- 1Department of Science and Technology, Institute for Biotechnology, IMC University of Applied Sciences, Krems, Austria
- 2Department of Science and Technology, Institute for Applied Chemistry, IMC University of Applied Sciences, Krems, Austria
- 3Research Division Biochemical Engineering, Research Group Integrated Bioprocess Development, Institute of Chemical, Environmental and Bioscience Engineering, Vienna University of Technology, Vienna, Austria
Modern society is heavily dependent on critical raw materials, such as rare earth elements (REEs), for use in electronic devices. The increasing demand for these materials has led to the need for environmentally friendly methods of processing non-recycled materials from e-waste and wastewater, as well as waste streams from cleaning and manufacturing facilities. Modern society’s dependence on such materials is growing by the day, and with it, the need for environmentally friendly processing of non-recycled materials from e-waste and wastewater in the form of “end-of-life” products, as well as waste streams from cleaning and manufacturing facilities, also increases. As these are problematic indications for modern isolation methods in the industry, these sources may be more suitable for new techniques as they have low concentration and high throughput for bioaccumulation. Chemical methods using nanomaterials are already being tested for their possibilities but still depend on acids and harsh chemicals. Microorganisms, on the other hand, can adsorb/absorb REEs in a more ecological way. Previous studies could already show that it is possible to accumulate REEs in the precipitates of bacterial cultures spiked with REEs to a value of over 50%. However, the question arose whether rare earths were spun into the pellets by centrifugation, adsorbed, or really incorporated in the cells. Therefore, we established a new easy-to-use experimental design in which the microorganisms were spiked with an REE standard and washed to minimize the falsification of measurements by peripheral binding of ions before being analyzed for REE contents by ICP-OES. The bioaccumulation of rare earths in microorganisms was monitored, yielding an uptake rate of up to 53.12% of the overall present ionic REE concentration. In this manuscript, we present the different concentration measurements that were taken during the process, before and after washing of the cells, to create a full picture of the localization, binding, incorporation, and occurrence of the ions of interest. The setup also showed a correlation between the introduction method of rare earths and the uptake of certain elements that might be correlated with the differentiation between light and heavy rare earth elements, while Y and Sc often seem to represent outliers.
1 Introduction
1.1 Bioaccumulation
Rare earth elements (REEs) belong to the lanthanide group (the sixth period of the periodic table of elements), which also includes scandium and yttrium (Giese, 2020). These elements share very similar chemical properties (Jowitt et al., 2018; Giese, 2020). With the exception of europium and cerium, they generally exist in a trivalent form and their ionic radius decreases with the increasing atomic number, which is also known as “lanthanide contraction” and may be a reason for the complexity of their separation and purification (Krishnamurthy and Gupta, 2015; Jowitt et al., 2018; Picone and Op den Camp, 2019).
In their 2015 publication, Gupta and Krishnamurthy updated an earlier report stating that the crustal abundance of REEs as transition metals, which is the representative of the proportional amount of the element in question found in the Earth’s crust, is even higher than that of carbon by approximately 20% (Krishnamurthy and Gupta, 2015). The comparative distribution usually follows the Oddo–Harkins rule, which still holds truth in modern times (Oddo, 1914; Harkins, 1917; Herrmann et al., 2016). REEs are not particularly rare but have long been poorly separated because of their similar chemical properties (Charalampides et al., 2015). It is elaborated that the larger ionic radii that come with the lower atomic numbers of the light REEs render them less compatible and allow for higher concentrations, which in turn, have an indirect proportionality to the abundance of the elements itself (Krishnamurthy and Gupta, 2015). The complexity of ore extraction, the problematic ways of isolation, and the low concentration in environmental sources still seem to render the term “rare” true (Hampel and Kolodney, 1961; Krishnamurthy and Gupta, 2015; Jowitt et al., 2018), which also might be the reason why they are mostly mined and refined in China (Charalampides and Vatalis, 2015).
Rare earths are often divided into LREEs (light rare earth elements), from lanthanum to europium or gadolinium, and HREEs (heavy rare earth elements), from gadolinium or terbium to lutetium and Yttrium (Jackson and Christiansen, 1993; Picone and Op den Camp, 2019; Giese, 2020). Some sources even introduce MREEs that include samarium, europium, and gadolinium (Sakamoto et al., 2008; Chen, 2011; Hatch, 2012; Suli et al., 2017).
Although considered inert to biological life, several studies have now found that REEs affect the enzymatic activity of certain proteins and can form active sites of enzymes like some alcohol dehydrogenases or be able to upregulate “normal” enzyme activities like with lanthanide-dependent methane dehydrogenases in methano- and methylotrophs (Huang et al., 2019; Picone and Op den Camp, 2019). It has also been demonstrated that the growth rate of microorganisms and the enzymatic activity of proteins are directly influenced by the lanthanides present with a preference for LREEs (Lumpe et al., 2018; Picone and Op den Camp, 2019). The uptake of lanthanides is also altered by several different biotic and abiotic factors and seems to be highly dependent on the proportion of species (Herrmann et al., 2016).
Kegl et al. suggest that nanomaterials, small materials with unique properties, could be useful for removing rare earths from wastewater. The authors discuss different types of nanomaterials that have been used for rare earth element removal, including carbon-based materials or metal oxides, acknowledging the need for acidic solvents for ionic content desorption and nanomaterial reuse and the influences of several factors that correlate with adsorption, such as pH, temperature, and contaminants in wastewater (Giese, 2020; Kegl et al., 2020).
These types of discoveries could shed new light on the possibilities of the biological uptake of lanthanides and on the separation of elemental metals. Following the results of Lumpe et al., 2018, the enzyme activity of REEs and the correlating lanthanide contraction, especially with LREEs, might be a viable strategy for the purification of single elements during fractionation of cell materials (Lumpe et al., 2018).
1.2 Environmental toll
According to the publication of Packey and Kingsnorth from 2016, there is significant financial and regulatory benefit for illegal mining. The environmental impact can be immense and can be even backed by local authorities, for the benefit of tax increases and employment in China (Packey and Kingsnorth, 2016) , responsible for more than 90% of global rare earth supply, and most legal mining is faced with trouble in uncovering new REE deposits (Binnemans et al., 2013). Adding to this, the Chinese REE market includes approximately 40% of the uncontrolled mining in the country, triggering significant unmonitored adverse effects in terms of environmental pollution, only overshadowed by increasing difficulties for the mining process itself, due to systematic depletion of most profitable ores (Mudd, 2010a; Mudd, 2010b; Norgate and Haque, 2010; Banks, 2013; Weng et al., 2016; Balaram, 2019).
Recycling is a widely used term for the active reuse, collection, or treatment of certain materials and products that may seem to be worthless, before they can be made useful again and, therefore, may have been discarded (Krishnamurthy and Gupta, 2015). For industrial purposes, recycling must have energy and cost-saving benefits to be included in a process, and for it to be beneficial to society, it must also have a positive impact on the environment. Several publications suggest different ways of rare earth element uptake, such as biosorption, as selective sequestration from the aquatic solution via non-metabolically mediated pathways or hypernymically termed bioaccumulation in a biological system (Tsezos, 2014; Krishnamurthy and Gupta, 2015; Giese, 2020). It can also be defined as the removal of substances from the solution by biological materials (Gadd, 2009; Fomina and Gadd, 2014; Breuker et al., 2020).
A well-documented circular economy system is the reuse of raw materials from spent lithium-ion batteries. They are industrially recycled, and even the wastewater from the production is treated to maximize material yields and financial benefits, which in turn, could be a formidable example of rare earth element treatment (Huang et al., 2018).
1.3 Importance in modern society
In a time when electric cars and smart devices are overwhelmingly present, the supply of rare earth elements is crucially relied on in the background (Balomenos et al., 2017; Jowitt et al., 2018). From smaller enterprises to big tech companies, everyone depends on the flow of these precious metals (Xavier et al., 2019), while few are the people knowing their importance. Scarce resources getting progressively more difficult to obtain due to an increase in demand, in contrast to the difficulties of ore exploitation and harsh conditions needed for metal extraction, draw an alarming picture (Binnemans et al., 2013; Jowitt et al., 2018). Ayman Elshkaki’s report summarizes the dependence on rare earths and focuses on possible future overproduction of rare earths in hopes of meeting the ever-increasing demand and the associated energy and water consumption and increased CO2 emissions (Elshkaki, 2021). More ecological models, such as the application of circular economy, for the reuse of rare earths in production lines seem to be a widely accepted option for improvement (Favot and Massarutto, 2019; Velázquez et al., 2019; Xavier et al., 2019).
Independently, the return in the form of recycled REEs observed is incredibly low. The regeneration of lanthanides from electronic supplies (i.e. microchips) is difficult, due to the low concentrations used, while highly concentrated products (i.e. neodymium magnets) are used for an extended period of time before again being included into the recycling procedure. However, end-of-life products increasingly gain importance as a sole or main means of source for raw materials (Binnemans et al., 2013; Blanchette and Lund, 2016; Li et al., 2017; Jowitt et al., 2018; Kumari et al., 2018).
Already, there were plenty of research efforts in recycling of rare earth elements; still, up until 2011, less than 1% of REEs were reintroduced into the production cycle. As already mentioned, this might be mainly due to the diminishingly low cost-to-benefit ratio and the lack of incentives (Binnemans et al., 2013; Krishnamurthy and Gupta, 2015). REEs are critical components in the production of technology and are rising in importance, due to the use in “green technology,” and further technological advances will keep pushing the demand in REEs and even exceed the current supply within the next few years, unrelated to the export restrictions from China. The only feasible solution from this point on would be the substitution of rare earth metals through the efficient recycling of REE-containing materials (Binnemans et al., 2013; Krishnamurthy and Gupta, 2015; Kumari et al., 2018).
2 Materials and methods
2.1 Laboratory work
Escherichia coli K12 was selected as the model organism for the uptake tests from a range of environmental strains as it is available to our research facility and most closely resembles the wild type. This was due to concerns about genetically modified organisms and the desire to implement a relatively common and easy-to-culture microorganism. LB broth (Roth, LB Broth (Lennox), granulated, Art. No:6669.3) was prepared to suffice for the whole experiment and adjusted to pH 6.7–6.8.
For the initial bolus testing setup, the Sy-Lab BacTrac4300® system, a multi-fermenter unit measuring impedance in up to 64 vials in a short sequence, was used as a measurement and a cultivation device. The temperature setting was adjusted to 30°C, while impedance measurements were taken every 10 min throughout 24 h. The vials were filled to 10 mL and adjusted to a starting OD600 of 0.1.
The microbes were prepared in an overnight culture in LB medium before inoculating into 100 mL of the medium in 250-mL baffled screw-top shaker flasks at a starting OD600 of 0.01 (Eppendorf BioSpectrometer kinetic, firmware-ver: 4.3.6.0). For the initial bolus testing experiment, OD600 was adjusted to 0.1. Samples were incubated at 30°C at 120 rpm on a horizontal shaking incubator (New Brunswick Scientific, INNOVA 42). The samples were treated with the rare earth element mix for ICP (Merck, 67349-100ML, TraceCERT®) to a final concentration of 2 ppm for the bolus testing experiment and 2.25 ppm for all the following experiments, respectively. The flasks were taken out for sterile sampling at previously defined timepoints (see respective setup descriptions) at which each sample’s OD600 and pH were measured and noted. A volume of 10 mL of each sample was spun down at 2500 g for 45 min at 4°C (Beckman Coulter, Allegra® X-12R Centrifuge) to split the supernatant from the pellet and stored accordingly. At the end of experiments, the bacteria-positive samples were taken and used for washing steps. A measure of 10 mL of the final volumes was filtered through a nitrocellulose filter (Sartorius, cellulose nitrate filter, pore size: .45 µm, order no: 11406–50----ACN) and washed twice with the same LB medium stock used previously, before being resuspended into another 10-mL medium (each step is stored in a fresh test tube for further analysis). Afterward, another 30–40 mL of the final volume was transferred into 50-mL falcon tubes to be spun down at 2500 g for 30 min at 4°C. The tubes were decanted, and each of the pellets were resuspended into 10-mL LB medium and spun down at the same settings. This washing procedure is then repeated for the second time, and the samples were stored at 4°C, before further treatment. Each flask, therefore, resulted in four filtration and four centrifugation samples in tubes of respective sizes.
For analytical measurement, all samples were treated with concentrated HNO3 (Honeywell Fluka, nitric acid, ACS Reagent, Reag. ISO, ≥69%, Cat. 30702-2.5 L) and incubated at 60°C o/n while arranging for the final concentration to be 2%. Each sample was sonicated (Bandelin, Sonorex RK 100 H, 35 kHz) for 30 min and filled up to a previously decided amount. For analysis, ICP-OES (Agilent 5110 ICP-OES with an attached Agilent Technologies SPS 4 Autosampler) was used for the quantitative and qualitative measurement. All test tubes were labeled, according to their description (see Table 1) and their respective time of sampling.
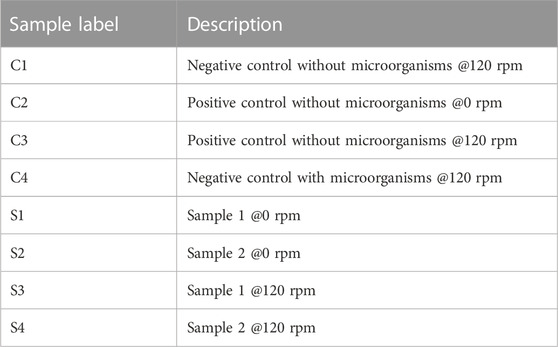
TABLE 1. Sample labels with corresponding descriptions (if not stated differently in the graphs and figures).
The analytical measurement was set up by the proprietary Agilent software application (Agilent Technologies ICP Expert, Version 7.5.4.11997). Parameters for samples were put to the axial measurement in triplicates, after 15 s stabilization time (for more precise information, see Table 2). The rare earth element mix for ICP (Merck, 67349-100ML, TraceCERT®), used to treat the samples, was also used as a standard for ICP measurement at concentrations of 0 (2% HNO3 as blank), 0.1, 0.5, 1, 2.5, and 5 ppm. Elements of interest were measured at four wavelengths each, as well as two argon and one carbon wavelength as internal standards (see Table 3).
For the purpose of facilitating explanations, this manuscript will be holding up the definition of light and heavy REEs (LREEs and HREEs), as stated by Picone and Op den Camp, 2019 and Giese, 2020. Therefore, LREEs are defined as La, Ce, Pr, Nd, Sm, Eu, and Gd, while HREEs will be defined as Tb, Dy, Ho, Er, Tm, Yb, and Lu. For evaluation, we used heatmaps for visual depiction of the full set of measured lanthanides (see Supplementary Data) while extracting six single representative measurements, namely, La, Nd, and Eu for LREEs, Dy and Yb for HREEs, and Y for the non-lanthanides of the rare earth elements.
To minimize possible interferences in the analytical measurements, a special water dispenser system (Sartorius Arium® Pro coupled with the Sartorius Arium Smart Station ultrapure water dispenser) was used to provide ultrapure ddH2O for all solutions.
The experiments were conducted without any environmental adjustment or genetic alteration to the microorganisms to ensure ecological tolerance, as a proof of concept for a possible application in waste processing.
2.2 Data analysis
Result analysis was performed using Microsoft Excel 2019 for calculation and simplification and GraphPad Prism 9 for the creation of homogenous and easily legible depictions.
The dataset created by the Sy-Lab BacTrac4300® system was composed of measurements of quadruplicates. The device readings were transferred to Excel, and an average [Excel function AVERAGE ()] and standard deviation were calculated based on a sample [Excel function StDev.S ()] taken. Due to the amount of measuring points, error bars depicting the standard deviation were deemed unsuitable.
The datasets created by ICP-OES were composed of measurements of triplicates at four wavelengths for each element, respectively, resulting in 12 values for each measured sample and element. The wavelengths in question were chosen in order to minimize interference and optimize the signal-to-noise ratio. All of these values were background normalized by the Agilent Technologies ICP Expert program before an average and a standard deviation based on a sample were applied in Excel. The measurement values, which were not relevant for depiction and solely served as an in-experiment control measure, were omitted in order to simplify the reading of resulting graphs. Some elements of choice were then selected as representatives for all the lanthanides measured as a means to further simplify the prohibitively large datasets. The calculated and simplified datasets were then transferred into GraphPad files and plotted, and the colors were adjusted to enhance the contrast. The standard deviation was given as positive and negative error bars for each measurement. Further statistical analysis of the datasets was not considered necessary for understanding the relationships of individual experimental measurements and was, therefore, removed.
3 Results
3.1 Initial bolus testing
As a possibility to evaluate the overall uptake of microorganisms, a screening experiment was set up, representatively shown in Figure 1. Figure 1A depicts the growth curves measured as changes in relative impedance every 10 min throughout 24 h of range. Each curve has a short-lived growth inhibition, shown by a slight dip in µ at the respective time of bolus introduction. Testing for the relative uptake, in comparison with the total retrieval, shows a benefit for the 3-h bolus incubation in contrast to 6 and 9 h (followed by 21, 18, and 15 h incubation time, see Figure 1B).
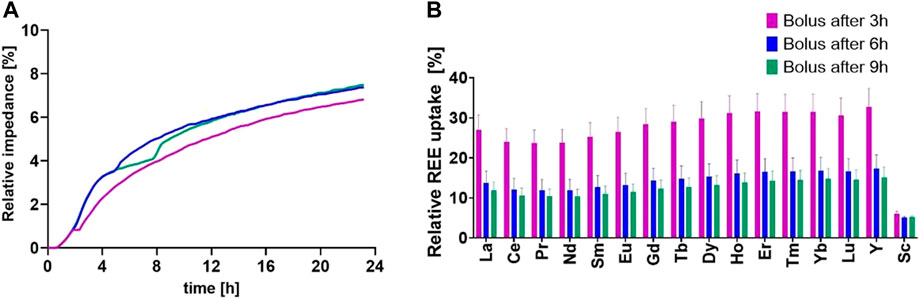
FIGURE 1. (A) Depiction of the growth of E. coli K12 shows change in relative impedance over the course of the bolus testing run. (B) Relative uptake of REEs in biomass in correlation with the total retrieval given for three different feeding timepoints after inoculation (100% = 2.25 ppm).
3.2 Bolus after 3 h
Following the assumption that the increased contact time might enable better uptake, the next experimental arrays were set up accordingly, adjusting OD600 to 0.01 to be able to observe the proceedings for approximately 3.5 additional generation times while introducing a shaking incubator for better oxygen distribution and enhanced growth. The remainder of parameters was left unaltered, although growth seemed to be further inhibited when comparing OD600 measurements over the course of the incubation, as can be seen in Figure 2. The most probable cause for this alteration is shown in the approximated pH values plotted, measured using the pH indicator paper for this first fully monitored trial. The samples incubated at 0 rpm only exhibited a slight growth and pH adjustment difference compared to the samples at 120 rpm, but this might be due to the low final biomass generated. The uptake depiction for six selected elements in Figure 3 proved higher than the previously finalized runs, peaking at 43% uptake in contrast to 27% in Figure 1B, in relation to total retrieval for lanthanum, in the cultivation setting with 120 rpm during incubation (further also described as “shaken “versus 0 rpm or non-shaken).
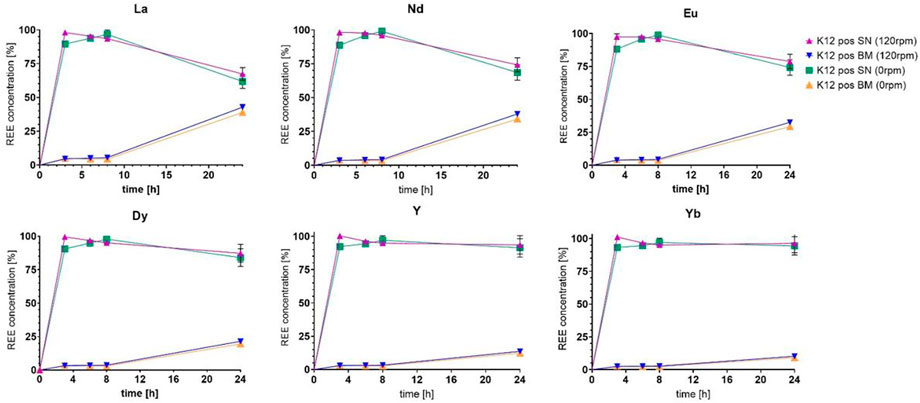
FIGURE 3. Comparison of the uptake of six chosen elements as the representation of the full set of lanthanides (concentrations given as a percentage of 2.25 ppm).
3.3 Bolus after 6 h
By delaying the bolus timing further back to 6 h after initial incubation, we could achieve significantly higher biomass proliferation, even comparable with the untreated control group. The adjustment abilities concerning the pH increased as well, especially for the shaken samples, as can be seen in Figure 4. As can be seen in Figure 5, we could further observe an improved uptake of lanthanides throughout the ranks, especially with lanthanum, where we surpassed 50% uptake somewhere between the 9 h and the 24 h sampling points, which also seemed to be the stagnation level, before dropping after the 27 h mark. The peak uptake was expressed in the case of La at 53.1% at 120 rpm and 34.7% at 0 rpm. If we observe Figure 4, we could argue that this could have to do with growth stagnation due to nutrient or oxygen depletion at an OD600 of 6.2 in a baffled shaker flask. The experimental setup was, therefore, further adjusted to a run time of 24 h.
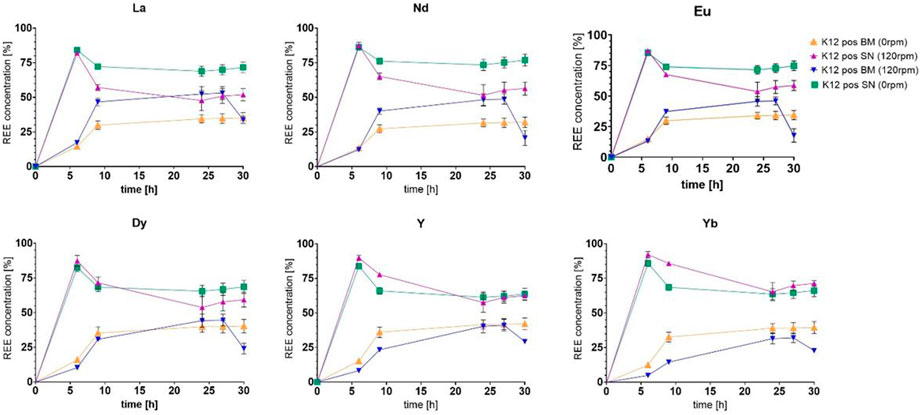
FIGURE 5. Comparison of the uptake of six chosen elements as the representation of the full set of lanthanides (concentrations given as a percentage of 2.25 ppm).
3.4 Comparison of cell origins
To minimize deviations in growth and uptake, we introduced a set of experiments in which the differences in the origins of o/n cultures could be elucidated. For one set, the o/n cultures were originated from streak plates (Figure 6A), while the other set was taken from cryo cell solutions (Figure 6B). The starting cell density was kept at a planned OD600 of 0.01, as all were residual parameters.
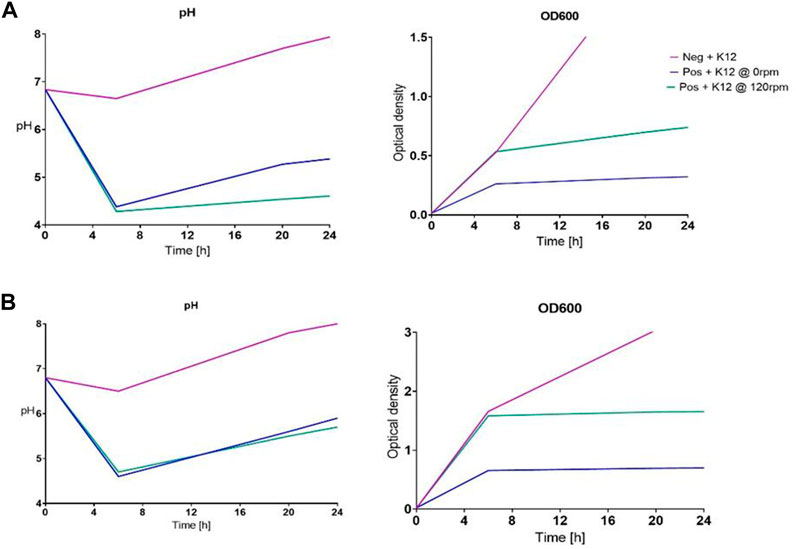
FIGURE 6. Depiction of pH and OD600 propagation over the course of the two experimental runs, comparing streak plate-originated o/n cultures against cryo-originated organisms: (A) taken from the streak plate and (B) taken from the cryo cell bank.
Even with such a seemingly minor change, we could observe an influence on the overall growth and proliferation time, which might then again be caused by an offset in generation cycles. On the other hand, the microorganisms did not seem to adjust the pH as efficiently as shown in previous trials (e.g. Figure 4), whichever sample was compared to the untreated control group. As expected, we could also determine growth differences between the trials at 120 rpm and 0 rpm. Surprisingly yet, the trials presented differences in the uptake, while the streak plate-originated cells only showed marginal differences in the uptake between 120 rpm and the 0 rpm subgroups, namely, 49.10% shaken and 37.14% non-shaken, for the exemplary lanthanum concentration (Figure 7A). At the same time, the discrepancies were enhanced with the elevated final cell densities in Figure 6B, such as 50.49% shaken and 18.48% non-shaken, as shown in Figure 8A. These differences in the uptake seem to decline with the increasing atomic number, which could lead to the conclusion that even though lanthanide contraction might be an influence, the heavier REEs still pose a more challenging adsorption and absorption target.
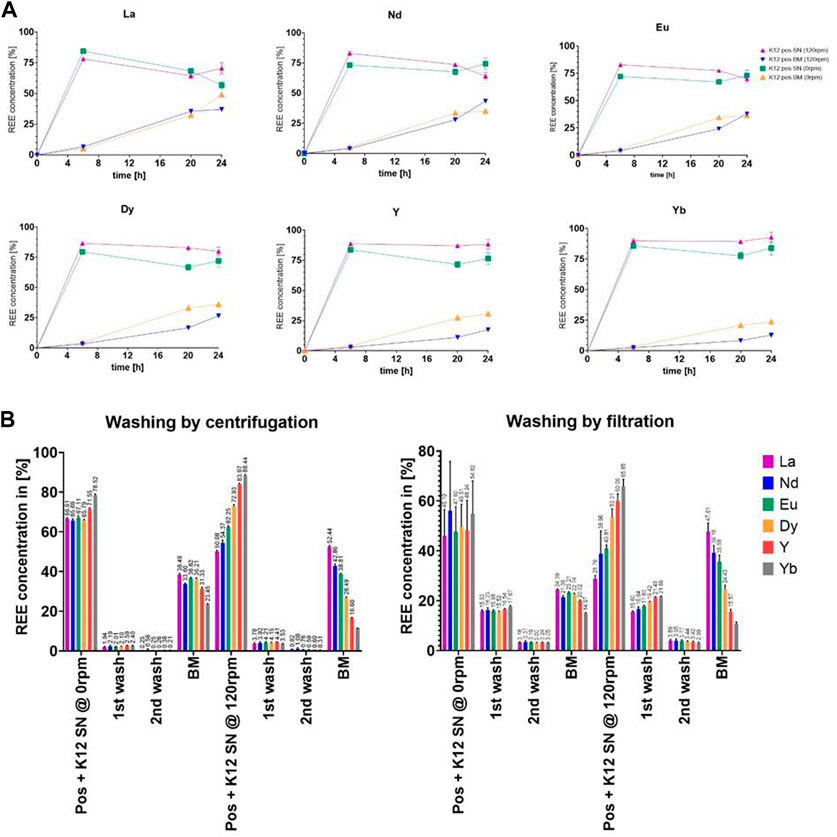
FIGURE 7. (A) Comparison of the uptake of six chosen elements as the representation of the full set of lanthanides (Y-axis: percentage of the total concentration [%]; X-axis: time [h]). (B) Analysis measurements of the uptake of six chosen elements after each washing step in the percentage of total retrieval. All measurements are given as percentages of the calculated final concentration of 2.25 ppm.
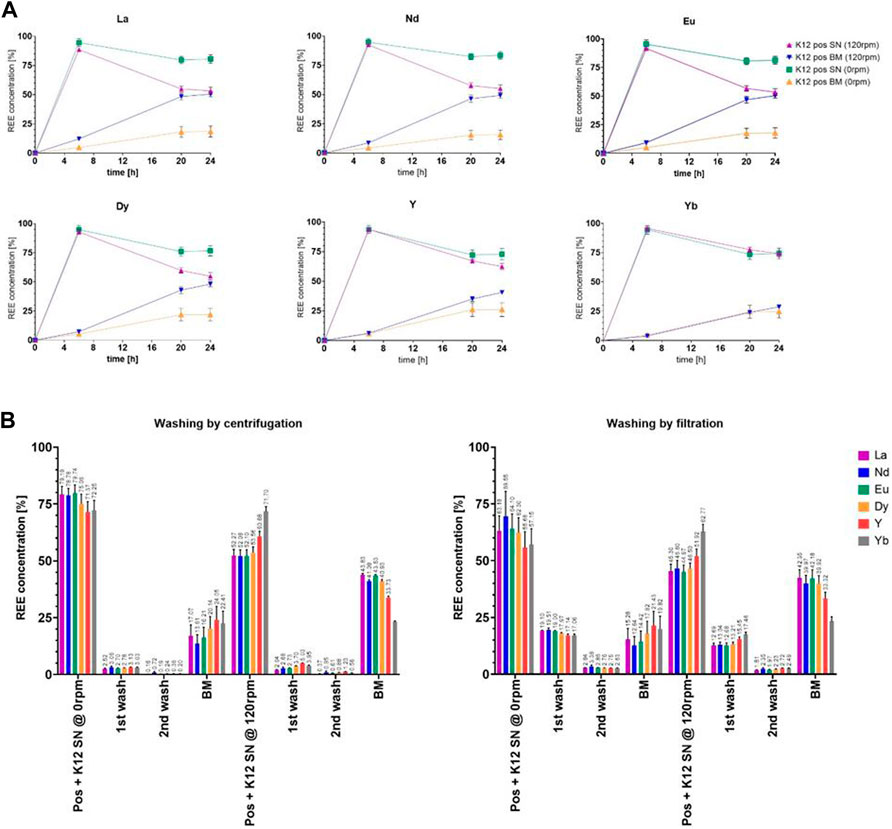
FIGURE 8. (A) Comparison of the uptake of six chosen elements as the representation of the full set of lanthanides (Y-axis: percentage of the total concentration [%]; X-axis: time [h]). (B) Analysis measurements of the uptake of six chosen elements after each washing step in the percentage of total retrieval. All measurements are given as percentages of the calculated final concentration of 2.25 ppm.
3.5 Localization of rare earth elements
In order to determine the REE whereabouts, we decided to introduce washing steps for samples taken after 24 h; see Figure 7B and Figure 8B. The results were compared between the 120 rpm and 0 rpm subgroups, as well as overall to each other (streak plate and cryo-originated cells). The better cut-off could be witnessed with separation by centrifugation, which led to the upkeeping of the original experimental set up. The uptake values measured in the biomass samples were comparable, while the concentrations in the supernatant differed greatly. The filter wash could not achieve the same cut-off as the washing by centrifugation, which fortified the plausibility of centrifugal separation in our experimental setup. The uptake values for the biomass show absorbed concentrations that are overall higher in shaken cultures but seem to differ in proportional distribution in the measured specimen. In contrast to this, the non-shaken cultures show a nearly homogenous distribution of all REEs.
Heatmaps depicting the uptake of each element at the sampled timepoints referenced with a color scale given in % of the total retrieval during each experiment can be found in the Supplementary Data provided with this manuscript.
4 Discussion
Filling the ranks of theories, we observed colloidal precipitate formation in smaller cultures. These phosphates seem to be insoluble in combination with trivalent lanthanides which could be confirmed with their respective solubility products, found in the literature. Most publications taken into consideration seem to be unaware of this fact, or simply omit it, due to possibly negligible importance. These precipitates, however, were not present in larger volumes, which led to believe that either the concentrations for molecule formation were too low or the microorganisms adjusted to their environment, by shifting the equilibrium of concentrations toward the ionic form since pH was being monitored, to ensure the preservation of ionic REE substrates for the uptake.
The comparison of heavy and light REEs shows that slightly different parameters might yield an easy-to-use capacity to trigger the pre-concentration of some or pre-thinning of other elemental rare earth elements before further processing. A series of such passages might even yield the wanted filtration effect with a certain amount of differentiation enabling close to pure elemental fractions for reintroduction into the production chain.
The concentrations used (around 2 ppm) are comparable to Jalali and Lebeau (2021), which in turn, led to some questions to further elucidate if the found differences in single elements uptake might prove advantageous for separation and isolation of pure compounds. The hypotheses proven in the preliminary results used in this manuscript have yet a long way to go to be applied in a useful setting, but we are looking forward to the upcoming challenges.
Rethinking might be due in terms of environmental toll. Mining loci, as well as the miners, who do not fall in line with guidelines of the legislative bodies and might not even be registered. Considering the environmental damage that even legal mining has, it is safe to say that change is of utmost importance. Our research is targeted toward tackling this. It might be the waste stream, a production facility or even a device that was thrown away, deemed to be irreparable or too costly to isolate single components or raw material. Recycling has become imminent to the well-being of our modern civilization and ongoing technological success.
Data availability statement
The original contributions presented in the study are included in the article/Supplementary Material; further inquiries can be directed to the corresponding author.
Author contributions
DS devised the project and the main conceptual outline. DR and WR were involved in the planning and execution of experiments, the processing of data, and performing the analysis. WR drafted and finalized the manuscript. All authors discussed the results, commented on the manuscript, and aided in interpreting results.
Funding
The writing of this manuscript benefitted from the financial support of the European Interreg Fund, V-A ATCZ, project number172. The compiling and writing were performed at the University of Applied Sciences Krems, Austria.
Acknowledgments
The authors thank Barbara Entler for providing the needed filtration apparatus and the filters for sample treatment.
Conflict of interest
The authors declare that the research was conducted in the absence of any commercial or financial relationships that could be construed as a potential conflict of interest.
Publisher’s note
All claims expressed in this article are solely those of the authors and do not necessarily represent those of their affiliated organizations, or those of the publisher, the editors, and the reviewers. Any product that may be evaluated in this article, or claim that may be made by its manufacturer, is not guaranteed or endorsed by the publisher.
Supplementary Material
The Supplementary Material for this article can be found online at: https://www.frontiersin.org/articles/10.3389/fenvs.2023.1112612/full#supplementary-material
References
Balaram, V. (2019). Rare earth elements: A review of applications, occurrence, exploration, analysis, recycling, and environmental impact. Geosci. Front. 10 (4), 1285–1303. doi:10.1016/j.gsf.2018.12.005
Balomenos, E., Davris, P., Deady, E., Yang, J., Panias, D., Friedrich, B., et al. (2017). The EURARE project: Development of a sustainable exploitation scheme for Europe’s rare earth ore deposits. Johns. Matthey Technol. Rev. 61, 142–153. doi:10.1595/205651317X695172
Banks, G. (2013). Little by little, inch by inch: Project expansion assessments in the Papua New Guinea mining industry. Resour. Policy 38 (4), 688–695. doi:10.1016/J.RESOURPOL.2013.03.003
Binnemans, K., Jones, P. T., Blanpain, B., Van Gerven, T., Yang, Y., Walton, A., et al. (2013). Recycling of rare earths: A critical review. J. Clean. Prod. 51, 1–22. doi:10.1016/J.JCLEPRO.2012.12.037
Blanchette, M. L., and Lund, M. A. (2016). Pit lakes are a global legacy of mining: An integrated approach to achieving sustainable ecosystems and value for communities. Curr. Opin. Environ. Sustain. 23, 28–34. doi:10.1016/j.cosust.2016.11.012
Breuker, A., Ritter, S. F., and Schippers, A. (2020). Biosorption of rare earth elements by different microorganisms in acidic solutions. Metals 10, 954. doi:10.3390/met10070954
Charalampides, G., Vatalis, K. I., Apostoplos, B., and Ploutarch-Nikolas, B. (2015). Rare earth elements: Industrial applications and economic dependency of europe. Procedia Econ. Finance 24, 126–135. doi:10.1016/s2212-5671(15)00630-9
Charalampides, G., and Vatalis, K. I. (2015). Global production estimation of rare earth elements and their environmental impacts on soils. J. Geoscience Environ. Prot. 03, 66–73. doi:10.4236/gep.2015.38007
Chen, Z. (2011). Global rare earth resources and scenarios of future rare earth industry. J. Rare Earths 29, 1–6. doi:10.1016/S1002-0721(10)60401-2
Elshkaki, A. (2021). Sustainability of emerging energy and transportation technologies is impacted by the coexistence of minerals in nature. Commun. Earth Environ. 2 (1), 1–13. doi:10.1038/s43247-021-00262-z
Favot, M., and Massarutto, A. (2019). Rare-Earth elements in the circular economy: The case of yttrium. J. Environ. Manag. 240, 504–510. doi:10.1016/J.JENVMAN.2019.04.002
Fomina, M., and Gadd, G. M. (2014). Biosorption: Current perspectives on concept, definition and application. Bioresour. Technol. 160, 3–14. doi:10.1016/j.biortech.2013.12.102
Gadd, G. M. (2009). Biosorption: Critical review of scientific rationale, environmental importance and significance for pollution treatment. J. Chem. Technol. Biotechnol. 84, 13–28. doi:10.1002/jctb.1999
Giese, E. C. (2020). Biosorption as green technology for the recovery and separation of rare earth elements. World J. Microbiol. Biotechnol. 36, 52. doi:10.1007/s11274-020-02821-6
Hampel, C. A., and Kolodney, M. (1961). Rare metals handbook, 2nd ed. J. Electrochem. Soc. 108, 248C. doi:10.1149/1.2427960
Harkins, W. D. (1917). The evolution of the elements and the stability of complex atoms. I. A new periodic system which shows a relation between the abundance of the elements and the structure of the nuclei of atoms. J. Am. Chem. Soc. 39, 856–879. doi:10.1021/ja02250a002
Hatch, G. P. (2012). Dynamics in the global market for rare earths. Elements 8, 341–346. doi:10.2113/gselements.8.5.341
Herrmann, H., Nolde, J., Berger, S., and Heise, S. (2016). Aquatic ecotoxicity of lanthanum - a review and an attempt to derive water and sediment quality criteria. Ecotoxicol. Environ. Saf. 124 (5), 213–238. doi:10.1016/j.ecoenv.2015.09.033
Huang, B., Pan, Z., Su, X., and An, L. (2018). Recycling of lithium-ion batteries: Recent advances and perspectives. J. Power Sources 399, 274–286. doi:10.1016/J.JPOWSOUR.2018.07.116
Huang, J., Yu, Z., Groom, J., Cheng, J. F., Tarver, A., Yoshikuni, Y., et al. (2019). Rare earth element alcohol dehydrogenases widely occur among globally distributed, numerically abundant and environmentally important microbes. ISME J. 13, 2005–2017. doi:10.1038/s41396-019-0414-z
Jackson, W. D., and Christiansen, G. (1993). International strategic minerals inventory summary report; rare-earth oxides. Circular. doi:10.3133/CIR930N
Jalali, J., and Lebeau, T. (2021). The role of microorganisms in mobilization and phytoextraction of rare earth elements: A review. Front. Environ. Sci. 9, 688430. doi:10.3389/fenvs.2021.688430
Jowitt, S. M., Werner, T. T., Weng, Z., and Mudd, G. M. (2018). Recycling of the rare earth elements. Curr. Opin. Green Sustain. Chem. 13, 1–7. doi:10.1016/j.cogsc.2018.02.008
Kegl, T., Kosak, A., Lobnik, A., Novak, Z., Kralj, A. K., and Ban, I. (2020). Adsorption of rare earth metals from wastewater by nanomaterials: A review. J. Hazard. Mater. 386, 121632. doi:10.1016/J.JHAZMAT.2019.121632
Krishnamurthy, N., and Gupta, C. K. (2015). Extractive metallurgy of rare earths. Second Edition. Washington, D.C: CRC Press. doi:10.1201/b19055
Kumari, A., Jha, M. K., and Pathak, D. D. (2018). “Review on the processes for the recovery of rare earth metals (REMs) from secondary resources,” in Minerals, metals and materials series (New York City: Springer International Publishing), 53–65. doi:10.1007/978-3-319-72350-1_5
Li, J., He, X., and Zeng, X. (2017). Designing and examining e-waste recycling process: Methodology and case studies. Environ. Technol. (United Kingdom) 38 (6), 652–660. doi:10.1080/09593330.2016.1207711
Lumpe, H., Pol, A., Op den Camp, H. J. M., and Daumann, L. J. (2018). Impact of the lanthanide contraction on the activity of a lanthanide-dependent methanol dehydrogenase-a kinetic and DFT study. Dalton Trans. 47, 10463–10472. doi:10.1039/c8dt01238e
Mudd, G. M. (2010a). Global trends and environmental issues in nickel mining: Sulfides versus laterites. Ore Geol. Rev. 38 (1–2), 9–26. doi:10.1016/J.OREGEOREV.2010.05.003
Mudd, G. M. (2010b). The environmental sustainability of mining in Australia: Key mega-trends and looming constraints. Resour. Policy 35 (2), 98–115. doi:10.1016/J.RESOURPOL.2009.12.001
Norgate, T., and Haque, N. (2010). Energy and greenhouse gas impacts of mining and mineral processing operations. J. Clean. Prod. 18 (3), 266–274. doi:10.1016/J.JCLEPRO.2009.09.020
Oddo, G. (1914). Die Molekularstruktur der radioaktiven Atome. Z. für Anorg. Chem. 87, 253–268. doi:10.1002/zaac.19140870118
Packey, D. J., and Kingsnorth, D. (2016). The impact of unregulated ionic clay rare earth mining in China. Resour. Policy 48, 112–116. doi:10.1016/j.resourpol.2016.03.003
Picone, N., and Op den Camp, H. J. (2019). Role of rare earth elements in methanol oxidation. Curr. Opin. Chem. Biol. 49, 39–44. doi:10.1016/j.cbpa.2018.09.019
Sakamoto, N., Kano, N., and Imaizumi, H. (2008). Determination of rare earth elements, thorium and uranium in seaweed samples on the coast in Niigata Prefecture by inductively coupled plasma mass spectrometry. Appl. Geochem. 23 (10), 2955–2960. doi:10.1016/j.apgeochem.2008.04.011
Suli, L. M., Ibrahim, W. H. W., Aziz, B. A., Deraman, M. R., and Ismail, N. A. (2017). A review of rare earth mineral processing technology. Chem. Eng. Res. Bull. 19, 20. doi:10.3329/cerb.v19i0.33773
Tsezos, M. (2014). Biosorption: A mechanistic approach. Adv. Biochem. Engineering/Biotechnology 141, 173–209. doi:10.1007/10_2013_250
Velázquez, O., Valio, A., Santasalo-Aarnio, A., Reuter, M. A., and Serna, R. (2019). A critical review of lithium-ion battery recycling processes from a circular economy perspective. Batteries 5 (4), 68. doi:10.3390/BATTERIES5040068
Weng, Z., Haque, N., Mudd, G. M., and Jowitt, S. M. (2016). Assessing the energy requirements and global warming potential of the production of rare earth elements. J. Clean. Prod. 139, 1282–1297. doi:10.1016/J.JCLEPRO.2016.08.132
Keywords: REEs (rare earth elements), WEEE (waste electrical and electronic equipment), EOL product recycling, ICP-OES, bioaccumulation, Bioab- and absorption
Citation: Rassy WY, Ripper D, Pomare E, Winkler S, Koppensteiner A, Spadiut O and Schild D (2023) Incorporation of ionic rare earth elements as a form of microbial environmental remediation. Front. Environ. Sci. 11:1112612. doi: 10.3389/fenvs.2023.1112612
Received: 30 November 2022; Accepted: 03 January 2023;
Published: 20 January 2023.
Edited by:
Sudhir Kumar Pandey, Guru Ghasidas Vishwavidyalaya, IndiaReviewed by:
Chunqiao Xiao, Wuhan Institute of Technology, ChinaStefan Fränzle, Technical University Dresden, Germany
Copyright © 2023 Rassy, Ripper, Pomare, Winkler, Koppensteiner, Spadiut and Schild. This is an open-access article distributed under the terms of the Creative Commons Attribution License (CC BY). The use, distribution or reproduction in other forums is permitted, provided the original author(s) and the copyright owner(s) are credited and that the original publication in this journal is cited, in accordance with accepted academic practice. No use, distribution or reproduction is permitted which does not comply with these terms.
*Correspondence: Dominik Schild, dominik.schild@fh-krems.ac.at