- School of Economics and Management, Beijing Jiaotong University, Beijing, China
In recent years, the rapid development of electric vehicles has gained widespread attention, and has especially brought new vitality to the fast-growing logistics service industry. Electric vehicles in urban logistics are not only ideal transportation tools but also do not affect the environment. This study thus implements the economic valuation of electric vehicles in urban logistics from the viewpoints of both manufacturers and business users through the investigation of pricing mechanism and discusses the potential for improving policy-making together with the real case in China. The net present value (NPV) approach is used to quantitatively analyze the investment decisions of urban logistics electric vehicles, with government regulation, incentive policy, and carbon emission trading taken into consideration. Our findings provide insights into the decision-making mechanism for commercializing electric vehicles in urban logistics that involves optimizing the government subsidy and transaction price between the manufacturers and business users. The results imply that with rationalized government policy incentives and coordinated transaction price, both manufacturers and business users are expected to achieve their break-even in limited time periods. The carbon cap placed on business users rather than manufacturers would be relatively more conducive to the marketization process.
1 Introduction
The consistent increase in energy price in international market and environmental concern leads to search alternate solution for transportation. Therefore, electrification of vehicles was essential for sustainable development of transportation. Moreover, urban transportation consumes a greater amount of fuel and energy than rural transportation due to the large number of vehicles used in urban areas (Liu et al., 2023). Therefore, it is necessary to empirically analyze the role of electric vehicles in urban transportation. Over the past decade, the development of new energy vehicles has attracted attention worldwide and has been promoted to realize an environment-friendly society and all-round sustainability. There has been a gradual acceptance of new energy vehicles such as electric passenger cars in the marketplace, which is promoted by local governments (Wan et al., 2015). Following this trend, electric vehicles in urban logistics have begun entering the market and becoming an important part of green transformation in the logistics industry (Quak et al., 2016; Strale, 2019). Electric vehicles in urban logistics are mainly used for relatively short-distance (intra-city) transportation service including branch line logistics transportation between outlets and distribution stations and the last kilometer of urban delivery logistics (Wang et al., 2019). With the rapid development of e-commerce, the express delivery industry has been penetrating into a larger number of households, creating huge demand for urban logistics service carried out by logistics electric vehicles. To achieve carbon neutrality goals, the government is pushing for the development of electric vehicles in urban logistics through incentive policies, since electric vehicles have advantages of lower electricity usage cost and less emission over the conventional fuel vehicles (Jones et al., 2019; Dean et al., 2022). However, there is an issue to be concerned regarding whether logistics service providers would prefer to use electric vehicles and whether manufacturers would be willing to invest in producing them. These will depend on whether their cost disadvantages can be mediated by the market penetration induced by government incentive policies, on their social responsibility propelled by environmental concern on energy conservation and emission reduction, and also on the market trend of gradual phasing out of conventional vehicles in the logistics industry. In China, for instance, reality reflects that there is a trend for conventional fuel vehicles to be replaced by electric vehicles in urban logistics, promoted by some large e-commercial retailers and logistics service providers such as JD.COM, Gome, SUNING.COM, and SF Express, responding to the government’s call on creating a green, low-carbon, and circular economy. The development of electric vehicles in the logistics industry is still in its initial stage. The realization of sustainable development requires the coordination of manufacturers and logistics service providers and the government’s regulation and incentive policies.
During the development of electric vehicles in urban logistics, two parties are involved, the manufacturers as suppliers and urban logistics service providers as business users. In terms of business characteristics, the market development process of electric vehicles in urban logistics is rather different from that of electric passenger vehicles, since the former is for business firms that are profit driven and the latter is for household users that are driven by usage satisfaction. The business feature of the former is characterized by not only profit drive, but also both parties are expected to comply with environmental regulations. During the process of transforming conventional fuel vehicles into electric vehicles in urban logistics, manufacturers face the challenge of cost disadvantage due to market competition, which is concerned with the achievement of its green investment break-even in an acceptable payback period (Porter, 2008). Without the government incentive policy, the cost disadvantage may drive the sales price to increase, which in turn may affect the purchase cost of the logistics service providers as the business users and makes them less motivated. In this sense, government incentives, such as subsidies, play a role in facilitating the market penetration of electric vehicles in logistics service. The marketization of electric vehicles in urban logistics can only be realized and sustained when both parties reach their break-even in an acceptable payback period. It requires the manufacturers and business users to act as partners and share government subsidies through transaction price coordination between them. Following up with the growth of sales through market penetration, government subsidies for promoting electric vehicles in urban logistics should be gradually reduced and possibly even withdrawn within a few years as scheduled. Thus, the economic feasibility of electric vehicles in urban logistics needs to be examined under different scenarios for managerial implication. In this regard, there are some issues that need to be addressed, for instance, what characters, unlike those of electric passenger cars, influence the marketability of electric vehicles in urban logistics? How can both manufacturers and logistic service providers reach break-even within limited time periods through a coordination process of transaction price determination? How can their business behaviors be effectively guided through government regulations and policy incentives to comply with environment standards? In the existing literature, limited research is dedicated to the marketization of electric vehicles in urban logistics from the perspective of logistics service providers, for whom it is necessary to consider profitability and economic feasibility. Moreover, investigation on its mechanism has both research and practical implications under government policies and carbon-trading context. The research in these aspects reflects the important innovation points of this study. There are several ways in which this paper makes contributions to the literature. First, it focuses on the economic evaluation of electric vehicles in urban logistics, which has been neglected past studies. Second, we applied the net present value approach for economic evaluation, which could provide better estimation and evaluation of electric vehicles in urban logistics. Third, we use data from China, which is among the top users of the electric vehicle, and this research provides a better understanding of the role of electric vehicles in the urban logistics. The remaining paper is organized as follows. Section 2 presents the literature review. Section 3 presents the net present value (NPV) models of both manufacturers and business users by introducing electric vehicle in urban logistics, taking into account government regulation and incentive policy, then the game approach is utilized to study their coordination process in determining the optimal transaction price, and the scenarios under the enforcement of carbon trading is also considered. Section 4 presents a numerical analysis together with sensitivity analysis based on the real case in China to illustrate the model, and the results are discussed in Section 5. The paper ends with the conclusion.
2 Literature review
2.1 Electric vehicles in urban logistics
As the current global environmental situation is gradually becoming grim and the energy problem is gradually becoming prominent, all countries in the world are paying attention to the research of electric vehicles. The large-scale promotion of the use of electric vehicles has the functions of ensuring the energy security, improving the local environment, and promoting economic development (Ahman, 2006). Marmiroli et al. (2020) compared the environmental assessment of electric and conventional light-duty vehicles. The results showed that electric vehicles have advantages in urban environments due to the large amount of stopping and regenerative breaking that occurs during urban transportation. The trend of replacing conventional fuel power with new energy power in the future automobile industry is gradually clear (Specchia et al., 2005). However, the market share of electric vehicles for urban logistics is much lower than the potential in some countries (İmre et al., 2021). To estimate the potential and possible directions of electric vehicle adoption, several scholars used the Bass diffusion model (Park et al., 2011; Lee et al., 2019; Brdulak et al., 2021). The Bass model captures the growth trend of a new product’s sales forecast. Yu et al. (2018) also used the diffusion model to assist the evaluation of policies’ effectiveness in the adoption of electric vehicles in China. Li et al. (2020) examined electric vehicles in urban logistics distribution systems under a sharing economy. They found that in the first simulation, sharing distribution networks can reduce trip times and costs. The second simulation estimated different timepoints of electricity price, which indicates that low electricity prices reduce traffic congestion and cost. Furthermore, they suggested that an increase in carbon tax reduces the CO2 emissions. Gao et al. (2022) evaluated inceptives for the adaptation electric vehicles in urban logistic, and they analyzed different factors including price, driving rate, and decision related to time window. This suggests that price and driving range of the electric vehicle motivate the consumer to adopt EV. Furthermore, wider time window also motives the consumer to adopt EV. Juvvala and Sarmah (2021) analyzed the policy electric vehicles in city logistics, and they used a real-life case study and use the MACO mathematical model test. The hypothesis found that the purchase subsidy and the zone entry fee play an important role in city logistics. Roumboutsos et al. (2014) found that electric vehicles help urban logistics significantly and improve urban terrific congestion, and they also suggested that electric vehicles reduce the pollution emissions. Based on a real-world application, Duarte et al. (2016) assessed the adequacy of electric vehicles for urban logistics in Lisbon. They compared the energy impacts of switching to electric mobility and found that the battery electric vehicles lead to low vehicle usage energy consumption. Quak et al. (2016) addressed recent advancements, challenges, and prospects for the large-scale use of electric freight vehicles in daily city logistics. Iwan et al. (2019) illustrated that electric vehicles are suitable for urban logistics by investigating the potentials of electric mobility in commercial transport in terms of travel patterns and daily mileage. Ehrler et al. (2021) analyzed a real-life case of electric vehicles for grocery logistics in Germany and argued the prerequisites and perspectives for a successful introduction of electric freight vehicles for urban logistics. Some scholars even look into the sharing economy to improve the routing model for urban logistics distribution using electric vehicles (Verena et al., 2019; Zuo et al., 2019).
2.2 Firms’ environmental behaviors and government policies
Firms should take the liability to reduce environmental externalities via green technology investments. While complying with government regulations, Yalabik and Fairchild (2011) argued that government subsidies are more effective than penalties in encouraging firms’ technology adoptions to become environment-friendly. Their findings suggest that the incentive policy is a stronger driving force for firms’ environmental innovation than the penalty policy. By using a lifecycle approach, Ding et al. (2014) drew the impact of government policies on how to motivate firms to reduce their pollution externalities by producing environment-friendly products. Ding et al. (2016) explored a decision-making mechanism for an environmentally sustainable supply chain that is jointly constrained by environmental carrying capacities and carbon caps, and also takes government policy incentives into account. They addressed the impact of government policy incentives on value transition and profit allotment in different settings of the collaborative supply chain system.
The industrialization of electric vehicles in urban logistics needs the government to issue policies on supporting the construction of infrastructure and encouraging the research and development of key technologies. Governments in different countries have taken differential policies to support measures in the development of electric vehicles, including research and development, demonstration projects, and market support (Ahman, 2006; Lebeau et al., 2016). Mirhedayatian and Yan (2018) evaluated the impacts of supporting policies for electric vehicles on company and environment. Juvvala and Sarmah (2021) carried out a real-life case study showing that car purchase subsidies and zone entry fees are important considerations when promoting electric vehicles in urban logistics. Luo et al. (2014) analyzed how the government’s subsidies for the purchase prices would affect the supply chain of new energy vehicles and proved that it is the most effective way to stimulate the sales of new energy vehicles.
2.3 Carbon trading
Carbon trading, as a market-based instrument, has been studied and used to optimize resource allocation and reduce carbon emissions. Most of the related research studies have been conducted at the macro level of country or industry, including carbon price, allocation approach of carbon emission rights, comparison of trading schemes in different countries, and the impact of emissions trading on economy and industries (Comodi et al., 2016; Kanamura, 2016; Bakhtyar et al., 2017; Zhang et al., 2017). Wang et al. (2019) proposed an ideal vehicle combination including electric vehicles and fuel vehicles to achieve low carbon emissions and distribution costs in the urban logistics network. There are only a few quantitative assessments of the impact of carbon trading and carbon emission constraint on energy-intensive firms, e.g., profits, cost transfers, innovation, and production planning (Gong and Zhou, 2013). To meet the regulatory requirements, Benjaafar et al. (2013) used relatively simple and extensive models to illustrate how to consider carbon emission in operational decisions with procurement, production, and inventory management. Toptal et al. (2014) studied the joint decision of manufacturing firms’ production planning and green technology investment under the constraint of carbon cap-and-trade policy and compared the impact of different policies on manufacturing firms’ optimal order quantity and green technology investment decision.
Existing studies of electric vehicles by and large have focused on the passenger vehicles with associated technologies, infrastructures, and government policies. The research on the marketization potential in urban electric logistics industry remains rare, combined with government policies and the carbon trading context. To fill this research gap, our study intends to explore the economic feasibility of electric vehicles in urban logistics by taking the view of both manufacturers and business users. The pricing model with an applicable government policy scheme is deduced to be compatible with the market development mechanism.
3 Modeling background and assumptions
3.1 Government policy for electric vehicles in urban logistics
It is the government’s responsibility to internalize environmental externalities by motivating business organizations to reduce emissions through regulation and incentive policies. In the logistics service industry, it is shown as the gradual replacement of conventional fuel vehicles with electric vehicles promoted by government incentive policies, leading to the marketization of electric vehicles in urban logistics. China has become world’s largest producer and seller of new energy vehicles, and world’s largest market for electric vehicles developed with policy support. With the consideration of fast development of electric vehicles in the Chinese market, promoted by government policies, we will focus our study on the reality in China. In terms of government policies in China, the purchase tax of new energy vehicles was exempted in 2014, and various related policies were subsequently issued with a clear indication to support the application of electric vehicles in urban logistics. According to the statistics of China Association of Automobile Manufacturers (CAAM), electric vehicles in urban logistics began to sprout in 2014 and achieved a blowout from 2015 to 2017, encouraged by government policies. However, the government issued a policy for electric vehicles with subsidy scheme change from 2018. Compared with previous years, the subsidy dropped sharply. Financial subsidy was reduced by 40% and over 50% in 2018 and 2019, respectively, and announced to be withdrawn in 2023, which may significantly impact the marketization of electric vehicles in urban logistics. Withdrawing subsidies is expected and will come sooner or later. The relevant issue is how to arrange the process of reducing the government subsidy so that it will be compatible with the requirement for the marketization of electric vehicles in urban logistics, leading the industry into a rational development stage in which fair market competition is compatible with low carbon emission and the internalization of environmental externalities.
3.2 Participants in the business of electric vehicles in urban logistics
3.2.1 Manufacturer: Supplier of electric vehicles in urban logistics
Suppliers of electric vehicles in urban logistics, one of the subjects in this study, are characterized by technical imperfections and immaturity at the early stage of their development of electric vehicles in urban logistics, so their initial investments and uncertainties are usually high, most likely leading to high unit costs and negative profits. To enable the production of electric vehicles in urban logistics to compete with conventional fuel vehicles in a competitive market, manufacturers are motivated by government incentive policies on one hand and enforced by environment regulation on the other hand. Along with the sales growth of the electric vehicles, the manufacturers expect to break-even in certain periods.
3.2.2 Logistics service provider: Business user of electric vehicles in urban logistics
Although electric vehicles in urban logistics have the advantage of low emissions that conventional fuel vehicles cannot match, the relative high-purchase cost and imperfect charging facilities are obstacles to their use. On the other hand, electric vehicles in urban logistics enjoy preferential incentive policies and relatively lower driving cost under the government’s promotion. Choosing between electric vehicles and conventional fuel vehicles in urban logistics has become a dilemma faced by the logistics service providers. As a business user, the usage cost of electric vehicles in urban logistics is an essential factor to consider. If its life cycle cost is lower than that of conventional logistics vehicle, electric vehicles in urban logistics will have economic advantage favored by business users. Otherwise, it will be difficult to compete in the market place.
3.3 Assumptions
Consider a supplier–buyer business relationship that involves business transactions of urban logistics vehicles between manufacturers and business users in the competitive market. For convenience, we consider all the manufacturers in the market of urban logistics vehicles as a whole, and all the business users as a whole. Manufacturing companies manufacture logistics vehicles on a made-to-order basis, and sell them directly to consumers. Similarly, logistics service providers purchase logistics vehicles for business operations. Due to the increase in the demand for logistics industry, electric vehicles are gradually replacing conventional fuel vehicles. The manufacturers are beginning to produce logistics electric vehicles to gradually replace the conventional fuel vehicles.
We assume that the production of electric logistics vehicles requires additional initial investment, resulting in a cost disadvantage compared with conventional fuel vehicles in the competitive market. To encourage the manufacturers and logistics service providers to improve their environmental performance by reducing environmental pollution, the government enforces regulation by imposing a penalty on manufacturing and using conventional fuel vehicles, and grants policy incentives to subsidize manufacturing and buying electric logistics vehicles. The government policy incentives will help reduce the cost disadvantage of the electric logistics vehicles in the marketplace.
We assume that in the competitive market, the purchase price of electric logistics vehicles for the business user is relatively similar to the market price, which may be lower than the manufacturer’s production cost of the electric logistics vehicles. The manufacturer will be compensated by the government subsidy only when it sells an electric logistics vehicle and shares the subsidy with the business users by coordinating the transaction price through negotiation. The purchase price of conventional fuel vehicles is determined by the market price.
We further assume that the electric logistics vehicles gradually replace the conventional ones in the market place, which means that the logistics service providers use both electric vehicles and conventional fuel vehicles simultaneously with an increasing usage of the former and decreasing usage of the latter. The usage costs of electric vehicles in urban logistics mainly include vehicle purchase cost and vehicle operation cost (including electricity cost, maintenance cost, insurance cost, and other expenses). Purchase cost includes purchase tax expense. We assume that purchase tax is free for electric vehicles in urban logistics, but is charged at a portion of the purchase price for conventional fuel vehicles.
Carbon trading is used to set the cap for carbon emission and then create a carbon market where emitters can buy and sell emission credits. The cap can be either placed on the source side (manufacturers) or placed on the end side (business users). However, the cap is not allowed to set for both the source side and the end side at the same time for the same industry to avoid double counting. The carbon cap is set at a much lower level than the average level of carbon emissions in producing and using logistics vehicles. Hence, emitters need to buy carbon credits to comply with the carbon cap whenever their carbon emissions exceed the cap. When discussing the scenario under carbon trading context, for convenience, we assume that the carbon cap-and-trade policy is implemented at the same time when the electric logistics vehicles are produced and used.
Regarding government policies, there are three alternatives to be considered: 1) the current implemented scheme in which the government subsidy is proportional to the production cost of electric vehicles, which is based on the fact that the manufacturer’s battery procurement cost is proportional to the production cost of electric vehicles; 2) the rationalized subsidy scheme in which the government subsidy is granted on the increment cost basis, which means the government subsidy only compensates for the additional costs incurred by the investment of electric vehicles compared with those of conventional fuel vehicles; and 3) the rationalized subsidy scheme together with the carbon cap enforced either on the manufacturers or business users. In the following section, these three schemes will be discussed.
4 Modeling the marketization of electric vehicles in urban logistics
4.1 Economic valuation
As mentioned previously, the break-even analysis of the investment of the electric vehicles in urban logistics involves two important parties, the manufacturers as suppliers and the urban logistics service providers as business users, and both are profit driven. Thus, the payback for their investments must be fully considered. The excessively long payback period of either party will cause them to give up the investments in electric vehicles in urban logistics. Only if they can achieve their break-even relatively simultaneously can there be enough driving force to make the marketization of electric vehicles in urban logistics sustainable. Hence, when studying the market development of electric vehicles in urban logistics from the viewpoints of manufacturers and business users, we need to comprehensively consider the operating conditions and payback periods of both the parties.
The investment in electric vehicles in urban logistics reduces emissions and internalizes environmental costs. Meanwhile, the internalization of environmental costs will have certain impacts on business cashflows. To make the marketization of electric vehicles in urban logistics economically feasible in the competitive market, the government imposes penalties on conventional fuel vehicles to have them bear environmental costs and increase their marginal costs. The incentive policies encourage the investment of electric vehicles in urban logistics by making up for the extra operating costs that manufacturers must pay to produce electric vehicles, thus reducing their marginal costs. It can be seen that in order to make electric vehicles in urban logistics competitive in the market and to raise the awareness of environmental protection, it is essential in the initial stage to implement government policies so that both the production and the usage of electric vehicles in urban logistics are well motivated toward their economic feasibility.
In the next section, our model formulation and analysis will be presented. For convenience, notations in Table 1 are used for defining variables and parameters.
4.2 Model analysis from the viewpoint of manufacturers producing electric vehicles in urban logistics
4.2.1 Manufacturer investment evaluation model
We first formulate an investment valuation model by the net present value (NPV) approach from the perspective of the manufacturers referring to Ding et al. (2014). It is considered that the lifecycle of the electric vehicles in urban logistics consists of the initial stage and the steady growth stage. At the initial stage of producing electric vehicles in urban logistics, the NPV of this project is negative, assuming that the sales volume of electric vehicles in urban logistics has not reached the expected level due to substantial upfront investment, business uncertainties, and lower market penetration. As the sale volume of logistics electric vehicles increases and the public gradually recognizes logistics electric vehicles driven by government incentive policies, the manufacturers’ cash flow will gradually increase until the time point t = n at which NPVm =0, which means they break-even. Beyond this point of time, electric vehicles in urban logistics come into its steady growth stage and earn economic profits. Our research will only focus on the decision issues in the initial stage.
In the early development stage, to enable the survival of electric vehicles in urban logistics in the market competition and promote its rapid spread, the government offers incentive policies in the form of subsidies (in Tet) for electric vehicles, and on the other hand, increases taxes or imposes fines (in To) on environmental pollution caused by conventional fuel vehicles. Since we assumed the electric vehicles in urban logistics will gradually replace conventional fuel vehicles, the manufacturers’ annual operating cash flows can be expressed as PetQet + Po(Qt − Qet) − [CetQet + Co(Qt − Qet)] + [TetQet − To(Qt − Qet)], and the investment valuation of producing electric vehicles in urban logistics should be based on the incremental net cash flow, which excludes the opportunity cost of only producing conventional fuel vehicles (PoQt − CoQt − ToQt). For the manufacturers, the net present value of producing electric vehicles in urban logistics during the initial stage, denoted by NPVm, can be expressed as:
4.2.2 Government subsidy model: Current vs. rationalized
In practice, it is found that manufacturers’ battery procurement cost is proportional to the production cost of electric vehicles, and is also proportional to battery capacity. The current government subsidy scheme for electric vehicles (in T’et) is based on the total capacity density of the power battery. The government subsidy is briefly proportional to the production cost of electric vehicle, which can be expressed as T’et = βCet (0 < β ≤ 1).
In principle, the government subsidy policy should compensate the cost advantage of electric vehicles in urban logistics compared with fuel vehicles, and further promote the market penetration process of electric vehicles in urban logistics. In order to do that, the government subsidy scheme should be granted on the incremental cost basis for producing electric vehicles in urban logistics. That is, the government only compensates for the additional costs incurred by investing in electric vehicles in urban logistics to be competitive relative to conventional fuel vehicles in the market place. Therefore, with the increasing sale volume of electric vehicles in urban logistics, the incremental cost of producing electric logistics vehicles per unit gradually decreases, and thus the government subsidy will also gradually decrease until it is completely withdrawn when the manufacturer reaches break-even. In this way, the government policy is more in line with reality and promotes the market penetration process of electric vehicles in urban logistics. Assuming that the government subsidy is proportional to the average increment cost of producing electric logistics vehicles, the proportion coefficient is defined by α. It reflects the variation of market demand, investment intensity, energy utilization, pollution reduction efficiency, and commercial risks of electric logistics vehicles. The formula for the rationalized government subsidy (in Tet) can be expressed as Tet = αC (0 < α ≤ 1). C is the average increment cost per unit of electric logistics vehicles, which is presented by allocating the total accumulated increment costs to the total sale volume during the initial stage. The rationalized government subsidy per unit can then be expressed as follows:
4.2.3 Electric logistics vehicles planning sales forecasting model
In order to estimate the appropriate value of government subsidies, we need to forecast the annual sales volume of electric vehicles in urban logistics based on the actual situation in China. The sales volume of electric vehicles in urban logistics theoretically undergoes a growth trend during a certain time period due to government promotion and environment regulation enforcement in the initial stage, and after that, with the withdrawal of government subsidy, the growth trend gradually slows down, and then the market reaches saturation. The dynamics of the adoption process can be explained by the Bass diffusion model (Bass, 1969), which is commonly used for market analysis and demand forecasting of new products. Therefore, we adopted the Bass diffusion model to estimate the market trend of electric vehicles in urban logistics. Its general form is as follows:
where m, p, and q are parameters. m represents the market potential of electric vehicles in urban logistics, p represents the innovation coefficient, q represents the imitation coefficient, n(t) represents the predicted annual sales in year t as Qet, and N(t) is the market holdings same as
According to the disclosed data from China Association of Automobile Manufacturers (CAAM) as shown in Table 2, the sales volume of China’s electric vehicles in urban logistics continued to rise from 2014 to 2018. However, affected by the “subsidy fraud” scandals in 2017, the actual sales in 2017 and 2018 should be less than those in the disclosed data because some manufacturers made fraudulent sales reports to seek more subsidies. Subsequently, the sharply shrinking subsidy from 2018 results in a sharp decline of the sales in 2019. When the subsidy standards stopped tightening in 2020, the sales rose again. These abnormal fluctuations in sales also reflected the problem brought by the current subsidy scheme, which is not conducive to the market development. If the government subsidy was rationalized as Eq. 2, the problem of excess initial subsidy with steep fall coming afterward would have been alleviated, and the same is true for the sales trend.
Using the Bass diffusion model, we forecast the sales volumes of the electric vehicles in urban logistics from 2022 and so on. We even the abnormal values in the existing data as mentioned previously and fit the equation model to match the trend as follows:
The predicted sales volumes of the electric vehicles in urban logistics from 2022 to 2025 are obtained from Eq. 5, and the forecasted sales trend (with smooth curve) is shown in Figure 1, where the parameters are set by m = 3,500,000, p = 0.005, and q = 0.32. Equation 5 will be used for estimating the government subsidy and the net present values of the manufacturers and business users.
Theoretically, the value of the rationalized government subsidy can then be calculated by Eq. 2. With the market promotion and sales growth of the electric vehicles in urban logistics, it is predicted that the NPV of investment of the electric logistics vehicles will gradually be close to zero from negative, and up to this point of time, the government will no longer subsidize electric logistics vehicles.
4.3 Model analysis from the view of business users of electric vehicles in urban logistics
Similarly, from the perspective of business users, the investment valuation of electric vehicles in urban logistics is also estimated in terms of the incremental difference in revenue and cost, compared with conventional fuel vehicles. For the convenience of comparison and analysis, we assume that all electric vehicles in urban logistics produced by the manufacturers are sold in the market. Regarding transportation cost measurement, we assume that it is charged in terms of the maximum allowable load carried by vehicles. Influenced by battery power consumption and charging time, the mileage of electric vehicles in urban logistics is shorter than that of conventional fuel vehicles on average. In addition, business users also bear the fine (in To) brought by conventional fuel vehicles that have not yet been replaced.
When electric vehicles in urban logistics are put into operation and begin to partially replace the conventional fuel vehicles in the market, for year t, the business users will hold electric logistics vehicles (in
4.4 Joint investment decision for electric vehicles in urban logistics
We refer to the sales price of manufacturers selling electric vehicles in urban logistics and the purchase price by business users collectively as the transaction price. As the suppliers and buyers of electric vehicles in urban logistics, both the manufacturers and the business users are facing the issue of jointly determining the optimal transaction price so that both of them can reach their break-even at relatively the same time, which is crucial to the sustainable development of electric vehicles in urban logistics market. Therefore, as the overall benefit of the integrated system including manufacturers and business users is optimum, both parties will come to collaboration only if the following holds:
These are the necessary prerequisites for a lasting collaborative relationship between the manufacturers and business users. The goal of the collaboration is to realize the unification of both individual interest and collective interest, and to finally achieve Pareto optimum. To satisfy Eqs 7, 8, the manufacturers and business users must negotiate a transaction price Pet that satisfies both parties. Since the determination of Pet goes through a negotiation process between the manufacturers and business users, we use the Rubinstein game approach (Rubinstein, 1982) to describe the bargaining process of negotiating Pet. First, we identify a feasible region that satisfies the interests of both parties. From Eqs 7, 8, we can obtain the lower and upper bounds of Pet denoted by Pe-min (from △NPVm ≥ 0) and Pe-max (from △NPVu ≥ 0), respectively, as follows:
where [Pe-min, Pe-max] is the feasible interval to find the optimal value of Pet within which the manufacturers and business users make a reasonable allocation of mutual benefits (profits). According to the Rubinstein’s game theory, for both parties to coordinate, the individual portion of shared profits under coordination must be not less than that of a non-coordination scenario. Assuming an indefinite bargaining game between the manufacturers and business users in our case, let x* (0 ≤ x* ≤ 1) be the profit share of the manufacturers and 1-x* be the profit share of the business users. The unique sub-game perfect Nash equilibrium, namely, the optimal profit sharing ratio of the manufacturer, is expressed as x* = (1-δ2)/(1-δ1δ2) (if δ1 = δ2, then x* = 1/(1+δ)), where δ1 and δ2 are the discount factors of the manufacturers and business users, respectively (here, discount factor is the patience degree of participants for 0 ≤ δ ≤ 1, which can be seen as the cost of bargain). Considering that the determination of profit sharing depends on the transaction price that satisfies both the manufacturers and business users, the ratio of the profit allotment between the two parties can then be expressed in terms of the transaction price as follows:
where Pe* denotes the optimal value of the transaction price and Pe-max and Pe-min are shown in Eqs 9, 10. Rewriting Eq. 11 with the coordination of participants, the optimal transaction price is obtained as follows:
As seen previously, the lower and upper bounds of Pet depend on the sales volume, initial investments of the manufacturers, production costs, government policies, incremental revenue, and operating cost of electric vehicles in urban logistics and also the price of fuel logistics vehicles. They have joint impacts on the determination of the optimal transaction price through the negotiation process. Given the production and sales volume and other parameters, the number of years for the manufacturers and business users to reach their break-evens can be obtained, which present their payback periods. The optimal coordinated transaction price is obtained when the game process comes to an equilibrium at which both parties reach their break-evens at relatively the same time.
4.5 Economic valuation of electric vehicles in urban logistics with carbon caps
In this section, in addition to the government subsidy offered to compensate for the cost disadvantages of electric vehicles, we further study the impact of introducing carbon trading on the economic valuation of the electric vehicles in urban logistics.
With the consideration of carbon emission and carbon cap, when introducing the electric logistics vehicles to gradually replace the conventional fuel vehicles, carbon credits for additional carbon emissions are involved. The carbon emissions at time period t (t = 1,2, … ,n) can be expressed as follows:
where (Qt − Qet) is the sales quantity of conventional fuel vehicles. Equation 13 states that the total carbon emissions in year t is the sum of the emissions associated with producing both the electric logistics vehicles and the conventional fuel vehicles, which equals to the annual carbon cap plus the purchased carbon credits from an external source in year t. Carbon emission costs incurred from purchasing carbon credits (i.e., carbon emission rights) at time period t can then be expressed as follows:
Equation 14 is derived from Eq. 13. As we assumed, the carbon cap can be either placed on the manufacturers or on the business users. Thus, we will discuss these two scenarios separately. If it is the manufacturer who participates in carbon trading, with the carbon credit purchase cost of Eq. 14 taken into consideration, we can obtain its NPV from Eq. 7 as follows:
If it is the business user who participates in carbon trading, with the carbon credit purchase cost of Eq. 14 taken into consideration, we can obtain its NPV from Eq. 8 as follows:
where the impact of the cost of purchasing carbon credits is similar to the penalty cost imposed on carbon emissions. This is true in reality because if the carbon caps were not set low enough, then firms would not actively reduce carbon emissions.
In a way similar to Section 4.4, the optimal transaction price between the manufacturer and business user can be determined through negotiation using the Rubinstein game approach. For the case of the manufacturers to participate in carbon trading, we use Eq. 15 to develop the lower bound of the transaction price as follows:
Together with the upper bound of the transaction price as shown in Eq. 10, we can determine the optimal transaction price with the carbon cap set on manufacturers. On the other hand, for the case of business users to participate in carbon trading, we use Eq. 16 to develop the upper bound of the transaction price as follows:
Together with the lower bound of the transaction price as shown in Eq. 9, we can determine the optimal transaction price with the carbon cap set on business users.
5 Numerical illustration
In this section, we conduct a case analysis based on the real data of China’s urban logistics vehicle market to demonstrate the economic valuation of electric vehicles in urban logistics and provide insight into government policies’ effectiveness. We compare the incremental cost difference between electric vehicles in urban logistics and conventional fuel vehicles, and NPV models are used to estimate the break-even time periods of the manufacturers and business users under the current market price and government subsidy mode. Then, the theoretically rationalized government subsidy coefficient and pricing formula are used to optimize the government subsidy and transaction price, and the break-even time periods are recalculated for comparison. In the similar way, the carbon trading scenario is discussed to gain insight into the effectiveness of government policies.
5.1 Data collection
Electric vehicles in urban logistics include minivan and light truck, mainly used in the fields of intra-city express and fresh food logistics. The data are estimated on average based on real market situation shown in Table 3. With the development and market penetration of the electric logistics vehicles, its variable production cost gradually reduces as the battery cost decreases with technological improvement.
5.2 Impact of current implemented government subsidy
Table 4 shows that under the current subsidy scheme and pricing, the NPVs of the electric vehicles in urban logistics for the manufacturers and business users will become positive in 2024 and 2020, respectively, i.e., the former will achieve its break-even in 10 years and the latter in 6 years. This incurs unbalanced payback periods between the two parties and will result in unwillingness from the manufacturers, implying that the current subsidy scheme may be inappropriate in a certain sense.
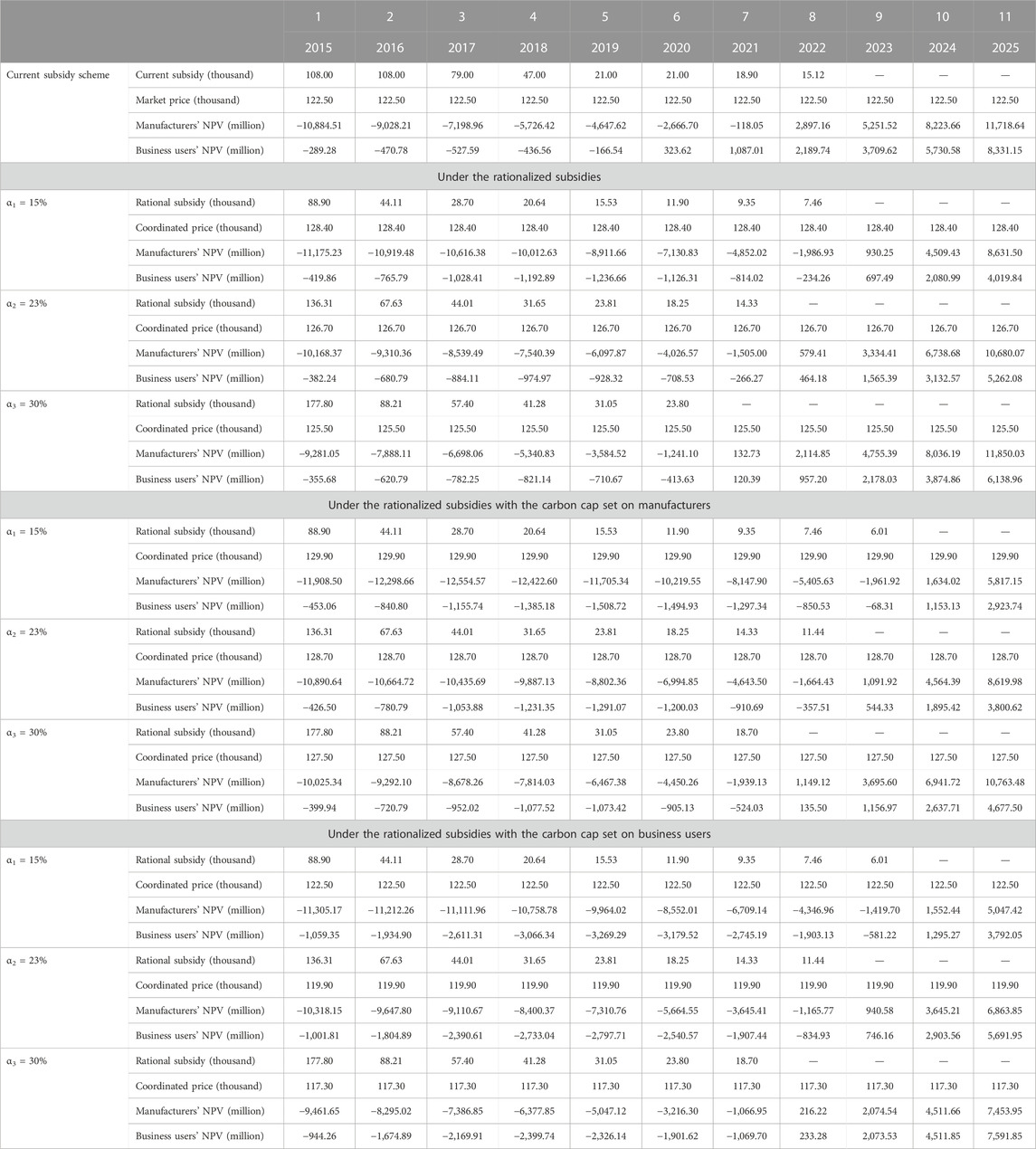
TABLE 4. Results of the NPV break-even of electric vehicles in urban logistics with different subsidy scenarios (RMB).
Under the current implemented government subsidy mode with T’et = βCet (0 < β ≤ 1), i.e., the government subsidy is proportional to the production cost of electric vehicles. Using the unit variable cost and current actual government subsidy for the electric logistics vehicles, the value of the coefficient β can be calculated to show its trend in Figure 2. The current government subsidy seems illogical since it incurs unbalance in the market due to its being directly proportional to the production cost of the electric vehicle, which cannot be compatible with the reality and does not properly consider the market competition with fuel vehicles. The initial growth trend of β indicated that the battery costs fell faster than subsidies and also meant that the subsidies were excess than needed. The delayed reaction of the government resulted in the sharp drop from 2017 to 2019. Due to the market competition, it would be unlikely for the manufacturers to make adjustment of the sales price for the subsidy decline. The loss caused by the subsidy decline basically should be covered by production cost reduction or sales increase, which needs longer time periods. Hence, this may not be acceptable by the manufacturers who have to take much longer to achieve their break-even. Noticing that it is the manufacturers that make the initial investment of producing the electric logistics vehicles by undertaking heavy upfront cost relative to the business users, this implies that the manufacturers originally take longer time to reach its break-even than the business users, which is evidenced in reality.
5.3 Rationalizing government subsidy and transaction price
From the aforementioned analysis, we can see that the current implemented government subsidy scheme needs to be rationalized to follow the market mechanism. Therefore, Eq. 2 is used to rationalize the government subsidy on the incremental cost basis, and Eq. 12 is used to coordinate the transaction price for both parties so as to ensure that both parties can reach their break-evens at relatively the same time and achieve sustainable development when the subsidy ceases. Based on the incremental cost, the value of the subsidy coefficient α is set to be less than 1 with three levels of 15%, 23%, and 30% for sensitivity analysis. The rationalized government subsidy, coordinated transaction price, and payback time periods for both parties are calculated with the collected data.
The comparison in Table 5 shows that for the rationalized scheme with α levels of 23%, the accumulated subsidies during the time periods toward the break-even are 336,000 RMB, which is rather less than that under the current implemented subsidy scheme of 418,000 RMB. Their payback periods are relatively the same, which means the manufacturer is overcompensated under the current subsidy scheme.
Under the rationalized government subsidy, Table 4 shows that both the manufacturers and business users reach their break-even at the same time in 9, 8, and 7 years at the subsidy coefficient level of 15%, 23%, improve routing for urban logistics distribution using and 30%, respectively. The investment break-even time periods are shortened with the increase of the government subsidy, which leaves the room for negotiation between business organizations and governments regarding how long is acceptable for the manufacturers and business users to break-even while facing the risks they undertake. Under the rationalized subsidy scheme with α levels of 23%, the coordinated transaction prices of the electric vehicles are 126,700 RMB, higher than 122,500 RMB under the current subsidy scheme. This implies that the reduced government subsidies compensate manufacturers by the increased transaction prices. In contrast, the higher purchase price paid by the business users prolongs their payback periods. It explains that under the rationalized scenario, the incomes associated with electric logistics vehicles are rebalanced between the manufacturers and business users.
5.4 Rationalizing government subsidy combined with the carbon cap
The impact of environmental standards on decision performance can be analyzed by observing the number of years to reach break-even changes as the carbon cap is introduced. With the carbon cap enforced on either the manufacturers or on the business users, it is expected that their payback time periods will be prolonged due to the imposed cost impact of paying for carbon credits, as shown by the case example in Table 4 that their payback time periods are extended for one more year . The total carbon emissions were 11.3 million tons in 2015 and will be 5.5 million tons in 2025, which is cut in half in 10 years for the given estimated data. We could see the positive effect brought by the marketization of electric logistic vehicles under the carbon trading context.
The government subsidy is not affected by the enforcement of the carbon cap as assumed in Eq. 2. Compared to the previous scenario not considering carbon trading, as shown in Table 6, the coordinated transaction price is higher when the carbon cap is enforced on the manufacturers due to the carbon emission costs undertaken by the manufacturers. In contrast, the coordinated transaction price is lower when the carbon cap is enforced on the business users. These can be explained by Eqs 17, 18 where the impacts of the carbon costs paid due to excess emission over the carbon cap for the manufacturers and business users are opposite to the transaction price.
6 Discussion
In our case study, the payoff of participants engaged in the production and use of electric vehicles in urban logistics to replace conventional fuel vehicles is quantitatively analyzed. Government incentives tend to last only for limited time periods and be withdrawn when the participating firms reach their break-even. Since its purpose is to let the marketization on the “right” track, investigation of how incentive policies work would help policymakers make the right decisions.
Under the current implemented subsidy and pricing model in China, the manufacturers undertaking heavy upfront investment in electric vehicles in urban logistics will take a longer time to achieve its break-even than the business users, which implies that the business users bear less risk than the manufacturers. Therefore, the manufacturers are compensated by the government subsidy when it sells an electric logistics vehicle, so they would share the subsidy with the business users by coordinating the transaction price through negotiation.
When the government subsidies are significantly reduced, the manufacturers may not be able to break-even in an acceptable time period, if the sale price of electric vehicles is unchanged. This may result in economic infeasibility to the supply side and may not be conducive to the sustainable development of the market. Thus, there is a need to negotiate the transaction price between the manufacturers and the business users to help rid the imbalance incurred by the steep drop or sharp withdrawal of the government subsidies. Also, the government subsidy scheme should also be rationalized based on economic logic compatible with the market mechanism.
Our research findings show the importance of coordination of the transaction price combining with the rationalized government subsidy scheme. When considering government policy incentives in an integrated supplier–buyer system (different from the traditional models in extant literature), the coordinated transaction price plays a core role and is interrelated with the rationalized government subsidies through the negotiation process. Compared with the current subsidy scheme, under the rationalized subsidy scheme based on the incremental cost and with the consideration of upfront investment by the manufacturers, the reduced government subsidies are given to the manufacturers by increasing the coordinated transaction price; this higher transaction price is paid by the business users, prolongs their payback periods, and thus rebalances the business performances of both parties. In this way, the unbalanced income distribution of electric logistics vehicles is redistributed between the manufacturers and business users through the rationalized government subsidy scheme together with the optimal coordinated transaction price. This explicitly proves that the effect of the rationalized government subsidies on the incremental cost basis helps rebalance the profits between the manufacturers and business users via the coordinated transaction price, and the transaction price negotiation is crucial in sustaining their business collaboration.
For a business firm in pursuit of maximizing economic benefits, its commitment to reducing carbon emissions and complying with environment standards depends on the trade-off that involves the supplier and the user. In terms of achieving sustainable economic and environmental development, business firms in a supplier–buyer system should jointly fulfill their social responsibilities of protecting the environment. In the case of commercializing electric vehicles in urban logistics, the environmental responsibilities undertaken by business organizations are embodied in the transaction price coordination between the manufacturers and business users. As more electric vehicles are produced, more carbon reductions are achieved and less carbon credit costs are paid. In practice, to make the carbon trading scheme work, the threshold of carbon emission should be low enough and the purchase price of carbon credits should be relatively uneconomic. In this way, business firms that purchase a large amount of carbon credits will face greater economic pressure, which in turn will stimulate business firms to innovate and further reduce carbon emissions. The results of this study support the findings of past studies such as those by Bi et al. (2020), Duarte et al. (2016), Li et al. (2020), and Gao et al. (2022).
7 Conclusion
In this study, we aim to investigate the marketization mechanism of electric vehicles in urban logistics with the consideration of its economic and environmental performances. From the perspectives of both the manufacturers and business users, we carry out economic evaluation through investment break-even analysis by using the net present value approach and illustrate the role of government policies (regulation, incentives, and carbon trading) in bringing the urban logistic industry to be low carbon and environment-friendly.
Our research contributions lie in the following aspects: 1) Different from the existing literature, from the viewpoints of both manufacturers and business users, we evaluate the economic feasibility of electric vehicles in urban logistics, which considers the profit-driven feature of the business user as a buyer, and thus varies from the case of electric passenger vehicles. As a notable feature of our study, by focusing on the electric logistics vehicles’ development, the distinction between buyers as business users and as passengers is identified, and this reflects that their motives and behaviors have different impacts on business transaction. 2) We address the pricing mechanism of electric vehicles in urban logistics that coordinates the transaction price between the manufacturers and the business users with government policies taken into consideration. The model proposed in this paper provides a framework for optimizing the government subsidy scheme and the transaction price of electric vehicles in urban logistics with enforcement of carbon trading system, and thus the manufacturers and the business users achieve their break-even in limited time periods and collaboratively reduce carbon emissions via investment in electric logistics vehicles production and operation. 3) By looking into the reality in China, we discuss the potential for improving policy making by analyzing the theoretical logic together with the real case, and investigating the inter-relations among market price, government policies, and production cost reduction with technology innovation, which is conducive to the policymaker making the right decision to be compatible with the market mechanism.
From the main findings, we can draw the following conclusions. 1) In order to realize the sustainable development of electric vehicles in the urban logistics market, it is important that government policies can effectively stimulate the benefit distribution of manufacturers and logistics service providers to achieve a win–win situation. 2) The government subsidy scheme should be rationalized based on economic logic compatible with the market development mechanism. 3) The impacts of the carbon emission costs paid over the carbon cap for the manufacturers and business users are opposite to the transaction price. It is relatively more conducive to the marketization process to introduce a carbon trading system to the business users rather than the manufacturers with green investment burden already. 4) Decline in government subsidies will have two-sided effects on the manufacturer, i.e., negative impact on its capital flow and possibly positive impact on driving its production cost reduction with technology innovation. 5) The manufacturers and the logistics service providers should not only actively participate in the production and usage of electric vehicles but also make compliance with environmental standards a high priority and a mandatory task and draw attention in improving their environmental performance by reducing pollutants and carbon emissions. Government regulation could have an incentive mechanism to encourage business firms to take their environmental responsibilities. This study has some limitations, for example, future studies could consider battery replacement and scrap issues from the perspective of an electric vehicle’s life cycle. Furthermore, it is also worthwhile to investigate the multi-player collaborative decision problem that includes upstream suppliers of batteries. The future studies may compare the electric vehicle for urban and rural logistics.
Data availability statement
The original contributions presented in the study are included in the article/Supplementary Material. Further inquiries can be directed to the corresponding author.
Author contributions
ZY, HD, and LC contributed to the conception and design of the study. ZY and LC collected the data. ZY, HD, and LC performed the case analysis. ZY wrote the first draft of the manuscript. All authors contributed to the manuscript revision, read, and approved the submitted version.
Funding
This research is supported by the National Natural Science Foundation of China with grant no. 71372012 and the Beijing Planning Office of Philosophy and Social Science Foundation of China with grant no. 13JGB021. ZY is grateful for the support provided by the China Scholarship Council (no. 202007090179).
Acknowledgments
The authors are most grateful to the reviewers for their constructive and helpful comments which helped to improve the work of this paper considerably.
Conflict of interest
The authors declare that the research was conducted in the absence of any commercial or financial relationships that could be construed as a potential conflict of interest.
Publisher’s note
All claims expressed in this article are solely those of the authors and do not necessarily represent those of their affiliated organizations, or those of the publisher, the editors, and the reviewers. Any product that may be evaluated in this article, or claim that may be made by its manufacturer, is not guaranteed or endorsed by the publisher.
References
Ahman, M. (2006). Government policy and the development of electric vehicles in Japan. Energy Policy 34, 433–443. doi:10.1016/j.enpol.2004.06.011
Bakhtyar, B., Fudholi, A., Hassan, K., Azam, M., Lim, C. H., Chan, N. W., et al. (2017). Review of CO2 price in Europe using feed-in tariff rates. Renew. Sustain. Energy Rev. 69, 685–691. doi:10.1016/j.rser.2016.11.146
Bass, F. M. (1969). A new product growth for model consumer durables. Manag. Sci. 15 (5), 215–227. doi:10.1287/mnsc.15.5.215
Benjaafar, S., Li, Y., and Daskin, M. (2013). Carbon footprint and the management of supply chains: Insights from simple models. IEEE Trans. Automation Sci. Eng. 10 (1), 99–116. doi:10.1109/tase.2012.2203304
Bi, K., Yang, M., Zhou, X., Zahid, L., Zhu, Y., and Sun, Z. (2020). Reducing carbon emissions from collaborative distribution: A case study of urban express in China. Environ. Sci. Pollut. Res. 27 (14), 16215–16230. doi:10.1007/s11356-020-08143-2
BrdulakChaberek, A. G., and Jagodziński., J. (2021). Bass model analysis in "crossing the chasm" in e-cars innovation diffusion scenarios. Energies 14 (11), 3216. doi:10.3390/en14113216
Comodi, G., Renzi, M., and Rossi, M. (2016). Energy efficiency improvement in oil refineries through flare gas recovery technique to meet the emission trading targets. Energy 109, 1–12. doi:10.1016/j.energy.2016.04.080
Dean, M. D., and Kockelman, K. M. (2022). Are electric vehicle targets enough? The decarbonization benefits of managed charging and second-life battery uses. Transp. Res. Rec. 2676, 03611981221082572. doi:10.1177/03611981221082572
Ding, H., He, M., and Deng, C. (2014). Lifecycle approach to assessing environmental friendly product project with internalizing environmental externality. J. Clean. Prod. 66, 128–138. doi:10.1016/j.jclepro.2013.10.018
Ding, H., Zhao, Q., An, Z., and Tang, O. (2016). Collaborative mechanism of a sustainable supply chain with environmental constraints and carbon caps. Int. J. Prod. Econ. 181, 191–207. doi:10.1016/j.ijpe.2016.03.004
Ding, H., Zhao, Q., An, Z., Xu, J., and Liu, Q. (2015). Pricing strategy of environmental sustainable supply chain with internalizing externalities. Int. J. Prod. Econ. 170, 563–575. doi:10.1016/j.ijpe.2015.05.016
Duarte, G., Rolim, C., and Baptista, P. (2016). How battery electric vehicles can contribute to sustainable urban logistics: A real-world application in lisbon, Portugal. Sustain. Energy Technol. Assessments 15, 71–78. doi:10.1016/j.seta.2016.03.006
Ehrler, V. C., Schöder, D., and Seidel, S. (2021). Challenges and perspectives for the use of electric vehicles for last mile logistics of grocery e-commerce – findings from case studies in Germany. Res. Transp. Econ. 87, 100757–100759. doi:10.1016/j.retrec.2019.100757
Gao, Y., Leng, M., Zhang, Y., and Liang, L. (2022). Incentivizing the adoption of electric vehicles in city logistics: Pricing, driving range, and usage decisions under time window policies. Int. J. Prod. Econ. 245, 108406. doi:10.1016/j.ijpe.2021.108406
Gong, X. T., and Zhou, S. X. (2013). Optimal production planning with emissions trading. Operations Res. 61 (4), 908–924. doi:10.1287/opre.2013.1189
İmre, Ş., Çelebi, D., and Koca, F. (2021). Understanding barriers and enablers of electric vehicles in urban freight transport: Addressing stakeholder needs in Turkey. Sustain. Cities Soc. 68, 102794. doi:10.1016/j.scs.2021.102794
Iwan, S., Allesch, J., Celebi, D., Kijewska, K., Hoé, M., Klauenberg, J., et al. (2019). Electric mobility in European urban freight and logistics – status and attempts of improvement. Transp. Res. Procedia 39, 112–123. doi:10.1016/j.trpro.2019.06.013
Jones, J., Genovese, A., and Tob-Ogu, A. (2019). Hydrogen vehicles in urban logistics: A total cost of ownership analysis and policy implications. Renew. Sustain. Energy Rev. 119.
Juvvala, R., and Sarmah, S. P. (2021). Evaluation of policy options supporting electric vehicles in city logistics: A case study. Sustain. Cities Soc. 74 (4), 103209. doi:10.1016/j.scs.2021.103209
Kanamura, T. (2016). Role of carbon swap trading and energy prices in price correlations and volatilities between carbon markets. Energy Econ. 54, 204–212. doi:10.1016/j.eneco.2015.10.016
Lebeau, P., Macharis, C., and Mierlo, J. (2016). Exploring the choice of battery electric vehicles in city logistics: A conjoint-based choice analysis. Transp. Res. Part E Logist. Transp. Rev. 91, 245–258. doi:10.1016/j.tre.2016.04.004
Lee, J. H., Hardman, S. J., and Tal, G. (2019). Who is buying electric vehicles in California? Characterising early adopter heterogeneity and forecasting market diffusion. Energy Res. Soc. Sci. 55, 218–226. doi:10.1016/j.erss.2019.05.011
Li, Y., Lim, M. K., Tan, Y., Lee, Y., and Tseng, M. L. (2020). Sharing economy to improve routing for urban logistics distribution using electric vehicles. Resour. Conservation Recycl. 153, 104585. doi:10.1016/j.resconrec.2019.104585
Liu, J., Li, J., Chen, Y., Lian, S., Zeng, J., Geng, M., et al. (2023). Multi-scale urban passenger transportation CO2 emission calculation platform for smart mobility management. Appl. Energy 331, 120407. doi:10.1016/j.apenergy.2022.120407
Luo, C., Leng, M., Huang, J., and Liang, L. (2014). Supply chain analysis under a price-discount incentive scheme for electric vehicles. Eur. J. Operational Res. 235, 329–333. doi:10.1016/j.ejor.2013.11.021
Marmiroli, B., Venditti, M., Dotelli, G., and Spessa, E. (2020). The transport of goods in the urban environment: A comparative life cycle assessment of electric, compressed natural gas and diesel light-duty vehicles. Appl. Energy 260, 114236. doi:10.1016/j.apenergy.2019.114236
Mirhedayatian, S. M., and Yan, S. (2018). A framework to evaluate policy options for supporting electric vehicles in urban freight transport. Transp. Res. Part D Transp. Environ. 58, 22–38. doi:10.1016/j.trd.2017.11.007
ParkKim, S. Y. J. W., and Lee, D. H. (2011). Development of a market penetration forecasting model for hydrogen fuel cell vehicles considering infrastructure and cost reduction effects. Energy Policy 39 (6), 3307–3315. doi:10.1016/j.enpol.2011.03.021
Quak, H., Nesterova, N., and Van Rooijen, T. (2016). Possibilities and barriers for using electric-powered vehicles in city logistics practice. Transp. Res. Procedia 12, 157–169. doi:10.1016/j.trpro.2016.02.055
Roumboutsos, A., Kapros, S., and Vanelslander, T. (2014). Green city logistics: Systems of Innovation to assess the potential of E-vehicles. Res. Transp. Bus. Manag. 11, 43–52. doi:10.1016/j.rtbm.2014.06.005
Rubinstein, A. (1982). Perfect equilibrium in A bargaining model. Econometrica 50 (1), 97–109. doi:10.2307/1912531
Specchia, S., Icardi, U. A., Specchia, V., and Saracco, G. (2005). Compact direct methanol fuel cells for portable applications: A modeling study. Int. J. Chem. React. Eng. 3 (1). doi:10.2202/1542-6580.1252
Strale, M. (2019). Sustainable urban logistics: What are we talking about? Transp. Res. Part A Policy Pract. 130, 745–751. doi:10.1016/j.tra.2019.10.002
Toptal, A., Ozlu, H., and Konur, D. (2014). Joint decisions on inventory replenishment and emission reduction investment under different emission regulations. Int. J. Prod. Res. 52 (1), 243–269. doi:10.1080/00207543.2013.836615
Verena, C. E., Dustin, S., and Saskia, S. (2019). Challenges and perspectives for the use of electric vehicles for last mile logistics of grocery e-commerce – findings from case studies in Germany. Res. Transp. Econ. 87, 100757. doi:10.1016/j.retrec.2019.100757
Wan, Z., Sperling, D., and Wang, Y. (2015). China’s electric car frustrations. Transp. Res. Part D Transp. Environ. 34, 116–121. doi:10.1016/j.trd.2014.10.014
Wang, J., Lim, M., Tseng, M., and Yang, Y. (2019). Promoting low carbon agenda in the urban logistics network distribution system. J. Clean. Prod. 211, 146–160. doi:10.1016/j.jclepro.2018.11.123
Yalabik, B., and Fairchild, R. J. (2011). Customer, regulatory, and competitive pressure as drivers of environmental innovation. Int. J. Prod. Econ. 131, 519–527. doi:10.1016/j.ijpe.2011.01.020
Yu, J., Yang, P., Zhang, K., Wang, F., and Miao, L. (2018). Evaluating the effect of policies and the development of charging infrastructure on electric vehicle diffusion in China. Sustainability 10 (10), 3394. doi:10.3390/su10103394
Zhang, X., Qi, T. Y., Ou, X. M., and Zhang, X. L. (2017). The role of multi-region integrated emissions trading scheme: A computable general equilibrium analysis. Appl. Energy 185, 1860–1868. doi:10.1016/j.apenergy.2015.11.092
Keywords: urban logistics (UL), electric vehicle (EV), economic valuation, government policy, environment, carbon trading (CT)
Citation: Yan Z, Ding H and Chen L (2023) The analyzing the role of electric vehicles in urban logistics: A case of China. Front. Environ. Sci. 11:1128079. doi: 10.3389/fenvs.2023.1128079
Received: 20 December 2022; Accepted: 02 February 2023;
Published: 21 February 2023.
Edited by:
Irfan Ullah, Nanjing University of Information Science and Technology, ChinaReviewed by:
Muhammad Zeeshan, Liaoning Technical University, ChinaFarman Ullah Khan, Bahria University, Pakistan
Copyright © 2023 Yan, Ding and Chen. This is an open-access article distributed under the terms of the Creative Commons Attribution License (CC BY). The use, distribution or reproduction in other forums is permitted, provided the original author(s) and the copyright owner(s) are credited and that the original publication in this journal is cited, in accordance with accepted academic practice. No use, distribution or reproduction is permitted which does not comply with these terms.
*Correspondence: Huiping Ding, aHBkaW5nQGJqdHUuZWR1LmNu