- 1Centre for Functional Ecology—Science for People & the Planet | TERRA—Associate Laboratory for Sustainable Land Use and Ecosystem Services, Department of Life Sciences, University of Coimbra, Coimbra, Portugal
- 2Centre for Functional Ecology—Science for People & the Planet, Escola Superior Agrária de Coimbra, Instituto Politécnico de Coimbra, Coimbra, Portugal
Conservation of biodiversity in protected areas is often threatened by invasive alien plants (IAPs), and its successful management requires proper planning, resources, and monitoring to adjust future interventions. Although follow-up controls and evaluation of different management actions through monitoring programs are crucial in an adaptive management strategy, they are often neglected. Acacia longifolia is one of the most widespread IAPs in the Portuguese coastal areas, causing several negative impacts. This study reports the results of different control methods of A. longifolia in two forest plantations over coastal dunes in two protected sites in Portugal, under different management regimes and for over 4 years. The best results were achieved after hand pulling, but due to its high cost, this method is not suitable for large areas. Mechanical cuts, which include the use of brush hogs, brush cutters, and chainsaws, resulted in variable levels of resprouting of A. longifolia stumps. Follow-up treatments, whether mechanical and/or chemical, reduced the number of A. longifolia resprouts and kept its cover below 20% and height below 50 cm. Furthermore, controlling with brush cutters/hogs reduced the seed bank of A. longifolia by 74% while that with a disc harrow allowed an even greater reduction (91%). In areas where no follow-up treatments were carried out, A. longifolia started producing flowers two and a half years after the initial treatment, restarting the cycle of seed production. Nevertheless, a biological control agent that reduces A. longifolia seed production (Trichilogaster acaciaelongifoliae) establishment was confirmed in the controlled areas, suggesting that new seed production by these plants will be soon controlled. Although species characteristic of the protected habitats are currently poorly represented, it is expected that they begin to emerge with the reduction of A. longifolia. Our results stress the importance of persistence of follow-up treatments if higher success in IAPs control is to be achieved, not only because of the resprouting ability of many species (despite this not being very common in A. longifolia) but also because invasive plants can produce extensive persistent seed banks. The role of the management regime on the level of success in controlling IAPs will be discussed.
1 Introduction
Invasive alien species are the fifth major threat to biodiversity worldwide (IPBES, 2019) and present a major challenge to the managers of protected areas that often have limited funds for the conservation of biodiversity (Pyšek et al., 2013). Despite economic assessments and reporting of invasive alien species in protected areas being relatively scarce, it is clear that post-invasion management represents the highest proportion of the observed costs (Foxcroft et al., 2013; Moodley et al., 2022). Therefore, management of invasive alien species is often hampered by the shortage of economic resources and also by the lack of clear policies and specialized staff (Braun et al., 2016; Paganelli et al., 2021).
Successful management of invasive alien plants (IAPs) is frequently difficult and implies prohibitive costs (McConnachie et al., 2012; Diagne et al., 2021; Novoa et al., 2021), thus effective responses are urgently needed. First, it is crucial to define a clear strategy, that is, whether it should be prevention, early detection and rapid response, or management per se (which includes control). Then, one must prioritize both the species and areas to control and adjust the objectives in light of the available budget (van Wilgen et al., 2012; Marchante H. et al., 2019). With rising costs, management is often the last option and also the most expensive, especially when needed in the long term (Simberloff et al., 2013), urging its better optimization.
Active management methodologies include mechanical or physical, chemical, and biological methods, alone or combined (integrated management), to eradicate, contain, or control populations of IAPs (Simberloff, 2013; Marchante H. et al., 2019). The term ‘eradication’ is often used colloquially and does not always mean the successful removal of every single individual (Simberloff, 2003). While there are several reports on animal eradication, plant eradication is less frequent (Simberloff et al., 2018), being an unrealistic goal, especially for widespread plants with vegetative propagation or long-lived seed banks (Rejmánek and Pitcairn, 2002; Mack and Foster, 2008). For long-standing invasions of such species, containment or control is the most appropriate goal to limit or reduce the spread to an acceptable level (Hulme, 2006). A plan to effectively contain or control an IAP that resprouts, coppices, or has a persistent seed bank such as the Acacia species must include three levels: 1) initial, 2) follow-ups, and 3) maintenance control (Le Maitre et al., 2011; Wilson et al., 2011; Gioria et al., 2012). Thorough follow-up efforts are mandatory until acceptable levels of germination or regrowth of the IAP allow keeping up with a maintenance control, over a longer term. Otherwise, without maintenance, previous control investments tend to be lost (Cheney et al., 2019). Ultimately, eradication may be achieved through long-term maintenance control, but it requires appropriate funding resources and high stakeholders’ commitment (Simberloff et al., 2018; Pyšek et al., 2020).
Despite often being neglected, evaluating the successes and failures of different management actions through monitoring programs is an essential component of effective IAPs management (Wilson et al., 2011; Foxcroft et al., 2017). Therefore, setting goals to monitor progress within a framework of adaptive management (Kettenring and Adams, 2011; Downey, 2013; Foxcroft et al., 2013) and engagement with stakeholders in real life contexts (Shackleton et al., 2019) may be the key to improve IAPs management (McConnachie et al., 2012; van Wilgen et al., 2012), especially if documented and shared (Wilson et al., 2011).
Acacia longifolia (Andrews) Willd., also known as the golden wattle or long-leaved wattle, is a shrub or small tree (Fabaceae: Mimosoideae) native from SE Australia that was introduced in Portugal in the late 19th century by the National Forest Administration (Maslin and McDonald, 2004; Fernandes, 2012). Used mainly for dune stabilization and soil improvement, it has become one of the most widespread IAPs along the Portuguese coastal dunes and maritime pine (Pinus pinaster Aiton) forests (César de Sá et al., 2017). This Acacia species is widely recognized as a transformer species [sensu Richardson et al. (2000)] with severe negative impacts on soil ecology (Marchante et al., 2008a; 2008b), displacing plant communities (Le Maitre et al., 2011; Marchante et al., 2015; Marchante E et al., 2019) and simplifying plant–insect interactions (López-Núñez et al., 2017). Acacia longifolia is a prolific producer of hard-coated seeds (up to 11,500 seeds/m2 × year) (Gibson et al., 2011) that can remain viable for 50 years or more (Pieterse and Cairns, 1987; Richardson and Kluge, 2008; Marchante et al., 2010) and are mostly incorporated in the top 10 cm of the soil (Wilson et al., 2011). Depending on the age of the invaded stand, A. longifolia soil-stored seed bank can vary between 500 (recently invaded areas) and 1,500 to up to 34,000 seeds/m2 (long invaded) (Pieterse, 1987; Marchante et al., 2010), which are triggered to germinate en mass by fire (or other disturbances) (Le Maitre et al., 2011).
The mechanical removal strategy is relatively effective for A. longifolia adult plants (Wilson et al., 2011), but without thorough follow-up treatments, the cleared areas are simply reinvaded with plants originating from seeds and sometimes from resprouts. As this species starts producing seeds at 2 years of age (Milton and Hall, 1981), the clearance results in low levels of success. As such, reducing seed production (using, e.g., biological control agents), and consequently, the seed bank, is crucial to prevent widespread reinvasion after removal, but dedicated seed studies are scarce (Richardson and Kluge, 2008; Le Maitre et al., 2011; Wilson et al., 2011), especially in Portugal (Marchante et al., 2010).
The applied project “GANHA—Sustainable management of Acacia spp. (POSEUR-03-2215-FC-000052)” focused on the integration of different techniques to control Acacia species, such as A. longifolia, in Portuguese Natura 2000 sites and protected areas. The added value of this project results from 1) the selection of effective control techniques considering the characteristics of A. longifolia; 2) persistence of follow-up treatments, avoiding the single-cut approach that often results in reinvasion; 3) use of the biocontrol agent Trichilogaster acaciaelongifoliae in reducing seed production; and 4) the promotion of a close collaboration between the operational staff, managers, and academics in an adaptive management approach. The project aimed a reduction of 70% of A. longifolia over 4 years and included management-focused research in monitoring IAPs initial and follow-up control treatments (Kettenring and Adams, 2011; Foxcroft et al., 2017). Therefore, this work reports findings arising from an applied IAPs management approach, which combined landowners' aims, interests, and decisions with their limitations, instead of a standard scientific experimental design.
The general aim of this study is to evaluate the efficacy of different control methods of A. longifolia in forest plantations with distinct production and management priorities in two Natura 2000 sites in Portugal. For this, different treatments were used to remove A. longifolia in order to assess its recovery during 4 years. In this applied study, our specific aims were 1) to compare the effectiveness of different treatments in reducing A. longifolia cover; 2) to determine the dimension and viability of the A. longifolia seed bank and the extent of its reduction due to follow-up controls and past management; and 3) to evaluate plant recovery, such as natives, after initial and follow-up controls, to assess the effectiveness of the number of follow-up controls. We hypothesize that 1) the combination of different methods to control A. longifolia reduces its cover and promotes the recovery of native vegetation; 2) areas with extensive A. longifolia seed banks are more reinvaded than areas with smaller seed banks; and 3) follow-up treatments reduce the recovery of A. longifolia.
2 Materials and methods
2.1 Study area
This study took place in forest plantations on coastal dunes in two Natura 20001 sites: PTCON0055—Dunas de Mira, Gândara e Gafanhas (Vagos Dunes Forest Perimeter, hereafter the Vagos dunes) and PTCON0034—Comporta/Galé (hereafter the Monte Feio) between 2017 and 2021 (Figure 1). The climate is Mediterranean with warm summers (Csb) in the north and hot summers (Csa) in the south (Beck et al., 2018).
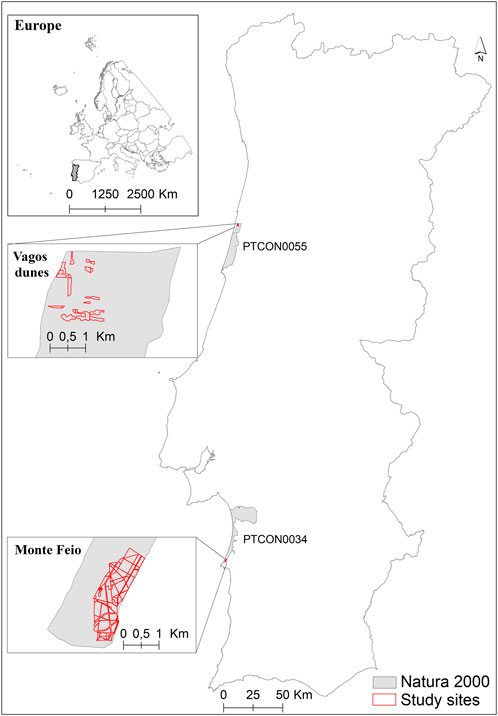
FIGURE 1. Location of the study and Natura 2000 selected sites in Portugal. Vagos dunes is a maritime pine forest (Pinus pinaster) managed by the public forest services, while Monte Feio is a private property where blue gum (Eucalyptus globulus) is planted for industrial forestry. Both areas are classified in Natura 2000 sites (PTCON0055 and PTCON0034) and are invaded by Acacia longifolia.
Vagos dunes (40.535906, −8.742057) host a maritime pine forest of Pinus pinaster (habitat 2270*) (Faulks, 2007). This native species was sown along with native shrubs and A. longifolia from 1929 to 1935 to curb the movement of sands (Plano de Povoamento Florestal, 1940). A. longifolia has become invasive replacing several protected psammophilous species and protected habitats (namely, 2150*, 2170, 2190, and 2260, whose characteristic species are Ulex europaeus subsp. latebracteatus, Salix arenaria, Scirpoides holoschoenus, and Stauracanthus genistoides, respectively) and threatening the pine habitat 2270* (dominated by Pinus pinaster). This area is managed by the public forest services prioritizing protection, conservation, and leisure, with sporadic actions for resin extraction and IAPs management. Maritime pine is a part of the native habitats to be conserved, coexisting with other protected habitats. Vagos dunes are one of the few pine forests in littoral dunes spared by the recent wildfires of 2017 in Portugal (ICNF, 2019).
Monte Feio at Sines (37.998038, −8.838421) is a private property, since 2000, where blue gum (Eucalyptus globulus Labill., an Australian species) was planted for industrial forestry, with regular IAPs management actions. Although there are conserved habitats in about one-third of the Monte Feio property (justifying the Natura 2000 classification), in the study area, the blue gum plantation is managed for pulp not focusing on the conservation of the native vegetation. In the plantation area, native species co-occur but are sporadic. A. longifolia in this site is both a nuisance to production goals and a threat to the remaining native dune plants and several Natura 2000 habitats (e.g., 2130*, 2150*, 2250, and 2330, whose characteristic species are Thymus carnosus, Ulex australis subsp. welwitschianus, Juniperus turbinata, and Corynephorus canescens var. maritimus, respectively). The management of the IAPs at both sites aims at not only protecting the classified habitats but also reducing fire risks and increasing blue gum production at Monte Feio.
Dune systems are fragile and as such interventions that cause high levels of disturbance should be avoided. Usually, managers of both sites mechanically clear cut A. longifolia stands to reduce the vegetation cover which, depending on the forest purpose, can be done more or less regularly in time. While pine trees have already surpassed their 70-year life span (AFBV, 2016), eucalyptus plantations require more interventions due to coppicing every 8–12 years (Cerasoli et al., 2016).
Besides A. longifolia, other IAPs occur sporadically in the study areas that include Acacia mearnsii, Acacia dealbata, Acacia saligna, and Carpobrotus edulis, among others.
2.2 Acacia longifolia control methodologies
The removal of A. longifolia within the GANHA project included one initial control and at least one or two follow-up treatments, executed between September 2017 and June 2020, comprising mainly mechanical methods that included selective or extensive cutting, either using a brush cutter or brush hog (Table 1). More details on the control methods used, such as main strengths, weaknesses, and outcomes, are provided in Supplementary Table S1. Seven monitoring groups, hereafter the groups, were defined based on the different removal methods applied in each area: in Vagos dunes, group VI differs from VII because, in the last, A. longifolia was in the undercover of pine trees, preventing the use of brush hog. In Monte Feio, A. longifolia was hand pulled in a small parcel due to the initial low levels of invasion (group I). Unlike other invasive Acacia species, A. longifolia often does not resprout after cutting. As such, herbicide was not applied immediately after cutting, but rather at follow-up controls, spraying glyphosate (360 g/L at 5%) on resprouts or saplings which were less than 50 cm tall (groups I–V). The last treatment interval between brush cutter/hog cuts and glyphosate was about 1 month in groups I–III and 7 months in groups IV and V. The control interventions were performed in 156.4 ha of Monte Feio (Sines), of which 108.3 ha were monitored in this work, and in 35.5 ha of Vagos dunes, which were all monitored. Monitoring took place from July 2017 to April 2021 and included the soil seed bank evaluation.
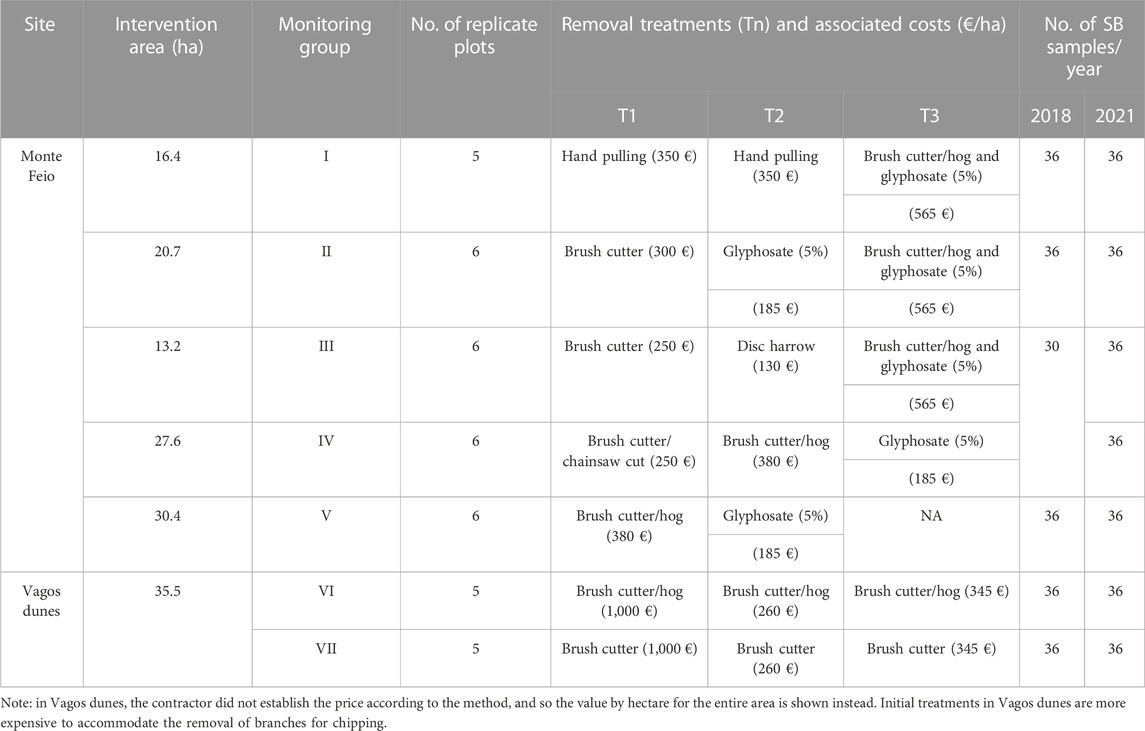
TABLE 1. Removal methods and associated monitoring groups in each site. Tn refers to the removal treatment applied: T1—initial control, T2—first follow-up control, and T3—second follow-up control. Information on the intervention costs (€/ha) paid to contractors is provided within brackets. Detailed information on each treatment is provided in Supplementary Table S1. SB means seed bank.
2.2.1 Monitoring of control effectiveness
A. longifolia areas in each site were characterized (cover percentage and height classes) previous to the removal treatments, and permanent monitoring plots were established (n = 10 in Vagos dunes and n = 29 in Monte Feio), proportional to the size of the areas of intervention and treatments. High coverage of Rubus sp. in Monte Feio and of A. longifolia in Vagos dunes (80%) represented an obstacle to circulation, thus monitoring plots V, VI, and VII were only settled after the initial control. Each plot with 8 m × 4 m was marked, and the central point was marked and georeferenced with a handheld GPS (ER Mapper with MM Field). The plots were monitored 4 months after each treatment (T1—initial control, T2—first follow-up control, and T3—second follow-up control) to assure their evaluation before the next follow-up treatment, except for groups IV and V in Monte Feio that were accessed 6 and 14 months after the treatment: T2 and T3 (IV) and T1 and T2 (V), respectively. Other monitoring visits were seldom carried out to minimize practical constraints, such as lack of manpower, which sometimes delayed planned treatments.
The total vascular plants cover percentage inside the plots was also accessed by visual estimation in each monitoring evaluation, distinguishing A. longifolia from the other species such as Pinus pinaster, Eucalyptus globulus, and shrubs or herbaceous plants; the cover by the other plants may contribute to the prevention of acacia recovery (Marchante et al., 2011a). Furthermore, the cover of A. longifolia resulting from seedlings or sprouts and its predominant height were recorded. The number of stumps in each plot was counted and checked for resprouts in the last monitoring visit to access the final treatment effect.
2.2.2 Seed bank density
Sampling was conducted at both sites in the initial and final phases of the project. The initial sampling periods were variable, from July 2017 to June 2019, depending on the timing of the first control treatment (T1), but always ensuring that they were done before or just after the first control treatments (in plots where dense vegetation cover did not allow sampling before treatments) and when trees did not have dehiscent pods. The final sampling was done between March and April 2021, after two (T3, in most monitoring groups) or one follow-up treatment (T2, in group V), corresponding to nine (groups I, II, and III) and 19 (groups IV, V, VI, and VII) months after the last control treatment. This difference in the final sampling time may have influenced the results, but for logistical and meteorological reasons, it was not possible to sample all the plots at the same time. However, the influence is not expected to be significant because the sampling was always done after the control treatments, and the plants did not produce seeds in the meantime, the soil seed bank was numerous before the start of the treatments, and Acacia seeds are long lived. Nevertheless, this difference was taken into account in the analysis of the results. A total of 72 samples were collected in each phase in Vagos dunes, and in Monte Feio, 138 and 180 samples were collected in the initial and final phases, respectively. A minimum of 36 samples were collected in each monitoring group, except for groups III and IV, where the areas were considered similar and the same type of treatments was initially expected to be carried out. A total of 30 samples were collected in these two groups.
As seeds are accumulated under the canopy of the parent trees (Marchante et al., 2010), samples were collected 2–3 m away from the cut stumps, whenever visible. In hand-pulled areas, we looked for Acacia phyllodes litter to select the sampling point. A. longifolia litter layer is responsible for differences in invaded seed banks due to the accumulation of seeds over time (Marchante et al., 2011b). To better understand this effect, and as a result of the accumulation of phyllodes and branches in the cleared areas, litter was separated from the soil (mostly sand). At each sampling point, unconsolidated litter within a circle of 17 cm diameter was collected with a shovel to a plastic bag with a numbered tag. Afterward, three soil samples were taken at the same point with a corer (7.5 cm diameter × 10 cm deep; area 44 cm2) and placed into plastic bags, as described above. Moisture samples were forced air oven dried (38°C for 24–48 h). All samples were characterized and sieved (using 5-mm and then 2-mm mesh; Filtra Vibracion, Spain, and Retsch, Haan, Germany, respectively) to extract and count A. longifolia seeds. The average litter, soil, and total (litter + soil) seed bank size were calculated and expressed as the number of seeds per area.
2.2.2.1 Seed viability
To test seed viability (Marchante et al., 2010; Strydom et al., 2019), a subsample of at least 250 seeds was taken from the seed pool from each site and sampling year, whenever available, and two replicas were made (litter + soil). The seeds were sterilized (Crisóstomo et al., 2007) prior to scarification (cut at the micropylar end with a scalpel) and placed in sterilized glass Petri dishes containing sterilized cotton and filter paper moistened with autoclaved water.
Petri dishes, each with 50 seeds, were sealed with a plastic film to avoid moisture loss and incubated at 25°C in the dark (Pieterse and Cairns, 1986; Strydom et al., 2019). The seeds were checked for germination after 3 days and thereafter for every other day until rotten. The germinated seeds, those with at least a 2-mm radicle, were counted and removed.
2.2.3 Initial and follow-up control treatment effects
In Vagos dunes, 24 additional 2-m quadrats were monitored in June 2020 (8 months after the last treatment) to assess the initial and follow-up removal treatment effects in more detail. Monitoring plots included 1) areas not cleared (zero treatments); 2) quadrats that only had the initial treatment (T1 and zero follow-ups); and 3) the cleared area with the initial treatment (T1) and two follow-up treatments (T2 and T3). Each plot was evaluated for A. longifolia: 1) cover; 2) height; and 3) total number of individuals separated by plants with flower buds/ pods or galls of T. acaciaelongifoliae. The establishment of T. acaciaelongifoliae in Vagos dunes had been confirmed in 2019 with 733 galls observed (López-Núñez et al., 2021). Since then, the biocontrol agent has spread reaching the study areas for the first time in June 2020.
2.3 Statistical analysis
The effectiveness of different removal methodologies over time (T1 to T3) was analyzed with linear mixed models (LMMs) for each monitoring group (I–VII). For this, A. longifolia and the other vegetation cover (log or root square transformed) were used as response variables, the treatment as a fixed factor, and the monitoring plots as a random factor. An identical analysis was performed to evaluate A. longifolia germination and sprouts’ height (root square transformed) as a response variable. The comparison between the monitoring groups was evaluated considering the proportion of stumps with sprouts accessed after the last treatment using a generalized linear model (GLM).
Differences between the initial and final soil seed bank density [log(x + 1) transformed] were accessed with LMMs using the monitoring groups as the fixed effect and the site as a random factor. The differences were further explored with generalized least square (GLS) fitted models to access what was the source (soil or litter) for each monitoring group. Another GLM was used to access seed viability differences between sites, sampling years, and source.
Finally, the effectiveness of the follow-up treatments was analyzed with LMMs for A. longifolia cover, number of plants, and its distribution by height classes as response variables, after being log(x + 1) transformed. The number of treatments was used as a fixed factor and the monitoring plots as a random factor.
The statistical analyses were performed with LMMs, GLSs, and GLMs using the R packages lme4 (Bates et al., 2015), lmerTest (Kuznetsova et al., 2017), nlme (Pinheiro et al., 2022), multcomp (Hothorn et al., 2008), and performance (Lüdecke et al., 2021) in R v.4.1.2 (R Core Team, 2021). The differences between A. longifolia cover, height, frequency of sprouting plants, and the initial and final soil seed banks in each or between the monitoring groups were further explored with a Tukey post-hoc test whenever significant effects were detected in the LMMs.
3 Results
3.1 Removal treatments effectiveness
The initial (T1) and follow-up treatments (T2 and T3) resulted in significant differences in the mean cover of A. longifolia in Monte Feio and Vagos dunes, and on the other vegetation only in Monte Feio (Figure 2; Supplementary Table S2). A. longifolia coverage generally diminished in Monte Feio, especially after hand pulling (monitoring group I, T1) and the last treatment with the brush cutter/hog followed by the application of glyphosate in A. longifolia germination and/or sprouts (groups I, II, and III), or just glyphosate (IV). On the contrary, A. longifolia cover showed a tendency to significantly increase in some areas of Vagos dunes after the first follow-up treatment (T2) in group VI. Yet, despite the observed variations, A. longifolia cover was almost always below 20%.
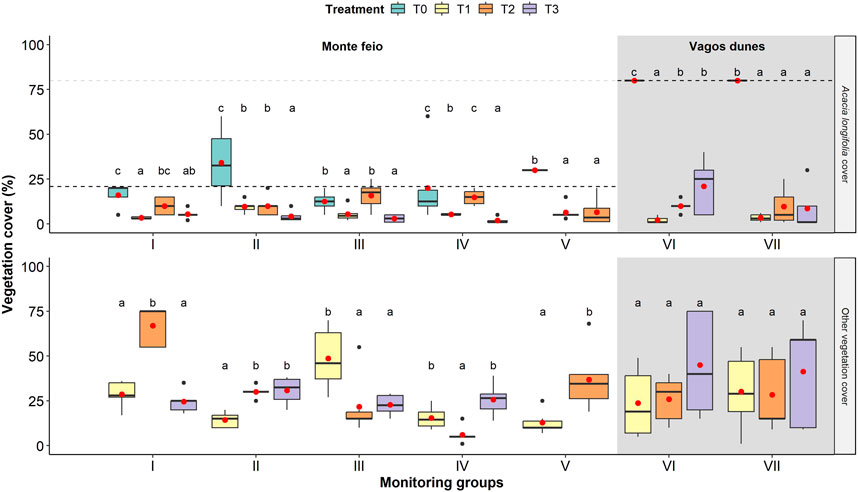
FIGURE 2. Acacia longifolia (left) and other vegetation (right) cover after each treatment (T1, T2, and T3) on different monitoring groups. Monitoring group V had only two treatments (T1 and T2). Letters above whiskers show results of Tukey post-hoc test. The thick horizontal line in each box indicates the median and the red point indicates the mean; the bottom and top of each box indicate the 25th and 75th percentiles, respectively. The vertical lines (whiskers) represent either the maximum/minimum value or 1.5× interquartile range, whichever is closer to the mean. Dark points represent outliers. For details regarding each monitoring group, see Table 1.
The follow-up controls not targeting exclusively A. longifolia, such as the brush hog, can also reduce other vegetation cover. Nevertheless, despite some variability, other vegetation often covered more than 20%, being noteworthy, the high soil cover after the initial selective control (T1) with the brush cutter in monitoring group III and after the hand-pull follow-up treatment (T2) in group I (Figure 2). This cover was mostly provided by Cistus psilosepalus. Several pioneer, anthropic, and/or nitrophilous native plants were also identified, such as Briza maxima, Holcus lanatus, or Andryala integrifolia, which prevailed at both sites, while shrub species such as Dittrichia viscosa, Helichrysum stoechas, or Ulex minor were only frequent in Monte Feio. Plants of coastal dune communities to be conserved were poorly represented: Corynephorus canescens var. maritimus and Juniperus turbinata at Monte Feio or Stauracanthus genistoides at Vagos dunes.
Although the second hand-pull treatment (T2 in group I) was not as effective as the first while concerning A. longifolia cover, it apparently eliminated all sprouted stumps (Supplementary Figure S1). Despite the initial hand-pull effort, this area was set to brush cutter/hog treatment (T3) that reduced both A. longifolia and other vegetation cover, but significantly promoted A. longifolia sprouts (F2 = 31.06, p < 0.001; Figure 3; Supplementary Table S2), even after glyphosate application.
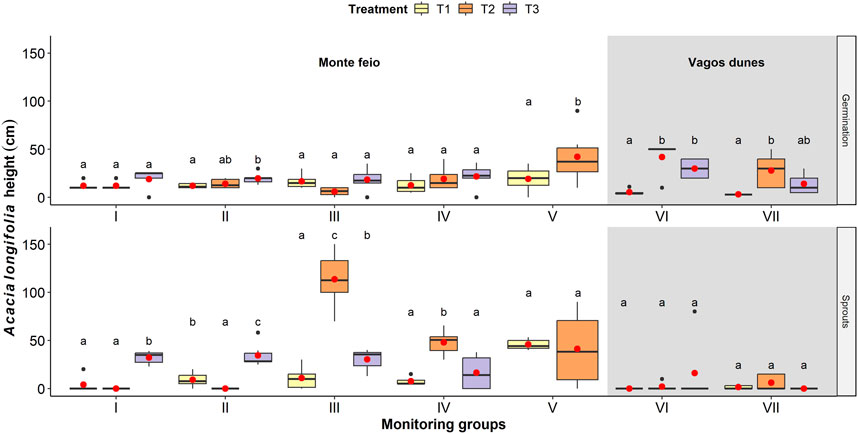
FIGURE 3. Acacia longifolia germination (left) and sprouts (right) dominant height after treatments (T1, T2, and T3) on different monitoring groups. Monitoring group V has only two treatments (T1 and T2). Letters above whiskers show results of Tukey post-hoc test. For details on the boxplots, see Figure 2.
On the contrary, the disc harrow (T2 in group III) and the brush cutter/hog treatments in eucalyptus plantation at Monte Feio (T2 in group IV) resulted in an increase of A. longifolia coverage reaching values similar to the initial treatment (T0; Figure 2). These were followed by a significant increase of A. longifolia sprouts’ height (F2 = 36.1, p < 0.001 and F2 = 10.5, p < 0.05; Figure 3; Supplementary Table S2) when compared to the previous treatment T1.
The formation of sprouts was significantly higher in Monte Feio whenever treatments included A. longifolia cuts as in monitoring groups I, II, III, and IV, but was not significant in Vagos dunes (groups VI and VII; Figure 3; Supplementary Table S2). The proportion of sprouted stumps (Figure 4) was also significantly different among the monitoring groups (χ2 = 15.568, df = 6, p < 0.001), being lower in Vagos dunes, followed by the Monte Feio group IV, that is, areas whose last treatment was solely glyphosate (T3) 6 months after the last cut (T2). In the other areas, the number of sprouted stumps was higher when there were less cuts (groups I and V) or when glyphosate spraying was applied about 1 month after the cuts (T3 in groups II and III). Furthermore, A. longifolia exceeded 50 cm in height especially due to sprouting in groups II to VI (Supplementary Figure S2).
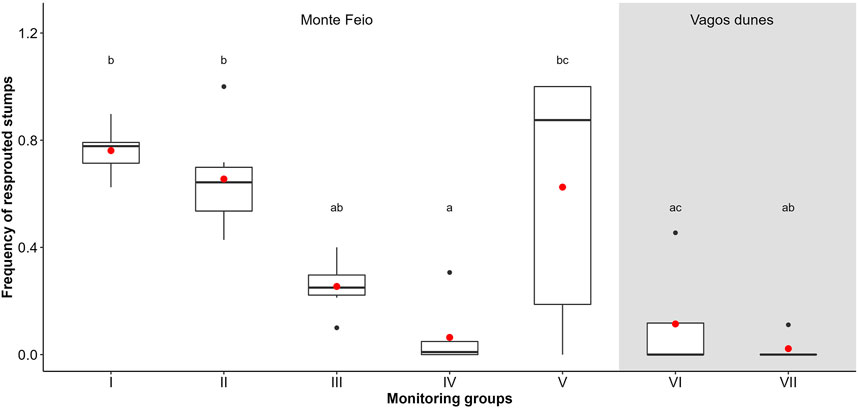
FIGURE 4. Frequency of Acacia longifolia sprouted stumps after the last treatment (T3 or T2 in monitoring group V) on different monitoring groups. Letters above whiskers show results of Tukey post-hoc test. For details on the boxplots, see Figure 2.
3.2 Seed bank density and viability
The average number of seeds stored in the seed bank when the experiment started was significantly higher in Vagos dunes (16,981 ± 2,167 seeds/m2) than in Monte Feio (763 ± 141 seeds/m2; F6 = 6.569, p < 0.001; Figure 5A). The seed bank decreased at both sites after the initial and follow-up treatments, but Vagos dunes continued to have more seeds in the seed bank than that of Monte Feio (4,670 ± 636 seeds/m2 and 345 ± 58 seeds/m2, respectively). Despite the differences between the sites, treatments also produced differences in the final seed bank between monitoring groups I and II, and II and III (F6 = 9.968, p < 0.001), whose sampling was executed 9 months after the last control treatment. In addition, differences were also found between groups III and V. The seed bank density was significantly reduced by 90.6% in group III (F1 = 14.739, p < 0.001), by 74.2% in group VI (F1 = 46.919, p < 0.0001), and by 67.4% in group VII (F1 = 64.669, p < 0.0001), while in the other groups, the reduction was not significant (Figure 5B; Supplementary Table S3).
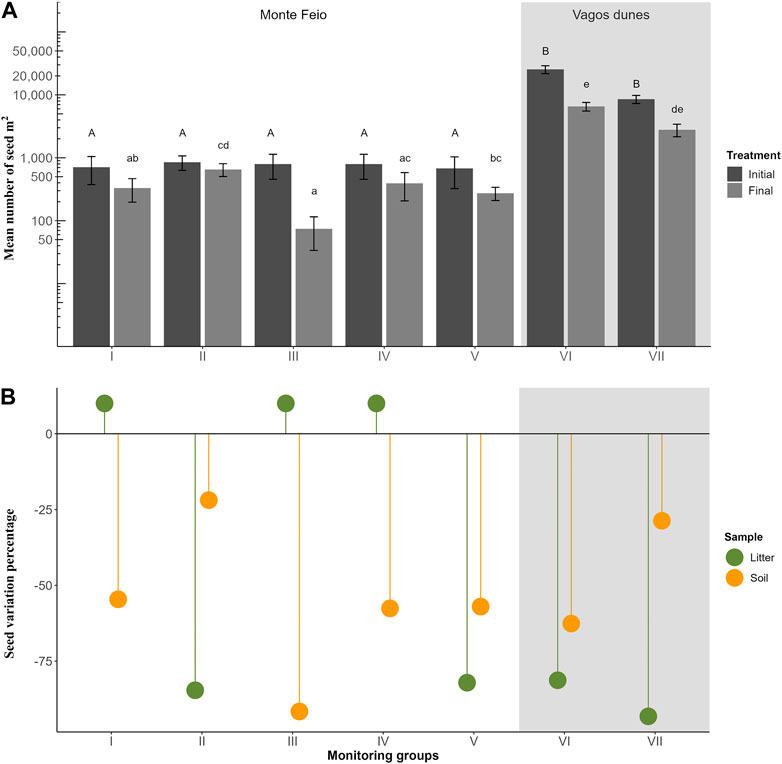
FIGURE 5. Density of Acacia longifolia seed bank on different monitoring groups. (A) Mean number of seeds per m2 ± SE (n = 36) before and right after the initial control treatments (Initial) and after the final follow-up treatments (Final) are represented on a logarithmic scale. Letters show the results of Tukey post-hoc test. (B) Variation in the number of seeds of A. longifolia in litter or soil layers is represented as percentage.
Although seeds had mainly accumulated in the soil, Vagos dunes’ initial seed bank had the highest number of seeds in the litter layer (Supplementary Table S3). After control treatments, the number of seeds in the litter layer was significantly reduced in most situations, reaching 81.3% reduction in monitoring group VI (F1 = 5.095, p < 0.05) and 93.2% in group VII (F1 = 22.373, p < 0.0001), while in groups I, III, and IV, the seeds were observed in this layer for the first time (Figure 5B; Supplementary Table S3). On the other hand, seeds on the soil layer were reduced in all situations, reaching the highest reduction by 91.6% in monitoring group III (F1 = 18.370, p < 0.0001).
Seed viability was high at both sites, being higher than 97.1% ± 1.94 in Vagos dunes and higher than 85.9% ± 6.23 in Monte Feio (Supplementary Table S4). Neither the sampling year nor the source of the seeds (soil or litter) influenced the viability of the seeds in Vagos dunes (year: χ2 = 0.136, df = 1, p = 0.053 and source: χ2 = 0.047, df = 1, p = 0.257). In Monte Feio, the seeds accumulated in the soil and sampled in 2021 showed a significantly higher viability (99.3% ± 0.42; year: χ2 = 0.359, df = 1, p < 0.05 and source: χ2 = 0.617, df = 1, p < 0.01).
3.3 Initial and follow-up control treatment effect
Although A. longifolia cover was significantly reduced with the number of treatments (F2 = 20.264, p < 0.0001; Figure 6A), the total number of acacia plants was similar (F2 = 0.924, p = 0.412), particularly those between 20 and 50 cm high (Figure 6B). The areas with two follow-up treatments had a significantly higher number of small saplings, less than 20 cm high, than those areas with no treatments (none) or one (initial) treatment (0–10 cm: F2 = 4.222, p < 0.1 and 10–20 cm: F2 = 5.953, p < 0.01). On the other hand, the number of bigger plants, above 50 cm high, was significantly higher in plots without treatments than in the plots with one or two treatments: 25.3 ± 6.4 plants/m2 between 50 and 150 cm (F2 = 7.220, p < 0.01); 10.5 ± 3.2 plants/m2 between 150 and 300 cm (F2 = 11.795, p < 0.001), and 2.4 ± 0.4 plants/m2 >300 cm (F2 = 20.154, p < 0.0001). However, plots with one (initial) treatment had a similar number of plants between 150 and 300 cm high than those plots without treatments.
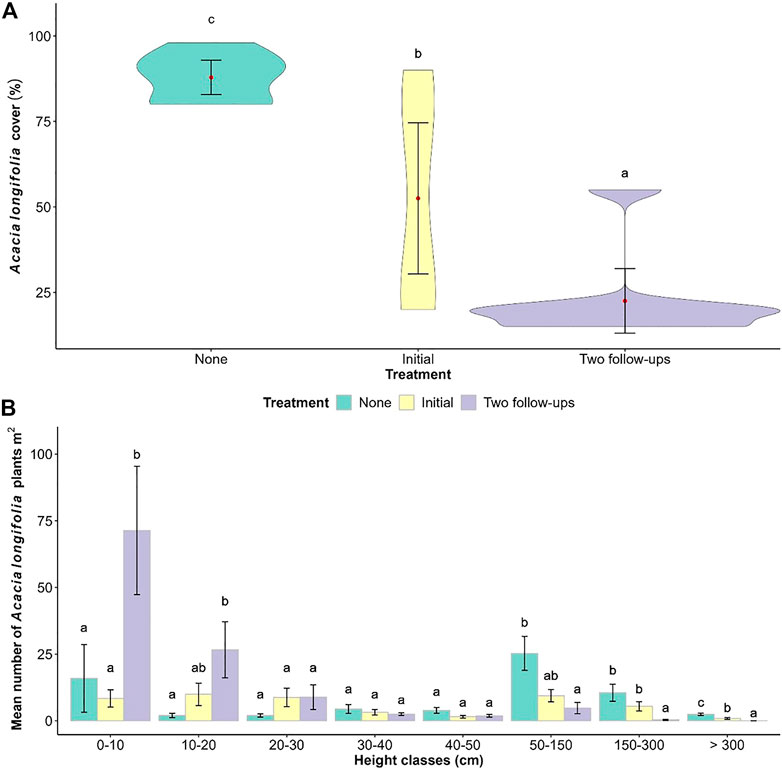
FIGURE 6. Effect of the number of control treatments (0 = none; 1 = initial treatment, and 3 = initial + two follow-ups) on Vagos dunes. (A) Violin plot distribution of A. longifolia cover; the red point indicates the mean and the vertical lines (whiskers) represent the standard error. (B) Mean number of A. longifolia plants per m2 ± SE (n = 8). Letters show results of Tukey post-hoc test.
In plots with no treatments, 87.0 and 76.6% of A. longifolia plants above 300 cm showed flower buds and pods, respectively, but to a lower extent, many of the smaller plants also developed such structures (Supplementary Table S5). Despite the lower percentage, flower buds were also exhibited in some of the plants of plots with only the initial treatment. No flower buds, pods, or galls of Trichilogaster acaciaelongifoliae were produced in plots with two follow-up treatments. Galls of T. acaciaelongifoliae were observed on few A. longifolia plants of all sizes (up to 11%), regardless of whether they had flower buds or not.
4 Discussion
A. longifolia is frequently subjected to active management in Portugal to prevent wildfires, improve accessibility, and protect or promote other plants in conservation or production areas (Marchante et al., 2023), as promoted by the national legislation (Decreto-Lei n.º 92/2019). However, clearing practices have not always achieved the best results, sometimes even aggravating the invasion and dispersal of A. longifolia (César de Sá et al., 2017), possibly due to ineffective clearing methods and lack of follow-up. Nonetheless, detailed analyses of management results are rarely published or made available, preventing a solid support to improving the management of this species (Marchante et al., 2023).
Our study allowed us to evaluate the effect of different combinations of treatments to control A. longifolia both in terms of vegetative and seminal outputs, including the seed bank. This, together with the inclusion of two invaded coastal dune forests with distinct management regimes and real-life management, allowed a more comprehensive view of the management outcomes in such situations. Although our findings must be interpreted with caution, they provide a better understanding of the trend in A. longifolia control efforts. Regardless of the variations between treatments, the persistence of the follow-up treatments proved successful in maintaining A. longifolia cover at a reduced level, frequently below 20%.
Hand-pulling methods are frequently disregarded in professional invasive plants control since they are labor and cost intensive, being used only in smaller areas or those with low levels of invasion, as observed in Monte Feio. While the second hand-pull treatment was not as effective as the first concerning A. longifolia cover, it successfully avoided sprout formation, and its conservative character helped promote the native species to recover, such as Cistus psilosepalus, similar to what had been observed in other situations (Holmes et al., 2020). Likewise, the use of brush cutter alone (group III) allowed the selective removal of A. longifolia, promoting native plants cover whose shadow could help hinder A. longifolia germination, yet, our results showed the opposite trend, that is, a higher A. longifolia cover from germinated plants. Despite using similar selective treatments (brush cutter or chainsaw) and having a higher cover of the native species than A. longifolia, the monitoring groups II and IV exhibited the lowest cover, suggesting a lower potential for native recovery in these areas, possibly due to the regular management of eucalyptus plantations. Furthermore, A. longifolia resprouted after cutting in Monte Feio, and these sprouts tended to grow higher than the germinated plants in the same areas. This has become clear after the disc harrow treatment, since this technique only removed A. longifolia in the space between the eucalyptus plantation lines, sparing those that were previously cut with a brush cutter next to eucalyptus trees. Sprouts could overpass 1 m height in just 1 year after the initial treatment, increasing A. longifolia cover, while the spaces between the plantation lines were heavily disturbed with the elimination of native and invasive plants, which resulted in an overall decrease of the other vegetation coverage.
The use of chemical treatment alone, as a follow-up control of A. longifolia, 7 months after brush cutter and brush hog treatments (T3 in group IV and T2 in V), was not always consistent in reducing the invasive plant cover, yet, the other vegetation was favored, possibly as a result of the limited and directed application of glyphosate. Furthermore, despite the longer period of 14 months between glyphosate spraying and our monitoring visit, A. longifolia coverage had remained much reduced, suggesting that this method was successful. As initially hypothesized, the combination of different methods reduced A. longifolia cover, especially when mechanical methods were followed by the use of chemicals for young sprouts and saplings. The use of chemicals in restoration practices can be controversial (Weidlich et al., 2020); however, Monte Feio’s eucalyptus plantation is certified by the Forest Stewardship Council (FSC), and glyphosate is only used in limited areas and in small concentrations. In Vagos dunes, chemicals were not used, yet regular follow-up cuts have revealed positive results.
Native plants that recovered at both sites were mostly pioneers favored by human activities and disturbances, while dune plants were seldom represented. Nonetheless, it was not possible to fully understand the effect of physical, mechanical, or chemical control methods on the restoration of the native vegetation cover as that would take more than 3 years (Boxriker et al., 2022).
The resprouting ability of A. longifolia is seldom reported (Marchante et al., 2004), and this study confirms that A. longifolia resprouting after cuts occurs, with some variations among the sites. Although the air temperature at the two sites is somewhat different, especially during summer, this difference is not expected to be related with climate since regrowth from cut stumps has been observed in São Jacinto dunes, which are close to Vagos dunes and have the same climate (Marchante et al., 2011a). If there is no chance of uprooting A. longifolia plants, and mechanical cut is the most affordable option, moto-manual either with the brush cutter or with a brush hog, then more the follow-up treatments, the better is the success, as observed in Monte Feio. Treatments with a higher number of cuts diminished the number of resprouted stumps, more pronouncedly along time. Interestingly and contrarily to Monte Feio’s, in Vagos dunes A. longifolia almost did not resprout, and the recovery of A. longifolia was mostly due to germination, which had increased with follow-up treatments. While differences in the resprouting ability among sites is not easy to explain and may be related to specific site characteristics, the different response in germination is certainly related with the much larger soil seed bank in Vagos dunes, whose germination was triggered with the brush hog clearing treatments. The seeds were stored in the first 10 cm of the soil (Strydom et al., 2012; Strydom et al., 2017) and those located on the litter registered the largest reduction.
Distinct management regimes and priorities at both study sites in the last 20 years may explain the huge differences in the number of seeds stored in the soil seed bank. As a consequence of more frequent silviculture practices in eucalyptus plantations in Monte Feio, A. longifolia does not reach the age to set large crops of seeds (Milton and Hall, 1981; Richardson and Kluge, 2008). On the other hand, while the initial soil seed bank size in Vagos dunes reflected several years of seeds accumulation without clearing operations or fire events, sometimes getting closer or even overcoming the maximum recorded value for A. longifolia (34,000 seeds/m2: Pieterse, 1987), the significant reduction of 74% with three interventions clearly demonstrate the importance of management persistence (Strydom et al., 2012). Fire is known to break A. longifolia seed dormancy and may even kill the seeds (Pieterse, 1987; Le Maitre et al., 2011); our study highlights that disturbances associated with control treatments also promote germination, contributing to deplete the seed bank. Indeed, the number of follow-ups and the level of disturbance promoted by the control treatments seem to reduce the seed bank more than time since the last treatment. However, even if less numerous, the seed bank persisted in the soil at the end of our monitoring, alerting the need to continue managing A. longifolia in both areas. Our data do not predict how many years are required to deplete the seed bank, but predict that in the absence of follow-up, A. longifolia will certainly germinate (sooner or later) and the invasion will persist, for even one germinating seed is enough to restart the invasion cycle. Distinct management regimes may also help in explaining the different results in terms of control success in the end, since the starting point and context was very different in the two areas.
The smaller experiment in Vagos dunes comparing areas with zero, one, and three follow-up treatments confirm that the number of seedlings was higher in plots with more cuts, which ultimately contributed to reduce the seed bank size. As the total number of A. longifolia plants was similar between these areas, we assume that the time since the last control was the main factor influencing A. longifolia cover and height. Our results also show that two and a half year A longifolia plants reached over 150 cm height and set flower buds, which would develop to pods 1 year later; this is approximately 1 year more than was previously reported by Milton and Hall (1981), but in Portugal, shorter generation times have also been sporadically observed. Even if seldom represented, galls of Trichilogaster acaciaelongifoliae have already been observed on these small plants and are likely to reduce seed production and vegetative vigor. This Australian bud-galling wasp is the best biocontrol agent to reduce A. longifolia seed formation and its vegetative growth, and has been successfully used in South Africa for more than 40 years (Impson et al., 2023). The agent was first released in Portugal in November 2015 (Marchante et al., 2017) and in the study areas in July 2018. The establishment success observed so far suggests it may be a good tool to help in A. longifolia control in Portugal as well (López-Núñez et al., 2021). As flower buds are replaced by T. acaciaelongifoliae galls, pods are not formed, and it is expected that A. longifolia seed banks replenishment will significantly decrease and this will stabilize the seed bank (Richardson and Kluge, 2008). Biological control is the most cost-effective, long-term option with a calculated benefit:cost ratio of 1,465 for A. longifolia (van Wilgen et al., 2004). Thus, combining biological control with clear cut operations of a species, such as A. longifolia, which additionally contributes in depleting the accumulated seed bank, may be the best approach to improve its management, becoming the key element in the restoration of invaded areas.
4.1 Study limitations
This study occurred in parallel with real-life management operations. While this is definitely an advantage as it approximates the academic results from the manager’s needs and real-life results, it also brings some limitations. During the project, some management decisions were made that implied a reduction of the number of monitoring plots/replicates for each methodology and an increase in the number of monitoring groups. For instance, the cut of areas that were previously hand pulled was not initially planned neither was the option for disk arrow or the generalized use of chemicals in all eucalyptus plots. The practical constraints of manpower hiring implied that interventions were not performed at exactly the same time, which implied some variability of the monitoring times. This issue was aggravated by COVID-19 pandemic, which incurred circulation restrictions in the beginning of 2020, with some plots being monitored a few months later than was planned.
5 Conclusion and management implications
For several years, the approach to control IAPs in Portugal, the Acacia species in particular, has been focused on using mechanical methods, sometimes combined with chemical methods, to reduce the density and extension of the invasion. Although in many situations follow-up treatments are scarce or not even planned, jeopardizing initial controls, this is slowly changing. The management regimes that prioritize regular control interventions prevent large seed sets and consequently accumulate less seeds in the seed banks with positive outcomes. This work shows that follow-up treatments are crucial in hampering reinvasion, to keep the A. longifolia cover below 25% and simultaneously reduce its persistent soil seed bank, when more disturbing methods are used, such as the brush hog or disk arrow. Biocontrol with T. acaciaelongifoliae reduces seed rain and may also contribute to the sustainability of control and restoration of invaded sites. Overall, the lessons learned from monitoring the different management actions and soil seed bank evaluations are key to improve the strategy against IAPs, which should include adapting methods for both the invaded situation and available budget. Natural restoration of native plant communities takes time, especially when follow-up treatments are required and unselective control methods favor pioneer vegetation stages. However, once the acceptable levels of germination or regrowth of the IAPs are achieved, the native dune species can be planted or sown to function as barriers for reinvasion.
Data availability statement
The original contributions presented in the study are included in the article/Supplementary Material. Further inquiries can be directed to the corresponding author.
Author contributions
LND, EM, and HM conceived and designed the study; LND monitored all sample plots, collected soil samples, and analyzed the data; LND led the writing of the manuscript with important contributions from all authors; and EM and HM supervised the research. All co-authors contributed critically to the drafts and gave their final approval for publication.
Funding
This research was funded by POSEUR-03-2215-FC-000052, and LND was funded by SFRH/BD/145222/2019. LND, EM, and HM were supported by the Portuguese Science Foundation—FCT/MCTES (Grant UIDB/04004/2020).
Acknowledgments
The authors kindly thank the Institute for Nature Conservation and Forests (ICNF) for the permission to conduct this study in the classified areas and the RAIZ—Forest and Paper Research Institute/The Navigator Company and Vagos Municipality for IAPs treatment supervision and report. The authors also thank many volunteers involved in fieldwork campaigns: A. Gil, L. Mota, F. A. López-Núñez, O. Sobral, A. M. Claro, M. Dinis, J. Palhas, P. Santos, D. Costa, C. Gonçalves, A. Carvalho, A. L. Pereira, F. Barreto, and M. M. Martins. We also thank F. A. López-Núñez for the priceless help with R analysis.
Conflict of interest
The authors declare that the research was conducted in the absence of any commercial or financial relationships that could be construed as a potential conflict of interest.
Publisher’s note
All claims expressed in this article are solely those of the authors and do not necessarily represent those of their affiliated organizations, or those of the publisher, editors, and reviewers. Any product that may be evaluated in this article, or claim that may be made by its manufacturer, is not guaranteed or endorsed by the publisher.
Supplementary material
The Supplementary Material for this article can be found online at: https://www.frontiersin.org/articles/10.3389/fenvs.2023.1113876/full#supplementary-material
Footnotes
1The Natura 2000 network is composed of classified sites that aim to maintain Europe’s most important species and habitats; priority natural habitats are those in danger of disappearance and are indicated by an asterisk (*) (Faulks, 2007).
References
AFBV- Associação Florestal do Baixo Vouga (2016). Plano de Gestão Florestal do Perimetro Florestal das Dunas de Vagos 2016-2026.
Bates, D., Mächler, M., Bolker, B. M., and Walker, S. C. (2015). Fitting linear mixed-effects models using lme4. J. Stat. Softw. 67, 1–48. doi:10.18637/JSS.V067.I01
Beck, H. E., Zimmermann, N. E., McVicar, T. R., Vergopolan, N., Berg, A., and Wood, E. F. (2018). Present and future Köppen-Geiger climate classification maps at 1-km resolution. Sci. Data 5, 180214. doi:10.1038/sdata.2018.214
Boxriker, M., Privett, S. D. J., Richardson, D. M., and Gaertner, M. (2022). Active restoration in South African fynbos–A long-term perspective from the Agulhas Plain. Trans. R. Soc. S. Afr. 77, 133–143. doi:10.1080/0035919X.2022.2087116
Braun, M., Schindler, S., and Essl, F. (2016). Distribution and management of invasive alien plant species in protected areas in Central Europe. J. Nat. Conserv. 33, 48–57. doi:10.1016/j.jnc.2016.07.002
Cerasoli, S., Caldeira, M., Pereira, J., Caudullo, G., and de Rigo, D. (2016). “Eucalyptus globulus and other eucalypts in Europe: Distribution, habitat, usage and threats,” in European atlas of forest tree species. Editors J. San-Miguel-Ayanz, D. de Rigo, G. Caudullo, T. Houston Durrant, and A. Mauri (Luxembourg: Publication Office of the European Union), 90–91.
César de Sá, N., Carvalho, S., Castro, P., Marchante, E., and Marchante, H. (2017). Using landsat time series to understand how management and disturbances influence the expansion of an invasive tree. IEEE J. Sel. Top. Appl. Earth Obs. Remote Sens. 10, 3243–3253. doi:10.1109/JSTARS.2017.2673761
Cheney, C., Esler, K. J., Foxcroft, L. C., and Van Wilgen, N. J. (2019). Scenarios for the management of invasive Acacia species in a protected area: Implications of clearing efficacy. J. Environ. Manage. 238, 274–282. doi:10.1016/j.jenvman.2019.02.112
Crisóstomo, J. J. A., Freitas, H., and Rodríguez-Echeverría, S. (2007). Relative growth rates of three woody legumes: Implications in the process of ecological invasion. Web Ecol. 7, 22–26. doi:10.5194/we-7-22-2007
Diagne, C., Leroy, B., Vaissière, A.-C., Gozlan, R. E., Roiz, D., Jarić, I., et al. (2021). High and rising economic costs of biological invasions worldwide. Nature 592, 571–576. doi:10.1038/s41586-021-03405-6
Downey, P. O. (2013). “Protecting biodiversity through strategic alien plant management: An approach for increasing conservation outcomes in protected areas,” in Plant invasions in protected areas: Patterns, problems and challenges. Editors L. C. Foxcroft, P. Pyšek, D. M. Richardson, and P. Genovesi (Dordrecht: Springer), 507–528.
Fernandes, M. M. (2012). Acácias e geografia histórica: Rotas de um percurso global (parte 1). Cad. Curso Doutor. em Geogr. FLUP 4, 23–40.
Foxcroft, L. C., Pyšek, P., Richardson, D. M., Genovesi, P., and MacFadyen, S. (2017). Plant invasion science in protected areas: Progress and priorities. Biol. Invasions 19, 1353–1378. doi:10.1007/s10530-016-1367-z
Foxcroft, L. C., Pyšek, P., Richardson, D. M., Pergl, J., and Hulme, P. E. (2013). “The bottom line: Impacts of alien plant invasions in protected areas,” in Plant invasions in protected areas: Patterns, problems and challenges. Editors L. C. Foxcroft, P. Pyšek, D. M. Richardson, and P. Genovesi (Dordrecht: Springer), 19–41.
Gibson, M. R. M., Richardson, D. M., Marchante, E., Marchante, H., Rodger, J. G., Stone, G. N., et al. (2011). Reproductive biology of Australian acacias: Important mediator of invasiveness? Divers. Distrib. 17, 911–933. doi:10.1111/j.1472-4642.2011.00808.x
Gioria, M., Pyšek, P., and Moravcová, L. (2012). Soil seed banks in plant invasions: Promoting species invasiveness and long-term impact on plant community dynamics. Preslia 84, 327–350.
Holmes, P. M., Esler, K. J., van Wilgen, B. W., and Richardson, D. M. (2020). Ecological restoration of ecosystems degraded by invasive alien plants in South African Fynbos: Is spontaneous succession a viable strategy? Trans. R. Soc. S. Afr. 75, 111–139. doi:10.1080/0035919X.2020.1781291
Hothorn, T., Bretz, F., and Westfall, P. (2008). Simultaneous inference in general parametric models. Biom. J. 50, 346–363. doi:10.1002/BIMJ.200810425
Hulme, P. E. (2006). Beyond control: Wider implications for the management of biological invasions. J. Appl. Ecol. 43, 835–847. doi:10.1111/j.1365-2664.2006.01227.x
ICNF (2019). Instituto da Conservação da Natureza e das Florestas). Cartografia Nacional de Áreas Ardidas em formato shapefile, 1990–2019. Available at: http://www2.icnf.pt/portal/florestas/dfci/inc/mapas (Accessed June 11, 2021).
Impson, F., Marchante, H., Marchante, E., and Al, E. (2023). “Biological control of Acacia species: History, progress, and prospectsNo Title,” in Wattles - Australian Acacia species around the world. Editors D. M. Richardson, J. J. Le Roux, and E. Marchante (Wallingford, United Kingdom: CABI International).
IPBES (2019). Summary for policymakers of the global assessment report on biodiversity and ecosystem services of the intergovernmental science-policy platform on biodiversity and ecosystem services. Bonn, Germany: IPBES secretariat. doi:10.1111/padr.12283
Kettenring, K. M., and Adams, C. R. (2011). Lessons learned from invasive plant control experiments: A systematic review and meta-analysis. J. Appl. Ecol. 48, 970–979. doi:10.1111/j.1365-2664.2011.01979.x
Kuznetsova, A., Brockhoff, P. B., and Christensen, R. H. B. (2017). lmerTest package: Tests in linear mixed effects models. J. Stat. Softw. 82, 1–26. doi:10.18637/JSS.V082.I13
Le Maitre, D. C., Gaertner, M., Marchante, E., Ens, E.-J., Holmes, P. M., Pauchard, A., et al. (2011). Impacts of invasive Australian acacias: Implications for management and restoration. Divers. Distrib. 17, 1015–1029. doi:10.1111/j.1472-4642.2011.00816.x
López-Núñez, F. A., Heleno, R. H., Ribeiro, S., Marchante, H., and Marchante, E. (2017). Four-trophic level food webs reveal the cascading impacts of an invasive plant targeted for biocontrol. Ecology 98, 782–793. doi:10.1002/ecy.1701
López-Núñez, F. A., Marchante, E., Heleno, R., Duarte, L. N., Palhas, J., Impson, F., et al. (2021). Establishment, spread and early impacts of the first biocontrol agent against an invasive plant in continental Europe. J. Environ. Manage. 290, 112545. doi:10.1016/j.jenvman.2021.112545
Lüdecke, D., Ben-Shachar, M., Patil, I., Waggoner, P., and Makowski, D. (2021). performance: An R package for assessment, comparison and testing of statistical models. J. Open Source Softw. 6, 3139. doi:10.21105/joss.03139
Mack, R. N., and Foster, S. K. (2008). “Eradicating plant invaders: Combining ecologically-based tactics and broad-sense strategy,” in Management of invasive weeds. Editor Inderjit (Dordrecht: Springer), 35–60. doi:10.1007/978-1-4020-9202-2_3
Marchante, E., Kjøller, A., Struwe, S., and Freitas, H. (2008a). Invasive Acacia longifolia induce changes in the microbial catabolic diversity of sand dunes. Soil Biol. biochem. 40, 2563–2568. doi:10.1016/j.soilbio.2008.06.017
Marchante, E., Kjøller, A., Struwe, S., and Freitas, H. (2008b). Short- and long-term impacts of Acacia longifolia invasion on the belowground processes of a Mediterranean coastal dune ecosystem. Appl. Soil Ecol. 40, 210–217. doi:10.1016/j.apsoil.2008.04.004
Marchante, E., Marchante, H., Freitas, H., Kjøller, A., and Struwe, S. (2019). Decomposition of an N-fixing invasive plant compared with a native species: Consequences for ecosystem. Appl. Soil Ecol. 138, 19–31. doi:10.1016/j.apsoil.2019.02.016
Marchante, E., Skulska, I., Colaço, C., Ulm, F., Gonzalez, L., Duarte, L., et al. (2023). “Management of invasive Australian Acacia species in the iberian peninsula,” in Wattles - Australian Acacia species around the world. Editors D. M. Richardson, J. J. Le Roux, and E. Marchante (Wallingford, United Kingdom: CABI International).
Marchante, H., Freitas, H., and Hoffmann, J. H. (2011a). Post-clearing recovery of coastal dunes invaded by Acacia longifolia: Is duration of invasion relevant for management success? J. Appl. Ecol. 48, 1295–1304. doi:10.1111/j.1365-2664.2011.02020.x
Marchante, H., Freitas, H., and Hoffmann, J. H. (2010). Seed ecology of an invasive alien species, Acacia longifolia (Fabaceae), in Portuguese dune ecosystems. Am. J. Bot. 97, 1780–1790. doi:10.3732/ajb.1000091
Marchante, H., Freitas, H., and Hoffmann, J. H. (2011b). The potential role of seed banks in the recovery of dune ecosystems after removal of invasive plant species. Appl. Veg. Sci. 14, 107–119. doi:10.1111/j.1654-109X.2010.01099.x
Marchante, H., López-Núñez, F. A., Freitas, H., Hoffmann, J. H., Impson, F., and Marchante, E. (2017). First report of the establishment of the biocontrol agent Trichilogaster acaciaelongifoliae for control of invasive Acacia longifolia in Portugal. EPPO Bull. 47, 274–278. doi:10.1111/epp.12373
Marchante, H., Marchante, E., Buscardo, E., Maia, J., and Freitas, H. (2004). Recovery potential of dune ecosystems invaded by an exotic Acacia species. Weed Technol. 18, 1427–1433. doi:10.1614/0890-037x(2004)018[1427:rpodei]2.0.co;2
Marchante, H., Marchante, E., Freitas, H., and Hoffmann, J. H. (2015). Temporal changes in the impacts on plant communities of an invasive alien tree, Acacia longifolia. Plant Ecol. 216, 1481–1498. doi:10.1007/s11258-015-0530-4
Marchante, H., Palhas, J., López-Núñez, F. A., and Marchante, E. (2019). “Invasive species impacts and management,” in Life on land. Encyclopedia of the UN sustainable development goals. Editors W. Leal Filho, A. Azul, L. Brandli, P. Özuyar, and T. Wall (Cham: Springer), 1–12. doi:10.1007/978-3-319-71065-5_85-1
Maslin, B., and McDonald, M. W. (2004). AcaciaSearch: Evaluation of Acacia as a woody crop option for southern Australia. Canberra: Rural Industries Research and Development Corporation.
McConnachie, M. M., Cowling, R. M., van Wilgen, B. W., and McConnachie, D. A. (2012). Evaluating the cost-effectiveness of invasive alien plant clearing: A case study from south Africa. Biol. Conserv. 155, 128–135. doi:10.1016/j.biocon.2012.06.006
Milton, S. J., and Hall, A. V. (1981). Reproductive biology of Australian acacias in the south-Western cape province, South Africa. Trans. R. Soc. S. Afr. 44, 465–487. doi:10.1080/00359198109520589
Moodley, D., Angulo, E., Cuthbert, R. N., Leung, B., Turbelin, A., Novoa, A., et al. (2022). Surprisingly high economic costs of biological invasions in protected areas. Biol. Invasions. 24, 1995–2016. doi:10.1007/s10530-022-02732-7
Novoa, A., Moodley, D., Catford, J. A., Golivets, M., Bufford, J., Essl, F., et al. (2021). Global costs of plant invasions must not be underestimated. NeoBiota 69, 75–78. doi:10.3897/neobiota.69.74121
Paganelli, D., Reino, L., Capinha, C., and Ribeiro, J. (2021). Exploring expert perception of protected areas’ vulnerability to biological invasions. J. Nat. Conserv. 62, 126008. doi:10.1016/j.jnc.2021.126008
Pieterse, P. (1987). Acacia longifolia and its control: To burn or not to burn? Veld Flora 73, 67–68.
Pieterse, P. J., and Cairns, A. L. P. (1986). An effective technique for breaking the seed dormancy of Acacia longifolia. South Afr. J. Plant Soil 3, 85–87. doi:10.1080/02571862.1986.10634196
Pieterse, P. J., and Cairns, A. L. P. (1987). The effect of fire on an Acacia longifolia seed bank and the growth, mortality and reproduction of seedlings establishing after a fire in the South West Cape. South Afr. J. Bot. 1, 34–38.
Pinheiro, J., Bates, D., DebRoy, S., and Sarkar, D. (2022). nlme: Linear and nonlinear mixed effects models. R package version 3.1-155. Available at: https://CRAN.R-project.org/package=nlmeorg (Accessed November 14, 2022).
Pyšek, P., Genovesi, P., Pergl, J., Monaco, A., and Wild, J. (2013). “Plant invasions of protected areas in Europe: An old continent facing new problems,” in Plant invasions in protected areas: Patterns, problems and challenges. Editors L. C. Foxcroft, P. Pyšek, D. M. Richardson, and P. Genovesi (Dordrecht: Springer), 209–240.
Pyšek, P., Hulme, P. E., Simberloff, D., Bacher, S., Blackburn, T. M., Carlton, J. T., et al. (2020). Scientists’ warning on invasive alien species. Biol. Rev. 95, 1511–1534. doi:10.1111/brv.12627
R Core Team (2021). R: A language and environment for statistical computing. Available at: https://www.r-project.org/.
Rejmánek, M., and Pitcairn, M. J. (2002). “When is eradication of exotic pest plants a realistic goal?,” in Turning the tide: The eradication of invasive species. Editors C. R. Veitch, M. N. Clout, and Switzerland Gland (Cambridge, UK: IUCN), 249–253.
Richardson, D. M., Pysek, P., Rejmánek, M., Barbour, M. G., Panetta, F. D., and West, C. J. (2000). Naturalization and invasion of alien plants: Concepts and definitions. Divers. Distrib. 6, 93–107. doi:10.1046/j.1472-4642.2000.00083.x
Richardson, D. M., and Kluge, R. L. (2008). Seed banks of invasive Australian Acacia species in South Africa: Role in invasiveness and options for management. Perspect. Plant Ecol. Evol. Syst. 10, 161–177. doi:10.1016/j.ppees.2008.03.001
Shackleton, R. T., Adriaens, T., Brundu, G., Dehnen-Schmutz, K., Estévez, R. A., Fried, J., et al. (2019). Stakeholder engagement in the study and management of invasive alien species. J. Environ. Manage. 229, 88–101. doi:10.1016/j.jenvman.2018.04.044
Simberloff, D. (2003). Eradication – preventing invasions at the outset. Weed Sci. 51, 247–253. doi:10.1614/0043-1745(2003)051[0247:epiato]2.0.co;2
Simberloff, D. (2013). Invasive species: What everyone needs to know. New York: Oxford University Press. doi:10.1093/wentk/9780199922017.001.0001
Simberloff, D., Keitt, B., Will, D., Holmes, N., Pickett, E., and Genovesi, P. (2018). Yes we can! Exciting progress and prospects for controlling invasives on islands and beyond. West. North Am. Nat. 78, 942–958. doi:10.3398/064.078.0431
Simberloff, D., Martin, J. L., Genovesi, P., Maris, V., Wardle, D. A., Aronson, J., et al. (2013). Impacts of biological invasions: What’s what and the way forward. Trends Ecol. Evol. 28, 58–66. doi:10.1016/j.tree.2012.07.013
Strydom, M., Esler, K. J., and Wood, A. R. (2012). Acacia saligna seed banks: Sampling methods and dynamics, Western Cape, South Africa. South Afr. J. Bot. 79, 140–147. doi:10.1016/j.sajb.2011.10.007
Strydom, M., Veldtman, R., Ngwenya, M. Z., and Esler, K. J. (2017). Invasive Australian Acacia seed banks: Size and relationship with stem diameter in the presence of gall-forming biological control agents. PLoS One 12, e0181763. doi:10.1371/journal.pone.0181763
Strydom, M., Veldtman, R., Ngwenya, M. Z., and Esler, K. J. (2019). Seed survival of Australian Acacia in the Western Cape of South Africa in the presence of biological control agents and given environmental variation. PeerJ 7, e6816. doi:10.7717/peerj.6816
van Wilgen, B. W., de Wit, M. P., Anderson, H., Le Maitre, D. C., and Kotze, I. M. (2004). Costs and benefits of biological control of invasive alien plants: Case studies from south Africa. S. Afr. J. Sci. 100, 113–122.
van Wilgen, B. W., Forsyth, G. G., Le Maitre, D. C., Wannenburgh, A., Kotzé, J. D. F., van den Berg, E., et al. (2012). An assessment of the effectiveness of a large, national-scale invasive alien plant control strategy in South Africa. Biol. Conserv. 148, 28–38. doi:10.1016/j.biocon.2011.12.035
Weidlich, E. W. A., Flórido, F. G., Sorrini, T. B., and Brancalion, P. H. S. (2020). Controlling invasive plant species in ecological restoration: A global review. J. Appl. Ecol. 57, 1806–1817. doi:10.1111/1365-2664.13656
Keywords: plant removal, hand pulling, mechanical control, monitoring, resprouts, seed bank, seed viability, seedlings
Citation: Duarte LN, Marchante E and Marchante H (2023) Managing an invasive tree in coastal dunes: The importance of follow-up treatments to improve the recovery of protected habitats. Front. Environ. Sci. 11:1113876. doi: 10.3389/fenvs.2023.1113876
Received: 01 December 2022; Accepted: 23 January 2023;
Published: 10 February 2023.
Edited by:
Emilio Badalamenti, University of Palermo, ItalyReviewed by:
Margarita Arianoutsou, National and Kapodistrian University of Athens, GreeceNicola J. Van Wilgen, South African National Parks, South Africa
Copyright © 2023 Duarte, Marchante and Marchante. This is an open-access article distributed under the terms of the Creative Commons Attribution License (CC BY). The use, distribution or reproduction in other forums is permitted, provided the original author(s) and the copyright owner(s) are credited and that the original publication in this journal is cited, in accordance with accepted academic practice. No use, distribution or reproduction is permitted which does not comply with these terms.
*Correspondence: Liliana Neto Duarte, bGlsaWFuYW5kQGdtYWlsLmNvbQ==