- 1College of Land Science and Technology, China Agricultural University, Beijing, China
- 2Institute of Plant Nutrition, Resources and Environment, Beijing Academy of Agriculture and Forestry Sciences, Beijing, China
- 3Beijing Engineering Technology Research Center for Slow/Controlled-Release Fertilizer, Beijing, China
- 4Dezhou Academy of Agricultural Sciences, Shandong, China
Accumulation of microplastics (MPs) in soil is a serious environmental concern. Addition of exogenous MPs can alter structure and physicochemical properties of and material transport in soil. MPs are particularly toxic to earthworms, which are soil ecosystem engineers, and exacerbate ecological risks; however, there is a lack of comprehensive and in-depth analyses of how MPs exhibit toxicity to/towards earthworms. In this study, we report a bibliometric analysis of 77 peer-reviewed papers published before December 2021 to systematically analyze how the addition of exogenous MPs contributes to earthworm toxicity and clarify the historical development and research hotspots in this field. We found that first, polyethylene and polystyrene are the most common materials used to study the toxic effects of MPs on earthworms. Second, the toxic mechanisms of MPs on earthworms mainly involve histopathological damage and oxidative stress, as well as serving as carriers of complex pollutants (e.g., heavy metals and organic pollutants) through combined adsorption–desorption. Third, oxidative stress is the typical reaction process of MPs toxicity in earthworms. When the content of MPs in soil exceeds 0.1%, earthworm growth is affected, and oxidative stress is induced, resulting in neural and DNA damage. Based on published studies, the prospects for future research on the ecological risks posed by MPs to earthworms have also been discussed. Overall, our findings help clarify the ecological risk of soil MPs toxicity to earthworms, reveal the mechanism of their toxic effects, and provide a theoretical basis for future studies focusing on establishing a healthy and ecologically sustainable soil environment.
1 Introduction
In 2004, Thompson et al. (2004) first defined plastic particles or debris in the ocean smaller than 5 mm as microplastics (MPs). According to one assessment, approximately 6.1 million tons of plastic waste was discharged into the terrestrial water environment in 2019 (OECD, 2022), and varying levels of MPs contamination have been reported in plankton, benthic organisms, and fish (Goldstein and Goodwin, 2013; Okamoto et al., 2022; Su et al., 2016). Soil is a more important “sink” for MPs than marine and freshwater environments (Li et al., 2020; Yu et al., 2021); the total amount of MPs entering the soil yearly is approximately 4–23 times greater than that entering the marine environment (Xu et al., 2020). Moreover, the distribution of MPs in soil shows significant spatial heterogeneity (Ding L. et al., 2021; Yu et al., 2021; Li J. et al., 2022). This may be related to the wide range of MPs sources, including agricultural film mulching, organic fertilizer application, sewage irrigation, atmospheric deposition, and fragmentation of larger plastics (Nizzetto et al., 2016; Magni et al., 2019; Zhou B. et al., 2020; Wu et al., 2021; Yu et al., 2021; Li S. et al., 2022; Zhang et al., 2022). Owing to their large specific surface area, MPs can act as carriers in the enrichment and migration of heavy metals and organic pollutants (e.g., pyrene) in the environment, resulting in compound pollution effects (Liu et al., 2018; Huang et al., 2021a; Cao et al., 2021; Jia et al., 2021). Once MPs enter the soil environment, they affect soil porosity, microbial diversity, community structural composition, and water-holding capacity (Wang J. et al., 2019; Pinto-Poblete et al., 2022; Wang W. et al., 2022; Zhao et al., 2022). Furthermore, the uptake of MPs by soil organisms affects digestive processes, resulting in bioaccumulation and biomagnification effects (Wang H. et al., 2019; Li M. et al., 2021; Xu & Yu, 2021; Zhao et al., 2022). Moreover, the activities of soil organisms drive the migration of MPs from the topsoil to the subsoil (Yu et al., 2019), thus increasing the risk of MPs entering groundwater (Zhang et al., 2018; Yu et al., 2020; Heinze et al., 2021).
As a common soil invertebrate, the earthworm improves soil structure and influences soil material circulation and energy flow through feeding, digestion, excretion, secretion, and burrowing. It is widely considered a soil ecosystem engineer (Liwarska-Bizukojc, 2021; Zhong et al., 2021; Boughattas et al., 2022). Earthworms are also important model organisms in ecotoxicology research, and their physiological activity and metabolic status reflect the degree of soil contamination (Bernard et al., 2015). For example, earthworms have been used in 60% of the studies investigating the biological effects of MPs on soil fauna; the commonly used earthworm species include Eisenia fetida, E. andrei, and Lumbricus terrestris (Spurgeon et al., 2005; Li M. et al., 2021). E. fetida and E. andrei are the recommended species for soil contamination studies according to international standards (OECD, 2004), owing to their high activity and reproduction rate, short life-cycle, and sensitivity to pollutants; however, they only inhabit surface soil layers rich in organic matter (Liu Y. et al., 2022; Xiao et al., 2022). Meanwhile, L. terrestris is an anecic earthworm that can excavate channels up to several meters deep, thus having a natural advantage when studying the migration of MPs in the soil profile (Yu et al., 2019; Baeza et al., 2020).
Although many studies have investigated the ecological risk of MPs toxicity to earthworms, there is a lack of systematic review of the literature. This study aimed to identify the historical development of the field and current research hotspots using the Web of Science core dataset to visualize and analyze data from the existing literature. We also highlight some shortcomings in existing research and identify future research priorities.
2 Materials and methods
2.1 Data sources
We retrieved relevant papers using the search terms {“microplastics”} and {“earthworm”} in the Web of Science core set database. We only retained documents of the “ARTICLE” type to limit our search to peer-reviewed original papers; there were no restrictions on language, data category, and publication year. After filtering, we obtained 77 records, including complete records and citation files in the “.txt” format, published between 2016 and 2021.
2.2 Data visualization and analysis
Statistical analysis and visualization of the literature were performed using the R language in the bibliometric package (version 3.2.1) and VOSviewer (version 1.6.17), respectively, and the keywords and co-cited references of the published literature were analyzed by mapping social networks (Small, 1973). In bibliometric research, these techniques can directly reflect research hotspots based on two-dimensional graphics (Liu J. et al., 2022). Specifically, we used the VOSviewer to perform a cluster analysis and create a social network map, in which the size of the nodes represents the frequency of occurrence, and the thickness of the lines between the nodes indicates the strength of the association (Zhang et al., 2020). Co-word analysis was also used to visualize the correlation and development between different research themes using the bibliometric data. Summary statistics of the highly cited literature were calculated using the MS Excel software, which was also to identify the current research hotspots and trends in research on MPs–earthworm interactions in soil.
3 Results
3.1 Current research hotspots
Highly cited papers reflect the hotspots, experimental methods, and trends in the research field, and the top 10 most frequently cited papers (nine research papers and one review) are listed in Table 1. The nine research papers were all conducted as simulated exposure experiments, with food and environmental exposure as the two most prominent experimental variables. Of these studies, 50% used low-density polyethylene (LDPE); the MP particle sizes typically ranged from 50 to 1,000 μm, the MPs exposure dose ranged from 0 to 2,000 mg/kg, and the earthworm species used were E. fetida and L. terrestris.
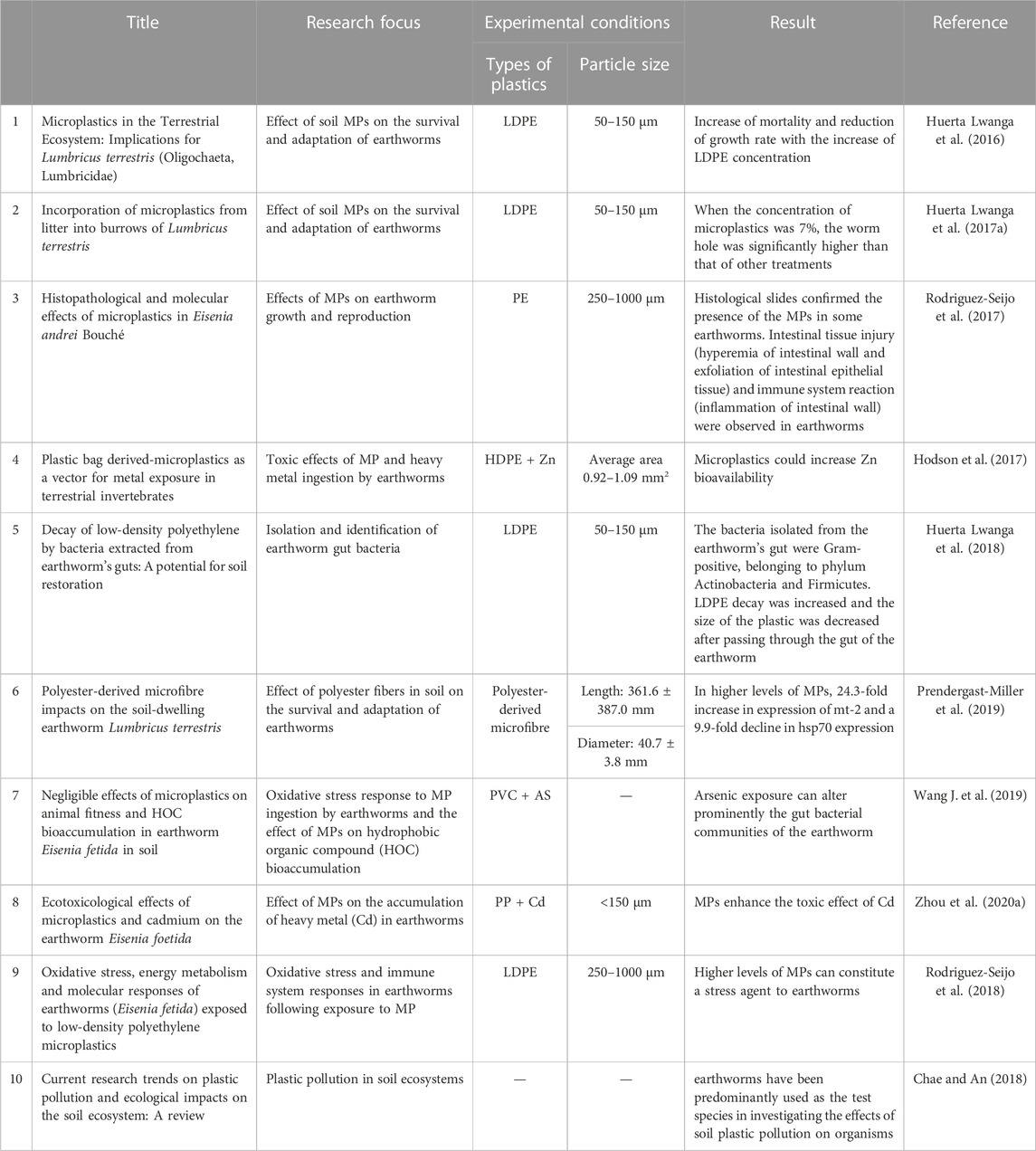
TABLE 1. Highly cited literature on the interactions between microplastics (MPs) and earthworms (published up to December 2021).
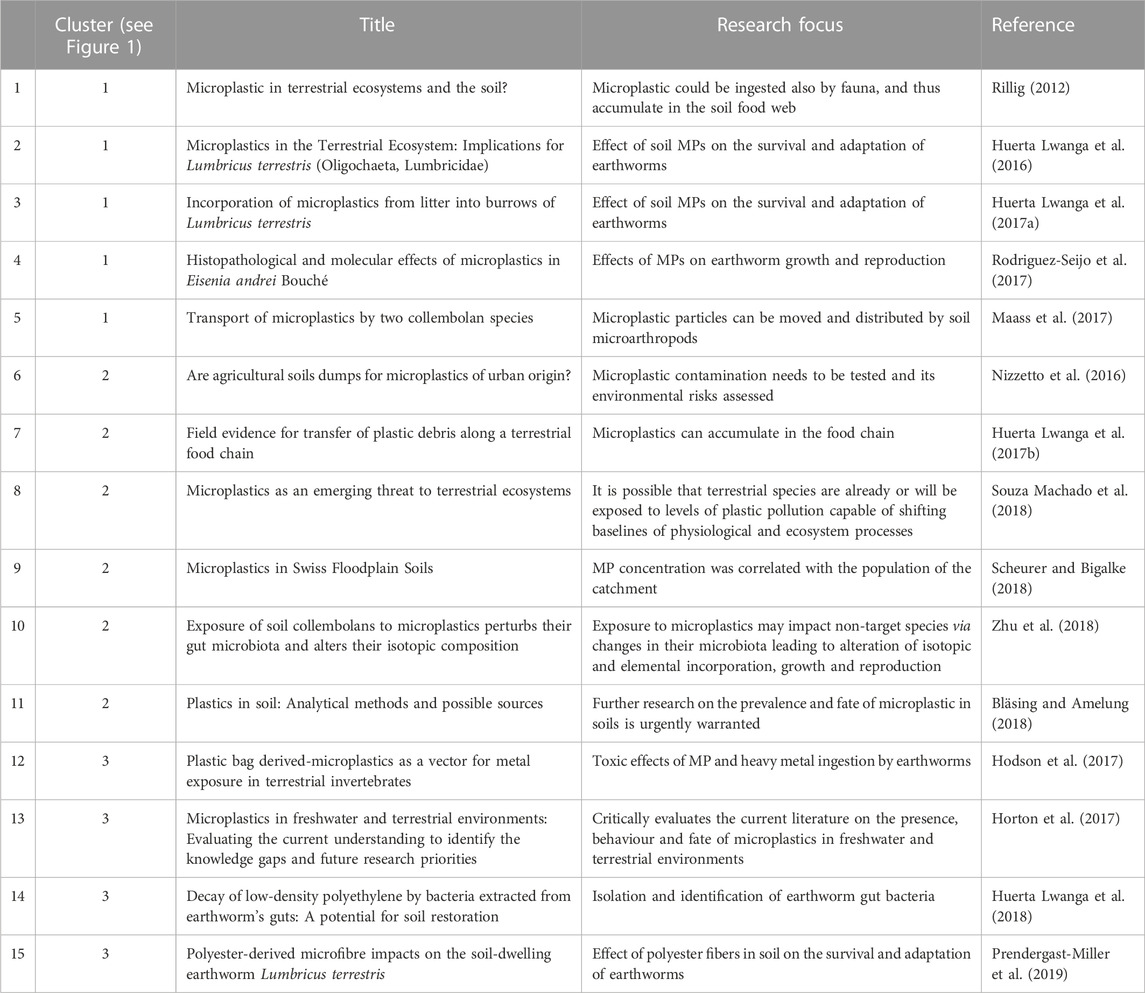
TABLE 2. Co-cited literature on the interactions between microplastics (MPs) and earthworms (published up to December 2021).
The main mechanism by which earthworms were harmed by MPs is ingestion, with a notable focus on its effects on earthworm growth (Huerta Lwanga et al., 2016; Huerta Lwanga et al., 2017a). The ingestion of MPs by earthworms affects the earthworm gut bacterial community (Wang H. et al., 2019), and earthworm gut bacteria can promote plastic degradation (Huerta Lwanga et al., 2018), thereby increasing the risk of MPs entering the groundwater (Zhang et al., 2018; Yu et al., 2020; Heinze et al., 2021). Ingestion of MPs may cause mechanical damage to the digestive organisms in earthworms, and Fourier-transform infrared-attenuated total reflectance (FTIR-ATR) spectroscopy and intestinal pathology analyses indicate that MPs can damage the intestinal tract of earthworms (Rodriguez-Seijo et al., 2017). MPs also stimulate oxidative stress in earthworms and reduce the stability of the cellular lysosomal system. Higher protein and lipid contents have also been noted in earthworms following the ingestion of MPs. Following MPs ingestion, various enzymes may be produced as a stress-response mechanism of the immune system, while on the other hand, earthworms may experience an increase in saturated fatty acids to reduce sensitivity to free radicals (Rodriguez-Seijo et al., 2018).
MPs have a large number of functional groups on their surfaces that can adsorb and immobilize heavy metals through complexation and electrostatic adsorption, respectively. Therefore, MPs may act as carriers that increase the accumulation of heavy metals in earthworms, reduce their growth rate, and increase their mortality, while also causing oxidative stress (Hodson et al., 2017; Prendergast-Miller et al., 2019; Zhou et al., 2020b). Owing to their different properties, MPs of different polymer types may enhance or reduce the damage caused by organic pollutants to earthworms (Wang J. et al., 2019; Xu et al., 2022). For example, non-polar polyethylene and polypropylene do not contain specific functional groups, and van der Waals forces play a major role in the adsorption of organic pollutants. Polystyrene can interact with aromatic compounds (e.g., polychlorinated biphenyls) via π-bonding, resulting in the stronger adsorption of organic matter (Ballent et al., 2016; Mei et al., 2020). Wang J. et al. (2019) found that MPs reduced the effects of hydrophobic organic compounds (HOCs) on earthworms through competitive sorption.
Existing studies have mostly used simulated experimental conditions. Some of these studies have used much higher MPs exposure doses than those found in natural soil to facilitate the observation of their impacts on earthworms. This has, however, limited the applicability of experimental findings to the natural environment. Moreover, the natural soil environment is often more complex than the soils used in experiments, and factors such as temperature, pH, and salinity can impact MPs adsorption, which remains understudied. The accumulation and biological effects of organic pollutants and heavy metals in earthworms can be altered by MPs through the adsorption-desorption process; however, we identified only three studies on this in the highly cited literature (Hodson et al., 2017; Wang H. et al., 2019; Zhou et al., 2020c).
3.2 Co-citation analysis
Co-citation refers to the frequency of two papers being cited simultaneously (Small, 1973), which reflects the relevance of research content and is used as the basis for cluster analysis. As shown in Figure 1, the 15 publications cited more than 20 times formed a co-citation map. These publications were grouped into three clusters. Within the first cluster (red in Figure 1), Rillig (2012) suggested that it is necessary to investigate MPs contamination in soil and explore the effects of MPs on soil function and biodiversity. The growth rate of earthworms is affected by the MPs content of the soil (Huerta Lwanga et al., 2016; Huerta Lwanga et al., 2017b). In the second cluster (green in Figure 1), articles focused on the MPs content, sources, and transport pathways in the soil. For example, Souza Machado et al. (2018) found that soil MPs content is associated with the number of people living near the sampling site, and Huerta Lwanga et al. (2017a) showed that MPs can be enriched through the soil–earthworm–chicken food chain. Within the third cluster (blue in Figure 1), Horton et al. (2017) performed a quantitative study to evaluate the single and compound effects of MPs in soil and reported that MPs can increase the accumulation of heavy metals in earthworms, reduce their growth rate, and increase their mortality.
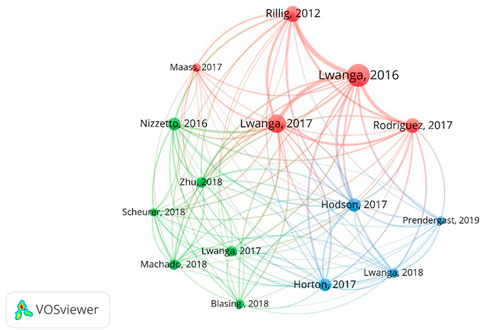
FIGURE 1. Co-citation map of studies published until December 2021 focusing on the interactions between microplastics and earthworms. The size of the nodes indicates the frequency of occurrence, and the thickness of the lines between the nodes indicates the strength of the association. Three main clusters were identified: Cluster 1, red; Cluster 2, green; and Cluster 3, blue (see also Table 2).
3.3 Keyword co-occurrence analysis
Keywords are used to reflect the core content of a study, and co-occurrence analysis of keywords is commonly used in bibliometric studies to clarify research hotspots (Zhang et al., 2020). In this study, 49 keywords were obtained, of which 34 occurred at least five times (Figure 2). In our keyword co-occurrence mapping, polyethylene and polystyrene were the two most representative MPs types in studies investigating toxicity to earthworms. Centrality measures the degree to which a particular node lies on the shortest path between the other nodes. A higher centrality value indicates a stronger influence of a specific node. The top 10 keywords for centrality were “microplastics” (centrality = 1.00, 66 occurrences), “soil” (0.97, 28 occurrences), “pollution” (0.94, 30 occurrences), “earthworm” (0.88, 31 occurrences), “exposure” (0.90, 27 occurrences), “accumulation” (0.90, 27 occurrences), “toxicity” (0.90, 22 occurrences), “oxidative stress” (0.72, 13 occurrences), “ecosystem” (0.63, 9 occurrences), and “ingestion” (0.58, 10 occurrences). MPs have become an emerging pollutant in soil ecosystems, and most studies have focused on the toxic effects on earthworms following MPs ingestion; the mechanism of toxicity is induction of oxidative stress, which is consistent with the results discussed in Section 3.1. Figure 2 also shows that research in this field draws from a range of disciplines. For example, within engineering, hot topics of research include adsorption and sorption, with respect to compound pollution caused by MPs and other contaminants; in metabolomics, hot topics include DNA (i.e., DNA damage); and in microbiology, hot topics include the gut microbiota.
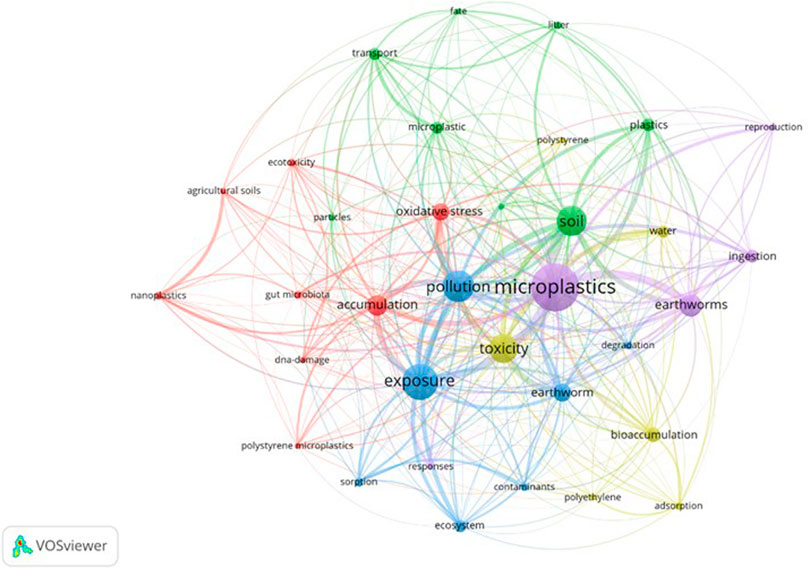
FIGURE 2. Keyword co-occurrence map of studies published until December 2021 focusing on the interactions between microplastics and earthworms. The size of the nodes indicates the frequency of occurrence, and the thickness of the lines between the nodes indicates the strength of the association.
3.4 Keyword co-word analysis
Keyword co-word analysis clusters keywords using multiple correspondence analysis methods, which show the connections between keywords in the form of a two-dimensional graph; the closer the connections between keywords, the closer their positions are in the graph. The keyword classification results are shown in Figure 3 and are divided into two clusters. Principal components 1 (Dim1) and 2 (Dim2) explained 24.70% and 19.04% of the total variance, respectively, with a cumulative explanation of 43.74%. Cluster 1 (blue in Figure 3) consists mainly of “Nile red” (a common dye used for the quantitative counting of MPs using fluorometric methods), “contaminants and pollutants,” “hydrophobic organic chemicals,” “sorption,” and “desorption.” The keywords in cluster 1 reflect a focus on the influence of the adsorption-desorption properties of MPs on the accumulation and bioavailability of HOCs in earthworms. Cluster 2 (red in Figure 3) includes “exposure,” “toxicity,” “accumulation,” “ecosystem,” and “damage,” representing studies primarily focused on the hazards to earthworms following exposure to MPs.
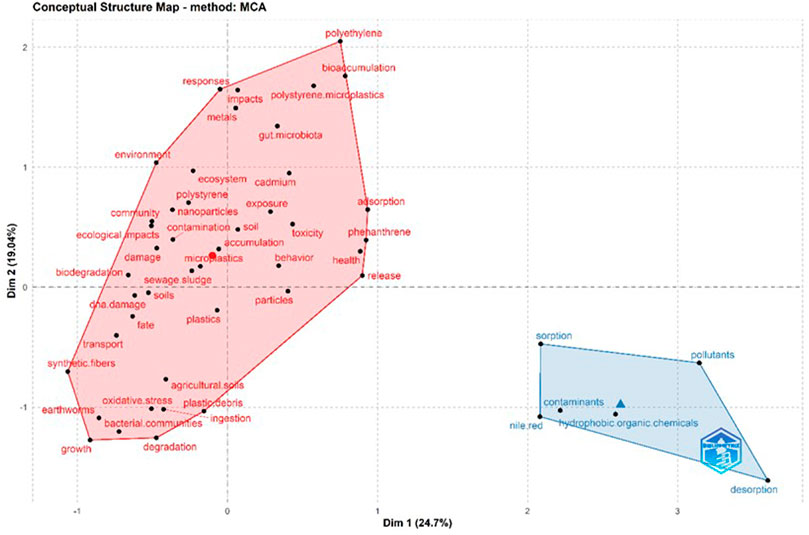
FIGURE 3. Keyword concept structure map. Two main clusters were identified: Cluster 1, blue and Cluster 2, red.
With further analysis of the co-word analysis results, we identified the inter-relationship of the research directions in this field (Figure 4). The horizontal axis in Figure 4 indicates the degree of relevance (centrality) as a measure of the importance of a research direction in the development of the entire research field. The vertical axis represents the degree of development (density), which is a measure of the current state of development of a research direction. Based on the degree of relevance and development in the field, the research directions within the current literature can be divided into the following four categories.
1. Motor themes (upper right quadrant in Figure 4). The research directions in this quadrant are well developed and have strong relevance to the whole field. The main research direction in this quadrant included the keywords “oxidative stress,” “DNA damage,” “agricultural soils,” and “comet assay.” This includes evidence of oxidative stress and DNA damage in earthworms following the ingestion of MPs, which corresponds with the keyword co-occurrence results, an understanding of which can be explained at the molecular level via metabolomics analyses (Li B. et al., 2021).
2. Niche themes (upper left quadrant in Figure 4). The research directions in this quadrant show a strong state of development, but are considered less important and more marginal to the wider field. The first theme in this quadrant is “dissolved organic matter” (DOM), reflecting a focus on the sorption and desorption of DOM by MPs. Importantly, DOM can enhance the adsorption of heavy metals by MPs and increase the bio-effectiveness of heavy metals in the earthworm gut (Zhou B. et al., 2020). The second theme includes “pesticides” and “chlorpyrifos,” indicating a focus on the compound effect of MPs and pesticides on earthworms, with some studies showing that MPs can significantly enhance the toxicity of pesticides (Cheng et al., 2020).
3. Emerging or declining themes (lower left quadrant in Figure 4). The research directions located in this quadrant are neither well developed nor marginal within the overall field of research. No keywords were positioned in this quadrant.
4. Basic themes (lower right quadrant in Figure 4). These themes represent the basic research directions of the whole field, with five keyword clusters identified. The first cluster includes the keywords “ingestion,” “earthworms,” “degradation,” “growth,” “bacterial communities,” and “avoidance behavior.” This direction of research is concerned with the negative effects of MPs ingestion on earthworms, often involving the study of the changes in the biomass and behavior of earthworms during experimental treatments (Ding W. et al., 2021). The second cluster includes the keywords “contaminants and pollutants,” “sorption,” “hydrophobic organic chemicals,” “desorption,” and “Nile.” This is consistent with Cluster 1 in the co-word analysis (Section 3.4), reflecting a focus on the effect of MPs adsorption-desorption properties on the accumulation and bio-effectiveness of HOCs in earthworms (Gong et al., 2019). The third cluster is formed by the keywords “exposure,” “soil,” “toxicity,” “accumulation,” and “bioaccumulation,” reflecting a focus on the effects of MPs on the accumulation of heavy metals in earthworms. For example, the concentration of MPs to which earthworms are exposed can influence heavy metals accumulation and bio-effectiveness, often resulting in a metabolic imbalance (Xu B. et al., 2021). The fourth cluster is formed by the keywords “transport,” “particles,” “biodegradation,” “environment,” “fate,” and “gut microbiota,” indicating a focus on the effects of MPs on the gut flora of earthworms. For example, MPs can change the composition of earthworm gut flora and affect metabolic activity by altering the biological effectiveness of heavy metals and organic pollutants (Yang et al., 2022). Lastly, the fifth cluster in this quadrant is formed by the keywords “plastics,” “release,” “behavior,” “health,” and “retention.” These keywords indicate a focus on the effects of plasticizer release from MPs on earthworms (Li et al., 2019). Notably, as different plasticizers can be added during MPs production (Li S. et al., 2021) and the factors affecting the leaching of plasticizers are not yet clear (e.g., MPs shape and particle size), more work is needed to examine the compound effects of MPs and plasticizers on earthworms.
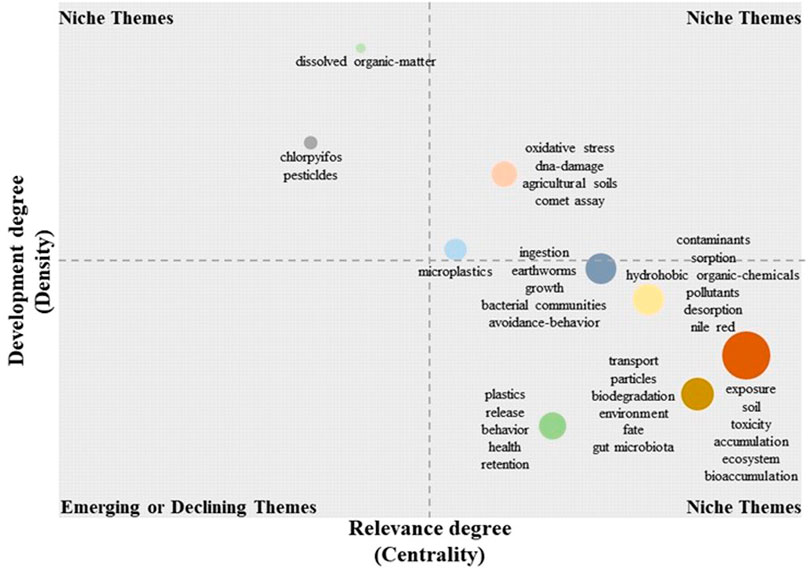
FIGURE 4. Keyword clustering of articles published until December 2021 focusing on the interactions between microplastics and earthworms. The minimum frequency of occurrence (per 1,000 documents) for each research direction is more than five. The size of the circles represents the number of articles published in the corresponding direction. The horizontal axis represents the relevance (centrality), which measures the importance of a research direction in the overall development of the research field. The vertical axis represents development (density), which measures the current state of development of a research direction. Research directions can be divided into the following categories: motor themes in the upper right corner (quadrant 1), niche themes in the upper left corner (quadrant 2), emerging or declining themes in the lower left corner (quadrant 3), and basic themes in the lower right corner (quadrant 4).
3.5 Temporal trends in keywords
Based on the results of the keyword co-word analysis, research within this field was divided into two main categories—the influence of MPs adsorption-desorption properties on the accumulation and bio-effectiveness of other contaminants in earthworms, and the toxic effects of MPs ingested by earthworms. To examine changes within the field over time, the use of keywords was examined across successive 2-year intervals. Overall, the number and range of keywords have increased over time. For example, in 2016–2017, the field was in its infancy, with fewer keywords mainly focusing on the toxic effects of MPs accumulation in the bodies of earthworms after ingestion (Figure 5). During this period, there were no keywords relating to the adsorption-desorption characteristics of MPs, indicating that this theme was not attracting much research attention. A notable increase in the number of keywords occurred during 2018–2019 (Figure 6). In addition to a focus on MPs accumulation in earthworms as well as their effects on MPs migration within soils, attention started to be given to the effects of MPs on earthworm growth and the damage associated with MPs ingestion. Other research directions emerging during this period included the effect of earthworm ingestion on intestinal flora, the toxicity of nano-MPs, and the adsorption properties of MPs. Between 2020 and 2021, the number of keywords increased in all research directions, with studies becoming increasingly more specific (Figure 7). In addition to the traditional research directions, keywords relating to oxidative stress and biomarkers appeared for the first time alongside a growing focus on the influence of the sorption and desorption properties of MPs on HOCs. These research themes are not only well developed, but also have high importance for the entire research field, constituting the dominant research direction for the last 2 years.
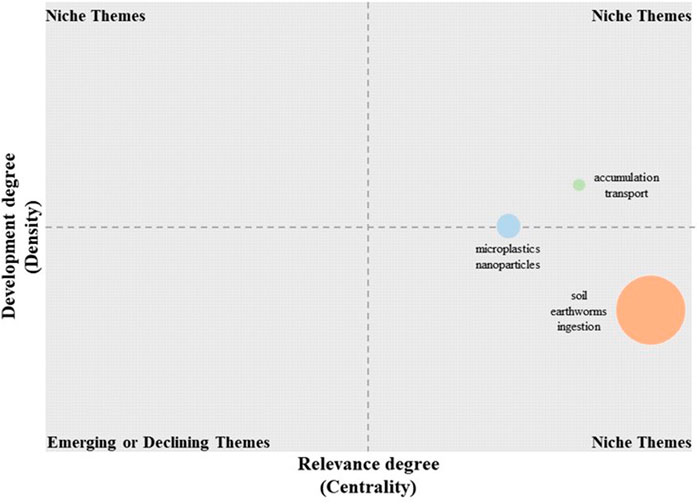
FIGURE 5. Keyword clustering of articles published from 2016 to 2017 focusing on microplastics and earthworms. The minimum frequency of occurrence (per 1,000 documents) for each research direction is more than five. The size of the circles represents the number of articles published in the corresponding direction. The horizontal axis represents relevance (centrality), which measures the importance of a research direction in the overall development of the research field. The vertical axis represents development (density), which measures the current state of development of a research direction.
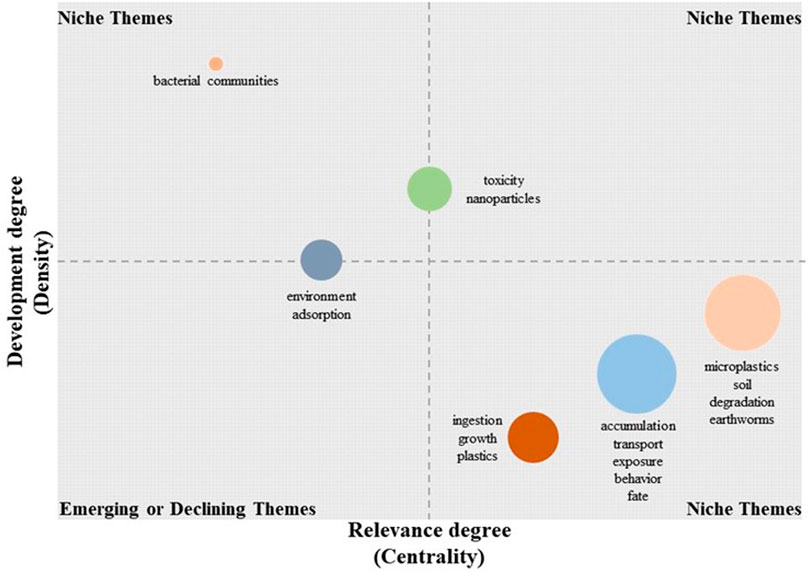
FIGURE 6. Keyword clustering of articles published from 2018 to 2019 focusing on microplastics and earthworms. The minimum frequency of occurrence (per 1,000 documents) for each research direction is more than five. The size of the circles represents the number of articles published in the corresponding direction. The horizontal axis represents relevance (centrality), which measures the importance of a research direction in the overall development of the research field. The vertical axis represents development (density), which measures the current state of development of a research direction.
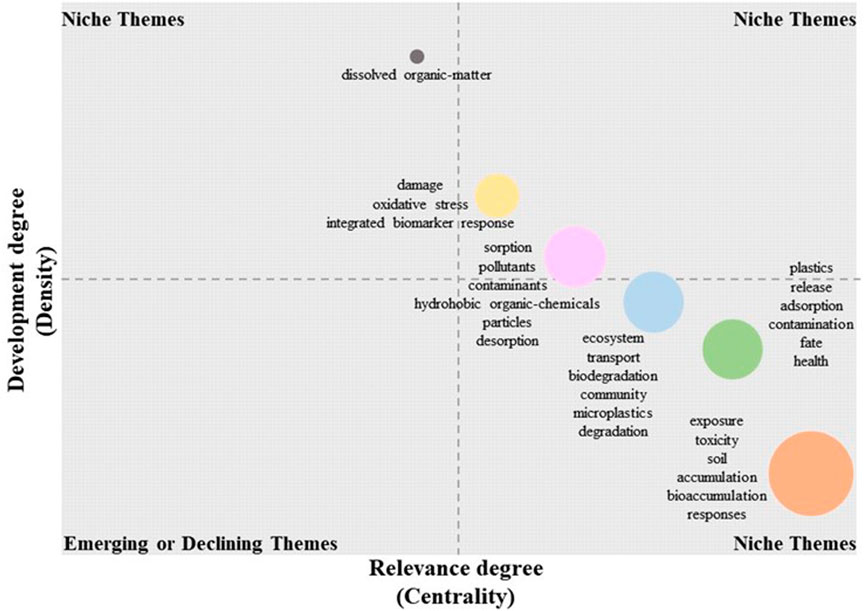
FIGURE 7. Keyword clustering of articles published from 2020 to 2021 focusing on microplastics and earthworms. The minimum frequency of occurrence (per 1,000 documents) for each research direction is more than five. The size of the circles represents the number of articles published in the corresponding direction. The horizontal axis represents relevance (centrality), which measures the importance of a research direction in the overall development of the research field. The vertical axis represents development (density), which measures the current state of development of a research direction.
4 Discussion
4.1 Toxic effects of MPs on earthworms
According to our analysis of highly cited articles and the keywords, MPs can cause toxicological effects on the digestive and metabolic systems of earthworms in the following ways.
(1) Earthworm mortality. Lower MPs content of soil corresponds to longer survival time of inhabiting earthworms. Huerta Lwanga et al. (2016) found that earthworm growth was significantly lower in 28%, 45%, and 60% polyethylene treatments compared to a 7% treatment and a blank control (0%), and mortality rates were correspondingly higher in all high-concentration treatment groups after 60 days. In contrast, Rodriguez-Seijo et al. (2017) found no significant difference in earthworm mortality among treatments after exposure to 62.5–1,000 mg kg−1 of polyethylene MPs, with generally no effect on growth when the MPs content was less than 0.1% w/w. Prendergast-Miller et al. (2019) reached a similar conclusion after exposing earthworms to a polyester microfiber environment (0.1% w/w) for 35 days. In addition, the toxic effects of MPs on earthworms are closely related to the type, size, and shape of MPs, and experimental conditions should also be comprehensively considered in subsequent experiments.
(2) Histopathological damage. MPs can cause histopathological damage to earthworms both in vivo and in vitro. Soil MPs can adhere to the surfaces of earthworms as they burrow. For example, Chen et al. (2020) observed bristle wounds and epidermal damage after 28 days of exposure to low-density polyethylene (1.5 g kg−1, <400 μm). MPs can cause histopathological damage to the digestive system of earthworms. For example, Rodriguez-Seijo et al. (2018) found that earthworms exposed to MPs (polyethylene <1 g kg−1, 25–1,000 μm) for 28 days showed significant intestinal tissue damage including the loss of intestinal epithelial tissue, congestion, and signs of inflammation in the intestinal wall.
(3) Oxidative stress response. The oxidative stress response is one of the hot topics of current research, in which MPs can affect the antioxidant system of earthworms and cause changes in antioxidant enzyme activity. After MPs ingestion by earthworms, the antioxidant system functions in three stages. The first stage is a rapid increase and excessive accumulation of reactive oxygen species—an indicator of oxidative stress—after the contaminant enters the body, which can result in oxidative damage. In the second stage, antioxidant enzyme activity (e.g., glutathione synthetase, GSH) and antioxidant molecules including superoxide dismutase, catalase, and glutathione scavenge reactive oxygen species. For example, Jiang et al. (2022) demonstrated that the toxic effects of polystyrene MPs on earthworms resulted in oxidative stress associated with altered activities of glutathione and superoxide dismutase. In the third stage, when oxidative stress exceeds the earthworm’s antioxidant capacity, increased malondialdehyde levels are associated with neurological damage. For example, earthworms exposed to LDPE MPs (particle size <400 μm) for 28 days were subjected to remarkable oxidative stress, with significant increases in catalase and malondialdehyde levels (Chen et al., 2020).
(4) DNA damage. The ingestion of MPs can cause DNA damage in earthworms. For example, using single-cell gel electrophoresis (comet assay), Jiang et al. (2022) found that earthworms exposed to polystyrene MPs showed higher levels of DNA damage, especially in a group treated with large particles and at high concentrations; Prendergast-Miller et al. (2019) found similar results, where environmental exposure to MPs (1% w/w) resulted in abnormal expression of the stress biomarker mt-2 and hsp70 genes, suggesting that the accumulation of reactive oxygen species may be the reason for abnormal gene expression in earthworms.
4.2 Combined toxicity of MPs with other pollutants
In addition to their toxicity, the small particle size, large specific surface area, and surface hydrophobicity of MPs meant that they can easily adsorb heavy metals and organic pollutants from the soil, thereby producing a compounding effect on earthworms (Hodson et al., 2017). For example, Huang et al. (2021a) experimentally demonstrated that MPs increase the accumulation of Cd in earthworms, and another study showed that co-exposure to MPs and Cd (>300 mg kg−1) for 42 days resulted in a higher mortality and significant increase in Cd accumulation in earthworms (Zhou et al., 2020b). Although some studies showed that MPs can also increase the bio-effectiveness of heavy metals in earthworms, some have drawn the opposite conclusion. MPs in the earthworm gut can reduce the accumulation and bio-effectiveness of As via adsorption (Wang J. et al., 2019). Furthermore, aging MPs adsorbed more heavy metals, probably in association with a higher number of oxidation-binding sites on their surfaces. Nevertheless, studies on the sorption-desorption properties of heavy metals by MPs remain limited; thus, further work is needed to better explain the complex and combined effects of MPs on earthworms.
MPs also have good adsorption capacities, with respect to organic pollutants, such as polycyclic aromatic hydrocarbons and polychlorinated biphenyls. Rochman et al. (2013) reported that the adsorption–desorption properties of different MPs were significantly different, with the relative order of polycyclic aromatic hydrocarbon adsorption as follows: high-density polyethylene > LDPE > polypropylene (PP) > polyvinyl chloride (PVC). Thereby, MPs can alter the accumulation and bio-effectiveness of organic pollutants in earthworms through sorption and release processes (Xu et al., 2019; Vieira et al., 2021; Xu G. et al., 2021; Xiang et al., 2022). This is reflected by higher levels of pyrene and phenanthrene residues in earthworms in MPs-contaminated soils (Xu G. et al., 2021; Liu Y. et al., 2022). Cheng et al. (2020) also observed that the combined exposure of LDPE and atrazine resulted in higher levels of atrazine in earthworms than their single exposures. Sun et al. (2021) obtained similar results for the combined exposure of earthworms using MPs and dufulin. However, MPs did not always enhance the bio-efficacy of other contaminants in earthworms. For example, Wang J. et al. (2019) found that MPs reduced the effects of organic pollutants (HOCs) via competitive sorption. The release of plasticizers from MPs can also have toxic effects on earthworms. Gaylor et al. (2013) reported that polybrominated diphenyl ether can accumulate in earthworms after leaching from polyurethane foam particles, while Li et al. (2019) found that hexabromocyclododecanes can reach concentrations of up to 307–371 ng g−1 in earthworms after 28 days of exposure to MPs.
Despite these advances, the enhancing and inhibiting effects of MPs on other contaminants in earthworms need to be further investigated under different exposure scenarios. Indeed, earthworms may ingest MPs and other adsorbed contaminants (Yang et al., 2006; Wang H. et al., 2019; Yang et al., 2019). In addition, MPs particle size, degree of aging, and external environmental conditions (temperature, pH, and salinity) can affect the adsorption–desorption processes, subsequently influencing the accumulation and bio-effectiveness of contaminants in earthworms (Liu et al., 2019; Wang Y. et al., 2022; Zhou et al., 2022).
5 Research prospects
Owing to the complexity of the soil environment and specificity of MPs, the ecological risk of MPs toxicity to earthworms requires further research. Based on our bibliometric analysis, the following research directions have been identified.
First, most experiments have used polyethylene and/or polystyrene, often employing one particle size only. However, the toxic effects of MPs on earthworms may be influenced by the type, size, and shape of the MPs used. Therefore, the toxic effects of multiple plastics need to be assessed, alongside the type, size, and shape of MPs. In addition, aging microplastics have stronger adsorption capacity and toxicity than original microplastics, thus requiring special attention from researchers.
Second, at present, most studies have used relatively high concentrations of MPs to observe their effects on earthworms under simulated experimental conditions. To better reflect natural soil conditions, representative plots should be selected for in situ tests in the field; alternatively, indoor tests with the representative MPs concentrations could be established. The influence of different types of earthworms on soil properties should also be considered. Most experiments to date have used E. fetida, an epigeic earthworm feeds on residues at the soil surface and does not generally enter the soil to feed. It also remains unclear whether this species feeds on MPs under natural conditions. In comparison, anecic earthworms dig vertical channels from the deep soil to the surface, mixing organic residues with soil in the gut and excreting them at the soil surface. This may affect the migration of MPs within the soil profile and towards waterbodies. Moreover, endogeic earthworms, which inhabit feed on soil organic matter within the soil interior, remain vastly understudied in this context.
Third, it is necessary to further consider the effects of the differences in MPs exposure time and scenarios. Although the exposure times of simulated experiments are often short, generally less than 2 months, MPs can have longer-term effects on earthworms, owing to their slow degradation rate in the environment. This necessitates long-term exposure experiments. In addition, different exposure scenarios (i.e., ingestion of MPs and other adsorbed contaminants) need to be further considered.
Fourth, earthworms are affected by the combined toxicity of MPs and other pollutants. For example, during the production and processing of plastics, numerous plasticizers are added, which can be easily released into the environment and have toxic effects on earthworms. In addition, MPs can adsorb heavy metals and organic pollutants from the environment, which can alter their accumulation and bio-effectiveness in earthworms. This co-toxicity adds complexity, as the ability of MPs to adsorb other contaminants is influenced by external conditions, which has yet to be fully understood.
Lastly, most studies have focused at the individual level (i.e., individual growth rate, mortality, biomass, and reproduction rate). In the future, we suggest that more attention should be paid to the toxic effects of MPs on earthworms at the cellular/molecular scale using genomics and metabolomics techniques.
6 Conclusion
The continuous accumulation of MPs, an emerging pollutant, in the environment has attracted extensive attention from researchers worldwide. In this bibliometric study, we identified polyethylene and polystyrene as the two most widely studied plastics in the context of earthworm toxicity. These and other MPs exhibit toxic effects through various mechanisms, thus increasing earthworm mortality associated with histopathological damage and oxidative stress. Furthermore, MPs show co-toxicity in earthworms via the adsorption–desorption of heavy metals and organic pollutants, which can increase pollutant accumulation and bio-effectiveness. Notably, when the concentration of MPs in soil exceeds 0.1% w/w, not only are the growth and reproduction of earthworms affected, but also tissue damage and oxidative stress can occur in association with nerve and DNA damage.
Author contributions
SG and QW: conceptualization, methodology, formal analysis, and writing—original draft. ZL and HL: methodology. YC, JZ, and XW: writing—review and editing. JL: data curation. BC: investigation. GZ: conceptualization and methodology. BZ and MZ: supervision.
Funding
This study was funded by the Beijing Academy of Agriculture and Forestry Sciences (grant number KJCX20210430), the Innovation Capacity Building Project of the Beijing Academy of Agriculture and Forestry Sciences (grant number KJCX20200419) and National Key Research and Development Plan Program of China (grant number 2016YFD0800906). This work was supported by Beijing Academy of Agriculture and Forestry Sciences and China Agricultural University.
Conflict of interest
The authors declare that the research was conducted in the absence of any commercial or financial relationships that could be construed as a potential conflict of interest.
Publisher’s note
All claims expressed in this article are solely those of the authors and do not necessarily represent those of their affiliated organizations, or those of the publisher, the editors and the reviewers. Any product that may be evaluated in this article, or claim that may be made by its manufacturer, is not guaranteed or endorsed by the publisher.
Supplementary material
The Supplementary Material for this article can be found online at: https://www.frontiersin.org/articles/10.3389/fenvs.2023.1126847/full#supplementary-material
Abbreviations
MPs, microplastics; LDPE, low-density polyethylene; HOCs, hydrophobic organic compounds; PCBs, polychlorinated biphenyls; DOM, dissolved organic matter; ROS, reactive oxygen species; PP, polypropylene; polyvinyl chloride.
References
Baeza, C., Cifuentes, C., Gonzalez, P., Araneda, A., and Barra, R. (2020). Experimental exposure of Lumbricus terrestristo microplastics. Water Air Soil Poll. 231, 308. doi:10.1007/s11270-020-04673-0
Ballent, A., Corcoran, P. L., Madden, O., Helm, P. A., and Longstaffe, F. J. (2016). Sources and sinks of microplastics in Canadian Lake Ontario nearshore, tributary and beach sediments. Mar. Pollut. Bull. 110, 383–395. doi:10.1016/j.marpolbul.2016.06.037
Bernard, E., Brulle, F., Dumez, S., Lemiere, S., Platel, A., Nesslany, F., et al. (2015). Antioxidant responses of annelids, brassicaceae and fabaceae to pollutants: A review. Ecotox Environ. Safe 114, 273–303. doi:10.1016/j.ecoenv.2014.04.024
Bläsing, M., and Amelung, W. (2018). Plastics in soil: Analytical methods and possible sources. Sci. Total Environ. 612, 422–435. doi:10.1016/j.scitotenv.2017.08.086
Boughattas, I., Zitouni, N., Hattab, S., Mkhinini, M., Missawi, O., Helaoui, S., et al. (2022). Interactive effects of environmental microplastics and 2,4-dichlorophe- noxyacetic acid (2,4-D) on the earthworm Eisenia andrei. J. Hazard Mater 424, 127578. doi:10.1016/j.jhazmat.2021.127578
Cao, Y., Zhao, M., Ma, X., Song, Y., Zuo, S., Li, H., et al. (2021). A critical review on the interactions of microplastics with heavy metals: Mechanism and their combined effect on organisms and humans. Sci. Total Environ. 788, 147620. doi:10.1016/j.scitotenv.2021.147620
Chae, Y., and An, Y. (2018). Current research trends on plastic pollution and ecological impacts on the soil ecosystem: A review. Environ. Pollut. 240, 387–395. doi:10.1016/j.envpol.2018.05.008
Chen, Y., Liu, X., Leng, Y., and Wang, J. (2020). Defense responses in earthworms (Eisenia fetida) exposed to low-density polyethylene microplastics in soils. Ecotox Environ. Safe 187, 109788. doi:10.1016/j.ecoenv.2019.109788
Cheng, Y., Zhu, L., Song, W., Jiang, C., Li, B., Du, Z., et al. (2020a). Combined effects of mulch film-derived microplastics and atrazine on oxidative stress and gene expression in earthworm (Eisenia fetida). Sci. Total Environ. 746, 141280. doi:10.1016/j.scitotenv.2020.141280
Ding, L., Wang, X., Ouyang, Z., Chen, Y., Wang, X., Liu, D., et al. (2021). The occurrence of microplastic in Mu Us Sand Land soils in northwest China: Different soil types, vegetation cover and restoration years. J. Hazard Mater 403, 123982. doi:10.1016/j.jhazmat.2020.123982
Ding, W., Li, Z., Qi, R., Jones, D. L., Liu, Q., Liu, Q., et al. (2021). Effect thresholds for the earthworm Eisenia fetida: Toxicity comparison between conventional and biodegradable microplastics. Sci. Total Environ. 781, 146884. doi:10.1016/j.scitotenv.2021.146884
Gaylor, M. O., Harvey, E., and Hale, R. C. (2013). Polybrominated diphenyl ether (PBDE) accumulation by earthworms (Eisenia fetida) exposed to biosolids-polyurethane foam microparticle-and penta-BDE-amended soils. Environ. Sci. Technol. 47, 13831–13839. doi:10.1021/es403750a
Gong, W., Jiang, M., Han, P., Liang, G., Zhang, T., and Liu, G. (2019). Comparative analysis on the sorption kinetics and isotherms of fipronil on nondegradable and biodegradable microplastics. Environ. Pollut. 254, 112927. doi:10.1016/j.envpol.2019.07.095
Heinze, W. M., Mitrano, D. M., Lahive, E., Koestel, J., and Cornelis, G. (2021). Nanoplastic transport in soil via bioturbation by Lumbricus terrestris. Environ. Sci. Technol. 55, 16423–16433. doi:10.1021/acs.est.1c05614
Hodson, M. E., Duffus-Hodson, C. A., Clark, A., Prendergast-Miller, M. T., and Thorpe, K. L. (2017). Plastic bag derived-microplastics as a vector for metal exposure in terrestrial invertebrates. Environ. Sci. Technol. 51, 4714–4721. doi:10.1021/acs.est.7b00635
Horton, A. A., Walton, A., Spurgeon, D. J., Lahive, E., and Svendsen, C. (2017). Microplastics in freshwater and terrestrial environments: Evaluating the current understanding to identify the knowledge gaps and future research priorities. Sci. Total Environ. 586, 127–141. doi:10.1016/j.scitotenv.2017.01.190
Huang, C., Ge, Y., Yue, S., Zhao, L., and Qiao, Y. (2021a). Microplastics aggravate the joint toxicity to earthworm Eisenia fetida with cadmium by altering its availability. Sci. Total Environ. 753, 142042. doi:10.1016/j.scitotenv.2020.142042
Huerta Lwanga, E., Gertsen, H., Gooren, H., Peters, P., Salanki, T., Van Der Ploeg, M., et al. (2017a). Incorporation of microplastics from litter into burrows of Lumbricus terrestris. Environ. Pollut. 220, 523–531. doi:10.1016/j.envpol.2016.09.096
Huerta Lwanga, E., Gertsen, H., Gooren, H., Peters, P., Salánki, T., Van Der Ploeg, M., et al. (2016). Microplastics in the terrestrial ecosystem: Implications forLumbricus terrestris (Oligochaeta, lumbricidae). Environ. Sci. Technol. 50, 2685–2691. doi:10.1021/acs.est.5b05478
Huerta Lwanga, E., Mendoza Vega, J., Ku Quej, V., Chi, J. D. L. A., Sanchez Del Cid, L., Chi, C., et al. (2017b). Field evidence for transfer of plastic debris along a terrestrial food chain. Sci. Rep-Uk 7, 14071. doi:10.1038/s41598-017-14588-2
Huerta Lwanga, E., Thapa, B., Yang, X., Gertsen, H., Salanki, T., Geissen, V., et al. (2018). Decay of low-density polyethylene by bacteria extracted from earthworm's guts: A potential for soil restoration. Sci. Total Environ. 624, 753–757. doi:10.1016/j.scitotenv.2017.12.144
Jia, H., Wu, D., Yu, Y., Han, S., Sun, L., and Li, M. (2021). Impact of microplastics on bioaccumulation of heavy metals in rape (Brassica napus L.). Chemosphere 288, 132576. doi:10.1016/j.chemosphere.2021.132576
Jiang, X., Yang, Y., Wang, Q., Liu, N., and Li, M. (2022). Seasonal variations and feedback from microplastics and cadmium on soil organisms in agricultural fields. Environ. Int. 161, 107096. doi:10.1016/j.envint.2022.107096
Li, B., Lan, Z., Wang, L., Sun, H., Yao, Y., Zhang, K., et al. (2019). The release and earthworm bioaccumulation of endogenous hexabromocyclododecanes (HBCDDs) from expanded polystyrene foam microparticles. Environ. Pollut. 255, 113163. doi:10.1016/j.envpol.2019.113163
Li, B., Song, W., Cheng, Y., Zhang, K., Tian, H., Du, Z., et al. (2021). Ecotoxicological effects of different size ranges of industrial-grade polyethylene and polypropylene microplastics on earthworms Eisenia fetida. Sci. Total Environ. 783, 147007. doi:10.1016/j.scitotenv.2021.147007
Li, J., Peng, D., Ouyang, Z., Liu, P., Fang, L., and Guo, X. (2022). Occurrence status of microplastics in main agricultural areas of Xinjiang Uygur Autonomous Region, China. Sci. Total Environ. 828, 154259. doi:10.1016/j.scitotenv.2022.154259
Li, J., Song, Y., and Cai, Y. (2020). Focus topics on microplastics in soil: Analytical methods, occurrence, transport, and ecological risks. Environ. Pollut. 257, 113570. doi:10.1016/j.envpol.2019.113570
Li, M., Liu, Y., Xu, G., Wang, Y., and Yu, Y. (2021). Impacts of polyethylene microplastics on bioavailability and toxicity of metals in soil. Sci. Total Environ. 760, 144037. doi:10.1016/j.scitotenv.2020.144037
Li, S., Ding, F., Flury, M., Wang, Z., Xu, L., Li, S., et al. (2022). Macro- and microplastic accumulation in soil after 32 years of plastic film mulching. Environ. Pollut. 300, 118945. doi:10.1016/j.envpol.2022.118945
Li, S., Ma, R., Zhu, X., Liu, C., Li, L., Yu, Z., et al. (2021). Sorption of tetrabromobisphenol A onto microplastics: Behavior, mechanisms, and the effects of sorbent and environmental factors. Ecotox Environ. Safe 210, 111842. doi:10.1016/j.ecoenv.2020.111842
Liu, J., Ma, Y., Zhu, D., Xia, T., Qi, Y., Yao, Y., et al. (2018). Polystyrene nanoplastics-enhanced contaminant transport: Role of irreversible adsorption in glassy polymeric domain. Environ. Sci. Technol. 52, 2677–2685. doi:10.1021/acs.est.7b05211
Liu, J., Qin, J., Zhu, L., Zhu, K., Liu, Z., Jia, H., et al. (2022). The protective layer formed by soil particles on plastics decreases the toxicity of polystyrene microplastics to earthworms (Eisenia fetida). Environ. Int. 162, 107158. doi:10.1016/j.envint.2022.107158
Liu, J., Zhang, T., Tian, L., Liu, X., Qi, Z., Ma, Y., et al. (2019). Aging significantly affects mobility and contaminant-mobilizing ability of nanoplastics in saturated loamy sand. Environ. Sci. Technol. 53, 5805–5815. doi:10.1021/acs.est.9b00787
Liu, L., Zou, G., Zuo, Q., Li, S., Bao, Z., Jin, T., et al. (2022). It is still too early to promote biodegradable mulch film on a large scale: A bibliometric analysis. Environ. Technol. Inno 27, 102487. doi:10.1016/j.eti.2022.102487
Liu, Y., Xu, G., and Yu, Y. (2022). Effects of polystyrene microplastics on accumulation of pyrene by earthworms. Chemosphere 296, 134059. doi:10.1016/j.chemosphere.2022.134059
Liwarska-Bizukojc, E. (2021). Effect of (bio)plastics on soil environment: A review. Sci. Total Environ. 795, 148889. doi:10.1016/j.scitotenv.2021.148889
Magni, S., Binelli, A., Pittura, L., Avio, C. G., Della Torre, C., Parenti, C. C., et al. (2019). The fate of microplastics in an Italian wastewater treatment plant. Sci. Total Environ. 652, 602–610. doi:10.1016/j.scitotenv.2018.10.269
Mei, W., Chen, G., Bao, J., Song, M., Li, Y., and Luo, C. (2020). Interactions between microplastics and organic compounds in aquatic environments: A mini review. Sci. Total Environ. 736, 139472. doi:10.1016/j.scitotenv.2020.139472
Nizzetto, L., Futter, M., and Langaas, S. (2016). Are agricultural soils dumps for microplastics of urban origin? Environ. Sci. Technol. 50, 10777–10779. doi:10.1021/acs.est.6b04140
OECD (2004). Guideline for testing of chemicals No. 222, earthworm reproduction test (Eisenia fetida/Eisenia andrei) [M]. Paris, France: OECD.
Okamoto, K., Nomura, M., Horie, Y., and Okamura, H. (2022). Color preferences and gastrointestinal-tract retention times of microplastics by freshwater and marine fishes. Environ. Pollut. 304, 119253. doi:10.1016/j.envpol.2022.119253
Pinto-Poblete, A., Retamal-Salgado, J., Lopez, M. D., Zapata, N., Sierra-Almeida, A., and Schoebitz, M. (2022). Combined effect of microplastics and Cd alters the enzymatic activity of soil and the productivity of strawberry plants. Plants-Basel 11, 536. doi:10.3390/plants11040536
Prendergast-Miller, M. T., Katsiamides, A., Abbass, M., Sturzenbaum, S. R., Thorpe, K. L., and Hodson, M. E. (2019). Polyester-derived microfibre impacts on the soil-dwelling earthworm Lumbricus terrestris. Environ. Pollut. 251, 453–459. doi:10.1016/j.envpol.2019.05.037
Rillig, M. C. (2012). Microplastic in terrestrial ecosystems and the soil? Environ. Sci. Technol. 46, 6453–6454. doi:10.1021/es302011r
Rochman, C. M., Hoh, E., Hentschel, B. T., and Kaye, S. (2013). Long-term field measurement of sorption of organic contaminants to five types of plastic pellets: Implications for plastic marine debris. Environ. Sci. Technol. 47, 1646–1654. doi:10.1021/es303700s
Rodriguez-Seijo, A., Da Costa, J. P., Rocha-Santos, T., Duarte, A. C., and Pereira, R. (2018). Oxidative stress, energy metabolism and molecular responses of earthworms (Eisenia fetida) exposed to low-density polyethylene microplastics. Environ. Sci. Pollut. R. 25, 33599–33610. doi:10.1007/s11356-018-3317-z
Rodriguez-Seijo, A., Lourenco, J., Rocha-Santos, T. A. P., Da Costa, J., Duarte, A. C., Vala, H., et al. (2017). Histopathological and molecular effects of microplastics in Eisenia andrei Bouche. Environ. Pollut. 220, 495–503. doi:10.1016/j.envpol.2016.09.092
Scheurer, M., and Bigalke, M. (2018). Microplastics in Swiss floodplain soils. Environ. Sci. Technol. 52, 3591–3598. doi:10.1021/acs.est.7b06003
Souza Machado, A. A., Kloas, W., Zarfl, C., Hempel, S., and Rillig, M. C. (2018). Microplastics as an emerging threat to terrestrial ecosystems. Glob. Change Biol. 24, 1405–1416. doi:10.1111/gcb.14020
Spurgeon, D. J., Ricketts, H., Svendsen, C., Morgan, A. J., and Kille, P. (2005). Hierarchical responses of soil invertebrates (earthworms) to toxic metal stress. Environ. Sci. Technol. 39, 5327–5334. doi:10.1021/es050033k
Su, L., Xue, Y., Li, L., Yang, D., Kolandhasamy, P., Li, D., et al. (2016). Microplastics in taihu lake, China. Environ. Pollut. 216, 711–719. doi:10.1016/j.envpol.2016.06.036
Sun, W., Meng, Z., Li, R., Zhang, R., Jia, M., Yan, S., et al. (2021). Joint effects of microplastic and dufulin on bioaccumulation, oxidative stress and metabolic profile of the earthworm (Eisenia fetida). Chemosphere 263, 128171. doi:10.1016/j.chemosphere.2020.128171
Thompson, R. C., Olsen, Y., Mitchell, R. P., Davis, A., Rowland, S. J., John, A. W. G., et al. (2004). Lost at sea: Where is all the plastic. Science 304, 838. doi:10.1126/science.1094559
Vieira, Y., Lima, E. C., Foletto, E. L., and Dotto, G. L. (2021). Microplastics physicochemical properties, specific adsorption modeling and their interaction with pharmaceuticals and other emerging contaminants. Sci. Total Environ. 753, 141981. doi:10.1016/j.scitotenv.2020.141981
Wang H., H., Ding, J., Xiong, C., Zhu, D., Li, G., Jia, X., et al. (2019). Exposure to microplastics lowers arsenic accumulation and alters gut bacterial communities of earthworm Metaphire californica. Environ. Pollut. 251, 110–116. doi:10.1016/j.envpol.2019.04.054
Wang J., J., Coffin, S., Sun, C., Schlenk, D., and Gan, J. (2019). Negligible effects of microplastics on animal fitness and HOC bioaccumulation in earthworm Eisenia fetida in soil. Environ. Pollut. 249, 776–784. doi:10.1016/j.envpol.2019.03.102
Wang, W., Yuan, W., Xu, E. G., Li, L., Zhang, H., and Yang, Y. (2022). Uptake, translocation, and biological impacts of micro(nano)plastics in terrestrial plants: Progress and prospects. Environ. Res. 203, 111867. doi:10.1016/j.envres.2021.111867
Wang, Y., Liu, C., Wang, F., and Sun, Q. (2022). Behavior and mechanism of atrazine adsorption on pristine and aged microplastics in the aquatic environment: Kinetic and thermodynamic studies. Chemosphere 292, 133425. doi:10.1016/j.chemosphere.2021.133425
Wu, R., Cai, Y., Chen, Y., Yang, Y., Xing, S., and Liao, X. (2021). Occurrence of microplastic in livestock and poultry manure in South China. Environ. Pollut. 277, 116790. doi:10.1016/j.envpol.2021.116790
Xiang, Y., Jiang, L., Zhou, Y., Luo, Z., Zhi, D., Yang, J., et al. (2022). Microplastics and environmental pollutants: Key interaction and toxicology in aquatic and soil environments. J. Hazard Mater 422, 126843. doi:10.1016/j.jhazmat.2021.126843
Xiao, X., He, E., Jiang, X., Li, X., Yang, W., Ruan, J., et al. (2022). Visualizing and assessing the size-dependent oral uptake, tissue distribution, and detrimental effect of polystyrene microplastics in Eisenia fetida. Environ. Pollut. 306, 119436. doi:10.1016/j.envpol.2022.119436
Xu, B., Huang, D., Liu, F., Alfaro, D., Lu, Z., Tang, C., et al. (2021). Contrasting effects of microplastics on sorption of diazepam and phenanthrene in soil. J. Hazard Mater 406, 124312. doi:10.1016/j.jhazmat.2020.124312
Xu, B., Liu, F., Cryder, Z., Huang, D., Lu, Z., He, Y., et al. (2020). Microplastics in the soil environment: Occurrence, risks, interactions and fate - a review. Crit. Rev. Env. Sci. Tec. 50, 2175–2222. doi:10.1080/10643389.2019.1694822
Xu, G., Yang, Y., and Yu, Y. (2021). Size effects of polystyrene microplastics on the accumulation and toxicity of (semi-)metals in earthworms. Environ. Pollut. 291, 118194. doi:10.1016/j.envpol.2021.118194
Xu, G., and Yu, Y. (2021). Polystyrene microplastics impact the occurrence of antibiotic resistance genes in earthworms by size-dependent toxic effects. J. Hazard Mater 416, 125847. doi:10.1016/j.jhazmat.2021.125847
Xu, J., Zhang, K., Wang, L., Yao, Y., and Sun, H. (2022). Strong but reversible sorption on polar microplastics enhanced earthworm bioaccumulation of associated organic compounds. J. Hazard Mater 423, 127079. doi:10.1016/j.jhazmat.2021.127079
Xu, P., Ge, W., Chai, C., Zhang, Y., Jiang, T., and Xia, B. (2019). Sorption of polybrominated diphenyl ethers by microplastics. Mar. Pollut. Bull. 145, 260–269. doi:10.1016/j.marpolbul.2019.05.050
Yang, J., Cang, L., Sun, Q., Dong, G., Ata-Ul-Karim, S. T., and Zhou, D. (2019). Effects of soil environmental factors and UV aging on Cu2+ adsorption on microplastics. Environ. Sci. Pollut. Res. Int. 26, 23027–23036. doi:10.1007/s11356-019-05643-8
Yang, J. Y., Yang, X. E., He, Z. L., Li, T. Q., Shentu, J. L., and B, P. J. S. (2006). Effects of pH, organic acids, and inorganic ions on lead desorption from soils. Environ. Pollut. 143, 9–15. doi:10.1016/j.envpol.2005.11.010
Yang, Y., Xu, G., and Yu, Y. (2022). Microplastics impact the accumulation of metals in earthworms by changing the gut bacterial communities. Sci. Total Environ. 831, 154848. doi:10.1016/j.scitotenv.2022.154848
Yu, L., Zhang, J., Liu, Y., Chen, L., Tao, S., and Liu, W. (2021). Distribution characteristics of microplastics in agricultural soils from the largest vegetable production base in China. Sci. Total Environ. 756, 143860. doi:10.1016/j.scitotenv.2020.143860
Yu, M., Van Der Ploeg, M., Huerta Lwanga, E., Yang, X., Zhang, S., Ma, X., et al. (2019). Leaching of microplastics by preferential flow in earthworm (Lumbricus terrestris) burrows. Environ. Chem. 16, 31–40. doi:10.1071/EN18161
Yu, M., Van Der Ploeg, M., Ma, X., Ritsema, C. J., and Geissen, V. (2020). Effects of microplastics and earthworm burrows on soil macropore water flow within a laboratory soil column setup. Vadose Zone J. 19. doi:10.1002/vzj2.20059
Zhang, L., Sintim, H. Y., Bary, A. I., Hayes, D. G., Wadsworth, L. C., Anunciado, M. B., et al. (2018). Interaction of Lumbricus terrestris with macroscopic polyethylene and biodegradable plastic mulch. Sci. Total Environ. 635, 1600–1608. doi:10.1016/j.scitotenv.2018.04.054
Zhang, Y., Lykaki, M., Markiewicz, M., Alrajoula, M. T., Kraas, C., and Stolte, S. (2022). Environmental contamination by microplastics originating from textiles: Emission, transport, fate and toxicity. J. Hazard Mater 430, 128453. doi:10.1016/j.jhazmat.2022.128453
Zhang, Y., Pu, S., Lv, X., Gao, Y., and Ge, L. (2020). Global trends and prospects in microplastics research: A bibliometric analysis. J. Hazard Mater 400, 123110. doi:10.1016/j.jhazmat.2020.123110
Zhao, S., Zhang, Z., Chen, L., Cui, Q., Cui, Y., Song, D., et al. (2022). Review on migration, transformation and ecological impacts of microplastics in soil. Appl. Soil Ecol. 176, 104486. doi:10.1016/j.apsoil.2022.104486
Zhong, H., Yang, S., Zhu, L., Liu, C., Zhang, Y., and Zhang, Y. (2021). Effect of microplastics in sludge impacts on the vermicomposting. Bioresour. Technol. 326, 124777. doi:10.1016/j.biortech.2021.124777
Zhou, B., Wang, J., Zhang, H., Shi, H., Fei, Y., Huang, S., et al. (2020a). Microplastics in agricultural soils on the coastal plain of Hangzhou Bay, east China: Multiple sources other than plastic mulching film. J. Hazard Mater 388, 121814. doi:10.1016/j.jhazmat.2019.121814
Zhou, Y., Liu, X., and Wang, J. (2020b). Ecotoxicological effects of microplastics and cadmium on the earthworm Eisenia foetida. J. Hazard Mater 392, 122273. doi:10.1016/j.jhazmat.2020.122273
Zhou, Y., Yang, Y., Liu, G., He, G., and Liu, W. (2020c). Adsorption mechanism of cadmium on microplastics and their desorption behavior in sediment and gut environments: The roles of water pH, lead ions, natural organic matter and phenanthrene. Water Res. 184, 116209. doi:10.1016/j.watres.2020.116209
Zhou, Z., Sun, Y., Wang, Y., Yu, F., and Ma, J. (2022). Adsorption behavior of Cu(II) and Cr(VI) on aged microplastics in antibiotics-heavy metals coexisting system. Chemosphere 291, 132794. doi:10.1016/j.chemosphere.2021.132794
Keywords: soil environment, microplastics, earthworms, bibliometric analysis, model organisms
Citation: Guo S, Wang Q, Li Z, Chen Y, Li H, Zhang J, Wang X, Liu J, Cao B, Zou G, Zhang B and Zhao M (2023) Ecological risk of microplastic toxicity to earthworms in soil: A bibliometric analysis. Front. Environ. Sci. 11:1126847. doi: 10.3389/fenvs.2023.1126847
Received: 18 December 2022; Accepted: 16 January 2023;
Published: 25 January 2023.
Edited by:
Cheng Zhang, Jiangnan University, ChinaReviewed by:
Song Ningning, Qingdao Agricultural University, ChinaXuetao Guo, Northwest A&F University, China
Copyright © 2023 Guo, Wang, Li, Chen, Li, Zhang, Wang, Liu, Cao, Zou, Zhang and Zhao. This is an open-access article distributed under the terms of the Creative Commons Attribution License (CC BY). The use, distribution or reproduction in other forums is permitted, provided the original author(s) and the copyright owner(s) are credited and that the original publication in this journal is cited, in accordance with accepted academic practice. No use, distribution or reproduction is permitted which does not comply with these terms.
*Correspondence: Baogui Zhang, zhangbg@cau.edu.cn; Meng Zhao, 1194704757@qq.com
†These authors have contributed equally to this work and share first authorship