- 1Department of Environment and Energy, Jeonbuk National University, Jeonju-si, Republic of Korea
- 2Department of Environmental Science and Engineering, Ewha Womans University, Seoul, Republic of Korea
- 3Division of Climate and Air Quality Research, National Institute of Environmental Research, Chungcheong Region Air Quality Research Center, Seosan, Republic of Korea
- 4Department of Atmospheric Environment Research, National Institute of Environmental Research, Seoul, Republic of Korea
- 5Bio-Chemical Analysis Team, Korea Basic Science Institute, Cheongju, Republic of Korea
- 6School of Civil and Environmental Engineering, Pusan National University, Busan, Republic of Korea
- 7Department of Earth and Environmental Sciences, Jeonbuk National University, Jeonju-si, Republic of Korea
The morphology and phase state are critical physical properties of aerosol particles. However, studies related to the analysis of these properties primarily focus on laboratory experiments, and studies on real aerosol particles are limited. Herein, fine particulate matter (PM2.5) filter samples were obtained to investigate and compare the morphology and phase state of ambient aerosol particles in South Korea. The PM2.5 samples were collected in the summer of June 2021 from two different environments: Seoul (urban) and Seosan (coastal-rural). Optical microscopy was combined with the poke-and-flow technique to determine the morphology and phase state of the PM2.5 as a function of relative humidity (RH) at 293 ± 1 K. At both sites, the PM2.5 droplets, which were extracted in purified water, showed a multiphase nature that was dependent on the RH and chemical composition. Based on the results and ambient average RH in Seoul, most of the PM2.5 was observed in a liquid state on polluted days under an inorganic-dominant condition, but in a semisolid state on clean days under an organic carbon-rich condition. In Seosan, the PM2.5 predominantly existed in a liquid state, due to the high RH caused by proximity to the Yellow Sea. Our study provides fundamental physical properties of PM2.5 for both urban and coastal-rural environments. The results have strong applications for atmospheric chemistry and predicting particle size distributions.
1 Introduction
In Asia, rapid industrialization and infrastructure developments have resulted in environmental issues associated with an increase in air pollution and a decrease in air quality (Masson-Delmotte et al., 2021). Among the various atmospheric pollutants, fine particulate matter (PM2.5) significantly affects climate change and human health (Song Y. et al., 2019; Bhattarai et al., 2020; Masson-Delmotte et al., 2021). These effects are influenced by the physicochemical properties of PM2.5, including chemical composition, size, phase state, and morphology (Seinfeld and Pandis. 2016). Field studies have reported that PM2.5 is predominately comprised of a mixture of thousands of organic molecules and some inorganic substances (Jimenez et al., 2009; Zhang et al., 2011; Kim et al., 2022).
PM2.5 can undergo phase transitions in the atmosphere, resulting in different morphologies and phase states. Depending on the relative humidity (RH), temperature, and particle chemical composition, PM2.5 morphology can shift between being homogeneous, core–shell, or partially engulfed (Reid et al., 2011; Song et al., 2013). Studies using atmospherically relevant particles have shown that aerosol particles often exhibit a phase-separated morphology when the oxygen-to-carbon ratio (O: C) of their organic materials is less than 0.8 (Bertram et al., 2011; Krieger et al., 2012; Song et al., 2012; Zuend and Seinfeld. 2012). These different morphologies can affect the heterogeneous chemical reaction rate, water uptake, and gas-to-particle partitioning (Zuend and Seinfeld. 2012; Lam et al., 2021; Mikhailov et al., 2021).
PM2.5 can exist in a liquid, semisolid, or solid phase state in the atmosphere, and the phase state is vital for predicting the size distribution, mass concentration, heterogeneous reactivity, and ice nucleation efficiency of its particles (Shiraiwa et al., 2013; Kim et al., 2019; Knopf et al., 2018; Li et al., 2018; Zaveri et al., 2018). The phase state of PM2.5 can be determined by the range of viscosity, where liquid (viscosity < 102 Pa s), semisolid (viscosity of 102–1012 Pa s), and solid (viscosity > 1012 Pa s) (Koop et al., 2011). Various laboratory studies have shown that the RH, water affinity, and chemical composition of aerosol particles significantly impact their phase states (Koop et al., 2011; Grayson et al., 2016; Rothfuss and Petters. 2017; Song M. et al., 2019; Champion et al., 2019; Petters et al., 2019; Song et al., 2021; Ham et al., 2019; Jeong et al., 2022). However, comparatively few field studies have observed the phase states of real-world aerosol particles (Bateman et al., 2016; Liu et al., 2021b; Cheng et al., 2021; Song et al., 2022). More data on the morphologies and phase states of real-world aerosol particles are required to accurately describe the impact of aerosols on atmospheric chemistry and the climate.
To achieve this, PM2.5 was simultaneously collected at two different sites in South Korea in June 2021 including Seoul (urban) and Seosan (coastal-rural). Seoul is a highly populated urban area and one of the world’s megacities, whereas Seosan is a coastal-rural area surrounded by an agricultural complex, the Yellow Sea, and coal power plants. Morphologies and phase states of PM2.5 droplets were observed at 293 ± 1 K upon dehydration, using optical microscopy and a poke-and-flow technique. The results of these two methods were combined with ambient RH conditions to estimate and compare the phase states of PM2.5 in these urban and coastal-rural areas during summer.
2 Materials and methods
2.1 Measurement sites
PM2.5 filter samples were simultaneously collected in Seoul (37.61°N, 126.93°E) and Seosan (36.78°N, 126.49°E) in South Korea during June 1–30, 2021 (Supplementary Figure S1). Seoul is a megacity with heavy traffic and dense residential areas (Kim et al., 2022). The sampling site in Seoul was operated by the National Institute of Environmental Research in Bulgwang-dong, Eunpyeong-gu. Seosan is a coastal-rural area surrounded by agricultural clusters, ∼15 km from the Yellow Sea, and ∼26 km from petrochemical complexes. This site has one of the highest annual PM2.5 levels in Korea, even though it is a rural area (Ju et al., 2020). The Seosan measurement site was operated by the National Institute of Environmental Research in Suseok-dong, Seosan-si, Chungcheongnam-do.
2.2 Collection and generation of PM2.5
PM2.5 was collected on quartz filters (8 × 10 inches, Pall Co., United States) every 23 h (from 10:00 a.m. to 09:00 a.m., local time) using high-volume air samplers at a flow rate of ∼1000 L/m (HV-1000R, SIBATA, Japan). The samples were immediately stored in a freezer at ∼255 K after collection. We focused on samples with high PM2.5 concentrations at least one of the two sites, i.e., a daily mean concentration ≥35 μg/m3, which is the standard daily level of PM2.5 in Korea (Ministry of Environment, 2021). Based on this, six cases (Cases 1–6) were selected from both sites (yellow shaded regions in Supplementary Figure S2), which included high PM2.5 episodes. The samples in Cases 1–6 that were considered unsuitable for analysis were excluded, such as the sample collected in Seosan on 28 June 2021, owing to the considerably low surface tension of the PM2.5 droplets on the substrate. In total, 17 filter samples (nine from Seoul and eight from Seosan) were investigated for phase states. Table 1 provides information on the daily mean concentrations, chemical compositions (Supplementary Section S1), O:C (Supplementary Section S2), and aerosol liquid water content (Supplementary Section S3) of PM2.5 for Cases 1–6 in Seoul and Seosan. An overview of the measurements at the two different sites is provided in Supplementary Section S4. The filter samples for Cases 1–6 were extracted as water-soluble organic and inorganic compounds in deionized water (18.2 MΩ cm, Merck Milli-Q®, Millipore, United States) for morphology and phase experiments within 3 months after collection. Previous studies showed that water-soluble organic and inorganic species were comprised of more than ∼70% in PM2.5 (Huang et al., 2012; Liu et al., 2021a). A nebulizer (MEINHARD®, United States) was used to nebulize the extracted PM2.5 droplets onto a hydrophobic substrate for morphology observation (Section 2.3) and poke-and-flow experiments (Section 2.4).
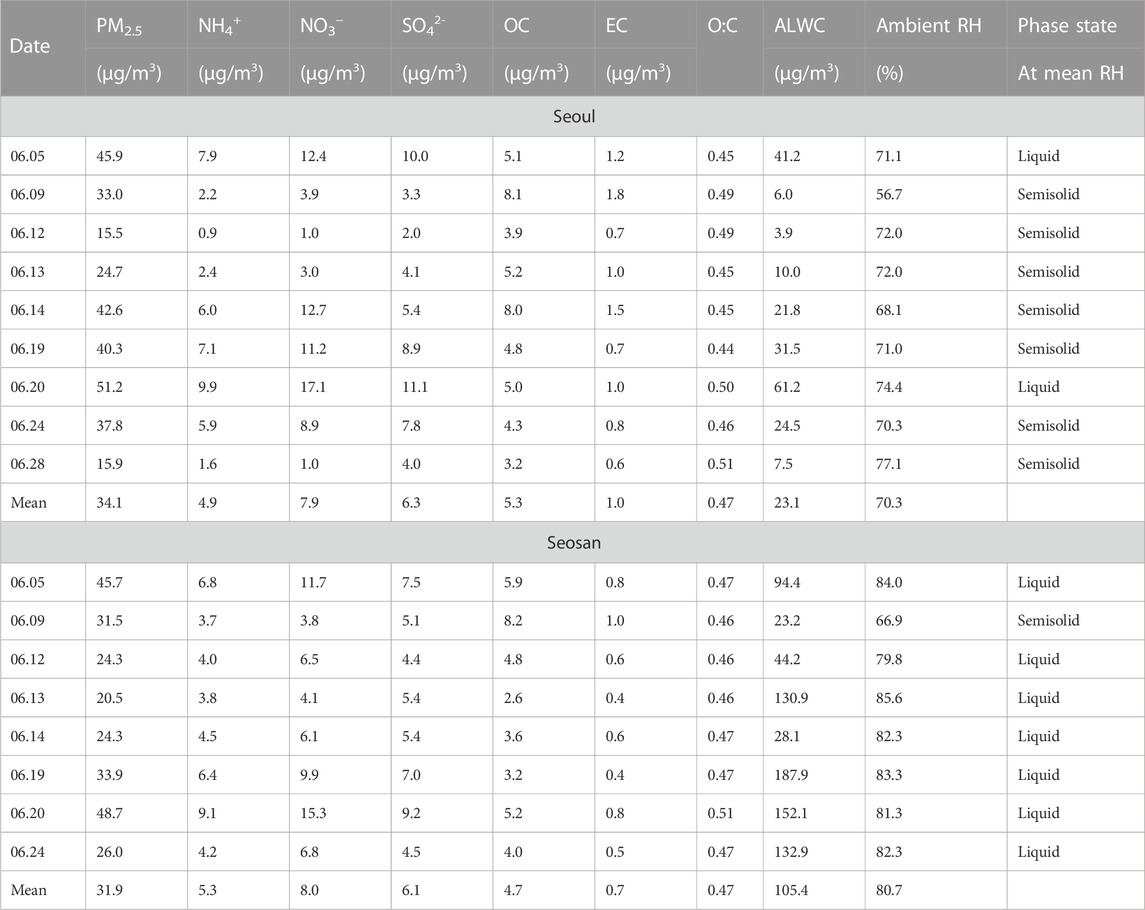
TABLE 1. A summary of the average daily mass concentration, chemical composition, oxygen-to-carbon ratio (O:C), and aerosol liquid water content (ALWC) of PM2.5, and ambient relative humidity (RH) based on filter sampling intervals in seoul and seosan for Case 1–6 in 2021. Phase state of the bulk of PM2.5 at mean ambient RH is also included.
2.3 Optical microscopy for PM2.5 droplets
A flow-cell was used to perform temperature- and RH-controlled measurements for PM2.5 droplets on a hydrophobic substrate (Song et al., 2012; Jeong et al., 2022). The PM2.5 droplets were equilibrated at ∼100% RH and 293 ± 1 K for ∼20 min at the start of the experiment. The RH was then lowered at a rate of 0.5% RH/min. Morphological analysis was carried out using an optical microscope; detailed procedures and methods are described in previous reports (Gaikwad et al., 2022; Song et al., 2022). An optical microscope (BX4 × 3, ×40 objective; Olympus, Japan) was used to monitor and capture morphology changes at 10 s intervals during the dehydration process, using a camera with a charge-coupled device (CCD) (DigiRetina 16, Tucsen, China). The RH in the flow-cell was calibrated at 293 ± 1 K by measuring the deliquescence RH of K2CO3 (at 44% RH) and NaCl (at 76% RH) (Winston and Bates. 1960), resulting in RH uncertainty of ±1.5%. The RH in the flow-cell was adjusted by the ratio of dry N2 gas to saturated H2O gas (total flow rate: 500 sccm). The temperature range of the experiment (293 ± 1 K) was similar to the actual mean temperature in Seoul (297 ± 3 K) and Seosan (295 ± 3 K).
2.4 Poke-and-flow technique for PM2.5 droplets
A poke-and-flow technique was adopted to analyze the PM2.5 phase state as semisolid or solid, hereafter referred to as (semi) solid as described by Renbaum-Wolff et al. (2013); Song M. et al. (2019). The procedure was detailed in previous studies (Renbaum-Wolff et al., 2013; Gaikwad et al., 2022). The PM2.5 droplets were conditioned at ∼ 100% RH. Subsequently, the RH was decreased to ∼40% at a rate of ∼1% RH/min, and the RH was then adjusted from approximately 40% to 0% at ∼ 10% decrements. At a given RH, the droplets were conditioned for ∼2 h before being poked. A sharp needle (Jung Rim Medical Industrial, South Korea) was used to poke the droplets at each decrement to determine the RH at which the particles cracked. Subsequently, the geometry was observed for ∼3 h to observe the occurrence of fluid flow. Based on previous studies using the poke-and-flow technique, when no flow was observed, the viscosity was determined to be > ∼1 × 108 Pa·s (Song et al., 2015; Grayson et al., 2016). However, this technique did not allow for measurements in the semisolid viscosity range of 102–108 Pa·s because the PM2.5 was supersaturated with inorganic substances such as ammonium sulfate and ammonium nitrate upon dehydration. The PM2.5 droplets were observed with an optical microscope (CKX5 × 3, ×40 objective; Olympus, Japan) before, during, and after being poked; the process was captured using a CCD camera (C11440-42U30, Hamamatsu, Japan). A temperature of ∼293 ± 1 K was maintained during the experiments, which reflected the ambient temperature range recorded at the two sampling sites.
3 Results and discussion
3.1 Morphology of PM2.5 droplets
PM2.5 was collected in the summer of 2021 in the urban and coastal-rural environments of Seoul and Seosan, respectively, including high PM2.5 episodes. The samples for each site were labeled as Cases 1–6 (per site), and the morphology of the PM2.5 was studied at a temperature of 293 ± 1 K. Figures 1A, B show the changes in PM2.5 morphology during the dehydration process in Seoul and Seosan, respectively, for each date. All components were dissolved in the droplets at an RH of ∼100–90%, exhibiting a single liquid state (as shown in the second column in Figures 1A, B). The evaporation of water from the droplets caused the size to decrease with decreasing RH. At an average of ∼87–89% RH, all PM2.5 droplets showed liquid-liquid phase separation (LLPS) (third column in Figures 1A, B). This LLPS has been observed in numerous laboratory studies and modeling work, and commonly occurs when the O: C ratio of organic materials in the presence of inorganic salts is below 0.8 (Bertram et al., 2011; Song et al., 2012; Zuend and Seinfeld. 2012). LLPS has also been observed in ambient aerosol particles from different environments, within a specified range of O: C ratios (Pöhlker et al., 2012; You et al., 2012; Gaikwad et al., 2022; Song et al., 2022). In this study, the average O: C of the PM2.5 droplets was ∼0.47 at both sites (Table 1), which is within the appearance range for LLPS.
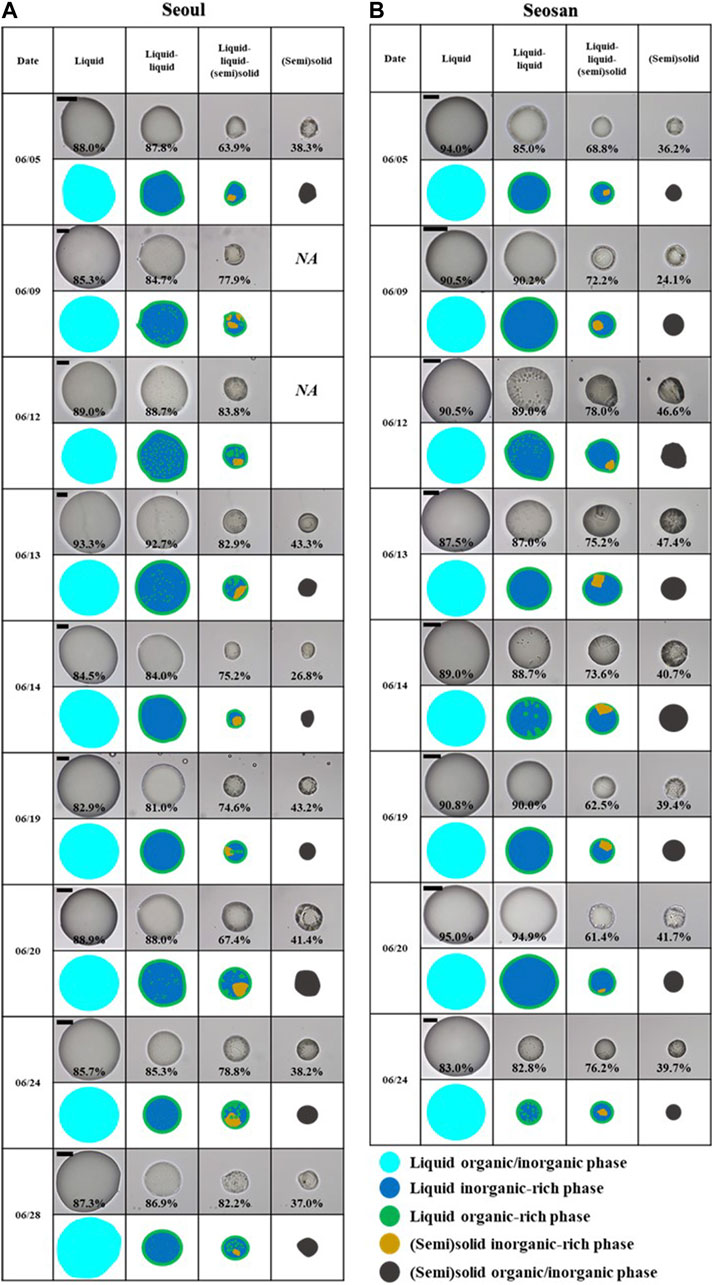
FIGURE 1. Optical images of the morphology changes of PM2.5 droplets at 293 ± 1 K with decreasing relative humidity (RH) for (A) seoul and (B) seosan. “NA” denotes the droplet morphology that was not observed optically. Cartoons are provided to assist in the interpretation of the observed morphologies. Cyan: liquid organic/inorganic phase; blue: liquid inorganic-rich phase; green: liquid organic-rich phase; dark yellow: (semi) solid inorganic-rich; and dark grey: (semi) solid organic/inorganic phase. The scale bar in the optical images denotes 20 µm.
The equilibrium morphology of liquid-liquid-phase separated droplets (i.e., core−shell or partially engulfed) can be determined by the surface and interfacial tensions and spreading coefficients of the two phases (Kwamena et al., 2010; Song et al., 2013). In the droplets from both sites, a core-shell morphology was observed on a hydrophobic substrate. Previous studies have shown that liquid-liquid-phase-separated particles with a core-shell morphology predominantly consist of organic and inorganic species on their outer and inner phases, respectively (Ciobanu et al., 2009; Reid et al., 2011; Song et al., 2013). This can be explained by the fact that organic compounds possess a lower surface tension than inorganic salts (Ciobanu et al., 2009).
With a further decrease in RH, crystals abruptly appeared inside the PM2.5 droplets (fourth column of Figures 1A, B), leading to a three-phase coexistence of a liquid-liquid-(semi) solid state, occurring at an average RH range of ∼76–27% for Seoul and ∼71–33% for Seosan. The crystals may be solid organic materials or inorganic substances such as ammonium sulfite and ammonium nitrate. This morphology has not yet been observed in laboratory-made aerosol particles. However, recently, the coexistence of the three phases consisting of liquid-liquid-(semi) solid has been discovered in the PM2.5 of different environments (Gaikwad et al., 2022; Song et al., 2022). This suggests that atmospheric aerosol particles could display more complicated and multi-phase morphologies than their laboratory counterparts. This finding can be used to predict more accurate results for heterogeneous reactions and in atmospheric chemistry (Riemer et al., 2019). As shown in Figure 1 (last column), a further decrease in RH led to the efflorescence of a large portion of the inorganic fractions.
3.2 Phase states of PM2.5 as a function of RH
To determine a (semi) solid state of the PM2.5, a poke-and-flow experiment was performed at ∼293 ± 1 K. All PM2.5 droplets cracked when poked by a needle at average RHs of ∼27% and ∼33% for particles collected in Seoul and Seosan, respectively (Figure 2). After cracking, no flow was observed for a period of ∼3 h, indicating a viscosity lower limit of ∼108 Pa·s (Song et al., 2015; Grayson et al., 2016), which corresponds to a (semi) solid state (Koop et al., 2011).
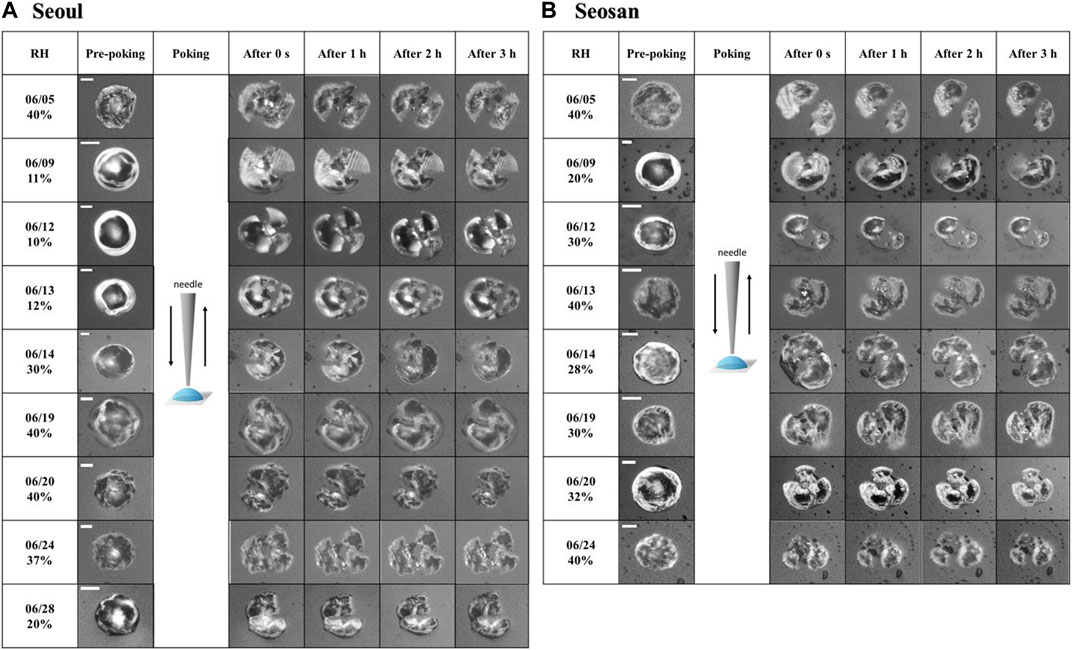
FIGURE 2. Optical images of the poke-and-flow experiment obtained pre-poking, poking, and post-poking of PM2.5 droplets in (A) Seoul and (B) Seosan for cases 1–6. The scale bar represents 10 µm.
From the result of the optical microscopy and poke-and-flow experiments, the phase states of the “bulk” of PM2.5 were determined as a function of RH at a temperature of ∼293 ± 1 K. The bulk of the PM2.5 was characterized as “liquid” for single liquid and liquid-liquid phase separated particles, “semisolid” for liquid-liquid-(semi) solid particles (although not all phases in the particles were necessarily semisolid), and “(semi) solid” for semisolid or solid particles (Song et al., 2022). Based on this characterization, Figure 3A presents the phase states of PM2.5 in Cases 1–6 from the two study sites (dates are shown for increasing daily PM2.5 concentrations). The bulk of the PM2.5 from Seoul exhibited a liquid state at mean RHs > ∼87%, semisolid state at ∼27% < mean RHs < ∼76%, and (semi) solid state at mean RHs < ∼27%. In Seosan, the bulk of the PM2.5 was a liquid state at mean RHs > ∼89%, semisolid state at ∼33% < mean RHs < ∼71%, and (semi) solid state at mean RHs < ∼33%. The average RH range of the liquid state was similar in the two sites, while the RH range of the (semi) solid state was slightly higher in Seosan (∼33%) than that in Seoul (∼27%).
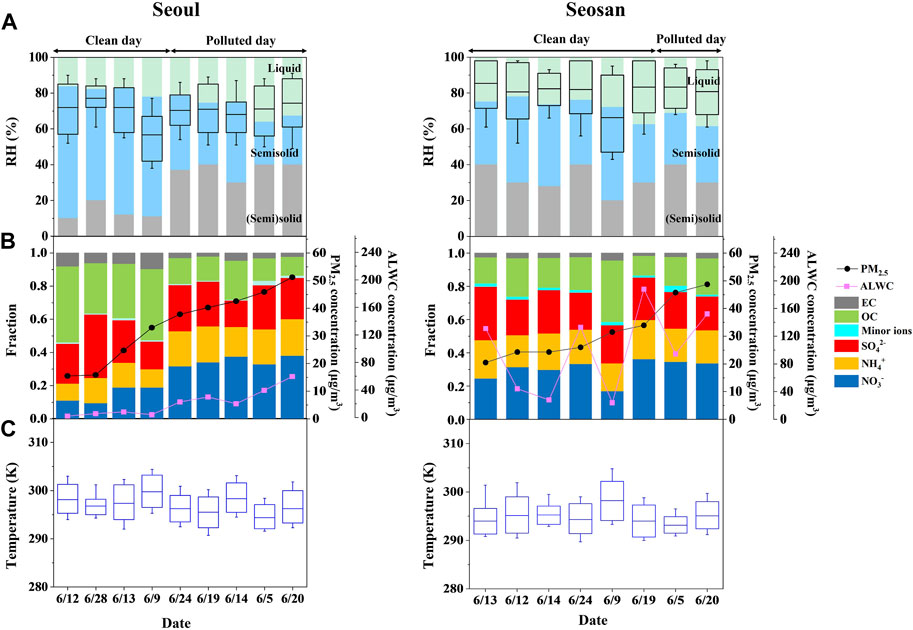
FIGURE 3. Daily average measurement results for PM2.5 in Seoul and Seosan on clean (PM2.5 < 35 μg/m3) and polluted (PM2.5 ≥ 35 μg/m3) days in cases 1–6. (A) Phase states of the bulk of PM2.5 as measured at 293 ± 1 K. Green: liquid; blue: semisolid; and grey: (semi) solid. The box plot indicates daily ambient relative humidity (RH). (B) PM2.5 mass concentrations, and chemical compositions (minor ions: Cl−, Na+, K+, Mg2+, and Ca2+) and aerosol liquid water content (ALWC). (C) Ambient temperature. In the box plot, the box represents the mean, 25th, and 75th percentiles, and the whisker represents the minimum and maximum values. Dates are arranged from low to high PM2.5 mass concentrations for cases 1–6.
Interestingly, a clear difference in the RH boundary of semisolid and (semi) solid state was observed in Seoul. It was ∼38% RH on polluted days (PM2.5 ≥ 35 μg/m3), but the RH value was dropped down to ∼13% on clean days (PM2.5 < 35 μg/m3) as shown in Figure 3A. This is most likely due to the different chemical compositions of Seoul PM2.5 on polluted and clean days; Figure 3B and S3a illustrate the abundance of major chemical compositions of PM2.5 in Seoul for polluted versus clean days, including organic carbon (OC), elemental carbon (EC), and inorganic substances (sulfate, nitrate, and ammonium). A distinct difference in PM2.5 chemical compositions was monitored in Seoul that inorganic fraction increased to ∼81% on polluted days while it was decreased to ∼55% with enhancement of organic fraction on clean days. Recent laboratory studies have shown the effect of inorganic salts on viscosity and phase state in aerosol particles (Richards et al., 2020; Jeong et al., 2022). Jeong et al. (2022) showed that the viscosity of inorganic-rich particles (i.e., ammonium sulfate) was approximately 8 orders of magnitude higher than that of organic-rich particles at low RH levels close to their efflorescence RH. In the current study, the effect of inorganic substances on phase state in real-world aerosol particles was consistent with the results of previous laboratory studies.
In contrast to the Seoul urban area, in Seosan, a significant variation on the RH boundaries for the phase states of the PM2.5 was not observed on the polluted versus clean days (Figure 3A). This could be resulted from the similar chemical compositions during the entire periods. Figure 3B and S3b show that the abundances of the major chemical species of the PM2.5 was insensitive depending on the concentrations. This can be extrapolated to a similar range in the RH boundary of the phase states over the entire period in the coastal-rural area.
3.3 Estimated phase states of PM2.5 in urban and coastal-rural areas
The primary impact on the phase state of aerosol particles on RH has been previously studied (Renbaum-Wolff et al., 2013; Song et al., 2015; Reid et al., 2018; Rovelli et al., 2019). From these studies, they explained that aerosol viscosity and phase states are strongly RH-dependent. To estimate the phase state of the bulk of the PM2.5, we first related our results to the ambient RH condition of the two sites. Box plots in Figure 3A display the daily ambient RH in Seoul and Seosan. The mean ambient RH during summer was ∼70% in Seoul and ∼81% in Seosan, with some variations (Figure 3A; Table 1).
Based on the measured phase states of the bulk of the PM2.5 (Section 3.2) and mean ambient RH in Seoul (box plot of Figure 3A), PM2.5 was estimated in a liquid or semisolid state. The liquid phase state of PM2.5 became more dominant as the PM2.5 concentration increased when the RH and ammonium nitrate were enhanced. The ambient RH in Seoul (Figure 3A) ranged higher than the deliquescence RH of ammonium nitrate (65.5% RH at 293 K) (Winston and Bates. 1960). This indicates the high ambient RH led to liquefy the PM2.5 dominated by ammonium nitrate (Seinfeld and Pandis. 2016). The result is consistent with findings of our recent study of Song et al. (2022) in Seoul during winter where the liquid phase state was dominant on high PM2.5 days and a semisolid state was dominant on low PM2.5 days. Liu et al. (2017) observed a similar phenomenon where the particle phase state was liquid during heavy haze events in Beijing. Under high RH conditions, rapid chemical reactions can occur between PM2.5 and surrounding gas molecules, accelerating the production of high PM2.5 mass concentrations.
Most of the PM2.5 in Seosan was in a liquid state at the mean ambient RH values, except on June 9, when the RH value was relatively low with an average of ∼67% (Figure 3A; Table 1). This coastal-rural area is close to the Yellow Sea (∼15 km); therefore, high ambient RH conditions are common. Due to the geographic characteristics of Seosan, PM2.5 was commonly existed in a liquid phase state.
In addition to RH, the chemical composition of PM2.5 is another important parameter for determining phase states. Several studies have shown that the phase states of aerosol particles were determined by the presence of organic materials, inorganic salts, and their ratios (Rovelli et al., 2019; Richards et al., 2020; Jeong et al., 2022). Using the RH and PM2.5 chemical compositions (Supplementary Section S3), the aerosol liquid water content (ALWC) at the two sites were calculated (Figure 3B). As discussed above, a high fraction of inorganic substances in PM2.5 was observed at both sites during high PM2.5 episodes (Supplementary Figure S3). High inorganic composition and RH could result in abundant liquid water molecules in the PM2.5, leading to PM2.5 pollution (Supplementary Figure S4). Moreover, a distinct difference in ALWC was observed at the two different sites, with Seosan exhibiting a significantly greater ALWC than Seoul, resulting in a predominantly liquid state of PM2.5 in Seosan. To gain more comprehensive results of the effect of chemical composition on the phase state of ambient aerosol particles, further studies are required with more extensive datasets. In addition, environmental factors such as wind and precipitation could also affect phase variation of PM2.5. These parameters should be also considered to explore phase behavior of PM2.5.
4 Conclusion and implications
In this study, optical microscopy was combined with the poke-and-flow technique to determine the morphology and phase state of PM2.5 at 293 ± 1 K collected in Seoul (urban) and Seosan (coastal-rural) in June 2021. Upon dehydration, the PM2.5 droplets from Seoul and Seosan exhibited different morphologies and phases, including single liquid, semisolid, and (semi) solid states. Based on the ambient average RH level and the results obtained from the laboratory experiments at 293 ± 1 K, which is considered similar to ambient temperature, the phase states of bulk PM2.5 in the urban and rural areas were estimated. The PM2.5 in Seoul was to be in both liquid and semisolid states and results showed that a liquid state was abundant during highly polluted summer days. However, PM2.5 was to be present in a liquid state due to the high ALWC, as well as meteorological and geographic characteristics of Seosan. Our results could have several limitations in providing of quantification of viscosity for PM2.5 particles, and potential effects of water-insoluble materials on the morphology and phase state. Therefore, to obtain more concrete conclusion for the phase state of PM2.5, more experimental dataset and incorporation with different methods are required.
The phase state of aerosol particles can be affected by particle size distribution. Several recent laboratory and modeling studies demonstrated that liquid particles allow the partitioning of gas molecules into the volume of the whole particle with fast reaction/uptake of ambient gas molecules (Shiraiwa et al., 2013; Slade and Knopf. 2014; Berkemeier et al., 2016; Houle et al., 2018). However, for solid particles, gas molecules can only partition onto the surface of the particles. As a result, the phase states of particles can influence particle growth, internal mixing, and particle size distribution (Zaveri et al., 2014; Song et al., 2022). To build on the results from this study, more detailed data is needed to understand the fundamental physical properties of PM2.5, and its environmental consequences, which are still largely unknown, and how the physical properties are linked to the chemical compositions that cause PM2.5 pollution.
Data availability statement
The original contributions presented in the study are included in the article/Supplementary Material, further inquiries can be directed to the corresponding author.
Author contributions
This study is designed by MS. All of the authors took measurements and analyzed the data. The manuscript was prepared by MS, SG, and DK with contributions from all co-authors. The published version of the manuscript has been read and approved by all authors.
Funding
Fine Particle Research Initiative in East Asia Considering National Differences (FRIEND) Project (NRF-2020M3G1A1114548) and research funds of Jeonbuk National University in 2022.
Acknowledgments
We thank to Sangmin Oh and Junsu Park for helping some filter sampling.
Conflict of interest
The authors declare that the research was conducted in the absence of any commercial or financial relationships that could be construed as a potential conflict of interest.
Publisher’s note
All claims expressed in this article are solely those of the authors and do not necessarily represent those of their affiliated organizations, or those of the publisher, the editors and the reviewers. Any product that may be evaluated in this article, or claim that may be made by its manufacturer, is not guaranteed or endorsed by the publisher.
Supplementary material
The Supplementary Material for this article can be found online at: https://www.frontiersin.org/articles/10.3389/fenvs.2023.1142941/full#supplementary-material
References
Bateman, A. P., Gong, Z., Liu, P., Sato, B., Cirino, G., Zhang, Y., et al. (2016). Sub-micrometre particulate matter is primarily in liquid form over Amazon rainforest. Nat. Geosci. 9 (1), 34–37. doi:10.1038/ngeo2599
Berkemeier, T., Steimer, S. S., Krieger, U. K., Peter, T., Pöschl, U., Ammann, M., et al. (2016). Ozone uptake on glassy, semi-solid and liquid organic matter and the role of reactive oxygen intermediates in atmospheric aerosol chemistry. Phys. Chem. Chem. Phys. 18 (18), 12662–12674. doi:10.1039/C6CP00634E
Bertram, A. K., Martin, S. T., Hanna, S. J., Smith, M. L., Bodsworth, A., Chen, Q., et al. (2011). Predicting the relative humidities of liquid-liquid phase separation, efflorescence, and deliquescence of mixed particles of ammonium sulfate, organic material, and water using the organic-to-sulfate mass ratio of the particle and the oxygen-to-carbon elemental ratio of the organic component. Atmos. Chem. Phys. 11 (21), 10995–11006. doi:10.5194/acp-11-10995-2011
Bhattarai, G., Lee, J. B., Kim, M. H., Ham, S., So, H. S., Oh, S., et al. (2020). Maternal exposure to fine particulate matter during pregnancy induces progressive senescence of hematopoietic stem cells under preferential impairment of the bone marrow microenvironment and aids development of myeloproliferative disease. Leukemia 34 (5), 1481–1484. doi:10.1038/s41375-019-0665-8
Champion, W. M., Rothfuss, N. E., Petters, M. D., and Grieshop, A. P. (2019). Volatility and viscosity are correlated in terpene secondary organic aerosol formed in a flow reactor. Environ. Sci. Technol. Lett. 6 (9), 513–519. doi:10.1021/acs.estlett.9b00412
Cheng, Z., Sharma, N., Tseng, K.-P., Kovarik, L., and China, S. (2021). Direct observation and assessment of phase states of ambient and lab-generated sub-micron particles upon humidification. RSC Adv. 11 (25), 15264–15272. doi:10.1039/D1RA02530A
Ciobanu, V. G., Marcolli, C., Krieger, U. K., Weers, U., and Peter, T. (2009). Liquid-liquid phase separation in mixed organic/inorganic aerosol particles. J. Phys. Chem. A 113 (41), 10966–10978. doi:10.1021/jp905054d
Gaikwad, S., Jeong, R., Kim, D., Lee, K., Jang, K., Kim, C., et al. (2022). Microscopic observation of a liquid-liquid-(semi)solid phase in polluted PM2.5. Front. Environ. Sci. 1126. doi:10.3389/fenvs.2022.947924
Grayson, J. W., Zhang, Y., Mutzel, A., Renbaum-Wolff, L., Böge, O., Kamal, S., et al. (2016). Effect of varying experimental conditions on the viscosity of <i&gt;α</i&gt;-pinene derived secondary organic material. Atmos. Chem. Phys. 16 (10), 6027–6040. doi:10.5194/acp-16-6027-2016
Ham, S., Babar, Z. B., Lee, J. B., Lim, H.-J., and Song, M. (2019). Liquid–liquid phase separation in secondary organic aerosol particles produced from <i&gt;α</i&gt;-pinene ozonolysis and <i&gt;α</i&gt;-pinene photooxidation with/without ammonia. Atmos. Chem. Phys. 19 (14), 9321–9331. doi:10.5194/acp-19-9321-2019
Houle, F. A., Wiegel, A. A., and Wilson, K. R. (2018). Changes in reactivity as chemistry becomes confined to an interface. The case of free radical oxidation of C30H62 alkane by OH. J. Phys. Chem. Lett. 9 (5), 1053–1057. doi:10.1021/acs.jpclett.8b00172
Huang, H., Ho, K. F., Lee, S. C., Tsang, P. K., Ho, S. S. H., Zou, C. W., et al. (2012). Characteristics of carbonaceous aerosol in PM2.5: Pearl delta river region, China. Atmos. Res. 104, 227–236. doi:10.1016/j.atmosres.2011.10.016
Jeong, R., Lilek, J., Zuend, A., Xu, R., Chan, M. N., Kim, D., et al. (2022). Viscosity and physical state of sucrose mixed with ammonium sulfate droplets. Atmos. Chem. Phys. Discuss. 1-21, 8805–8817. doi:10.5194/acp-22-8805-2022
Jimenez, J. L., Canagaratna, M., Donahue, N., Prevot, A., Zhang, Q., Kroll, J. H., et al. (2009). Evolution of organic aerosols in the atmosphere. Science 326 (5959), 1525–1529. doi:10.1126/science.1180353
Ju, S., Yu, G. H., Park, S., Lee, J., Lee, S., Jee, J., et al. (2020). Pollution characteristics of PM2.5 measured during fall at a seosan site in chungcheong province. J. Korean Soc. Atmos. 36 (3), 329–345. doi:10.5572/KOSAE.2020.36.3.329
Kim, N. K., Kim, Y. P., Ghim, Y. S., Song, M., Kim, C. H., Jang, K. S., et al. (2022). Spatial distribution of PM2.5 chemical components during winter at five sites in Northeast Asia: High temporal resolution measurement study. Atmos. Environ. 290, 119359. doi:10.1016/j.atmosenv.2022.119359
Kim, Y., Sartelet, K., and Couvidat, F. (2019). Modeling the effect of non-ideality, dynamic mass transfer and viscosity on SOA formation in a 3-D air quality model. Atmos. Chem. Phys. 19 (2), 1241–1261. doi:10.5194/acp-19-1241-2019
Knopf, D. A., Alpert, P. A., and Wang, B. (2018). The role of organic aerosol in atmospheric ice nucleation: A review. ACS Earth Space Chem. 2 (3), 168–202. doi:10.1021/acsearthspacechem.7b00120
Koop, T., Bookhold, J., Shiraiwa, M., and Poschl, U. (2011). Glass transition and phase state of organic compounds: Dependency on molecular properties and implications for secondary organic aerosols in the atmosphere. Phys. Chem. Chem. Phys. 13 (43), 19238–19255. doi:10.1039/C1CP22617G
Krieger, U. K., Marcolli, C., and Reid, J. P. (2012). Exploring the complexity of aerosol particle properties and processes using single particle techniques. Chem. Soc. Rev. 41 (19), 6631–6662. doi:10.1039/C2CS35082C
Kwamena, N. O., Buajarern, J., and Reid, J. P. (2010). Equilibrium morphology of mixed organic/inorganic/aqueous aerosol droplets: Investigating the effect of relative humidity and surfactants. J. Phys. Chem. A 114 (18), 5787–5795. doi:10.1021/jp1003648
Lam, H. K., Xu, R., Choczynski, J., Davies, J. F., Ham, D., Song, M., et al. (2021). Effects of liquid–liquid phase separation and relative humidity on the heterogeneous OH oxidation of inorganic–organic aerosols: Insights from methylglutaric acid and ammonium sulfate particles. Atmos. Chem. Phys. 21 (3), 2053–2066. doi:10.5194/acp-21-2053-2021
Li, Z., Smith, K. A., and Cappa, C. D. (2018). Influence of relative humidity on the heterogeneous oxidation of secondary organic aerosol. Atmos. Chem. Phys. 18 (19), 14585–14608. doi:10.5194/acp-18-14585-2018
Liu, Y., Li, H., Cui, S., Nie, D., Chen, Y., and Ge, X. (2021a). Chemical characteristics and sources of water-soluble organic nitrogen species in PM2. 5 in nanjing, China. Atmosphere 12 (5), 574. doi:10.3390/atmos12050574
Liu, Y., Meng, X., Wu, Z., Huang, D., Wang, H., Chen, J., et al. (2021b). The particle phase state during the biomass burning events. Sci. Total Environ. 792, 148035. doi:10.1016/j.scitotenv.2021.148035
Liu, Y., Wu, Z., Wang, Y., Xiao, Y., Gu, F., Zheng, J., et al. (2017). Submicrometer particles are in the liquid state during heavy haze episodes in the urban atmosphere of beijing, China. Environ. Sci. Technol. Lett. 4 (10), 427–432. doi:10.1021/acs.estlett.7b00352
Masson-Delmotte, V., Zhai, P., Connors, S. L., Péan, C., and Berger, S. (2021). IPCC, 2021: Climate change 2021: The physical science basis. Contribution of Working Group I to the Sixth Assessment Report of the Intergovernmental Panel on Climate Change. United Kingdom and New York, NY, USA: Cambridge University Press, Cambridge, 3–32. doi:10.1017/9781009157896.001
Mikhailov, E. F., Pöhlker, M. L., Reinmuth-Selzle, K., Vlasenko, S. S., Krüger, O. O., Fröhlich-Nowoisky, J., et al. (2021). Water uptake of subpollen aerosol particles: Hygroscopic growth, cloud condensation nuclei activation, and liquid–liquid phase separation. Atmos. Chem. Phys. 21 (9), 6999–7022. doi:10.5194/acp-21-6999-2021
Petters, S. S., Kreidenweis, S. M., Grieshop, A. P., Ziemann, P. J., and Petters, M. D. (2019). Temperature-and humidity-dependent phase states of secondary organic aerosols. Geophys. Res. Lett. 46 (2), 1005–1013. doi:10.1029/2018GL080563
Pöhlker, C., Wiedemann, K. T., Sinha, B., Shiraiwa, M., Gunthe, S. S., Smith, M., et al. (2012). Biogenic potassium salt particles as seeds for secondary organic aerosol in the Amazon. Science 337 (6098), 1075–1078. doi:10.1126/science.1223264
Reid, J. P., Bertram, A. K., Topping, D. O., Laskin, A., Martin, S. T., Petters, M. D., et al. (2018). The viscosity of atmospherically relevant organic particles. Nat. Commun. 9 (1), 956. doi:10.1038/s41467-018-03027-z
Reid, J. P., Dennis-Smither, B. J., Kwamena, N. O. A., Miles, R. E., Hanford, K. L., and Homer, C. J. (2011). The morphology of aerosol particles consisting of hydrophobic and hydrophilic phases: Hydrocarbons, alcohols and fatty acids as the hydrophobic component. Phys. Chem. Chem. Phys. 13 (34), 15559–15572. doi:10.1039/C1CP21510H
Renbaum-Wolff, L., Grayson, J. W., Bateman, A. P., Kuwata, M., Sellier, M., Murray, B. J., et al. (2013). Viscosity of alpha-pinene secondary organic material and implications for particle growth and reactivity. Proc. Natl. Acad. Sci. U. S. A. 110 (20), 8014–8019. doi:10.1073/pnas.1219548110
Richards, D. S., Trobaugh, K. L., Hajek-Herrera, J., Price, C. L., Sheldon, C. S., Davies, J. F., et al. (2020). Ion-molecule interactions enable unexpected phase transitions in organic-inorganic aerosol. Sci. Adv. 6 (47), eabb5643. doi:10.1126/sciadv.abb5643
Riemer, N., Ault, A., West, M., Craig, R., and Curtis, J. (2019). Aerosol mixing state: Measurements, modeling, and impacts. Rev. Geophys 57 (2), 187–249. doi:10.1029/2018RG000615
Rothfuss, N. E., and Petters, M. D. (2017). Influence of functional groups on the viscosity of organic aerosol. Environ. Sci. Technol. 51 (1), 271–279. doi:10.1021/acs.est.6b04478
Rovelli, G., Song, Y.-C., Maclean, A. M., Topping, D. O., Bertram, A. K., and Reid, J. P. (2019). Comparison of approaches for measuring and predicting the viscosity of ternary component aerosol particles. Anal. Chem. 91 (8), 5074–5082. doi:10.1021/acs.analchem.8b05353
Seinfeld, J. H., and Pandis, S. N. (2016). Atompspheric chemistry and physics from air pollution to climate change. John Wiley & Sons.
Shiraiwa, M., Yee, L. D., Schilling, K. A., Loza, C. L., Craven, J. S., Zuend, A., et al. (2013). Size distribution dynamics reveal particle-phase chemistry in organic aerosol formation. Proc. Natl. Acad. Sci. 110 (29), 11746–11750. doi:10.1073/pnas.1307501110
Slade, J. H., and Knopf, D. A. (2014). Multiphase OH oxidation kinetics of organic aerosol: The role of particle phase state and relative humidity. Geophys. Res. Lett. 41 (14), 5297–5306. doi:10.1002/2014GL060582
Song, M., Jeong, R., Kim, D., Qiu, Y., Meng, X., Wu, Z., et al. (2022). Comparison of phase states of PM2.5 over megacities, Seoul and beijing, and their implications on particle size distribution. Environ. Sci. Technol. 56 (24), 17581–17590. doi:10.1021/acs.est.2c06377
Song, M., Liu, P. F., Hanna, S. J., Li, Y. J., Martin, S. T., and Bertram, A. K. (2015). Relative humidity-dependent viscosities of isoprene-derived secondary organic material and atmospheric implications for isoprene-dominant forests. Atmos. Chem. Phys. 15 (9), 5145–5159. doi:10.5194/acp-15-5145-2015
Song, M., Maclean, A. M., Huang, Y. Z., Smith, N. R., Blair, S. L., Laskin, J., et al. (2019). Liquid-liquid phase separation and viscosity within secondary organic aerosol generated from diesel fuel vapors. Atmos. Chem. Phys. 19 (19), 12515–12529. doi:10.5194/acp-19-12515-2019
Song, M., Marcolli, C., Krieger, U. K., Lienhard, D. M., and Peter, T. (2013). Morphologies of mixed organic/inorganic/aqueous aerosol droplets. Faraday Discuss. 165, 289–316. doi:10.1039/C3FD00049D
Song, M., Marcolli, C., Krieger, U. K., Zuend, A., and Peter, T. (2012). Liquid-liquid phase separation and morphology of internally mixed dicarboxylic acids/ammonium sulfate/water particles. Atmos. Chem. Phys. 12 (5), 2691–2712. doi:10.5194/acp-12-2691-2012
Song, Y. C., Lilek, J., Lee, J. B., Chan, M. N., Wu, Z. J., Zuend, A., et al. (2021). Viscosity and phase state of aerosol particles consisting of sucrose mixed with inorganic salts. Atmos. Chem. Phys. 21 (13), 10215–10228. doi:10.5194/acp-21-10215-2021
Song, Y., Huang, B., He, Q., Chen, B., Wei, J., and Mahmood, R. (2019). Dynamic assessment of PM2.5 exposure and health risk using remote sensing and geo-spatial big data. Environ. Pollut. 253, 288–296. doi:10.1016/j.envpol.2019.06.057
Winston, P. W., and Bates, D. H. (1960). Saturated solutions for the control of humidity in biological research. Ecology 41 (1), 232–237. doi:10.2307/1931961
You, Y., Renbaum-Wolff, L., Carreras-Sospedra, M., Hanna, S. J., Hiranuma, N., Kamal, S., et al. (2012). Images reveal that atmospheric particles can undergo liquid-liquid phase separations. Proc. Natl. Acad. Sci. U. S. A. 109 (33), 13188–13193. doi:10.1073/pnas.1206414109
Zaveri, R. A., Easter, R. C., Shilling, J. E., and Seinfeld, J. H. (2014). Modeling kinetic partitioning of secondary organic aerosol and size distribution dynamics: Representing effects of volatility, phase state, and particle-phase reaction. Atmos. Chem. Phys. 14 (10), 5153–5181. doi:10.5194/acp-14-5153-2014
Zaveri, R. A., Shilling, J. E., Zelenyuk, A., Liu, J., Bell, D. M., D'Ambro, E. L., et al. (2018). Growth kinetics and size distribution dynamics of viscous secondary organic aerosol. Environ. Sci. Technol. 52 (3), 1191–1199. doi:10.1021/acs.est.7b04623
Zhang, Q., Jimenez, Z. L., Canagaratna, M. R., Ulbrich, I. M., Ng, N. L., Worsnop, D. R., et al. (2011). Understanding atmospheric organic aerosols via factor analysis of aerosol mass spectrometry: A review. Ananl. Bioanal. Chem. 401, 3045–3067. doi:10.1007/s00216-011-5355-y
Keywords: liquid, semisolid, solid, air pollution, aersol
Citation: Gaikwad S, Kim D, Jeong R, Lee JY, Lee K, Ahn J, Jang K-S, Kim C and Song M (2023) Morphology and phase state of PM2.5 in urban and coastal-rural areas during summer. Front. Environ. Sci. 11:1142941. doi: 10.3389/fenvs.2023.1142941
Received: 12 January 2023; Accepted: 24 April 2023;
Published: 09 May 2023.
Edited by:
Ying Li, Chinese Academy of Sciences (CAS), ChinaReviewed by:
Yimeng Song, Yale University, United StatesMasao Gen, Tohoku University, Japan
Yangxi Chu, Chinese Research Academy of Environmental Sciences, China
Copyright © 2023 Gaikwad, Kim, Jeong, Lee, Lee, Ahn, Jang, Kim and Song. This is an open-access article distributed under the terms of the Creative Commons Attribution License (CC BY). The use, distribution or reproduction in other forums is permitted, provided the original author(s) and the copyright owner(s) are credited and that the original publication in this journal is cited, in accordance with accepted academic practice. No use, distribution or reproduction is permitted which does not comply with these terms.
*Correspondence: Mijung Song, mijung.song@jbnu.ac.kr