twelve species at Byarri and 13 at Cuatro Ciénegas. Small truncatelloidean gastropods are particularly diverse at all sites. Of these, Pyrgulopsis (Hydrobiidae; nine species) and Tryonia (Cochliopidae; four species) constitute the only two families of aquatic gastropods represented at Ash Meadows while at Cuatro Ciénegas these same families are present, with eight cochliopids and three hydrobiids, together with a lithoglyphid and an assimineid. At Byarri nine species of Tateidae are present, along with a bithyniid. Crustacea are represented at Byarri (three species) and Cuatro Ciénegas (seven species). Aquatic arachnids are represented by a species of mite at Cuatro Ciénegas. Aquatic insects are well represented at Ash Meadows, with three beetle (Coleoptera) and two true bug (Hemiptera) species. There are single endemic dragonfly (Odonata) species at both Byarri and Cuatro Ciénegas. There were five endemic fish species in three families at Ash Meadows (although the goodeid Emepetrichthyes merriami is now extinct). There are two species in two families of endemic fish at Byarri, and eight species in four families at Cuatro Ciénegas. Three species of turtle and one species of lizard at Cuatro Ciénegas are the only aquatic or semi-aquatic reptiles.
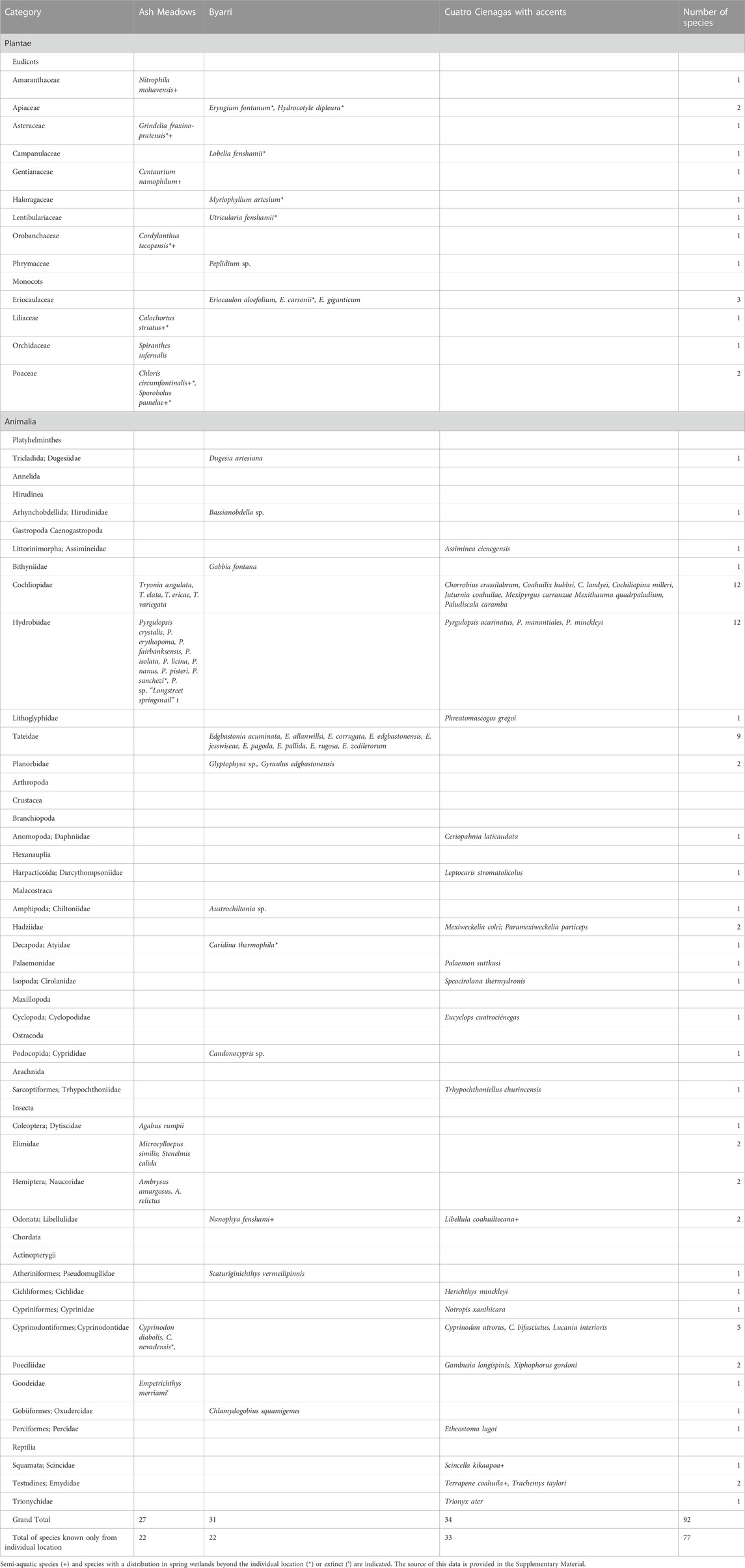
TABLE 2. Endemic aquatic and semi-aquatic species at three locations of spring complexes arranged in their taxonomic groups.
The currently known terrestrial endemic species are represented by at least two species at Ash Meadows, five at Byarri and 26 at Cuatro Ciénegas (Table 3). Plants are represented across eleven eudicot families, with two species at Ash Meadows, four species at Byarri and 17 species at Cuatro Ciénegas. Endemic terrestrial arthropods are represented by one species of spider at Byarri, and by two species of scorpions, four beetle species and a single moth species at Cuatro Ciénegas. There are two endemic species of lizards in terrestrial environments at Cuatro Ciénegas, but no endemic terrestrial vertebrate species at the other two spring locations.
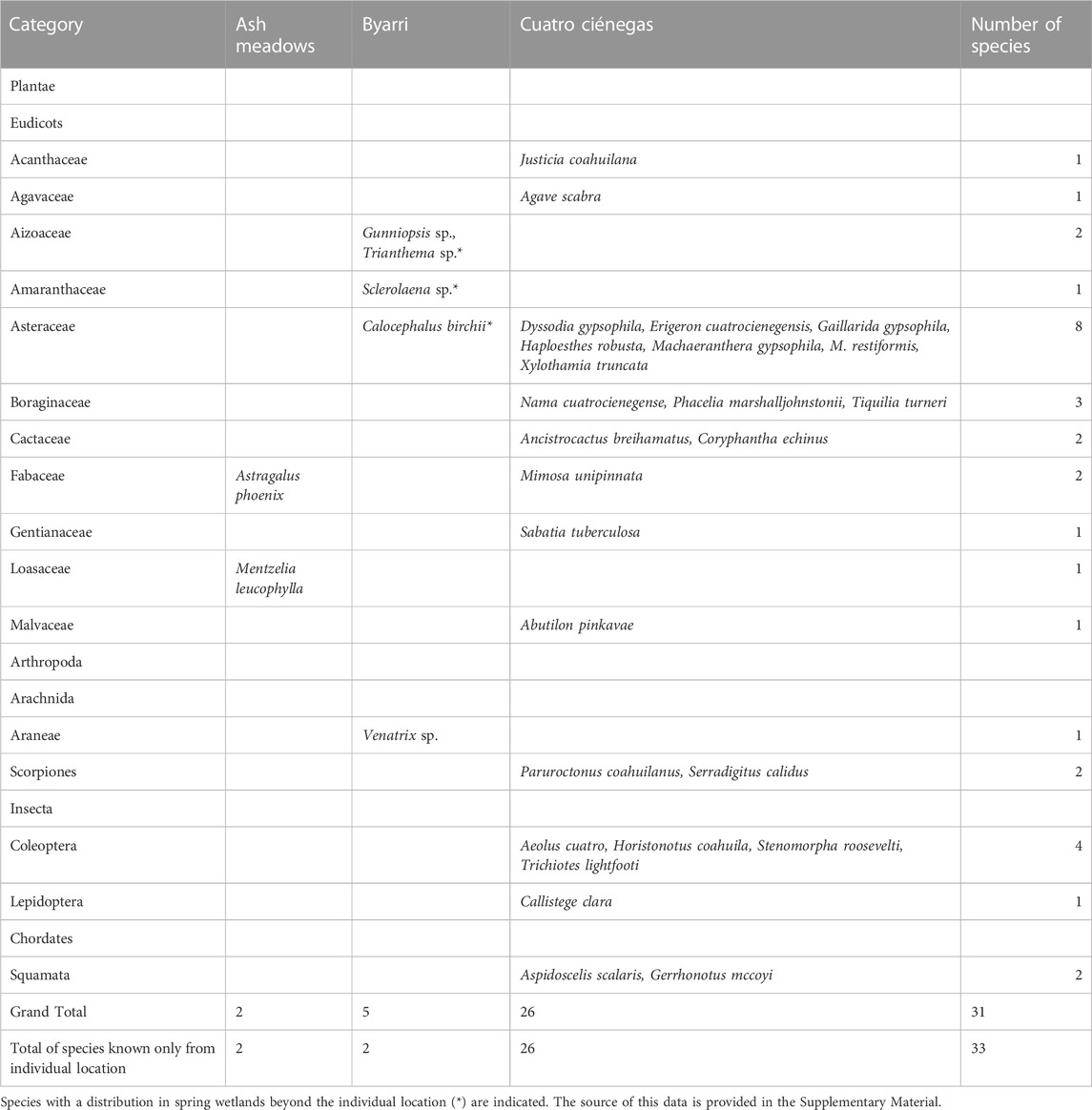
TABLE 3. Endemic terrestrial species at three locations of spring complexes sorted arranged in their taxonomic group.
The fish genus Empetrichthys is endemic to Ash Meadows and another four genera from Ash Meadows (Cyprinodon, Microcylloepus, Ambrysus, and Stenelmis) occur only in spring-fed wetland and streams within the Great Basin catchment. One genus (Scaturiginichthys) is endemic to Byarri and another four genera from Byarri (Candonocypris, Edgbastonia, Eriocaulon and Hydrocotyle) occur only in spring wetlands from the Lake Eyre catchment. Five genera (Chorroibius, Mexipyrgus, Mexithauma, Paludiscola and Phreatomascogos) are endemic to Cuatro Ciénegas and another Pyrgulopsis from Cuatro Ciénegas occur only in spring wetlands from the Rio Grande catchment.
Discussion
This compilation of macro-organisms from these three locations on two continents demonstrates that an extraordinary concentration of local (fine-scale) endemism occurs in habitats associated with arid-land springs. The largest of the three locations, Cuatro Ciénegas, is only 399 km2 in area but provides habitat for 60 species known only from spring-associated habitats at that location. Ash Meadows and Byarri are even smaller in area (Table 1) and both have 24 species restricted to those locations. There are an additional five species at Ash Meadows and 12 species at Byarri that are only known from habitats associated with springs, but also occur further afield than those two individual locations (see Supplementary Material). Eukaryote endemism at these sites is associated with a broad range of phyla (plants, platyhelminths, annelids, molluscs, arthropods and chordates), but these levels of endemism may even be greater when comprehensive surveys are complete and taxonomy resolved. There is also likely a vast but largely undescribed array of microbial taxa and eDNA will be an important tool to reveal that diversity (Souza et al., 2006; Moreno-Letelier et al., 2012; Paulson and Martin, 2019). A species of cyanobacteria that forms macro-colonies at Byarri is the single described endemic prokaryote from any of three sites (McGregor and Sendall, 2017). Endemic species are not only associated with the aquatic environments of the spring wetlands but also with the scalded saline areas around the wetlands.
Many of these endemic species are related to taxa that are distributed regionally, particularly in the terrestrial environments (see Supplementary Material). However, five aquatic and semi-aquatic endemic species at Ash Meadows, five at Byarri and six at Cuatro Ciénegas are from genera without congeneric relatives in non-spring habitats in the major catchments where they currently occur. These genera have either evolved in springs as paleo-endemics, been isolated after the extinction of ancestors, or have radiated after unlikely long-distance dispersal across major catchment divides.
Spring-associated endemic aquatic plant species are diverse at Byarri but are relatively few in North America (Table 1). Endemic beetles and Hemiptera have only been described from Ash Meadows. Endemic snails, particularly those in the caenogastropod superfamily Truncatelloidea occur at all locations. Scaturiginichthys (Byarri) and Empetrichthyes (Ash Meadows) are fish genera entirely restricted to spring habitats. A lizard and three species of semi-aquatic turtle are confined to Cuatro Ciénegas and are the only other non-fish chordates at any of the locations. The last association is probably dependent on the especially large size of the wetlands at Cuatro Ciénegas, which have twice the discharge of Ash Meadows, and about 30 times greater discharge than Byarri.
The high diversity of endemic aquatic plant species at Byarri and their scarcity at the other two locations is difficult to explain. These plant species are perennial and form short, rhizomatous mat-like colonies, a growth form that occurs widely throughout the springs of arid and semi-arid Australia (Fensham et al., 2004). This growth form may be particularly well adapted to small wetlands with a constant flow, but like grasses are also adapted to grazing. Such islands of green forage in a barren landscape are occasionally subject to extreme grazing pressure from livestock and feral and native herbivores. Populations of endemic plants, such as Eriocaulon carsonii can be greatly diminished by intense grazing and pugging by feral pigs, only to recover when these pressures are reduced (R. Fensham pers. obs.). The springs-dependent endemic plants of Australia have been subject to intense herbivory pressure from marsupials including extinct Pleistocene megafauna (Johnson, 2006) over evolutionary time; thus, the perennial mat-forming growth form may partly be an adaptation to withstand herbivory.
Spring wetlands represent tiny aquatic islands surrounded by a vast “sea” of harsh desert habitat and are extremely isolated from other aquatic habitats. Thus, a species that has dispersed or become trapped in a spring-fed wetland has evolved into a new form under reduced competition with its ancestral relatives. For this allopatric evolution to occur, the spring habitats must be relatively persistent and isolated over at least recent evolutionary time. The magnitude of these timescales is partly informed by the age of spring wetland complexes. For example, the spring deposits at Ash Meadows lie in the Great Basin, which was subjected to rain shadow aridification beginning in Miocene time. Ash Meadows contains spring mound deposits dating to 3.2 my ago. Surface water connections existed with Owens Valley and through the Pleistocene advances and retreats of Lakes Tecopa and Manly in the lower Amargosa River drainage (Phillips, 2008). Thus, while largely surrounded by Paleozoic limestones and dolomites (Denny and Drewes, 1965), its Neogene sedimentological history indicates that groundwater expression has been commonplace in Ash Meadow for several million years. Also, periodic but intensifying aridification, has reduced spring density and discharge over time (Denny and Drewes, 1965; Hay et al., 1986; Menges, 2008).
In Australia, the aridification of the interior of the continent commenced about 4 Ma, and formation of the major dune fields, that are still in place today, commenced about 1 Ma (Fujioka et al., 2009). This time-scale coincides with the evolutionary lineages that represent much of the contemporary arid-zone flora (Byrne et al., 2008). The hydrological gradient of the Great Artesian Basin is the result of large synclinal structure uplifted and exposed from the development of the Great Dividing Range in the vicinity of its eastern margin (Habermehl, 2020). The timing of this orogeny remains controversial, but Ollier (1982) opined that it mostly occurred between 90 and 30 Ma. The suggested age of the springs towards the western margin in the Lake Eyre region is late Tertiary (Jessup and Norris, 1971), and a <2 Ma time frame is consistent with the uranium series disequilibrium dating of travertine deposits that are <1 my old (Priestley et al., 2018).
Deep phylogenetic divergence of species lineages of snails (Ponder et al., 1995; Perez et al., 2005; Murphy et al., 2012; Ponder et al., 2019) and isopods (Guzik et al., 2012) in isolated springs complexes of the Great Artesian Basin in Australia suggest they are derived from ancestors much older than 2 Ma. At Cuatro Ciénegas sub-populations of the endemic snail Mexipyrgus churinceanus occupy isolated drainage areas within the springs complex and appear to represent distinctive lineages that shared a common ancestor 2.5 Ma (Johnson, 2005). These timescales support the understanding that at least some of the species represented in desert springs are paleo-endemics representing relictual lineages from previous, more mesic environments.
There is also clear evidence that some of the endemics in spring-fed wetland habitats are neo-endemics. The endangered devils hole pupfish (Cyprinodon diabolis) is characterised by its small population size and its lack of pelvic fins. It has a population less than 1000 individuals and breeds exclusively on a shallow 3.5 by 5 m ledge near the surface of a subaqueous cavern, which is its sole habitat. Long thought to be a Pleistocene relic (Echelle, 2008), recent genetic studies (subject to considerable debate) indicate that the species diverged from its sister taxon Cyprinodon nevadenesis less than 10,000 ya (Sağlam et al., 2017; Sağlam et al., 2018) and possibly less than 1,000 ya (Martin et al., 2016; Martin et al., 2017). At Ash Meadows, lineages of hydrobiid snails in the genus Pyrgulopis exhibit both deep structure, suggesting divergence during the Pliocene, and also shallow structuring indicating divergence younger than 0.7 Ma (Hershler and Liu, 2008). The latter in some cases appear to have involved cross-catchment dispersal.
To what extent is the endemism of the springs associated with their unusual geochemistry? The terrestrial endemic plant species are associated with unusual alkaline soda rich (NaHCO3) or neutral gypsum-rich (CaSO4) saline environments. These environments are a product of the evaporites of the groundwater and the endemic halophytes have a specific adaptation to these specialised environments (Fensham et al., 2021).
Aquatic conditions in the springs themselves are generally more benign than the surrounding ‘scalds’, with relatively neutral or slightly alkaline pH at all three sites (Table 1). A simple experiment where the spring endemic plant Eriocaulon carsonii was grown under a range of pH conditions indicated that the species is equally able to tolerate alkaline (pH 7.0-7.7) and neutral (pH 6.4-7.0) conditions but is intolerant of acid conditions (pH of 4.1–4.4). Experiments on endemic snails indicate conditions become lethal when salinity is more than 15,000 μS/cm and temperatures <5°C and >40°C, conditions corresponding to the variation within the aquatic environments of the springs but exceeded in the shoreline conditions where evaporation concentrates salt (Ponder et al., 1989; Rossini, et al., 2017b). There is no evidence from these experiments that the snails are impeded in ‘soft’ waters with low salinity and neutral pH. Breeding populations of the endemic fish Scaturiginichthys vermeilipinnis can be maintained in water from Brisbane main water supply (P. Johnson pers. comm.). Disparities between physical and microbial environments of natural and artificial habitats may account for the limited recruitment of populations of the devils hole pupfish in the artificial habitat (Sackett et al., 2018). The extent that the geochemical environment of springs is a critical determinant of the habitat of endemic species requires further study.
The species list generated here includes taxa that are not yet formally described and excludes species at the sub-species level. A comprehensive taxonomy of the species in spring wetlands supported by molecular data will help to resolve the true levels of endemism in spring habitats and may identify cryptic species that are difficult to distinguish using morphology alone but may be only distant relatives due to the long periods of isolation in separate spring complexes. Such potential for cryptic speciation already has been recognised among amphipods that occupy individual artesian springs in South Australia (Guzik et al., 2012) and the American Southwest (Adams et al., 2018). Populations of a grass species, Cenchrus purpurascens, in isolated spring wetlands are morphologically indistinguishable from other populations across Asia and Australia (where it has been considered an exotic weed) but have been shown to have diverged in the order of 105–106 ya (Toon et al., 2018). In other cases, as for the devils hole pupfish, further study will reveal when species have morphologically diverged.
Conclusion
The concept of species endemism only has meaning in reference to a defined area or habitat (Shipley and McGuire, 2022). Endemism can be associated with specialised habitats at local scales, for example, cloud-forests, distinctive geological formations, coral reefs or wetlands. In this context and in lieu of comparative studies, the spring ecosystems at Ash Meadows, Byarri and Cuatro Ciénegas have some of the highest documented concentrations of endemic species in the world and highlights the critical importance of desert springs as specialised habitats. There is evidence from genetic analyses of these organisms that some pre-date Tertiary aridification and subsequent isolation in the springs, while others result from much more recent dispersal and rapid divergence in these unusual but constant habitats. The biota of spring habitats consists of both paleo-endemic taxa that evolved before 106 ya, as well as neo-endemic taxa that are likely much younger. There are many springs that do not have high concentrations of endemics and the environment, distribution and history of these springs will undoubtedly help explain the situations where the endemics occur. Our understanding of the biology of these three locations on two continents may be at least partly a product of the extent to which they have been biologically surveyed. Desert spring complexes around the world await investigation.
Data availability statement
The original contributions presented in the study are included in the article/Supplementary Material, further inquiries can be directed to the corresponding author.
Author contributions
RF conceived and designed the project. WP and LS contributed to defining species distributions. RF wrote the manuscript and WP, LS, and VS provided editorial input. All authors contributed to the article and approved the submitted version.
Acknowledgments
This paper was written while the senior author was a visiting scholar in the Departement Fysische Geografie, Universiteit Utrecht and he thanks all the staff and students who made him very welcome. Boris Laffineur drafted Figure 1 and he and Jen Silcock are thanked for her helpful comments on the manuscript.
Conflict of interest
The authors declare that the research was conducted in the absence of any commercial or financial relationships that could be construed as a potential conflict of interest.
Publisher’s note
All claims expressed in this article are solely those of the authors and do not necessarily represent those of their affiliated organizations, or those of the publisher, the editors and the reviewers. Any product that may be evaluated in this article, or claim that may be made by its manufacturer, is not guaranteed or endorsed by the publisher.
Supplementary material
The Supplementary Material for this article can be found online at: https://www.frontiersin.org/articles/10.3389/fenvs.2023.1143378/full#supplementary-material
References
Adams, N. E., Inoue, K., Seidel, R. A., Lang, B. K., and Berg, D. J. (2018). Isolation drives increased diversification rates in freshwater amphipods. Mol. Phylogenet. Evol. 127, 746–757. doi:10.1016/j.ympev.2018.06.022
Byrne, M., Yeates, D. K., Kearney, M., Bowler, J., Williams, M. A. J., Cooper, S., et al. (2008). Birth of a biome: Insights into the assembly and maintenance of the Australian arid zone biota. Mol. Ecol. 17, 4398–4417. doi:10.1111/j.1365-294x.2008.03899.x
Davis, J. A., Kerezsy, A., and Nicol, S. (2017). Springs: Conserving perennial water is critical in arid landscapes. Biol. Conserv. 211, 30–35. doi:10.1016/j.biocon.2016.12.036
Denny, C. S., and Drewes, H. (1965). The history of a desert basin and its bordering highlands. Bulletin 1181-L. Washington DC: U.S. Geological Survey. Available at: https://pubs.usgs.gov/bul/1181l/report.pdf.
Dublyansky, Y. V., and Spötl, C. (2015). Condensation-corrosion speleogenesis above a carbonate-saturated aquifer: Devils Hole Ridge, Nevada. Geomorphology 229, 17–29. doi:10.1016/j.geomorph.2014.03.019
Echelle, A. A. (2008). “The Western NorthNorth American pupfish clade (Cyprinodontidae: Cyprinodon): Mitochondrial DNA divergence and drainage history,” in Late cenozoic drainage history of the southwestern Great Basin and lower Colorado river region: Geologic and biotic perspectives. Geological society of America special paper 439. Editors M. C. Reheis, R. Hershler, and D. M. miller (The Geological Society of America), 27–38. doi:10.1130/2008.2439(02
Erman, N. A., and Erman, D. C. (1995). Spring permanence, Trichoptera species richness, and the role of drought. J. Kans. Entomol. Soc. 68 (2), 50–64.
Espinosa-Asuar, L., Monroy-Guzmán, C., Madrigal-Trejo, D., Navarro-Miranda, M., Sánchez-Pérez, J., Buenrostro Muñoz, J., et al. (2022). Diversity of an uncommon elastic hypersaline microbial mat along a small-scale transect. PeerJ 10, e13579. doi:10.7717/peerj.13579
Fensham, R. J., Fairfax, R. J., and Sharpe, P. R. (2004). Spring wetlands in seasonally arid Queensland. Floristics, environmental relations, classification and conservation values. Aust. J. Bot. 52, 583–595. doi:10.1071/bt03171
Fensham, R. J., and Laffineur, B. (2022). Response of spring wetlands to restored aquifer pressure in the Great Artesian Basin, Australia. J. Hydrol. 612, 128152. doi:10.1016/j.jhydrol.2022.128152
Fensham, R. J., Laffineur, B., and Shand, P. (2021). Plant species associations with alkaline environments associated with artesian spring wetlands. Science of the Total Environment 887, 163936. doi:10.1007/s11104-021-04903-2
Fensham, R. J., Silcock, J. L., Kerezsy, A., and Ponder, W. F. (2011). Four desert waters: Setting arid zone wetland conservation priorities through understanding patterns of endemism. Biol. Conserv. 144, 2459–2467. doi:10.1016/j.biocon.2011.06.024
Fick, S. E., and Hijmans, R. J. (2017). WorldClim 2: New 1-km spatial resolution climate surfaces for global land areas. Int. J. Climatol. 37 (12), 4302–4315. doi:10.1002/joc.5086
Fujioka, T., Chappell, J., Fifield, L. K., and Rhodes, E. J. (2009). Australian desert dune fields initiated with Pliocene - Pleistocene global climatic shift. Geology 37 (1), 51–54. doi:10.1130/G25042A.1
Guzik, M. T., Adams, M. A., Murphy, N. P., Cooper, S. J. B., and Austin, A. D. (2012). Desert Springs: Deep phylogeographic structure in an ancient endemic crustacean (Phreatomerus latipes). Plos One 7 (7), e37642. doi:10.1371/journal.pone.0037642
Habermehl, M. A. (2020). Review: The evolving understanding of the Great Artesian Basin (Australia), from discovery to current hydrogeological interpretations. Hydrogeol. J. 28 (1), 13–36. doi:10.1007/s10040-019-02036-6
Hay, R. L., Pexton, R. E., Teague, T. T., and Kyser, T. K. (1986). Spring-related carbonate rocks, Mg clays, and associated minerals in Pliocene deposits of the Amargosa Desert, Nevada and California. Bull. Geol. Soc. Am. 97 (12), 1488–1503. doi:10.1130/0016-7606(1986)97<1488:SCRMCA>2.0.CO;2
Hendrickson, D. A., and Minckley, W. L. (1985). Cienegas-Vanishing climax communities of the American southwest. Desert Plants 6, 131–175.
Hershler, R., and Liu, H. P. (2008). “Ancient vicariance and recent dispersal of springsnails (Hydrobiidae: Pyrgulopsis) in the Death Valley system, California-Nevada,” in Late cenozoic drainage history of the southwestern Great Basin and lower Colorado river region: Geologic and biotic perspectives. Geological society of America special paper 439. Editors M. C. Reheis, R. Hershler, and D. M. Miller (The Geological Society of America), 439, 91–101. Issue 04. doi:10.1130/2008.2439(04
Japan Aerospace Exploration Agency. (2021). ALOS world 3D 30 meter DEM. V3.2. Hatoyama: JAXA Earth Observation Research Center. doi:10.5069/G94M92HB
Jessup, R. W., and Norris, R. M. (1971). Cainozoic stratigraphy of the Lake Eyre Basin and part of the arid region lying to the south J. Geol. Soc. Australia 18 (3), 303–332. doi:10.1080/00167617108728769
Johannesson, K. H., Cortés, A., and Kilroy, K. C. (2004). Reconnaissance isotopic and hydrochemical study of Cuatro Ciénegas groundwater, Coahuila, México. J. S. Am. Earth Sci. 17 (2), 171–180. doi:10.1016/j.jsames.2004.01.002
Johnson, C. (2006). Australia’s mammal extinctions: A 50,000 year history. Cambridge University Press.
Johnson, S. G. (2005). Age, phylogeography and population structure of the microendemic banded spring snail, Mexipyrgus churinceanus. Mol. Ecol. 14, 2299–2311. doi:10.1111/j.1365-294x.2005.02580.x
Laczniak, R. J., DeMao, G. A., Reiner, S. R., LaRue Smith, J., and Nylund, W. E. (1999). Estimates of ground-water discharge as determined from measurements of evapotranspiration, Ash Meadows area, Nye County, Nevada. Water-Resources Investigations Report 99-4079. Available at: https://babel.hathitrust.org/cgi/pt?id=mdp.39015046280064&view=1up&seq=3&skin=2021.
Leal Nares, O. A., Herrera, G. R., and de la Maza Benignos, M. (2022). “Cuatro Cienagas: An aquifer at risk of overexploitation,” in Conflicts between biodiversity conservation and humans. The case of the chihuahua desert and Cuatro Cienagas. Editors V. Souza, M. C. Mandujano, I. Pisanty, and L. E. Eguiarte (Sporinger), 93–103.
Martin, C. H., Crawford, J. E., Turner, B. J., and Simons, L. H. (2016). Diabolical survival in death valley: Recent pupfish colonization, gene flow and genetic assimilation in the smallest species range on Earth. Proc. R. Soc. B Biol. Sci., 283, 20152334. doi:10.1098/rspb.2015.2334
Martin, C. H., Höhna, S., Crawford, J. E., Turner, B. J., Richards, E. J., and Simons, L. H. (2017). The complex effects of demographic history on the estimation of substitution rate: Concatenated gene analysis results in no more than twofold overestimation. Proc. R. Soc. B Biol. Sci. 284 (1860), 20170537–20171102. doi:10.1098/rspb.2017.0537
McGregor, G. B., and Sendall, B. C. (2017). Iningainema pulvinus gen nov., sp nov. (Cyanobacteria, Scytonemataceae) a new nodularin producer from Edgbaston Reserve, north-eastern Australia. Harmful Algae 62, 10–19. doi:10.1016/j.hal.2016.11.021
Menges, C. M. (2008). “Multistage late Cenozoic evolution of the Amargosa River drainage, southwestern Nevada and eastern California,” in Late cenozoic drainage history of the southwestern Great Basin and lower colrado river region geologic and biotic perspecitves. Special paper 439. Editors M. C. Reheis, R. Hershler, and D. M. Miller (The Geological Soceity of America), 39–90.
Moreno-Letelier, A., Olmedo-Alvarez, G., Eguiarte, L. E., and Souza, V. (2012). Divergence and phylogeny of firmicutes from the Cuatro Ciénegas Basin, Mexico: A window to an ancient ocean. Astrobiology 12 (7), 674–684. doi:10.1089/ast.2011.0685
Murphy, N. P., Breed, M. F., Guzik, M. T., Cooper, S. J. B., and Austin, A. D. (2012). Trapped in desert springs: Phylogeography of Australian desert spring snails. J. Biogeogr. 39 (9), 1573–1582. doi:10.1111/j.1365-2699.2012.02725.x
Nekola, J. C. (1999). Paleorefugia and neorefugia: The influence of colonization history on community pattern and process. Ecology 80 (8), 2459–2473. doi:10.1890/0012-9658(1999)080[2459:PANTIO]2.0.CO;2
Ollier, C. D. (1982). The great escarpment of eastern Australia tectonic and geomorphic significance. Aust. J. Earth Sci. 29, 13–23. doi:10.1080/00167618208729190
Paulson, E. L., and Martin, A. P. (2019). Inferences of environmental and biotic effects on patterns of eukaryotic alpha and beta diversity for the spring systems of Ash Meadows, Nevada. Oecologia 191 (4), 931–944. doi:10.1007/s00442-019-04526-6
Perez, E. P., Ponder, W. F., Colgan, D. J., Clark, S. A., and Lydeard, C. (2005). Molecular phylogeny and biogeography of spring-associated hydrobiid snails of the Great Artesian Basin, Australia. Mol. Phylogenet. Evol. 34, 545–556. doi:10.1016/j.ympev.2004.11.020
Phillips, F. M. (2008). “Geological and hydrological history of the paleo-Owens River drainage since the late Miocene,” in Late cenozoic drainage history of the southwestern Great Basin and lower Colorado river region: Geologic and biotic perspectives. Special paper 439 (Geological Society of America), 115–150.
Pinkava, D. J. (1984). Arizona-Nevada Academy of Sciences vegetation and flora of the Bolson of Cuatro Cienegas region, Coahuil, Mexico: IV. Summary, endemism and corrected catalogue. J. Arizona-Nevada Acad. Sci. 19 (1), 23–47.
Ponder, W. F., Eggler, P., and Colgan, D. J. (1995). Genetic differentiation of aquatic snails (Gastropoda: Hydrobiidae) from artesian springs in and Australia. Biol. J. Linn. Soc. 56 (4), 553–596. doi:10.1016/0024-4066(95)90004-7
Ponder, W. F., Hershler, R., and Jenkins, B. (1989). An endemic radiation of Hydrobiidae from artesian springs in northern south Australia: Their taxonomy, physiology, distribution and anatomy. Malacologia 31 (1), 1–140.
Ponder, W. F., and Slatyer, C. (2007). “Freshwater molluscs in the Australian arid zone,” in Animals of arid Australia: Out on their own? Editors C. Dickman, D. Lunney, and S. Burgin (Royal Zoological Society of NSW), 1–13.
Ponder, W. F., Zhang, W. H., Hallan, A., and Shea, M. E. (2019). New taxa of Tateidae (Caenogastropoda, Truncatelloidea) from springs associated with the Great Artesian Basin and Einasleigh Uplands, Queensland, with the description of two related taxa from eastern coastal drainages. Zootaxa 4583 (1), zootaxa.4583.1.1–67. doi:10.11646/zootaxa.4583.1.1
Priestley, S. C., Karlstrom, K. E., Love, A. J., Crossey, L. J., Polyak, V. J., Asmerom, Y., et al. (2018). Uranium series dating of Great Artesian Basin travertine deposits: Implications for palaeohydrogeology and palaeoclimate. Palaeogeogr. Palaeoclimatol. Palaeoecol. 490, 163–177. doi:10.1016/j.palaeo.2017.10.024
Rossini, R. A., Fensham, R. J., Stewart-Koster, B., Gotch, T., and Kennard, M. J. (2018). Biogeographical patterns of endemic diversity and its conservation in Australia’s artesian desert springs. Divers. Distib. 24 (9), 1199–1216. doi:10.1111/ddi.12757
Rossini, R. A., Fensham, R. J., and Walter, G. H. (2017a). Spatiotemporal variance of environmental conditions in Australian artesian springs affects the distribution and abundance of six endemic snail species. Aquat. Ecol. 51 (4), 511–529. doi:10.1007/s10452-017-9633-4
Rossini, R. A., Tibbetts, H. L., Fensham, R. J., and Walter, G. H. (2017b). Can environmental tolerances explain convergent patterns of distribution in endemic spring snails from opposite sides of the Australian arid zone? Aquat. Ecol. 51 (4), 605–624. doi:10.1007/s10452-017-9639-y
Sackett, J. D., Huerta, D. C., Kruger, B. R., Hamilton-Brehm, S. D., and Moser, D. P. (2018). A comparative study of prokaryotic diversity and physicochemical characteristics of Devils Hole and the Ash Meadows fish conservation facility, a constructed analog PLoS ONE 13 (3), 1–21. doi:10.1371/journal.pone.0194404
Sağlam, İ. K., Baumsteiger, J., and Miller, M. R. (2017). Failure to differentiate between divergence of species and their genes can result in over-estimation of mutation rates in recently diverged species. Proc. R. Soc. B Biol. Sci. 284 (1860), 20170021–20170023. doi:10.1098/rspb.2017.0021
Sağlam, İ. K., Baumsteiger, J., Smith, M. J., Linares-Casenave, J., Nichols, A. L., O’Rourke, S. M., et al. (2018). Best available science still supports an ancient common origin of Devils Hole and Devils Hole pupfish. Mol. Ecol. 27 (4), 839–842. doi:10.1111/mec.14502
Schweickert, R. A., and Lahren, M. M. (1997). Strike-slip fault system in Amargosa Valley and yucca mountain, Nevada. Tectonophysics 272 (1), 25–41. doi:10.1016/S0040-1951(96)00274-0
Scoppettone, G. D., Johnson, D. M., Hereford, M. E., Rissler, P., Fabes, M., Salgado, A., et al. (2012). Relative abundance and distribution of fishes and crayfish at ash meadows national wildlfie Refuge, Nye county, Nevada, 2010-11. Open-File Report 2012-1141. U.S. Geological Survey.
Shipley, B. R., and McGuire, J. L. (2022). Interpreting and integrating multiple endemism metrics to identify hotspots for conservation priorities. Biol. Conserv. 265, 109403. doi:10.1016/j.biocon.2021.109403
Souza, V., and Eguiarte, L. E. (2022). Cuatro Ciénegas Basin: An endangered Hyperdiverse Oasis. Springer.
Souza, V., Espinosa-Asuar, L., Escalante, A. E., Eguiarte, L. E., Farmer, J., Forney, L., et al. (2006). An endangered oasis of aquatic microbial biodiversity in the Chihuahuan desert. Proc. Natl. Acad. Sci. U. S. A. 103 (17), 6565–6570. doi:10.1073/pnas.0601434103
Stebbins, G. L., and Major, J. (1965). Endemism and speciation in the California flora. Ecol. Monogr. 35 (1), 1–35. doi:10.2307/1942216
Stevens, L. E., Johnson, R. R., and Estes, C. (2020). “The watershed continuum: A conceptual fluvial-riparian ecosystem model,” in Riparian research and management: Past, present, future. U.S. Department of agriculture, forest service, rocky mountain research station general technical Report RMRS-GTR-303. Editors R. R. Johnson, S. W. Carothers, D. M. Finch, K. J. Kingsley, and J. T. Stanley (U.S. Department of Agriculture), Vol. 2, 80–137.
Stevens, L. E., and Meretsky, V. J. (2008). Aridland springs in North America: Ecology and conservation. The University of Arizona Press and The Arizona-Sonora Desert Museum.
Thomas, J. M., Welch, A. H., and Dettinger, M. D. (1996). Geochemistry and isotope hydrology of representative aquifers in the Great Basin Region of Nevada, Utah, and adjacent states. Regional aquifer-system analysis-Great Basin Nevada-Utah U.S. Geological Survey Professional Paper 1409-C.
Toon, A., Sacre, E., Fensham, R. J., and Cook, L. G. (2018). Immigrant and native? The case of the swamp foxtail Cenchrus purpurascens in Australia. Divers. Distib. 24, 1169–1181. doi:10.1111/ddi.12751
Winograd, I. J., Riggs, A. C., and Coplen, T. B. (1998). The relative contributions of summer and cool-season precipitation to groundwater recharge, Spring Mountains, Nevada, USA. Hydrogeol. J. 6 (1), 77–93. doi:10.1007/s100400050135
Wolaver, B. D., Crossey, L. J., Karlstrom, K. E., Banner, J. L., Cardenas, M. B., Gutiérrez Ojeda, C., et al. (2013). Identifying origins of and pathways for spring waters in a semiarid basin using He, Sr, and C isotopes: Cuatrociénegas Basin, Mexico. Geosphere 9 (1), 113–125. doi:10.1130/GES00849.1
Wolaver, B. D., and Diehl, T. M. (2011). Control of regional structural styles and faulting on Northeast Mexico spring distribution. Environ. Earth Sci. 62 (7), 1535–1549. doi:10.1007/s12665-010-0639-7
Wolaver, B. D., Sharp, J. M., Rodriguez, J. M., and Ibarra Flores, J. C. (2008). Delineation of regional arid karstic aquifers: An integrative data approach. Ground Water 46 (3), 396–413. doi:10.1111/j.1745-6584.2007.00405.x
Keywords: endemism, neo-endemic, paleo-endemic, springs, wetlands
Citation: Fensham RJ, Ponder WF, Souza V and Stevens LE (2023) Extraordinary concentrations of local endemism associated with arid-land springs. Front. Environ. Sci. 11:1143378. doi: 10.3389/fenvs.2023.1143378
Received: 12 January 2023; Accepted: 24 May 2023;
Published: 12 June 2023.
Edited by:
Kirsten Work, Stetson University, United StatesReviewed by:
Marco Cantonati, University of Bologna, ItalyMichelle Guzik, University of Adelaide, Australia
Copyright © 2023 Fensham, Ponder, Souza and Stevens. This is an open-access article distributed under the terms of the Creative Commons Attribution License (CC BY). The use, distribution or reproduction in other forums is permitted, provided the original author(s) and the copyright owner(s) are credited and that the original publication in this journal is cited, in accordance with accepted academic practice. No use, distribution or reproduction is permitted which does not comply with these terms.
*Correspondence: Roderick J. Fensham, rod.fensham@qld.gov.au