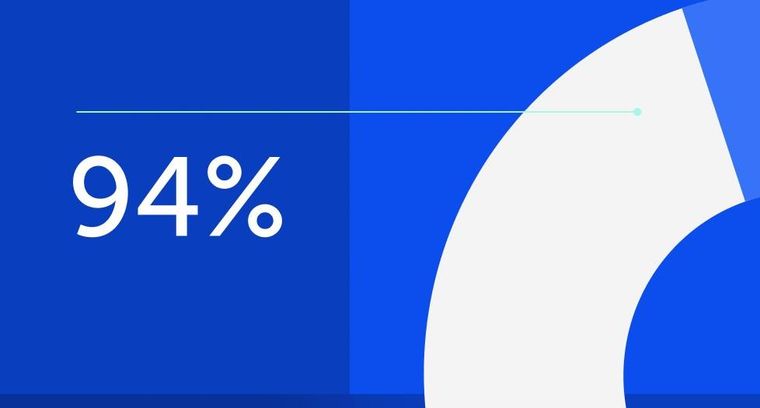
94% of researchers rate our articles as excellent or good
Learn more about the work of our research integrity team to safeguard the quality of each article we publish.
Find out more
ORIGINAL RESEARCH article
Front. Environ. Sci., 13 March 2023
Sec. Freshwater Science
Volume 11 - 2023 | https://doi.org/10.3389/fenvs.2023.1124797
This article is part of the Research TopicAssessment and Restoration of River Ecosystem IntegrityView all 7 articles
Groundwater-influenced ditches, so called hinterland drainage systems (HDS), accompany many heavily modified water bodies (HMWBs). Herein, we evaluate the usefulness of including HDS as functional habitats in river restoration approaches by assessing their role as salmonid recruitment areas in HMWBs. We tested the functionality of spawning grounds in a groundwater-influenced HDS compared to the quality of those not influenced by groundwater (OHB 1 and OHB 2), based on bioindication using brown trout eggs. Eggs were placed in each compartment of a two-layered container of 20 cm diameter and 30 cm total depth (known as the HydroEcoSedimentary Tool or HEST) pre-filled with sediment, mimicking hyporheic conditions. A total of 81 HESTs were evenly distributed across the three sites to assess egg and larvae mortality whilst exposed at two substrate depths (5–10 cm and 17–22 cm). In addition, key physico-chemical variables such as dissolved oxygen, temperature, pH, turbidity, electric conductance and substratum composition were analyzed. Mean egg mortality in the HDS was 33% lower than in both non-groundwater-influenced spawning grounds, whereas mean larval mortality was highest in the HDS (95%) compared to 74% at OHB 1 and 66% at OHB 2. The exposure depth of eggs had a significant influence on mortality, with more dead eggs and larvae in the deeper substrate layers, most likely attributed to an increase of fine sediment and a decrease of oxygen availability over time. Particularly the HDS revealed high mortality rates of larvae since it had a 17% lower mean oxygen level compared to the other spawning grounds. These findings suggest that if additional measures such as substratum restoration were undertaken to increase oxygen availability within HDS, these groundwater-dominated ditches could be incorporated as part of an efficient river restoration strategy.
A large proportion of European rivers have undergone heavy structural modifications including engineering modifications for shipping purposes, flood protection or hydropower use (Auerswald et al., 2019). In the context of the European Water Framework Directive (WFD, European Commission, 2000) such rivers are defined as “Heavily Modified Water Bodies” (HMWBs). The changes in river morphology in these HMWB has led to a severe loss of riverine fish habitat (Habersack et al., 2016) and subsequently to a decline of riverine fish species (Pander and Geist, 2010a; Mueller et al., 2018; Pander and Geist, 2018). The loss of fish habitat particularly affects critical early life stages (Aarts et al., 2004) and is considered a main factor of decline of European freshwater fish populations (Mueller et al., 2018). To reach the target of a “good ecological potential” in these waters as formulated in the WFD, habitat restoration is high on the agenda. Ideally, such restoration should be integrative and evidence-based (Geist and Hawkins, 2016), with the possibility of using popular target species as surrogates of the entire fish community in conservation (Geist, 2015). It is not unusual that HMWB, despite pronounced deficits in habitat quality, can still comprise a large diversity of freshwater fish species, often including relict and over-aged populations of typical riverine species that formerly dominated the fish community composition in these systems (Pander and Geist, 2010a). Successful restoration efforts in such areas offers a great potential to inverse the declines and extinctions of fish populations in these systems in the near future, by triggering reproduction and recruitment (Pander and Geist, 2016; Pander and Geist, 2018).
The structural transformation of natural rivers into HMWB resulted in multiple ecological and socio-economic consequences within and beyond the river that are widely irreversible (Auerswald et al., 2019) due to the evolved restrictions hindering comprehensive restoration of the riverine ecosystem and its ecosystem services (Pander and Geist, 2013). The structural modifications often created new, mostly channelized river courses adjacent to the main stems, which were mainly built as ditches to drain the hinterland (Figure 1A). These ditches, so-called hinterland drainage systems (HDS) exist along almost all European HMWBs and the intention when building them was to regulate increasing groundwater levels after the damming of the main river (Figures 1B, C). In most cases, these artificial and channel-like ditches arise from small natural creeks, run parallel to the levees of the main river at both sides, and finally drain into the tailwater of the main river below hydropower dams (Figures 1A, B). HDS mostly contain groundwater and drainage water that originates from drainage systems of the adjacent agricultural land (Figure 1A). Thereby the influence of agriculture is largely driven by cultivation techniques, crop rotations and intensity of farming and can vary greatly from place to place. Their substrate is largely dependent on the local geology and can range from gravelly substrate along alpine and pre-alpine areas, to finer substrate in the lowlands of larger river valleys. Especially in light of climate change with increasing periods of water scarcity and warm water temperatures during summertime, these HDS may provide important cool-water refuges, similar to cold-water spots in upwelling zones of natural river systems (Kuhn et al., 2021). During wintertime, when in mid European countries outside temperatures are usually very low, these systems can be much warmer than natural rivers. Due to the groundwater influence, these HDS are very clear, and can thus comprise a large diversity of macrophytes that are known to be important habitat structures for many macroinvertebrate and fish species (Pander et al., 2022a). However, in HDS, particularly during summer, periods of low oxygen supply may occur due to their high percentage of low-oxygen groundwater. The lower oxygen concentrations during summer result from two main factors, 1) the lower dissolution of oxygen at warmer water temperatures, and 2) the greater inflow and contribution of low-oxygen groundwater to the total discharge at low water levels during that period. Since HDS can be strongly influenced by agricultural drainages, contamination of these water bodies with nutrients and pesticides can occur (Cooper, 1993; Needelman et al., 2007) and further reduce habitat quality. Hydrodynamic conditions in HDS can vary widely from being near natural river systems to being completely embanked channels with a largely anthropogenic influenced hydrograph. The potential pros and cons of HDS have all contributed to a controversy about the usefulness of including HDS into restoration concepts of riverine fish populations. On the one hand, the cool temperatures of groundwater-influenced systems during summer may be important in a global warming-affected environment (Poff et al., 2002). On the other hand, the uniform river morphology and the adverse effects of agriculture on these systems, as well as the low availability of oxygen (Smiley et al., 2008; Kløve et al., 2011) during summer, give rise to doubts about the functionality of HDS as critical habitats for riverine fish species (Malcolm et al., 2003; Smiley et al., 2008).
FIGURE 1. Construction scheme of a typical Heavily Modified Water Body (HMWB) with hinterland drainage systems (HDS). (A) top view, (B) side view and (C) detail of the cross section, WL, water level; GWL, ground water level; AWL, actual water level of the main river after the dam construction; HWL, historic water level of the natural main river.
In this study, we evaluate the usefulness of including HDS as functional habitats in river restoration approaches by assessing their role as salmonid recruitment areas in the upper Danube catchment. We assessed the quality of spawning grounds in a groundwater-influenced HDS in comparison with two functional non-groundwater-influenced spawning grounds based on bioindication with brown trout eggs. The study area is located within a large floodplain restoration project in the upper Danube River (Stammel et al., 2012), which is known to provide several spawning grounds for lithophilic fish along its course (Pander et al., 2015a; Pander et al., 2017; Pander et al., 2022b). To compare the spawning ground quality with other sites, we used the HydroEcoSedimentary Tool (HEST, Casas-Mulet et al., 2021) as a standard method of active bioindication with brown trout eggs (Pander and Geist, 2013), The HEST tool is an integrated approach to assess hydro-sedimentary and ecologically relevant interstitial processes together and has recently proven its functionality to detect negative influences of hydropeaking on spawning grounds (Pander et al., 2022b). In particular, we hypothesize that the large amount of groundwater in the HDS impairs spawning ground quality, leading to unfavorable conditions in the interstitial spaces of the spawning grounds, and resulting in an impaired recruitment success for brown trout.
The study was carried out in two rivers, the Ottheinrichbach (OHB), and the Längenmühlbach hinterland draining system (HDS). Both are located in the middle section of a large scale floodplain restoration project at the upper River Danube in Bavaria, southern Germany (48°44′55.7″N, 11°16′35.7″E; Fischer and Cyffka, 2014; Stammel et al., 2012; Figure 2A). The catchment area of the upper River Danube is composed of low permeable tertiary layers and the typical karst aquifer in the carbonate rock of the jurassic. The OHB was mainly used as a bypass to restore the impaired fish migration within the Danube due to the construction of the power plant Bergheim, to provide additional habitat for keystone species, and to re-wet the former floodplain of one of the last remaining fluvial forests along this large European River (Stammel et al., 2012; Stammel et al., 2016). Since this river runs exclusively through alluvial forest, there is no major agricultural influence of eutrophication on the OHB. Before the hydropower plant Bergheim was built, the HDS had been a small natural tributary of the Danube. During the construction of the Bergheim plant, its lower course and confluence were reconstructed. The HDS got a new confluence in the tailwater of the power plant and now ensures the drainage of the hinterland so that intensive agriculture can be carried out. This is even the case after a new damming target, comprising much higher water levels of the Danube compared to the pre-damming phase (Figures 1A–C). The study site is known as an important fish nursery area (Pander et al., 2015a; Pander et al., 2017; Pander et al., 2022b) and both the OHB and HDS represent ideal model streams for tributaries located along heavily modified water bodies affected by hydropower production. The OHB has a fluctuating discharge between 0.5–5 m3 s−1 and can be additionally flooded with another 25 m3 s−1 to mimic natural dynamics of flood scenarios that were historically present in the alluvial forest (Stammel et al., 2012; Fischer and Cyffka, 2014). The discharge of the HDS mostly consists of groundwater originating from drainages of the agricultural land of the hinterland. Additionally, it is strongly influenced by precipitation events. In spite the agricultural land use with mainly maize and wheat fields (3.65 km2), the eutrophication level of the HDS is low. Since the HDS is also used to provide the OHB with flooding water from the Danube, its discharge can vary between 0.5–25 m3 s−1. In the OHB, two known spawning grounds (OHB 1 and OHB 2) and in the HDS one spawning ground of gravel-spawning fish species were chosen for active bioindication with brown trout eggs (Pander and Geist, 2013) (Figure 2B). OHB 1 (23 m2) is located in the upper reach of the OHB (48°44′12″N, 11°15′56″E) approximately 280 m downstream of a weir where the OHB is supplied with Danube water. OHB 1 has a flow gradient of 0.64%. OHB 2 is located in the middle reach of the OHB (48°44′25″N, 11°18′23″E), has a larger flow gradient (0.80%) and covers 36 m2. The HDS (48°44′49″N, 11°14′23″E) reach covers 20 m2 and comprises a flow gradient of 0.62%. A summary of key physico-chemical variables characterizing both the HDS and OHB is provided in Table 1.
FIGURE 2. Illustration of the study area within Europe (A) and location of the three investigated spawning grounds (B), one in the hinterland draining system Längenmühlbach (HDS) and two in the Ottheinrichbach (OHB 1 and OHB 2) within a large scale floodplain restoration at the Danube river. Colored triangles indicate the location of the three assessed spawning grounds; blue arrows indicate the flow directions. The lowermost Panel (C) indicates placement and construction of the HydroEcoSedimentary Tool (HEST) in OHB 2 as an example.
TABLE 1. Important physico-chemical variables of the Längenmühlbach (HDS) and Ottheinrichbach (OHB) during spring and summer. T, temperature; O2, dissolved oxygen [mg·L−1]; pH, pH-value; EC, electric conductance [µS·cm−1]; Turb H, turbidity [NTU]; D, water depth [m]; V, riverbed velocity [m·s−1]; W, river width [m]; DG, mean particle diameter of spawning ground substrate [mm].
In order to assess the potential effects of the groundwater influence on salmonid spawning habitat quality, we compared the strongly groundwater-influenced reach in the HDS with the two reaches in the OHB without groundwater influence. At each spawning ground, we installed 27 HydroEcoSedimentary Tools (HESTs) loaded with brown trout eggs (Salmo trutta fario L.) (Figure 2C) in each of their two levels or compartments. HESTs were made from interlocked plastic containers (AUER Packaging GmbH, Amerang, Germany), creating top (T) and bottom (B) compartments. We placed a temperature logger in each compartment, and installed a 1.5 m long Sahleberg® TubeTec silicon tubing of 4.5 mm inner diameter (Sahleberg GmbH, Feldkirchen, Germany) to enable water sample extraction. Three sets of different HEST types were applied to distinguish longitudinal, (L) lateral (X), and vertical (V) infiltration of fines (Figure 2C). Each of the infiltration openings was covered with Jaera® perforated metallic plates (2 mm diameter round holes, JAERA GmbH & Co. KG, Laatzen, Germany). For full details of the HEST design, see Casas-Mulet et al. (2021).
Before exposure, each HEST was pre-filled with sediment truncated at the 6.3 mm fraction. We followed granulometry curves from the natural streambed material, described in Pander et al. (2015a), to mimic the corresponding sediment sizes at each spawning ground. The prefilled sediment was prepared in our institute and originated from nearby rivers. Brown trout eggs were purchased from a local fish farm (Forellenhof Nadler, Eching, Germany). They were inserted into the HESTs at the eye-point stage to assess their survival, analogously to their use for bioindication of open (Pander and Geist, 2010b) and interstitial water quality (Pander et al., 2009). After receiving the eggs from the fish farm, they were acclimatized for 30 min in common upflow incubation trays as commonly used in fish hatcheries (Pander and Geist, 2010b) including an overall temperature adjustment of 2°C in the laboratory of the Aquatic Systems Biology Unit, Freising, Germany. During the adjustment, temperature and oxygen saturation were continuously checked with a handheld WTW® Multimeter 340i (WTW GmbH, Weilheim, Germany). Dead eggs were subsequently removed, and the remaining live eggs were distributed into the HESTs. Each HEST compartment (T and B) was loaded with 30 eggs that were randomly picked and placed by forming a small pit in the HESTs, which was then carefully covered with a 5 cm thick layer of substrate. The full HESTs were kept submerged in a large tank (700 L) with aerated water, so the quality of the eggs was preserved during transport (45 min) and until their exposure the next day (see Casas-Mulet et al., 2021 for further details).
On 18 December 2019, 27 HESTs were installed at the spawning grounds into the streambed of the OHB and HDS. They were located in a homogeneous riffle section of the channel, buried in the streambed and placed with enough distance between individual HESTs to avoid potential disturbance. They were completely buried with the top of the HEST at the streambed level, and with approximately 10 cm of water level above the top when installed. Sunshine was not considered a factor, since all HESTs in all sites had the same level of exposure. A hole big enough to fit each of the HESTs was dug with a common spade, keeping disturbance of the surrounding streambed to a minimum. Each HEST was then inserted so that its top was even with the gravel surface, and the rivers natural coarse sediment was used to fill up the gap to the same level. The HESTs were installed in closed mode and after fines were settled, they were opened to start the experiment (Casas-Mulet et al., 2021). After exposure, the HESTs were retrieved and immediately assessed in the laboratory for egg and larvae survival. The HEST compartments were opened carefully, data loggers and larger stones were removed, and the sediment content was emptied into a tray, rinsed with water and examined for larvae and eggs. A distinction was made between live and dead eggs as well as between live and dead larvae, as described in Casas-Mulet et al. (2021). All samples were preserved in a solution of 70% (v/v) ethanol.
Ecologically relevant physico-chemical parameters were measured in situ for each HEST and in the open water of the river after installation of the HEST and before retrieval. The interstitial water of each HEST was extracted following the procedure described in Casas-Mulet et al. (2021) using 100 mL, Omnifix Solo plastic syringes (B. Braun Melsungen AG, Melsungen, Germany). Samples of interstitial water were immediately transferred into clean 100 mL vials and temperature T) [°C], dissolved oxygen (O2) [mg·L−1], electrical conductance (EC) [µS·cm−1, corrected to 20°C], and pH were measured with a hand-held WTW® Multimeter 340i (WTW GmbH, Weilheim, Germany). In addition, turbidity (Turb) [NTU] was assessed using a WTW® Turb 355 IR measuring set. HOBO® temperature loggers (UA-002-064, Onset, United States) installed in the HESTs, and in the water column of each spawning ground, were used to record temperature data at 30 min intervals throughout the exposure time. To assess fine sediment deposition in the HESTs, we wet-sieved (DIN, 1990) the sediment contents of each HEST compartment after retrieval. We used an AS 200 Retsch sieving machine (Retsch, Haan, Germany) equipped with sieves ISO 3310-1 of screen sizes 20.0, 6.3, 2.0, 0.85, 0.20 and 0.045 mm. Coarse fractions (>20.0, >6.3, >2.0 mm) were dried at air temperature for 24 h, and finer fractions (<2.0 mm) were oven-dried at 100°C for 24 h. All fractions were then weighted with a scale (Dini Argeo S.r.l., Modena, Italy) to the nearest 0.1 g. Deposition of fines [g] and mean particle grain size (DG) [mm] in the HESTs was calculated. Water depth (D) [m] and near-bed flow velocity (v) [m·s−1] was measured with a magnetic inductive flow meter (Ott MF pro, Ott, Kempten, Germany) directly at the HESTs 5 cm below the water surface as well as 3–5 cm above them.
Total percent mortality was calculated as the ratio of difference between the surviving eggs or larvae and the initially loaded egg number for each of the HESTs retrieved from the three spawning grounds at the three time points after 18, 35, and 64 days of exposure. For univariate multiple-group comparisons of egg and larval mortality as well as abiotic habitat variables, each dataset was tested for normal distribution (Shapiro-Wilk test) and homoscedasticity (Levene test). Since data did not fulfil the criteria for parametric testing (normal distribution), the non-parametric Kruskal Wallis test was applied to test for significant differences. A subsequent post hoc Wilcoxon test with Bonferroni correction for multiple comparisons was used to determine whether values differed significantly between HESTs, compartments (depths) and/or infiltration directions. Since no significant differences between longitudinal, lateral and vertical HEST measurements were observed, the data was pooled for further investigation. Univariate statistics were carried out using statistical and graphical open-source software R (version 4.0.3R, www.R-project.org/, last accessed on 27 July 2022).
To test for relations between the abiotic predictor variables measured in the HESTs and the response variables egg mortality and larval mortality, general linear mixed effect models (GLMMs) were performed in R (R packages car and tidyverse). To meet model assumptions regarding normal distribution of model residuals and homogeneity of variances, predictor variables were square root transformed and subsequently tested for multi-collinearity using the Pearson method (Boslaugh and Watters, 2008). Since the Pearson method revealed values below 0.63, the variables were considered not to be significantly correlated according to Nettleton (2014) who suggests a threshold value of >0.7 indicative of collinearity. Subsequently, the predictor variables EC, pH, T, O2, Turb, deposition of fines, mean particle grain size, water depth and flow velocity were stepwise considered in the model for egg as well as larval mortality. To account for site variability, spawning ground was included as random effect. To consider potential interactions between turbidity, dissolved oxygen and deposition of fines, these three variables were stepwise integrated in the model using the interaction term. Model selection followed the top-down strategy (Diggle et al., 2002) as described in Zuur et al. (2009). The Akaike Information Criteria (AIC) based on the restricted maximum likelihood (REML) was chosen as model selection tool. The p-values for predictor variables of the best fitting model were obtained by Wald Chi-square tests. Significance was accepted at p < 0.05.
To visualize differences of HESTs in egg and larval mortality between the three spawning grounds, a non-metric multidimensional scaling (NMDS) analyses using PRIMER v7 (Plymouth Marine Laboratory, Plymouth, United Kingdom) was plotted. For this multivariate comparison, a resemblance matrix was calculated based on Bray–Curtis similarities were computed (Bray and Curtis, 1957; Clarke, 1993) using the full data resolution of egg and larval mortality from the different retrieval time points of HESTs at the three spawning grounds (Clarke et al., 2014). To test for significant differences of mortality rates between HESTs and between top and bottom compartments, one-way analysis of similarities (ANOSIM) based on Bray–Curtis similarities calculated from egg and larval mortality data as described above was used. To analyze the interaction between the measured abiotic habitat variables and the ordination of HESTs in the NMDS, the abiotic habitat variables were displayed in the NMDS using the overlay function in PRIMER. For all statistical tests, a significance level of p < 0.05 (= 95% probability) was applied.
To test for relations between larval mortality and oxygen concentration found in the HESTs, the oxygen concentration was used as predictor variable and plotted against the response variable larval mortality. To avoid false-negative conclusions due to potentially non-linear relations, Spearman rank correlation for monotonic trend were computed and a smooth curve was displayed. This analysis was also performed using R.
Univariate comparisons of egg and larval mortality revealed significant differences between the groundwater-influenced HDS and the two non-groundwater-influenced OHB 1 and OHB 2 that were considered as a reference (Figure 3; Table 2). In the groundwater-influenced HDS, egg mortality was significantly lower than in the two OHB. In contrast, larval mortality in the HDS was significantly highest, with a mean larval mortality of 95% compared to the non-groundwater-influenced OHB where it averaged 70% (Table 3). Egg and larval mortality between the two non-groundwater-influenced OHB 1 and OHB 2 were not significantly different (Table 2).
FIGURE 3. Box-whisker-plots (25% quantile, median. 75% quantile, whisker: minimum and maximum values, circles represent outliers) of egg and larval mortality [%] at the three different spawning grounds in the Längenmühlbach (HDS) and the Ottheinrichbach (OHB 1 and OHB 2).
TABLE 2. Univariate comparisons of egg and larval mortality for the three different spawning grounds. HDS, groundwater-influenced Längenmühlbach; OHB 1 and OHB 2, non-groundwater-influenced spawning grounds in the Ottheinrichbach. Kruskal-Wallis ANOVA df = 2. Wilcoxson-signed ranks post hoc test was Bonferroni corrected for multiple comparisons.
TABLE 3. Egg mortality and larval mortality detected in the top and bottom compartments of the HEST in the assessed Ottheinrichbach (OHB) and the groundwater-influenced hinterland draining system Längenmühlbach (HDS). Upper layer indicates mean mortality rates for eggs and larvae respectively, whilst the lower layer indicates minimum and maximum values.
Multivariate analyses of brown trout egg and larval mortality (Figure 4; Table 4) revealed significant differences (ANOSIM global test: R = 0.14, p < 0.001) between the groundwater-influenced HDS and OHB 1 (ANOSIM: R = 0.22, p < 0.001) as well as among HDS and OHB 2 (ANOSIM: R = 0.23, p < 0.001). No significant differences in egg and larval mortality were found between OHB 1 and OHB 2 (ANOSIM: R = 0.01, p > 0.05). In addition, significant differences between top and bottom compartments could only be detected for HDS (ANOSIM: R = 0.09, p < 0.05) with 7% higher mortality for eggs in top compartments and 8% higher mortality for larvae in bottom compartments (Table 4).
FIGURE 4. Non-metric multidimensional scaling (NMDS) comprising comparisons of egg and larval mortality of the HydroEcoSedimentary Tool (HEST) between the three assessed spawning grounds HDS, groundwater influenced hinterland draining system; OHB 1 and OHB 2, Ottheinrichbach spawning grounds; O2, dissolved oxygen [mg·L−1]; pH, pH-value; TL, temperature data [°C] from loggers exposed in the HEST; EC, electric conductance [µS·cm−1] (corrected to 20°C); Turb, turbidity [NTU]; DG, mean particle diameter [mm]; S0045, sediment grain size of 0.045 mm; S02, sediment grain size of 0.20 mm; S085, sediment grain size of 0.85 mm. Abiotic habitat variables were correlated on the NMDS ordination plot and are displayed as blue lines. The length of the blue lines is proportional to the degree of correlation with the arrangement of egg and larval mortality (the blue circle = indicates 100% correlation). 2D-Stress, stress value after Kruskal.
TABLE 4. Results of multivariate comparisons of the assessed spawning grounds in the Ottheinrichbach (OHB) and the groundwater-influenced hinterland draining system Längenmühlbach (HDS) with ANOSIM considering T, top compartment as well as B, bottom compartment of the HEST. Values in bold indicate statistically significant differences.
Temperature, O2, and pH differed significantly between the groundwater-influenced HDS and both non-groundwater-influenced OHB spawning grounds (Kruskal-Wallis ANOVA p < 0.001 and subsequently applied Wilcoxson signed-ranks post hoc tests, see Table 5). Mean temperature was 1.9 fold higher in the groundwater-influenced HDS (T = 7.12°C; O2 = 9.62 mgL−1) and mean O2 value (Figure 5) was 17% lower compared to the mean of both OHB (T = 3.77°C, O2 = 11.62 mgL−1). The pH value was lowest in the groundwater-influenced HDS (mean = 7.9) compared to the OHB spawning grounds where it was around 8.3 (Table 5). In addition, HDS, OHB 1, and OHB 2 differed significantly in EC and water depth. The groundwater-influenced HDS had the highest EC values (770 μS cm−1) and the values in the OHB 1 and OHB 2 were 28% and 18% lower, respectively. Lowest mean water depth (13 cm) was found in HDS, followed by OHB 1 (18 cm) and highest water depth was found in OHB 2 (22 cm). Since current speed did not vary significantly between all spawning grounds, we do not assume that the lower water depth in the HDS affected the egg or larval development. A significant increase of the net weight of fines <0.85 mm in the buckets could be detected over the exposure time with a cumulative mean across all spawning grounds of 80.4 g after 18 days, 97.4 g after 35 days and 141.6 g after 64 days of exposure (Figure 5). However, turbidity, mean particle diameter after excavation and infiltration rate did not differ significantly between spawning grounds. In terms of the infiltration direction, no significant differences were found in fine sediment deposition between spawning grounds and HEST types. Turbidity values measured in the extracted water samples of the HESTs ranged between 11 NTU and 1100 NTU and were not significantly different between spawning grounds (Table 5).
TABLE 5. Abiotic spawning ground characteristics of the assessed Ottheinrichbach (OHB) and the groundwater-influenced hinterland draining system Längenmühlbach (HDS). T, temperature; O2, dissolved oxygen [mg·L−1]; pH, pH-value; EC, electric conductance [µS·cm−1]; Turb H, turbidity [NTU] measured in the HESTs compartments; D, water depth [m]; V, riverbed velocity [m·s−1]; DG, mean particle diameter after retrieving the HESTs [mm]; IR, sediment infiltration rate [kg·d−1]. Different letters (a, b, c) behind the respective mean values indicate significant differences between pairwise comparisons of spawning grounds.
FIGURE 5. Box-whisker-plots (25% quantile, median. 75% quantile, whisker: minimum and maximum values, circles represent outliers) of net fine sediment deposition in the HEST [g] and oxygen availability in the interstitial of the HEST [%] at the groundwater-influenced hinterland draining system Längenmühlbach (HDS) and the non-groundwater-influenced spawning grounds in the Ottheinrichbach (OHB 1 and OHB 2).
The GLMMs for egg- and larval mortality revealed slightly different results regarding the predictor variables and their interactions (Table 6). For egg mortality, the predictors dissolved oxygen, temperature, deposition of fines and pH, with site as random factor and interactions between dissolved oxygen and deposition of fines resulted in the best fit with lowest AIC (749.92). For larval mortality, the best fitting model (AIC = 638.94) was more complex and additionally included the variables turbidity and interactions between turbidity and dissolved oxygen (Table 6).
TABLE 6. Statistical output of the two best fitting general linear mixed effects models (GLMMs) including the predictor variables dissolved oxygen, temperature, deposition of fines and pH for egg mortality and dissolved oxygen, temperature, deposition of fines, pH and turbidity for larval mortality. In both GLMMs spawning ground was set as random effect and interactions between dissolved oxygen and the deposition of fines were considered. In the GLMM for larval mortality was additionally the interaction between dissolved oxygen and turbidity considered since this led to a better fit. Values in bold indicate statistically significant differences.
Correlating oxygen availability in the buckets with larval mortality revealed that mortality increased sharply with decreasing oxygen supply in the interstitial zone, with more than 90% larval mortality whenever oxygen values were below 11 mg L−1 (Figure 6).
FIGURE 6. Correlation plot of the oxygen concentration measured in the HESTs against larval mortality. The smooth curves are locally weighted regression fits (Cleveland and Devlin, 1988) to the data points. LCL, lower critical limit for egg to fry survival according to Smialek et al. (2021).
Restoration of HMWBs is challenging in many ways. The most severe issues are the many restrictions going along with restoration of fish habitat in these waters (Pander and Geist, 2013), potentially hindering the restoration of river dynamic processes including flow, sediment (Hauer et al., 2018) and deadwood dynamics (Gurnell, 2012) which are all important factors for gravel-dependent riverine fish populations (Roni et al., 2014). The comparison of the groundwater-influenced HDS and the known functioning spawning grounds in the non-groundwater-influenced OHB clearly show the potential of HDS for the restoration of important key habitats for riverine fish species. Due to the lower oxygen availability in the groundwater-influenced HDS, it was highly unexpected that the egg development was much higher there compared to the spawning grounds in the OHB. The larval mortality of the brown trout, on the other hand, was much higher in the HDS with almost 100% of dead larvae in the HESTs. The differences in egg versus larval mortality in the HDS can be explained by the higher oxygen requirement of larvae compared to the eggs in general (Serigstad, 1987; Smialek et al., 2021). In addition, with a potentially limited transport of metabolites due to the clogging, the larval stages were prevented to develop further. The finding of higher oxygen demand of larvae compared to eggs is also supported by the results herein illustrating >90% larval mortality when O2 decreased under 11 mg L−1 in the HDS. Recently published results using the HEST, assessing the effects of hydropeaking on spawning grounds, suggest a slightly lower susceptibility of eggs to low oxygen concentrations, with sharply increasing egg mortality when oxygen levels fall under 10 mg L−1 (Pander et al., 2022b, also reviewed for salmonids in Smialek et al., 2021). Nevertheless, lower larval survival can also be attributed to a successive decrease of oxygen availability over the exposure time of the brown trout that happened simultaneously in all spawning grounds. In line with the findings of the GLMM, it is likely that accumulating fines have a substantial influence on mortality in the HESTs during exposure. Sedimentation of fines impaired the oxygen availability for eggs and larvae across all spawning grounds. This effect may been accelerated by fine particulate matter that usually also infiltrates the streambed and often cannot be separated easily from non-organic materials. If this was the case, oxygen consuming organic matter potentially further reduced the content of dissolved oxygen in the interstitial spaces. The longer the HESTs were exposed in the spawning grounds, the more fine material could accumulate, which is in line with observed accumulation of fines in gravel-filled boxes in the streambed (Denic and Geist, 2015; Hoess and Geist, 2020). In our study, this fine sediment accumulation resulted in almost doubling mean net deposition of fine sediment between the first (after 18 of exposure days) and the last assessment time point (after 64 days of exposure) in the HESTs, and consequently, in reduced oxygen availability in the interstitial (Geist and Auerswald, 2007). This interaction of fine sediment and decreasing oxygen availability in the hyporheic zone is also strongly supported by our model-output. Because oxygen availability in the groundwater-influenced HDS was generally much lower compared to the OHB spawning grounds, this effect was probably severe enough to lower oxygen availability in the groundwater-influenced HDS to the extent of not meeting the minimum oxygen requirements for the larvae stages. In a natural gravel bed, larvae may already emerge earlier to escape phases of low oxygen availability (emergency emergence) or change interstitial depth to reach more oxygenated layers, albeit their survival then remains questionable (Sternecker and Geist, 2010). However, escaping from the HESTs was not possible for the brown trout larvae in our experiment. They were only able to change interstitial depth inside the compartments and as the significant higher mortality in bottom compartments (which are more prone to oxygen depletion due to colmation) indicate, this was only successful for a very small number of larvae. Although oxygen availability in the water can be reduced during higher temperatures, it is unlikely that the higher water temperature in the groundwater-influenced HDS during the period of the study played any major role. Laboratory experiments with brown trout eggs of the same origin indicate that the measured temperate values in the HESTs were not even close to the thermal limits of successful egg or larval development (Smialek et al., 2021). Equally, pH and EC values, although strongly differed between the HDS and OHB, they were all within the range as known to be uncritical for a successful egg and larval development, suggesting an unlikely negative impact on the eggs or on the larvae of the brown trout.
The low mortality during the egg stage found in this study suggests that important habitats such as spawning grounds can potentially be restored in HDS if the exchange between the groundwater-influenced hyporheic interstitial and the open water can be secured throughout the whole development period of the fish eggs, until the larvae emerge. This is also in line with findings of Malcolm et al., 2003, who assessed salmonid egg survival in a degraded and groundwater-influenced gravel-bed stream. In order to achieve these conditions, it is essential that during several months of the spawning season and development time of the fish eggs, the spawning grounds do not get altered by fine sediment accumulations that potentially impair the exchange between hyporheic interstitial and the open water of the watercourse. Specific measures that can help achieve these conditions include loosening the streambed gravel (Shackle et al., 1999; Meyer et al., 2008; Sternecker et al., 2013; Nagel et al., 2020) or introducing of suitable spawning substrate shortly before the spawning season (Pedersen et al., 2009; Pander et al., 2015b). Management of hydrodynamic processes can also be an important tool to enhance the washing out of fines and sorting of gravel, increasing surface water–hyporheic exchange (Auerswald and Geist, 2018). Additional measures at the broader scale include the retention of fines in the river’s catchment, which can reduce fine sediment input in HDS (Knott et al., 2019) and lead to a longer permeable spawning substrate and favorable conditions for egg development. Addressing within-river processes of sediment dynamics through restoration measures appears equally important (Auerswald and Geist, 2018). For instance, sinuosity and macrophytes present in HDS can strongly affect the patchiness and quality of the streambed (Braun et al., 2012) and can be addressed by restoration measures. Also, the restoration of river dynamic processes such as gravel and deadwood transport (Beechie et al., 2010; Hauer et al., 2018; Pander and Geist, 2018) that at the same time result in substrate sorting and the creation of new natural spawning grounds and juvenile habitats can be beneficial. As evident from our dataset, restoration measures are mandatory in these systems, however, full connectivity to the HMWBs is also required so that spawners can migrate to these spawning grounds and lay their eggs.
The results of this study indicate that HDS hold a large potential to restore critical fish habitat such as spawning grounds along HMWBs. Recruitment success of brown trout can be improved if it is feasible to increase oxygen availability in the interstitial of highly groundwater-influenced HDSs over the full developmental time of brown trout eggs and larvae. The use of HDS as habitats for spawning and larval development needs to be better integrated in restoration strategies of main rivers and floodplains to improve fish populations in HMWBs. It has to be considered that the potential of HDS to restore spawning grounds and overall habitat quality is not enough if not combined with further measures to improve overall habitat quality, particularly of the hyporheic zone and of ensuring connectivity between HDS and the main river. Whilst restoration in the main channel of HMWBs is highly restricted, fish passes and side channels such as HDS may offer a great opportunity to restore required critical fish habitats beyond the HMWB itself, despite all restrictions that hinder restoration in these systems to the full extent. If the recommended restoration measures are realized, then HDS may not only add important sites for recruitment of gravel-dependent species, but also act as critical cold water refuge habitats during warm periods, which increase in light of climate change.
The raw data supporting the conclusion of this article will be made available by the authors, without undue reservation.
The animal study was reviewed and approved by Technical University of Munich, Animal Research Center; not considered an animal experiment since only fish eggs and early-life stage larvae were used.
Conceptualization, JP, RC-M, and JG; methodology, JP and RC-M; formal analysis, JP; writing—original draft preparation, JP and RC-M; editing and artwork, JP; review JG; project administration, JP and JG; resources, JG, JP, and RC-M; funding acquisition, RC-M. All authors have read and agreed to the published version of the manuscript.
This research was partly funded by the Alexander von Humboldt Foundation through a fellowship awarded to RC-M, carried out at TUM. This study was also partly supported by the framework of the project AquaKlif in the bayklif network for investigation of regional climate change funded by the Bavarian State Ministry of Science and the Arts.
We would like to thank Catarina Eirich, Julia Reinbeck and Martin Gauger who contributed to the sampling in the Ottheinrichbach and Längenmühlbach. We are also grateful to all the volunteers and student assistants who helped with the installation and retrieval of the HESTs in the field and later sample processing. We thank the AuenZentrum Neuburg-Ingolstadt for providing water level data, and, in particular to B. Cyffka for coordination of the permissions needed from the landowners. We also would like to thank the water authorities, in particular B. Kuegel from the local water authority, for their kind support of the study.
The authors declare that the research was conducted in the absence of any commercial or financial relationships that could be construed as a potential conflict of interest.
All claims expressed in this article are solely those of the authors and do not necessarily represent those of their affiliated organizations, or those of the publisher, the editors and the reviewers. Any product that may be evaluated in this article, or claim that may be made by its manufacturer, is not guaranteed or endorsed by the publisher.
Aarts, B. G., Van Den Brink, F. W., and Nienhuis, P. H. (2004). Habitat loss as the main cause of the slow recovery of fish faunas of regulated large rivers in Europe: The transversal floodplain gradient. Riv. Res. Appl. 20 (1), 3–23. doi:10.1002/rra.720
Auerswald, K., and Geist, J. (2018). Extent and causes of siltation in a headwater stream bed: Catchment soil erosion is less important than internal stream processes. Land Degrad. Dev. 29, 737–748. doi:10.1002/ldr.2779
Auerswald, K., Moyle, P., Seibert, S. P., and Geist, J. (2019). HESS Opinions: Socio-economic and ecological trade-offs of flood management - benefits of a transdisciplinary approach. Hydrol. Earth Syst. Sci. 23, 1035–1044. doi:10.5194/hess-23-1035-2019
Beechie, T. J., Sear, D. A., Olden, J. D., Pess, G. R., Buffington, J. M., Moir, H., et al. (2010). Process-based principles for restoring river ecosystems. BioScience 60 (3), 209–222. doi:10.1525/bio.2010.60.3.7
Boslaugh, S., and Watters, P. A. (2008). Statistics in a nutshell: A desktop quick reference. 2nd edition. Sebastopol, CA: O'Reilly Media, 571.
Braun, A., Auerswald, K., and Geist, J. (2012). Drivers and spatio-temporal extent of hyporheic patch variation: Implications for sampling. PLoS ONE 7, e42046. doi:10.1371/journal.pone.0042046
Bray, J. R., and Curtis, J. T. (1957). An ordination of the upland forest communities of southern Wisconsin. Ecol. Monogr. 27, 325–349. doi:10.2307/1942268
Casas-Mulet, R., Pander, J., Prietzel, M., and Geist, J. (2021). The HydroEcoSedimentary tool: An integrated approach to characterise interstitial hydro-sedimentary and associated ecological processes. Riv. Res. Appl. 37 (7), 988–1002. doi:10.1002/rra.3819
Clarke, K. R., Gorley, R. N., Somerfield, P. J., and Warwick, R. M. (2014). Change in marine communities: An approach to statistical analysis and interpretation. 3rd ed. Plymouth, UK: PRIMER-E, 144.
Clarke, K. R. (1993). Non-parametric multivariate analyses of changes in community structure. Aust. J. Ecol. 18, 117–143. doi:10.1111/j.1442-9993.1993.tb00438.x
Cooper, C. M. (1993). Biological effects of agriculturally derived surface water pollutants on aquatic systems—A review. J. Environ. Qual. 22 (3), 402–408. doi:10.2134/jeq1993.00472425002200030003x
Denic, M., and Geist, J. (2015). Linking stream sediment deposition and aquatic habitat quality in pearl mussel streams: Implications for conservation. Riv. Res. Appl. 31, 943–952. doi:10.1002/rra.2794
Diggle, P. J., Heagerty, P. J., Liang, K. Y., and Zeger, S. L. (2002). Analysis of longitudinal data. 2nd ed. Oxford, England: Oxford University Press, 398.
European Parliament (2000). Directive 2000/60/EC of the European Parliament and of the Council establishing a framework for the Community action in the field of water policy. Off. J. Eur. Union 327, 1–73.
Fischer, P., and Cyffka, B. (2014). Floodplain restoration on the upper Danube by re-establishing back water dynamics: First results of the hydrological monitoring. Erdkunde 68, 3–18. doi:10.3112/erdkunde.2014.01.02
Geist, J., and Auerswald, K. (2007). Physicochemical stream bed characteristics and recruitment of the freshwater pearl mussel (Margaritifera margaritifera). Freshw. Biol. 52, 2299–2316. doi:10.1111/j.1365-2427.2007.01812.x
Geist, J., and Hawkins, S. J. (2016). Habitat recovery and restoration in aquatic ecosystems: Current progress and future challenges. Aquat. Conserv. Mar. Freshw. Ecosyst. 26 (5), 942–962. doi:10.1002/aqc.2702
Geist, J. (2015). Seven steps towards improving freshwater conservation. Aquat. Conserv. Mar. Freshw. Ecosyst. 25, 447–453. doi:10.1002/aqc.2576
Habersack, H., Hein, T., Stanica, A., Liska, I., Mair, R., Jäger, E., et al. (2016). Challenges of river basin management: Current status of, and prospects for, the River Danube from a river engineering perspective. Sci. Total Environ. 543, 828–845. doi:10.1016/j.scitotenv.2015.10.123
Hauer, C., Leitner, P., Unfer, G., Pulg, U., Habersack, H., and Graf, W. (2018). “The role of sediment and sediment dynamics in the aquatic environment,” in Riverine ecosystem management. Editors S. Schmutz, and J. Sendzimir (Cham, Switzerland: Springer), 151–169. doi:10.1007/978-3-319-73250-3
Hoess, R., and Geist, J. (2020). Spatiotemporal variation of streambed quality and fine sediment deposition in five freshwater pearl mussel streams, in relation to extreme drought, strong rain and snow melt. Limnologica 85, 125833. doi:10.1016/j.limno.2020.125833
Kløve, B., Ala-Aho, P., Bertrand, G., Boukalova, Z., Ertürk, A., Goldscheider, N., et al. (2011). Groundwater dependent ecosystems. Part I: Hydroecological status and trends. Environ. Sci. Policy 14 (7), 770–781. doi:10.1016/j.envsci.2011.04.002
Knott, J., Mueller, M., Pander, J., and Geist, J. (2019). Effectiveness of catchment erosion protection measures and scale-dependent response of stream biota. Hydrobiologia 830, 77–92. doi:10.1007/s10750-018-3856-9
Kuhn, J., Casas-Mulet, R., Pander, J., and Geist, J. (2021). Assessing stream thermal heterogeneity and cold-water patches from UAV-based imagery: A matter of classification methods and metrics. Remote Sens. 13 (7), 1379. doi:10.3390/rs13071379
Malcolm, I. A., Youngson, A. F., and Soulsby, C. (2003). Survival of salmonid eggs in a degraded gravel-bed stream: Effects of groundwater–surface water interactions. Riv. Res. Appl. 19 (4), 303–316. doi:10.1002/rra.706
Meyer, E. I., Niepagenkemper, O., Molls, F., and Spänhoff, B. (2008). An experimental assessment of the effectiveness of gravel cleaning operations in improving hyporheic water quality in potential salmonid spawning areas. Riv. Res. Appl. 24 (2), 119–131. doi:10.1002/rra.1051
Mueller, M., Pander, J., and Geist, J. (2018). Comprehensive analysis of > 30 years of data on stream fish population trends and conservation status in Bavaria, Germany. Biol. Conserv. 226, 311–320. doi:10.1016/j.biocon.2018.08.006
Nagel, C., Mueller, M., Pander, J., and Geist, J. (2020). Making up the bed: Gravel cleaning as a contribution to nase (Chondrostoma nasus L.) spawning and recruitment success. Aquat. Conserv. Mar. Freshw. Ecosyst. 30, 2269–2283. doi:10.1002/aqc.3458
Needelman, B. A., Kleinman, P. J., Strock, J. S., and Allen, A. L. (2007). Drainage Ditches: Improved management of agricultural drainage ditches for water quality protection: An overview. J. Soil Water Conserv. 62 (4), 171–178.
Nettleton, D. (2014). Commercial Data Mining. Processing, analysis and modeling for predictive analytics projects. New York, USA: Elsevier, 287.
Pander, J., Casas-Mulet, R., and Geist, J. (2022b). Hydropeaking impairs upstream salmonid spawning habitats in a restored Danube tributary. Riv. Res. Appl., 1–12. doi:10.1002/rra.3953
Pander, J., and Geist, J. (2016). Can fish habitat restoration for rheophilic species in highly modified rivers be sustainable in the long run? Ecol. Eng. 88, 28–38. doi:10.1016/j.ecoleng.2015.12.006
Pander, J., and Geist, J. (2013). Ecological indicators for stream restoration success. Ecol. Indic. 30, 106–118. doi:10.1016/j.ecolind.2013.01.039
Pander, J., and Geist, J. (2010b). Salmonid-egg floating boxes as bioindication for riverine water quality and stocking success. J. Fish. Biol. 76 (10), 2584–2590. doi:10.1111/j.1095-8649.2010.02645.x
Pander, J., and Geist, J. (2010a). Seasonal and spatial bank habitat use by fish in highly altered rivers – A comparison of four different restoration measures. Ecol. Freshw. Fish. 19, 127–138. doi:10.1111/j.1600-0633.2009.00397.x
Pander, J., and Geist, J. (2018). The contribution of different restored habitats to fish diversity and population development in a highly modified river: A case study from the river günz. Water 10 (9), 1202. doi:10.3390/w10091202
Pander, J., Habersetzer, L., Casas-Mulet, R., and Geist, J. (2022a). Effects of stream thermal variability on macroinvertebrate community: Emphasis on native versus non-native gammarid species. Front. Environ. Sci. 10, 869396. doi:10.3389/fenvs.2022.869396
Pander, J., Mueller, M., and Geist, J. (2015b). A comparison of four stream substratum restoration techniques concerning interstitial conditions and downstream effects. Riv. Res. Appl. 31, 239–255. doi:10.1002/RRA.2732
Pander, J., Mueller, M., and Geist, J. (2015a). Succession of fish diversity after reconnecting a large floodplain to the upper Danube River. Ecol. Eng. 75, 41–50. doi:10.1016/j.ecoleng.2014.11.011
Pander, J., Mueller, M., Knott, J., Egg, L., and Geist, J. (2017). Is it worth the money? The functionality of engineered shallow stream banks as habitat for juvenile fishes in heavily modified water bodies. Riv. Res. Appl. 33 (1), 63–72. doi:10.1002/rra.3065
Pander, J., Schnell, J., Sternecker, K., and Geist, J. (2009). The ‘egg sandwich’: A method for linking spatially resolved salmonid hatching rates with habitat variables in stream ecosystems. J. Fish. Biol. 74, 683–690. doi:10.1111/j.1095-8649.2008.02145.x
Pedersen, M. L., Kristensen, E. A., Kronvang, B., and Thodsen, H. (2009). Ecological effects of re introduction of salmonid spawning gravel in lowland Danish streams. Riv. Res. Appl. 25, 626–638. doi:10.1002/rra.1232
Poff, N. L., Brinson, M. M., and Day, J. W. (2002). Aquatic ecosystems and global climate change, 44. Arlington, VA, USA: Pew Center on Global Climate Change, 1–36.
Roni, P., Pess, G. R., Beechie, T. J., and Hanson, K. M. (2014). “Fish-habitat relationships and the effectiveness of habitat restoration,” in NOAA tech. Memo. NMFS-NWFSC-127 (USA: U.S. Dept. Commer, Silver Spring), 169.
Serigstad, B. (1987). Oxygen uptake of developing fish eggs and larvae. Sarsia 72 (3-4), 369–371. doi:10.1080/00364827.1987.10419739
Shackle, V. J., Hughes, S., and Lewis, V. T. (1999). The influence of three methods of gravel cleaning on Brown trout, Salmo trutta, egg survival. Hydrol. Process. 13 (3), 477–486. doi:10.1002/(SICI)1099-1085(19990228)13:3<477::AID-HYP751>3.0.CO;2-%23
Smialek, N., Pander, J., and Geist, J. (2021). Environmental threats and conservation implications for Atlantic salmon and Brown trout during their critical freshwater phases of spawning, egg development and juvenile emergence. Fish. Manag. Ecol. 28 (5), 437–467. doi:10.1111/fme.12507
Smiley, P. C., Gillespie, R. B., King, K. W., and Huang, C. H. (2008). Contribution of habitat and water quality to the integrity of fish communities in agricultural drainage ditches. J. Soil Water Conserv. 63 (6), 218–219. doi:10.2489/jswc.63.6.218A
Stammel, B., Cyffka, B., Geist, J., Müller, M., Pander, J., Blasch, G., et al. (2012). Floodplain restoration on the upper Danube (Germany) by re-establishing water and sediment dynamics: A scientific monitoring as part of the implementation. Riv. Res. Appl. 20, 55–70. doi:10.1127/1868-5749/2011/020-0033
Stammel, B., Fischer, P., Gelhaus, M., and Cyffka, B. (2016). Restoration of ecosystem functions and efficiency control: Case study of the Danube floodplain between Neuburg and ingolstadt (Bavaria/Germany). Environ. Earth Sci. 75, 1174. doi:10.1007/s12665-016-5973-y
Sternecker, K., and Geist, J. (2010). The effects of stream substratum composition on the emergence of salmonid fry. Ecol. Freshw. Fish. 19, 537–544. doi:10.1111/j.1600-0633.2010.00432.x
Sternecker, K., Wild, R., and Geist, J. (2013). Effects of substratum restoration on salmonid habitat quality in a subalpine stream. Environ. Biol. Fish. 96, 1341–1351. doi:10.1007/s10641-013-0111-0
Keywords: brown trout, streambed colmation, fish egg survival, river restoration, groundwater ditches, cold-water refugia, aquatic conservation, climate change
Citation: Pander J, Casas-Mulet R and Geist J (2023) Contribution of a groundwater-influenced hinterland drainage system to the restoration of salmonid spawning grounds at the upper river Danube. Front. Environ. Sci. 11:1124797. doi: 10.3389/fenvs.2023.1124797
Received: 15 December 2022; Accepted: 28 February 2023;
Published: 13 March 2023.
Edited by:
Jingfu Wang, Institute of Geochemistry (CAS), ChinaReviewed by:
Qingguang Li, Guizhou University, ChinaCopyright © 2023 Pander, Casas-Mulet and Geist. This is an open-access article distributed under the terms of the Creative Commons Attribution License (CC BY). The use, distribution or reproduction in other forums is permitted, provided the original author(s) and the copyright owner(s) are credited and that the original publication in this journal is cited, in accordance with accepted academic practice. No use, distribution or reproduction is permitted which does not comply with these terms.
*Correspondence: Juergen Geist, Z2Vpc3RAdHVtLmRl
Disclaimer: All claims expressed in this article are solely those of the authors and do not necessarily represent those of their affiliated organizations, or those of the publisher, the editors and the reviewers. Any product that may be evaluated in this article or claim that may be made by its manufacturer is not guaranteed or endorsed by the publisher.
Research integrity at Frontiers
Learn more about the work of our research integrity team to safeguard the quality of each article we publish.