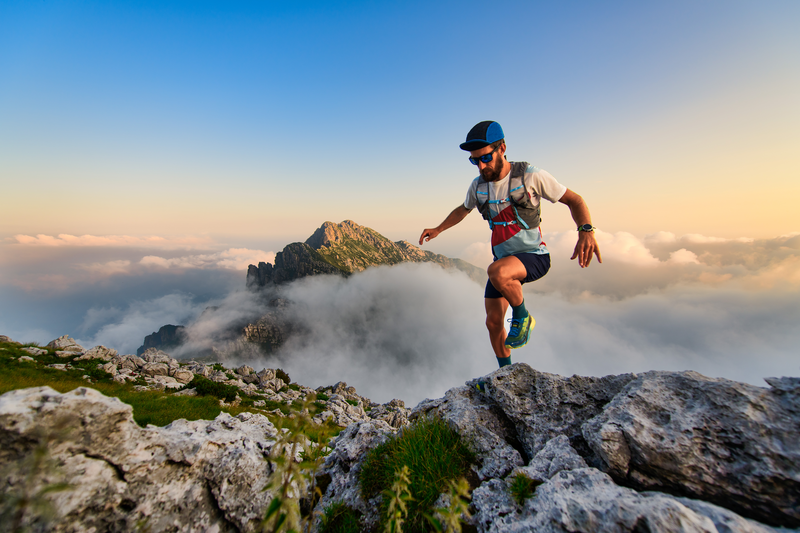
94% of researchers rate our articles as excellent or good
Learn more about the work of our research integrity team to safeguard the quality of each article we publish.
Find out more
ORIGINAL RESEARCH article
Front. Environ. Sci. , 10 March 2023
Sec. Soil Processes
Volume 11 - 2023 | https://doi.org/10.3389/fenvs.2023.1130455
This article is part of the Research Topic Soil Degradation and Restoration in Arid and Semi-Arid Regions View all 10 articles
Soil secondary salinization in the Yellow River Diversion Irrigation Area of Northwest China seriously threatens local agricultural production. Drip irrigation technology is one of the largest contributors to low-yielding saline-alkali land; however, research on the high spatio-temporal scale variability of soil moisture and salinity in drip irrigation is still lacking. Herein, four treatments, CK (flood irrigation, 900 mm), W1 (small volume drip irrigation, 360 mm), W2 (medium volume drip irrigation, 450 mm), and W3 (large volume drip irrigation, 540 mm), were set up to investigate the characteristics and laws of soil moisture and salinity under different irrigation methods. The results showed that the soil moisture of drip irrigation was 5.02%–17.88% (W1), 7.36%–21.06% (W2), and 13.79%–27.88% (W3) higher than that of flood irrigation, resulting in a vertical distribution of soil moisture being low at the top and high at the bottom. Under drip irrigation, the soil salinity formed a desalination zone centered on the drip emitter and this zone gradually expanded to deeper soil with continuous drip irrigation, gradually transforming the soil from surface aggregation type to the bottom accumulation type. The desalination rates of W1, W2, and W3 were 18.46%, 20.84%, and 22.94%, respectively, whereas the salt leaching rate of CK was slower and the salt distribution was not uniform; therefore, the desalination rate was only 5.32%. By precisely controlling the irrigation water volume and flow, drip irrigation significantly reduced surface evaporation and subsurface leakage of water and improved water use efficiency, thus increasing grain yield. Compared with flood irrigation, the yield increase rates of W1, W2, and W3 were 6.6%, 16.18%, and 18.32%, respectively. Therefore, drip irrigation with an appropriate irrigation volume in the saline land in northern Ningxia can improve water saving, salt suppression, and maize yield.
Soil salinization is a major global environmental problem with severe negative impacts on crop planting and sustainable agricultural development in arid and semi-arid regions (Chhabra, 2004; Ashraf, 2007). In saline-alkali areas with high groundwater tables, the movement of water and salt to the surface caused by capillary upwelling and high evaporation is the main cause of salinization (Northey et al., 2006). The Yellow River Diversion Irrigation Agricultural Area is located in the semi-arid region of Northwest China. People have diverted water from the Yellow River for hundreds of years allowing the Yellow River Basin in ancient China to achieve prosperity in agriculture and the development of civilization under climatic conditions with an average annual precipitation of only 200 mm (Wang et al., 1993; Xiong et al., 1996). However, excessive irrigation leads to the accumulation of salt in groundwater and secondary salinization, and insufficient fertilization leads to a decrease in water and fertilizer use efficiency and fertilizer use efficiency, resulting in agricultural non-point source pollution (Liu et al., 2014; Wang et al., 2014). At present, hundreds of years of irrigation by the Yellow River have raised the groundwater table, and the dry and high evaporation climate conditions in this region have further exacerbated the local soil salinization process, seriously threatening normal agricultural production (Wang et al., 1993; Xiong et al., 1996).
The essence of saline soil improvement measures is to regulate the movement of soil water and salt, to promote the downward leaching of soil salt, and to prevent salt from migrating upward to the surface with the soil solution due to transpiration (Guan et al., 2019; Stavi et al., 2021; Gu et al., 2022). The traditional water-saving measure is to remove soil salt by flooding before sowing, but this method wastes valuable water resources and increases the risk of environmental pollution (Pimentel et al., 2004). Drip irrigation can accurately control the amount of water and nutrients applied to the soil, ensuring adequate levels of water, nutrients and aeration in the soil-root zone (Burt and Isbell, 2005; Rajak et al., 2006). Drip irrigation also removes excess salt from the root zone through small area irrigation and slow infiltration, forming a desalination zone with sufficient water and less salt near the emitter, creating a suitable low-salinity microenvironment for the normal growth of plants (Burt and Isbell, 2005). It has become a key irrigation technology in the promotion of water conservation, salt suppression, and productivity improvement in saline-alkali land (Stavi et al., 2021) and is one of the most effective methods for developing low-yield salinized farmland, being widely used in salinized farmlands globally. Nevertheless, improper drip irrigation methods can cause in many problems, particularly in highly saline soils (Darwish et al., 2005). Therefore, when designing a drip irrigation system for planting crops in saline-alkali soils, the amount and timing of drip irrigation must be carefully optimized.
Proper management and evaluation of drip irrigation requires a good understanding of salt movement patterns and crop water consumption. However, the mechanism of this water-salt transport is not well understood, especially because research on the strong variability of soil moisture and salinity at the spatial and temporal levels is lacking. Therefore, it is an urgent need to study the distribution characteristics and laws of soil moisture and salinity under different irrigation methods. To improve the theory of water-salt movement and drip irrigation regulation, and realize the sustainable development and utilization of saline soil by drip irrigation technology, this study carried out field experiments on salinized soils of different degrees in the Yellow River Irrigation Area of Ningxia. This study mainly focused on: 1) the spatial and temporal dynamic transport of soil water and salt under drip irrigation, 2) plant responses to different drip irrigation systems, and 3) clarifying the desalination mechanism under drip irrigation scheduling.
The experiment was carried out in the Saline Land Water-saving and Salt-control Technology Demonstration Area (38°84′N, 106°57′E, 1,100 m), in Pingluo County, Ningxia Hui Autonomous Region, Northwest China. The whole region has a temperate continental semi-arid climate, with an annual average temperature of 9.5 °C, a minimum temperature of −22.6 °C, and a maximum temperature of 37.8 °C. The annual sunshine hours were 2,900 h, and the frost-free period was 180 days. The mean annual precipitation and potential evaporation (PE) were 180 mm and 1900 mm, respectively. The soil type was saline-irrigated silt with a medium loam texture. The physical and chemical properties of the initial soil are listed in Table 1. Before the experiment, the groundwater had an average depth of 1.4 m, a pH of 8.4, and an electrical conductivity (EC) of 15 dS m-1.
The experiment was conducted from April 2022 to September 2022, and the test crop was silage maize (Zea mays L.) of variety Dajingjiu 26. The planting method was set in wide (60 cm) and narrow (40 cm) with a planting density of 22 × 50 cm (Figure 1). Based on the local traditional irrigation quota and fertilization habits, four treatments were set up: 1) CK (traditional flood irrigation, 900 mm of irrigation during the growth season), 2) W1 (360 mm of drip irrigation during the growth season), 3) W2 (450 mm of drip irrigation throughout the growing season), and 4) W3 (540 mm of drip irrigation throughout the growing season). Each treatment was repeated three times, and a total of 12 plots (randomly arranged) were set up with an area of 20 × 20 m2.
FIGURE 1. Planting pattern and soil sampling positions in the field experiment. (A), Cross-section view of plot; (B), Top view of the plot details.
The flood irrigation treatment (CK) used urea, superphosphate, and potassium sulfate as fertilizers. The amounts of N, P, and K were 400 kg N ha-1, 200 kg P2O5 ha-1, and 225 kg K2O ha-1, respectively, of which 65% N was used as base fertilizer and 35% as topdressing. Rotary tillage was carried out at a depth of 20–25 cm immediately after the application of base fertilizer. The remaining urea was top dressed twice during the maize growth period as required. The drip irrigation treatments (W1, W2, and W3) used water-soluble fertilizer (24-12-14) specialized for maize drip irrigation. There were 10 times of irrigation in the maize growing season, including 7 times of drip irrigation fertilization. The total fertilization amount was the same as that of CK, and the specific fertilization and irrigation were shown in Table 2.
Irrigation water was pumped from an irrigation canal connected to the Yellow River with a pH, EC, and SARe of 7.5, 0.8 dS m-1, and 1.87 mmol L-1, respectively. The drip irrigation system was composed of a solenoid valve, pressure meter, flow meter, screen filter, fertilizer tank, and drip lines. Each treatment (three plots) was independently installed using a drip irrigation field control system to control the irrigation quota. Drip irrigation pipes were arranged in narrow rows, with one pipe for every two rows of maize (Figure 1). The distance between the drip irrigation emitters was 20cm, and the flow rate of the emitters was 1.27 L h-1. As in the local high-yielding maize fields, field management included weeding and insecticide control.
The entire experimental period was divided into Stage A (end of April to end of May), Stage B (end of May to end of June), Stage C (end of June to end of July), and Stage D (end of July to early September) during the maize growing season to collect the soil samples. For each sample, sites near the drip emitter were randomly selected in each plot, and soil samples were collected using an auger with a diameter of 4.0 cm in and a height of 15 cm. Samples were collected at distances of 0, 10, 20, 30, 40, and 50 cm from the emitters, and at sampling depths of 10, 20, 30, 40, 50, 60, 70, 80, 90, and 100 cm (Figure 1). Sixty soil samples were obtained from each sampling site. The distribution of soil sampling points for the flood irrigation treatment was similar that of the drip irrigation treatments, with 60 soil samples were randomly selected from each plot. After removing the surface organic impurities and fine roots from fresh samples, the soil water content was determined using the oven-drying method. The remaining soil subsamples were air-dried and sieved through a 1 mm sieve, and then three replicates were mixed into one sample to make a saturated soil slurry extract using the standard method (Robbins and Wiegand, 1990). The pH and ECe were measured using a conductivity meter (DDS-12A, REX) and a pH meter (PHS-3C, REX), respectively. Na+, Ca2+ and Mg2+ were measured using an inductively coupled plasma optical emission spectrometer (Optima 5300DV), and SARe (sodium adsorption ratio) was calculated as follows:
where the concentration of each cation is in mmol L-1.
The soil desalination rate (SDR) was used to characterize the desalination process under different treatments:
Where SDR is the soil desalination rate (%), S0 is the initial soil ECe (dS m-1), Si is the soil ECe for each soil layer at different stages (dS m-1).
At the mature stage of maize, three maize plants were randomly selected from each plot to measure their plant height and stem diameter. In this way, nine maize plants are collected from each treatment, and a total of 36 maize plants are collected. After on-site measurement, the whole plant shall be sampled and transported back to the laboratory immediately, dried to constant weight at 70°C, measured the weight of dry matter and 1,000 grains on the ground, and then calculated the grain yield. Based on the aboveground dry matter mass and grain yield, the nutritional quality index of silage maize was calculated using the following formula:
Where N is the nutritional quality index of silage maize (%), Y is the grain yield (kg ha−1), and M is the aboveground dry matter mass per unit area (kg ha−1). The water use efficiency in the maize growth season was calculated according to the following equation (Zhang et al., 2019):
Where WUE is the water use efficiency (kg ha−1 mm−1), Y is the grain yield (kg ha−1), and ET is the maize evapotranspiration during the growth period (mm) calculated by the soil water balance equation (Zhang et al., 2019) as:
Where ET is the maize evapotranspiration during the growth period (mm), I is the amount of irrigation (mm), P is the total precipitation (mm) collected by the field rain gauge. ΔS is the change in soil water storage (mm) estimated using the space-weighted mean method, G is the contribution of groundwater (mm), R is the surface runoff (mm), L is the underground leakage (mm) calculated by soil leakage water monitor, and E is the evaporation of surface water (mm) monitored by the micro-Lysimeter evaporator. Because the terrain of the test area was flat and the average depth of maize roots was much greater than the average depth of groundwater, G and R were ignored in this study.
All data were recorded and classified in Microsoft Office Excel 2016, and analysis of variance (ANOVA) was performed using IBM SPSS Statistics ver.19.0 (IBM Co., Armonk, NY, United States). Tukey’s honestly significant difference (HSD) test was used to determine significant differences between the means at p ≤ 0.05. Figures were created using Origin 2022 (Origin Lab Co., Northampton, MA, United States).
Changes in the spatial distribution of soil moisture with sampling time are shown in Figure 2. The soil moisture distribution changed significantly with the irrigation measures, indicating that irrigation changed the soil moisture infiltration characteristics. In Stage A, the wetted area under the drip emitters of the drip irrigation treatments (W1, W2, and W3) expanded both horizontally and vertically, and the water content of the 0–40 cm soil layer under W1, W2, and W3 increased by 3.78%–27.09%, 3.17%–37.79%, and 6.86%–44.87%, respectively, compared with CK. At the end of Stages B, C, and D, the soil wet zone continued to expand horizontally and vertically, and the soil water content in the 0–100 cm soil layer increased by 3.13%–6.36%, 3.28%–8.51%, and 5.01%–9.35%, respectively, compared with Stage A. The soil water content in the 0–20 cm soil layer of the drip irrigation treatment was significantly higher than that of CK, and the W1, W2, and W3 treatments were 5.02%–17.88%, 7.36%–21.06%, and 13.79%–27.88% higher than that of CK, respectively. Overall, irrigation increased soil moisture, resulting in a vertical distribution of soil moisture being low at the top and high at the bottom. The water content of the shallow soil in the flood irrigation treatment was significantly lower than that in the drip irrigation treatment. This is because the frequent side flow of irrigation water causes strong surface evaporation, indicating that drip irrigation has a better effect on maintaining surface soil moisture.
FIGURE 2. Spatial distribution of soil water (mass water content). (A), Stage A, end of April to end of May; (B), Stage B, end of May to end of June; (C), Stage C, end of June to end of July; (D), Stage D, end of July to early September.
The spatial distribution of ECe at different stages is shown in Figure 3, demonstrating the trend in soil salinity during the growing season. Soil moisture movement controls the redistribution of soil salts. Owing to the small amount of irrigation at Stage A, the soil salinity in the 0–40 cm soil layer of each treatment did not change much during this period. Nevertheless, starting from Stage B, the soil salinity decreased sharply to lower levels in all treatments; in particular, the ECe of the 0–20 cm soil layer in the W3 treatment decreased by 5.68% compared with other treatments. In Stage C, compared with the CK, the desalination zone gradually appeared 0–40 cm below the drip emitters in the drip irrigation treatments, and the soil salts gradually leached to the deep layer. At the end of Stage D, with continuous irrigation, the soil desalination zone continued to expand, and its lower boundary edge further moved from to 30–50 cm to 70–90 cm. There was a clear decreasing trend in soil salinity in the 0–100 cm soil layer under the drip irrigation treatments. Compared with CK, the ECe of 0–40 cm soil layer in W1, W2, and W3 decreased by 23.53%–32.57%, 26.49%–35.64%, and 26.30%–36.86%, respectively. In general, with the prolongation of the maize growth period, the soil salts gradually leached down with soil water infiltration under the drip irrigation treatments and gradually formed a desalination zone centered on the drip emitters. In contrast, the soil salt leaching rate of CK was lower, and the salt distribution was uneven.
FIGURE 3. Spatial distribution of soil salinity (electrical conductivity of saturated extract). (A), Stage A, end of April to end of May; (B), Stage B, end of May to end of June; (C), Stage C, end of June to end of July; (D), Stage D, end of July to early September.
Table 3 shows the trends in soil pH and SARe at the later stage of the field trial (end of Stage D). Except for the 10–20 cm soil layer, the drip irrigation treatments significantly reduced the soil pH value of each soil layer by 0.72%–2.13%, 0.83%–3.33%, and 0.81%–3.39% in W1, W2, and W3, respectively, compared with CK. When irrigation water removed salts from the surface soil, the pH of the 0–40 cm soil layer was significantly higher than that of the 40–80 cm soil layer. Similar to the soil ECe, it showed the vertical distribution characteristics of low salt content in the upper soil layer and high salt content in the lower soil layer as a whole, and the deep soil layer SARe was significantly higher than that of the shallow soil. The soil SARe values of the drip irrigation treatments were significantly lower than those of CK by 1.4%–6.63% (W1), 1.63%–7.02% (W2), and 1.43%–7.02% (W3). Consequently, the effect of salt washing of drip irrigation was positive, but the difference between the drip irrigation treatments was insignificant.
As illustrated in Table 4, there were significant differences in soil salinity and desalination rate in the different soil layers of each treatment. At the start of the field experiment, there were no significant differences in soil salinity between the treatments. At the later stage of the field experiment, there was a significant difference in the soil salts among the treatments. In the 0–10 cm, 10–20 cm, 20–30 cm and 40–50 cm soil layers, the soil salinity of the drip irrigation treatments was significantly lower than CK, but the difference was not significant between the drip irrigation treatments. As the soil layer deepened, the difference in soil salinity between treatments gradually decreased. Overall, the soil salinity of CK showed a trend of high up and low down, while that of the drip irrigation treatments showed the reverse trend. Compared with the drip irrigation treatments, the overall desalination efficiency of CK was lower, and the desalination rate of each soil layer was unevenly distributed, with an average of only 5.32%. The desalination rates of W1, W2, and W3 were 18.46%, 20.84%, and 22.94%, respectively. The drip irrigation treatments significantly reduced soil salinity in the tillage layer (0–40 cm), and the desalination rates were 32.45% (W1), 35.01% (W2), and 36.23% (W3), respectively. Notably, with the deepening of the soil layer, the soil became denser, the porosity decreased, and the soil desalination rate gradually decreased. Especially in the 90–100 cm soil layer, the soil desalination rate became negative, and the soil salinity tended to increase.
As shown in Table 5, the soil water storage (ΔS) varied from 36.59 to 45.59 mm throughout the growing season, and there was no significant difference among the treatments. Compared with the flood irrigation treatment, the drip irrigation treatments produced uniform infiltration flow and reduced underground leakage (L) by precisely controlling the volume and flow of irrigation water. Due to the frequent flow of surface irrigation water caused by large amounts of flood irrigation, the surface evaporation (E) of CK was significantly higher than that of the drip irrigation treatments by 57.64%–68.29%, while there was no significant difference between the drip irrigation treatments. It can be seen that underground leakage and surface evaporation of CK accounted for 35.8% of irrigation water and rainfall, significantly higher than W1 (32.61%), W2 (28.73%), and W3 (23.88%). Compared with traditional flood irrigation, drip irrigation significantly improved the water use efficiency of maize. The water use efficiency of W1 and W2 was the highest, reaching 23.88–25.68 kg ha-1 mm-1, followed by W3, and CK was the lowest at only 15.98 kg ha-1 mm-1.
It can be seen from Table 6 that different treatments had no significant effect on maize plant height, stem diameter, and aboveground biomass, but the drip irrigation treatments significantly increased maize grain yield, with a general trend of W3≥W2≥W1≥CK. Compared with CK, the grain yield increase rates of W1,W2, and W3 were 6.6%, 16.18%, and 18.32%, respectively, indicating that drip irrigation significantly increased maize grain yield, and the yield increase effect was better with the increase of irrigation quota. According to the calculation of the nutritional quality index from maize grain yield and aboveground biomass, the drip irrigation treatments significantly improved the nutritional quality of silage maize, and the nutritional quality indices of W2 and W3 were 10.73%–12.31%, and 11.30%–14.97% higher than that of CK, respectively. Thus, under the condition of high-frequency water-fertilizer drip irrigation, the water and fertilizer supply can better meet the water and fertilizer needs of maize growth, improving the grain yield and nutritional quality of silage maize.
Irrigation schedules play an important role in controlling the soil moisture and salinity in irrigated arid areas (Ren et al., 2019). Many studies have been carried out in arid and semi-arid areas on the technology of combining drip irrigation with water and fertilizer to form a water-saving irrigation technology system (Zheng et al., 2016; Wang et al., 2018; Hou et al., 2019). In this study, the water content of the soil layers changed significantly with time under different irrigation patterns and gradually increased with increasing soil depth, demonstrating that the irrigation measures significantly improved the soil water infiltration performance. Compared with traditional flood irrigation, drip irrigation of medium and large volumes significantly increased the soil water content of shallow (0–20 cm) and deep (70–100 cm) soils, indicating that high-frequency drip irrigation replenished the water loss of shallow soils due to high evaporation and transpiration in arid and semi-arid areas, thus maintaining the surface soil moisture at a high level (Dong et al., 2021). Therefore, the large amount of low-frequency irrigation water treated by flood irrigation and the high-frequency uniform irrigation water treated by drip irrigation may be the main reason for the difference in soil water content between the two treatments. The uniform and stable infiltration of irrigation water under drip irrigation increased the water content of deep soil and maintained the high content of soil water under the high evaporation climate environment. However, in the traditional flood irrigation treatment, owing to the large amount of irrigation water used simultaneously, the irrigation water tended to accumulate on the soil surface, which made the irrigation water prone to uneven underground leakage, which was not conducive to water infiltration and lead to uneven distribution of soil water (Figure 2). In addition, flood irrigation treatment resulted in evaporation loss of a large amount of water, increased evapotranspiration of farmland, reduced water use efficiency (Table 5) and wasted valuable water resources.
Soil water movement drives the diffusion of soil salinity, whereas ECe reflects the total amount of soil ions, which decreases with the leaching of soil salts by drip irrigation (Qi et al., 2018; Su et al., 2022). Consistent with previous research (Dong et al., 2022), our study observed a significant decrease in soil salinity under drip irrigation as the irrigation intervention continued from stages A to D (Figure 3). This is because the soil salts were dissolved by the infiltrating water during the drip irrigation process, and the high-frequency underground leachate diffused downward smoothly, gradually forming a desalination zone centered on the drip emitter. This finding is consistent with those of other studies showing that soil salts accumulate around the desalination zone under drip irrigation conditions (Burt and Isbell, 2005). One interesting finding is that with the infiltration of water, soil salinity gradually migrated to deeper soil layers, and the characteristics of soil salinity gradually changed from a surface aggregation pattern to a bottom accumulation pattern (Figure 3; Table 5), forming the vertical distribution characteristics of the upper lower and lower higher as a whole. A possible explanation for this might be that the blocked downward movement of salts was caused by the compacted and reduced porosity of the deep soil, and the close distance to the groundwater table, where the salts in groundwater can easily enter the soil through capillary action (Shah et al., 2011; Sun et al., 2022). Another important finding was the uneven distribution of water and soil salinity across soil layers under flood irrigation treatment, which indicating that under the flood irrigation condition, the irrigation water was easy to accumulate on the surface, and cannot form uniform underground seepage in the soil, resulting in the uneven distribution of salt in the soil, and ultimately reducing the soil desalination efficiency.
During the desalination process, the soil pH value decreased significantly in the 0–50 cm soil layer (Table 3), indicating that irrigation measures reduced shallow soil alkalinization. In contrast to earlier findings (Dong et al., 2021), drip irrigation could increase the soil pH within a certain range by moving the soil salts. These differences may be partly explained by the fact that the change in pH was not only the result of an exchange reaction between H+ and Na+, but also that the transport of soil moisture in the irrigated soil can change the multi-ion composition of the soil, thus affecting the change of soil pH; the specific reasons for this need to be further studied. In this study, with the extension of the maize growing season, the soil SARe decreased gradually (Table 3), which was similar to the distribution of soil ECe. Previous studies have interpreted this phenomenon as that under saline water conditions, because Na+ has a lower charge and smaller hydration radius than Ca2+ and Mg2+, it is less likely to be adsorbed by soil colloids and migrate downwards during drip irrigation (Zhao et al., 2019), so Na+ leaches more than Mg2+ and Ca2+ (White, 2005). Therefore, in the process of soil desalination in this study, more Na+ was leached and less Mg2+ and Ca2+ were washed away during the soil desalination process, resulting in a lower SARe calculated with Na+ as the numerator and Mg2+ and Ca2+ as the denominators. Furthermore, the SARe of each soil layer in the drip irrigation treatments was significantly lower than that in the flood irrigation treatment, indicating that the steady and continuous infiltration flow of drip irrigation accelerated the leaching of soil ions and significantly inhibited the process of soil salinization.
Water plays an indispensable role in plant growth, and improving water use efficiency in arid regions is an effective way to increase productivity (Qu et al., 2020). This study found that the surface evaporation of CK was nearly four times that of W1 (Table 5), indicating that the surface evaporation of traditional flood irrigation was greatly increased by frequent horizontal flow because of the large amount of irrigation water spread on the farmland at one time. It is worth noting that the amount of underground leakage under flood irrigation was significantly higher than that under drip irrigation. The reason may be that the terrain of this area was flat, and there was no surface runoff during irrigation. Therefore, a large amount of flood irrigation water accumulated on the surface, causing uneven infiltration, damaging the soil structure, and forming gaps and tunnels connected with groundwater in many places, thus increasing the underground leakage flow. These gaps and tunnels were likely responsible for salts that had leached into the groundwater returning to the surface with transpiration at the end of the maize growth season, causing soil re-salinization (Li et al., 2021). However, under the drip irrigation treatment, the irrigation water conducted a small amount of high frequency drip irrigation on the soil, without surface water accumulation, maintaining the soil structure, so the underground seepage flow was low. Overall, nearly half (41.57%) of the total input water was lost from the farmland ecosystem in the diffuse irrigation treatment, which was significantly higher than that in the drip irrigation treatments, significantly reducing the water use efficiency. Similar to other studies in the dry and saline inland areas of Northwest China (Wang et al., 2012), this study found that drip irrigation treatments reduced deep leakage and surface evaporation, and improved the water use efficiency of farmland by uniformly distributing water and precisely controlling water volume.
In this experiment, maize varieties were used as silage. Plant height and stem diameter are the key characteristics of maize growth, and the quality of aboveground dry matter mass is an important indicator of maize silage yield (Rüegg et al., 1998), and the nutritional quality of silage maize depends on the proportion of grain yield and aboveground dry matter mass (Lima et al., 2022). This study found that there were no significant differences in plant height, stem diameter and aboveground dry matter mass between irrigation modes (Table 4); however, compared with the traditional flood irrigation, the drip irrigation treatments significantly increased the grain yield and improved the nutritional quality and economic value of silage maize. With the increase in irrigation amount, the yield increase effect of drip irrigation treatments was greater than that of the flood irrigation treatment. In addition, the water use efficiency of maize differed significantly between irrigation modes, with the highest water use efficiency achieved by small-volume drip irrigation, followed by medium-volume drip irrigation, large-volume drip irrigation, and flood irrigation treatment. In summary, the irrigation frequency, fertilizer ratio and application amount of irrigation water under the drip irrigation treatment were consistent with the law of fertilizer and water demand of maize (Fan et al., 2020). Drip irrigation treatments could provide sufficient nutrients and water for the middle and later stages of maize growth, promote the nutrient absorption of maize roots, reduce the evapotranspiration of farmland water, improve the water use efficiency of maize, thus improve the yield of maize and nutritional quality. In this test, it was found that the medium and large water drip irrigation treatment was the best in terms of soil water content, salt content and maize yield. Compared with the traditional flood irrigation treatment, the soil water content under the medium and large water drip irrigation treatment was increased by 14%–21% (Figure 2), the soil salt was reduced by 21%–23% (Table 4), and the grain yield was increased by 17%–18% (Table 6). Therefore, the initial recommended irrigation water in Yellow River basin was 450–540 mm.
Drip irrigation significantly improves the infiltration performance of soil water. Through uniform infiltration of irrigation water and precise water and fertilizer control, soil salts were smoothly infiltrated into deeper soil, gradually forming a desalination zone centered on the drip emitter, which gradually changed the soil salinity characteristics from the surface aggregation mode to the bottom aggregation mode, thus significantly reducing surface evaporation and underground leakage, and improving water utilization efficiency and yield. In contrast, flood irrigation led to an uneven distribution of soil water and salt, poor desalination, and a significantly lower water use efficiency and yield than drip irrigation. Therefore, using drip irrigation with an appropriate irrigation volume in the Yellow River irrigated regions can achieve the goal of water saving and salt control, whilst effectively improving land productivity. However, our study was limited by the short duration of the research, which is only 1 year. Soil improvement in saline-alkali land is a long-term process. In the future, long-term and continuous drip irrigation is required to observe its long-term impact on soil structure, water, salinity and plant growth.
The raw data supporting the conclusion of this article will be made available by the authors, without undue reservation.
YW and RW contributed to the design of the study, and YW wrote the first draft of the manuscript. MG, HC, and XF conducted field sampling, laboratory analysis and statistical analysis. LW wrote sections of the manuscript. All authors contributed to manuscript revision, read, and approved the submitted version.
This study was funded by the National key research and development program of China (2021YFD1900600).
We would like to thank other Ningxia University students who participated in the field and data collection and were not listed in the author list.
The authors declare that the research was conducted in the absence of any commercial or financial relationships that could be construed as a potential conflict of interest.
All claims expressed in this article are solely those of the authors and do not necessarily represent those of their affiliated organizations, or those of the publisher, the editors and the reviewers. Any product that may be evaluated in this article, or claim that may be made by its manufacturer, is not guaranteed or endorsed by the publisher.
Ashraf, M. (2007). Variation in nutritional composition and growth performance of some halophytic species grown under saline conditions. Afr. J. Range Forage Sci. 24 (1), 19–23. doi:10.2989/102201107780178203
Burt, C. M., and Isbell, B. (2005). Leaching of accumulated soil salinity under drip irrigation. Trans. ASAE 48 (6), 2115–2121. doi:10.13031/2013.20097
Chhabra, R. (2004). Classification of salt-affected soils. Arid Land Res. Manag. 19 (1), 61–79. doi:10.1080/15324980590887344
Darwish, T., Atallah, T., El Moujabber, M., and Khatib, N. (2005). Salinity evolution and crop response to secondary soil salinity in two agro-climatic zones in Lebanon. Agric. Water Manag. 78 (1-2), 152–164. doi:10.1016/j.agwat.2005.04.020
Dong, S., Wan, S., Kang, Y., and Li, X. (2021). Establishing an ecological forest system of salt-tolerant plants in heavily saline wasteland using the drip-irrigation reclamation method. Agric. Water Manag. 245, 106587. doi:10.1016/j.agwat.2020.106587
Dong, S., Wang, G., Kang, Y., Ma, Q., and Wan, S. (2022). Soil water and salinity dynamics under the improved drip-irrigation scheduling for ecological restoration in the saline area of Yellow River basin. Agric. Water Manag. 264, 107255. doi:10.1016/j.agwat.2021.107255
Fan, J., Lu, X., Gu, S., and Guo, X. (2020). Improving nutrient and water use efficiencies using water-drip irrigation and fertilization technology in Northeast China. Agric. Water Manag. 241, 106352. doi:10.1016/j.agwat.2020.106352
Gu, Y. Y., Zhang, H. Y., Liang, X. Y., Fu, R., Li, M., and Chen, C. J. (2022). Effect of different biochar particle sizes together with bio-organic fertilizer on rhizosphere soil microecological environment on saline–alkali land. Front. Environ. Sci. 1156. doi:10.3389/fenvs.2022.949190
Guan, Z., Jia, Z., Zhao, Z., and You, Q. (2019). Dynamics and distribution of soil salinity under long-term mulched drip irrigation in an arid area of northwestern China. Water 11 (6), 1225. doi:10.3390/w11061225
Hou, Y., Wang, Z., Ding, H., Li, W., Wen, Y., Zhang, J., et al. (2019). Evaluation of suitable amount of water and fertilizer for mature grapes in drip irrigation in extreme arid regions. Sustainability 11 (7), 2063. doi:10.3390/su11072063
Li, Y., Li, M., Liu, H., and Qin, W. (2021). Influence of soil texture on the process of subsurface drainage in saturated-unsaturated zones. Int. J. Agric. Biol. Eng. 14 (1), 82–89. doi:10.25165/j.ijabe.20211401.5699
Lima, L. M., Bastos, M. S., Ávila, C. L., Ferreira, D. D., Casagrande, D. R., and Bernardes, T. F. (2022). Factors determining yield and nutritive value of maize for silage under tropical conditions. Grass Forage Sci. 77 (3), 201–215. doi:10.1111/gfs.12575
Liu, R., Kang, Y., Zhang, C., Pei, L., Wan, S., Jiang, S., et al. (2014). Chemical fertilizer pollution control using drip fertigation for conservation of water quality in Danjiangkou Reservoir. Nutrient Cycl. Agroecosyst. 98 (3), 295–307. doi:10.1007/s10705-014-9612-2
Northey, J., Christen, E., Ayars, J., and Jankowski, J. (2006). Occurrence and measurement of salinity stratification in shallow groundwater in the Murrumbidgee Irrigation Area, south-eastern Australia. Agric. Water Manag. 81 (1-2), 23–40. doi:10.1016/j.agwat.2005.04.003
Pimentel, D., Berger, B., Filiberto, D., Newton, M., Wolfe, B., Karabinakis, E., et al. (2004). Water resources: Agricultural and environmental issues. BioScience 54 (10), 909–918. doi:10.1641/0006-3568(2004)054[0909:wraaei]2.0.co;2
Qi, Z., Feng, H., Zhao, Y., Zhang, T., Yang, A., and Zhang, Z. (2018). Spatial distribution and simulation of soil moisture and salinity under mulched drip irrigation combined with tillage in an arid saline irrigation district, northwest China. Agric. Water Manag. 201, 219–231. doi:10.1016/j.agwat.2017.12.032
Qu, W., Tan, Y., Li, Z., Aarnoudse, E., and Tu, Q. (2020). Agricultural water use efficiency-A case study of inland-river basins in northwest China. Sustainability 12 (23), 10192. doi:10.3390/su122310192
Rajak, D., Manjunatha, M., Rajkumar, G., Hebbara, M., and Minhas, P. (2006). Comparative effects of drip and furrow irrigation on the yield and water productivity of cotton (Gossypium hirsutum L.) in a saline and waterlogged vertisol. Agric. Water Manag. 83 (1-2), 30–36. doi:10.1016/j.agwat.2005.11.005
Ren, D., Wei, B., Xu, X., Engel, B., Li, G., Huang, Q., et al. (2019). Analyzing spatiotemporal characteristics of soil salinity in arid irrigated agro-ecosystems using integrated approaches. Geoderma 356, 113935. doi:10.1016/j.geoderma.2019.113935
Rüegg, W., Richner, W., Stamp, P., and Feil, B. (1998). Accumulation of dry matter and nitrogen by minimum-tillage silage maize planted into winter cover crop residues. Eur. J. Agron. 8 (1-2), 59–69. doi:10.1016/s1161-0301(97)00013-0
Shah, S., Vervoort, R., Suweis, S., Guswa, A. J., Rinaldo, A., and Van Der Zee, S. (2011). Stochastic modeling of salt accumulation in the root zone due to capillary flux from brackish groundwater. Water Resour. Res. 47 (9). doi:10.1029/2010wr009790
Stavi, I., Thevs, N., and Priori, S. (2021). Soil salinity and sodicity in drylands: A review of causes, effects, monitoring, and restoration measures. Front. Environ. Sci. 330, 712831. doi:10.3389/fenvs.2021.712831
Su, F., Wu, J., Wang, D., Zhao, H., Wang, Y., and He, X. (2022). Moisture movement, soil salt migration, and nitrogen transformation under different irrigation conditions: Field experimental research. Chemosphere 300, 134569. doi:10.1016/j.chemosphere.2022.134569
Sun, G., Zhu, Y., Gao, Z., Yang, J., Qu, Z., Mao, W., et al. (2022). Spatiotemporal patterns and key driving factors of soil salinity in dry and wet years in an arid agricultural area with shallow groundwater table. Agriculture 12 (8), 1243. doi:10.3390/agriculture12081243
Wang, R., Kang, Y., Wan, S., Hu, W., Liu, S., Jiang, S., et al. (2012). Influence of different amounts of irrigation water on salt leaching and cotton growth under drip irrigation in an arid and saline area. Agric. Water Manag. 110, 109–117. doi:10.1016/j.agwat.2012.04.005
Wang, R., Wan, S., Kang, Y., and Dou, C. (2014). Assessment of secondary soil salinity prevention and economic benefit under different drip line placement and irrigation regime in northwest China. Agric. Water Manag. 131, 41–49. doi:10.1016/j.agwat.2013.09.011
Wang, Z., Bian, Q., Zhang, J., and Zhou, B. (2018). Optimized water and fertilizer management of mature jujube in Xinjiang arid area using drip irrigation. Water Sci. Technol. 10 (10), 1467. doi:10.3390/w10101467
White, R. E. (2005). Principles and practice of soil science: The soil as a natural resource. John Wiley & Sons.
Xiong, S., Xiong, Z., and Wang, P. (1996). Soil salinity in the irrigated area of the Yellow River in Ningxia, China. Arid Land Res. Manag. 10 (1), 95–101. doi:10.1080/15324989609381423
Zhang, T., Zhan, X., He, J., and Feng, H. (2019). Moving salts in an impermeable saline-sodic soil with drip irrigation to permit wolfberry production. Agric. Water Manag. 213, 636–645. doi:10.1016/j.agwat.2018.11.011
Zhao, X., Xia, J., Chen, W., Chen, Y., Fang, Y., and Qu, F. (2019). Transport characteristics of salt ions in soil columns planted with Tamarix chinensis under different groundwater levels. Plos One 14 (4), e0215138. doi:10.1371/journal.pone.0215138
Keywords: saline-alkali soil, soil moisture, soil salinity, drip irrigation, flood irrigation
Citation: Wang Y, Gao M, Chen H, Fu X, Wang L and Wang R (2023) Soil moisture and salinity dynamics of drip irrigation in saline-alkali soil of Yellow River basin. Front. Environ. Sci. 11:1130455. doi: 10.3389/fenvs.2023.1130455
Received: 23 December 2022; Accepted: 02 March 2023;
Published: 10 March 2023.
Edited by:
Yuncong Li, University of Florida, United StatesReviewed by:
Yiming Wang, Institute of Soil Science (CAS), ChinaCopyright © 2023 Wang, Gao, Chen, Fu, Wang and Wang. This is an open-access article distributed under the terms of the Creative Commons Attribution License (CC BY). The use, distribution or reproduction in other forums is permitted, provided the original author(s) and the copyright owner(s) are credited and that the original publication in this journal is cited, in accordance with accepted academic practice. No use, distribution or reproduction is permitted which does not comply with these terms.
*Correspondence: Rui Wang, YW13YW5ncnVpQDEyNi5jb20=
Disclaimer: All claims expressed in this article are solely those of the authors and do not necessarily represent those of their affiliated organizations, or those of the publisher, the editors and the reviewers. Any product that may be evaluated in this article or claim that may be made by its manufacturer is not guaranteed or endorsed by the publisher.
Research integrity at Frontiers
Learn more about the work of our research integrity team to safeguard the quality of each article we publish.