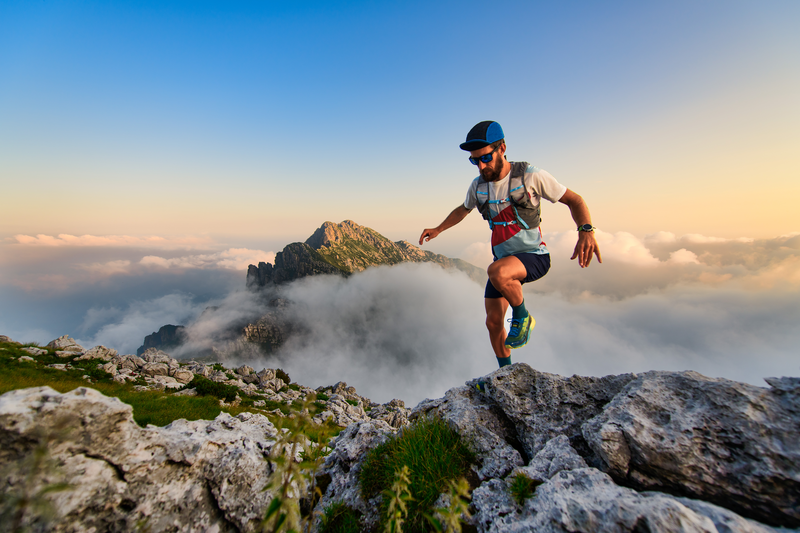
94% of researchers rate our articles as excellent or good
Learn more about the work of our research integrity team to safeguard the quality of each article we publish.
Find out more
REVIEW article
Front. Environ. Sci. , 16 February 2023
Sec. Soil Processes
Volume 11 - 2023 | https://doi.org/10.3389/fenvs.2023.1114752
With the global food deficit increasing and rising climate change issues, there is a need to find green solutions to improve soil fertility and productivity while enhancing soil biochemical quality and reducing the ecological impact of agriculture. Biochar is a potentially cost-effective, carbonaceous resource with many agricultural and environmental applications. As a soil amendment, it improves soil physical and biochemical properties and increases soil fertility and productivity—particularly over the long-term—increasing soil aggregation, water retention, pH, and microbial activities, thus, improving overall soil quality, potentially helping to reduce chemical fertilizer needs over time. The extent of biochar’s impact on soil physiochemical properties varies depending on biochar source, type, size, inherent soil characteristics, cropping system, etc. Moreover, biochar has significant potential in soil and water remediation, especially through its unique adsorption and chemical properties capable to capture and immobilize pollutants such as metal(loid)s, organic pollutants, and hazardous emerging contaminants such as microplastics. Further, biochar has also emerged as a key strategic, cost-effective material to tackle global issues such as climate change mitigation, reducing the net greenhouse gas emission to minimize global warming potential. However, a knowledge gap remains as to understanding the long-term persistence of biochar on agroecosystem, optimal biochar application rate for the diversity of biochar-soil-crop-environmental conditions, interaction of biochar with inherent soil carbon stock, specific mechanisms of biochar’s effect on soil biotic properties, quantification of carbon sequestration, greenhouse gas emissions, synergy or potential antagonistic effects with other carbon sources such as compost, manure, residues, etc., its modification for environmental applications and associated environmental and human risks over long-term. Further research is needed to evaluate the long-term impacts of types and sizes of biochar on overall soil quality to recommend suitable application practices based on soil management and cropping system. Also, its environmental applications need to be finetuned for wider and target specific applications to tackle pressing environmental issues such as soil and water pollution.
Sustaining agricultural productivity is a significant challenge globally due to the accelerated depletion of soil organic matter and nutrients. With the population continuing to rise—reaching a landmark figure of 8 billion recently—contemporary agroecosystem faces a significant hurdle to keep producing more food while protecting its most valuable resource—soil (Kopittke et al., 2019; Ortiz-Bobea et al., 2021). Soil provides almost 99% of the food for human (Kopittke et al., 2019) while providing numerous ecosystem functions (McBratney et al., 2017); therefore, degradation of soil fertility and productivity threatens food security universally (Ortiz-Bobea et al., 2021). A looming climate change issue amplifies this challenge (Kilian et al., 2021; Ortiz-Bobea et al., 2021), demonstrated by rising temperatures (Arnell et al., 2019), atmospheric carbon dioxide levels (Walker et al., 2021) and extreme weather events such as droughts (Cook et al., 2018; Mukherjee et al., 2018), further confronting the agricultural production systems (Barry and Hoyne, 2021; Priestley et al., 2021; Biesbroek et al., 2022; Pörtner and Roberts, 2022). Food security is likely to be further challenged after the COVID pandemic (Laborde et al., 2020) and global unrest worldwide, (Ben Hassen and El Bilali, 2022). In such a scenario, it becomes crucial for present agriculture to adapt and innovate to constantly improve resource use efficiency and agricultural productivity while minimizing the environmental repercussions of farming. Due to its multidisciplinary application potential, biochar is at the forefront of material innovation to tackle global food insecurity, looming climate change issues and address environmental issues (Oni et al., 2019; Das et al., 2021).
Biochar is a pyrolyzed mass produced through high-heat pyrolysis of organic matter such as plant residues in a deoxygenated environment (Lehmann, 2007; Ahmed et al., 2016a; Weber and Quicker, 2018; Ge et al., 2020). While there are different processes to produce biochar—mainly with variation in temperature, pyrolysis time, moisture, and raw material, the most common method to produce biochar industrially for agricultural and environmental use is through the slow pyrolysis or organic feedstock (350°C–700°C) in an oxygen-limiting environment. Biochar can be prepared from different organic raw materials such as agricultural by-products (rice husk, corn stalks, forage, crop, and root residues), agroforestry sources (tree bark, wood, sawdust, shells, and roots), livestock-based sources (animal manure, beddings), industrial by-products (bagasse, carbon black), agro-urban wastes (biosolids, sewage) and almost any carbon-containing stocks (Das et al., 2021). Cellulose and lignin are the principal component of biochar (produced from vegetal biomass) that is high in carbon content and low in hydrogen and oxygen. The development and application of different forms of biochar and nano-biochar and biochar-based nanocomposites have received increased attention in recent years as a soil conditioners to reduce soil bulk density and increase soil porosity and water/nutrient holding due to their functional groups, pore properties, surface activity and high adsorption capacity (Hagemann et al., 2017; Liu et al., 2018).
While biochar is produced through the pyrolysis of carbon feedstock, it has several nuances. The slower the pyrolysis process, the slower the heating rate and the longer the residence time for biochar production (Ge et al., 2020). In general, in pyrolysis at higher temperatures (600°C–700°C), there is an increase in fixed carbon content, alkalinity, and amount of basic functional groups present, while at the lower temperature (100°C–300°C), there is an increase in porosity, adsorption capacity, amount of acidic functional groups and biochar yield (Sun et al., 2017). In recent years, more innovative ways, such as microwave vacuum pyrolysis (i.e., pyrolysis using a microwave in a vacuum environment), have been reported to reduce fossil fuel usage in conventional pyrolysis (Ge et al., 2020). This method has several advantages, especially in overcoming the low inherent porosity of biochar, to use it as an adsorbent for environmental remediation. Especially with slight modification and improvisation such as steaming, biochar produced with microwave vacuum pyrolysis has been shown to be an adsorbent for soil herbicides such as 2,4 2,4-dichlorophenoxyacetic acid (2,4 D) (Lam et al., 2019).
This review reports comprehensively the development, advances, and prospects of biochar applications, including the knowledge gaps remaining in biochar’s agricultural and environmental applications (Figure 1).
Soil amendment with biochar is regarded as one of the important ways to improve soil nutrient and water retention and promote soil ecological functioning, as well as a significant tool to combat climate change issues by storing the CO2 back in the earth(Woolf et al., 2010; Lorenz and Lal, 2014; Han et al., 2022). Because of the highly stable carbon content with high porosity and specific surface area, its application can provide a multitude of benefits as a soil amendment (Lehmann, 2007; Oni et al., 2019; Das et al., 2021; Zhang et al., 2021). It has been reported that biochar improves the soil physical, chemical, and biological properties of soil (Joseph et al., 2010; Burrell et al., 2016; Zhang et al., 2021)—particularly over a long-term application (Joseph et al., 2021)—therefore enhancing agricultural productivity (Diatta et al., 2020). Also, the greatly porous carbonaceous material with a high specific surface area can be used as soil amendments to sorb organic as well as inorganic contaminants such as herbicides and pesticide residues, metal(oid)s and newly emerging pollutants such as microplastics, thereby improving the soil quality for agricultural and environmental use (Joseph et al., 2010; Hardie et al., 2014; Ahmed et al., 2016a; Burrell et al., 2016; Amonette et al., 2021; Das et al., 2021; Islam et al., 2021). The application of biochar as soil amendment can be discussed as its effect in amending soil physical, chemical and biological characteristics, as well as a combined effect leading to soil health and increased crop productivity (Figure 2).
FIGURE 2. Biochar in soil physico-chemical properties (soil health) and environmental attributes (Biochar-Soil-Crop-Environment nexus).
In general, biochar’s advantage is well demonstrated for coarse-textured, sandy soils as they improve the soil physical properties, increase water retention, and reduces bulk density(Das et al., 2021; Joseph et al., 2021). Besides the soil type, the feedstock used to make biochar affects how effective it is as a soil amendment. One of the most significant effects of biochar applied as a soil amendment is the improvement of soil aggregate stability (Herath et al., 2013; Liu et al., 2014; Burrell et al., 2016; Du et al., 2017; Bai et al., 2019; Zhang Q. et al., 2020; Islam et al., 2021; Yan et al., 2022). Wood chip, wheat straw, and vineyard pruning biochar (pH 8.3–9.7, application rate 3% by soil weight) improved the soil structure and aggregate stability in a 1-year crop (mustard-red clover) and 2 years of fallow, with the effect being more pronounced on coarse-textured soil compared to fine textured soil (Burrell et al., 2016). 6-year of continuous straw and straw-derived biochar amendment increased the soil aggregation in the top 0–40 cm in a rice-wheat rotation system (Bai et al., 2019; Zhang Q. et al., 2020). Wheat straw biochar (pH 10.35, application rate 2–40 t ha-1) applied in upland red soil for 1 year in a rapeseed-potato cultivation system increased the soil aggregate stability, microaggregate content, while increasing the rapeseed and sweet potato yields (Liu et al., 2014). The application of biochar has also been reported to improve soil water retention through increased pore size and aggregate stability (Hardie et al., 2014; Rasa et al., 2018; Wang et al., 2019; Razzaghi et al., 2020; Kang et al., 2022). Another advantage of biochar amendment can be the improvement in soil bulk density (Bhattarai et al., 2015; Ahmad Bhat et al., 2022) and reduction of soil compaction, although the impact seems to be short-lived (< 2 years) and at higher rates of biochar application (>10 ton ha-1) (Blanco-Canqui, 2021) and more effective in sandy soils with small size biochar (Verheijen et al., 2019). Biochar derived from crop residues, have a more pronounced effect in improving soil bulk density and reducing soil compaction (Bhattarai et al., 2015; Blanco-Canqui, 2021). Long-term experiments have often reported minimal change in soil physical characteristics compared to the lab and short-term field experiments (Blanco-Canqui, 2017; Islam et al., 2021). Compared to the biochar control and the larger particle size fraction, the biochar amendment with small and medium particle size considerably decreased the bulk density of the soil and enhanced overall porosity, soil pH, and electrical conductivity (Zeeshan et al., 2020). It can be generally reported that biochar typically improves the soil physical environment, more so in sandy (coarse-textured) soils over long-term applications (Blanco-Canqui, 2017); while more long-term application rates are necessary to conclusively quantify the effect on different indicators exclusively to determine the management approach suitable for the soil-crop system.
As soon as biochar is incorporated into the soil, it starts to interact with soil chemistry, starting with dissolution (<1 month), development of reactive surfaces (1–6 months), and degradation or aging (> 6 months) (Joseph et al., 2021). In addition to increasing soil fertility, particularly over a long term, its ability to store carbon and nitrogen may lessen both immediate and long-term environmental deterioration and its harmful impacts on human and animal health (Verheijen et al., 2019). Biochar functions as an excellent adsorbent for nutrients and pollutants because of the surface characteristics and attached functional groups (Carey et al., 2015; Gong et al., 2019; Yuan et al., 2019). Consequently, biochar improves the soil’s ability to absorb and hold onto nutrients and agricultural chemicals while lowering their vaporization and leaching into surface and groundwater, enhancing nutrient retention in soil. Over time and through weathering and decomposition, biochar may contribute crucial nutrients to the soil solution. As mentioned previously, biochar enhances the soil physical environment by lessening the bulk density of the soil (compaction), improving water retention, drainage, aeration, and root penetration, as well as the soil’s ability to retain water and workability, thanks to its low density and up to highly porous structure (Downie et al., 2012). Biochar, through its persistence, enables a long-lasting impact on soil quality and carbon sequestration due to its greater half-life of carbon, potentially over thousands of years (Lehmann et al., 2003; Oliveira et al., 2017). Due to its potential for decreased nutrient losses (Joseph et al., 2010; Zimmerman et al., 2011; Ahmad Bhat et al., 2022)) and higher fertilizer efficiency, biochar has been a strategic tool for agro-environmental applications (Lehmann et al., 2003; Lehmann, 2007; Amonette et al., 2021; Joseph et al., 2021) (Lehmann and Joseph, 2015). Its integration into poor soil enhances soil fertility, crop development, and productivity more than non-biochar soil (Steiner et al., 2007; Major et al., 2010; Zeeshan et al., 2020). However, these factors heavily rely on the origin and properties of the applied biochar (Zimmerman et al., 2011; Enders and Lehmann, 2012; Bhattarai et al., 2015; Blanco-Canqui, 2017; Weber and Quicker, 2018), as well as soil characteristics, environmental plant responses, biochar-soil interactions, and its ability to absorb nutrients (Herath et al., 2013; Joseph et al., 2021; Ahmad Bhat et al., 2022). It may have an impact on crop development both directly and indirectly depending on its natural nutritional content and capability for nutrient and water retention as well as the reduction of moisture stress (Karhu et al., 2011; Vaccari et al., 2011; Verheijen et al., 2019; Diatta et al., 2020; Kang et al., 2022). In some cases, it is possible to anticipate poor crop performance for the first 30 days following cultivation on acidic soil (pH = 5.5), which may be caused by short-term N retention in N-deficient soil (Clough et al., 2013; Joseph et al., 2021). According to Widowati et al. (2014), rice husk biochar caused significant N leaching, whereas wood biochar had the maximum water retention and minimized NO3−1 leaching within the first 30 days following application Jones et al. (2011, 2012) claim that biochar alters soil physical characteristics like bulk density, soil organic matter breakdown, and the organic carbon liberation, suggesting a potential method for soil carbon sequestration. It effectively delivers nutrients into the soil while reducing nutrient leaching (Biederman and Harpole, 2013). With biochar amendment, soil contained superior extractable nutrients (Laird et al., 2010) and fertility (Ali et al., 2022b). Biochar amendment with small and medium particle size considerably decreased the soil pH and electrical conductivity (Zeeshan et al., 2020). Additionally, the tiny particle size fraction greatly enhanced the soil’s organic matter (OM) content and saturation (Gorovtsov et al., 2020; Han et al., 2022).
Overall, due to its chemistry and properties, biochar can be used to increase agricultural production, especially in soils with low fertility and soil degradation, where it can be especially beneficial. Biochar made from biomass waste has been linked to better soil water-holding capacity as well as decreased run-off of agricultural pesticides and fertilizers (Woolf et al., 2010; Verheijen et al., 2019). It has improved crop yield by increasing soil base saturation (Glaser et al., 2002; Major et al., 2010), increasing soil water holding capacity (Karhu et al., 2011; Blanco-Canqui, 2017; Verheijen et al., 2019; Razzaghi et al., 2020), and retaining nutrients in the portion of the soil column containing roots and enhancing nutrient use efficiency (Steiner et al., 2007; Laird et al., 2010).
The effect of biochar on soil physical and chemical properties across different experiments has been summarized in Table 1.
Biochar have been reported to improve the key soil biological properties, mainly owing to their alkaline pH and structure, improving the soil porosity, aggregation, and water-holding capacity that promotes soil nutrient bioavailability and microbial growth (Lehmann, 2007; Warnock et al., 2007; Joseph et al., 2010; Lehmann et al., 2011; Beheshti et al., 2018; Gorovtsov et al., 2020). While biochar is often considered an apt soil amendment for long-term application use, it can interact with soil biological properties such as plant roots and root microbes as early as it is applied in the soil (Joseph et al., 2010), even on soils contaminated with microplastics (Palansooriya et al., 2022) and metal(loid)s (Gorovtsov et al., 2020). The precise mechanism by which biochar directly affects the soil microorganisms remains unclear, although a synergistic effect in increasing overall soil physical, chemical, and biological properties with biochar, particularly soil pH, aggregation, and water retention, is often attributed as key factors in promoting soil microbe growth, increase in soil biomass carbon, soil respiration, and microbial diversity (Joseph et al., 2010, 2021; Lehmann et al., 2011; Beheshti et al., 2018; Diatta et al., 2020; Gorovtsov et al., 2020).
In fact, biochar has been attributed to increasing crop’s systemic resistance and even helping to control soil pathogens by improving soil biological quality (Tan et al., 2022). Biochar has also been attributed to improving the soil mycorrhizal population through the improvement of soil carbon sequestration and the addition of soil fertility (Warnock et al., 2007). Also, for beneficial soil bacteria to flourish and perform, biochar-treated soil may provide a better environment. Inoculating lentils with rhizobia considerably improved soil organic matter (SOM), micronutrient fertility (Fe, Zn, Mn, and Cu), and soil qualities (Ali et al., 2022a). These advantages could change depending on the feedstock and pyrolysis temperature (Sun et al., 2017; Huang et al., 2018; Wang et al., 2021). The biochar particle size fraction may, however, significantly influence soil properties. As it stands, although there is an acknowledgment that biochar improves the soil biological environment, mainly driven by biochar’s alkaline pH and soil aggregating capacity (Lehmann et al., 2011; Joseph et al., 2021), the impact of biochar as a soil amendment is more focused on soil physical and chemical properties particularly in improving soil fertility and quality, but soil biological impact, particularly on soil microorganisms and fauna is a significant aspect that needs to be explored more in the future.
There has been growing interest in the concept of soil health, which has been as “the continued capacity to function as a vital living ecosystem that sustains plants, animals, and humans while maintaining or enhancing water and air quality” (Doran and Zeiss, 2000; Stott, 2019; Lehmann et al., 2020). This definition emphasizes soil management so that they are available for future generations. Physical (e.g., soil structure, aggregate stability, etc.), chemical (pH, electrical conductivity (EC), cation exchange capacity (CEC), nutrient cycling and holding capacity, etc.), and biological indicators (microbial biomass and activity, community structure) are used to evaluate different soil management—all of which can be significantly affected with biochar application as discussed above (Cardoso et al., 2013; Das et al., 2021). Biochar can be used as a soil amendment to enhance the soil quality to have optimal biological activity with enhanced nutrient bioavailability for plants, improved structural aggregation, enhanced water holding capacity, and beneficial microbial activity (Doran and Zeiss, 2000; Das et al., 2021; Tan et al., 2022). Soil organic matter (SOM) and clay minerals that act as storage and exchange sites for nutrients are key components of soil health, significantly affected as early as biochar is incorporated in the soil, thereby affecting soil health (Joseph et al., 2010; Amonette et al., 2021). Soils that have enriched microbial diversity and activity are often more resilient to management practices, which have been demonstrated across different soils with biochar applications (Beheshti et al., 2018; Wang et al., 2019; Diatta et al., 2020; Palansooriya et al., 2022). Biochar plays a key role in managing soil health through mobilizing soil microbes are a vital component of soil health as they’re involved in several critical processes, including the decomposition of organic substrates to form SOM, biogeochemical nutrient cycling and mineralization, soil structure, and aggregation, disease suppression, regulation of plant growth-promoting hormones, greater soil water holding and availability to plants, neutralization of toxic compounds and metal(loid)s to plants (Lehmann et al., 2011; Liu et al., 2016; Gorovtsov et al., 2020; Amonette et al., 2021; Tan et al., 2022). Microbial biomass, respiration, and enzymatic activity are vital to maintaining healthy soil structure and having aggregate stability, which can be amended in soils by applying biochar. These soil organic carbon, soil structure, and other biochemical soil properties drive microbial abundance, and community composition in soil biological activity from soil microbes mediated with biochar is essential to reverse the fixation of nutrients through mineralization, increasing the availability of nutrients required by plants (Jones et al., 2012; Beheshti et al., 2018).
One of the most important aspects of biochar application is its potential for environmental remediation. Its unique property makes it a useful remediation material in soil, water, and gas medium, which makes it a highly versatile resource (Oliveira et al., 2017). Because biochar can be produced locally and commercially from a wide variety of feedstock, including crop residues, wood chips, animal manure, solid byproducts, and so on, it is a relatively low-cost option for use as an absorbent in soil and water. Recently, with technology that can optimize the production of biochar that contains desired physical and chemical properties, biochar can be optimized for multitude of environmental application, which includes treatment of soil pollution, targeted removal of metal(loid)s and contaminants from soil, solid waste management and wastewater treatment (Zhou et al., 2021).
Soil contamination with organic pollutants and metal(loid)s is increasingly becoming a global problem (Zhang et al., 2013; Yuan et al., 2019) One of the significant research and application of biochar is as a soil amendment to improve soil quality, especially ideal for marginal and poor-quality soils through its unique characteristics. For the agro-ecosystem application context, biochar is often used as a soil amendment; therefore, traditionally, maximizing the carbon content is considered the most important factor (Joseph et al., 2010; 2021; Diatta et al., 2020). Besides, its structure, sizes, pH range, particle size, specific surface area, porosity, etc., are among the factors that play a significant role in the quality of biochar produced for soil amendment and environmental applications. The quality of biochar and its effectiveness for agricultural and environmental applications is affected by biochar’s physical (shape, size, specific surface area, pore size and distribution, stability, density, persistence, etc.), chemical (composition of elements, pH and electrical conductivity, cation exchange capacity, etc.,) and biological (cropping system, management, etc.,) properties and interactions (Joseph et al., 2010; Jones et al., 2012; Blanco-Canqui, 2017; Amonette et al., 2021). Biochar obtained through pyrolysis at higher temperatures (550°C–750°C) has been shown useful in the phytoremediation of metals such as Zn(II), Pb(II), and Cd(II) through converting them into oxide compounds (Huang et al., 2018). Use of microwave steam activation to produce biochar from palm shells, Lam et al. (2019) demonstrated the application of biochar as a treatment for hazardous leachates in landfills, achieved through significantly increased adsorption capacity. However, biochar’s ability for adsorption depends on its reactive surface area, pH, surface groups, ion-exchange capacity and pore size distribution (Kołodyńska et al., 2012; Zhang et al., 2013; Qiu et al., 2022).
One of the mechanism biochar helps in metal and organic pollutant contamination is through reducing their bioavailability in soil (Qiu et al., 2022). Biochar—through its alkaline pH helps to raise soil pH and stabilize metals—potentially reducing the bioavailability and leachability of heavy metals and organic pollutants in soils through high adsorption and different physicochemical reactions (Zhang et al., 2013). In fact, higher the specific surface area of biochar with a high variable charge component, the greater the surface sorption capacity that will affect the soil’s ability to retain water, nutrients, and organic molecules (Brown et al., 2006; Zhang et al., 2013). The surface oxygenation of biochar, when it is applied to soil, changes the O2-containing functional groups (carboxyl, OH, phenol, and carbonyl groups) on the vast interior surface area of the biochar, inducing an induced negative charge and raising the soil pH and cation exchange capacity (CEC) (Cheng et al., 2006). Biochar-related cation release into the soil reduces soil acidity and may significantly alter the soil’s N (NH4+ and NO3−) and AB-DTPA extractable P and K contents (Chintala et al., 2014). Biochar can help stabilize metal(loid)s through sorption by exchange of cations associated with biochar such as Ca2+, Mg2+, surface complexation with different functional groups and inter-spherical complexation with free hydroxyl of mineral oxides and simply physical adsorption leading to surface precipitation (Zhang et al., 2013; Qiu et al., 2022). Besides the mineral components present in biochar such as phosphates and carbonates can also help in stabilizing of metal(loids) (Cao et al., 2009; Kołodyńska et al., 2012). Recently, modification of biochar with metal oxides or biochar-nanocomposites has been reported to further improve adsorption of contaminants, however concern of them persisting and becoming untoward in the ecosystem remains to be duly studied (Zhang A. et al., 2020).
In addition, carbon in biochar, which is highly stable, can be sequestered for more than 1,000 years once applied to the soils which can contribute to climate change mitigation through sequestration of recalcitrant carbon in soil (Lai et al., 2013; Lin et al., 2015; Yang et al., 2020; Shin et al., 2021). Potential to increase soil quality by adding fertility and soil amendment while providing an alternative for carbon sequestration in the soil makes biochar a suitable prospect for sustainable agriculture and climate management (Woolf et al., 2010; Lorenz and Lal, 2014). Because of its neutral characteristics that consist of carbon with high persistence in soil, it can be an effective tool to mitigate climate change through the sequestration of atmospheric carbon dioxide while potentially improving soil fertility (Lorenz and Lal, 2014; Gross et al., 2021). Recent report has shown that over 920 kg of CO2 equivalent can potentially be sequestered throughout the country when just one ton of crop residue is converted into biochar (Yang et al., 2021). In coastal saline conditions, biochar derived from corn stalks applied at the rate of 16-ton ha-1 significantly increased the carbon sequestration (−3.84 – −3.17 t CO2-eq. ha−1 t−1C) without increasing the greenhouse gases emissions significantly while increasing soil fertility (Lin et al., 2015). Application of biochar at the rate of 2%–5% has been reported to increase the carbon sequestration significantly by 46%–58% from rice and beet fields (Lai et al., 2013). Biochar derived from apple wood residues and re-applied in apple orchards promoted the soil organic carbon sequestration, which increased by 316.52%–354.78% in a 2-year study in the Loess Plateau of China (Han et al., 2022). Soil carbon sequestration was increased significantly (1.87–13.37 t C/ha) under wood-derived biochar application, compared to rice straw application which reduced carbon sequestration, driven by highly recalcitrant carbon contained in biochar vs. labile form in rice straw (Thammasom et al., 2016).
In addition, higher rate of biochar application (30 t ha-1) have been reported to increase the carbon sequestration potential in corn fields grown under drip irrigation and mulching system by augmented soil organic carbon (SOC) sequestration in upper 15-cm soil depth by 16% (Yang et al., 2020), although such high application rates may not be feasible in an agricultural context. The application of biochar-activated fertilizer pellets increased the soil carbon sequestration potential to 1.23 t ha-1 while increasing rice production (Shin et al., 2021). Further, with agricultural land diminishing, growing a high carbon dioxide capturing aquatic crop such as algae in photobioreactors or ponds, converting it into biochar, and applying it back to soil can not only aid in soil quality but also help in significant CO2 sequestration (Roberts et al., 2015; Cole et al., 2017; Mona et al., 2021). A recent global meta-analysis study of global biochar application has revealed that biochar, when applied without and together with chemical fertilization, enhanced the soil organic carbon (SOC) sequestration potential by 32%–35% (Xu et al., 2021), rising to 40% (Liu et al., 2016). The results suggest that the biochar of higher pH (8–9) had a more positive increase of SOC sequestration, although a significant variation existed depending on the soil properties, land-use type, agricultural practice, and other biochar characteristics (Liu et al., 2016). Overall, results indicate a more prominent potential of biochar in soil carbon sequestration from lab-based studies, with lesser effect in field conditions, due to differential interactions and management practices (Liu et al., 2016; Xu et al., 2021).
Recent studies have elucidated that the application of biochar as a soil amendment can contribute to reducing greenhouse gas emissions from soil to the atmosphere (Yang et al., 2020; Gross et al., 2021; Shin et al., 2021; Xu et al., 2021). Applying wood chip biochar in rice and beet fields has been reported to significantly reduce greenhouse gas (N2O and CO2) emissions when used at 2%–5% in the soil (Lai et al., 2013). One of the mechanisms through which biochar aids in reducing greenhouse gas emissions (N2O reduction of 20%–30% in dry conditions and 40%–50% in wet vegetated conditions) from agricultural soil is indirectly through increasing soil moisture retention and plant N uptake (Saarnio et al., 2013). Biochar, when applied at higher rates (30 t ha-1), decreased the methane (CH4) gas emission by 134% in corn fields grown under drip irrigation and mulching (Yang et al., 2020). Recently, fertilizers activated with biochar in the form of pellets have been reported to reduce the emission of greenhouse gases (N2O emission lowered, no significant change in CH4 emission) in a rice cultivation system through reducing nitrogen mineralization and nitrification processes (Shin et al., 2021). A high amount of recalcitrant carbon present in wood-derived biochar, especially compared to carbon sources containing more labile carbon such as rice straw, is key in mitigating greenhouse gas (GHG) emissions enabling the reduction of greenhouse gas (CO2 and CH4) emission intensity (Thammasom et al., 2016). Applying biochar enriched with nitrogen at 4 ton ha-1 promoted rice yields while reducing greenhouse gas emissions (Yin et al., 2021). Biochar derived from apple wood residues and re-applied in apple orchards reduced the net greenhouse gas emission (net global warming potential) by 368.93%–480.91% in a 2-year study in the Loess Plateau of China (Han et al., 2022). A meta-analysis of global biochar application has revealed that biochar, when applied without and with chemical fertilization, reduced the global warming potential by 27.1% and 14.3%, respectively, by reducing the net greenhouse emissions (Xu et al., 2021).
One of the emerging biochar applications is in water pollution management, especially the treatment of contaminated wastewater from different sources. This could be achieved through pre-treatment of substrates (feedstocks) as well as post-treatment for contaminant removal, with engineered biochar with higher porosity, specific surface area, abundant functional groups, elevated adsorption capacity, etc., Often being more effective than pristine organic biochar (Xiang et al., 2020). The use of biochar derived from coconut shells has been reported to improve the Pb contamination significantly from lead-contaminated water through the complexation of Pb in the surface and porous structure of biochar (Hao et al., 2021). KOH-activated highly porous biochar has been found effective in removing contaminants such as chromium and naphthalene from wastewater. Significantly increased adsorption and removal of sulfamethoxazole was attained from wastewater using the biochar derived from hydrothermal carbonization of sugarcane bagasse, assisted with charge-assisted hydrogen bonding and π-π interaction with graphitized carbon (Prasannamedha et al., 2021). Biochar derived from bone milling enhanced the efficiency of metals Cd(II), Cu(II), and Pb(II) adsorption in aquatic systems through increased surface complexation, cation exchange, chemical precipitation, electrostatic interaction, and cation-π bonding (Xiao et al., 2020). A significant model for wastewater treatment, particularly from industrial and municipal wastes, could be growing algae which captures excess nitrogen and phosphorus from the water and aid in sewage treatment, while the biochar produced from algae can be reused as a soil amendment to increase agricultural production (Roberts et al., 2015; Cole et al., 2017; Mona et al., 2021). Using relatively inexpensive, carbon-negative, and highly adsorbent biochar has been demonstrated to remove and immobilize organic pollutants and micro and nanoplastics from wastewater (Kumar et al., 2023). The honeycomb structure of biochar can help capture and immobilize the microplastics (diameter 10–22 μm, 95%) in the wastewater, a significant emerging pollutant (Wang et al., 2020). Using biochar (straw collected from the lake) has been suggested for removing metals attached to microplastics in water systems, using biochar’s catalytic and magnetic removal properties, thereby significantly reducing the risk of microplastic contamination in drinking water (Ye et al., 2020). The microplastic contained in a water medium could also be effectively adsorbed and removed by using the magnetic properties of biochar activated with different metals (Fe, Mg, Zn, etc.), treating the wastewater effectively through a series of filtration methods (Singh et al., 2021; Wang et al., 2021; 2022; Kumar et al., 2023).
One of the important areas of biochar application moving forward could be the treatment of newly emerging highly hazardous wastes such as organic pollutants and microplastics, a critical global pollution challenge (Palansooriya et al., 2022; Kumar et al., 2023). With the extensive use of plastics in agricultural and urban settings, more microplastic residues contaminate the soil and aquatic environments; therefore, its remediation is critical to improving soil and water quality (Wang et al., 2020, 2022; Ye et al., 2020). The use of biochar (corn straw and hard wood-derived) as a filtration method has been reported to significantly (>95%) remove and immobilize microplastics of diameter 10–22 μm through capturing and entangling the microplastic particles in the extensive honeycomb structures of biochar (Wang et al., 2020). Another approach to separation and removal of microplastics from aquatic systems is catalytic removal by utilizing the magnetic biochar activating oxidation processes (Ye et al., 2020). Also, biochar produced from corncob with high pyrolysis temperature was reported to offer the important potential to adsorb polystyrene nanoplastics, achieved through pore filling, hydrophobic interactions, and hydrogen bonding (Abdoul Magid et al., 2021). Through adsorption, chemical bonding, and electrostatic attractions, biochar offers potential adsorption of micro and nanoplastics from aquatic ecosystems (Kumar et al., 2023). Another relevant approach for microplastic removal from aqueous solutions has been suggested to use the magnesium and zinc-modified magnetic biochar by utilization of magnetic and thermal degradation approaches (Wang et al., 2021). Using iron modified biochar pyrolyzed at 850 and 550°C demonstrated strong adsorption of microplastics (30–1000 nm, carboxyl, and amine functional groups) utilizing the magnetic property of biochar (Singh et al., 2021). Similarly, the application of peanut shell biochar, with or without modification with magnesium oxide metalloid, attained a significant removal (>75.53%) for microplastics from a porous medium (Wang et al., 2022).
Biochar application in soil and water remediation for metal(loids) and other contaminants such as microplastics has been summarized in Table 2.
Recently, with the advancement of technology, increased biochar engineering is being achieved to tailor biochar preparation for a specific application. This has been attained through feedstock treatment during biochar preparation, modifying the preparatory conditions (e.g., temperature), and several other physical and chemical methods to optimize functionality, pore structure, and specific surface area as desired (Ahmed et al., 2016b). The most common method of modification during preparation include steam activation (Rajapaksha et al., 2015), heat treatment (Li et al., 2014), acidic (Zhang et al., 2015) and alkaline (Ma et al., 2014) modifications, impregnation with metal salts or oxides (Agrafioti et al., 2014; Han et al., 2015; Shen et al., 2015). This modified biochar is especially used in the removal of particular contaminant(s) from the soil, water, and gaseous medium, and specific method may be the most suitable for a particular contaminant or to attain specific characteristics (Ahmed et al., 2016a; Ahmed et al., 2016b). Activation of biochar post-preparation using chemicals such as KOH has been demonstrated as an effective option to increase biochar’s adsorption capacity and porosity, thereby elevating the removal of contaminants such as chromium and naphthalene (Qu et al., 2021). By using KOH, there was an increase in the specific surface area up to 2,183.80 m2/g and well-developed micropores with an average particle size of 2.75 nm and main pore diameters distribution from 1–2 nm, also attaining higher electrostatic attraction, complexation, ion exchange and reduction action (Qu et al., 2021). Using the micro-nano technology to engineer the bone biochar through ball-milling was found to significantly increase biochar’s adsorption capacity (Xiao et al., 2020). Biochar produced specifically using bioenergy systems could increase the presence of oxidizing agents, increasing the surface functionality of biochar that helps to immobilize Pb and As contaminants from soil. Activation of fertilizer pellets with biochar has been demonstrated to reduce fertilizer needs by 60%, increasing crop yields with the potential of carbon sequestration in soil by lowering the nitrogen mineralization and nitrification processes (Shin et al., 2021). Enrichment of nitrogen with biochar helped to decrease the CO2 and CH4 gas emissions, promoting rice yield, fertilizer use efficiency, and conversion of Fe (III) to Fe(II) (Yin et al., 2021). Activation of biochar with different metals, such as Zn, Mg, Fe, etc., has been successfully elucidated to adsorb (magnetically and chemically) the microplastic contaminants from aquatic environments (Singh et al., 2021; Wang et al., 2021; 2022; Kumar et al., 2023).
As with other amendments, one of the critical challenges with biochar is that each biochar is different, hence attaining different results. Because of the diversity of biochar, it is difficult to pinpoint the key advantage of biochar blanketly, and each biochar must be treated as a unique material, and its merit must be tested for desired results (Joseph et al., 2021; Xu et al., 2021). The poor quality of raw material and the indifferent process parameters generate a low quality of biochar. Moreover, variations in soil type, crops, management practices, and external factors add another layer of complexity to its application. The rate and timing of biochar application, physical and chemical properties, and size of biochar particles also play a crucial role in determining its function in the soil-crop system (Joseph et al., 2021; Xu et al., 2021). Even when the quality of biochar is properly selected and application is carefully performed, biochar may not yield desired results, especially in the first few years or even longer (Burrell et al., 2016; Bai et al., 2019). The purpose of the application of biochar often dictates the amount of benefit it can attain in the soil. The substrate available could determine the most suitable carbon source to use in some instances (Oliveira et al., 2017; Zhou et al., 2021; Ahmad Bhat et al., 2022). For example, biochar could be the best alternative if there is an abundance of lignocellulosic (wood) feedstock from forest or orchard crops. The availability of technology and farming system also determines the necessity of carbon sources; for example, if livestock is to be fed in a system, using plant residues as animal feed and manure as biochar could be an option (Joseph et al., 2021; Xu et al., 2021).
The purpose of the application, availability of resources, and environmental and management conditions often dictate the superiority of carbon sources in an agroecosystem–in some instances—a mixture of two or more can yield the best results (Joseph et al., 2021; Xu et al., 2021). The addition of organic sources of fertilizers, such as manure, compost, etc., has the potential to add a significant amount of contaminants depending on the feedstock used, such as fertilizers, pesticides and herbicide residues, antibiotics, organic pollutants such as polycyclic aromatic hydrocarbons (PAHs) and polychlorinated biphenyls (PCBs) and trace elements such as metal(loid)s in the soil which can affect soil fertility, microbial diversity, ultimately affecting human health (Zhou et al., 2021). This can be of significant concern in an organic system with accumulation over time. In fact, if used correctly, different carbon sources can complement each other. Also, biochar derived from manures, such as poultry and farmyard manure, often has a more significant increase in soil biochemical quality and crop yield than biochar derived from crop and wood residues (Bhattarai et al., 2015). For instance, using compost with biochar can help minimize the toxicity of metal(loid)s and organic contaminants (biochar) while adding enough nutrients for crop production. Adding carbon-rich compounds such as activated carbon composite together with manure during composting can complement manure application by immobilizing metals such as Cr, Cd, Pb, and As, improving compost quality (Lin et al., 2021). Using algal-based compost and biochar together can not only help improve soil fertility (through the recovery of nitrogen and phosphorus from municipal wastewater), enabling agriculture but also help in sewage treatment and CO2 sequestration (Cole et al., 2017; Mona et al., 2021).
Despite several work reported on application of biochar for soil, water, contaminant remediation, most of the work is either conducted in lab or greenhouse, and very few in-situ application of biochar has been explored in actual ecosystem conditions (O’Connor et al., 2018). Although modification of biochar for specific applications is gaining interest, mainly to utilize the high adsorption capacity of modified biochar for contaminants remediation, the addition of modified sources of carbon (biochar) with chemical could have some eco-toxicological effect on soil-water systems, which needs to be well understood. Moreover, the application of carbon-rich compounds such as biochar has been reported to the half-life of antibiotics in compost, increasing its overall quality (Lin et al., 2021). Using biochar can be particularly useful in utilizing the secondary wastes from agriculture and wood residues (instead of burning) and preventing the potential risk of pollution. More importantly, biochar can help to effectively manage and recycle potentially hazardous waste, reducing the risks of burning or leaching from landfill sites. In contrast, in some instances, biochar can potentially yield a negative impact, essentially defeating the purpose of its use such as reducing the nutrient cycling (Clough et al., 2013; Glaser and Lehr, 2019). Concerns exist on application of biochar for long term could raise the pH and temperatures of the soil accumulated over time. It has been shown to reduce nutrient availability (Clough et al., 2013; Glaser and Lehr, 2019), affect the soil chemical properties, negatively impact the soil rhizosphere biology (Ren et al., 2018; Pei et al., 2020) and promote the accumulation of organic and inorganic pollutants in soil. Concerns remain whether biochar absorbs root exudates from plants, thereby affecting inherent soil microbiology and plant-microbe signaling and interactions (Ren et al., 2018; Pei et al., 2020). The actual carbon footprint of biochar, compared to other sources of carbon such as compost remains to be properly studied and outlined. Therefore, these issues need to be systematically researched and documented for application of biochar extensively in the future for agricultural and environmental uses.
Biochar has excellent potential in agricultural and environmental fields and can be a cost-effective, efficient method to improve soil fertility, soil quality, soil and water remediation, soil carbon sequestration, and greenhouse gas mitigation. For agricultural applications, because the positive is reliant on the type of biochar, soil properties, management practices, etc., it remains critical to treat each biochar as unique, characterize them, and test it for crop-soil management practices, particularly to apply it on a large scale. With the advancement of technology, especially nanotechnology, there is an opportunity to produce nano biochar with highly increased specific surface area and reactive potential, thereby increasing the effectivity of the carbonaceous material in a relatively short term in the field. The smaller size of biochar, reduced up to a nanoscale, could offer significant potential for environmental remediation with the tremendous increase in specific surface area for adsorption and chemical reaction of contaminants in the soil as well as water systems. However, whether this kind of nano-biochar will be suitable for soil carbon sequestration and greenhouse gas mitigation, often driven by more recalcitrant carbon in biochar, remains to be further explored. Significant progress over the years has been made in the environmental application of biochar as a low-cost, carbon-neutral source of pollution control in soil and water systems by utilizing its unique adsorption capacity. With the advancement of technology, such as nanotechnology, this potential could be further capitalized by understanding the interaction of specifically generated biochar with metal(loid)s, organic contaminants, microplastics, etc., from the soil, water, and gaseous medium. Developing the concrete protocol and contaminant capturing/filtration system could be necessary for utilizing universal biochar in wastewater treatments in municipal wastes, drinking water sources, and landfill sites. With the increasing prospect of production and use of hazardous chemicals globally, biochar can help minimize environmental risks, thereby reducing risks to human health.
A knowledge gap remains as to understanding the long-term persistence of biochar on agroecosystem, the optimal biochar application rate for the diversity of biochar-soil-crop-environmental conditions, the interaction of biochar with inherent soil carbon stock, specific mechanisms of biochar’s effect on soil biotic properties, quantification of carbon sequestration, greenhouse gas emissions, synergy or potential antagonistic effects with other carbon sources such as compost, manure, residues, etc., its modification for environmental applications and associated environmental and human risks over long-term (Amonette et al., 2021). In the future, there needs to be a more comprehensive analysis of synergies between different carbon sources, such as biochar, compost, and manure in an agricultural setting, elucidating the opportunity cost of using one source vs. another in a specific agroecosystem management approach. Specifically, comprehensive knowledge of when the biochar works and when it doesn’t need to be demonstrated across a diversity of conditions for an efficient decision-management system to assist the agronomic and environmental applications (Joseph et al., 2021). Identifying soil quality indicators affected by biochar-based management systems could be pivotal in capitalizing on the application potential of biochar as carbon sequestration to achieve carbon credit from a system (Jones et al., 2012). Quantifying the carbon sequestration potential of different biochar and its interaction with net greenhouse gas emissions could be crucial in amplifying the application potential of biochar as a soil amendment (Amonette et al., 2021; Joseph et al., 2021). Activation of biochar with fertilizers to increase the nutrient use efficiency and harnessing its climate change mitigation potential in a synergistic approach could be a suitable alternative in the future.
- Biochar is a highly calcitrant carbonaceous material generated by pyrolysis at oxygen-limited conditions through almost any carbon (cellulose-lignin) feedstock, including residues from agriculture, forestry, livestock farming, industrial wastes, etc., therefore a potentially cost-effective, carbon-negative resource with a multitude of agricultural and environmental applications.
- One of the most important applications of biochar remains as a soil amendment, which improves soil physical, and biochemical properties and increases soil fertility and productivity, particularly over the long-term, potentially increasing soil aggregation, water retention, pH, and microbial activities, thus, improving overall soil quality. However, biochar’s effect is more noticeable in coarse-textured soil, although large variability exists depending on biochar type and soil-crop-environment factors. Biochar can complement chemical fertilizer and organic sources such as compost and manure; however, the optimum application method to achieve maximum synergistic effect remains poorly known.
- Long-term biochar application can promote nutrient retention and soil productivity, helping reduce chemical fertilizer needs over time. Through increased aeration, soil moisture, and soil productivity, biochar also helps to promote soil biological quality. The extent of biochar impact on soil physiochemical properties varies depending on biochar source, type, size, inherent soil characteristics, and cropping system, among others.
- Biochar has significant potential in soil and water remediation, especially through its unique adsorption and chemical properties that can help to capture and immobilize pollutants such as metal(loid)s, organic pollutants, and hazardous emerging contaminants such as microplastics, therefore significantly improving soil and water quality. More recently, optimizing biochar through an activation with nutrients, metals, etc., has enabled the creation of a quality of biochar suitable for specific purposes, even allowing treatment of pollution in landfills and hazardous sites. Its environmental applications need to be finetuned for wider and target specific applications to tackle pressing environmental issues such as soil and water pollution.
- Biochar has also emerged as a key strategic, cost-effective material to tackle global issues such as climate change mitigation, reducing the net greenhouse gas emission to minimize global warming potential, which can be achieved while potentially improving soil quality and productivity, enabling the promotion of food security.
- As with other amendments, one of the critical challenges with biochar is that each biochar is different, hence attaining different results. Because of the diversity of the biochar, it is difficult to pinpoint the key advantage of biochar blanketly, and each biochar must be treated as a unique material, and its merit must be tested for desired results.
- A knowledge gap remains as to understanding the long-term persistence of biochar on agroecosystem, the optimal biochar application rate for the diversity of biochar-soil-crop-environmental conditions, the interaction of biochar with inherent soil carbon stock, specific mechanisms of biochar’s effect on soil biotic properties, quantification of carbon sequestration, greenhouse gas emissions, synergy or potential antagonistic effects with other carbon sources such as compost, manure, residues, etc., its modification for environmental applications and associated environmental and human risks over long-term.
- Future research should prioritize long-term studies, synthesizing cross-site mechanistic experiments across different systems and biochar types, life cycle assessment of biochar in soil-plant-environment, quantification of biochar persistence and soil carbon sequestration potential, validation of net greenhouse gas emission across different biochar-management systems, economic analysis of biochar amendment and application, with potential for carbon market integration.
JN: Conceptualization, First draft of manuscript, review and editing WA: Writing, Review and editing FM: Writing, review and validation AK: Writing, review and editing ZZ: Supervision, Fund acquisition.
The authors declare that the research was conducted in the absence of any commercial or financial relationships that could be construed as a potential conflict of interest.
All claims expressed in this article are solely those of the authors and do not necessarily represent those of their affiliated organizations, or those of the publisher, the editors and the reviewers. Any product that may be evaluated in this article, or claim that may be made by its manufacturer, is not guaranteed or endorsed by the publisher.
Abdoul Magid, A. S. I., Islam, Md. S., Chen, Y., Weng, L., Li, J., Ma, J., et al. (2021). Enhanced adsorption of polystyrene nanoplastics (PSNPs) onto oxidized corncob biochar with high pyrolysis temperature. Sci. Total Environ. 784, 147115. doi:10.1016/j.scitotenv.2021.147115
Agrafioti, E., Kalderis, D., and Diamadopoulos, E. (2014). Arsenic and chromium removal from water using biochars derived from rice husk, organic solid wastes and sewage sludge. J. Environ. Manag. 133, 309–314. doi:10.1016/j.jenvman.2013.12.007
Ahmad Bhat, S., Kuriqi, A., Dar, M. U. D., Bhat, O., Sammen, S. Sh., Towfiqul Islam, A. R. Md., et al. (2022). Application of biochar for improving physical, chemical, and hydrological soil properties: A systematic review. Sustainability 14, 11104. doi:10.3390/su141711104
Ahmed, M. B., Zhou, J. L., Ngo, H. H., and Guo, W. (2016a). Insight into biochar properties and its cost analysis. Biomass Bioenergy 84, 76–86. doi:10.1016/j.biombioe.2015.11.002
Ahmed, M. B., Zhou, J. L., Ngo, H. H., Guo, W., and Chen, M. (2016b). Progress in the preparation and application of modified biochar for improved contaminant removal from water and wastewater. Bioresour. Technol. 214, 836–851. doi:10.1016/j.biortech.2016.05.057
Ali, A., Ahmad, W., Munsif, F., Khan, A., Nepal, J., Wójcik-Gront, E., et al. (2022a). Residual effect of finely-ground biochar inoculated with bio-fertilization impact on productivity in a lentil–maize cropping system. Agronomy 12, 2036. doi:10.3390/agronomy12092036
Ali, I., Yuan, P., Ullah, S., Iqbal, A., Zhao, Q., Liang, H., et al. (2022b). Biochar amendment and nitrogen fertilizer contribute to the changes in soil properties and microbial communities in a paddy field. Front. Microbiol. 13
Amoakwah, E., Frimpong, K. A., Okae-Anti, D., and Arthur, E. (2017). Soil water retention, air flow and pore structure characteristics after corn cob biochar application to a tropical sandy loam. Geoderma 307, 189–197. doi:10.1016/j.geoderma.2017.08.025
Amonette, J. E., Blanco-Canqui, H., Hassebrook, C., Laird, D. A., Lal, R., Lehmann, J., et al. (2021). Integrated biochar research: A roadmap. J. Soil Water Conservation 76, 24A–29A. doi:10.2489/jswc.2021.1115A
Arnell, N. W., Lowe, J. A., Challinor, A. J., and Osborn, T. J. (2019). Global and regional impacts of climate change at different levels of global temperature increase. Clim. Change 155, 377–391. doi:10.1007/s10584-019-02464-z
Bai, N., Zhang, H., Li, S., Zheng, X., Zhang, J., Zhang, H., et al. (2019). Long-term effects of straw and straw-derived biochar on soil aggregation and fungal community in a rice–wheat rotation system. PeerJ 6, e6171. doi:10.7717/peerj.6171
Barry, D., and Hoyne, S. (2021). Sustainable measurement indicators to assess impacts of climate change: Implications for the New Green Deal Era. Curr. Opin. Environ. Sci. Health 22, 100259. doi:10.1016/j.coesh.2021.100259
Beheshti, M., Etesami, H., and Alikhani, H. A. (2018). Effect of different biochars amendment on soil biological indicators in a calcareous soil. Environ. Sci. Pollut. Res. 25, 14752–14761. doi:10.1007/s11356-018-1682-2
Ben Hassen, T., and El Bilali, H. (2022). Impacts of the Russia-Ukraine war on global food security: Towards more sustainable and resilient food systems? Foods 11, 2301. doi:10.3390/foods11152301
Bhattarai, B., Neupane, J., Dhakal, S. P., Nepal, J., Gnyawali, B., Timalsina, R., et al. (2015). Effect of biochar from different origin on physio-chemical properties of soil and yield of garden pea (pisum sativum L.) at paklihawa, rupandehi, Nepal. World J. Agric. Res. 3, 10. doi:10.12691/wjar-3-4-3
Biederman, L. A., and Harpole, W. S. (2013). Biochar and its effects on plant productivity and nutrient cycling: a meta-analysis. GCB Bioenergy, 5 (2), 202–214.
Biesbroek, R., Wright, S. J., Eguren, S. K., Bonotto, A., and Athanasiadis, I. N. (2022). Policy attention to climate change impacts, adaptation and vulnerability: A global assessment of national communications (1994–2019). Clim. Policy 22, 97–111. doi:10.1080/14693062.2021.2018986
Blanco-Canqui, H. (2017). Biochar and soil physical properties. Soil Sci. Soc. Am. J. 81, 687–711. doi:10.2136/sssaj2017.01.0017
Blanco-Canqui, H. (2021). Does biochar application alleviate soil compaction? Review and data synthesis. Geoderma 404, 115317. doi:10.1016/j.geoderma.2021.115317
Brown, R. A., Kercher, A. K., Nguyen, T. H., Nagle, D. C., and Ball, W. P. (2006). Production and characterization of synthetic wood chars for use as surrogates for natural sorbents. Org. Geochem. 37, 321–333. doi:10.1016/j.orggeochem.2005.10.008
Burrell, L. D., Zehetner, F., Rampazzo, N., Wimmer, B., and Soja, G. (2016). Long-term effects of biochar on soil physical properties. Geoderma 282, 96–102. doi:10.1016/j.geoderma.2016.07.019
Butnan, S., Deenik, J. L., Toomsan, B., and Vityakon, P. (2017). Biochar properties affecting carbon stability in soils contrasting in texture and mineralogy. Agric. Nat. Resour. 51, 492–498. doi:10.1016/j.anres.2018.03.002
Cao, X., Ma, L., Gao, B., and Harris, W. (2009). Dairy-manure derived biochar effectively sorbs lead and atrazine. Environ. Sci. Technol. 43, 3285–3291. doi:10.1021/es803092k
Cardoso, E. J. B. N., Vasconcellos, R. L. F., Bini, D., Miyauchi, M. Y. H., Santosdos, C. A., Alves, P. R. L., et al. (2013). Soil health: Looking for suitable indicators. What should be considered to assess the effects of use and management on soil health? Sci. Agric. (Piracicaba, Braz.) 70, 274–289. doi:10.1590/S0103-90162013000400009
Carey, D. E., McNamara, P. J., and Zitomer, D. H. (2015). Biochar from pyrolysis of biosolids for nutrient adsorption and turfgrass cultivation. Water Environ. Res. 87, 2098–2106. doi:10.2175/106143015X14362865227391
Cheng, C.-H., Lehmann, J., Thies, J. E., Burton, S. D., and Engelhard, M. H. (2006). Oxidation of black carbon by biotic and abiotic processes. Org. Geochem. 37, 1477–1488. doi:10.1016/j.orggeochem.2006.06.022
Chintala, R., Mollinedo, J., Schumacher, T. E., Malo, D. D., and Julson, J. L. (2014). Effect of biochar on chemical properties of acidic soil. Arch. Agron. Soil Sci. 60 (3), 393–404.
Clough, T., Condron, L., Kammann, C., and Müller, C. (2013). A review of biochar and soil nitrogen dynamics. Agronomy 3, 275–293. doi:10.3390/agronomy3020275
Cole, A. J., Paul, N. A., de Nys, R., and Roberts, D. A. (2017). Good for sewage treatment and good for agriculture: Algal based compost and biochar. J. Environ. Manag. 200, 105–113. doi:10.1016/j.jenvman.2017.05.082
Cook, B. I., Mankin, J. S., and Anchukaitis, K. J. (2018). Climate change and drought: From past to future. Curr. Clim. Change Rep. 4, 164–179. doi:10.1007/s40641-018-0093-2
Das, S., Mohanty, S., Sahu, G., Rana, M., and Pilli, K. (2021). “Biochar: A sustainable approach for improving soil health and environment,” in Soil erosion - current challenges and future perspectives in a changing world Editors A. Vieira, and S. Carlos Rodrigues (London, UK: IntechOpen). doi:10.5772/intechopen.97136
Diatta, A. A., Fike, J. H., Battaglia, M. L., Galbraith, J. M., and Baig, M. B. (2020). Effects of biochar on soil fertility and crop productivity in arid regions: A review. Arab. J. Geosci. 13, 595. doi:10.1007/s12517-020-05586-2
Downie, A., Crosky, A., and Munroe, P. (2012). “Physical properties of biochar,” in Biochar for environmental management. Abingdon, Oxfordshire, United Kingdom: Routledge, 45–64.
Doran, J. W., and Zeiss, M. R. (2000). Soil health and sustainability: Managing the biotic component of soil quality. Appl. Soil Ecol. 15, 3–11. doi:10.1016/S0929-1393(00)00067-6
Du, Z.-L., Zhao, J.-K., Wang, Y.-D., and Zhang, Q.-Z. (2017). Biochar addition drives soil aggregation and carbon sequestration in aggregate fractions from an intensive agricultural system. J. Soils Sediments 17, 581–589. doi:10.1007/s11368-015-1349-2
Enders, A., and Lehmann, J. (2012). Comparison of wet-digestion and dry-ashing methods for total elemental analysis of biochar. Commun. Soil Sci. Plant Analysis 43, 1042–1052. doi:10.1080/00103624.2012.656167
Ge, S., Foong, S. Y., Ma, N. L., Liew, R. K., Wan Mahari, W. A., Xia, C., et al. (2020). Vacuum pyrolysis incorporating microwave heating and base mixture modification: An integrated approach to transform biowaste into eco-friendly bioenergy products. Renew. Sustain. Energy Rev. 127, 109871. doi:10.1016/j.rser.2020.109871
Glaser, B., and Lehr, V.-I. (2019). Biochar effects on phosphorus availability in agricultural soils: A meta-analysis. Sci. Rep. 9, 9338. doi:10.1038/s41598-019-45693-z
Glaser, B., Lehmann, J., and Zech, W. (2002). Ameliorating physical and chemical properties of highly weathered soils in the tropics with charcoal - a review. Biol. Fertil. Soils 35, 219–230. doi:10.1007/s00374-002-0466-4
Gong, H., Tan, Z., Zhang, L., and Huang, Q. (2019). Preparation of biochar with high absorbability and its nutrient adsorption–desorption behaviour. Sci. Total Environ. 694, 133728. doi:10.1016/j.scitotenv.2019.133728
Gorovtsov, A. V., Minkina, T. M., Mandzhieva, S. S., Perelomov, L. V., Soja, G., Zamulina, I. V., et al. (2020). The mechanisms of biochar interactions with microorganisms in soil. Environ. Geochem Health 42, 2495–2518. doi:10.1007/s10653-019-00412-5
Gross, A., Bromm, T., and Glaser, B. (2021). Soil organic carbon sequestration after biochar application: A global meta-analysis. Agronomy 11, 2474. doi:10.3390/agronomy11122474
Hagemann, N., Joseph, S., Schmidt, H.-P., Kammann, C. I., Harter, J., Borch, T., et al. (2017). Organic coating on biochar explains its nutrient retention and stimulation of soil fertility. Nat. Commun. 8, 1089. doi:10.1038/s41467-017-01123-0
Hale, S. E., Nurida, N. L., Jubaedah, , Mulder, J., Sørmo, E., Silvani, L., et al. (2020). The effect of biochar, lime and ash on maize yield in a long-term field trial in a Ultisol in the humid tropics. Sci. Total Environ. 719, 137455. doi:10.1016/j.scitotenv.2020.137455
Han, Z., Sani, B., Mrozik, W., Obst, M., Beckingham, B., Karapanagioti, H. K., et al. (2015). Magnetite impregnation effects on the sorbent properties of activated carbons and biochars. Water Res. 70, 394–403. doi:10.1016/j.watres.2014.12.016
Han, J., Zhang, A., Kang, Y., Han, J., Yang, B., Hussain, Q., et al. (2022). Biochar promotes soil organic carbon sequestration and reduces net global warming potential in apple orchard: A two-year study in the Loess Plateau of China. Sci. Total Environ. 803, 150035. doi:10.1016/j.scitotenv.2021.150035
Hao, N., Cao, J., Ye, J., Zhang, C., Li, C., and Bate, B. (2021). Content and morphology of lead remediated by activated carbon and biochar: A spectral induced polarization study. J. Hazard. Mater. 411, 124605. doi:10.1016/j.jhazmat.2020.124605
Hardie, M., Clothier, B., Bound, S., Oliver, G., and Close, D. (2014). Does biochar influence soil physical properties and soil water availability? Plant Soil 376, 347–361. doi:10.1007/s11104-013-1980-x
Herath, H. M. S. K., Camps-Arbestain, M., and Hedley, M. (2013). Effect of biochar on soil physical properties in two contrasting soils: An Alfisol and an Andisol. Geoderma 210, 188–197. doi:10.1016/j.geoderma.2013.06.016
Huang, H., Yao, W., Li, R., Ali, A., Du, J., Guo, D., et al. (2018). Effect of pyrolysis temperature on chemical form, behavior and environmental risk of Zn, Pb and Cd in biochar produced from phytoremediation residue. Bioresour. Technol. 249, 487–493. doi:10.1016/j.biortech.2017.10.020
Islam, M. U., Jiang, F., Guo, Z., and Peng, X. (2021). Does biochar application improve soil aggregation? A meta-analysis. Soil Tillage Res. 209, 104926. doi:10.1016/j.still.2020.104926
Jones, D. L., Murphy, D. V., Khalid, M., Ahmad, W., Edwards-Jones, G., and DeLuca, T. H. (2011). Short-term biochar-induced increase in soil CO2 release is both biotically and abiotically mediated. Soil Biol. Biochem. 43, 1723–1731. doi:10.1016/j.soilbio.2011.04.018
Jones, D. L., Rousk, J., Edwards-Jones, G., DeLuca, T. H., and Murphy, D. V. (2012). Biochar-mediated changes in soil quality and plant growth in a three year field trial. Soil Biol. Biochem. 45, 113–124. doi:10.1016/j.soilbio.2011.10.012
Joseph, S. D., Camps-Arbestain, M., Lin, Y., Munroe, P., Chia, C. H., Hook, J., et al. (2010). An investigation into the reactions of biochar in soil. Soil Res. 48, 501. doi:10.1071/SR10009
Joseph, S., Cowie, A. L., Van Zwieten, L., Bolan, N., Budai, A., Buss, W., et al. (2021). How biochar works, and when it doesn’t: A review of mechanisms controlling soil and plant responses to biochar. GCB Bioenergy 13, 1731–1764. doi:10.1111/gcbb.12885
Kang, M. W., Yibeltal, M., Kim, Y. H., Oh, S. J., Lee, J. C., Kwon, E. E., et al. (2022). Enhancement of soil physical properties and soil water retention with biochar-based soil amendments. Sci. Total Environ. 836, 155746. doi:10.1016/j.scitotenv.2022.155746
Karhu, K., Mattila, T., Bergström, I., and Regina, K. (2011). Biochar addition to agricultural soil increased CH4 uptake and water holding capacity – results from a short-term pilot field study. Agric. Ecosyst. Environ. 140, 309–313. doi:10.1016/j.agee.2010.12.005
Kilian, B., Dempewolf, H., Guarino, L., Werner, P., Coyne, C., and Warburton, M. L. (2021). Crop science special issue: Adapting agriculture to climate change: A walk on the wild side. Crop Sci. 61, 32–36. doi:10.1002/csc2.20418
Kołodyńska, D., Wnętrzak, R., Leahy, J. J., Hayes, M. H. B., Kwapiński, W., and Hubicki, Z. (2012). Kinetic and adsorptive characterization of biochar in metal ions removal. Chem. Eng. J. 197, 295–305. doi:10.1016/j.cej.2012.05.025
Kopittke, P. M., Menzies, N. W., Wang, P., McKenna, B. A., and Lombi, E. (2019). Soil and the intensification of agriculture for global food security. Environ. Int. 132, 105078. doi:10.1016/j.envint.2019.105078
Kumar, R., Verma, A., Rakib, Md. R. J., Gupta, P. K., Sharma, P., Garg, A., et al. (2023). Adsorptive behavior of micro(nano)plastics through biochar: Co-Existence, consequences, and challenges in contaminated ecosystems. Sci. Total Environ. 856, 159097. doi:10.1016/j.scitotenv.2022.159097
Laborde, D., Martin, W., Swinnen, J., and Vos, R. (2020). COVID-19 risks to global food security. Science 369, 500–502. doi:10.1126/science.abc4765
Lai, W.-Y., Lai, C.-M., Ke, G.-R., Chung, R.-S., Chen, C.-T., Cheng, C.-H., et al. (2013). The effects of woodchip biochar application on crop yield, carbon sequestration and greenhouse gas emissions from soils planted with rice or leaf beet. J. Taiwan Inst. Chem. Eng. 44, 1039–1044. doi:10.1016/j.jtice.2013.06.028
Laird, D., Fleming, P., Wang, B., Horton, R., and Karlen, D. (2010). Biochar impact on nutrient leaching from a Midwestern agricultural soil. Geoderma 158, 436–442. doi:10.1016/j.geoderma.2010.05.012
Lam, S. S., Su, M. H., Nam, W. L., Thoo, D. S., Ng, C. M., Liew, R. K., et al. (2019). Microwave pyrolysis with steam activation in producing activated carbon for removal of herbicides in agricultural surface water. Ind. Eng. Chem. Res. 58, 695–703. doi:10.1021/acs.iecr.8b03319
Lehmann, J., Zech, W., Glaser, B., Nehls, T., and Glaser, B. (2003). Nutrient availability and leaching in an archaeological anthrosol and a ferralsol of the central amazon basin: Fertilizer, manure and charcoal amendments. Plant Soil 249, 343–357. doi:10.1023/A:1022833116184
Lehmann, J., and Joseph, S. (2015). Biochar for environmental management: science, technology and implementation. Abingdon, Oxfordshire, United Kingdom: Routledge.
Lehmann, J., Rillig, M. C., Thies, J., Masiello, C. A., Hockaday, W. C., and Crowley, D. (2011). Biochar effects on soil biota – a review. Soil Biol. Biochem. 43, 1812–1836. doi:10.1016/j.soilbio.2011.04.022
Lehmann, J., Bossio, D. A., Kögel-Knabner, I., and Rillig, M. C. (2020). The concept and future prospects of soil health. Nat. Rev. Earth Environ. 1, 544–553. doi:10.1038/s43017-020-0080-8
Lehmann, J. (2007). Bio-energy in the black. Front. Ecol. Environ. 5, 381–387. doi:10.1890/1540-9295(2007)5[381:BITB]2.0.CO;2
Li, Y., Shao, J., Wang, X., Deng, Y., Yang, H., and Chen, H. (2014). Characterization of modified biochars derived from bamboo pyrolysis and their utilization for target component (furfural) adsorption. Energy 28, 5119–5127. doi:10.1021/ef500725c
Lin, X. W., Xie, Z. B., Zheng, J. Y., Liu, Q., Bei, Q. C., and Zhu, J. G. (2015). Effects of biochar application on greenhouse gas emissions, carbon sequestration and crop growth in coastal saline soil: Biochar’s effects on GHGs, soil C cycle and crop growth. Eur. J. Soil Sci. 66, 329–338. doi:10.1111/ejss.12225
Lin, H., Sun, W., Yu, Y., Ding, Y., Yang, Y., Zhang, Z., et al. (2021). Simultaneous reductions in antibiotics and heavy metal pollution during manure composting. Sci. Total Environ. 788, 147830. doi:10.1016/j.scitotenv.2021.147830
Liu, Z., Chen, X., Jing, Y., Li, Q., Zhang, J., and Huang, Q. (2014). Effects of biochar amendment on rapeseed and sweet potato yields and water stable aggregate in upland red soil. Catena 123, 45–51. doi:10.1016/j.catena.2014.07.005
Liu, S., Zhang, Y., Zong, Y., Hu, Z., Wu, S., Zhou, J., et al. (2016). Response of soil carbon dioxide fluxes, soil organic carbon and microbial biomass carbon to biochar amendment: A meta-analysis. GCB Bioenergy 8, 392–406. doi:10.1111/gcbb.12265
Liu, G., Zheng, H., Jiang, Z., Zhao, J., Wang, Z., Pan, B., et al. (2018). Formation and physicochemical characteristics of nano biochar: Insight into chemical and colloidal stability. Environ. Sci. Technol. 52, 10369–10379. doi:10.1021/acs.est.8b01481
Lorenz, K., and Lal, R. (2014). Biochar application to soil for climate change mitigation by soil organic carbon sequestration. J. Plant Nutr. Soil Sci. 177, 651–670. doi:10.1002/jpln.201400058
Ma, Y., Liu, W.-J., Zhang, N., Li, Y.-S., Jiang, H., and Sheng, G.-P. (2014). Polyethylenimine modified biochar adsorbent for hexavalent chromium removal from the aqueous solution. Bioresour. Technol. 169, 403–408. doi:10.1016/j.biortech.2014.07.014
Major, J., Rondon, M., Molina, D., Riha, S. J., and Lehmann, J. (2010). Maize yield and nutrition during 4 years after biochar application to a Colombian savanna oxisol. Plant Soil 333, 117–128. doi:10.1007/s11104-010-0327-0
McBratney, A. B., Morgan, C. L., and Jarrett, L. E. (2017). “The value of soil’s contributions to ecosystem services,” in Global soil security (Cham: Springer), 227–235. doi:10.1007/978-3-319-43394-3_20
Mona, S., Malyan, S. K., Saini, N., Deepak, B., Pugazhendhi, A., and Kumar, S. S. (2021). Towards sustainable agriculture with carbon sequestration, and greenhouse gas mitigation using algal biochar. Chemosphere 275, 129856. doi:10.1016/j.chemosphere.2021.129856
Mukherjee, S., Mishra, A., and Trenberth, K. E. (2018). Climate change and drought: A perspective on drought indices. Curr. Clim. Change Rep. 4, 145–163. doi:10.1007/s40641-018-0098-x
O’Connor, D., Peng, T., Zhang, J., Tsang, D. C. W., Alessi, D. S., Shen, Z., et al. (2018). Biochar application for the remediation of heavy metal polluted land: A review of in situ field trials. Sci. Total Environ. 619–620, 815–826. doi:10.1016/j.scitotenv.2017.11.132
Oliveira, F. R., Patel, A. K., Jaisi, D. P., Adhikari, S., Lu, H., and Khanal, S. K. (2017). Environmental application of biochar: Current status and perspectives. Bioresour. Technol. 246, 110–122. doi:10.1016/j.biortech.2017.08.122
Oni, B. A., Oziegbe, O., and Olawole, O. O. (2019). Significance of biochar application to the environment and economy. Ann. Agric. Sci. 64, 222–236. doi:10.1016/j.aoas.2019.12.006
Ortiz-Bobea, A., Ault, T. R., Carrillo, C. M., Chambers, R. G., and Lobell, D. B. (2021). Anthropogenic climate change has slowed global agricultural productivity growth. Nat. Clim. Chang. 11, 306–312. doi:10.1038/s41558-021-01000-1
Palansooriya, K. N., Sang, M. K., Igalavithana, A. D., Zhang, M., Hou, D., Oleszczuk, P., et al. (2022). Biochar alters chemical and microbial properties of microplastic-contaminated soil. Environ. Res. 209, 112807. doi:10.1016/j.envres.2022.112807
Pandit, N. R., Mulder, J., Hale, S. E., Schmidt, H. P., and Cornelissen, G. (2017). Biochar from “Kon Tiki” flame curtain and other kilns: Effects of nutrient enrichment and kiln type on crop yield and soil chemistry. PLoS ONE 12, e0176378. doi:10.1371/journal.pone.0176378
Pandit, N. R., Mulder, J., Hale, S. E., Zimmerman, A. R., Pandit, B. H., and Cornelissen, G. (2018). Multi-year double cropping biochar field trials in Nepal: Finding the optimal biochar dose through agronomic trials and cost-benefit analysis. Sci. Total Environ. 637, 1333–1341. doi:10.1016/j.scitotenv.2018.05.107
Pei, J., Li, J., Fang, C., Zhao, J., Nie, M., and Wu, J. (2020). Different responses of root exudates to biochar application under elevated CO2. Agric. Ecosyst. Environ. 301, 107061. doi:10.1016/j.agee.2020.107061
Pörtner, H.-O., and Roberts, D. C. (2022). Climate change 2022: Impacts, adaptation and vulnerability. Geneva, Switzerland: IPCC. IPCC Sixth Assessment Report, 168.
Prasannamedha, G., Kumar, P. S., Mehala, R., Sharumitha, T. J., and Surendhar, D. (2021). Enhanced adsorptive removal of sulfamethoxazole from water using biochar derived from hydrothermal carbonization of sugarcane bagasse. J. Hazard. Mater. 407, 124825. doi:10.1016/j.jhazmat.2020.124825
Priestley, R. K., Heine, Z., and Milfont, T. L. (2021). Public understanding of climate change-related sea-level rise. PLoS ONE 16, e0254348. doi:10.1371/journal.pone.0254348
Qiu, M., Liu, L., Ling, Q., Cai, Y., Yu, S., Wang, S., et al. (2022). Biochar for the removal of contaminants from soil and water: A review. Biochar 4, 19. doi:10.1007/s42773-022-00146-1
Qu, J., Wang, Y., Tian, X., Jiang, Z., Deng, F., Tao, Y., et al. (2021). KOH-activated porous biochar with high specific surface area for adsorptive removal of chromium (VI) and naphthalene from water: Affecting factors, mechanisms and reusability exploration. J. Hazard. Mater. 401, 123292. doi:10.1016/j.jhazmat.2020.123292
Rajapaksha, A. U., Vithanage, M., Ahmad, M., Seo, D.-C., Cho, J.-S., Lee, S.-E., et al. (2015). Enhanced sulfamethazine removal by steam-activated invasive plant-derived biochar. J. Hazard. Mater. 290, 43–50. doi:10.1016/j.jhazmat.2015.02.046
Rasa, K., Heikkinen, J., Hannula, M., Arstila, K., Kulju, S., and Hyväluoma, J. (2018). How and why does willow biochar increase a clay soil water retention capacity? Biomass Bioenergy 119, 346–353. doi:10.1016/j.biombioe.2018.10.004
Razzaghi, F., Obour, P. B., and Arthur, E. (2020). Does biochar improve soil water retention? A systematic review and meta-analysis. Geoderma 361, 114055. doi:10.1016/j.geoderma.2019.114055
Ren, X., Wang, F., Cao, F., Guo, J., and Sun, H. (2018). Desorption of atrazine in biochar-amended soils: Effects of root exudates and the aging interactions between biochar and soil. Chemosphere 212, 687–693. doi:10.1016/j.chemosphere.2018.08.124
Roberts, D. A., Paul, N. A., Cole, A. J., and de Nys, R. (2015). From waste water treatment to land management: Conversion of aquatic biomass to biochar for soil amelioration and the fortification of crops with essential trace elements. J. Environ. Manag. 157, 60–68. doi:10.1016/j.jenvman.2015.04.016
Saarnio, S., Heimonen, K., and Kettunen, R. (2013). Biochar addition indirectly affects N2O emissions via soil moisture and plant N uptake. Soil Biol. Biochem. 58, 99–106. doi:10.1016/j.soilbio.2012.10.035
Saffari, N., Hajabbasi, M. A., Shirani, H., Mosaddeghi, M. R., and Owens, G. (2021). Influence of corn residue biochar on water retention and penetration resistance in a calcareous sandy loam soil. Geoderma 383, 114734. doi:10.1016/j.geoderma.2020.114734
Shen, B., Chen, J., Yue, S., and Li, G. (2015). A comparative study of modified cotton biochar and activated carbon based catalysts in low temperature SCR. Fuel 156, 47–53. doi:10.1016/j.fuel.2015.04.027
Shin, J., Park, D., Hong, S., Jeong, C., Kim, H., and Chung, W. (2021). Influence of activated biochar pellet fertilizer application on greenhouse gas emissions and carbon sequestration in rice (Oryza sativa L.) production. Environ. Pollut. 285, 117457. doi:10.1016/j.envpol.2021.117457
Singh, N., Khandelwal, N., Ganie, Z. A., Tiwari, E., and Darbha, G. K. (2021). Eco-friendly magnetic biochar: An effective trap for nanoplastics of varying surface functionality and size in the aqueous environment. Chem. Eng. J. 418, 129405. doi:10.1016/j.cej.2021.129405
Steiner, C., Teixeira, W. G., Lehmann, J., Nehls, T., de Macêdo, J. L. V., Blum, W. E. H., et al. 2007). Long term effects of manure, charcoal and mineral fertilization on crop production and fertility on a highly weathered Central Amazonian upland soil. Plant Soil 291, 275–290. doi:10.1007/s11104-007-9193-9
Stott, D. (2019). Recommended soil health indicators and associated laboratory procedures. United States: USDA. Soil Health Technical note, 76.
Sun, J., He, F., Pan, Y., and Zhang, Z. (2017). Effects of pyrolysis temperature and residence time on physicochemical properties of different biochar types. Acta Agric. Scand. Sect. B — Soil & Plant Sci. 67, 12–22. doi:10.1080/09064710.2016.1214745
Tan, S., Narayanan, M., Thu Huong, D. T., Ito, N., Unpaprom, Y., Pugazhendhi, A., et al. (2022). A perspective on the interaction between biochar and soil microbes: A way to regain soil eminence. Environ. Res. 214, 113832. doi:10.1016/j.envres.2022.113832
Thammasom, N., Vityakon, P., Lawongsa, P., and Saenjan, P. (2016). Biochar and rice straw have different effects on soil productivity, greenhouse gas emission and carbon sequestration in Northeast Thailand paddy soil. Agric. Nat. Resour. 50, 192–198. doi:10.1016/j.anres.2016.01.003
Vaccari, F. P., Baronti, S., Lugato, E., Genesio, L., Castaldi, S., Fornasier, F., et al. (2011). Biochar as a strategy to sequester carbon and increase yield in durum wheat. Eur. J. Agron. 34, 231–238. doi:10.1016/j.eja.2011.01.006
Verheijen, F. G. A., Zhuravel, A., Silva, F. C., Amaro, A., Ben-Hur, M., and Keizer, J. J. (2019). The influence of biochar particle size and concentration on bulk density and maximum water holding capacity of sandy vs sandy loam soil in a column experiment. Geoderma 347, 194–202. doi:10.1016/j.geoderma.2019.03.044
Walker, A. P., De Kauwe, M. G., Bastos, A., Belmecheri, S., Georgiou, K., Keeling, R. F., et al. (2021). Integrating the evidence for a terrestrial carbon sink caused by increasing atmospheric CO 2. New Phytol. 229, 2413–2445. doi:10.1111/nph.16866
Wan, S., Wu, J., Zhou, S., Wang, R., Gao, B., and He, F. (2018). Enhanced lead and cadmium removal using biochar-supported hydrated manganese oxide (HMO) nanoparticles: Behavior and mechanism. Sci. Total Environ. 616, 1298–1306. doi:10.1016/j.scitotenv.2017.10.188
Wang, S., Gao, B., Li, Y., Zimmerman, A. R., and Cao, X. (2016). Sorption of arsenic onto Ni/Fe layered double hydroxide (LDH)-biochar composites. RSC Adv. 6, 17792–17799. doi:10.1039/C5RA17490B
Wang, D., Li, C., Parikh, S. J., and Scow, K. M. (2019). Impact of biochar on water retention of two agricultural soils – a multi-scale analysis. Geoderma 340, 185–191. doi:10.1016/j.geoderma.2019.01.012
Wang, Z., Sedighi, M., and Lea-Langton, A. (2020). Filtration of microplastic spheres by biochar: Removal efficiency and immobilisation mechanisms. Water Res. 184, 116165. doi:10.1016/j.watres.2020.116165
Wang, J., Sun, C., Huang, Q.-X., Chi, Y., and Yan, J.-H. (2021). Adsorption and thermal degradation of microplastics from aqueous solutions by Mg/Zn modified magnetic biochars. J. Hazard. Mater. 419, 126486. doi:10.1016/j.jhazmat.2021.126486
Wang, X., Dan, Y., Diao, Y., Liu, F., Wang, H., and Sang, W. (2022). Transport and retention of microplastics in saturated porous media with peanut shell biochar (PSB) and MgO-PSB amendment: Co-Effects of cations and humic acid. Environ. Pollut. 305, 119307. doi:10.1016/j.envpol.2022.119307
Warnock, D. D., Lehmann, J., Kuyper, T. W., and Rillig, M. C. (2007). Mycorrhizal responses to biochar in soil – concepts and mechanisms. Plant Soil 300, 9–20. doi:10.1007/s11104-007-9391-5
Weber, K., and Quicker, P. (2018). Properties of biochar. Fuel 217, 240–261. doi:10.1016/j.fuel.2017.12.054
Widowati, W., Asnah, A., and Utomo, W. H. (2014). The use of biochar to reduce nitrogen and potassium leaching from soil cultivated with maize. J. Degrade. Min. Land Manage. 2 (1), 211.
Woolf, D., Amonette, J. E., Street-Perrott, F. A., Lehmann, J., and Joseph, S. (2010). Sustainable biochar to mitigate global climate change. Nat. Commun. 1, 56. doi:10.1038/ncomms1053
Xiang, W., Zhang, X., Chen, J., Zou, W., He, F., Hu, X., et al. (2020). Biochar technology in wastewater treatment: A critical review. Chemosphere 252, 126539. doi:10.1016/j.chemosphere.2020.126539
Xiao, J., Hu, R., and Chen, G. (2020). Micro-nano-engineered nitrogenous bone biochar developed with a ball-milling technique for high-efficiency removal of aquatic Cd(II), Cu(II) and Pb(II). J. Hazard. Mater. 387, 121980. doi:10.1016/j.jhazmat.2019.121980
Xu, H., Cai, A., Wu, D., Liang, G., Xiao, J., Xu, M., et al. (2021). Effects of biochar application on crop productivity, soil carbon sequestration, and global warming potential controlled by biochar C:N ratio and soil pH: A global meta-analysis. Soil Tillage Res. 213, 105125. doi:10.1016/j.still.2021.105125
Yan, M., Li, X., Liu, Y., Li, Y., He, L., and Zhang, J. (2022). Biochar enhanced soil aggregation and C-related enzyme activity in post-mining land on the Loess Plateau, China. Land Degrad. Dev. 33, 1054–1061. doi:10.1002/ldr.4230
Yang, X., Igalavithana, A. D., Oh, S.-E., Nam, H., Zhang, M., Wang, C.-H., et al. (2018). Characterization of bioenergy biochar and its utilization for metal/metalloid immobilization in contaminated soil. Sci. Total Environ. 640, 704–713. doi:10.1016/j.scitotenv.2018.05.298
Yang, W., Feng, G., Miles, D., Gao, L., Jia, Y., Li, C., et al. (2020). Impact of biochar on greenhouse gas emissions and soil carbon sequestration in corn grown under drip irrigation with mulching. Sci. Total Environ. 729, 138752. doi:10.1016/j.scitotenv.2020.138752
Yang, Q., Mašek, O., Zhao, L., Nan, H., Yu, S., Yin, J., et al. (2021). Country-level potential of carbon sequestration and environmental benefits by utilizing crop residues for biochar implementation. Appl. Energy 282, 116275. doi:10.1016/j.apenergy.2020.116275
Ye, S., Cheng, M., Zeng, G., Tan, X., Wu, H., Liang, J., et al. (2020). Insights into catalytic removal and separation of attached metals from natural-aged microplastics by magnetic biochar activating oxidation process. Water Res. 179, 115876. doi:10.1016/j.watres.2020.115876
Yin, X., Peñuelas, J., Sardans, J., Xu, X., Chen, Y., Fang, Y., et al. (2021). Effects of nitrogen-enriched biochar on rice growth and yield, iron dynamics, and soil carbon storage and emissions: A tool to improve sustainable rice cultivation. Environ. Pollut. 287, 117565. doi:10.1016/j.envpol.2021.117565
Yuan, P., Wang, J., Pan, Y., Shen, B., and Wu, C. (2019). Review of biochar for the management of contaminated soil: Preparation, application and prospect. Sci. Total Environ. 659, 473–490. doi:10.1016/j.scitotenv.2018.12.400
Zeeshan, M., Ahmad, W., Hussain, F., Ahamd, W., Numan, M., Shah, M., et al. (2020). Phytostabalization of the heavy metals in the soil with biochar applications, the impact on chlorophyll, carotene, soil fertility and tomato crop yield. J. Clean. Prod. 255, 120318. doi:10.1016/j.jclepro.2020.120318
Zhang, X., Wang, H., He, L., Lu, K., Sarmah, A., Li, J., et al. (2013). Using biochar for remediation of soils contaminated with heavy metals and organic pollutants. Environ. Sci. Pollut. Res. 20, 8472–8483. doi:10.1007/s11356-013-1659-0
Zhang, M., Liu, Y., Li, T., Xu, W., Zheng, B., Tan, X., et al. (2015). Chitosan modification of magnetic biochar produced from Eichhornia crassipes for enhanced sorption of Cr(vi) from aqueous solution. RSC Adv. 5, 46955–46964. doi:10.1039/C5RA02388B
Zhang, A., Li, X., Xing, J., and Xu, G. (2020a). Adsorption of potentially toxic elements in water by modified biochar: A review. J. Environ. Chem. Eng. 8, 104196. doi:10.1016/j.jece.2020.104196
Zhang, Q., Song, Y., Wu, Z., Yan, X., Gunina, A., Kuzyakov, Y., et al. (2020b). Effects of six-year biochar amendment on soil aggregation, crop growth, and nitrogen and phosphorus use efficiencies in a rice-wheat rotation. J. Clean. Prod. 242, 118435. doi:10.1016/j.jclepro.2019.118435
Zhang, Y., Wang, J., and Feng, Y. (2021). The effects of biochar addition on soil physicochemical properties: A review. CATENA 202, 105284. doi:10.1016/j.catena.2021.105284
Zhao, T., Yao, Y., Li, D., Wu, F., Zhang, C., and Gao, B. (2018). Facile low-temperature one-step synthesis of pomelo peel biochar under air atmosphere and its adsorption behaviors for Ag(I) and Pb(II). Sci. Total Environ. 640, 73–79. doi:10.1016/j.scitotenv.2018.05.251
Zhou, Y., Qin, S., Verma, S., Sar, T., Sarsaiya, S., Ravindran, B., et al. (2021). Production and beneficial impact of biochar for environmental application: A comprehensive review. Bioresour. Technol. 337, 125451. doi:10.1016/j.biortech.2021.125451
Keywords: biochar, carbon sequestration, environmental quality, soil fertility, soil health, bioremediation, water quality, greenhouse gas emission
Citation: Nepal J, Ahmad W, Munsif F, Khan A and Zou Z (2023) Advances and prospects of biochar in improving soil fertility, biochemical quality, and environmental applications. Front. Environ. Sci. 11:1114752. doi: 10.3389/fenvs.2023.1114752
Received: 02 December 2022; Accepted: 30 January 2023;
Published: 16 February 2023.
Edited by:
Lanfang Han, Guangdong University of Technology, ChinaReviewed by:
Rubén Forján, Universidad de Oviedo Mieres, SpainCopyright © 2023 Nepal, Ahmad, Munsif, Khan and Zou. This is an open-access article distributed under the terms of the Creative Commons Attribution License (CC BY). The use, distribution or reproduction in other forums is permitted, provided the original author(s) and the copyright owner(s) are credited and that the original publication in this journal is cited, in accordance with accepted academic practice. No use, distribution or reproduction is permitted which does not comply with these terms.
*Correspondence: Zhiyou Zou, NDEwMDAzMzkxQHFxLmNvbQ==
Disclaimer: All claims expressed in this article are solely those of the authors and do not necessarily represent those of their affiliated organizations, or those of the publisher, the editors and the reviewers. Any product that may be evaluated in this article or claim that may be made by its manufacturer is not guaranteed or endorsed by the publisher.
Research integrity at Frontiers
Learn more about the work of our research integrity team to safeguard the quality of each article we publish.