- 1Beijing Forestry University, Beijing, China
- 2Beijing Laboratory of Urban and Rural Ecological Environment (BFU), Beijing, China
The accelerated urbanization process has caused problems such as habitat loss, isolation, and habitat quality decline, resulting in a sharp reduction in the richness and abundance of urban species. Constructing suitable habitat environmental conditions is the most direct and effective way to protect animals. In urban environment, habitat construction can be achieved by integrating species protection and landscape planning, which is also an important manifestation of biodiversity conservation at the ecosystem level. Understanding how to incorporate animal habitats into city plan and design is critical and urban planners would benefit from a review that holistically describes the steps and methods of animal habitat constructions. We conducted a review to highlight the animal habitats space resources and network structures. We synthesized the findings of research studies in the last 20 years to illuminate the investigation, assessment, planning and management of animal habitats. As habitat degradation and fragmentation in anthropogenic environments, our findings suggest city planners should consider ecological background investigation, habitat suitability assessment, habitat planning strategies and animal habitat management as four key steps of mitigation to alleviate these impacts. This study will provide a useful reference to improve animal survival quality and communication. Through this study, the consolidated research can aid in sustainable development and innovation to promote the ecological function of urban green space and the harmonious coexistence of humans and animals.
1 Introduction
With the acceleration of urbanization, a large amount of land is used for development and construction (Fahrig, 2019). As a result, various ecological and environmental problems have emerged, such as biodiversity loss, landscape fragmentation, ecosystem services decline, and even regional or global environmental changes (Wu, 2010; Liang et al., 2019). The biodiversity crisis in modern cities is mainly due to the greater degree of habitat degradation and fragmentation (Haddad et al., 2015; Driscoll et al., 2013; Fahrig, 2003; Fischer and Lindenmayer, 2007; Laurance, 2018; Hilty et al., 2020). Habitat degradation means that animals do not have sufficient and high-quality habitat space (Thomas and Blakemore, 2007; Conrad et al., 2012). Habitat fragmentation means that the exchange of genes and individuals can be hindered by barriers created by fragmentation (Cheptou et al., 2017; Wang et al., 2020; Yang et al., 2021). Animal habitats constructions in urban areas are an effective approach because they can ensure the sufficient animal habitats, improve the quality of animal habitats and eliminate obstacles formed between habitat fragments (Pascual-Hortal and Saura, 2007; Albert et al., 2017; Schwarz et al., 2017; Russo and Holzer, 2021). The construction of animal habitats is not simply to increase the green area or improve the connectivity of patches, but to optimize the spatial layout through identifying suitable habitat sources and building a reasonable connectivity network based on understanding the habitat preferences of key species (Northrup et al., 2022), which will give full play to the ecological service function of urban green space as animal habitats.
At present, many scholars have conducted research on urban biodiversity and animal habitats, including key influencing factors (Soga and Koike, 2013; Gan, 2018) and distribution patterns (Farinha-Marques et al., 2017), scientific assessment methods (Wang et al., 2019), planning strategies (Xu and Shen, 2009; Ma et al., 2021; Su and Dai, 2021), design methods and management measures (Deng et al., 2015) and so on. Generally speaking, they focused on different scientific questions and how to integrate all the studies above is critical. The literature reviews on animal habitats constructions tend to be discipline-based and urban planners would benefit from a review that holistically describes the steps and methods of animal habitat constructions. We conducted this review of research to highlight the animal habitats space resources and network structures. We synthesized the findings of research studies in the last 20 years to illuminate the investigation, assessment, planning and management of animal habitats. Our study will provide a useful reference for urban planners and land managers so they can better carry out the ecological practices to strengthen the ecosystem services function of habitat in a metropolitan environment.
2 Methods
We reviewed papers published in international peer-reviewed journals of the Web of Science by using the topic “animal habitat construction” and consulting to the group composed of professionals working in Beijing Forestry University that have diverse ecological resource and zoological science backgrounds to summarize the content involved in the animal habitat constructions. These ideas were then consolidated into a scheme consisting of 4 key steps to restore and enhance the habitat functions in cities (Table 1). We broadly categorize these key steps as ecological background investigation, habitat suitability assessment, habitat planning strategies, and habitat management (Figure 1). The ecological background investigation includes animal resources survey and environmental resources survey. Habitat suitability assessment is based on the selection characteristics of species on environmental factors, comprehensively evaluates environmental factors, and finally obtains habitat suitability classification and spatial layout, including habitat patches assessment and connection evaluation. Habitat planning strategies can provide animals with effective living space and communication space according to the spatial pattern of habitat suitability assessment results, including the habitat patches quality improvement and the ecological corridor construction. Habitat patches should cover the main suitable habitat patches, and the area should be able to maintain the space requirements of a certain number of species. At the same time, patches should be integrated and connected to reduce fragmentation at the landscape level and enhance connectivity to facilitate regional population exchanges. Finally, habitat interference management and citizen science management should be carried out to ensure that habitats are rational and functional.
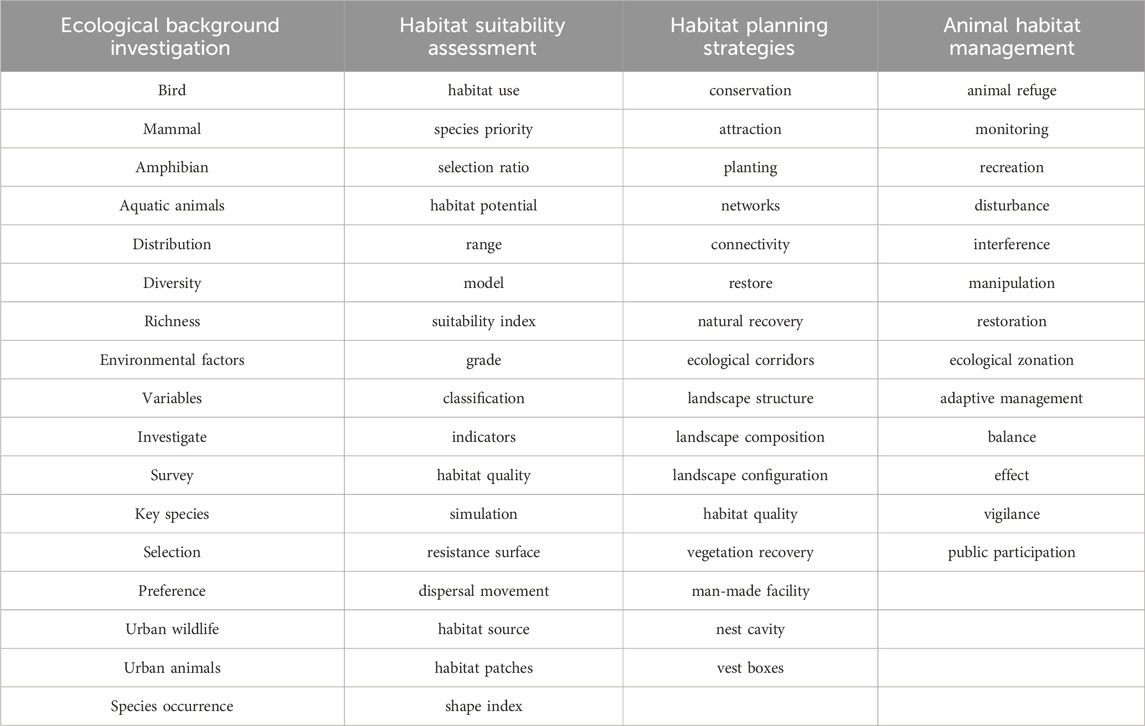
TABLE 1. Literature search keywords, 2000–2023. Each topic broadly categorized under ecological background investigation, habitat suitability assessment, habitat planning strategies, and animal habitat management and the keywords used for each topic to identify appropriate literature for the review.
In total, we used 63 keywords in the Web of Science to identify pertinent literature for each step (Table 1). We limited our initial search to peer-reviewed articles, research studies, and other publications published after the year 2000. Earlier publications with significant findings that contributed to the review were later included. We also include a handful of recently published articles that were suggested by our peer reviewers. In an effort to address the literature review topic succinctly, we narrowed the pertinent papers down to a total of 240 papers across all 4 topics. Through this procedure, we were able to provide a reference for future land management decisions and highlight gaps in the literature.
3 Results
3.1 Ecological background investigation
3.1.1 Animal resources survey
3.1.1.1 Animal groups selection
Urban green spaces (Wolch et al., 2014; Li et al., 2017) is vital in providing suitable animal habitats. There are many types of urban animals, and their habitat requirements are also heterogeneous, leading to the assembly of different communities in different urban settings (Aronson et al., 2016).
First, birds, as one of the critical components of biodiversity, are an important element of the food chains (Hedblom and Murgui, 2017). Urban birds have been widely used as environmental bioindicators to assess habitat quality and environmental change (Herrera-Dueñas et al., 2014; Pollack et al., 2017; Bernat-Ponce et al., 2021). Second, small mammals, mainly as primary and secondary consumers in food chains, also play an important role in ecosystem cycles. They are sensitive to environmental changes, because their habitats in cities are often fragmented or replaced by human activities (Fernández and Simonetti, 2013; Łopucki and Kitowski, 2017). Their group size can reflect the quality of habitat and the degree of human disturbance, and they can also be selected as indicator groups of urban biodiversity. Third, amphibians are animals with both aquatic and terrestrial life forms (Cayuela et al., 2020). With a diversity of habitat requirements, they are highly susceptible to negative impacts from habitat conditions changes. In addition, amphibians have strong skin permeability, so they are extremely sensitive to ultraviolet rays, chemical fertilizers, pesticides, herbicides, etc. They can also be used as indicator groups for urban biodiversity. Fourth, mainly as upper organisms of the aquatic food chain, fishes have a significant impact on the existence and abundance of other populations (Hong et al., 2023). They are also sensitive to changes in the water environment and can be used as indicator groups for urban biodiversity (Tonkin et al., 2017; Lyon et al., 2019).
Therefore, this paper selects birds, small mammals, amphibians and fishes as representatives of animal groups. Their habitats and activity spaces cover forests, shrubs, grasslands and waters, which can represent urban habitat types more comprehensively.
3.1.1.2 Focus species selection
In terms of habitat construction, it is not practical to monitor or survey every species. One or more species should be selected as typical indicators for habitat assessment and construction (Simberloff, 1998). Therefore, this paper adheres to the principle of focal species selection (Lambeck, 1997; Hess and King, 2002; Nicholson et al., 2013; Dondina et al., 2020). The selection differs from conservation biology, which is specific to endangered or protected species. While it is out of biological diversity enhancement, the focus species are selected based on their habitat type representation, conservation status, and role in ecological balance. By protecting the habitat required by the focal species, it extends to the most species protection in this group (Lindenmayer et al., 2014).
3.1.1.3 Species occurrence data
Through website or literature data collation, interviews, field surveys and other methods, we can obtain data on the types, quantities, distribution and activity patterns of existing animal species to understand the animal resources in the study area. The survey methods are shown in Table 2.
3.1.2 Environmental resources survey
An environmental resources survey obtains environmental data and clarifies habitat characteristics based on on-site investigation, map surveying, mapping, etc. Through literature reference (Arques et al., 2014; Bradfield et al., 2022; Callaghan et al., 2018; Cayuela et al., 2020; Churko et al., 2020; Curzel et al., 2021; Fahrig, 2003; Fidino et al., 2020; Fleury and Galetti, 2006; Gallo et al., 2017; Huang et al., 2021; Lerman et al., 2021; Matthies et al., 2017; Miller et al., 2022; Narango et al., 2017; Nielsen et al., 2014; Rico-Silva et al., 2021; Taylor et al., 2016; Yates et al., 1997), we determined the commonly used ecological variables around the species occurrence points, as shown in Table 3. In addition, we can calculate variance inflation factors for environmental variables (VIF, Quinn, and Keough, 2002) to avoid high multicollinearity affecting analysis results (Guisan and Zimmermann, 2000).
3.2 Habitat suitability assessment
3.2.1 Habitat patches assessment
3.2.1.1 Habitat preference analysis
Establishing animal habitats requires information about where animals are, why they are there, and when they might be (Aarts et al., 2008). Habitat selection studies provide insights into resource selection at landscape scales and improve our understanding of potential drivers of animal distributions (McIntyre, 1997; Razgour et al., 2011). So habitat preferences analysis is critical to enhancing the biodiversity of urban fringes’ green spaces. We can use SPSS for statistical analysis. For example, Pearson’s chi-square test (independence test) is used to compare the relationship between the specific location and the land category. Multiple correlations or regression analyses based on the generalized linear model (GLM) are used to compare the relationship between the environmental variables (such as green patch size and side length) and species richness.
According to IUCN Habitats Classification Scheme and land cover map (Lumbierres et al., 2022; IUCN, 2023), from the perspective of landscape planning and design in urban areas, we can divide the habitats of urban animals into three types:
(1) Green space habitat: it has a certain canopy coverage, mainly covered by trees, shrubs and grassland;
(2) Water and wetland habitat: it mainly includes lakes, ponds, streams, ditches, etc., and the vegetation is mostly aquatic plants such as reeds and irises;
(3) Artificial facility habitat: it is artificially placed some animal auxiliary facilities to help animals rebuild their habitat.
3.2.1.2 Habitat patches quality assessment
According to the results of species habitat preference analysis, the most important factors were selected as dominant habitat selection factors. ArcGIS can be used to preprocess data and build related spatial layers. Then weights are assigned to each factor, and in the current studies, analytic hierarchy, expert consultation and CRITIC are practical methods (Dong et al., 2013; Maleki et al., 2016; Yang et al., 2022). Finally, by overlaying factor layers using ArcGIS software, we can evaluate the animal habitats’ suitability. If the habitat suitability index is high, the regional biodiversity is equally high.
In addition, we can also choose simulation model to evaluate the quality of habitat patches. The Maximum Entropy Model (MaxEnt), based on the maximum entropy principle and niche theory, is a modeling method that uses mathematical models to induce or simulate the ecological niche requirements of species based on their specific living environment (Phillips et al., 2006). It is currently an effective tool for predicting and evaluating the spatial distribution pattern of suitable habitats for species. This model has a low requirement for the independence of data and can achieve high prediction accuracy even with a small number of distribution points (Pearson et al., 2007; Chen et al., 2012). Researches have shown that the MaxEnt model has significant advantages in terms of accuracy and convenience compared to many niche models (Elith et al., 2006; Gogol-Prokurat, 2011; Xu et al., 2015).
3.2.2 Habitat connection evaluation
Landscape connectivity refers to the degree to which landscape influences the movement of species between habitat patches, including structural connectivity and functional connectivity.
3.2.2.1 Structural connectivity evaluation
In terms of structural connectivity evaluation, MSPA is used to identify regions with significant connectivity from the pixel level (Wang and Pei, 2020; Rincón et al., 2021). Because habitats are landscape entities with considerable range and landscape characteristics, some quantitative information can be provided based on landscape indicators, such as fragmentation and proximity on the landscape spatial patterns (Zhang and Wang, 2006; Cui et al., 2020; Huang et al., 2022), which could be an important basis for connectivity assessment. Therefore, we can use Fragstats software and select the metrics including LSI (landscape shape index), PD (patch density), ENN_MN (mean euclidean nearest-neighbor distance), PROX_MN (mean proximity index), AI (aggregation index) (Zhang and Wang, 2006; Zhao et al., 2019; Cui et al., 2020; Huang et al., 2021).
3.2.2.2 Functional connectivity evaluation
However, the focus of recent studies has shifted from structural connectivity, and not only the spatial habitats arrangement, but also the functional connection of real biological movements and processes (Albert et al., 2017; Matos et al., 2019; Laliberté and St-Laurent, 2020). Graph theory is a technical approach for analyzing the functional connectivity and ecological conservation (Urban et al., 2009; Zetterberg et al., 2010; Clauzel et al., 2018). It presents habitat patches in the form of nodes (Urban et al., 2009; Galpern et al., 2011), and it is the most common and straightforward used method for measuring and assessing various aspects of habitat connectivity (Minor and Urban, 2008; Zhang, 2017). In graph theory, topological metrics introduced by network analysis methods are often used to evaluate functional connectivity (Huang et al., 2021b), simulating the relationships between network nodes (Nogués and Cabarga-Varona, 2014). Network analysis metrics include network ring Path α, the mean number of connections β, the ratio of the number of possible connections γ, and the network density φ (Cook, 2002; Zhao et al., 2019; Huang et al., 2021b). In the application, we can use the Conefor software (http://www.conefor.org/) (Saura and Torné, 2009), and the following metrics are commonly selected, including the area-weighted flux (AWF), the overall connectivity index (IIC) and the possible connectivity index (PC), etc. (Matos et al., 2019; Cui et al., 2020; Guo et al., 2020; Huang et al., 2021b). Among all kinds of connectivity indices, the Integral Index of Connectivity (IIC) and COHESION are the most popular and suitable indices for evaluating landscape connectivity (Saura and Pascual-Hortal, 2007; Zhang et al., 2019). The cohesion index measures the structural connectivity of a landscape (Zhang, 2017), and the IIC index is one of the best binary indices for functional connectivity analysis (Saura Santiago and lucía, 2008; Mahmoudzadeh et al., 2022).
Finally, the scientific combination of the two methods can effectively evaluate the structural and functional connectivity of habitats.
3.3 Habitat planning strategies
3.3.1 Habitat patches quality improvement
The existence of a habitat patch by itself does not fully guarantee higher species richness and abundance (Watling and Donnelly, 2006; Lee and Rhim, 2017). The environment within a habitat patch is critical to improving the habitat quality (Laidlaw, 2000; Prevedello and Vieira, 2010). Habitat quality indicates the capacity of an ecosystem to deliver the resources and conditions needed for wildlife and is a crucial determinant of biodiversity (Hall et al., 1997; Terrado et al., 2016; Aznarez et al., 2022). According to the physiological characteristics and habitat preferences of species, more favorable conditions should be created, and the stability of ecological habitat structure should be enhanced, mainly including the improvement of the quality of green space habitat, water and wetland habitat, and artificial facility habitat.
3.3.1.1 Green space habitat
Mixed woodland with trees, shrubs and grass has a complex plant community structure, which can provide a variety of habitats for animals. Plant flowers, fruits, and seeds of plants are also important food sources for animals. For example, birds like to jump between trees to find food, hedgehogs tend to move in bushes, and frogs need the abundant grass to shade. Therefore, green space habitat should avoid increasing the density of single plant species and planting structure, which would result in biotic homogenization. Such habitat cannot support the needs of various animals (McKinney, 2002; McKinney, 2006; Choudaj and Wankhade, 2022).
① Native plants selection
The current studies (Potgieter et al., 2017; Potgieter et al., 2019; Langmaier and Lapin, 2020; Choudaj and Wankhade, 2022) find that increase in the richness and diversity of native species increased the richness and diversity of animals. In the same case, a decline in the richness of animals as an increase in the density of exotic plants. Exotic species can replace native species and lead to loss of local biodiversity (Florianová and Münzbergová, 2017). In particular, they can destroy native habitats, limit biodiversity by reducing breeding, nesting and foraging space, and alter prey-predator relationships. This is because exotic plants do not satisfy the local habitat selection mechanism and do not have the structure adaptation. While native plants require little maintenance so that they can save money and water. They are less sensitive to local weather and rarely invasive so that they are a better food source for native animals (Mohammad et al., 2013). At the same time, tall native plants with thick trunks and dense foliage, are also good places for animals to hide from natural enemies and build nests. Therefore, native plants are the preferred plant types for restoring natural habitats.
② Multi-layer planting
Vegetation composition is a major factor in animal habitat selection in urban environments. Building a multi-layer plant community structure with an “arbor-shrub-herb” transition can provide animals with various ecological niches and positively impact animal habitats.
(1) Arbor forest layer
Maintain the forest core area. A large area of forest core area is important. Many previous studies have shown that populations persist longer in larger patches of suitable habitats than in smaller patches (Stacey and Taper, 1992; Capizzi et al., 2003; Jorge, 2008). Because these species require large areas of woodland to establish breeding grounds, build nests and raise young. In urban environments with high human influence, the minimum size of a circular forest is 3.1 ha and that of a square forest is 4.5 ha. The forest cores are not only essential for protecting habitat, but also play a vital role in dispersing seeds, maintaining populations and gene pools, and sustaining life cycles (Cho et al., 2021).
Keep the fallen and dead leaves. The rich vegetation cover of woodlands will shed a large amount of litter in autumn and winter, and a particular area of fallen and dead leaves should be reserved, with some small stones used to form caves or gaps. It can provide refuge for frogs, and the soil layer under the fallen leaves is relatively cool and humid, which is beneficial for frogs to dig and live and keep their bodies moist. The fallen and dead leaves is also an excellent habitat choice for arthropods and molluscs, and can be food of birds, small mammals and amphibians.
Preserve the dead and fallen trees. People often like to remove old trees, including dead branches. Because their poor health affects the aesthetics of the landscape, but these trees are important for nature conservation because the removal of old dead trees will exacerbate forest degradation and cause ecological imbalance, and they are also suitable habitats and shelters for animals (Oleksa and Gawronski, 2006; Oleksa et al., 2013; Horák, 2017). For example, the larvae of termites and certain beetles feed on broken branches and low tree branches, and they can become food for animals such as birds, hedgehogs, and squirrels (Fergus, 2019). Hollows of various sizes may form in dead or aged tree trunks and branches, which are valuable homes for wildlife. Many birds and mammals use burrows for nesting, rearing young, resting in cold or stormy weather to store food, hiding from predators, or hibernating.
For over-dense woodlands, the gaps are equally important. Because the gaps between the woodlands can not only provide a resting place for flying birds, but also allow sunlight shine directly on the ground, promoting the growth of low-level shrubs and grasses (Niu et al., 2021). The plant community has sufficient growth and natural succession space. Their wide leaves and dense branches can protect young animals from predation, as well as, flower buds and berries and attracted insects are also a good source of food for them.
(2) Shrub layer
The shrubs and grasslands mainly distribute on the edge of woodlands and open spaces, steep slopes of mountains, and demolished plots with poor soil (Niu et al., 2022), it is necessary to reduce intervention and create a natural community of multi-layered shrubs with different densities and heights.
Appropriately increasing the number of fruit-bearing tree species in early spring and winter (Zhou et al., 2018; Niu et al., 2022), and more functional plants such as jujube (Ziziphus jujuba), hairy cherry (Prunus tomentosa), Vitex (Vitex negundo), large flowered vetch (Vicia bungei), etc. are supposed to be planted. Their nuts, berries, and insects that spread pollen and nectar in the forest are important food sources for birds, small mammals and amphibians (Sulaiman et al., 2013; Bergner et al., 2015).
Planting low shrubs with clump-like plants and dense branches and leaves is an excellent place for animals to build nests and avoid natural enemies (Rhim et al., 2015). Scattered stones are piled up around the shrubs, and the surrounding soil environment is loosened, which can create the shaded and moist terrestrial environment for amphibians to hide and overwinter.
(3) grassland layer
The grassland is a significant habitat for insects. Thus it is also an important predation area for insectivorous birds, herbivorous seed birds, small mammals and amphibians (Murgui, 2009; Pithon et al., 2021). A variety of grass species should be selected. The common types are cool-season grasses and warm-season grasses. Warm-season grasses have stiffer stalks that are less likely to be overwhelmed by snow, providing shelter in winter and nesting space in spring. However, the warm-season grasses germinate late in early spring, but the cool-season grasses can be planted as food supplements and temporary shelters for small mammals and amphibians before the warm-season grasses starting to grow or after the dormancy period (Wang, 2021). In addition, wild flower seeds can also be sown to increase the richness of the grassland further.
The grassland habitat with variable micro-topography can produce different light, water and soil conditions locally, and promote the generation of differences in plant communities, which is conducive to enhancing the richness of animals. The large flat lawns should be avoided. On the contrary, micro-terrain design can be used to create belt-shaped valley spaces and shallow pits on the ground, where rainwater can gather to form various seasonal water bodies such as puddles and streams. Studies have shown that frogs and toads such as philodendron have a high hatching rate in seasonal water bodies at a depth of 3–4 cm (Lin et al., 2018).
(4) Aquatic and wet plants layer
For wet plants in the water, from the shore to the water, it is advisable to follow the plant mix of “terrestrial trees, shrubs and grasses - wet plants - emergent plants - floating plants - submerged plants.” Water-resistant reeds or multi-branched shrubs are planted in nearshore mud pools to provide breeding places for birds. The growth of emergent plants provides hiding space for amphibians and small mammals to breed and feed. The floating and submerged plants can become food for plankton and aquatic insects, eventually food sources for amphibians. The overall water area should maintain a relatively large aquatic vegetation coverage, providing a large hiding space and a sufficient area of resting spots for birds and amphibians, as well as (Babbitt and Tarr, 2002; Hartel et al., 2007) preventing amphibians from being exposed to excessive ultraviolet rays after landing (Wu et al., 2011). However, the vegetation cannot completely cover the surface of the pond, because the complete coverage of vegetation reduces the water temperature and dissolved oxygen concentration, leading to a reduction in the number of algae and epiphytes in the water, thereby reducing the food source and foraging success rate of amphibian larvae (Skelly et al., 2002; Maerz et al., 2005; Thurgate and Pechmann, 2007).
③ Plant protection
The plant protection for habitats can determine whether habitat patches quality has achieved the target, and intervene or modify the strategies based on long-term monitoring results to maintain the interests of the site. First, from the perspective of protecting animal food sources, nest sites, shelters and large-scale non-directional insect removal operations should be avoided in near-naturalized habitats, and tree holes should not be blocked. Excessive pruning should only be done on necessary roads (Yang et al., 2023). A low-disturbance method should be adopted to protect the self-growing ground cover plants, simulating the form and community structure of natural secondary forests, shrubs and grasslands (Xian et al., 2020; Zhou et al., 2018). Second, the healthy growth of plants is an important basis for ensuring the stability of animal habitats. The monitoring content of vegetation growth includes whether the branches are strong, whether the leaves are whole in color, whether there are dead and diseased leaves, etc (Yin et al., 2017). The plants with weak growth should be updated and replaced in time.
3.3.1.2 Water and wetland habitat
Water is the most important habitat type for animals to survive, as well as humans. All animals require the consumption of water to survive and reproduce. And they prefer to choose the area close to the water source as their habitat (Zhang et al., 2017). Therefore, the design of water bodies and their surrounding environment is particularly important.
① Shaping diverse water forms
Firstly, water bodies with irregular shorelines attract more wildlife. Creating diverse water forms such as rivers, lakes, islands, beaches, pits, and ponds, as well as different slopes and water depths of river banks, can create rich water environments and water landscapes to increase the diversity of water habitats (Lin et al., 2016). Secondly, the larger the water body area is, the more likely it is to support more plants and wildlife. However, when the water area exceeds a certain level, it is advisable to build islands, which are essential habitats and stops for wetland birds (Li et al., 2014; Yuan et al., 2021). Thirdly, the water body slope is also a significant habitat space. During the low water level period, a larger area of land will be exposed above the water surface, showing a mud-like shape and providing a substrate for the germination and growth of wetland plants. For most wetlands, a slope of 1:10 is suitable (Fergus, 2019; Wang, 2021). A gentle slope can make it easier for amphibians to enter and exit the wetland and make it easier for mammals to drink water (Huang et al., 2018). In order to increase diversity, different slopes can be designed in other wetland areas, but most slopes should be natural and gentle (Fergus, 2019).
② Creating a water buffer zone
The hard river banks should be naturalized, and the near-water area should be transformed into the grass and scrub habitats to restore the aquatic ecosystem by reducing erosion and stabilizing the revetment. First of all, grass, wildflowers, shrubs and trees are planted on both sides of the water body as a buffer zone. The width of the buffer zone can be set between 7 and 15 m or even wider, which can intercept runoff, control soil particles, chemicals, pollutants, etc. from entering the wetland, and protect the wetland from the sediment or pollutants of surface runoff (Fergus, 2019). Secondly, the buffer zone can also be used as a moving corridor and breeding area for wild animals, especially to create extremely favorable habitat conditions for wild waterfowl animals, and some mammals also like to move in the riparian zone (Fergus, 2019). Thirdly, a certain buffer area of exposed mudflats is needed. Because this type of shoreline can effectively meet the avoidance needs of small and medium-sized frogs during the day (Li, 2018; Lin et al., 2018). In winter, amphibians can also dig holes in the mud pool sediments for hibernation.
③ Retaining small water bodies
Small water bodies, such as ponds, make up a large proportion of the global freshwater surface (Downing et al., 2006). Given their ubiquity and variability in habitat types, ponds are considered “key systems for biodiversity conservation” (Oertli, 2018) and support greater diversity than similarly sized rivers and lakes. Because they can serve as shelters and corridor/stepping stone connectors (Le Viol et al., 2012; Maynou et al., 2017; Davies et al., 2008). Evidence from urban small water ponds suggests that they support a remarkable diversity as a miniature life network (Bishop et al., 2000; Le Viol et al., 2009; Hammer et al., 2012; Hassall and Anderson, 2015; Hill et al., 2017; Meland et al., 2020). Thus, retaining small water ponds can maintain or promote regional biodiversity by providing additional habitat. At the same time, you can put stones and sunken wood in the pond to attract amphibians to breed, and to attract birds to stop and rest.
④ Water protection
Animals usually use water bodies as the main space for feeding and drinking, and some birds and amphibians use aquatic plants and other materials for nesting on the water’s surface. Therefore, conservation methods such as salvaging aquatic plants and spraying pesticides in the park to keep the water clean should not be completely applied. Instead, pollution discharge should be controlled from the source, and the degree of manual management should be reduced. The self-purification ability of the water body should be restored to maintain the integrity of the aquatic ecosystem.
In addition, numerous studies indicate that regular management of ponds is critical to maintaining their biodiversity conservation function (Briers, 2014; Hassall and Anderson, 2015; Holtmann et al., 2018). Fish stocking, eutrophication, and woody overgrowth should be avoided in these ponds. The connectivity of the existing stormwater network can be improved by creating new ponds, which can be built from water bodies that can serve as stepping stones. General stormwater pond management strategies include maintaining ponds during early successional stages to facilitate the emergence of pioneer habitat specialists (Holtmann et al., 2018) through sludge prevention of terrestrialization and logging of woody plants along shorelines to provide sunny microhabitats. These practices also increase the macrophyte diversity and enhance the aerobic decomposition processes to counteract eutrophication (Sayer et al., 2012; Deacon and Samways, 2021). In addition, they conform to a reconciliation ecology since they help maintain the ponds’ technical function (Šigutová et al., 2022).
3.3.1.3 Artificial facility habitat
Another method to help mitigate habitat loss from urbanization is to use artificial structures to create new refuges and attraction facilities for animals. Humans can temporarily provide a range of different types of facilities, such as nest boxes, bat boxes, insect hotels, toad houses, etc. (Gaston et al., 2005; Davies et al., 2009), to introduce or translocate species, and monitor species abundance and distribution (Beyer and Goldingay, 2006; Williams et al., 2013; Goldingay et al., 2020). Obviously, any facility’s physical structure should meet the focal species’ requirements.
① Artificial nest boxes
Artificial nest boxes are built for animals, allowing animals to rest, reproduce or avoid natural enemies. For small mammals such as hedgehogs, they prefer to build nests in hedgerows and woodlands (Haigh et al., 2012; Bearman-Brown et al., 2020), because the nests built under the shelter of the ground can reduce the risk of detection by predators (Evans et al., 2002), and also protect them from adverse weather conditions, such as reducing rain, wind and direct sunlight (Jackson and Green, 2000). At the same time, hedgehogs are sensitive to changes in the microclimate inside the nest: high levels of humidity can cause the decay of nesting materials (Morris, 1973), the presence of parasites (Heeb et al., 2000) and impaired evaporative heat mechanisms in animals (McComb et al., 2022). During their hibernation, low temperatures in the nest would accelerate individual heat loss and lead to rapid depletion of fat stores (Soivio et al., 1968; Jansen, 2004; Gazzard and Baker, 2022). Therefore, when setting up artificial nest boxes, the leaves of broad-leaved trees are preferred because they can be “woven” to form a layered structure, which is believed to maintain the temperature inside the nest and gas transfer (Gazzard and Baker, 2022). Second, nests are placed in sheltered places, such as under shrub cover. The distance between nests varies between 2–323 m (Jensen, 2004; Yarnell et al., 2019). Third, bedding materials such as leaves, hay, or shredded newspaper inside the nest box had a significant positive effect on resting but a (non-significant) negative effect on reproduction. This is because females with dependent young are susceptible during breeding. Pregnant females may interpret the presence of bedding if their nest is disturbed as an indication of other hedgehogs, and the mother may abandon the breeding nest or kill the young (Gazzard and Baker, 2022).
For the design of artificial nest boxes for birds, the shape and size need to be determined in conjunction with the size of the target bird. The materials generally use wood, clay, etc., which are functional and durable. The served birds are secondary-cavity nester birds that use existing caves as nest sites. The nest box style and layout area should be determined according to the ecological habits of the species. For example, the nest boxes of Beijing swifts and barn swallows are arranged on building facades, and the nest boxes of mandarin ducks and woodpeckers are hung on tall trees.
② Dead hedges
Dead hedges are piled up with stones, branches, etc. to form a small porous ecological environment. The interior is filled with soil with seeds, and thorny vines are planted to protect the plants in the pile from herbivores. Dead hedges are equivalent to a kind of “artificial bushes,” which can be set up in open areas lacking hidden spots and understory spaces lacking bushes, providing shelter for birds and small animals. The common size is 6 m*6 m wide and 1.2–2.4 m high. It consists of a three-layer structure: the substrate layer, the middle layer and the top layer. The substrate layer is made of wood, wood stones and plants arranged in a grid, or the stones are arranged in a triangular shape. The middle layer is made of small pieces of branches and sticks stacked repeatedly, and the top layer is covered with thorny vines or branches. Dead hedges should be arranged at a distance of 10–45 m so that nearby animals can quickly reach and use them (Wang, 2021).
③ Rock piles
Rocks add important cracks, cavities, and other structures to habitats that provide cover for animals. Rock squirrels are especially fond of rock structures. They are good at climbing and jumping on undulating rocks. In natural environments, rock squirrels also eat, rest or groom on rocks. Weasels and hedgehogs also roost and breed in caves or rock piles. Placing rock mounds in scrubland, fences, or wetland revetments can create a very important structural element for habitats, which can also be supplemented by planting some food-producing vines or shrubs between the rocks (Fergus, 2019). Some amphibians like to hide in aquatic cave environments. Stone slabs can be built in places with sufficient water sources to create dark and hidden spaces, such as caves and rock crevices, which are convenient for amphibians to survive and reproduce.
3.3.2 Ecological corridor construction
3.3.2.1 Ecological corridor location
Ecological corridors (ECs) have been identified as one conservation tool to provide linkages or restore connections between isolated ecological spaces in human-modified landscapes (Matthews, 2008) to solve ecological fragmentation problems and maintain biodiversity. Methods for constructing ecological corridors include minimum cumulative resistance (MCR) models (Pirnat, 2000; Dong et al., 2020; Xiao et al., 2020), least cost paths (LCP) (Adriansen et al., 2003; Zhang et al., 2019; Morandi et al., 2020), circuit theory (McRae et al., 2008; Song and Qin, 2016) or landscape indexes (Xiao et al., 2020). A small number of studies have developed new tools, such as Moreno et al. (2020), by applying GIS, GPS and the Normalized Difference Vegetation Index (NDVI) derived from Santinel-2 to determine vegetation cover, chlorophyll activity and plant vigor to identify the potential of green corridor. Many studies (Rudd et al., 2002; Kong et al., 2010; Yang et al., 2017; Zhang et al., 2019; Zhao et al., 2019) applied the Gravity Models to simulate the interactions between nodes connected by corridors, whereby the priority of these corridors could be determined.
3.3.2.2 Ecological corridor width
The ecological corridor is not a line but a strip space containing the ecological land along the central line. Although there are several methods to determine the location of ecological corridors, in practice, the width of ecological corridors still needs to be improved. Their boundaries are often subjectively identified or estimated (Avon and Bergès, 2016; Liang et al., 2018; Dong et al., 2020). According to the research of Shen and Wang (2022), five indicators are selected from the following three dimensions as the key factors that significantly affect the width of ecological corridor construction (Table 4). 1) The inherent characteristics of ecological corridors, including path length and the capacity of connecting important ecological spaces; 2) The internal conditions of the ecological corridor, including the number of ecological spaces and the ecological functions on the path; 3) The external environmental factors of the ecological corridor, including the land use types along the path.
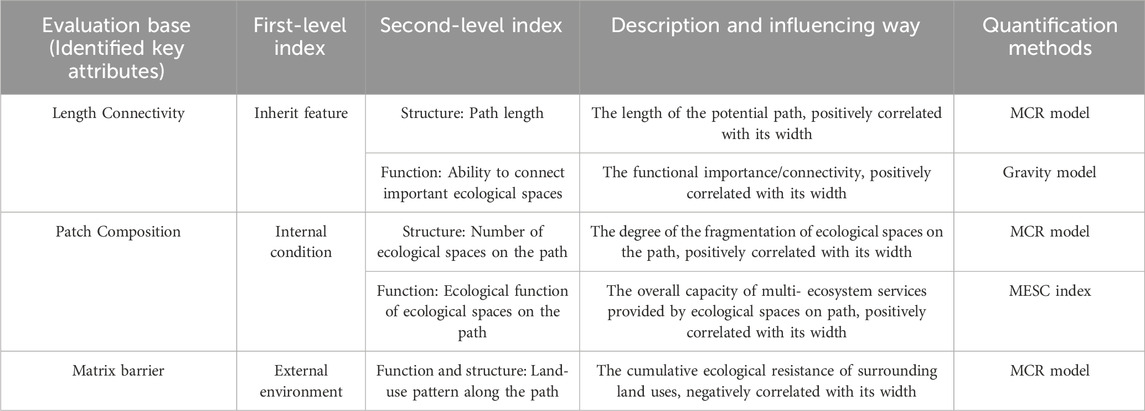
TABLE 4. Impact index system of the width of urban ecological corridors (Shen and Wang, 2022).
In addition to being affected by the above indicators, the appropriate width of the ecological corridor should be limited to a certain range. According to the landscape ecology, ecological corridors with widths>12 m and with tree, shrub, and grass structures are considered to have ecological significance. These qualities are also necessary for species living within them (Shen and Wang, 2022). Therefore, 12 m is the minimum effective width of the ecological corridor. Generally speaking, the wider the ecological corridor is, the better the internal habitat quality is, and the greater the overall ecological benefits. However, the corridor cannot be as wide as possible, due to the consideration of land use, cost economy, and species spanning range. According to the studies on ecological corridor planning and design (Zhu et al., 2005; Teng et al., 2010; Zhang et al., 2017; Xiao et al., 2020), we found that 1,000 m is an appropriate width for ecological corridor planning within the urban-rural gradient in China. Therefore, in this paper, the width of the ecological corridor can be between 12 m and 1,000 m.
3.3.2.3 Stepping stone network
Stepping stones are small habitat patches that have crucial ecological linkage functions. Although these landscape patches are small and have weak ecological functions, they can serve as transfer stations for biological migration and become an important part of the ecological corridor network (Baum et al., 2004). According to the range of potential ecological corridors, we can use ArcGIS’s “overlap” tool to screen the stepping stones in buffer zones of habitat sources and potential corridors. Then, the stepping-stone network can be built with the Build Network and Map Linkages tools in Linkage Mapper (Zhang and Song, 2020). The network simulates biological communication between important habitats and stepping stones. Finally, the stepping stone network is embedded in the ecological corridor network to form a multi-level network structure. This network structure delineates the connections between landscape patches in the area (Wang et al., 2022).
3.4 Animal habitat management
Nowadays, most habitat designs are difficult to play a substantive role or exert ecological benefits in the long run. An important reason is the lack of habitat management, which requires the joint efforts of the whole society. Habitat management can monitor and give feedback on the rationality of habitat construction, help designers or managers improve the habitat environment, and provide more experience for future biological protection work. In the study, it is divided into two parts.
3.4.1 Interference management
The urban environment is the home where humans and animals live together. But human activities may cause disturbances in many species’ predation, foraging, and mating activities (Bateman and Fleming, 2014; Mikula et al., 2018; Gallo et al., 2019). Previous studies have found that many animals tend to avoid human interaction and prefer habitats with lower human population densities (George and Crooks, 2006; Gehrt et al., 2009). Therefore, we need to control the type and intensity of people’s activities.
3.4.1.1 Zoning of source protection
In general, the “one-size-fits-all” approach of the functional positioning leads to little practical significance. Depending on the species and environmental factors in the area, the functional orientation of the habitat should also vary. The precedence principle (Minor and Urban, 2008; Liang et al., 2018) is used to identify priority areas for animal habitat conservation, as described below. With large amounts of human infiltration into natural green areas, protection in the form of protected areas alone cannot be fully effective (Nori et al., 2015). The presence of sufficiently wide buffer zones and the concentration of pedestrian traffic is proven to be more effective in protecting urban biodiversity.
In order to balance the needs of animal habitats and human activities, it is necessary to delineate areas with different development intensities. According to the evaluation results of habitat suitability, 1) Set the area with concentrated distribution of animals and high suitability in the habitat network as the core habitat areas. The core habitat areas provide suitable habitat conditions for the survival and reproduction of animals. At the same time, it is also the core area of animal protection and strictly restricts construction and interference from human activities; 2) Set up areas with low animal distribution density, low habitat suitability and non-moving paths as ecological recreation areas. Based on the low intensity of recreational activities and damage to the ecological background, various recreational services should be created as much as possible to balance ecological habitat protection and recreational functions. 3) The ecological buffer zones between the core habitat areas and the ecological recreation areas buffer the interference of human activities in the core habitat area. It is the primary place for urban residents to observe, experience nature, and carry out popular science education.
3.4.1.2 Classification of ecological corridor
① Determine the backbone of the corridor
The corridors most frequently used by animals were calculated using the kernel density model (Teng et al., 2011) and identified as backbone corridors, constituting the ecological corridor network. The kernel density distribution is a spatial analysis method. It calculates the distribution density of event points in the adjacent space, which can directly reflect the aggregation or discrete distribution characteristics of point groups (Yang et al., 2022). The kernel density analysis provides information about the density of each corridor, with larger values indicating the most likely path animals would have traveled, and it is used to estimate the distribution and habitat range of animals (Worton, 1989; Feberg, 2007; Li et al., 2010; Teng et al., 2011).
② Corridors classification
Corridors are classified according to objectives of city planning and the balance of ecological protection and economic development. This is necessary to adapt ecological corridors to limited land and financial resources. Referring to the definition of corridor classification and the trend of diffusion of animals between corridor nodes (Yang et al., 2022), corridors are divided into three levels to correspond to three ecological security levels: primary corridors, secondary corridors, and tertiary corridors. 1) The primary corridor has the largest core density and the widest width, connecting many important large-scale ecological spaces, most of which are located in areas with fewer human activities and disturbances. It also assumes diverse functions for ecosystem services, such as shaping the landscape, connecting the source of habitat and adjusting the microclimate. 2) The secondary corridor has the second highest core density and broader width, which connects stepping stones. 3) The tertiary corridor has the most negligible core density and the narrowest width. It connects more ecological spaces in the green space on the edge of the city and can be built as traffic green belts or scenic trails. The ecological culverts, ecological overpasses and other structures can be used to guide the safe migration of species on both sides of the road to prevent interruption of landscape flow and ecosystem service flow. It can also be combined with leisure greenways or short-distance leisure places for urban residents.
3.4.2 Citizen science management
Citizen science is led by scientists in academic, government, nonprofit, or commercial organizations and is conducted jointly by professional scientists and paid technicians or students (Nichols and Williams, 2006; Sullivan et al., 2009; McCormick, 2012; Hemmi and Graham, 2014; McKinley et al., 2017). Volunteers help to generate scientific information for conservation scientists, natural resource and environmental managers, and other decision-makers. Or stimulate public input and participation in natural resource and environmental management and policy-making. Citizen science has emerged as a powerful tool for addressing many of the challenges environmentalists face (McKinley et al., 2017; Table 5). For example, citizen science is being used to understand the effectiveness of urban wildlife habitats (Mowry et al., 2021) by collecting big data from citizen scientists. These data can be used to implement adaptive management in response to uncertainty caused by environmental changes, improved sampling methods, and species adaptation (Richardson et al., 2020). We advocate adopting an adaptive management strategy, establishing a long-term data network, and revising management plans annually based on modeling results. With the latest species and environmental information, policymakers can adjust their management strategies, remain flexible, and respond to changes in the urban environment and the species they monitor.

TABLE 5. Projects types and contents of citizen science management (McKinley et al., 2017).
4 Conclusion
Constructing suitable habitat environmental conditions is the most direct and effective way to protect animals. In this paper, we synthesized research from a variety of environmental fields and consolidated the findings to act as a reference for future planning, management, and policymaking in city. This paper has three implications for the construction and research of urban animal habitats. 1) In terms of resources investigation, various methods are used to obtain data, such as data collection, interview, expert consultation, field survey and technical survey. The accuracy and richness of multi-source data can provide reliable basic support. 2) In terms of evaluation and planning, the hotspots of animal distribution and the suitable patches evaluated by the model can be combined to delineate the specific spatial range of source areas and ecological corridors. The habitat characteristics of animals’ preferences are an important basis for habitat construction. And on the basis of satisfying the green coverage and the plants ecological function, highlighting the dominant position of animals in green space is the critical way to ensure the success of habitat construction. 3) Finally, in terms of habitat management, by encouraging positive human intervention and avoiding negative behaviors, the balance between humans and animals can be realized to achieve the maximum function and sustainability.
Overall, our review has highlighted the 4 key steps of animal habitat constructions throughout recent literature to create a blueprint for future development and management. However, to incorporate these benefits into urban green space, further researches are needed to construction methods. For example, regions can use their own multi-source data to draw habitat maps, explore and evaluate the suitable habitat region by combining geographic information systems and biodiversity research, which can provide scientific basis for biodiversity protection and urban ecological planning. Besides the important habitat sources under centralized protection, we also need to pay attention to small and micro green spaces, brownfields and green roofs, etc., and actively call on community residents to share the responsibility for daily management and maintenance. In addition, the current literature focuses on the scientific research of a single species or a single animal group. And each species is part of the ecosystem and there are fluidity and integrity in the ecological cycle. Future research studies need to consider the ecological relationship between species and explore the construction models and methods of urban composite habitats from the perspective of integrating multiple species.
Author contributions
ZL and HY conceived the idea. QC and ZW collected the data. ZL and YW wrote the manuscript with input from HY. All authors contributed to the article and approved the submitted version.
Funding
The study was funded by Special Fund for Beijing Common Construction Project “Beijing urban and rural energy-saving green space construction technology and efficient breeding of functional plant materials (2019GJ-03)” at the Beijing Laboratory of Urban and Rural Ecological Environment, the hot tracking project “research on the application level and promotion strategy of plant landscape resources in urban human settlement environment (2022BLRD05)” at the Beijing Forestry University.
Conflict of interest
The authors declare that the research was conducted in the absence of any commercial or financial relationships that could be construed as a potential conflict of interest.
Publisher’s note
All claims expressed in this article are solely those of the authors and do not necessarily represent those of their affiliated organizations, or those of the publisher, the editors and the reviewers. Any product that may be evaluated in this article, or claim that may be made by its manufacturer, is not guaranteed or endorsed by the publisher.
References
Aarts, G., MacKenzie, M., McConnell, B., Fedak, M., and Matthiopoulos, J. (2008). Estimating space-use and habitat preference from wildlife telemetry data. Ecography 31, 140–160. doi:10.1111/j.2007.0906-7590.05236.x
Albert, C. H., Rayfield, B., Dumitru, M., and Gonzalez, A. (2017). Applying network theory to prioritize multispecies habitat networks that are robust to climate and land-use change. Conserv. Biol. 31, 1383–1396. doi:10.1111/cobi.12943
Aronson, M. F., Nilon, C. H., Lepczyk, C. A., Parker, T. S., Warren, P. S., Cilliers, S. S., et al. (2016). Hierarchical filters determine community assembly of urban species pools. Ecology 97, 2952–2963. doi:10.1002/ecy.1535
Arques, J., Belda, A., Peiró, V., Seva, E., and Martínez-Pérez, J. E. (2014). Main ecological gradients and landscape matrix affect wild rabbit Oryctolagus cuniculus (Linnaeus, 1758) (Mammalia: leporidae) abundance in a coastal region (South-East Spain). Ital. J. Zool. 81, 440–450. doi:10.1080/11250003.2014.9226
Avon, C., and Bergès, L. (2016). Prioritization of habitat patches for landscape connectivity conservation differs between least-cost and resistance distances. Landsc. Ecol. 31, 1551–1565. doi:10.1007/s10980-015-0336-8
Aznarez, C., Svenning, J. C., Taveira, G., Baró, F., and Pascual, U. (2022). Wildness and habitat quality drive spatial patterns of urban biodiversity. Landsc. Urban Plan. 228, 104570. doi:10.1016/j.landurbplan.2022.104570
Babbitt, K., and Tarr, T. (2002). Effects of habitat complexity and predator identity on predation of Rana clamitans larvae. Amphibia-Reptilia 23, 13–20. doi:10.1163/156853802320877591
Bateman, P. W., and Fleming, P. A. (2014). Does human pedestrian behaviour influence risk assessment in a successful mammal urban adapter? J. Zoology 294, 93–98. doi:10.1111/jzo.12156
Baum, K. A., Haynes, K. J., Dillemuth, F. P., and Cronin, J. T. (2004). The matrix enhances the effectiveness of corridors and stepping stones. Ecology 85, 2671–2676. doi:10.1890/04-0500
Bearman-Brown, L. E., Baker, P. J., Scott, D., Uzal, A., Evans, L., and Yarnell, R. W. (2020). Over-winter survival and nest site selection of the west-European hedgehog (Erinaceus europaeus) in arable dominated landscapes. Animals 10, 1449. doi:10.3390/ani10091449
Bergner, A., Avcı, M., Eryiğit, H., Jansson, N., Niklasson, M., Westerberg, L., et al. (2015). Influences of forest type and habitat structure on bird assemblages of oak (Quercus spp.) and pine (Pinus spp.) stands in southwestern Turkey. For. Ecol. Manag. 336, 137–147. doi:10.1016/j.foreco.2014.10.025
Bernat-Ponce, E., Gil-Delgado, J. A., and López-Iborra, G. M. (2021). Recreational noise pollution of traditional festivals reduces the juvenile productivity of an avian urban bioindicator. Environ. Pollut. 286, 117247. doi:10.1016/j.envpol.2021.117247
Beyer, G. L., and Goldingay, R. L. (2006). The value of nest boxes in the research and management of Australian hollow-using arboreal marsupials. Wildl. Res. 33, 161–174. doi:10.1071/WR04109
Bishop, C. A., Struger, J., Shirose, L. J., Dunn, L., and Campbell, G. D. (2000). Contamination and wildlife communities in stormwater detention ponds in Guelph and the greater Toronto area, Ontario, 1997 and 1998 Part II—contamination and biological effects of contamination. Water Qual. Res. J. 35, 437–474. doi:10.2166/wqrj.2000.027
Bradfield, A. A., Nagy, C. M., Weckel, M., Lahti, D. C., and Habig, B. (2022). Predictors of mammalian diversity in the New York metropolitan area. Front. Ecol. Evol. 10, 903211. doi:10.3389/fevo.2022.903211
Briers, R. A. (2014). Invertebrate communities and environmental conditions in a series of urban drainage ponds in Eastern Scotland: implications for biodiversity and conservation value of SUDS. Clean–Soil, Air, Water 42, 193–200. doi:10.1002/clen.201300162
Callaghan, C. T., Major, R. E., Lyons, M. B., Martin, J. M., and Kingsford, R. T. (2018). The effects of local and landscape habitat attributes on bird diversity in urban greenspaces. Ecosphere 9, e02347. doi:10.1002/ecs2.2347
Capizzi, D., Battistini, M., and Amori, G. (2003). Effects of habitat fragmentation and forest management on the distribution of the edible dormouseGlis glis. Acta Theriol. 48, 359–371. doi:10.1007/BF03194175
Cayuela, H., Valenzuela-Sánchez, A., Teulier, L., Martínez-Solano, Í., Léna, J. P., Merilä, J., et al. (2020). Determinants and consequences of dispersal in vertebrates with complex life cycles: a review of pond-breeding amphibians. Q. Rev. Biol. 95, 1–36. doi:10.1086/707862
Chen, X. M., Lei, Y. C., Zhang, X. Q., and Jia, H. Y. (2012). Effects of sample sizes on accuracy and stability of maximum entropy model in predicting species distribution. Sci. Silvae Sin. 48, 53–59.
Cheptou, P. O., Hargreaves, A. L., Bonte, D., and Jacquemyn, H. (2017). Adaptation to fragmentation: evolutionary dynamics driven by human influences. Philosophical Trans. R. Soc. B Biol. Sci. 372, 20160037. doi:10.1098/rstb.2016.0037
Cho, H. J., Lee, E. J., and Rhim, S. J. (2021). Effect of patch size and habitat variables on small rodents population in urban woods. Pak. J. Zool. 54, 1557–1562. doi:10.17582/journal.pjz/20200915030932
Choudaj, K., and Wankhade, V. (2022). Study of the interrelationship between woody plants and birds in Pune urban area, insights on negative impacts of exotic plants. Trop. Ecol. 10, 1–12. doi:10.1007/s42965-022-00269-3
Churko, G., Walter, T., Szerencsits, E., and Gramlich, A. (2020). Improving wetland connectivity through the promotion of wet arable land. Wetl. Ecol. Manag. 28, 667–680. doi:10.1007/s11273-020-09739-8
Clauzel, C., Jeliazkov, A., and Mimet, A. (2018). Coupling a landscape-based approach and graph theory to maximize multispecific connectivity in bird communities. Landsc. Urban Plan. 179, 1–16. doi:10.1016/j.landurbplan.2018.07.002
Conrad, J. M., Gomes, C. P., van Hoeve, W. J., and Suter, J. F. (2012). Wildlife corridors as a connected subgraph problem. J. Environ. Econ. Manag. 63, 1–18. doi:10.1016/j.jeem.2011.08.001
Cook, E. A. (2002). Landscape structure indices for assessing urban ecological networks. Landsc. urban Plan. 58, 269–280. doi:10.1016/S0169-2046(01)00226-2
Cui, L., Wang, J., Sun, L., and Lv, C. (2020). Construction and optimization of green space ecological networks in urban fringe areas: a case study with the urban fringe area of Tongzhou district in Beijing. J. Clean. Prod. 276, 124266. doi:10.1016/j.jclepro.2020.124266
Curzel, F. E., Bellocq, M. I., and Leveau, L. M. (2021). Local and landscape features of wooded streets influenced bird taxonomic and functional diversity. Urban For. Urban Green. 66, 127369. doi:10.1016/j.ufug.2021.127369
Davies, B., Biggs, J., Williams, P., Whitfield, M., Nicolet, P., Sear, D., et al. (2008). Comparative biodiversity of aquatic habitats in the European agricultural landscape. Agric. Ecosyst. Environ. 125, 1–8. doi:10.1016/j.agee.2007.10.006
Davies, Z. G., Fuller, R. A., Loram, A., Irvine, K. N., Sims, V., and Gaston, K. J. (2009). A national scale inventory of resource provision for biodiversity within domestic gardens. Biol. Conserv. 142, 761–771. doi:10.1016/j.biocon.2008.12.016
Deacon, C., and Samways, M. J. (2021). A review of the impacts and opportunities for African urban dragonflies. Insects 12, 190. doi:10.3390/insects12030190
Deng, Y. R., Xiao, R. B., Huang, L. J., Hu, H. J., Li, Z. S., and Ding, X. L. (2015). Approaches and case study of urban biodiversity restoration. Landsc. Archit. 11, 25–32. doi:10.14085/j.fjyl.2015.06.0025.08
Dondina, O., Orioli, V., Chiatante, G., and Bani, L. (2020). Practical insights to select focal species and design priority areas for conservation. Ecol. Indic. 108, 105767. doi:10.1016/j.ecolind.2019.105767
Dong, J., Peng, J., Liu, Y., Qiu, S., and Han, Y. (2020). Integrating spatial continuous wavelet transform and kernel density estimation to identify ecological corridors in megacities. Landsc. Urban Plan. 199, 103815. doi:10.1016/j.landurbplan.2020.103815
Dong, Z., Wang, Z., Liu, D., Li, L., Ren, C., Tang, X., et al. (2013). Assessment of habitat suitability for waterbirds in the West Songnen Plain, China, using remote sensing and GIS. Ecol. Eng. 55, 94–100. doi:10.1016/j.ecoleng.2013.02.006
Downing, J. A., Prairie, Y. T., Cole, J. J., Duarte, C. M., Tranvik, L. J., Striegl, R. G., et al. (2006). The global abundance and size distribution of lakes, ponds, and impoundments. Limnol. Oceanogr. 51, 2388–2397. doi:10.4319/lo.2006.51.5.2388
Driscoll, D. A., Banks, S. C., Barton, P. S., Lindenmayer, D. B., and Smith, A. L. (2013). Conceptual domain of the matrix in fragmented landscapes. Trends Ecol. Evol. 28, 605–613. doi:10.1016/j.tree.2013.06.010
Duro, D. C., Coops, N. C., Wulder, M. A., and Han, T. (2007). Development of a large area biodiversity monitoring system driven by remote sensing. Prog. Phys. Geogr. 31, 235–260. doi:10.1177/0309133307079054
Elith, J., Graham, H., Anderson, C. P. R., Dudík, M., Ferrier, S., Guisan, A., et al. (2006). Novel methods improve prediction of species’ distributions from occurrence data. Ecography 29, 129–151. doi:10.1111/j.2006.0906-7590.04596.x
Evans, M. R., Lank, D. B., Boyd, W. S., and Cooke, F. (2002). A comparison of the characteristics and fate of Barrow's Goldeneye and Bufflehead nests in nest boxes and natural cavities. Condor 104, 610–619. doi:10.1093/condor/104.3.610
Fahrig, L. (2003). Effects of habitat fragmentation on biodiversity. Annu. Rev. Ecol. Evol. Syst. 34, 487–515. doi:10.1146/annurev.ecolsys.34.011802.132419
Fahrig, L. (2003). Effects of habitat fragmentation on biodiversity. Annu. Rev. Ecol. Evol. Syst. 34, 487–515. doi:10.1146/annurev.ecolsys.34.011
Fahrig, L. (2019). Habitat fragmentation: a long and tangled tale. Glob. Ecol. Biogeogr. 28, 33–41. doi:10.1111/geb.12839
Farinha-Marques, P., Fernandes, C., Guilherme, F., Lameiras, J. M., Alves, P., and Bunce, R. G. (2017). Urban Habitats Biodiversity Assessment (UrHBA): a standardized procedure for recording biodiversity and its spatial distribution in urban environments. Landsc. Ecol. 32, 1753–1770. doi:10.1007/s10980-017-0554-3
Fergus, C. (2019). Make a home for wildlife: creating habitat on your land backyard to many acres. United States: Stackpole Books.
Fernández, I. C., and Simonetti, J. A. (2013). Small mammal assemblages in fragmented shrublands of urban areas of Central Chile. Urban Ecosyst. 16, 377–387. doi:10.1007/s11252-012-0272-1
Fidino, M., Gallo, T., Lehrer, E. W., Murray, M. H., Kay, C., Sander, H. A., et al. (2020). Landscape-scale differences among cities alter common species’ responses to urbanization. Ecol. Appl. 31, 2. doi:10.1002/eap.2253
Fieberg, J. (2007). Kernel density estimators of home range: smoothing and the autocorrelation red herring. Ecology 88, 1059–1066. doi:10.1890/06-0930
Fischer, J., and Lindenmayer, D. B. (2007). Landscape modification and habitat fragmentation: a synthesis. Glob. Ecol. Biogeogr. 16, 265–280. doi:10.1111/j.1466-8238.2006.00287.x
Fleury, M., and Galetti, M. (2006). Forest fragment size and microhabitat effects on palm seed predation. Biol. Conserv. 131, 1–13. doi:10.1016/j.biocon.2005.10.049
Florianová, A., and Münzbergová, Z. (2017). Invasive Impatiens parviflora has negative impact on native vegetation in oak-hornbeam forests. Flora 226, 10–16. doi:10.1016/j.flora.2016.11.006
Gallo, T., Fidino, M., Lehrer, E. W., and Magle, S. (2019). Urbanization alters predator-avoidance behaviours. J. Animal Ecol. 88, 793–803. doi:10.1111/1365-2656.12967
Gallo, T., Fidino, M., Lehrer, E. W., and Magle, S. B. (2017). Mammal diversity and metacommunity dynamics in urban green spaces: implications for urban wildlife conservation. Ecol. Appl. 27, 2330–2341. doi:10.1002/eap.1611
Galpern, P., Manseau, M., and Fall, A. (2011). Patch-based graphs of landscape connectivity: a guide to construction, analysis and application for conservation. Biol. Conserv. 144, 44–55. doi:10.1016/j.biocon.2010.09.002
Gan, L. (2018). Built environment factors affecting urban biodiversity and planning optimization approaches. Urban Plan. Int. 33, 67–73.
Gaston, K. J., Smith, R. M., Thompson, K., and Warren, P. H. (2005). Urban domestic gardens (II): experimental tests of methods for increasing biodiversity. Biodivers. Conservation 14, 395–413.
Gazzard, A., and Baker, P. J. (2022). What makes a house a home? Nest box use by West European hedgehogs (Erinaceus europaeus) is influenced by nest box placement, resource provisioning and site-based factors. PeerJ 10, e13662. doi:10.7717/peerj.13662
Gehrt, S. D., Anchor, C., and White, L. A. (2009). Home range and landscape use of coyotes in a metropolitan landscape: conflict or coexistence? J. Mammal. 90, 1045–1057. doi:10.1644/08-MAMM-A-277.1
George, S. L., and Crooks, K. R. (2006). Recreation and large mammal activity in an urban nature reserve. Biol. Conserv. 133, 107–117. doi:10.1016/j.biocon.2006.05.024
Gogol-Prokurat, M. (2011). Predicting habitat suitability for rare plants at local spatial scales using a species distribution model. Ecol. Appl. 21, 33–47. doi:10.1890/09-1190.1
Goldingay, R. L., Rohweder, D., and Taylor, B. D. (2020). Nest box contentions: are nest boxes used by the species they target? Ecol. Manag. Restor. 21, 115–122. doi:10.1111/emr.12408
Guisan, A., and Zimmermann, N. E. (2000). Predictive habitat distribution models in ecology. Ecol. Model. 135, 147–186. doi:10.1016/S0304-3800(00)00354-9
Guo, X., Zhang, X., Du, S., Li, C., Siu, Y. L., Rong, Y., et al. (2020). The impact of onshore wind power projects on ecological corridors and landscape connectivity in Shanxi, China. J. Clean. Prod. 254, 120075. doi:10.1016/j.jclepro.2020.120075
Haddad, N. M., Brudvig, L. A., Clobert, J., Davies, K. F., Gonzalez, A., Holt, R. D., et al. (2015). Habitat fragmentation and its lasting impact on Earth’ s ecosystems. Sci. Adv. 1, e1500052. doi:10.1126/sciadv.1500052
Haigh, A., O’Riordan, R. M., and Butler, F. (2012). Nesting behaviour and seasonal body mass changes in a rural Irish population of the Western hedgehog (Erinaceus europaeus). Acta Theriol. 57, 321–331. doi:10.1007/s13364-012-0080-2
Hall, L. S., Krausman, P. R., and Morrison, M. L. (1997). The habitat concept and a plea for standard terminology. Wildl. Soc. Bull., 173–182.
Hamer, A. J., Smith, P. J., and McDonnell, M. J. (2012). The importance of habitat design and aquatic connectivity in amphibian use of urban stormwater retention ponds. Urban Ecosyst. 15, 451–471. doi:10.1007/s11252-011-0212-5
Hartel, T., Nemes, S., Cogălniceanu, D., Öllerer, K., Schweiger, O., Moga, C. I., et al. (2007). The effect of fish and aquatic habitat complexity on amphibians. Hydrobiologia 583, 173–182. doi:10.1007/s10750-006-0490-8
Hassall, C., and Anderson, S. (2015). Stormwater ponds can contain comparable biodiversity to unmanaged wetlands in urban areas. Hydrobiologia 745, 137–149. doi:10.1007/s10750-014-2100-5
Hedblom, M., and Murgui, E. (2017). Urban bird research in a global perspective. Switzerland: Springer Cham, 3–10. doi:10.1007/978-3-319-43314-1_1
Heeb, P., Kölliker, M., and Richner, H. (2000). Bird–ectoparasite interactions, nest humidity, and ectoparasite community structure. Ecology 81, 958–968. doi:10.1890/0012-9658(2000)081[0958:BEINHA]2.0.CO;2
Hemmi, A., and Graham, I. (2014). Hacker science versus closed science: building environmental monitoring infrastructure. Inf. Commun. Soc. 17, 830–842. doi:10.1080/1369118X.2013.848918
Herrera-Duenas, A., Pineda, J., Antonio, M. T., and Aguirre, J. I. (2014). Oxidative stress of house sparrow as bioindicator of urban pollution. Ecol. Indic. 42, 6–9. doi:10.1016/j.ecolind.2013.08.014
Hess, G. R., and King, T. J. (2002). Planning open spaces for wildlife: I. Selecting focal species using a Delphi survey approach. Landsc. urban Plan. 58, 25–40. doi:10.1016/S0169-2046(01)00230-4
Hill, M. J., Biggs, J., Thornhill, I., Briers, R. A., Gledhill, D. G., White, J. C., et al. (2017). Urban ponds as an aquatic biodiversity resource in modified landscapes. Glob. change Biol. 23, 986–999. doi:10.1111/gcb.13401
Hilty, J., Worboys, G. L., Keeley, A., and Tabor, G. M. (2020). Guidelines for conserving connectivity through ecological networks and corridors. Best. Pract. Prot. area Guidel. Ser. 30, 122. doi:10.2305/IUCN.CH.2020.PAG.30.en
Holtmann, L., Juchem, M., Brüggeshemke, J., Möhlmeyer, A., and Fartmann, T. (2018). Stormwater ponds promote dragonfly (Odonata) species richness and density in urban areas. Ecol. Eng. 118, 1–11. doi:10.1016/j.ecoleng.2017.12.028
Hong, Y. Z., Meng, S. L., and Chen, C. J. (2023). Research progress of fish habitat protection technologies. J. Agric. 13, 82–87. doi:10.11923/j.issn.2095-4050.cjas2021-0221
Horák, J. (2017). Insect ecology and veteran trees. J. Insect Conservation 21, 1–5. doi:10.1007/s10841-017-9953-7
Huang, B. X., Chiou, S. C., and Li, W. Y. (2021). Landscape pattern and ecological network structure in urban green space planning: a case study of Fuzhou city. Land 10, 769. doi:10.3390/land10080769
Huang, K., Mu, Z. Q., Yue, Q., Yu, F., Yuan, X., Bo, S. Q., et al. (2018). Microhabitat utilization of Anuran species in Shanghai country parks. Sichuan J. Zoology 37, 426–434. doi:10.11984/j.issn.1000-7083.20170148
Huang, L., Wang, J., and Cheng, H. (2022). Spatiotemporal changes in ecological network resilience in the Shandong Peninsula urban agglomeration. J. Clean. Prod. 339, 130681. doi:10.1016/j.jclepro.2022.130681
Huang, X., Wang, H., Shan, L., and Xiao, F. (2021). Constructing and optimizing urban ecological network in the context of rapid urbanization for improving landscape connectivity. Ecol. Indic. 132, 108319. doi:10.1016/j.ecolind.2021.108319
IUCN (2023). Habitats classification scheme. Available at: https://www.iucnredlist.org/resources/habitat-classification-scheme (Accessed May 25, 2023).
Jackson, D. B., and Green, R. E. (2000). The importance of the introduced hedgehog (Erinaceus europaeus) as a predator of the eggs of waders (Charadrii) on machair in South Uist, Scotland. Biol. Conserv. 93, 333–348. doi:10.1016/S0006-3207(99)00135-4
Jensen, A. B. (2004). Overwintering of European hedgehogsErinaceus europaeus in a Danish rural area. Acta theriol. 49, 145–155.
Jorge, M. L. S. (2008). Effects of forest fragmentation on two sister genera of Amazonian rodents (Myoprocta acouchy and Dasyprocta leporina). Biol. Conserv. 141, 617–623. doi:10.1016/j.biocon.2007.11.013
Kong, F., Yin, H., Nakagoshi, N., and Zong, Y. (2010). Urban green space network development for biodiversity conservation: identification based on graph theory and gravity modeling. Landsc. urban Plan. 95, 16–27. doi:10.1016/j.landurbplan.2009.11.001
Laidlaw, R. K. (2000). Effects of habitat disturbance and protected areas on mammals of Peninsular Malaysia. Conserv. Biol. 14, 1639–1648. doi:10.1111/j.1523-1739.2000.99073.x
Laliberté, J., and St-Laurent, M. H. (2020). Validation of functional connectivity modeling: the Achilles’ heel of landscape connectivity mapping. Landsc. Urban Plan. 202, 103878. doi:10.1016/j.landurbplan.2020.103878
Lambeck, R. J. (1997). Focal Species: a Multi-Species Umbrella for Nature Conservation: especies Focales: una Sombrilla Multiespecífica para Conservar la Naturaleza. Conserv. Biol. 11, 849–856. doi:10.1046/j.1523-1739.1997.96319.x
Langmaier, M., and Lapin, K. (2020). A systematic review of the impact of invasive alien plants on forest regeneration in European temperate forests. Front. Plant Sci. 11, 524969. doi:10.3389/fpls.2020.524969
Laurance, W. (2018). If you can't build well, then build nothing at all. Nature 563, 295–296. doi:10.1038/d41586-018-07348-3
Lee, E. J., and Rhim, S. J. (2017). Influence of vegetation area and edge length on mammals in urban woods. Animal Cells Syst. 21, 294–299. doi:10.1080/19768354.2017.1348983
Lerman, S. B., Narango, D. L., Avolio, M. L., Bratt, A. R., Engebretson, J. M., Groffman, P. M., et al. (2021). Residential yard management and landscape cover affect urban bird community diversity across the continental USA. Ecol. Appl. 31, e02455. doi:10.1002/eap.2455
Le Viol, I., Chiron, F., Julliard, R., and Kerbiriou, C. (2012). More amphibians than expected in highway stormwater ponds. Ecol. Eng. 47, 146–154. doi:10.1016/j.ecoleng.2012.06.031
Le Viol, I., Mocq, J., Julliard, R., and Kerbiriou, C. (2009). The contribution of motorway stormwater retention ponds to the biodiversity of aquatic macroinvertebrates. Biol. Conserv. 142, 3163–3171. doi:10.1016/j.biocon.2009.08.018
Li, B. (2018). “The influence of landscape structure and habitat type on Amphibian in different urbanization area of shanghai,” ([Shanghai]: East China Normal University). [dissertation/doctoral thesis].
Li, F., Liu, X., Zhang, X., Zhao, D., Liu, H., Zhou, C., et al. (2017). Urban ecological infrastructure: an integrated network for ecosystem services and sustainable urban systems. J. Clean. Prod. 163, S12–S18. doi:10.1016/j.jclepro.2016.02.079
Li, H., Li, D., Li, T., Qiao, Q., Yang, J., and Zhang, H. (2010). Application of least-cost path model to identify a giant panda dispersal corridor network after the Wenchuan earthquake—case study of Wolong Nature Reserve in China. Ecol. Model. 221, 944–952. doi:10.1016/j.ecolmodel.2009.12.006
Li, W., Cui, L. J., and Zhao, X. S. (2014). Measures of conservation and restoration for the development of wetland park. Wetl. Sci. Manag. 10, 13–16.
Liang, J., He, X., Zeng, G., Zhong, M., Gao, X., Li, X., et al. (2018). Integrating priority areas and ecological corridors into national network for conservation planning in China. Sci. total Environ. 626, 22–29. doi:10.1016/j.scitotenv.2018.01.086
Liang, L. W., Wang, Z. B., Fang, C. L., and Sun, Z. (2019). Spatiotemporal differentiation and coordinated development pattern of urbanization and the ecological environment of the Beijing-Tianjin-Hebei urban agglomeration. Acta Ecol. Sin. 39, 1212–1225. doi:10.5846/stxb201809162015
Lin, J. T., Yang, D. D., and Cao, L. (2016). Exploration and conception of aqueous system protection strategy of qilihai wetland. J. Tianjin Univ. Sci. 18, 445–450.
Lin, S. S., Chen, Y. T., and Sun, Y. J. (2018). Diversity of Amphibian and construction of micro-habitats in shenzhen's urban park. Guangdong Landsc. Archit. 40, 4–9.
Lindenmayer, D. B., Lane, P. W., Westgate, M. J., Crane, M., Michael, D., Okada, S., et al. (2014). An empirical assessment of the focal species hypothesis. Conserv. Biol. 28, 1594–1603. doi:10.1111/cobi.12330
Łopucki, R., and Kitowski, I. (2017). How small cities affect the biodiversity of ground-dwelling mammals and the relevance of this knowledge in planning urban land expansion in terms of urban wildlife. Urban Ecosyst. 20, 933–943. doi:10.1007/s11252-016-0637-y
Lumbierres, M., Dahal, P. R., Di Marco, M., Butchart, S. H., Donald, P. F., and Rondinini, C. (2022). Translating habitat class to land cover to map area of habitat of terrestrial vertebrates. Conserv. Biol. 36, e13851. doi:10.1111/cobi.13851
Lyon, J. P., Bird, T. J., Kearns, J., Nicol, S., Tonkin, Z., Todd, C. R., et al. (2019). Increased population size of fish in a lowland river following restoration of structural habitat. Ecol. Appl. 29, e01882. doi:10.1002/eap.1882
Ma, Y., Li, F., and Yang, R. (2021). The impact of urbanization on biodiversity and its regulation countermeasures. Chin. Landsc. Archit. 37, 6–13. doi:10.19775/j.cla.2021.05.0006
Maerz, J. C., Blossey, B., and Nuzzo, V. (2005). Green frogs show reduced foraging success in habitats invaded by Japanese knotweed. Biodivers. Conservation 14, 2901–2911. doi:10.1007/s10531-004-0223-0
Mahmoudzadeh, H., Masoudi, H., Jafari, F., Khorshiddoost, A. M., Abedini, A., and Amir, M. (2022). Ecological networks and corridors development in urban areas: an example of Tabriz, Iran. Front. Environ. Sci. 1269. doi:10.3389/fenvs.2022.969266
Maleki, S., Soffianian, A. R., Koupaei, S. S., Saatchi, S., Pourmanafi, S., and Sheikholeslam, F. (2016). Habitat mapping as a tool for water birds conservation planning in an arid zone wetland: the case study Hamun wetland. Ecol. Eng. 95, 594–603. doi:10.1016/j.ecoleng.2016.06.115
Matos, C., Petrovan, S. O., Wheeler, P. M., and Ward, A. I. (2019). Landscape connectivity and spatial prioritization in an urbanising world: a network analysis approach for a threatened amphibian. Biol. Conserv. 237, 238–247. doi:10.1016/j.biocon.2019.06.035
Matthews, S. N. (2008). A new perspective on corridor design and implementation. Landsc. Ecol. 23, 497–498. doi:10.1007/s10980-008-9192-0
Matthies, S. A., Rueter, S., Schaarschmidt, F., and Prasse, R. (2017). Determinants of species richness within and across taxonomic groups in urban green spaces. Urban Ecosyst. 20, 897–909. doi:10.1007/s11252-017-0642-9
Maynou, X., Martín, R., and Aranda, D. (2017). The role of small secondary biotopes in a highly fragmented landscape as habitat and connectivity providers for dragonflies (Insecta: odonata). J. Insect Conservation 21, 517–530. doi:10.1007/s10841-017-9992-0
McComb, L. B., Lentini, P. E., Harley, D. K. P., Lumsden, L. F., Eyre, A. C., and Briscoe, N. J. (2022). Climate and behaviour influence thermal suitability of artificial hollows for a critically endangered mammal. Anim. Conserv. 25, 401–413. doi:10.1111/acv.12750
McCormick, S. (2012). After the cap: risk assessment, citizen science and disaster recovery. Ecol. Soc. 17. doi:10.5751/ES-05263-170431
McIntyre, N. E. (1997). Scale-dependent habitat selection by the darkling beetle Eleodes hispilabris (Coleoptera: tenebrionidae). Am. Midl. Nat. 138, 230–235. doi:10.2307/2426671
McKinley, D. C., Miller-Rushing, A. J., Ballard, H. L., Bonney, R., Brown, H., Cook-Patton, S. C., et al. (2017). Citizen science can improve conservation science, natural resource management, and environmental protection. Biol. Conserv. 208, 15–28. doi:10.1016/j.biocon.2016.05.015
McKinney, M. L. (2002). Urbanization, Biodiversity, and Conservation: the impacts of urbanization on native species are poorly studied, but educating a highly urbanized human population about these impacts can greatly improve species conservation in all ecosystems. Bioscience 52, 883–890. doi:10.1641/0006-3568(2002)052[0883:UBAC]2.0.CO;2
McKinney, M. L. (2006). Urbanization as a major cause of biotic homogenization. Biol. Conserv. 127, 247–260. doi:10.1016/j.biocon.2005.09.005
McRae, B. H., Dickson, B. G., Keitt, T. H., and Shah, V. B. (2008). Using circuit theory to model connectivity in ecology, evolution, and conservation. Ecology 89, 2712–2724. doi:10.1890/07-1861.1
Meland, S., Sun, Z., Sokolova, E., Rauch, S., and Brittain, J. E. (2020). A comparative study of macroinvertebrate biodiversity in highway stormwater ponds and natural ponds. Sci. Total Environ. 740, 140029. doi:10.1016/j.scitotenv.2020.140029
Mikula, P., Šaffa, G., Nelson, E., and Tryjanowski, P. (2018). Risk perception of vervet monkeys Chlorocebus pygerythrus to humans in urban and rural environments. Behav. Process. 147, 21–27. doi:10.1016/j.beproc.2017.12.011
Miller, K. F., Wilson, D. J., Hartley, S., Innes, J. G., Fitzgerald, N. B., Miller, P., et al. (2022). Invasive urban mammalian predators: distribution and multi-scale habitat selection. Biology 11, 1527. doi:10.3390/biology11101527
Minor, E. S., and Urban, D. L. (2008). A graph-theory framework for evaluating landscape connectivity and conservation planning. Conservation biology 22, 297–307. doi:10.1111/j.1523-1739.2007.00871.x
Mohamad, N. H. N., Idilfitri, S., and Thani, S. K. S. O. (2013). Biodiversity by Design: the attributes of ornamental plants in urban forest parks. Procedia-social Behav. Sci. 105, 823–839. doi:10.1016/j.sbspro.2013.11.085
Morandi, D. T., de Jesus França, L. C., Menezes, E. S., Machado, E. L. M., da Silva, M. D., and Mucida, D. P. (2020). Delimitation of ecological corridors between conservation units in the Brazilian Cerrado using a GIS and AHP approach. Ecol. Indic. 115, 106440. doi:10.1016/j.ecolind.2020.106440
Moreno, R., Ojeda, N., Azócar, J., Venegas, C., and Inostroza, L. (2020). Application of NDVI for identify potentiality of the urban forest for the design of a green corridors system in intermediary cities of Latin America: case study, Temuco, Chile. Urban For. Urban Green. 55, 126821. doi:10.1016/j.ufug.2020.126821
Mowry, C. B., Lee, A., Taylor, Z. P., Hamid, N., Whitney, S., Heneghen, M., et al. (2021). Using community science data to investigate urban Coyotes (Canis latrans) in Atlanta, Georgia, USA. Hum. Dimensions Wildl. 26, 163–178. doi:10.1080/10871209.2020.1806415
Murgui, E. (2009). Seasonal patterns of habitat selection of the House Sparrow Passer domesticus in the urban landscape of Valencia (Spain). J. Ornithol. 150, 85–94. doi:10.1007/s10336-008-0320-z
Narango, D. L., Tallamy, D. W., and Marra, P. P. (2017). Native plants improve breeding and foraging habitat for an insectivorous bird. Biol. Conserv. 213, 42–50. doi:10.1016/j.biocon.2017.06.029
Nichols, J. D., and Williams, B. K. (2006). Monitoring for conservation. Trends Ecol. Evol. 21, 668–673. doi:10.1016/j.tree.2006.08.007
Nicholson, E., Lindenmayer, D. B., Frank, K., and Possingham, H. P. (2013). Testing the focal species approach to making conservation decisions for species persistence. Divers. Distributions 19, 530–540. doi:10.1111/ddi.12066
Nielsen, A. B., Van Den Bosch, M., Maruthaveeran, S., and van den Bosch, C. K. (2014). Species richness in urban parks and its drivers: a review of empirical evidence. Urban Ecosyst. 17, 305–327. doi:10.1007/s11252-013-0316-1
Niu, T. G., Hao, S., and Wen, S. (2022). Biodiversity restoration in large urban blue-green space: a case study of Beijing nanyuan forest and wetland park. J. Chin. Urban For. 20, 69–74.
Niu, T. G., Zhao, N., and Xu, D. D. (2021). Exploration of near-natural forest construction in beijing-tianjin-hebei plain. Chin. Landsc. Archit. (S1), 145–149. doi:10.19775/j.cla.2021.S1.0145
Nogués, S., and Cabarga-Varona, A. (2014). Modelling land use changes for landscape connectivity: the role of plantation forestry and highways. J. Nat. Conservation 22, 504–515. doi:10.1016/j.jnc.2014.08.004
Nori, J., Lemes, P., Urbina-Cardona, N., Baldo, D., Lescano, J., and Loyola, R. (2015). Amphibian conservation, land-use changes and protected areas: a global overview. Biol. Conserv. 191, 367–374. doi:10.1016/j.biocon.2015.07.028
Northrup, J. M., Vander Wal, E., Bonar, M., Fieberg, J., Laforge, M. P., Leclerc, M., et al. (2022). Conceptual and methodological advances in habitat-selection modeling: guidelines for ecology and evolution. Ecol. Appl. 32, e02470. doi:10.1002/eap.2470
Oertli, B. (2018). Freshwater biodiversity conservation: the role of artificial ponds in the 21st century. Aquatic Conservation Mar. Freshw. Ecosyst. 28, 264–269. doi:10.1002/aqc.2902
Oleksa, A., and Gawronski, R. (2006). Forest insects in an agricultural landscape—presence of old trees is more important than the existence of nearby forest. Ecol. Quest. 7, 29–36.
Oleksa, A., Gawroński, R., and Tofilski, A. (2013). Rural avenues as a refuge for feral honey bee population. J. Insect Conservation 17, 465–472. doi:10.1007/s10841-012-9528-6
Pascual-Hortal, L., and Saura, S. (2007). Impact of spatial scale on the identification of critical habitat patches for the maintenance of landscape connectivity. Landsc. Urban Plan. 83, 176–186. doi:10.1016/j.landurbplan.2007.04.003
Pearson, R. G., Raxworthy, C. J., Nakamura, M., and Townsend Peterson, A. (2007). Predicting species distributions from small numbers of occurrence records: a test case using cryptic geckos in Madagascar. J. Biogeogr. 34, 102–117. doi:10.1111/j.1365-2699.2006.01594.x
Phillips, S. J., Anderson, R. P., and Schapire, R. E. (2006). Maximum entropy modeling of species geographic distributions. Ecol. Model. 190, 231–259. doi:10.1016/j.ecolmodel.2005.03.026
Pimm, S. L., Alibhai, S., Bergl, R., Dehgan, A., Giri, C., Jewell, Z., et al. (2015). Emerging technologies to conserve biodiversity. Trends Ecol. Evol. 30, 685–696. doi:10.1016/j.tree.2015.08.008
Pirnat, J. (2000). Conservation and management of forest patches and corridors in suburban landscapes. Landsc. Urban Plan. 52, 135–143. doi:10.1016/S0169-2046(00)00128-6
Pithon, J. A., Duflot, R., Beaujouan, V., Jagaille, M., Pain, G., and Daniel, H. (2021). Grasslands provide diverse opportunities for bird species along an urban-rural gradient. Urban Ecosyst. 24, 1281–1294. doi:10.1007/s11252-021-01114-6
Pollack, L., Ondrasek, N. R., and Calisi, R. (2017). Urban health and ecology: the promise of an avian biomonitoring tool. Curr. Zool. 63, 205–212. doi:10.1093/cz/zox011
Potgieter, L. J., Gaertner, M., Kueffer, C., Larson, B. M., Livingstone, S. W., O’Farrell, P. J., et al. (2017). Alien plants as mediators of ecosystem services and disservices in urban systems: a global review. Biol. Invasions 19, 3571–3588. doi:10.1007/s10530-017-1589-8
Potgieter, L. J., Gaertner, M., O'Farrell, P. J., and Richardson, D. M. (2019). Perceptions of impact: invasive alien plants in the urban environment. J. Environ. Manag. 229, 76–87. doi:10.1016/j.jenvman.2018.05.080
Prevedello, J. A., and Vieira, M. V. (2010). Does the type of matrix matter? A quantitative review of the evidence. Biodivers. Conservation 19, 1205–1223. doi:10.1007/s10531-009-9750-z
Quinn, G. P., and Keough, M. J. (2002). Experimental design and data analysis for biologists. Cambridge: Cambridge University Press.
Razgour, O., Hanmer, J., and Jones, G. (2011). Using multi-scale modelling to predict habitat suitability for species of conservation concern: the grey long-eared bat as a case study. Biol. Conserv. 144, 2922–2930. doi:10.1016/j.biocon.2011.08.010
Rhim, S. J., Son, S. H., Hwang, H. S., Kang, J. H., Sung, J. H., Park, G. E., et al. (2015). Characteristics of mammal abundance relative to habitat variables in temperate forests. For. Sci. Technol. 11, 61–64. doi:10.1080/21580103.2014.920733
Richardson, S., Mill, A. C., Davis, D., Jam, D., and Ward, A. I. (2020). A systematic review of adaptive wildlife management for the control of invasive, non-native mammals, and other human–wildlife conflicts. Mammal. Rev. 50, 147–156. doi:10.1111/mam.12182
Rico-Silva, J. F., Cruz-Trujillo, E. J., and Colorado, Z. G. J. (2021). Influence of environmental factors on bird diversity in greenspaces in an Amazonian city. Urban Ecosyst. 24, 365–374. doi:10.1007/s11252-020-01042-x
Rincón, V., Velázquez, J., Gutiérrez, J., Hernando, A., Khoroshev, A., Gómez, I., et al. (2021). Proposal of new Natura 2000 network boundaries in Spain based on the value of importance for biodiversity and connectivity analysis for its improvement. Ecol. Indic. 129, 108024. doi:10.1016/j.ecolind.2021.108024
Rudd, H., Vala, J., and Schaefer, V. (2002). Importance of backyard habitat in a comprehensive biodiversity conservation strategy: a connectivity analysis of urban green spaces. Restor. Ecol. 10, 368–375. doi:10.1046/j.1526-100X.2002.02041.x
Russo, A., and Holzer, K. A. (2021). Biodiverse cities: exploring multifunctional green infrastructure for ecosystem services and human well-being. Urban Serv. Ecosyst. Green Infrastructure Benefits Landsc. Urban Scale, 491–507. doi:10.1007/978-3-030-75929-2_26
Saura, S., and Pascual-Hortal, L. (2007). A new habitat availability index to integrate connectivity in landscape conservation planning: comparison with existing indices and application to a case study. Landsc. urban Plan. 83, 91–103. doi:10.1016/j.landurbplan.2007.03.005
Saura, S., and Torné, J. (2009). Conefor Sensinode 2.2: a software package for quantifying the importance of habitat patches for landscape connectivity. Environ. Model. Softw. 24, 135–139. doi:10.1016/j.envsoft.2008.05.005
Saura Santiago, T. J., and lucía, P. (2008). Conefor sensinode 2.2. Lleida, Spain: processing, 0–56. Available from: http://www.conefor.org/files/usuarios/CS22manual.pdf.
Sayer, C., Andrews, K., Shilland, E., Edmonds, N., Edmonds-Brown, R., Patmore, I., et al. (2012). The role of pond management for biodiversity conservation in an agricultural landscape. Aquatic Conservation Mar. Freshw. Ecosyst. 22, 626–638. doi:10.1002/aqc.2254
Schwarz, N., Moretti, M., Bugalho, M. N., Davies, Z. G., Haase, D., Hack, J., et al. (2017). Understanding biodiversity-ecosystem service relationships in urban areas: a comprehensive literature review. Ecosyst. Serv. 27, 161–171. doi:10.1016/j.ecoser.2017.08.014
Shen, J., and Wang, Y. (2022). An improved method for the identification and setting of ecological corridors in urbanized areas. Urban Ecosyst., 1–20. doi:10.1007/s11252-022-01298-5
Šigutová, H., Pyszko, P., Valušák, J., and Dolný, A. (2022). Highway stormwater ponds as islands of Odonata diversity in an agricultural landscape. Sci. Total Environ. 837, 155774. doi:10.1016/j.scitotenv.2022.155774
Simberloff, D. (1998). Flagships, umbrellas, and keystones: is single-species management passé in the landscape era? Biol. Conserv. 83, 247–257. doi:10.1016/S0006-3207(97)00081-5
Skelly, D. K., Freidenburg, L. K., and Kiesecker, J. M. (2002). Forest canopy and the performance of larval amphibians. Ecology 83, 983–992. doi:10.1890/0012-9658(2002)083[0983:FCATPO]2.0.CO;2
Soga, M., and Koike, S. (2013). Large forest patches promote breeding success of a terrestrial mammal in urban landscapes. PLoS One 8, e51802. doi:10.1371/journal.pone.0051802
Soivio, A., Tähti, H., and Kristoffersson, R. (1968). Studies on the periodicity of hibernation in the hedgehog (Erinaceus europaeus L.): III. Hibernation in a constant ambient temperature of—5° C. Ann. Zool. Fenn. 5 (No. 2), 224–226.
Song, L. L., and Qin, M. Z. (2016). Identification of ecological corridors and its importance by integrating circuit theory. J. Appl. Ecol. 27, 3344–3352. doi:10.13287/j.1001-9332.201610.035
Stacey, P. B., and Taper, M. (1992). Environmental variation and the persistence of small populations. Ecol. Appl. 2, 18–29. doi:10.2307/1941886
Su, R., and Dai, D. X. (2021). “Planning approaches for urban biodiversity conservation——berlin and London experiences. Urban planning society of China,” in Spatial governance for high-quality development——2021 Chinese cities proceedings of planning annual conference (08 urban ecological planning) (China: China Architecture and Building Press), 558–567. doi:10.26914/c.cnkihy.2021.027920
Sulaiman, S., Mohamad, N. H. N., and Idilfitri, S. (2013). Contribution of vegetation in urban parks as habitat for selective bird community. Procedia-Social Behav. Sci. 85, 267–281. doi:10.1016/j.sbspro.2013.08.358
Sullivan, B. L., Wood, C. L., Iliff, M. J., Bonney, R. E., Fink, D., and Kelling, S. (2009). eBird: a citizen-based bird observation network in the biological sciences. Biol. Conserv. 142, 2282–2292. doi:10.1016/j.biocon.2009.05.006
Taylor, J. J., Lepczyk, C. A., and Brown, D. G. (2016). Patch and matrix level influences on forest birds at the rural–urban interface. Landsc. Ecol. 31, 1005–1020. doi:10.1007/s10980-015-0310-5
Teng, M., Wu, C., Zhou, Z., Lord, E., and Zheng, Z. (2011). Multipurpose greenway planning for changing cities: a framework integrating priorities and a least-cost path model. Landsc. urban Plan. 103, 1–14. doi:10.1016/j.landurbplan.2011.05.007
Teng, M. J., Zhou, Z. X., Wang, P., Wu, C. G., and Xu, Y. R. (2010). The function types of green corridor and the key issues in its planning based upon structure design and management. Acta Ecol. Sin. 30, 1604–1614.
Terrado, M., Sabater, S., Chaplin-Kramer, B., Mandle, L., Ziv, G., and Acuña, V. (2016). Model development for the assessment of terrestrial and aquatic habitat quality in conservation planning. Sci. total Environ. 540, 63–70. doi:10.1016/j.scitotenv.2015.03.064
Thomas, R. H., and Blakemore, F. B. (2007). Elements of a cost–benefit analysis for improving salmonid spawning habitat in the River wye. J. Environ. Manag. 82, 471–480. doi:10.1016/j.jenvman.2006.01.004
Thurgate, N. Y., and Pechmann, J. H. (2007). Canopy closure, competition, and the endangered dusky gopher frog. J. Wildl. Manag. 71, 1845–1852. doi:10.2193/2005-586
Tonkin, Z., Kitchingman, A., Lyon, J., Kearns, J., Hackett, G., O’Mahony, J., et al. (2017). Flow magnitude and variability influence growth of two freshwater fish species in a large regulated floodplain river. Hydrobiologia 797, 289–301. doi:10.1007/s10750-017-3192-5
Urban, D. L., Minor, E. S., Treml, E. A., and Schick, R. S. (2009). Graph models of habitat mosaics. Ecol. Lett. 12, 260–273. doi:10.1111/j.1461-0248.2008.01271.x
Wang, D., Ji, X., Jiang, D., and Liu, P. (2022). Importance assessment and conservation strategy for rural landscape patches in Huang-Huai plain based on network robustness analysis. Ecol. Inf. 69, 101630. doi:10.1016/j.ecoinf.2022.101630
Wang, H., and Pei, Z. (2020). Urban green corridors analysis for a rapid urbanization city exemplified in Gaoyou City. Jiangsu. For. 11, 1374. doi:10.3390/f11121374
Wang, H. K., Guo, X., Wang, M., Zhuang, H., Ahmad, S., Khan, T. U., et al. (2020). Gravity model toolbox: an automated and open-source ArcGIS tool to build and prioritize ecological corridors in urban landscapes. Glob. Ecol. Conservation 22, e01012. doi:10.1016/j.gecco.2020.e01012
Wang, Y., Kotze, D. J., Vierikko, K., and Niemelä, J. (2019). What makes urban greenspace unique–Relationships between citizens’ perceptions on unique urban nature, biodiversity and environmental factors. Urban For. Urban Green. 42, 1–9. doi:10.1016/j.ufug.2019.04.005
Wang, Y., Qu, Z., Zhong, Q., Zhang, Q., Zhang, L., Zhang, R., et al. (2022). Delimitation of ecological corridors in a highly urbanizing region based on circuit theory and MSPA. Ecol. Indic. 142, 109258. doi:10.1016/j.ecolind.2022.109258
Wang, Z. H. (2021). “Country park design based on habitat of small mammals,” ([Beijing: Beijing Forestry University). [dissertation/master's thesis].
Watling, J. I., and Donnelly, M. A. (2006). Fragments as islands: a synthesis of faunal responses to habitat patchiness. Conserv. Biol. 20, 1016–1025. doi:10.1111/j.1523-1739.2006.00482.x
Williams, R. L., Goodenough, A. E., Hart, A. G., and Stafford, R. (2013). Using long-term volunteer records to examine dormouse (Muscardinus avellanarius) nestbox selection. PloS one 8, e67986. doi:10.1371/journal.pone.0067986
Wolch, J. R., Byrne, J., and Newell, J. P. (2014). Urban green space, public health, and environmental justice: the challenge of making cities ‘just green enough. Landsc. urban Plan. 125, 234–244. doi:10.1016/j.landurbplan.2014.01.017
Worton, B. J. (1989). Kernel methods for estimating the utilization distribution in home-range studies. Ecology 70, 164–168. doi:10.2307/1938423
Wu, D., Yue, F., Luo, Z. K., and Wang, T. H. (2011). The distribution and habitat selection patterns of Amphibian population in Dalian Lake Wetland, Shanghai. J. Fudan Univ. Nat. Sci. 50 (3), 268–273. doi:10.15943/j.cnki.fdxb-jns.2011.03.015
Wu, J. (2010). Urban sustainability: an inevitable goal of landscape research. Landsc. Ecol. 25, 1–4. doi:10.1007/s10980-009-9444-7
Xian, L., Xu, B. Y., Weng, S. F., et al. (2020). Application of food source tree species and the construction of ecological landscape in urban green space of Guangzhou. J. Central South Univ. For. Technol. 40, 142–147. doi:10.14067/j.cnki.1673-923x.2020.02.017
Xiao, L., Cui, L., Jiang, Q. O., Wang, M., Xu, L., and Yan, H. (2020). Spatial structure of a potential ecological network in Nanping, China, based on ecosystem service functions. Land 9, 376. doi:10.3390/land9100376
Xiao, S., Wu, W., Guo, J., Ou, M., Pueppke, S. G., Ou, W., et al. (2020). An evaluation framework for designing ecological security patterns and prioritizing ecological corridors: application in Jiangsu Province, China. Landsc. Ecol. 35, 2517–2534. doi:10.1007/s10980-020-01113-6
Xiao, W. H., Zhou, Q. S., Zhu, C. D., Wu, D. H., and Xiao, Z. S. (2020). Advances in techniques and methods of wildlife monitoring. Chin. J. Plant Ecol. 44, 409. doi:10.17521/cjpe.2019.0165
Xu, S. Y., and Shen, Q. J. (2009). Urban biodiversity conservation: the planning ideal and the approach. Mod. Urban Res. 24, 12–18.
Xu, Z. L., Peng, H. H., and Peng, S. Z. (2015). The development and evaluation of species distribution models. Acta Ecol. Sin. 35, 557–567. doi:10.5846/stxb201304030600
Yang, G. D., Bai, J. Y., Xu, M. Y., et al. (2023). Study on birds species characteristics and habitat plant landscape in south China botanica garden. For. Environ. Sci. 39, 82–88.
Yang, H., Chen, W., and Chen, X. (2017). Regional ecological network planning for biodiversity conservation: a case study of China's poyang lake eco-economic region. Pol. J. Environ. Stud. 26, 1825–1833. doi:10.15244/pjoes/68877
Yang, J., Xing, Z., and Cheng, C. (2022). How urban fringe expansion affects green habitat diversity? Analysis from urban and local scale in hilly city. J. Environ. Public Health 2022, 1–11. doi:10.1155/2022/8566686
Yang, L., Qiu, H. F., and Wu, J. (2021). Strategies for habitat protection and ecological restoration of bird communities on the east lake green heart in wuhan. Chin. Landsc. Archit. 37, 93–98. doi:10.19775/j.cla.2021.06.0093
Yang, X. T., Zhong, Z. Q., Xiu, C., Lin, S. N., Li, Y., Zeng, X. W., et al. (2022). Application of A Species dispersal model to guide the establishment of A functional ecological corridor for Platalea minor in the coastal areas of guangdong. For. Environ. Sci. 38, 12–22.
Yarnell, R. W., Surgey, J., Grogan, A., Thompson, R., Davies, K., Kimbrough, C., et al. (2019). Should rehabilitated hedgehogs be released in winter? A comparison of survival, nest use and weight change in wild and rescued animals. Eur. J. Wildl. Res. 65, 1–10. doi:10.1007/s10344-018-1244-4
Yates, M. D., Loeb, S. C., and Guynn, D. C. (1997). The effect of habitat patch size on small mammal populations. Proc. ofannual Conf. Southeast. Assoc. Fish Wildl. Agencies 51, 501–510.
Yin, H., Ma, Y. M., and Xie, X. M. (2017). Study on the damaged plants in green space of residential area: case study in Beijing. J. Beijing For. Univ. 39, 101–108. doi:10.13332/j.1000-1522.20170310
Yuan, J., Wang, B., Wen, C., et al. (2021). Research on the river lsland wetland ecological landscape construction based on habitat restoration: a case study of tongzhousha river lsland, zhangjiagang. Chin. Landsc. Archit. 37, 150–155.
Zetterberg, A., Mörtberg, U. M., and Balfors, B. (2010). Making graph theory operational for landscape ecological assessments, planning, and design. Landsc. urban Plan. 95, 181–191. doi:10.1016/j.landurbplan.2010.01.002
Zhang, H., Gao, J. X., Ma, M. X., Shao, F. Z., Wang, Q., Li, G. Y., et al. (2017). Influence of road on breeding habitat of Nipponia nippon based on MaxEnt model. J. Appl. Ecol. 28, 1352–1359. doi:10.13287/j.1001-9332.201704.023
Zhang, L., and Wang, H. (2006). Planning an ecological network of Xiamen Island (China) using landscape metrics and network analysis. Landsc. Urban Plan. 78, 449–456. doi:10.1016/j.landurbplan.2005.12.004
Zhang, W. (2017). “Effects of habitat and landscape changes on the dynamics and genetic structure of Amphibians during urbanization,” ([Shanghai]: East China Normal University). [dissertation/doctoral thesis].
Zhang, X. (2017). “A preliminary study on the behavior and habitat selection of red-bellied squirrels in urban green spaces,” ([Harbin]: Northeast Forestry University). [dissertation/master's thesis].
Zhang, Y., and Song, W. (2020). Identify ecological corridors and build potential ecological networks in response to recent land cover changes in Xinjiang, China. Sustainability 12, 8960. doi:10.3390/su12218960
Zhang, Z. (2017). “Enhancing landscape connectivity in detroit through multifunctional green corridor modeling and design,” ([Ann Arbor]: University of Michigan). [dissertation/ doctoral thesis].
Zhang, Z., Meerow, S., Newell, J. P., and Lindquist, M. (2019). Enhancing landscape connectivity through multifunctional green infrastructure corridor modeling and design. Urban For. urban Green. 38, 305–317. doi:10.1016/j.ufug.2018.10.014
Zhao, S. M., Ma, Y. F., Wang, J. L., and You, X. Y. (2019). Landscape pattern analysis and ecological network planning of Tianjin City. Urban For. urban Green. 46, 126479. doi:10.1016/j.ufug.2019.126479
Zhou, W., Chen, T. Z., and Rao, X. L. (2018). Plant landscape planning of urban green spaces for birds attraction and protection. J. Chin. Urban For. 16, 25–29. doi:10.3969/j.issn.1672-4925.2018.01.006
Zhu, C., Fang, Y., Zhou, K. X., Mu, S. J., and Jiang, J. L. (2015). IUCN Red List of Ecosystems, a new tool for biodiversity conservation. Acta Ecol. Sin. 35, 2826–2836. doi:10.5846/stxb201309082235
Keywords: animal habitat, biodiversity, investigation, suitability assessment, planning strategy, management
Citation: Liu Z, Yin H, Wang Y, Cheng Q and Wang Z (2024) Research progress on animal habitat constructions from the perspective of urban biodiversity improvement. Front. Environ. Sci. 11:1133879. doi: 10.3389/fenvs.2023.1133879
Received: 29 December 2022; Accepted: 28 December 2023;
Published: 10 January 2024.
Edited by:
Bo Hong, Northwest A&F University, ChinaReviewed by:
Zhengkai Zhang, Northwest A&F University, ChinaDaniela Campobello, University of Palermo, Italy
Copyright © 2024 Liu, Yin, Wang, Cheng and Wang. This is an open-access article distributed under the terms of the Creative Commons Attribution License (CC BY). The use, distribution or reproduction in other forums is permitted, provided the original author(s) and the copyright owner(s) are credited and that the original publication in this journal is cited, in accordance with accepted academic practice. No use, distribution or reproduction is permitted which does not comply with these terms.
*Correspondence: Hao Yin, eWluaGFvQGJqZnUuZWR1LmNu