- 1DSI/Mintek Nanotechnology Innovation Centre, Advanced Materials Division, Mintek, Randburg, South Africa
- 2Department of Chemical Engineering, Faculty of Engineering and the Built Environment, University of Johannesburg, Doornfontein, South Africa
- 3Department of Quality Operations Management, Faculty of Engineering and the Built Environment, University of Johannesburg, Doornfontein, South Africa
- 4Council for Scientific and Industrial Research (CSIR), Holistic Climate Change-Climate Services Group, Pretoria, South Africa
- 5Department of Chemical Engineering, School of Mines and Mineral Sciences, Copper Belt University, Kitwe, Zambia
Across the world population growth, expansion of economic activities and climate change have become a concern for future water supply. To address the issue, many countries are investigating strategies to augment current water supplies. Water reclamation has been identified as a plausible sustainable solution to meet potable water supply demand, in turn achieving SDG 6.3. This study identifies some of the critical success factors for consideration of municipal waste water reclamation. This was undertaken in the growing township of Diepsloot, in Johannesburg (South Africa). Diepsloot is densely populated with over 350,000 people as recorded in 2022. As a result, water shortages are common due to insufficient bulk water facilities to supply the area. A direct potable water reclamation plant from treated municipal wastewater has been proposed to augment the water supply. Aqueous Material Balance (AqMB)®, a process modelling simulator software for water treatment processes to predict water quality and quantity, was used to design and simulate the water reclamation plant process. Our findings show that, the quantity and quality of water, as well as the choice of treatment technology are key. The simulations treatment process proposed here-in indicated successful removal of the contaminants to acceptable SANS 241:2015 drinking water standards. The variation in seasonal feed data did not show any difference in the performance of the proposed process. Furthermore, the plant has the potential to provide 109 L/day of clean water per person for a population of 350,000. Therefore, direct potable water reclamation shows great potential to augment current water supply to support growing populations where natural water sources are scarce.
1 Introduction
Potable clean water supply has remained one of the major global challenges of the 21st Century. Many cities are grappling to meet the ever rising demand. Water is a required natural resource for human development and ecosystems survival (Macedonio et al., 2012; Zeng et al., 2013). However; with the struggle between water demand and supply, the 2018 Sustainable Development Goals (SDG) report indicates that there are still too many people without access to safely managed water supply and sanitation. The United Nations (UN) in 2015 reported that a global population of 29% lacked access to drinking water supply that is safely managed. Furthermore, if current water challenges trends continue; such as population growth, consumption, pollution, and climate change, there is a possibility that the world will face water deficit of about 40% by 2030 (WWAP, 2015; UN, 2018). In order to meet the water demand for the future, alternative ways of water supply have been identified, such as more utilisation of groundwater, desalination of seawater and wastewater re-use (i.e., water reclamation) (DWS, 2013). Water reclamation is a process of transforming treated wastewater discharge into water that can be reused for different activities such as human consumption, irrigation, and recreation. It releases pressure on conventional water sources such as groundwater and surface water (Khan, 2013; Estevez-Olea, 2015). A number of countries have successfully implemented water reclamation for non-potable (Australia, Israel, Singapore) and potable (Namibia, South Africa, Unites States of America) reuse (USA, 2019). Moreover, in communities where bulk or centralized water supply does not meet the demand due to population expansion, such as in growing urban and peri-urban areas, water reclamation can be useful in supporting decentralization of water supply by augmenting the existing centralized supply.
1.1 Drivers for water reclamation
Water scarcity is one of the drivers of water reclamation. Freshwater scarcity has become a major threat and a limiting factor towards socio-economic and sustainable development to some parts of the world. Water scarcity occurs when water availability falls below 1,000 m3 per capita per year, while absolute scarcity occurs when water availability falls below 500 m3 per capita per year. Water scarcity has been reported to affect approximately 2.4 billion people, or 36% of the global population. If alternative water supply systems or appropriate measures are not implemented, 52% of the world’s population will face severe water scarcity by 2050 (Wang et al., 2014a; Mekonnena and Hoekstra, 2016; Liu et al., 2017). Climate change is another common driver towards the implementation of water reclamation systems. Climate change has changed the world’s hydrological cycles mostly because of changes in temperature and precipitation, which could lead to even more severe water shortages. Temperature rises are likely to have an impact on evapotranspiration and atmospheric water storage, potentially altering the magnitudes, frequencies, and intensities of rainfall, as well as its seasonal and inter-annual variability, and geographical distribution (Wang et al., 2014a; Du et al., 2021). Water availability also contributes towards the implementation of water reclamation systems, the world’s total renewable freshwater resources available (surface water and groundwater) for the environment and human use is 45,000 km3 annually. Roughly 6,000 m3 annually of water per inhabitant is available, if only the available water was to be divided equally amongst the inhabitants. However; due to increase in water withdrawal and consumption over the last decades globally, the current human demand has exceeded the available renewable approximately by a factor of 10 (Oelkers et al., 2011).
1.2 Types of potable water reclamation systems
Potable water reclamation is a system that is used to remove contaminants and pollutants from the treated wastewater discharge through advanced water treatment processes with the aim of producing water that meets drinking water standards. These systems can be oriented into three different types, namely,: De Facto Potable Water Reclamation (DFPWR), Indirect Potable Water Reclamation (IPWR), and Direct Potable Water Reclamation (DPWR). DFPWR is an unplanned water reclamation process. This occurs when the municipal wastewater treatment plant discharges the treated effluent into the river or ocean that is used as a supplying source of drinking water. IPWR is a type of water reclamation in which municipality treats wastewater to an advanced stage with the purpose of expanding drinking water supply. The reclaimed water is stored in environmental buffers such as rivers, reservoirs, aquifers or lakes prior to further treatment at the water treatment plant (Rodriguez et al., 2009; Khan, 2013; EPA, 2017; Warsinger et al., 2018). DPWR is a planned water reclamation system in which treated municipal wastewater effluent is discharged directly into a water reclamation plant for potable water treatment instead of being discharged into a river or groundwater. The reclaimed water is then blended with conventional sources of water such as groundwater or surface water before distribution to consumers. Since DPWR does not require environmental buffers, furthermore the cost of transporting and combining treated water with other drinking sources is lower compared to IPWR. As a result, DPWR might be more cost-effective than IPWR. This type of reclamation has been implemented with great success in Windhoek’s Goreangab, Big Spring Texas, and Beaufort West (Leverenz et al., 2011; Khan, 2013; EPA, 2017; Landsteiner et al., 2018).
1.3 Study area
1.3.1 Location and demographic characteristics
Diepsloot Township (Figure 1), situated in Gauteng province of South Africa, is a low-income settlement established in 1994 initially as a relocation area for people who were moved from an informal settlement in Zevenfontein and in Alexander Township, Johannesburg. It is situated in the north of Johannesburg, and the Jukskei River which feeds into the Crocodile River passes through Diepsloot Township. The township is densely populated, with over 350,000 people (Sobantu and Nel., 2019; Diepsloot Youth Programme, 2023; World Population Review, 2023).
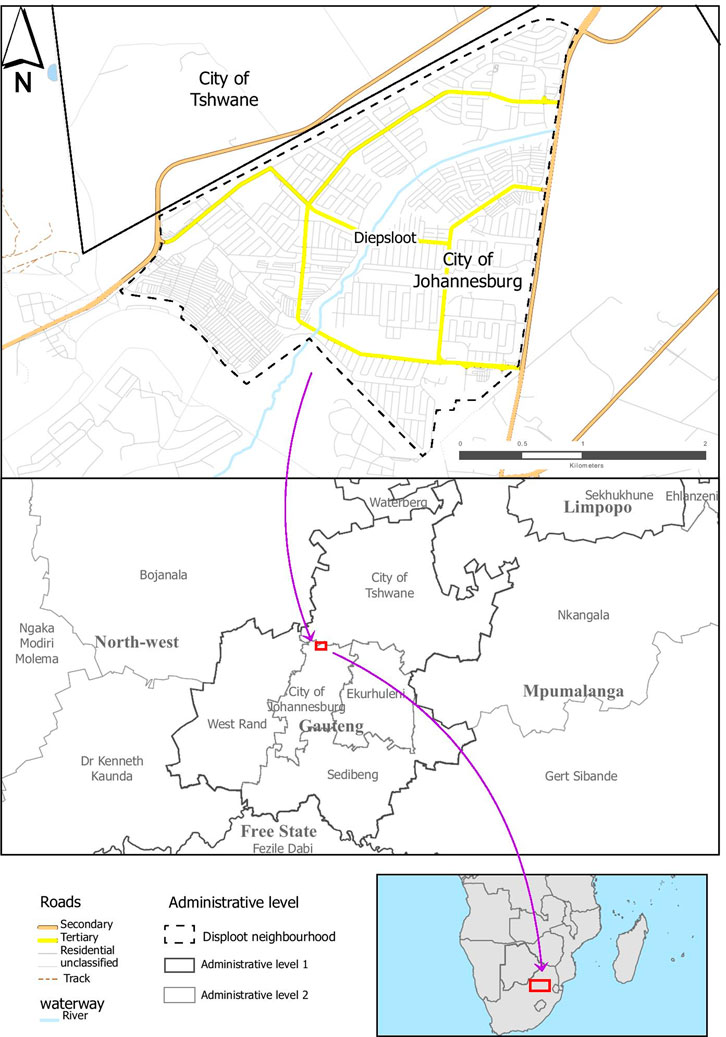
FIGURE 1. Map of Diepsloot Township (Sesan et al., 2022).
1.3.2 Water supply
Water shortages are common due to insufficient bulk water facilities to supply the area. Some residence experience water supply interruptions which can extend for longer than 2 days (Himlin et al., 2008; Tshililo et al., 2022). Rand Water is a water utility that provides potable water to the Gauteng province and parts of Mpumalanga, Free State, and North West provinces. In the study area, the primary bulk water supply is supplied by Rand Water and distributed by Johannesburg Water (GAPP, 2020; Wikipedia, 2022). Apart from the centralized water supplied by Rand Water, other obvious water sources that can be considered for decentralized water supply in the study area are the groundwater and Jukskei River. The quantity of these sources must be known in order for one to be able to assess the possibility of their utilization as sustainable sources for water supply. Table 1 shows the quantity of water per annum in A2H023 site; which is one of the Department of Water and Sanitation monitoring sites along the Jukskei River. The monitoring site is situated at Nietgedacht, 7 km away from the study area. The data shows that the quantity of water available may not be adequate for future demand, as a portion of water available in Jukskei River is used by the downstream users (Magalies Water Board) which draws water from Hartbeespoort dam (Sibali et al., 2008).
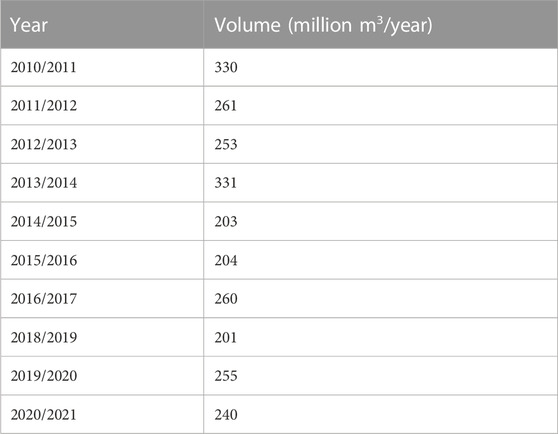
TABLE 1. Quantities of water in A2H023 site along the Jukskei River (DWS, 2022a).
1.3.3 Groundwater
Groundwater is one of the freshwater resources that is accessible and used as a source of water supply for domestic use and irrigation. It is a major source of drinking water in Africa, with nearly 75% of the population depending on groundwater. In recent years, the over-exploitation of groundwater in some parts of South Africa has become a serious challenge. As a result, groundwater levels are declining and will require to be recharged (Taylor et al., 2013; Wang et al., 2014b; DWS, 2022b). Figure 2 shows the groundwater status in Gauteng province reported by Department of Water and Sanitation in May 2022. It was observed that the volumes of water abstracted was higher than those of the groundwater available, implying that groundwater may not be adequate as the sole source for a sustainable water supply.
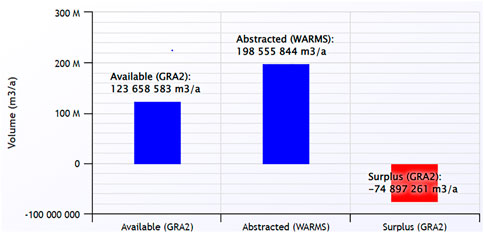
FIGURE 2. Groundwater quantity status in Gauteng Province (Groundwater Resource Assessment (GRA) and Water use Authorization & Registration Management System (WARMS) (DWS, 2022b).
1.3.4 Wastewater treatment works
Diepsloot was chosen as the research site for this study because it is densely populated, water shortages are common, and also due to its close proximity to a Wastewater Treatment Works (WWTW). The Northern Wastewater Treatment Works (NWWTW), operated by Johannesburg Water, is located 2 km outside Diepsloot and is the largest wastewater treatment plant in the City of Johannesburg Municipality. The plant treats over 400,000 m3 of municipal wastewater on a daily basis. A portion of the discharge is used for irrigation in some of the nearby farm lands, while another portion is pumped to a nearby coal-operated Power Station (Prinsloo, 2008). The remaining effluent (estimated at 220,000 m3/day) is discharged into the Jukskei River. The majority of the sewage collected and treated at NWWTW comes from residential areas in Alexandra, Sandton, Randburg, and the northern parts of Johannesburg. The treatment steps followed at the NWWTW are summarized in Figure 3. The sewage enters the pre-treatment stage to eliminate any foreign material or bigger rubbish such as paper and plastic. Followed by the balancing tank which acts as a buffer against significant changes in inflow volume and contaminant concentration. This stage is followed by separation of suspended solids and any floating particles such as oils, fats and grease in primary clarifiers. The effluent is sent to biological reactor to remove nitrogen and phosphorus. Further removal is done in the secondary treatment to remove dissolved and suspended organic solids. Lastly, disinfection is done to eliminate pathogens, viruses and bacteria. The secondary effluent is then discharged into the Jukskei River (Mather and Shaw, 1993; Amdany et al., 2014; Naidoo and Olaniran, 2014; Jwara et al., 2020; Makoane, 2012). The typical effluent quality of this plant is reported in Table 2, the results of the analyses is provided by the Department of Water and Sanitation. The effluent quality shows that further treatment will be required in order for the water to meet SANS 241:2015 drinking water standards.
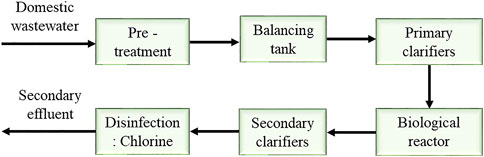
FIGURE 3. Treatment process at NWWTW (Makoane, 2012).
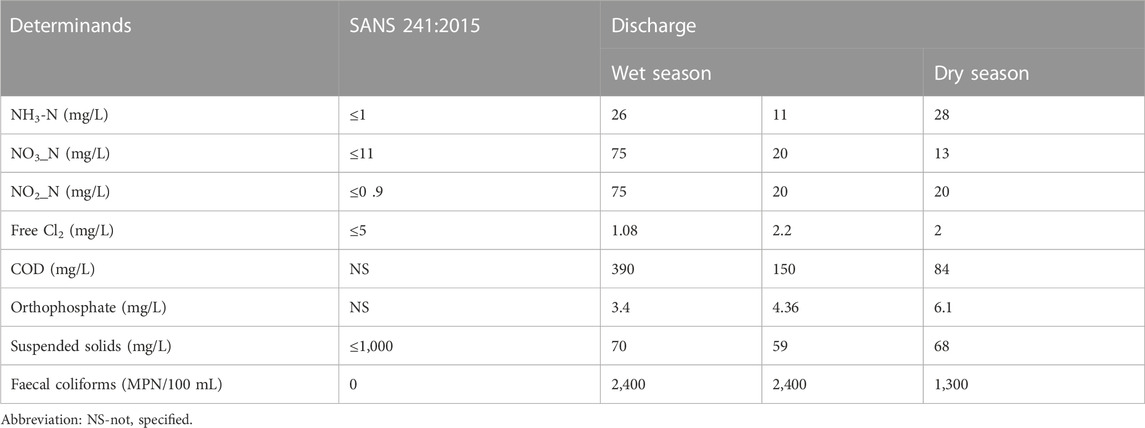
TABLE 2. Water quality of NWWTW discharge into the Jukskei River (DWS, 2022c).
For this study, a direct water reclamation plant using the treated wastewater discharge from the NWWTW as the feed is proposed. Thus the aim of this work was to assess the feasibility of direct water reclamation from treated municipal wastewater discharge for supply of potable water to Diepsloot Township. A stepwise sequential approach was used to: i) Assess quality of treated wastewater discharge for reuse, ii) Investigate the required technology options for direct water reclamation for potable reuse, and iii) Assess capacity of the treatment plant. Feasibility studies of reclamation of treated municipality wastewater for potable reuse, have in South Africa been carried for Western Cape and KwaZulu Natal provinces, and have since been successfully implemented for bulk water supply (Swartz and Menge, 2022). While only one study has been reported for the semi-arid Gauteng province, the study which was based on assessment of the viability of non-potable reuse of treated municipal wastewater discharge (Skosana, 2017) and not on potable re-use. To the best of our knowledge, this is the first study on feasibility of water reclamation for potable reuse, to be reported for the semi-arid Gauteng Province of South Africa, for the benefit of township communities potentially through sustainable decentralized water supply. The study proposes a suite of treatment technologies, backed by simulations results generated from real municipality secondary effluent quality data.
2 Methods
2.1 Sampling and analysis
Treated municipal wastewater discharge has the remains of organic micro contaminants, including personal care products, pharmaceutically active compounds, and pesticides since the conventional treatments do not remove them entirely. Their presence becomes a major concern when considering water reclamation (Fatta-Kassinos et al., 2016). Hence, the quality of the discharge from the WWTW should be examined as it plays a major role in selecting the appropriate treatment technology protocol. In this study samples were collected from the Jukskei River during wet season, to determine the quality of the feed for water reclamation plant. Four sampling points were chosen; two downstream of the NWWTW (Heron Bridge College and Lion Park Quarry) and another two upstream (William Nicol South and Lonehill Main Road). The GPS coordinates of the sampling points are presented in Supplementary Material (as Supplementary Table S1), while Figure 4 presents the maps of the sampling sites.
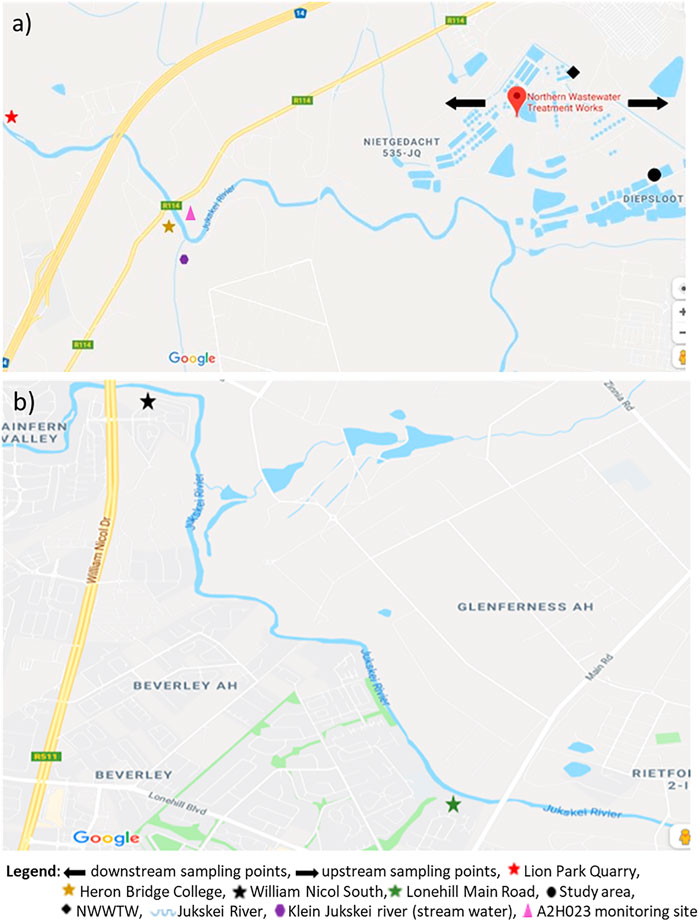
FIGURE 4. A map showing the location of the water sampling sites along the Jukskei River: (A) Downstream and (B) Upstream of NWWTW (Google maps, 2022).
In addition to the sampling locations, the maps in Figure 4 also show the relative location of NWWTW within the study area. The Department of Water and Sanitation sampling procedure was followed (WRC, 2003). Two samples were collected at each sampling point; one sample for microbial analysis were collected first using sterile glass bottles and then followed by the collection of a sample for chemical analysis (in plastic bottles). The samples were stored at below 4°C analysed within 6 h of their collection. The water quality was compared to the South African National Standard (SANS) 241:2015 for drinking water quality.
On-site measurement of pH, electrical conductivity, temperature, and turbidity of the samples were conducted at the sampling points. Turbidity was measured using Eutech instruments Turbidimeter TN-100, while pH and electrical conductivity were measured using Eutech instruments Cyberscan PC 300. Chemical analyses were conducted using Automated Photometric, Inductively Coupled Plasma Optical Emission Spectroscopy (ICP- OES), and Inductively Coupled Plasma Mass Spectrometry (ICP-MS).
2.2 Treatment process modelling
Aqueous Material Balance (AqMB)®, a process modelling simulator software for water treatment processes to predict water quality and quantity was used. This software includes a wide range of unit operations that are utilised for designing water treatment process such as clarifier, membrane separators, adsorption unit, etc. (Scott et al., 2019). According to the World Health Organization (WHO, 2013), a person requires a minimum of 70 L/day of clean water to meet basic needs, such as drinking, cooking, personal washing, laundry, cleaning home, growing food (domestic use) and for waste disposal (sanitation). Thus, the proposed treatment plant must have a design capacity of 35,808 m3/day to supply a population of 350,000 with 100 L/day per person. The proposed value of 100 L/day per person was based on hierarchy of water requirements developed by the WHO as a guideline to determine the minimum amount of water required per person per day.
3 Results and discussion
3.1 Water quality analysis of collected samples
Samples were collected at Jukskei River to determine other contaminants that were not reported by Department of Water and Sanitation. A visual presentation of the samples is shown in Figure 5. There was a visible difference in the colour of the water upstream and downstream of the NWWTW. The samples collected downstream contained suspended solids giving them a brownish cloudy appearance, while those collected upstream appeared clearer. The presence of the suspended solids and the resultant colour in the samples collected downstream can be attributed to the presence of the effluent discharge from the NWWTW and possibly from other activities in the surrounding areas.
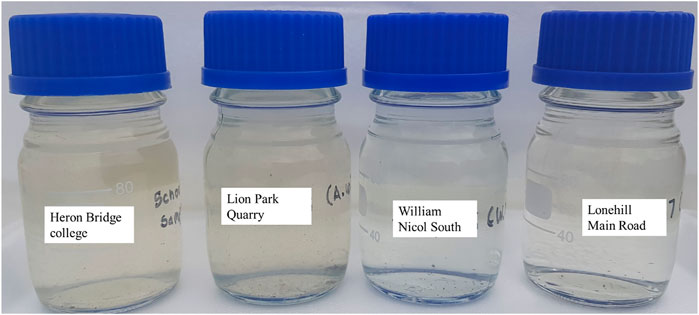
FIGURE 5. Physical appearance of the Jukskei River samples collected downstream (Heron Bridge College and Lion Park Quarry) and upstream (William Nicol South and Lonehill Main Road) of the NWWTW.
Table 3 shows the physical, chemical and microbial analyses results of the river samples. The results indicate that the samples collected downstream were found to have elevated levels of turbidity and electrical conductivity when compared to the upstream samples, in line with the physical appearance of the samples as presented in Figure 5. Nevertheless, the pH and the electrical conductivity of all the samples were found to be within SANS 241:2015 limits. The upstream and downstream samples seemed to have more or less same levels of most of the chemical determinands, with the exception of NO2_N and Fe which were both found to be higher in the downstream samples. The higher levels of these determinands in the downstream samples stem from the NWWTW discharge as ferric chloride is usually used in coagulation during municipal wastewater treatment, while nitrate and nitrite are generally from the agriculture run offs (How et al., 2021). Nevertheless, most of the chemical determinands were observed to be within SANS 241:2015 limits for all samples collected (except for NO2_N in the downstream samples). A more striking difference was observed in the microbial analysis as significant levels of faecal coliforms were obtained downstream, which is attributed to the discharge from the NWWTW. According to the results in Table 2, it can be observed that the faecal coliform levels discharged into the river is similar to those found from the samples collected downstream of the NWWTW. However, the concentrations of nitrate and nitrite were higher compared to those found in the downstream samples reported in Table 3. The lower nitrate and nitrite concentrations can be due to dilution of the discharge by the stream water (Figure 4), and additionally due to removal by aquatic or algae plants in the river (Andersen et al., 2004). Therefore, the water quality of the discharge is similar to that of the water downstream, although it can be argued from the results presented herein that allowing the discharge to be in contact with the natural environment in the river before reclamation is done may be advantageous. As a result, the quality of the feed water for reclamation can be expected to be the same as that of the downstream samples.
Water reclamation for potable reuse generally requires blending with conventional water sources. According to Ketteringham (2007), it is a requirement from the South African Department of Health to blend the reclaimed water with any other conventional sources of water, such as surface or groundwater, at a ratio of 1 part reclaimed water: 4 parts conventional source water before exposure for human consumption. The purpose of blending is to dilute total dissolved solids and anthropogenic dissolved organic carbon. Moreover, blending is conducted for psychological reasons (mainly public acceptance). The blending process can be done pipe-to-pipe in the distribution network or in a storage tank. It is generally recommended to implement blending in a storage tank process since it is safer and more efficient, than a pipe-to-pipe blending (Lahnsteiner et al., 2018). Due to low volumes of fresh water resources within the vicinity of the study location, groundwater was chosen as a blending source. The quality of the ground water in the vicinity of Diepsloot is presented in Supplementary Material (Supplementary Table S2), as reported by Kruidenier (2019). The results show the determinands are within SANS 241:2015 limits, which implies the groundwater may be suitable for blending of reclaimed water.
3.2 Treatment technology protocol
3.2.1 Selection of treatment technologies
The choice of technologies to be used and the level of treatment are governed by the contaminants of concern in the area, the quality of the treated wastewater discharge, intended end uses of the reclaimed water, and regulations. Potable reuse requires advanced water treatment technologies (Hummer and Eden, 2016). The main purpose of advanced treatment technologies is to advance the removal of the remaining microorganisms, nutrients, pollutants, and suspended solids in the feed. Hence, when designing a direct potable reuse treatment train, multiple processes that have equivalent ability to remove contaminants must be employed. In case of failure from one process to remove certain contaminants, the downstream processes need to be able to remove them down to acceptable limits. Furthermore, the system needs to be robust and resilient to increase the capability of addressing a wide range of contaminants. Water reclamation treatment train for potable reuse typically consists of multiple barriers combined to remove pathogens and organics. For the removal of pathogens, the following multiple barriers are normally combined: coagulation, disinfection (chlorine or ultraviolet [UV]), filtration (granular or membrane) and softening. Furthermore, the organics are removed by combined multiple barriers of advanced treatments processes such as Reverse Osmosis (RO) membrane, granular activated carbon, advanced oxidation processes (ozone, and UV) etc. (Schimmoller et al., 2015; Roccaro, 2018). From the water quality data reported of NWWTW and downstream samples of NWWTW, it can be noted disinfection of the feed and further treatment will need to be conducted to keep turbidity, faecal coliforms, and nitrates below the SANS 241:2015 limits. This was therefore used as a guideline to propose the appropriate treatment protocol for the water reclamation plant and the choice of technology to use for production of potable water.
3.2.2 Treatment protocol and design data for water reclamation plant
A summary of simulated main unit operations design data are shown in Table 4. The proposed water reclamation plant consists of two inlets streams (feed water from the WWTW and another feed from the groundwater) as shown in Figure 6. The two inlet feed streams feed into clarifiers separately, whereby alum and polymer are added to enhance the settling of suspended solids. The clarifier is applied to remove turbidity, suspended solids, protozoa, viruses, and bacteria (Chaitra et al., 2017; Omarova et al., 2018). The Ultrafiltration (UF) membrane is intended for removing suspended solids, bacteria, turbidity, colloidal contaminants, protozoa, and viruses (Bergamasco et al., 2011). Furthermore, UF is used as a pretreatment stage for RO membrane. The treatment train included RO since the technology is considered one of the best in removing contaminants such as emerging organic contaminants, pharmacologically active components, personal care products, dissolved solids, mono and divalent ions, and trace organics from the municipal wastewater (Aravinthan, 2014; Roccaro, 2018). The advanced treated secondary effluent was mixed with the treated groundwater for blending purposes using a mixing ratio of 1:4. The final stage of the treatment train is disinfection using ozone, with the purpose of eliminating residual pathogenic organisms that may still be present in the water (Somani and Ingole, 2011).
3.3 Treatment plant water quality
3.3.1 Process capability assessment on secondary effluent from NWWTW
The water quality data reported for NWWTW for wet season and the composition of the water samples collected downstream of NWWTW were used as a guideline to model the water reclamation plant. The proposed treatment process was designed to treat effluent from NWWTW and groundwater to acceptable limits of SANS 241:2015 drinking water standards. The results generated by the software after simulation of the proposed treatment process are shown in Table 5. Contaminants that do not meet SANS 241:2015 requirements; such as turbidity, nitrites, and bacteria are highlighted in red. It was observed that the clarifier was able to remove turbidity completely and remove the bacteria to 99.68%, the remaining 0.32% of the bacteria was removed by the UF membrane. Lastly, 99.67% of nitrites were removed by the RO membrane. Furthermore, it was observed that the clarifier was able to remove the bacteria completely from groundwater (Supplementary Table S3). The treatment process proposed here-in successfully removed the contaminants to acceptable SANS 241:2015 drinking water standards. Furthermore, it was noticed that after passing the treated feed through the RO membrane most of the minerals were removed (Supplementary Table S3). The removals of these caused the water to be demineralised and not ideal for drinking water purposes, as a result remineralisation process will need to be done after RO process. However, for this study remineralisation process will not be required since reclaimed water is expected to be blended with groundwater (Khan, 2013; Rosborg and Kozisek, 2019).
The performance of the proposed process was assessed for season variability (Supplementary Table S4) using water quality data for NWWTW collected during dry season. The feed showed elevated levels of NH3, NO3 and NO2 when compared to the SANS 241: 2015 and the feed for wet season. Furthermore, the dry season secondary effluent contained lower levels of bacteria when compared to wet season effluent, although the levels were higher than allowed for drinking water. The proposed treatment process was still able to reduce all contaminants exceeding SANS 241:2015 requirements to acceptable levels for drinking water.
3.3.2 Process capability assessment on other secondary effluent in South Africa
Secondary effluent data from Darvill WWTW in KwaZulu Natal province (Freese et al., 2002) and Mmabatho WWTW in North West province (Akoth, 2018) were used to assess the capability of the proposed treatment process. The determinands that exceeded SANS 241:2015 requirements are highlighted in red (Supplementary Table S5, S6). The effluent from the Darvill WWTW (Supplementary Table S5) contained As, Pb, true colour, cryptosporidium, giardia, and viruses; which in were not found or reported in the secondary effluent data from the NWWTW, and were found in considerable amounts. On the other hand, the secondary effluent from Mmabatho WWTW (Supplementary Table S6) contained the highest level of NO3 in this study, and significantly high levels of bacteria (almost twice as much as reported in the NWWTW effluent). Regardless of the disparity of the levels of these contaminants, the proposed treatment process was able to reduce all contaminants exceeding SANS 241:2015 requirements down to acceptable levels for drinking water.
3.4 Treatment plant capacity
The simulated treatment plant was able to produce 1,584 m3/h of treated water as presented in (Supplementary Table S3), which is 38,016 m3/day as shown in Figure 7. The volume of treated water per person per day was calculated using Eq. 1
The plant will be able to provide 109 L/day of clean water per person for a population of 350,000.
4 Conclusion
Based on water shortages or scarcity experienced worldwide, particularly in South Africa over the recent years, water reclamation presents a sustainable, reliable and promising future. Water reclamation presents many advantages such as the release of pressure on water sources, and reduction in energy cost for water transportation or transfer. Direct potable water reclamation shows great potential as an alternative of water supply; this practice has been operated successfully at the New Goreangab (Namibia), Beaufort West (South Africa), and Big Spring (United States) Water Reclamation Plants. The proposed here-in water reclamation plant will require the following treatment processes such as clarification, membrane filtration (UF and RO), and disinfection to improve the water quality to SANS 241:2015 drinking water standards. Based on the results obtained after the simulation process, the treatment process proposed here-in was able to remove contaminants to acceptable levels as guided by SANS 241:2015. Moreover, the simulated treatment plant will be able to produce 38,016 m3/day, providing 109 L/day of clean water per person for a population of 350,000. Thus, water reclamation for direct potable reuse shows a great potential to augment the current water supply and in turn achieving SDG 6.3 goal. However, validation of the results obtained from this study with empirical data would be beneficial for a comprehensive and informed conclusion to be made. Furthermore, despite the myriad advantages of water reclamation, a cost-benefit analysis and a full economic evaluation of the proposed water reclamation plant are necessary, in addition to public buy-in and acceptance, before implementation of the proposed solution can be considered.
Data availability statement
The original contributions presented in the study are included in the article/Supplementary Material, further inquiries can be directed to the corresponding author.
Author contributions
Conceptualization: KS, KJ, SM, and JS. Data curation: KS. Formal analysis: LN, KS, KM, and KJ. Funding acquisition, KS, SM, and JS. Investigation: LN and MB. Methodology: LN, KS, KM, and KJ, Project administration: KM. Resources: KS and KM. Supervision: KS, KM, and KJ. Validation: KS, KM, and KJ. Writing–original draft: LN. Writing—review and editing: KS, KM, KJ, SM. All authors contributed to the article and approved the submitted version.
Funding
The study was funded in part by the International Science Council (ISC), Network of African Science Academics (NASAC) and the Swedish International Development and Cooperation Agency (SIDA) through the Leading Integrated Research for Agenda (LIRA) 2030 in Africa program (Grant number LIRA2030-GR06/17). Mintek provided the bursary for LN. The Department of Science and Technology/Mintek Nanotechnology Innovation Centre provided part of the funding for research activities.
Acknowledgments
The authors would like to extend gratitude to International Science Council (ISC), Network of African Science Academics (NASAC), and the Swedish International Development and Cooperation Agency (SIDA) for funding through the Leading Integrated Research for Agenda (LIRA) 2030 in Africa program. The DSI/Mintek Nanotechnology Innovation Centre and University of Johannesburg are also acknowledged for financial support and permission to publish the work.
Conflict of interest
The authors declare that the research was conducted in the absence of any commercial or financial relationships that could be construed as a potential conflict of interest.
Publisher’s note
All claims expressed in this article are solely those of the authors and do not necessarily represent those of their affiliated organizations, or those of the publisher, the editors and the reviewers. Any product that may be evaluated in this article, or claim that may be made by its manufacturer, is not guaranteed or endorsed by the publisher.
Supplementary material
The Supplementary Material for this article can be found online at: https://www.frontiersin.org/articles/10.3389/fenvs.2023.1143367/full#supplementary-material
References
Akoth, M. (2018). “Impact of wastewater effluent disposal on surface water quality in mahikeng,” in Master of science in environmental sciences and management (South Africa: North West University).
Amdany, R., Chimuka, L., and Cukrowska, E. (2014). Determination of naproxen, ibuprofen and triclosan in wastewater using the polar organic chemical integrative sampler (POCIS): A laboratory calibration and field application. Water sa. 40 (3), 407–414. doi:10.4314/wsa.v40i3.3
Andersen, C. B., Lewis, G. P., and Sargent, K. A. (2004). Influence of wastewater treatment effluent on concentrations and fluxes of solutes in the bush river, South Carolina, during extreme drought conditions. Environ. Geosci. 11 (1), 28–41. doi:10.1306/eg.10200303017
Aravinthan, V. (2014). Reclaimed wastewater as a resource for sustainable water management. ResearchGate, 1–11.
Bain, R., Johnston, R., Mitis, F., Chatterley, C., and Slaymaker, T. (2018). Establishing sustainable development goal baselines for household drinking water, sanitation and hygiene services. Water 10, 1711. doi:10.3390/w10121711
Bergamasco, R., Konradt-Moraes, L. C., Vieira, M. F., Fagundes-Klen, M. R., and Vieira, A. M. S. (2011). Performance of a coagulation–ultrafiltration hybrid process for water supply treatment. Chem. Eng. J. 166, 483–489. doi:10.1016/j.cej.2010.10.076
Chaitra, H., Aravind, H. B., and Senapati, A. (2017). Removal of total suspended solids and turbidity by actiflo ® process using micro-sand. Int. Res. J. Eng. Technol. (IRJET) 4 (7), 2964–2970.
Department of Water and Sanitation (DWS). (2022a). Hydrology data, Available at: https://www.dws.gov.za/hydrology/Verified/HyData.aspx?Station=A2H023100.00&DataType=Monthly&StartDT=1965-10-23&EndDT=2022-02-09&SiteType=RIV&Format=Old (Accessed November 30, 2022).
Department of Water and Sanitation (DWS). (2022b). National integrated water information system (NIWIS), Groundw. Availab. Status, Available at: https://www.dws.gov.za/niwis2/Ground/WaterStatus (Accessed May 10, 2022).
Department of Water and Sanitation (DWS) (2013). National strategy for water re-use. Department of water affairs, South Africa. Available at: https://www.dws.gov.za/documents/Other/Strategic%20Plan/NWRS2-Final-email-version.pdf (Accessed November 20, 2022).
Department of Water and Sanitation (DWS) (2022c). South Africa's effluent discharge into river map. Integr. Regul. Inf. Syst. (IRIS). Available at: https://ws.dws.gov.za/iris/myriver.aspx?c2VvcD0xJndvcmtzX2lkPTE5NDQ= (Accessed December 19, 2022).
Du, P., Xu, M., and Li, R. (2021). Impacts of climate change on water resources in the major countries along the belt and Road. PeerJ 9, e12201–e12221. doi:10.7717/peerj.12201
Environmental Protection Agency (EPA) (2017). 2017 potable reuse compendium. Available at: https://www.epa.gov/sites/default/files/2018–01/documents/potablereuse compendium_3.pdf (Accessed November 2, 2022).
Estevez-Olea, A. (2015). “Life cycle assessment of reclaimed water for potable and nonpotable reuse in California,” in Master's projects and capstones (San Francisco: University of San Francisco).
Fatta-Kassinos, D., Dionysiou, D. D., and Kümmerer, K. (2016). Advanced treatment technologies for urban wastewater reuse. Handb. Environ. Chem. 45, 5–38.
Freese, S. D., Nozaic, D. J., Bailey, I., and Trollip, D. L. (2002). Alternative disinfectants for wastewater effluents: Viable or prohibitively expensive? biennial conference of the water institute of southern Africa (WISA).
GAPP (2020). Greater lanseria master plan. Available at: https://www.gov.za/sites/default/files/gcis _document/202011/greater-lanseria-masterplans.pdf (Accessed December 2, 2022).
Google maps (2022). Jukskei river. Available at: https://www.google.com/maps/place/Jukskei+Rivier/@-26.0348508,27.7006112,10z/data=!3m1!4b1!4m6!3m5!1s0x1e95773d683176ef:0x22e3b9b042a8363d!8m2!3d-26.0097552!4d28.0369438!16zL20vMDZ6cGp4 (accessed August 25, 2022).
Himlin, R., Engel, H., and Mathoho, M. (2008). “Land use management and democratic governance in the city of Johannesburg,” in Case study: Kliptown and Diepsloot (Johannesburg: Wits Center for Urban and Built Environment Studies and Planacts).
How, S. W., Ting, C. X., Yap, J. Y., Kwang, C. Y., Tan, C. K., Yoochatchaval, W., et al. (2021). Effect of carbon-to-nitrogen ratio on high rate nitrate removal in an up flow sludge blanket reactor for polluted raw water pre-treatment application. Sustain. Environ. Res. 31 (16).
Hummer, N., and Eden, S. (2016). Potable reuse of water, arroyo. Tucson: University of Arizona Water Resources Research Center.
Jwara, T. Y. S., Musonge, P., and Bakare, B. F. (2020). “Chemical oxygen demand fractionation of primary wastewater effluent for process optimization and modelling,” in 18th South Africa int'l conference on agricultural (Durban: Chemical, Biological & Environmental Sciences ACBES-20), 265–271.
Ketteringham, W. (2007). Treatment of effluent to potable standards for supply from the faure water treatment plant. South Africa: Ninham Shand (Pty) Ltd, UWP Consulting (Pty) Ltd & Department of Water Affairs and Forestry.
Khan, S. (2013). Drinking water through recycling: The benefits and costs of supplying direct to the distribution system. Melbourne Victoria: Australian Academy of Technological Sciences and Engineering.
Kruidenier, J. H. B. (2019). Hydrogeological and contamination risk assessment study for the planned filling station in cosmo city extension 52, to be located on remainder of portions 98 and 182 of the farm Nietgedacht 535 Jq., located in the Gauteng province. Johannesburg: HK Geohydrological Services Pty Ltd.
Lahnsteiner, J., van Rensburg, P., and Esterhuizen, J. (2018). Direct potable reuse – a feasible water management option. IWA Publ. J. Water Reuse Desalination 8 (1), 14–28. doi:10.2166/wrd.2017.172
Leverenz, H. L., Tchobanoglous, G., and Asano, T. (2011). Direct potable reuse: A future imperative. IWA Publ. 01.1, 2–10. doi:10.2166/wrd.2011.000
Liu, J., Yang, H., Gosling, S. N., Kummu, M., Flörke, M., Pfister, S., et al. (2017). Water scarcity assessments in the past, present, and future. Earth’s Future 5, 545–559. doi:10.1002/2016ef000518
Macedonioa, F., Drioli, E., Gusev, A. A., Bardowe, A., Semiat, R., and Kurihara, M. (2012). Efficient technologies for worldwide clean water supply. Chem. Eng. Process. 51, 2–17. doi:10.1016/j.cep.2011.09.011
Makoane, R. (2012). Overview of northern wastewater works wastewater treatment processes used at N works, Johannesburg: Johannesburg Water Pty Ltd, Available at: https://www.ais.unwater.org/ais/pluginfile.php/231/mod_page/content/188/Session4_Overview_Of_NorthernWastewaterWorks.pdf (Accessed December 19, 2022).
Mather, A. J., and Shaw, I. S. (1993). Alternative method for the control of balancing tank at a wastewater treatment plant, Water Sci. Technol., 28 (11-12), 523–530. doi:10.2166/wst.1993.0692
Mekonnena, M. M., and Hoekstra, A. Y. (2016). Four billion people facing severe water scarcity. Sci. Adv. 2 (2), e1500323–e1500326. doi:10.1126/sciadv.1500323
Naidoo, S., and Olaniran, A. O. (2014). Treated wastewater effluent as a source of microbial pollution of surface water resources. Int. J. Environ. Res. Public Health 11, 249–270. doi:10.3390/ijerph110100249
Oelkers, E. H., Hering, J. G., and Zhu, C. (2011). Water: Is there a global crisis. Elements 7, 157–162. doi:10.2113/gselements.7.3.157
Omarova, A., Tussupova, K., Berndtsson, R., Kalishev, M., and Sharapatova, K. (2018). Protozoan parasites in drinking water: A system approach for improved water, sanitation and hygiene in developing countries. Int. J. Environ. Res. Public Health 15, 495. doi:10.3390/ijerph15030495
Prinsloo, L. (2008). Creamer media engineering news: Construction giant receives water treatment works upgrade contract. Available at: https://www.engineeringnews.co.za/article/construction-giant-receives-water-treatment-works-upgrade-contract-2008-03-28/rep_id:4136 (Accessed May 2, 2022).
Roccaro, P. (2018). Treatment processes for municipal wastewater reclamation: The challenges of emerging contaminants and direct potable reuse. Curr. Opin. Environ. Sci. Health 2, 46–54. doi:10.1016/j.coesh.2018.02.003
Rodriguez, C., Van Buynder, P., Lugg, R., Blair, P., Devine, B., Cook, A., et al. (2009). Indirect potable reuse: A sustainable water supply alternative. Int. J. Environ. Res. Public Health 6, 1174–1203. doi:10.3390/ijerph6031174
Rosborg, I., and Kozisek, F. (2019). Drinking water minerals and mineral balance, springer nature Switzerland AG.
Schimmoller, L. J., Kealy, M. J., and Foster, S. K. (2015). Triple bottom line costs for multiple potable reuse treatment schemes. Environ. Sci. Water Res. Technol. 1, 644–658. doi:10.1039/c5ew00044k
Sesan, T., Sanfo, S., Sikhwivhilu, K., Dakyaga, F., Aziz, F., and Yirenya Tawiah, D. (2022). Mediating Knowledge Co Production for Inclusive Governance and Delivery of Food, Water and Energy Services in African Cities. Urban Forum 33, 281–658307.
Scott, M., Millar, G. J., and Altaee, A. (2019). Process design of a treatment system to reduce conductivity and ammoniacal nitrogen content of landfill leachate. J. Water Process Eng. 31, 100806. doi:10.1016/j.jwpe.2019.100806
Sibali, L. L., Okwonkwo, J. O., and McCrindle, R. I. (2008). Determination of selected organochlorine pesticide (OCP) compounds from the Jukskei River catchment area in Gauteng, South Africa. Water sa. 34, 611–621. doi:10.4314/wsa.v34i5.180659
Skosana, G., and du Preez, H. (2017). Assessment of the viability of the reuse of sedibeng district municipal secondary effluent in southern Gauteng, South Africa. J. Water Resour. Prot. 9, 1043–1061. doi:10.4236/jwarp.2017.98069
Sobantu, M., and Nel, H. (2019). Voluntary housing delivery: The contribution of partnerships to the success of a community based organisation (wassup) in Diepsloot low-income community, Johannesburg. South Afr. Soc. Work. W 55, 284.
Somani, S. B., and Ingole, N. W. (2011). Alternative approach to chlorination for disinfection of drinking water - an overview. Amravati: International Journal of Advanced Engineering Research and Studies, 47–50.
Swartz, C. D., Menge, J. G., du Plessis, J. A., and Wolfaardt, G. M. (2022). A water reclamation and reuse guide for South African municipal engineers. Water Research Commission Report No. TT 882/22, ISBN 978-0-6392-0158-0.
The Diepsloot Youth Programme (2023). Diepsloot. Available at: https://www.dyp.co.za/what-and-where-is-diepsloot/ (accessed April 10, 2023).
Tshililo, F. P., Mutanga, S., Sikhwivhilu, K., Siame, J., Hongoro, C., Managa, L. R., et al. (2022). Analysis of the determinants of household’s water access and payments among the urban poor. A case study of Diepsloot township. Phys. Chem. Earth 127, 103183. doi:10.1016/j.pce.2022.103183
United Nations (2018). The sustainable development goals report. Available at: https://unstats.un.org/sdgs/files/report/2018/thesustainabledevelopmentgoalsreport2018-en.pdf (Accessed November 11, 2022).
United Nations World Water Assessment Programme (WWAP). (2015). The United Nations World Water Development Report 2015: Water for a Sustainable World. Paris: UNESCO
United States of America (USA) (2019). Draft national water reuse action plan: Appendix G - selected international profiles. https://www.epa.gov/sites/default/files/2019-09/documents/water-reuse-2019-appendix-g.pdf.
Wang, H., Wang, T., Zhang, B., Li, F., Toure, B., Omosa, I. B., et al. (2014b). Water and wastewater treatment in Africa - current practices and challenges: Water and wastewater treatment in Africa. Water 42 (8), 1029–1035. doi:10.1002/clen.201300208
Wang, X.-j., Zhang, J.-y., Shahid, S., Guan, E.-h., Wu, Y.-x., Gao, J., et al. (2014a). Adaptation to climate change impacts on water demand, mitigation and adaptation strategies for global change. Springer Science + Business Media Dordrecht.
Warsinger, D. M., Chakraborty, S., Tow, E. W., Plumlee, M. H., Bellona, C., Loutatidou, S., et al. (2018). A review of polymeric membranes and processes for potable water reuse. Prog. Polym. Sci. 81, 209–237. doi:10.1016/j.progpolymsci.2018.01.004
Water Research Commission (WRC) (2003). Quality of domestic water supplies volume 2: Sampling guide, department of water affairs and forestry. WRC Report No. TT/117/99. ISBN 1 86845 543 2.
Wikipedia. (2022). Rand water, Available at: https://en.wikipedia.org/wiki/Rand_Water (Accessed November 10, 2022).
World Health Organization. (2013). How much water is needed in emergencies? Technical notes on drinking-water, sanitation and hygiene in emergencies. Available at: https://cdn.who.int/media/docs/default-source/wash-documents/who-tn-09-how-much-water-is-needed.pdf?sfvrsn=1e876b2a_6 [Accessed September 14, 2022].
World Population Review (2023). World population review. Available at: https://worldpopulationreview.com/countries/south-africa-population (accessed April 10, 2023).
Keywords: direct water reclamation, municipal wastewater, potable reuse, water quality, process simulation
Citation: Nemadodzi L, Sikhwivhilu K, Jalama K, Moothi K, Bambo M, Mutanga S and Siame J (2023) Salient ingredients for direct water reclamation from treated municipal wastewater for potable reuse: Diepsloot Township case study. Front. Environ. Sci. 11:1143367. doi: 10.3389/fenvs.2023.1143367
Received: 13 January 2023; Accepted: 20 April 2023;
Published: 22 May 2023.
Edited by:
Irene Voukkali, Open University of Cyprus, CyprusReviewed by:
Asude Hanedar, Namik Kemal University, TürkiyeNiti B. Jadeja, University of Virginia, United States
Copyright © 2023 Nemadodzi, Sikhwivhilu, Jalama, Moothi, Bambo, Mutanga and Siame. This is an open-access article distributed under the terms of the Creative Commons Attribution License (CC BY). The use, distribution or reproduction in other forums is permitted, provided the original author(s) and the copyright owner(s) are credited and that the original publication in this journal is cited, in accordance with accepted academic practice. No use, distribution or reproduction is permitted which does not comply with these terms.
*Correspondence: Keneiloe Sikhwivhilu, a2VuZWlsb2VzQG1pbnRlay5jby56YQ==