- 1State Key Laboratory of Comprehensive Utilization of Low-Grade Refractory Gold Ores, Zijin Mining Group Co., Ltd., Shanghang, China
- 2Fujian Province Colleges and University Engineering Research Center of Solid Waste Resource Utilization, Longyan University, Longyan, China
- 3State Key Laboratory of Structural Chemistry, Fujian Institute of Research on the Structure of Matter, Chinese Academy of Sciences, Fuzho, China
Analyzing the pollution level and ecological risk of heavy metals in tailings is a necessary step for conducting revegetation after a tailings pond’s closure. Herein, we determined the heavy metal pollution status and ecological risk in one low-grade copper sulfide tailings pond using chemical and mineralogical analysis, chemical extraction, and ecological risk assessment. The results showed that the low-grade copper sulfide tailings displayed a low pollution status and exhibited a very low ecological risk. Among six heavy metals (Cu, Pb, Zn, As, Cr, and Cd), only Cu (53.7 mg/kg) slightly exceeded its standard value limit (50 mg/kg), and was the main pollutant in the tailings. Due to its high toxicity, As had the maximum contribution to the potential ecological risk in the tailings. Pb, Zn, Cr, and Cd in the tailings were practically of no pollution, and at low or none potential ecological risk. In order to conduct revegetation in the tailings pond, more attention should be paid to the acidity change of tailings and its impact on the chemical activity and bioavailability of Cu and As. This research provides a theoretical basis for heavy metals risk control and revegetation in the low-grade copper sulfide tailings pond.
1 Introduction
Mineral resources provide an important material foundation for society development and national security (Wu et al., 2021). However, the mining processes generate a large amount of tailings, which is about 2–12 times of the metal extracted from the ore (Jiang et al., 2021). At present, over 10 billion tons of mine tailings are produced globally each year (Xie and van Zyl, 2020). Billions of tons of tailings are increasingly accumulated in tailings ponds and are inevitably exposed to the environment for a long-term period. These tailings usually contain various kinds of heavy metals such as Pb, As, Cr, Cd, Mn, Zn, and Cu, which are commonly associated with the metal ore and would be released into the surrounding farmland soil, groundwater system and nearby rivers by directly penetrating, surface runoff and/or groundwater recharge (Barcelos et al., 2020). Therefore, heavy metal contents in the environment near tailings ponds might continue to increase, leading to severe environmental pollution (Khoeurn et al., 2019; Wang et al., 2019; Kan et al., 2021; Luo et al., 2021; Qi et al., 2022a).
Previous studies have demonstrated the great impacts of tailings on environmental quality and the ecological landscape (Nie et al., 2022). For example, affected by Hg mining deposits, the average contents of Hg and Ni in agricultural soil in Gongguan of Shaanxi province exceeded the national secondary standard value by 3.9, and 4.0 times, respectively (Zhu et al., 2018). Affected by artisanal gold mining, the mean concentrations of Hg and Cd in six villages in Tongguan (east of Shaanxi Province of China) were respectively 3.9 and 5.1 times of their maximum allowable concentrations for agricultural soils (Xiao et al., 2017). The translocation and accumulation of heavy metals in tailings may not only threaten the regional ecological security (Dubey et al., 2018; Ismail et al., 2019), but also affect the human health through food chain via causing some chronic diseases, metabolism disorders, deformities, and cancers (Al osman et al., 2019; Sanaei et al., 2021). For instance, Wang et al. found that Cu, Cr, Pb, Cd, and As in vegetables and crops near four typical mining and smelting zones in central China were 6.7%, 6.7%, 66.7%, 80.0%, and 26.7% higher than the national standards, respectively (Wang et al., 2021b).
It is worth noting that only the total contents of heavy metals are not enough for assessing the ecological risk of tailings (Moore et al., 2014; Jiang et al., 2021). The toxicity, bioavailability, mobility, or potential risks of heavy metals should also depend on their chemical fractions (Zhao et al., 2021). Heavy metals’ chemical fractions in tailings can be determined by various extractants with different leaching strength (Cheng et al., 2018; Gitari et al., 2018; Roebbert et al., 2018; Wu et al., 2018). Among the various extraction protocols, the modified BCR sequential extraction is one of the most widely used method and applied in various environmental components such as soil, sediment, sewage sludge, and mining waste (Matong et al., 2016; Li et al., 2018; Wang et al., 2021a; Gao et al., 2021; Liu et al., 2022). By using this technique, the bioavailability of As, Cr, Cd, Mn, Pb, and Zn were also successfully estimated from environmental matrices (Barcelos et al., 2020). In addition, in the farmland soils around several typical mining areas, Zhao et al. (2021) analyzed the association relationship between multiple heavy metals and Fe fractions.
For characterizing the ecological risk of contaminants to the environment, various risk assessment methods have been developed in many studies under multiple matrices (Wu et al., 2018; Buch et al., 2021; Dash et al., 2021; Masri et al., 2021; Demirak et al., 2022; Zhang et al., 2022). Among these methods, risk assessment code (RAC), geo-accumulation index (Igeo), and potential ecological risk index (RI) have been widely used based on the total content and chemical fraction of heavy metals. For example, Cheng et al. reported a moderate soil Cd contamination around a coal mining area of Huaibei coalfield by applying RAC, Igeo and RI (Cheng et al., 2018). Using the improved Igeo and RI, Luo et al. (2021) found that Sb and As exhibited a serious risk and Cr, Cd, Cu, Pb, and Zn posed a low risk in Qinglong antimony mine tailings. In addition, the Nemerow integrated pollution index (PN) is also frequently applied to assess the pollution level of multiple heavy metals (Zhang et al., 2018; Chai et al., 2021).
Currently, one low-grade copper sulfide mine tailings pond has to face the problem of ecological restoration after its closure. In addition to copper, this low-grade copper sulfide ore consists of a large number of minerals, which contain potentially toxic elements, such as As, Pb, Zn, Mn, Cr, and Cd. In the process of copper mining, these coexisting heavy metals are discarded and enter the mine tailings pond. Heavy metals play a significant impact on the quality of the soil, and can be enriched in organisms (Xing et al., 2022). In order to successfully achieve ecological restoration of the tailings pond, investigating the pollution levels in the tailings and assessing the potential ecological risks has become an urgent problem.
Therefore, the objectives of this study are as follows: 1) performing the chemical and mineralogical analysis of the low-grade copper sulfide mine tailings; 2) determining the concentrations and chemical fraction of various heavy metals (Cu, Pb, Zn, As, Cr, and Cd); 3) investigating the pollution degree and ecological risk of these metals. This research is expected to provide a comprehensive information on heavy metals in the low-grade copper sulfide mine tailings and lay a basis for the tailings’ pollution control and vegetation reconstruction.
2 Materials and methods
2.1 The tailings sample and reagents
About 5.0 t tailings slurry were sampled from the total outlet of the transfer pump of the low-grade copper sulfide mine tailings pond. The water content of tailings slurry was about 40%. The collected tailings were air-dried, evenly mixed, grinding and sieved by a 10-mesh nylon mesh screen.
All reagents used in this study were analytically pure and were directly used. Only hydrochloric acid (purity 37%), nitric acid (purity 65%), and perchloric acid (purity 70%) were purchased from Xilong Scientific Co., Ltd. (Chengdu, China), and other reagents, including standard metal solutions (1000 mg/L) were purchased from Macklin Biochemical Technology Co., Ltd. (Shanghai, China). All the solutions were prepared in deionized water.
2.2 Chemical and mineralogical analysis
Analysis of physicochemical properties is necessary to characterize the tailings sample. The pH was measured using a pH meter (PHS-3C, Ramag, China) at a 2.5:1 water-to-tailings ratio. The cation exchange capacity was estimated at pH equal to seven by ammonium acetate exchange method (Belay et al., 2022). The mineral phase of the tailings was determined by X-ray diffraction spectroscopy (X’Per Powder, PANalytical, Netherlands) scanning from 10° to 80° at a scan speed of 1°/min. The chemical composition of the tailings was determined by X-ray fluorescence spectroscopy (S8 TIGER, Bruker, Germany). The particle size analysis of the tailings was performed with a laser particle size analyzer (BT-9300H(T), China) with a detection limit of 0.01 μm.
2.3 Tailings digestion and chemical fraction
2.3.1 Tailings digestion
The total contents of various heavy metals in the tailings were analyzed by aqua regia and HClO4 digestion method (Ying et al., 2022). 30 ml of acid solutions (15 ml HCl and 5 ml HNO3 + 10 ml HClO4) was added to 0.5 g tailings, and then heated to 200°C. Digested solutions were diluted by 1% HNO3 aquation into 100 ml volumetric flasks. All glassware was immersed in 20% nitric acid solution to at least 24 h before usage.
2.3.2 Chemical fractions of heavy metals
Chemical fractions of heavy metals in the low-grade copper sulfide tailings were determined by the modified BCR sequential extraction (Zhao et al., 2021; Demirak et al., 2022). This method separates the chemical fractions of heavy metals into four forms: weak acid soluble fraction, reducible fraction, oxidizable fraction and residual fraction (Jayarathne et al., 2018). Weak acid soluble fraction (F1): added 20 ml of 0.11 M CH3COOH solution to one 50 ml tube containing 0.5 g of tailings, continuously shaken for 16 h at 25°C. Reducible fraction (F2): added 20 ml of 0.5 M NH2OH∙HCl solution (adjusted to pH = 1.5 with HCl) to the former tube and shook for 16 h at 25°C. Oxidizable fraction (F3): added 3 ml of 30% H2O2 solution, shook intermittently for 1 h at 25°C, and then continued to digest for another 1 h at 85°C until the volume in the tube dropped to 2–3 ml. Repeated the above steps until the volume was reduced to 1 ml. Finally, put 20 ml of 1.0 M CH3COONH4 solution into the cooled tube and shook for 16 h. Residual fraction (F4): used aqua regia and HClO4 to extract the residual heavy metals.
Centrifuged all the above suspensions at 1500 r/min for 10 min, and then filtered by 0.45 μm membrane. The dissolved heavy metals were determined by a flame atomic absorption spectrophotometer (Shimadzu AA-7000F, Japan), while As was measured by atomic fluorescence spectrophotometer (AFS-9800, Beijing KeChuang HaiGuang Instrument Co., Ltd, China), and reported on a dry weight basis (mg/kg).
The accuracy of the modified BCR sequential extraction method was verified by comparing the sum of four chemical fractions with the total contents obtain from direct tailings digestion. The recovery of the sequential extraction method was calculated according to Eq. 1 (Demirak et al., 2022):
where
2.4 Pollution status and ecological risk of heavy metals
The risk assessment code (
2.4.1 Risk assessment code ( )
Chemical fractions of heavy metals largely affect their harm to the environment (Moore et al., 2014). In particular, the weak acid soluble fraction of metals is weakly bound to the minerals in soil, they would easily enter aqueous solution and affect the organisms. Therefore, the
where
2.4.2 Geo-accumulation index (Igeo)
The geo-accumulation index (
where
2.4.3 The Nemerow integrated pollution index ( )
The Nemerow integrated pollution index (
where
2.4.4 Potential ecological risk index ( )
The potential ecological risk index (
where
3 Results and discussion
3.1 Physicochemical properties of tailings
The physicochemical characteristics of tailings affect the accumulation and mobility of the heavy metals in tailings. The low-grade copper sulfide mine tailings was found to be acidic with a pH of 4.5. Cation exchange capacity was determined to be 13.1 cmol/kg. The d10, d50, and d90 values of the tailings particle sizes were 1.61, 16.18, and 72.4 μm, respectively. According to the particle size distribution results, it can be seen that the permeability of the tailings is very poor.
The XRD test (Figure 1) indicated that the tailings in the low-grade copper sulfide mine was composed by quartz, alunite, dickite, pyrite, and sericite. The results obtained by XRF (Table 2) showed that heavy metal elements in the tailings included Cu, Pb, Ba, As, Mn, Cr, Zn, Ni, and so on. Considering the content and the toxicity of various heavy metals, Cu, Pb, Zn, As, Cr, and Cd were chosen to be analyzed by tailings digestion and the modified BCR protocol.
3.2 Contents and chemical fractions of heavy metals in the low-grade copper sulfide tailings
3.2.1 Contents of heavy metals
Heavy metal concentrations, as shown in Table 3, were investigated to reveal the pollution status in the low-grade copper sulfide tailings pond. In comparison to the national soil pollution risk screening value limit (GB 15618-2018), only the Cu content (53.7 mg/kg) in tailings slightly exceeded the standard limit (50 mg/kg). The As content (33.9 mg/kg) in tailings was close to the standard limit (40 mg/kg), so more attention should be paid to it. The contents of Pb and Zn were significantly less than their relevant standard limits. The Cr and Cd contents in tailings were not detected. Based on the above analysis, Cu was considered to be the main pollutant in the tailings.
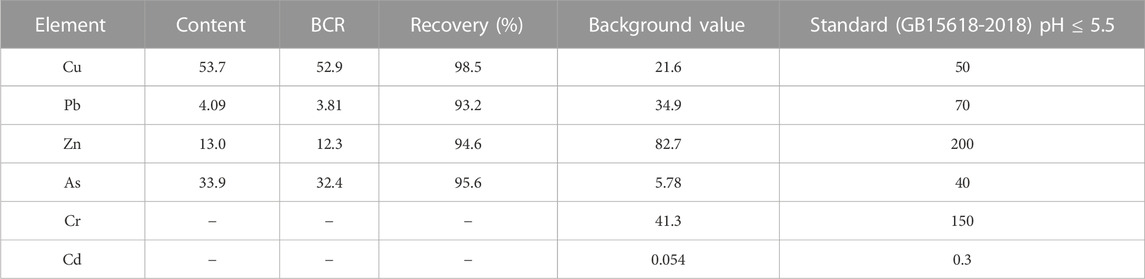
TABLE 3. Contents of six heavy metals in the tailings obtained by digestion and BCR, background values in Fujian Province and national soil pollution risk screening value (mg/kg).
3.2.2 Chemical fractions of heavy metals
Chemical fractions of heavy metals in the tailings were carried out to characterize their chemical activity and bioavailability as well as their risks to the environment. As shown in Table 3, recovery rates of all heavy metals were 93.2%–98.3%, suggesting that the sums of four chemical fractions were consistent with the total contents. Therefore, the modified BCR sequential extraction was reliable and repeatable. The chemical fractions of heavy metals were depicted in Figure 2. Various chemical fractions of Cr and Cd were not detected; thus, they were not provided here.
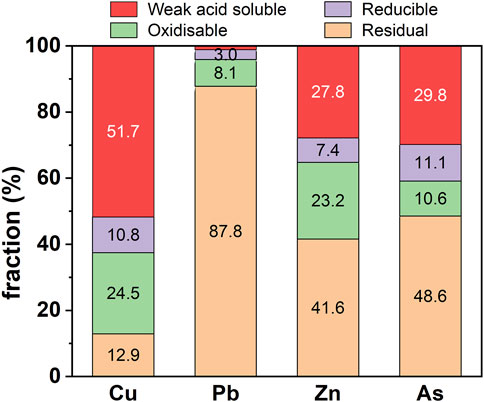
FIGURE 2. Heavy metals chemical fractions in the low-grade copper sulfide tailings using the modified BCR sequential extraction.
Cu. As shown in Figure 2, Cu was distributed in all four chemical fractions, and the considerable proportion (51.7%) of total Cu existed in the weak acid soluble fraction. This part of Cu existed in the form of ion-exchange and carbonate binding, and was loosely combined with soil matrices. Once the environmental conditions change, such as the pH, Cu in the weak acid soluble fraction would easily release into the environment. Therefore, among four kinds of chemical fractions, the weak acid soluble metal fraction is the most unstable, toxic, and bioavailable component (Matong et al., 2016; Demirak et al., 2022). The portion (24.5%) of oxidizable fraction was also noticeable. In oxidizable fraction, Cu combined with various organics and sulfide to form highly stable organic-copper compounds (Ma et al., 2016). About 12.9% and 10.8% of Cu had been found in residual and reducible fractions, respectively.
Pb. A proportion of 87.8% of Pb existed in the residual fraction, which was hard to release into pore-waters through dissociation due to its combination with aluminosilicate minerals (Burachevskaya et al., 2019; Ying et al., 2022). Only 8.1%, 3.0%, and 1.1% of Pb were found in oxidizable, reducible, and weak acid soluble fraction, respectively. Therefore, it is believed that Pb has weak chemical activity and bioavailability in the low-grade copper sulfide tailings.
Zn and As. Zn and As were very similar in chemical fractions, and mainly existed in the residual fraction with the proportions of 41.6% and 48.6%. This part of Zn and As were difficult to re-enter into the environment. In addition, there were about 30% of Zn and As in the weak acid soluble fraction, which were labile and easy to release under acidic conditions. 23.2% of Zn and 10.6% of As were observed in the oxidizable fraction. The reducible fractions of Zn and As were 7.4% and 11.1%, indicating that only small amounts of Zn and As were bound with Fe and Mn (oxyhydr) oxides.
3.3 Heavy metal pollution and ecological risk assessment
3.3.1 Risk assessment code ( )
Based on the chemical fraction results obtained by the modified BCR sequential extraction, the potential risks to the environment were estimated using the
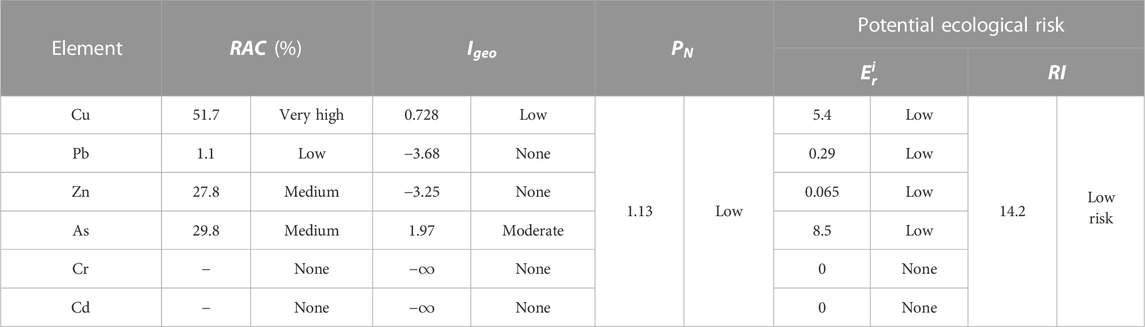
TABLE 4. The degrees of contamination and ecological risk of heavy metals in the low-grade copper sulfide tailings.
3.3.2 Geo-accumulation index ( )
For the purpose of determining heavy metals contamination levels in the tailings, the geo-accumulation index
3.3.3 The Nemerow integrated pollution index ( )
The Nemerow integrated pollution index (
3.3.4 Potential ecological risk index ( )
The potential ecological risk index (
4 Conclusion
Chemical and mineralogical analysis, chemical extraction, and ecological risk assessment were employed to evaluate the pollution level and ecological risk in the low-grade copper sulfide tailings pond. According to the results of
Among the six heavy metals (Cu, Pb, Zn, As, Cr, Cd) contained in the tailings, only Cu content exceeded the standard limit. Furthermore, the
It is urgent to pay more attention to the acidity change of tailings and its impact on the chemical activity and bioavailability of Cu and As for conducting revegetation in the low-grade copper sulfide tailings pond.
Data availability statement
The original contributions presented in the study are included in the article/supplementary material, further inquiries can be directed to the corresponding author.
Author contributions
PZ: Conceptualization, methodology, formal analysis, data curation, writing-original draft, visualization, supervision, funding acquisition. JC: Writing-review and editing. TL: Writing-review and editing. QW: Conceptualization, resources, project administration. ZW: Validation, resources. SL: Investigation, resources, data curation.
Funding
The study was financially supported by the Natural Science Foundation of Fujian Province (No. 2022J05250), and the Qimai Natural Science Foundation of Longyan City, China (No. XLQM002).
Acknowledgments
The authors express special thanks to doctor Dean Song and Ruiming Zhang for their efforts in the revision of the manuscript. The authors would like to thank the editors and reviewers for their pertinent comments and suggestions.
Conflict of interest
PZ, JC, QW, and ZW were employed by the company Zijin Mining Group Co., Ltd.
The remaining authors declare that the research was conducted in the absence of any commercial or financial relationships that could be construed as a potential conflict of interest.
Publisher’s note
All claims expressed in this article are solely those of the authors and do not necessarily represent those of their affiliated organizations, or those of the publisher, the editors and the reviewers. Any product that may be evaluated in this article, or claim that may be made by its manufacturer, is not guaranteed or endorsed by the publisher.
References
Al osman, M., Yang, F., and Massey, I. Y. (2019). Exposure routes and health effects of heavy metals on children. BioMetals 32 (4), 563–573. doi:10.1007/s10534-019-00193-5
Barcelos, D. A., Pontes, F. V. M., da Silva, F. A. N. G., Castro, D. C., dos Anjos, N. O. A., and Castilhos, Z. C. (2020). Gold mining tailing: Environmental availability of metals and human health risk assessment. J. Hazard. Mater. 397, 122721. doi:10.1016/j.jhazmat.2020.122721
Belay, S. A., Assefa, T. T., Worqlul, A. W., Steenhuis, T. S., Schmitter, P., Reyes, M. R., et al. (2022). Conservation and conventional vegetable cultivation increase soil organic matter and nutrients in the Ethiopian highlands. Water 14 (3), 476. doi:10.3390/w14030476
Buch, A. C., Niemeyer, J. C., Marques, E. D., and Silva-Filho, E. V. (2021). Ecological risk assessment of trace metals in soils affected by mine tailings. J. Hazard. Mater. 403, 123852. doi:10.1016/j.jhazmat.2020.123852
Burachevskaya, M., Minkina, T., Mandzhieva, S., Bauer, T., Chaplygin, V., Zamulina, I., et al. (2019). Study of copper, lead, and zinc speciation in the Haplic Chernozem surrounding coal-fired power plant. Appl. Geochem. 104, 102–108. doi:10.1016/j.apgeochem.2019.03.016
Chai, L., Wang, Y., Wang, X., Ma, L., Cheng, Z., and Su, L. (2021). Pollution characteristics, spatial distributions, and source apportionment of heavy metals in cultivated soil in Lanzhou, China. Ecol. Indic. 125, 107507. doi:10.1016/j.ecolind.2021.107507
Cheng, S., Liu, G., Zhou, C., and Sun, R. (2018). Chemical speciation and risk assessment of cadmium in soils around a typical coal mining area of China. Ecotoxicol. Environ. Saf. 160, 67–74. doi:10.1016/j.ecoenv.2018.05.022
Dash, S., Borah, S. S., and Kalamdhad, A. S. (2021). Heavy metal pollution and potential ecological risk assessment for surficial sediments of Deepor Beel, India. Ecol. Indic. 122, 107265. doi:10.1016/j.ecolind.2020.107265
Demirak, A., Kocakaya, M., and Keskin, F. (2022). Chemical fractions of toxic metals and assessment of risks on the environment and health in Mugla topsoils. Int. J. Environ. Sci. Technol. 19 (6), 5631–5648. doi:10.1007/s13762-021-03547-0
Dubey, S., Shri, M., Gupta, A., Rani, V., and Chakrabarty, D. (2018). Toxicity and detoxification of heavy metals during plant growth and metabolism. Environ. Chem. Lett. 16 (4), 1169–1192. doi:10.1007/s10311-018-0741-8
Gao, Y., Jia, J., Xi, B., Cui, D., and Tan, W. (2021). Divergent response of heavy metal bioavailability in soil rhizosphere to agricultural land use change from paddy fields to various drylands. Environ. Sci. Process. Impacts 23 (3), 417–428. doi:10.1039/D0EM00501K
Gitari, M. W., Akinyemi, S. A., Ramugondo, L., Matidza, M., and Mhlongo, S. E. (2018). Geochemical fractionation of metals and metalloids in tailings and appraisal of environmental pollution in the abandoned Musina Copper Mine, South Africa. Environ. Geochem. Health 40 (6), 2421–2439. doi:10.1007/s10653-018-0109-9
Ismail, A., Riaz, M., Akhtar, S., Goodwill, J. E., and Sun, J. (2019). Heavy metals in milk: Global prevalence and health risk assessment. Toxin Rev. 38 (1), 1–12. doi:10.1080/15569543.2017.1399276
Jayarathne, A., Egodawatta, P., Ayoko, G. A., and Goonetilleke, A. (2018). Assessment of ecological and human health risks of metals in urban road dust based on geochemical fractionation and potential bioavailability. Sci. Total Environ. 635, 1609–1619. doi:10.1016/j.scitotenv.2018.04.098
Jiang, L., Sun, H., Peng, T., Ding, W., Liu, B., and Liu, Q. (2021). Comprehensive evaluation of environmental availability, pollution level and leaching heavy metals behavior in non-ferrous metal tailings. J. Environ. Manag. 290, 112639. doi:10.1016/j.jenvman.2021.112639
Kan, X., Dong, Y., Feng, L., Zhou, M., and Hou, H. (2021). Contamination and health risk assessment of heavy metals in China’s lead–zinc mine tailings: A meta–analysis. Chemosphere 267, 128909. doi:10.1016/j.chemosphere.2020.128909
Khoeurn, K., Sakaguchi, A., Tomiyama, S., and Igarashi, T. (2019). Long-term acid generation and heavy metal leaching from the tailings of Shimokawa mine, Hokkaido, Japan: Column study under natural condition. J. Geochem. Explor. 201, 1–12. doi:10.1016/j.gexplo.2019.03.003
Li, Y.-C., Min, X.-B., Ke, Y., Chai, L.-Y., Shi, M.-Q., Tang, C.-J., et al. (2018). Utilization of red mud and Pb/Zn smelter waste for the synthesis of a red mud-based cementitious material. J. Hazard. Mater. 344, 343–349. doi:10.1016/j.jhazmat.2017.10.046
Liu, H., Qu, M., Chen, J., Guang, X., Zhang, J., Liu, M., et al. (2022). Heavy metal accumulation in the surrounding areas affected by mining in China: Spatial distribution patterns, risk assessment, and influencing factors. Sci. Total Environ. 825, 154004. doi:10.1016/j.scitotenv.2022.154004
Luo, G., Han, Z., Xiong, J., He, Y., Liao, J., and Wu, P. (2021). Heavy metal pollution and ecological risk assessment of tailings in the Qinglong Dachang antimony mine, China. Environ. Sci. Pollut. Res. 28 (25), 33491–33504. doi:10.1007/s11356-021-12987-7
Ma, X., Zuo, H., Tian, M., Zhang, L., Meng, J., Zhou, X., et al. (2016). Assessment of heavy metals contamination in sediments from three adjacent regions of the Yellow River using metal chemical fractions and multivariate analysis techniques. Chemosphere 144, 264–272. doi:10.1016/j.chemosphere.2015.08.026
Masri, S., Lebron, A., Logue, M., Valencia, E., Ruiz, A., Reyes, A., et al. (2021). Risk assessment of soil heavy metal contamination at the census tract level in the city of santa ana, CA: Implications for health and environmental justice. Environ. Sci. Process. Impacts 23, 812–830. doi:10.1039/d1em00007a
Matong, J. M., Nyaba, L., and Nomngongo, P. N. (2016). Fractionation of trace elements in agricultural soils using ultrasound assisted sequential extraction prior to inductively coupled plasma mass spectrometric determination. Chemosphere 154, 249–257. doi:10.1016/j.chemosphere.2016.03.123
Moore, F., Nematollahi, M. J., and Keshavarzi, B. (2014). Heavy metals fractionation in surface sediments of Gowatr bay-Iran. Environ. Monit. Assess. 187 (1), 4117. doi:10.1007/s10661-014-4117-7
Nemati, K., Bakar, N. K. A., Abas, M. R., and Sobhanzadeh, E. (2011). Speciation of heavy metals by modified BCR sequential extraction procedure in different depths of sediments from Sungai Buloh, Selangor, Malaysia. J. Hazard. Mater. 192 (1), 402–410. doi:10.1016/j.jhazmat.2011.05.039
Nie, W., Luo, M., Wang, Y., and Li, R. (2022). 3D visualization monitoring and early warning system of a tailings dam—gold copper mine tailings dam in zijinshan, fujian, China. Front. Earth Sci. 10, 800924. doi:10.3389/feart.2022.800924
Qi, Y., Fan, C., Quan, X., Xi, F., Liu, Z., Cao, Q., et al. (2022a). In-situ recycling strategy for co-treatment of antimony-rich sludge char and leachate: Pilot-scale application in an engineering case. Chem. Eng. J. 446, 137315. doi:10.1016/j.cej.2022.137315
Qi, Y., Wei, X., Zhao, M., Pan, W., Jiang, C., Wu, J., et al. (2022b). Heavy metal pollution characteristics and potential ecological risk assessment of soils around three typical antimony mining areas and watersheds in China. Front. Environ. Sci. 10. doi:10.3389/fenvs.2022.913293
Roebbert, Y., Rabe, K., Lazarov, M., Schuth, S., Schippers, A., Dold, B., et al. (2018). Fractionation of Fe and Cu isotopes in acid mine tailings: Modification and application of a sequential extraction method. Chem. Geol. 493, 67–79. doi:10.1016/j.chemgeo.2018.05.026
Sanaei, F., Amin, M. M., Alavijeh, Z. P., Esfahani, R. A., Sadeghi, M., Bandarrig, N. S., et al. (2021). Health risk assessment of potentially toxic elements intake via food crops consumption: Monte Carlo simulation-based probabilistic and heavy metal pollution index. Environ. Sci. Pollut. Res. 28 (2), 1479–1490. doi:10.1007/s11356-020-10450-7
Su, C., Meng, J., Zhou, Y., Bi, R., Chen, Z., Diao, J., et al. (2022). Heavy metals in soils from intense industrial areas in south China: Spatial distribution, source apportionment, and risk assessment. Front. Environ. Sci. 10. doi:10.3389/fenvs.2022.820536
Wang, J., Shi, L., Zhai, L., Zhang, H., Wang, S., Zou, J., et al. (2021a). Analysis of the long-term effectiveness of biochar immobilization remediation on heavy metal contaminated soil and the potential environmental factors weakening the remediation effect: A review. Ecotoxicol. Environ. Saf. 207, 111261. doi:10.1016/j.ecoenv.2020.111261
Wang, L., Zeraatpisheh, M., Wei, Z., and Xu, M. (2022). Heavy metal pollution and risk assessment of farmland soil around abandoned domestic waste dump in Kaifeng City. Front. Environ. Sci. 10. doi:10.3389/fenvs.2022.946298
Wang, P., Sun, Z., Hu, Y., and Cheng, H. (2019). Leaching of heavy metals from abandoned mine tailings brought by precipitation and the associated environmental impact. Sci. Total Environ. 695, 133893. doi:10.1016/j.scitotenv.2019.133893
Wang, Z., Bao, J., Wang, T., Moryani, H. T., Kang, W., Zheng, J., et al. (2021b). Hazardous heavy metals accumulation and health risk assessment of different vegetable species in contaminated soils from a typical mining city, central China. Int. J. Environ. Res. Public Health 18 (5), 2617. doi:10.3390/ijerph18052617
Wu, J., Lu, J., Li, L., Min, X., and Luo, Y. (2018). Pollution, ecological-health risks, and sources of heavy metals in soil of the northeastern Qinghai-Tibet Plateau. Chemosphere 201, 234–242. doi:10.1016/j.chemosphere.2018.02.122
Wu, W., Zhou, J., Niu, J., and Lv, H. (2021). Study on coupling between mineral resources exploitation and the mining ecological environment in Shanxi Province. Environ. Dev. Sustain. 23 (9), 13261–13283. doi:10.1007/s10668-020-01209-8
Xiao, R., Wang, S., Li, R., Wang, J. J., and Zhang, Z. (2017). Soil heavy metal contamination and health risks associated with artisanal gold mining in Tongguan, Shaanxi, China. Ecotoxicol. Environ. Saf. 141, 17–24. doi:10.1016/j.ecoenv.2017.03.002
Xie, L., and van Zyl, D. (2020). Distinguishing reclamation, revegetation and phytoremediation, and the importance of geochemical processes in the reclamation of sulfidic mine tailings: A review. Chemosphere 252, 126446. doi:10.1016/j.chemosphere.2020.126446
Xing, J., Gao, L., and He, L. (2022). Species distribution and concentration pollution of soil heavy metals in coal mine reclamation areas. Front. Environ. Sci. 10. doi:10.3389/fenvs.2022.925074
Ying, H., Zhao, W., Feng, X., Gu, C., and Wang, X. (2022). The impacts of aging pH and time of acid mine drainage solutions on Fe mineralogy and chemical fractions of heavy metals in the sediments. Chemosphere 303, 135077. doi:10.1016/j.chemosphere.2022.135077
Zhang, P., Qin, C., Hong, X., Kang, G., Qin, M., Yang, D., et al. (2018). Risk assessment and source analysis of soil heavy metal pollution from lower reaches of Yellow River irrigation in China. Sci. Total Environ. 633, 1136–1147. doi:10.1016/j.scitotenv.2018.03.228
Zhang, X., Gong, Z., Allinson, G., Xiao, M., Li, X., Jia, C., et al. (2022). Environmental risks caused by livestock and poultry farms to the soils: Comparison of swine, chicken, and cattle farms. J. Environ. Manag. 317, 115320. doi:10.1016/j.jenvman.2022.115320
Zhao, W., Gu, C., Ying, H., Feng, X., Zhu, M., Wang, M., et al. (2021). Fraction distribution of heavy metals and its relationship with iron in polluted farmland soils around distinct mining areas. Appl. Geochem. 130, 104969. doi:10.1016/j.apgeochem.2021.104969
Keywords: mine tailings, heavy metal, chemical fraction, pollution level, ecological risk assessmemt
Citation: Zhao P, Chen J, Liu T, Wang Q, Wu Z and Liang S (2023) Heavy metal pollution and risk assessment of tailings in one low-grade copper sulfide mine. Front. Environ. Sci. 11:1132268. doi: 10.3389/fenvs.2023.1132268
Received: 27 December 2022; Accepted: 01 February 2023;
Published: 22 February 2023.
Edited by:
Xiaohu Wen, Northwest Institute of Eco-Environment and Resources (CAS), ChinaReviewed by:
Yuanfeng Qi, Qingdao University of Technology, ChinaWenjun Huang, Shanghai Jiao Tong University, China
Jia Duo, Xinjiang Institute of Ecology and Geography (CAS), China
Copyright © 2023 Zhao, Chen, Liu, Wang, Wu and Liang. This is an open-access article distributed under the terms of the Creative Commons Attribution License (CC BY). The use, distribution or reproduction in other forums is permitted, provided the original author(s) and the copyright owner(s) are credited and that the original publication in this journal is cited, in accordance with accepted academic practice. No use, distribution or reproduction is permitted which does not comply with these terms.
*Correspondence: Qiankun Wang, d2FuZ19xaWFua3VuQHppamlubWluZy5jb20=