- 1School of Marine Sciences, Ningbo University, Ningbo, Zhejiang, China
- 2University of Pisa Marine Graduate School, Zhejiang Ocean University, Zhoushan, Zhejiang, China
- 3Leibniz Institute DSMZ-German Collection of Microorganisms and Cell Cultures, Braunschweig, German
Archaea play a significant role in regulating the biogeochemical processes of marine ecosystems. In this study, the high-throughput sequencing was used to explore the archaeal communities in the seawater from the hypoxic and non-hypoxic zones of the Changjiang Estuary. Thaumarchaeota dominated the archaeal communities in the hypoxic zones (bottom water), and Euryarchaeota were mainly distributed in the non-hypoxic zones (surface water). However, the diversity and richness of the archaeal communities showed no obvious difference in the hypoxic and non-hypoxic zones. Moreover, Thaumarchaeota and Nitrosopumilales were positively correlated with salinity, but negatively correlated with dissolved oxygen (DO) and temperature. Temperature, phosphorus, and dissolved oxygen had significant correlations with archaeal richness, while ammonia nitrogen was correlated with archaeal diversity. The networks of archaeal communities possessed a high proportion of positive interspecific interaction and revealed that the Marine Group II species may play distinct roles in both hypoxic and non-hypoxic zones. The current work assessed the influence of dissolved oxygen on the archaeal community patterns and network interaction, thereby illumining the community structure shift of archaea caused by the hypoxia phenomenon in the Changjiang Estuary, which laid a foundation for the future studies on the ecological functions of archaea in estuary ecosystems.
1 Introduction
Archaea have been confirmed to be abundant in extreme environments and marine ecosystems (Wishner et al., 1995; Massana et al., 2000; Madrid et al., 2001), including the seawater, estuaries, and sediments. Previous studies have reported that archaea could play a key role in cycling nitrogen, sulfur, and carbon (Cornelia et al., 2006; Jingxu et al., 2015). Thaumarchaeota [formerly Marine Group (MG) I] and MG II are the most abundant archaeal groups in the marine ecosystem (Ken et al., 2004; Zhang, 2012), and members of Thaumarchaeota play an important role in aerobic ammonia oxidation (Martin et al., 2005).
The Changjiang Estuary is a complex and dynamic region that interfaces the terrestrial and marine environments. The mixture of freshwater and Taiwan Current makes this area a complex and an ideal region for conducting studies on the microorganism distribution. Hypoxia often occurs near the bottom waters of the Changjiang Estuary and is a common phenomenon observed in many estuaries (Li et al., 2002; Orita et al., 2015). The hypoxia in this region might be caused by the water stratification and the decomposition of organic matter that comes from the upstream sediments and dissolved organic compounds (Huabing et al., 2012). Previous studies have reported that hypoxia is the most important factor that shapes the bacterial communities (Heike and Osvaldo, 2010; Huabing et al., 2012). However, the response of these archaeal communities to this changing environment remains unclear.
Several studies on the archaeal communities have been conducted in the Changjiang Estuary. Liu et al. (2011) studied the archaeal community in the seawater of the hypoxic zone in Changjiang Estuary, which demonstrated that Euryarchaeota and Crenarchaeota were the predominant phyla and salinity was the major environmental factor that shaped the archaeal community (Liu et al., 2011). Zeng et al. (2007) found that MG I and II were the most abundant groups in the East China Sea (Zeng et al., 2007). A previous study revealed that the ammonia-oxidizing archaea (AOA) community was significantly correlated with nitrite in the Changjiang Estuary (Hui et al., 2014). However, extensive information on the archaeal community in the Changjiang Estuary is lacking, as the relationships between archaeal richness, diversity, and environmental factors remain to be uncovered.
In this study, high-throughput sequencing was used to explore the archaeal community structure and to reveal the influence of dissolved oxygen (DO) on the archaeal community pattern and network interaction in the seawater of Changjiang Estuary. The findings of this study could enhance our understanding of the hypoxia impact on the archaeal community shift in the seawater of the Changjiang Estuary.
2 Materials and methods
2.1 Sampling and locations
In July 2016, water samples were collected using the SBE 32 sampler (Sea-Bird Electronics, United States) from twelve sites of the Changjiang Estuary including hypoxic and non-hypoxic zones (Figure 1). Samples from each site were collected from the surface layers (10 m depth) and bottom layers (40–55 m depth), and six duplicate samples were collected for each depth. Samples were collected for DNA isolation using a vacuum pump suction filter under 20 kPa. One liter of seawater per sample was successively filtered by using the 3- and 0.22-μm pore size polycarbonate nucleopore membranes (Merck Millipore Ltd., United States), and then the membranes were stored in 5-ml sterile cryopreservation tubes at −20°C during the cruise and at −80°C after returning to the laboratory.
2.2 DNA extraction, PCR amplification, and sequencing
The genomic DNA from each water sample was exacted using the FastPrep®-24 rapid nucleic acid extraction kit (MP Biomedicals, United States) according to the manufacturer’s instructions. The concentration of purified DNA was measured using Nanodrop 2000 (Thermo, United States). The DNA solution from each duplicate sample was pooled together to avoid the extraction bias. The 16S rRNA genes were amplified using the archaeal primers 515F (5ʹ-GTGCCAGCMGCCGCGG-3ʹ) and 907R (5ʹ-CCGTCAATTCMTTTRAGTTT-3ʹ) (Muyzer et al., 1993). The PCR products were detected by the 1.2% agarose gel electrophoresis and were purified by using the MiniBEST Agarose Gel DNA Extraction Kit Version 4.0 (TaKaRa, Japan) following the manufacturer’s instructions. The purity and concentration of the PCR products were determined by Nanodrop 2000 (Thermo, United States). The PCR products were sent to Shanghai Majorbio Bio-pharm Technology Co., Ltd., (China) for the high-throughput sequencing using the Miseq platform (Illumina, United States).
2.3 Measurement of environmental parameters
The environmental parameters, including pH, salinity, DO, temperature, chemical oxygen demand (COD), and concentration of chlorophyll a (Chl a) in the seawater were determined by using an SBE2 sampler (Washington Seabird, United States) in situ. The concentrations of nitrite (N-NO2), nitrate (N-NO3), and ammonia nitrogen (N-NH4) in the seawater samples were determined by using the ultraviolet spectrophotometry method (Qin et al., 2022). The concentration of phosphate (P-PO4) in the samples was measured by using a QuAAtro continuous flow analyzer (SEAL Analytical, Germany) (Wu et al., 2019). The silicate concentration in the seawater were determined by using the method of ion exclusion chromatography with conductivity detection (Li and Chen, 2000).
2.4 Data analysis
The DNA sequences were processed using QIIME, USEARCH, and FLASH software. First, the paired-end reads were combined with FLASH (Hawley et al., 2014). Then, the adapters were trimmed and the low-quality sequences were discarded using QIIME. Chimera sequences were assessed and filtered with USEARCH. Finally, the clean sequences were aligned against the SILVA 132 database and were clustered into operational taxonomic units (OTUs) with 97% sequence identity. In order to assess the archaeal richness and diversity, the Chao1, ACE, Shannon, and Simpson indexes were calculated after normalizing the sequences to 30,125 reads by using QIIME. Principal coordinate analysis (PCoA), clustering analysis, redundancy analysis (RDA), and Mantel test were conducted by using R packages of ape, ggtree, vegan, and linkET, respectively. Variation partition analysis (VPA) was analyzed by using R package of vegan. Networks of archaeal communities were constructed using the Molecular Ecological Network Analyses (MENA) Pipeline with a St value of 0.850 (http://129.15.40.240/mena/) (Deng, 2012). The OTUs with Zi values ≥ 2.5 or Pi values ≥ 0.62 were identified as the keystone taxa (Olesen et al., 2007). The networks were visualized by using Cytoscape (https://cytoscape.org/).
3 Results
3.1 Statistical information of the environmental properties
The environmental factors changed vertically between the hypoxic and non-hypoxic zones (Figure 2). N-NO3, N-NO2, N-NH4, and temperature were significantly higher in the non-hypoxic zones (Student’s t-test, p < 0.05). In contrast, P-PO4 and salinity were significantly lower in the non-hypoxic zones (Student’s t-test, p < 0.05). The DO was significantly lower in the hypoxic zones (Student’s t-test, p < 0.05). Silicate was not significantly different between the two zones.
3.2 Richness and diversity of archaeal communities
The 24 samples yielded a total of 1,050,783 raw reads. A total of 921,221 clean reads were retained for the downstream analysis. All normalized sequences were grouped into 1,375 OTUs. Samples in hypoxic zones were comprised of 128–241 OTUs, while samples in non-hypoxic zones were comprised of 141–238 OTUs. A total of 234 OTUs were shared between the hypoxic and non-hypoxic zones (Figure 3A). Additionally, 124 OTUs were significantly different between the two zones. Compared to the non-hypoxic zone, 55 OTUs were enriched and 69 OTUs were depleted in the hypoxic zone (Figure 3B). No significant differences were detected in the richness and diversity indexes between the hypoxic and non-hypoxic zones (Student’s t-test, p > 0.05), except for the chao1 index (Student’s t-test, p < 0.05) (Figure 4).
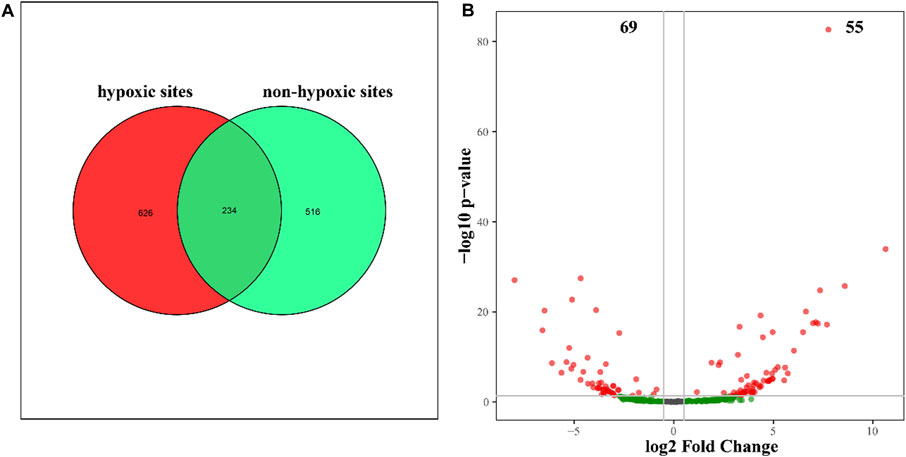
FIGURE 3. Unique and shared OTUs between the hypoxic and non-hypoxic zones. (A) Venn diagram showing the OTUs unique to the hypoxic (red) and non-hypoxic (green) zones and the shared OTUs (dark green). (B) Volcano plot showing the significant differences between enriched (red) and depleted (green) hypoxic OTUs.
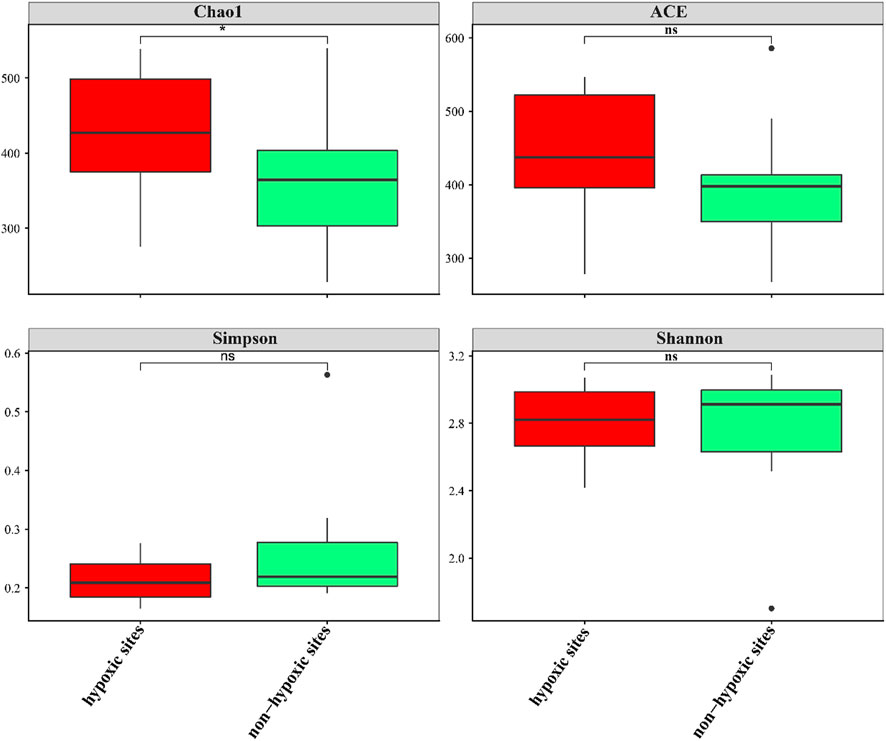
FIGURE 4. Box plots of the alpha diversity of the archaeal communities between the hypoxic and non-hypoxic zones.
3.3 Archaeal community composition
The results of PCoA and clustering analysis revealed that the samples formed two groups based on the hypoxic and non-hypoxic zones (Figure 5; Supplementary Figure S1A). Hypoxic samples were clearly separated from non-hypoxic samples, indicating that there was a distinct archaeal structure between the two zones. Moreover, archaeal communities were dissimilar between the hypoxic and non-hypoxic zones (R = 0.993, p = 0.001), where the archaeal communities in the hypoxic zone had a higher similarity composition (Supplementary Figure S1B).
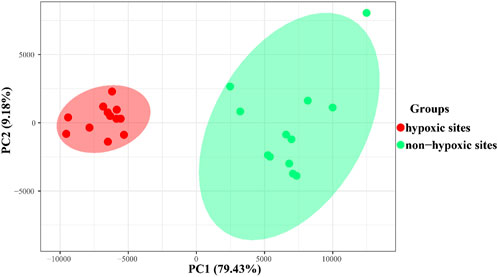
FIGURE 5. PCoA plot showing the archaeal similarities within the hypoxic and non-hypoxic zones and dissimilarities between zones based on Bray-Curtis distances.
Euryarchaeota was abundant in the non-hypoxic zones, while Thaumarchaeota was prevalent in the hypoxic zones (Figure 6A). Moreover, the two major classes exhibited opposing trends, with MG II and Nitrosopumilales dominating the non-hypoxic and hypoxic zones, respectively (Figure 6B). Further analysis at the phylum and order levels showed that the abundance of the archaeal community was significantly different between the two zones (Supplementary Table S1).
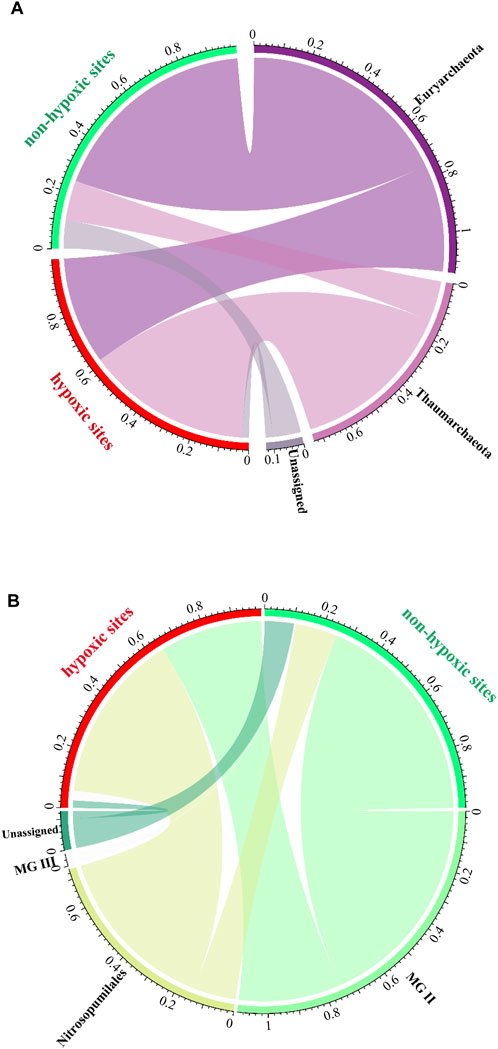
FIGURE 6. Archaeal community composition of the hypoxic and non-hypoxic zones at the phylum (A) and order (B) levels.
3.4 Relationship between local environmental factors and archaeal communities
The archaeal richness was significantly and negatively correlated with DO (p < 0.05) and temperature, but was positively correlated with P-PO4 (Supplementary Figure S2). N-NH4 and N-NO3 were positively correlated with the archaeal Simpson indexes, while salinity was negatively correlated with the Simpson indexes. The archaeal Shannon indexes were negatively correlated with N-NH4 (Supplementary Figure S2). The Mantel test revealed that the environmental factors except silicate significantly influenced the archaeal communities (p < 0.05; Figure 7). The RDA result showed that DO (p = 0.001), N-NO3 (p = 0.001), N-NH4 (p = 0.001), P-PO4 (p = 0.001), temperature (p = 0.001), N-NO2 (p = 0.001), and salinity (p = 0.001) significantly influenced the archaeal communities (Figure 8A; Table 1). The VPA result indicated that the salinity and temperature accounted for the most archaeal variation in the hypoxic and non-hypoxic zones (Figure 8B). In the hypoxic zone, DO (p = 0.026) was the most important factor that shaped the archaeal composition (Supplementary Figure S3; Supplementary Table S2). DO and temperature were negatively correlated with Thaumarchaeota and Nitrosopumilales, but was positively correlated with Euryarchaeota and MG II. Salinity was positively correlated with Thaumarchaeota, Nitrosopumilales, and MG III, but was negatively correlated with MG II and Euryarchaeota. N-NH4, N-NO3, and N-NO2 were positively correlated with Euryarchaeota and MG II, but was negatively correlated with Thaumarchaeota, MG III, and Nitrosopumilales (Supplementary Figure S4).
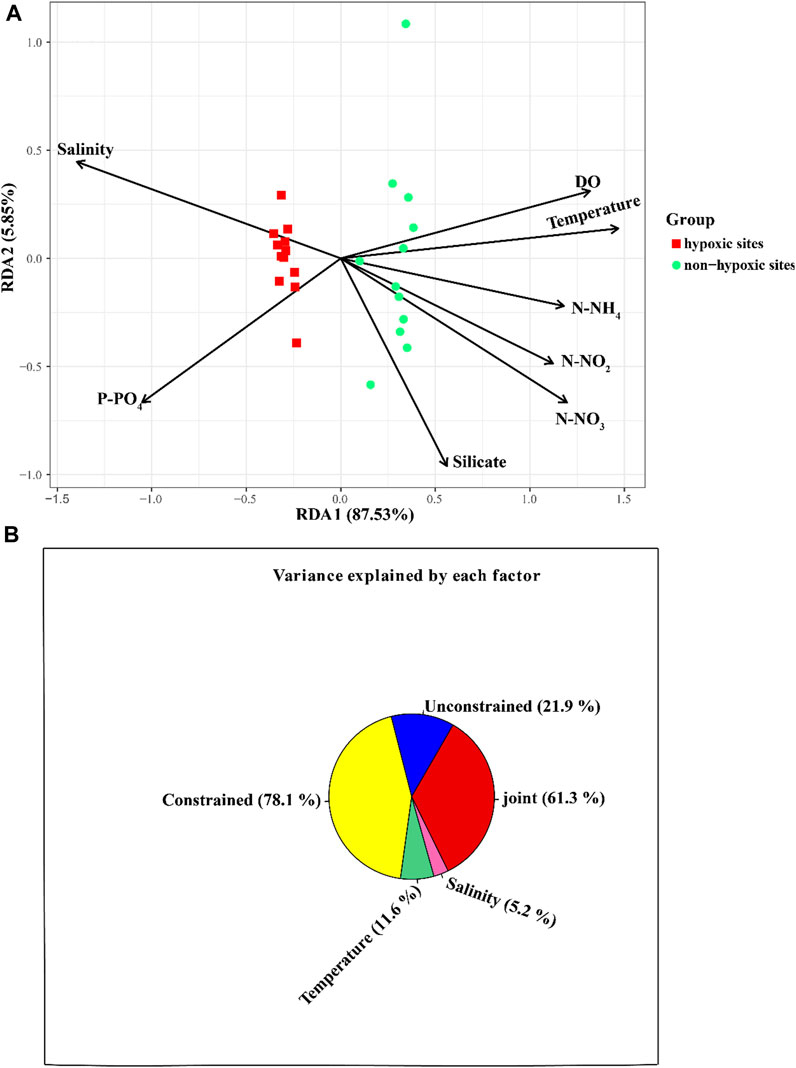
FIGURE 8. Relationship between environmental factors and archaeal community at the OTU level. (A) RDA showing the relationship between environmental variables and archaeal community in the Changjiang Estuary. (B) VPA analysis for contribution of environmental factors to archaeal composition.
3.5 Archaeal community networks in the hypoxic and non-hypoxic zones
The archaeal community networks in the hypoxic zones had 108 nodes and 486 edges, while those in the non-hypoxic zones had 105 nodes and 462 edges (Figure 9; Supplementary Table S3). The modularity values of the archaeal networks in hypoxic and non-hypoxic zones were 0.35 and 0.42, respectively (Figure 9; Supplementary Table S3). Both the hypoxic (84%) and non-hypoxic (86%) networks possessed a high proportion of positive correlation for the interspecific interaction of the archaeal communities. The Zi-Pi plot revealed that four module hubs were identified between the two networks (Supplementary Figure S5). No keystone taxa were identified in the archaeal networks from the non-hypoxic samples, and MGII was identified as the keystone taxa in the archaeal networks from the hypoxic samples.
4 Discussion
4.1 Archaeal community richness and diversity in the Changjiang Estuary
Archaeal community richness and diversity in the estuary were previously investigated (Gordon et al., 2015; Jiwen et al., 2015; Liu et al., 2018). Gordon et al. (2015) found that archaeal richness and diversity decreased with the depth (Gordon et al., 2015). However, Jiwen et al. (2015) found that archaeal richness and diversity were not significantly different between the surface and bottom water samples (Jiwen et al., 2015). A separate study on the archaea in the global estuaries found that the archaeal richness and diversity varied with latitude (Liu et al., 2018). Previous studies have investigated archaeal richness and diversity in the Changjiang Estuary (Zeng et al., 2007; Liu et al., 2011; Hui et al., 2014). However, there is limited knowledge on the environmental factors that influence archaeal richness and diversity in the Changjiang Estuary to date. In this study, high-throughput sequencing was used to compare the archaeal communities of the hypoxic and non-hypoxic zones. Compared to a previous study (Zeng et al., 2007), the Shannon and Simpson indexes of the archaea in this study were considerably higher, which may be due to the different sequencing methods. Zeng et al. (2007) used PCR-restriction fragment length polymorphism to examine the archaeal community pattern in the Changjiang Estuary. However, high-throughput sequencing, as used in this study, can detect more phyla than traditional tools. Additionally, the archaeal richness in the hypoxic zone was slightly higher than that in the non-hypoxic zone. In our previous study, the bacterial richness was significantly higher in the hypoxic zone than in the non-hypoxic zone (Wu et al., 2019). Clearly, both bacterial and archaeal richness were higher in the hypoxic zone. Furthermore, archaeal richness was not significantly different between the hypoxic and non-hypoxic zones (Student’s t-test, p > 0.05). This is in agreement with the findings of a previous study (Gillies et al., 2015). The Spearman correlation analysis indicated that archaeal richness exhibited a positive correlation with P-PO4, but was significantly and negatively correlated with DO. This suggests that the archaeal richness in the Changjiang Estuary was greatly impacted by DO. Furthermore, the Simpson index was positively correlated with N-NH4 and N-NO3, while the Shannon index was negatively correlated with N-NH4, indicating that nutrient concentrations may be important factors that influenced archaeal diversity in the Changjiang Estuary.
4.2 Archaeal community structure in the Changjiang Estuary
The temporal and spatial changes of the archaeal community structure in many marine ecosystems and lakes have been well-documented (Massana et al., 2000; Church et al., 2003; Jiang et al., 2009; Meyerhof, 2011; Yan et al., 2015). The PCoA and clustering analysis revealed that the archaeal community between the hypoxic and non-hypoxic zones was different. Furthermore, samples from the hypoxic zone possessed a similar archaeal community structure. Liu et al. (2015) studied shifts in archaea plankton community structure in the Pearl Estuary and found that the archaeal community in the hypoxic and non-hypoxic sites grouped together (Jiwen et al., 2015). A separate study found that the archaeal community structure in hypoxic samples was distinct from non-hypoxic samples (Gillies et al., 2015). A remarkable difference in bacterial community structure between the hypoxic and non-hypoxic zones has also been observed (Wu et al., 2019), indicating that specific bacterial and archaeal community structures exist in the hypoxic and non-hypoxic zones of the Changjiang Estuary.
Currently, the relationship between the archaeal community structure and environmental factors is not well understood. A previous study indicated that temperature, nutrients, and DO may have an effect on archaeal communities in the seawater. In this study, the RDA and Mantel test revealed that salinity, DO, and nutrient content significantly influenced the archaeal community in the Changjiang Estuary. VPA indicated that temperature and salinity were the major environmental factors that shaped the archaeal community, which is consistent with a previous study that found that salinity had a significant effect on the archaeal community in the Changjiang Estuary (Liu et al., 2011). Hongyue et al., 2008 also reported that AOA significantly correlated with salinity in the sediments of the Changjiang Estuary. Liu et al. (2011) pointed out that this may be caused by the freshwater of the Changjiang River. Furthermore, DO was the most influential environmental factor that shaped the archaeal community in the hypoxic zone (p = 0.024). In contrast, Liu et al. (2011) found that DO had no significant influence on the archaeal community in the Changjiang Estuary, and samples from the hypoxic area did not group together. Therefore, we speculated that the different sampling sites and time may result in different findings.
In this study, Euryarchaeota (57.0%) and Thaumarchaeota (36.6%) dominated both the hypoxic and non-hypoxic zones. This is consistent with the findings of previous studies, which indicated that Thaumarchaeota and Euryarchaeota were the two major phyla in the estuaries (Liu et al., 2011; Yan et al., 2015) and marine system (Liu et al., 2011). In this study, Euryarchaeota abundance was significantly higher in the non-hypoxic zones, while Thaumarchaeota dominated the hypoxic zone samples. At the order level, Nitrosopumilales (36.6%) and MG II (55%) were the predominant groups, which is in agreement with previous studies (Zeng et al., 2007; Liu et al., 2011). In this study, MG III was also detected with a low proportion (2%). MG III was reported to be distantly related to Thermoplasmales (López-Garcı́;A et al., 2001). Quaiser et al. (2013) pointed out that MG III is usually detected in deep waters. This is in agreement with our study that MGIII was found with significantly higher abundance in bottom waters (Student’s t-test, p < 0.05).
Only a small proportion of OTUs was shared between the archaeal communities in the hypoxic and non-hypoxic zones, and 55 OTUs were significantly enriched in the hypoxic zones. These results further confirmed that the hypoxic and non-hypoxic habitats differed remarkably in terms of their archaeal community structure. However, the knowledge on how environmental factors influence phyla in these zones is still limited.
In this study, spearman correlation analysis indicated that Euryarchaeota positively correlated with DO, N-NO2, N-NO3, and N-NH4 but negatively correlated with P-PO4 and salinity. Thaumarchaeota was positively correlated with P-PO4 and salinity, but was negatively correlated with N-NO2, N-NO3, N-NH4, and DO. Gillies et al. (2015) also found that Thaumarchaeota was negatively correlated with DO (R = −0.061) but was positively correlated with P-PO4 (R = 0.75). These findings are logical, considering previous studies have reported that Thaumarchaeota plays a key role in the nitrogen cycle (Schleper and Nicol, 2010; Walker et al., 2010).
Nitrosopumilales was an isolated culture of AOA (Kirchman et al., 2005). In this study, Nitrosopumilales was abundant in the Changjiang Estuary and was abundant in the hypoxic zone. Thus, we speculated that the high proportions of Thaumarchaeota and Nitrosopumilales may play important roles in the nitrification process of the hypoxic zone in the Changjiang Estuary. However, this hypothesis requires further investigation by analyzing the abundance of AOA or amoA genes in the area.
MG II was significantly abundant in the non-hypoxic zone (surface water). This mathed the findings of previous studies that found that MG II was prevalent in surface waters (Rinke et al.; Massana et al., 2000; Jiwen et al., 2015). Tseng et al. (2015) also found that MG II was predominant in the surface waters of the South China Sea (Tseng et al., 2015). Moreover, a previous study reported that MG II had the ability to degrade protein and lipids (Jia et al., 2012). Liu et al. (2017) also observed that MG II dominated surface waters, while Thaumarchaeota was mainly distributed in deep waters (Liu et al., 2017). In this study, MG II was positively correlated with DO and nutrient levels, but was negatively correlated with salinity. Additionally, the spearman correlation analysis indicated that DO, nutrient levels, and salinity may be the key environmental factors that shaped the archaeal community in the Changjiang Estuary, which was also supported by the RDA results.
4.3 Network analysis in the hypoxic zones and non-hypoxic zones
In this study, the archaeal community networks in the hypoxic and non-hypoxic zones were similar in size, and the higher positive correlation in the networks indicated that there was less competition among the archaeal species from the hypoxic and non-hypoxic zones. In our previous study, bacteria in the hypoxic zone experienced more competition than in the non-hypoxic zone (Wu et al., 2019). Clearly, archaea and bacteria respond differently to hypoxia.
The community networks are considered modular when the modularity values are >0.4 (Newman, 2006). The modularity value of the archaeal community network in the non-hypoxic zone was 0.42, which revealed that the non-hypoxic network was modular. Additionally, the number of modules, clustering coefficient, and network centralization values were 4, 0.346, and 0.229 in the hypoxic network and 5, 0.380, and 0.237 in the non-hypoxic network, respectively. Zi and Pi were described previously (Olesen et al., 2007) and were used to describe a node that connects to other nodes or modules. Thus, Zi and Pi divided the OTUs into four categories: module hubs, peripherals, network hubs, and connectors, which was important for microorganisms (Chen et al., 2018). In ecology, generalists play key roles in promoting the exchange of energy and nutrients, as well as in maintaining the balance of microbial communities (Chen et al., 2018). In this study, both the hypoxic and non-hypoxic networks had no network hubs, indicating that there was no node with many links within its modules and other modules. Only five generalists were found in the hypoxic zone, while thirteen generalists were found in the non-hypoxic zone. Therefore, we speculated that the archaeal community in the non-hypoxic zone was more stable and ordered than that in the hypoxic zone.
Interestingly, OTU 41 was a generalist in the hypoxic network, but a specialist in the non-hypoxic network. Moreover, the generalists OTUs (OTU 406 and OTU 1173) in the non-hypoxic network were specialists in the hypoxic network. OTU 406 and OTU 1173 belonged to MG II. As generalist in the non-hypoxic network, MG II played key roles in connecting other archaea and forming a stable ecological system in the non-hypoxic zone. However, as specialist in the hypoxic network, MG II was rarely connected other archaea. These results suggest these OTUs exhibit role-shifts. A previous study also reported that the same bacteria could serve different functions under two different environments (Chen et al., 2018). Most generalists in the hypoxic and non-hypoxic networks belonged to MG II, indicating that MG II plays a significant role in exchanging energy, metabolites, and nutrients, as well as maintaining the balance of the microbial community in the Changjiang Estuary.
5 Conclusion
There was a distinct archaeal communities’ pattern between the hypoxic and non-hypoxic zones in the seawater of the Changjiang Estuary. RDA results showed that temperature and salinity were the major environmental factors that shaped the archaeal communities in the seawater of the Changjiang Estuary. The archaeal richness was significantly influenced by DO, while archaeal diversity was influenced by nutrient levels, and MG II displayed different functions in the hypoxic and non-hypoxic zones. All of these can enhance our understanding of archaeal communities in the seawater of the Changjiang Estuary.
Data availability statement
The datasets presented in this study can be found in online repositories. The names of the repository/repositories and accession number(s) can be found in the article/Supplementary Material.
Author contributions
RW designed this work. CT, DW, and RW revised the manuscript; JW collected the samples, performed the experiments, and wrote this paper.
Funding
This work was supported by National Key R&D Program of China (2019YFD0901305) and the Fundamental Research Fund for the Provincial Universities of Zhejiang (2021JD003).
Conflict of interest
The authors declare that the research was conducted in the absence of any commercial or financial relationships that could be construed as a potential conflict of interest.
Publisher’s note
All claims expressed in this article are solely those of the authors and do not necessarily represent those of their affiliated organizations, or those of the publisher, the editors and the reviewers. Any product that may be evaluated in this article, or claim that may be made by its manufacturer, is not guaranteed or endorsed by the publisher.
Supplementary material
The Supplementary Material for this article can be found online at: https://www.frontiersin.org/articles/10.3389/fenvs.2023.1139237/full#supplementary-material
References
Chen, S., Qi, G., Luo, T., Zhang, H., Jiang, Q., Wang, R., et al. (2018). Continuous-cropping tobacco caused variance of chemical properties and structure of bacterial network in soils. Land Degrad. Dev. 29 (11), 4106–4120. doi:10.1002/ldr.3167
Church, M. J., DeLong, E. F., Ducklow, H. W., Karner, M. B., Preston, C. M., Karl, D., et al. (2003). Abundance and distribution of planktonic archaea and bacteria in the waters west of the Antarctic Peninsula. Limnol. Oceanogr. 48 (5), 1893–1902. doi:10.4319/lo.2003.48.5.1893
Cornelia, W., Ben, A., Coolen, M. J. L., Lydie, H., Judith, V. B., Peer, T., et al. (2006). Archaeal nitrification in the ocean. Proc. Natl. Acad. Sci. 103 (33), 12317–12322. doi:10.1073/pnas.0600756103
Deng, Y., Jiang, Y. H., Yang, Y., He, Z., Luo, F., and Zhou, J. (2012). Molecular ecological network analyses. BMC Bioinforma. 13 (1), 113. doi:10.1186/1471-2105-13-113
Gillies, L. E., Thrash, J. C., Derada, S., Rabalais, N. N., and Mason, O. (2015). Archaeal enrichment in the hypoxic zone in the northern Gulf of Mexico. Environ. Microbiol. 17 (10), 3847–3856. doi:10.1111/1462-2920.12853
Gordon, W., O'Sullivan, L. A., Yiyu, M., Williams, A. S., Sass, A. M., Watkins, A. J., et al. (2015). Archaeal community diversity and abundance changes along a natural salinity gradient in estuarine sediments. FEMS Microbiol. Ecol. 91 (2), 1–18. doi:10.1093/femsec/fiu025
Hawley, E. R., Malfatti, S. A., Pagani, I., Huntemann, M., Chen, A., Foster, B., et al. (2014). Metagenomes from two microbial consortia associated with Santa Barbara seep oil. Mar. Genomics 18, 97–99. doi:10.1016/j.margen.2014.06.003
Heike, S., and Osvaldo, U. (2010). Bacterial diversity in the oxygen minimum zone of the eastern tropical South Pacific. Environ. Microbiol. 10 (5), 1244–1259. doi:10.1111/j.1462-2920.2007.01539.x
Hongyue, D., Xiaoxia, Z., Jin, S., Tiegang, L., Zhinan, Z., and Guanpin, Y. J. M. (2008). Diversity and spatial distribution of sediment ammonia-oxidizing crenarchaeota in response to estuarine and environmental gradients in the Changjiang Estuary and East China Sea. Microbiology 154 (7), 2084–2095. doi:10.1099/mic.0.2007/013581-0
Huabing, L., Peng, X., and Wu, Q. L. (2012). Characterization of the bacterial community composition in a hypoxic zone induced by Microcystis blooms in Lake Taihu, China. FEMS Microbiol. Ecol. 79 (3), 773–784. doi:10.1111/j.1574-6941.2011.01262.x
Hui, H., Yu, Z., Mi, T., and Yu, Z. (2014). Community composition and abundance of ammonia-oxidizing archaea in sediments from the Changjiang Estuary and its adjacent area in the East China Sea. Geomicrobiol. J. 33 (5), 416–425. doi:10.1080/01490451.2014.986695
Jia, G., Zhang, J., Chen, J., Peng, P. A., and Zhang, L. (2012). Archaeal tetraether lipids record subsurface water temperature in the South China Sea. Org. Geochem. 50 (50), 68–77. doi:10.1016/j.orggeochem.2012.07.002
Jiang, H., Dong, H., Deng, S., Yu, B., Huang, Q., and Wu, Q. (2009). Response of archaeal community structure to environmental changes in lakes on the Tibetan Plateau, Northwestern China. Geomicrobiol. J. 26 (4), 289–297. doi:10.1080/01490450902892662
Jingxu, Z., Yuyin, Y., Lei, Z., Yuzhao, L., Shuguang, X., Yong, L., et al. (2015). Distribution of sediment bacterial and archaeal communities in plateau freshwater lakes. Appl. Microbiol. Biotechnol. 99 (7), 3291–3302. doi:10.1007/s00253-014-6262-x
Jiwen, L., Shaolan, Y., Meixun, Z., Biyan, H., and Xiao-Hua, Z. (2015). Shifts in archaeaplankton community structure along ecological gradients of Pearl Estuary. FEMS Microbiol. Ecol. 90 (2), 424–435. doi:10.1111/1574-6941.12404
Ken, T., Hanako, O., Yohey, S., Hisako, H., Satoshi, N., Takuro, N., et al. (2004). Spatial distribution of marine crenarchaeota group I in the vicinity of deep-sea hydrothermal systems. Appl. Environ. Microbiol. 70 (4), 2404–2413. doi:10.1128/aem.70.4.2404-2413.2004
Kirchman, D., Dittel, A., Malmstrom, R. R., and Cottrell, M. T. (2005). Biogeography of major bacterial groups in the Delaware Estuary. Limnol. Oceanogr. 50, 1697–1706. doi:10.4319/lo.2005.50.5.1697
Li, D., Zhang, J., Huang, D., Wu, Y., and Liang, J. (2002). Oxygen depletion off the Changjiang (yangtze river) estuary. Sci. China Ser. D Earth Sci. 45 (12), 1137–1146. doi:10.1360/02yd9110
Li, H. B., and Chen, F. (2000). Determination of silicate in water by ion exclusion chromatography with conductivity detection. J. Chromatogr. A 874 (1), 143–147. doi:10.1016/s0021-9673(00)00078-9
Liu, H., Zhang, C. L., Yang, C., Chen, S., Cao, Z., Zhang, Z., et al. (2017). Marine group II dominates planktonic archaea in water column of the Northeastern South China Sea. Front. Microbiol. 8, 1098. doi:10.3389/fmicb.2017.01098
Liu, M., Xiao, T., Wu, Y., Zhou, F., and Zhang, W. (2011). Temporal distribution of the archaeal community in the Changjiang Estuary hypoxia area and the adjacent East China Sea as determined by denaturing gradient gel electrophoresis and multivariate analysis. Can. J. Microbiol. 57 (6), 504–513. doi:10.1139/w11-037
Liu, X., Pan, J., Liu, Y., Li, M., and Gu, J. (2018). Diversity and distribution of Archaea in global estuarine ecosystems. Sci. Total Environ. 637, 349–358. doi:10.1016/j.scitotenv.2018.05.016
López-Garcı́A, P., López-López, A., Moreira, D., and Rodrı́Guez-Valera, F. (2001). Diversity of free-living prokaryotes from a deep-sea site at the Antarctic Polar Front. FEMS Microbiol. Ecol. 36 (2), 193–202. doi:10.1016/s0168-6496(01)00133-7
Madrid, V. M., Taylor, G. T., Scranton, M. I., and Chistoserdov, A. Y. (2001). Phylogenetic diversity of bacterial and archaeal communities in the anoxic zone of the Cariaco Basin. Appl. Environ. Microbiol. 67 (4), 1663–1674. doi:10.1128/aem.67.4.1663-1674.2001
Martin, K. N., Bernhard, A. E., Torre, J. R., de la Torre, J. R., Walker, C. B., Waterbury, J. B., et al. (2005). Isolation of an autotrophic ammonia-oxidizing marine archaeon. Nature 437 (7058), 543–546. doi:10.1038/nature03911
Massana, R., Delong, E. F., and Pedrós-Alió, C. (2000). A few cosmopolitan phylotypes dominate planktonic archaeal assemblages in widely different oceanic provinces. Appl. Environ. Microbiol. 66 (5), 1777–1787. doi:10.1128/aem.66.5.1777-1787.2000
Meyerhof, M. (2011) ProQuest ID: 2011_mmeyerhof. Diversity and distribution of archaea and bacteria in marine lakes, palauMerced, CA, USA: UC Merced.
Muyzer, G., de Waal, E. C., and Uitterlinden, A. G. (1993). Profiling of complex microbial populations by denaturing gradient gel electrophoresis analysis of polymerase chain reaction-amplified genes coding for 16S rRNA. Appl. Environ. Microbiol. 59 (3), 695–700. doi:10.1128/aem.59.3.695-700.1993
Newman, M. (2006). Modularity and community structure in networks. Proc. Natl. Acad. Sci. 103 (23), 8577–8582. doi:10.1073/pnas.0601602103
Olesen, J. M., Jordi, B., Dupont, Y. L., and Pedro, J. (2007). The modularity of pollination networks. Proc. Natl. Acad. Sci. 104 (50), 19891–19896. doi:10.1073/pnas.0706375104
Orita, R., Umehara, A., Komorita, T., Choi, J. W., Montani, S., Komatsu, T., et al. (2015). Contribution of the development of the stratification of water to the expansion of dead zone: A sedimentological approach. Estuar. Coast. Shelf Sci. 164 (2), 204–213. doi:10.1016/j.ecss.2015.07.028
Qin, J., Wu, K., Chen, L., Wang, X., Zhao, Q., Liu, B., et al. (2022). Achieving high selectivity for nitrate electrochemical reduction to ammonia over MOF-supported RuxOy clusters. J. Mater. Chem. A 10 (8), 3963–3969. doi:10.1039/d1ta09441f
Quaiser, A., Zivanovic, Y., Moreira, D., and Lópezgarcía, P. (2013). Comparative metagenomics of bathypelagic plankton and bottom sediment from the sea of Marmara. ISME J. 5 (2), 285–304. doi:10.1038/ismej.2010.113
Rinke, C., Rubino, F., Messer, L. F., Youssef, N., Parks, D. H., Chuvochina, M., et al. (2019). A phylogenomic and ecological analysis of the globally abundant Marine Group II archaea (Ca. Poseidoniales ord. nov.). ISME J. 13 (3), 663–675. doi:10.1038/s41396-018-0282-y
Schleper, C., and Nicol, G. (2010). Ammonia-oxidising archaea-physiology, ecology and evolution. Adv. Microb. Physiology 57, 1–41. doi:10.1016/B978-0-12-381045-8.00001-1
Tseng, C. H., Chiang, P. W., Lai, H. C., Shiah, F. K., Hsu, T. C., Chen, Y. L., et al. (2015). Prokaryotic assemblages and metagenomes in pelagic zones of the South China Sea. BMC Genomics 16 (1), 1–16. doi:10.1186/s12864-015-1434-3
Walker, C. B., de la Torre, J. R., Klotz, M. G., Urakawa, H., Pinel, N., Arp, D. J., et al. (2010). Nitrosopumilus maritimus genome reveals unique mechanisms for nitrification and autotrophy in globally distributed marine crenarchaea. Proc. Natl. Acad. Sci. 107 (19), 8818–8823. doi:10.1073/pnas.0913533107
Wishner, K. F., Ashjian, C. J., Gelfman, C., Gowing, M. M., Kann, L., Levin, L. A., et al. (1995). Pelagic and benthic ecology of the lower interface of the Eastern Tropical Pacific oxygen minimum zone. Deep Sea Res. Part I Oceanogr. Res. Pap. 42 (1), 93–115. doi:10.1016/0967-0637(94)00021-j
Wu, D. M., Dai, Q. P., Liu, X. Z., Fan, Y. P., and Wang, J. X. (2019). Comparison of bacterial community structure and potential functions in hypoxic and non-hypoxic zones of the Changjiang Estuary. PloS one 14 (6), e0217431. doi:10.1371/journal.pone.0217431
Yan, L., Liu, Q., Li, C., Yi, D., Zhang, W., Zhang, W., et al. (2015). Bacterial and archaeal community structures in the Arctic deep-sea sediment. Acta Oceanol. Sin. 34 (2), 93–113. doi:10.1007/s13131-015-0624-9
Zeng, Y., Li, H., and Jiao, N. (2007). Phylogenetic diversity of planktonic archaea in the estuarine region of East China Sea. Microbiol. Res. 162 (1), 26–36. doi:10.1016/j.micres.2006.03.007
Keywords: archaeal community, Changjiang Estuary, 16S rRNA, dissolved oxygen, network
Citation: Wang J, Tian C, Wu D and Wang R (2023) Dissolved oxygen shapes the archaeal communities in the seawater of Changjiang Estuary. Front. Environ. Sci. 11:1139237. doi: 10.3389/fenvs.2023.1139237
Received: 06 January 2023; Accepted: 07 February 2023;
Published: 27 March 2023.
Edited by:
Xiaoning Zhao, Nanjing University of Information Science and Technology, ChinaCopyright © 2023 Wang, Tian, Wu and Wang. This is an open-access article distributed under the terms of the Creative Commons Attribution License (CC BY). The use, distribution or reproduction in other forums is permitted, provided the original author(s) and the copyright owner(s) are credited and that the original publication in this journal is cited, in accordance with accepted academic practice. No use, distribution or reproduction is permitted which does not comply with these terms.
*Correspondence: Rixin Wang, wrx_zjou@163.com