- 1State Key Laboratory Managing Biotic and Chemical Threats to the Quality and Safety of Agro-products, Institute of Agr-product Safety and Nutrition, Zhejiang Academy of Agricultural Sciences, Hangzhou, China
- 2School of Agriculture, Ningxia University, Ningxia, China
- 3School of Agricultural Sciences, College of Agricultural, Life and Physical Sciences, Southern Illinois University, Carbondale, IL, United States
- 4Cultivated Land Quality Monitoring and Protection Center, Ministry of Agriculture and Rural Affairs, Beijing, China
Chemical nitrogen (N) fertilizer is generally applied at high rates to maximize crop yields and also many non-grain farmlands are being converted into grain production to ensure grain production safety in China now. To test the effects of chemical N on crop production and soil health, a 3-year trial with urea and controlled-release fertilizer was conducted in a maize (Zea mays L.)-wheat (Triticum aestivum L.) system shifted from vegetable farmland by evaluating the effects of N fertilization on crop yields, typical soil properties, and soil bacterial community using 16S rRNA gene sequencing. The maximum crop yield and aboveground N uptake efficiency was achieved at 150 kg N ha-1 for wheat and 200 kg N ha-1 for maize. After three cropping seasons, the soil N properties were notably altered. For each kg of N applied, soil pH decreased at a rate of 0.0004–0.0012 pH units. The soil total N (TN) and total carbon (TC) content reached a higher stability at certain cumulative N application rates. However, the C/N ratio linearly decreased as N rate increased. Consequently, N application significantly decreased soil bacterial diversity owing to the decrease of α-diversity indexes of Shannon and Pielou_e. At the phylum level, chemical N application significantly depleted members of Acidobacteria, Chloroflexi and Nitrospirae, but the growth of Actinobacteria, Gemmatimonadetes, Firmicutes and Patescibacteria were improved. Compared with urea, the controlled-release fertilizer did not significantly change crop yields, soil bacterial diversity, soil TN and TC content. Overall, our results indicated that chemical N promoted crop yields at optimum application rates, but excessive N application could result in a decline in soil health, specifically accelerated soil acidification, a decrease in soil bacterial diversity, and soil C and N imbalance.
1 Introduction
Large amounts of chemical N fertilizer have been applied to crops worldwide in order to improve crop production and maximize crop yield (Savci, 2012). Maize and wheat are the most important cereal crops, and a summer maize-winter wheat rotation is cultivated globally to meet food demands. To ensure high crop yields, chemical fertilizers are commonly used in a maize-wheat rotation system (Kong et al., 2014). In Minnesota, growers often fertilize maize crops with either urea or anhydrous ammonia at around 150 kg N ha-1 (Bierman et al., 2012). In Italy, ammonium nitrate is the most widely used source of N fertilizer in maize and wheat production systems and the application rates have been recorded as 170 kg N ha-1 for wheat and 230 kg N ha-1 for maize (Fantin et al., 2017). Kong et al. (2014) report that the average increase in yield between 1985 and 2009 ranged from 1,582 kg ha−1 to 5,860 kg ha−1 for wheat and 4,492 kg ha−1 to 5,610 kg ha−1 for maize in the Huang-Huai-Hai region of China and the average rates of fertilizer use were about 430 kg ha−1 yr−1 of N. The response of crop production to chemical N is different in different regions and at different N rates. There is no doubt that in the future, N chemical fertilizer will continue to be widely used to improve crop yields to feed the growing global population (Davison, 2009). Therefore, to improve chemical N management, it is imperative to identify correct N application rates for both maize and wheat, and to understand the effects of long-term chemical N application on soil health.
Soil pH is a good indicator of soil health and it is changed notably when chemical N fertilizer is applied. Previous research reported that ammonium-based chemical N fertilizer applications decrease soil pH, causing acidification of the soil, which limit crop growth and yields (Whitten et al., 2000; Caires et al., 2006; Kalkhoran et al., 2019). In a 5-year trial, Sadeghpour et al. (2017) found that cumulative urea ammonium nitrate (UAN) application to maize, especially at rates above the optimum N fertilizer requirement, decreased soil pH by 0.5 units. In a long-term wheat and maize rotation experiment, Hui et al. (2022) also found that soil pH was significantly decreased from 7.93 in a no fertilizer (CK) treatment to 6.53 with an annual application of 300 kg N ha-1. In Australia, it is estimated that about A$1,585 million per year is lost from agricultural production owing to soil acidity (Hajkowicz and Young, 2005). Soil acidification has also been found to cause soil nutrient imbalances, and alter soil microbial biomass activities (Hati et al., 2008; Kalkhoran et al., 2019). To date, the effects of chemical N rate on the decreasing strength of soil pH are still unknown.
Long-term chemical N application significantly influences many soil health indexes, including soil TN, soil organic matter (SOM), and soil microbial community (Wrage et al., 2001; Lucas et al., 2011). Literature is inconsistent regarding the effects of long-term chemical N fertilizer on soil TN and TC. For example, Hui et al. (2022) reported that soil TN concentration was 70% higher in 300 kg N ha-1y-1 compared with CK in a long-term wheat-maize rotation experiment, but a decreasing trend was found for soil organic carbon (SOC)as the N application increased (Hui et al., 2022). However, other studies report that N fertilization did not change soil TC, TN, and SOM content regardless of N fertilizer rates (Mack et al., 2004; Zeng et al., 2016). Similarly, reports in literature are conflicting regarding the effects of chemical N fertilizer on microbial communities. Several studies report that N fertilizer addition decreases soil microbial activities (Allison et al., 2007; Ramirez et al., 2012; Geisseler and Scow, 2014; Yu et al., 2019). In particular, ammonium-based N fertilizers are reported to decrease the abundance of bacteria and archaea in the soil (Wessén et al., 2010). In contrast, Li et al. (2022) report that N fertilization increased soil microbial biomass and altered microbial composition according to a 6-year experiment. These conflicting reports may have been caused by fertilizer type and site-specific investigations. To clearly understand the effect of chemical N fertilizer on soil health indexes, more research is needed.
Currently, many non-grain farmlands, such as vegetable fields and orchard fields, are being converted to grain production to ensure adequate grain supplies in China. There are only a few studies that report the responses of grain production and soil health indicators to N chemical fertilization following a change in land usage from non-grain farmland to grain crops. Therefore, we conducted a 3-year maize-wheat rotation experiment in a typical intensive vegetable cropping field with five different urea N rates and a controlled-release N fertilizer. The aims of this study were 1) to determine the correct N fertilizer rates for maize and wheat, 2) to assess the strength of soil acidification under chemical N application, and 3) to examine the soil health regulations under chemical N application in a grain production system that was previously vegetable farmland.
2 Materials and methods
2.1 Experimental site
The field experiment was conducted from July 2017 to May 2020 at the Yangdu Experiment station of Zhejiang Academy of Agriculture Science, Jiaxing city, Zhejiang, China (N:30°26′22″, E:120°24′40″). The experiment site has a subtropical monsoon climate with an annual average temperature of 15.9°C, an annual average rainfall of 1,168.8 mm, and an annual average sunshine of 2017.0 h in recent 5 years. Prior to conducting this trial, the site was used for intensive vegetable production for 5 years. The soil type was paddy and yellow earth with a clay texture, sand, silt, and clay contents of 8.13%, 24.13%, and 67.74%, respectively. Soil pH (1 soil:2.5 water) was 5.3. Soil organic matter, total phosphorus (TP), total potassium (TK), and TN concentrations were 15.7 g kg-1, 1.06 g kg-1, 21.8 g kg-1, and 1.13 g kg-1, respectively.
2.2 Experimental design and treatments
The experiment was laid out with six treatments and there were 5 N rates for urea and one rate for controlled-release fertilizer with three replicates. In total, there were 18 plots in a randomized complete block design. For urea applications, the N rates of the maize/wheat rotation were 0/0,50/50,150/100,200/150, and 300/200 kg N ha-1 and were named as CK, U100, U250,U350, and U500, respectively. Another treatment, named C250, involved controlled-release fertilizer with 150 kg N ha-1 during the maize season and 100 kg N ha-1 in the wheat season. Each plot was 6 m in length and 3 m in width. All the fertilizer was applied at the seedling stage or the heading stage at a rate of 4:6.
For the last winter wheat season of 2019–2020, because of the COVID-19 outbreak, we were unable go to the field, so we simply asked the farmer to implement a one-time fertilizer application at the same urea rate of 83 kg N ha-1 for all the plots. Other field managements such as weed and pest control were conducted in a similar manner to local farming practices. In general, maize was planted at the end of May and harvested at the beginning of September, and wheat was planted at the end of October and harvested at the beginning of May. The planting varieties were ZheTianLuo7 for maize, ZheNJ1018 for wheat_17_18, and JiaFengMai11 for wheat in other years. All the aboveground crop residue was removed from the field.
2.3 Soil-plant sampling and analysis
All maize and wheat were harvested at physiological maturity by hand. Crop yields were measured at each harvest; however, results for the first summer maize and last winter wheat were not included in analyses, owing to some issues with field management. For soil properties and soil microbial analysis, five soil cores of 0–20 cm depth were evenly collected in an S-line from each plot. In the maize season, all the soils were taken from bulk areas because the different characteristics of non-rhizophere and rhizophere zones. In the wheat season, soil samples were taken from the field in an even pattern due to the wheat seeds having been evenly spread in field, making it difficult to discriminate the non-rhizophere and rhizophere zones.
Regarding the analysis of soil properties, about 1 kg of soil was taken at the harvest of summer maize in 2019, and winter wheat in the 2018–2019 and 2019–2020 seasons. Those soils stored in a plastic bag and transplanted into lab in an air temperature condition. Impurities such as stone particles and plant residues were removed, and the samples were air dried in the laboratory. Soil samples were sieved through 2 mm and 0.18 mm stainless steel screens for subsequent soil pH and soil chemical property analyses. Soil pH was measured by the potentiometry method using a pH meter with a rate of 1:2.5 to soil: water (HJ 962–2018). Crop samples of straw and grain (±500 g) were dried at 70°C and sieved through 0.18 mm stainless steel screens. The TN and TC content of soil and crop samples were measured using an elemental analyzer (Enlmetar Vario Max CN, Germany).
Regarding soil microbial diversity measurements, about 50 g of soil was sampled using a 1 cm diameter stainless-steel soil drill and placed into a sealed plastic bag. The sampled soils stored in a constant temperature box with ice for transportation. The collected soil samples were mixed, and stored at −80°C for DNA extraction. These soils were sampled on 2 February 2019, 15 May 2019, 10 August 2019, and 8 November 2019.
2.4 DNA extraction, 16S rRNA gene amplification, and MiSeq sequencing
For soil DNA extraction, 0.5 g stored fresh soil was weighed and was extracted using a Power Soil DNA Isolation Kit (MoBio Laboratories, United States) according to the manufacturer’s protocol. The extracted DNA was quantified with a NanoDrop™ 2000 spectrophotometer (Thermo Fisher Scientific, United States), and validated on 1.2% (w/v) ethidium bromide-stained agarose gels. The V3–V4 regions of the bacterial 16S rRNA genes were amplified using the primer sets 338F (5′-ACTCCTACGGGAGGCAGCA-3′) and 806R (5′-GGACTACHVGG GTWTCTAAT-3′) with a barcode (Caporaso et al., 2011). PCR reactions, containing 25 μL of 2×Premix Taq (TakaraBiotechnology, Dalian, China), 1 μL of each primer (10 mM), and 3 μL DNA (20 ngμl-1) of template, were amplified by thermo cycling (30 cycles of 30 s denaturation at 94°C, 30 s annealing at 52°C, and 30 s extension at 72°C) after initialization at 94°C for 5 min, followed by a 10-min final elongation at 72°C. The PCR products were purified using Vazyme VAHTSTM DNA Clean Beads (Vazyme Biotech Co., Ltd., China). Sequencing libraries were generated with a TruSeq Nano DNA LT Library Prep Kit (Illumina). The library quality was evaluated on an Agilent Bioanalyzer 2100 system with an Agilent High Sensitivity DNA Kit (Agilent Technologies, Germany). Finally, the library was sequenced on an Illumina NovaSeq platform.
2.5 Data analysis
The raw data were filtered, spliced, and chimeras were removed through QIIME2 (2019.4) software, and then clustered into OTU (Operational Taxonomic Units) for species classification (S1). The OTU similarity was set to 97%. By comparing the Silva132 data base, the species classification information corresponding to each OTU was obtained. Microbial community abundance, alpha adversity, and beta adversity were accessed by QIIME2 (2019.4) and the plots were graphed using R (v.3.1.1). Alpha adversity included Chao1, Shannon, Simpson, Observed species, Faith’s PD, Pielou’s evenness, and Good’s cover. Kruskal–Wallis and Dunn’s test were used to test the significance of different treatments on alpha adversity. Only the α-diversity index, which showed significant differences between treatments, were used in results. Beta adversity was expressed by weighted UniFrac principal coordinates analysis (PCoA). Pearson’s correlations were analyzed using SigmaStat v.12.0. For crop and soil pH responses to N fertilizer, the figures and relationships of polynomial regression were also conducted by SigmaStat v.12.0. Differences between the treatments of urea U250 and controlled-release fertilizer C250 were tested using the Mann-Whitney rank sum test.
Crop N use efficiency (NUE) of aboveground biomass was calculated with the following equation (Yu et al., 2022):
3 Results
3.1 Crop yields and crop N uptake
Nitrogen fertilization significantly influenced crop yields of wheat and maize (Figure 1A); crop yield response to N was best explained by a quadratic relationship. For the no-N control, wheat grain yield was 899–3,072 kg ha-1 and maize grain yield was 1,560 kg ha-1 (Figure 1A). Wheat grain yields in 2017–2018 (7,000 kg ha-1) and 2018–2019 (5,000 kg ha-1) were maximized at 150 kg ha-1 (Figure 1A), indicating that any rate above this could result in excess N in the soil and potential N losses. Wheat in 2017–2018 showed a significantly greater gain in yield than that of 2018–2019, which may have been caused by the wheat varieties. Wheat grain yields in 2019–2020, which involved the application of urea at 83 kg N ha-1 for all treatments, were only 1938–3,635 kg ha-1 and notably lower than wheat yields in other years (data not shown). The N rate at which maize yield was maximized was 200 kg ha-1, maize grain yield at this N rate was up to 5,000–6,000 kg ha-1 (Figure 1A). Maize and wheat grain yields were similar between urea vs. controlled-release fertilizer except for maize in 2019, suggesting that the controlled release types were not necessarily increasing yield (S1).
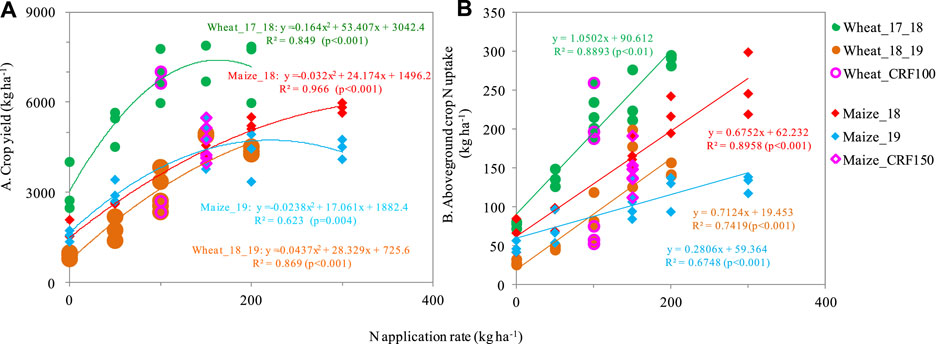
FIGURE 1. Responses of crop grain yield (A) and aboveground crop N uptake (B) to N fertilization rate and N fertilizer type.
Crop N uptake was also obviously affected by N application, and it increased with an increase in N application rate (Figure 1B). For wheat, the N use efficiency (NUE) averaged 105.0% (91.3%–120.5%) in the wheat_17_18 season and 49.0% (28.6%–76.7%) in the wheat_18_19 season (Figure 1B). Meanwhile, maize showed a significantly lower NUE with an average of 54.6% (40.9%–66.0%) for maize_18% and 33.9% (23.5%–41.8%) for maize_19 (Figure Figure1B). The rotation of winter wheat_17_18 and summer maize_18 showed a much higher NUE than the following rotation (Figure 1B). Considering the different N application rates, NUE reached a higher value of over79.7% for wheat at 150 kg ha-1, and 31.1%–66.0% for maize at 200 kg ha-1. Furthermore, the controlled-release fertilizer was not significantly different from the urea application except for maize_19 with a lower N uptake at urea application (S1).
3.2 Soil properties
The soil pH, TN, and TC ranged 4.49–6.43, 0.81–1.22 g kg-1, and 6.71–8.88 g kg-1, respectively. All these soil properties were obviously influenced by N rate (Figure 2). Soil pH was linearly decreased as N fertilization amount increased. For each kg of N applied, soil pH decreased by 0.0004–0.007 units (Figure 2A). Soil TN and TC were also affected by N fertilization. Mostly, a quadratic trend explained the increase in TN and TC in the soil following N fertilization, indicating that soil TN and TC increased with N fertilization but only to a certain N rate (Figures Figure2B, C). The maize harvest season had a slightly higher soil TN and TC than the wheat seasons. For soil TC, the wheat_19_20 season was found significantly lower value than that of wheat_18_19 (Figure 2C). The maximum soil TN and TC were 1.22 g N kg-1 and 8.88 g C kg-1 at the U350 treatment. However, the soil C/N ratio ranged from 6.47 to 8.74 and it decreased as N fertilizer increased (Figure 3D).
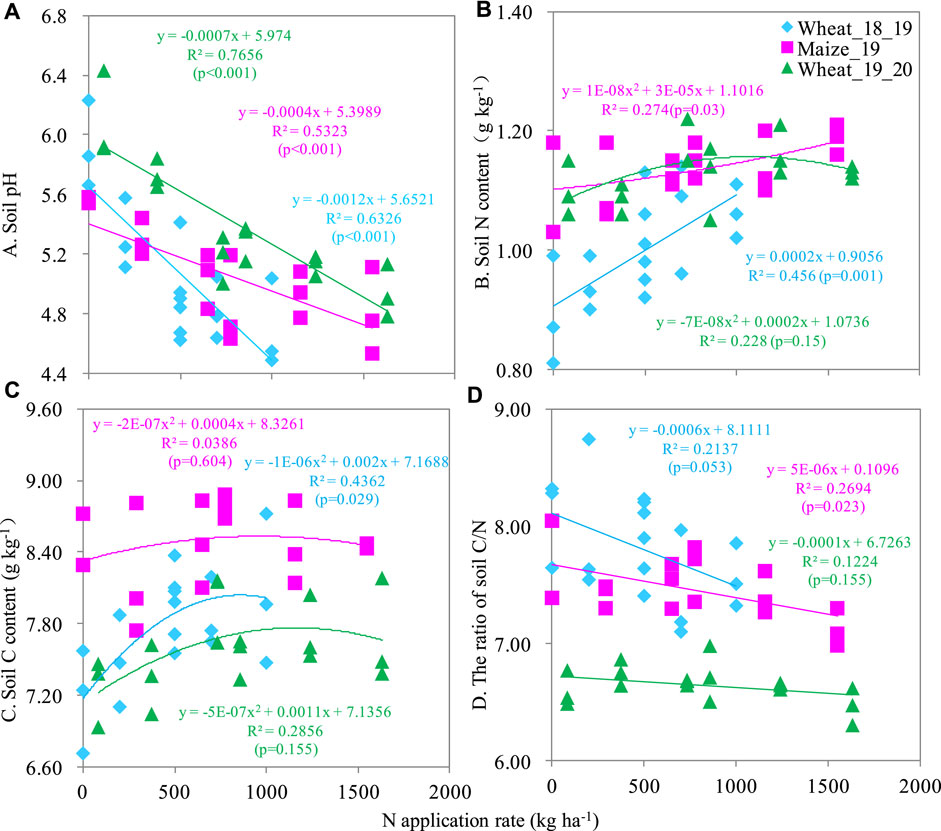
FIGURE 2. The relationship between N application rate and soil pH (A), TN (B), TC (C), and C/N ratio (D).
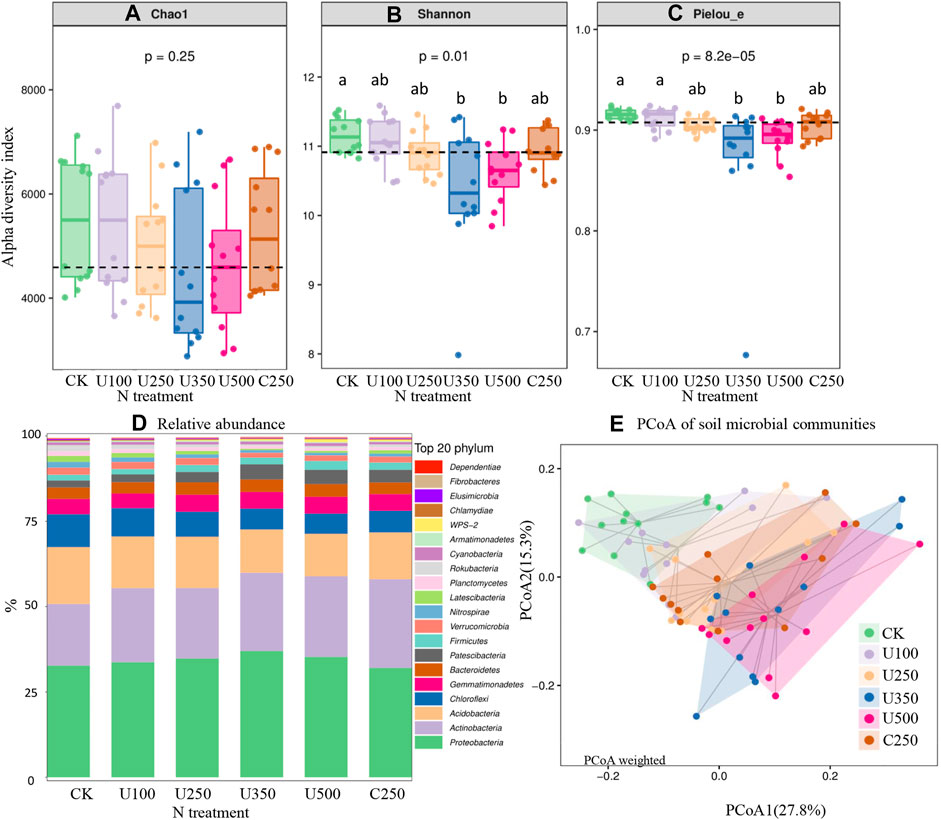
FIGURE 3. The effects of N application on soil alpha diversity index of Chao (A), Shannon (B), and Pielou_e (C), relative abundance (D), and PCoA of soil microbial communities (E).
3.3 Soil microbial community
Results of the influence of N application on soil microbial community are shown in Figure 3. Although no significant differences were observed from the Chao index, the data distribution indicated that increasing N rate tended to decrease soil microbial diversity (Figure 3A). At high N rates (U350 and U500), Shanon and Pielou_e indices were lower than at other N rates (Figures 3B, C). Compared with urea application U250, the controlled-release fertilizer C250 did not cause notable changes in α-diversity (Figures 3A,B, C).
At the phylum level, the relative abundances of the top 20 phyla in each treatment ranged from 99.39% to 99.69% and the other 24 phyla were only 0.31%–0.61% (Figure 3D). The main bacterial communities were Proteobacteria (32.1%–37.0%), Actinobacteria (18.1%–26.0%), Acidobacteria (12.4%–16.6%), Chloroflexi (5.9%–9.7%), Gemmatimonadetes (4.3%–4.9%), Bacteroidetes (3.3%–3.7%), and Verrucomicrobia (2.0%–4.4%) (Figure 3D). Among these bacterial communities, an increase in N rate decreased Acidobacteria and Chloroflexi bacterial communities. The other five major phyla increased with N application. Furthermore, the relative abundance of Nitrospirae ranged from 0.77% to 1.70% and decreased with an increase in N rate. Nitrogen fertilizer type (urea U250 vs. controlled-release N C250) showed similar relative abundances for most bacteria (Figure 3D). However, there was around a 5% higher relative abundance of Acidobacteria found in controlled-release N C250 than in urea U250, and around 1%–3% higher relative abundance of Proteobacteria, Acidobacteria, and Chloroflexi observed in urea U250 than controlled-release N C250 (Figure 3D).
Principal component analysis of bacterial community structure, based on the weighted UniFrac metric, indicated that the primary axis (27.8%) distinguished the effects of different N application rates, and the second axis (15.3%) distinguished different fertilizer types (Figure 3E). Based on the results, U350 and U500 were significantly different from CK. However, no differences were found between urea and controlled-release N fertilizer.
3.4 Relationship of factors
Correlation analysis indicated that soil pH negatively affected crop grain yield, soil TN, and TC contents (Table 1). Soil TN and TC concentrations had a significant and positive effect on crop yields and soil TN and TC were positively correlated (Table 1).
For the top 10 phyla of soil microbial communities, soil pH showed significant negative effects on Actinobacteria, Gemmatimonadetes, Firmicutes, and Patescibacteria and positive effects on Acidobacteria, Chloroflexi, and Nitrospirae (Table 1). Correlations among crop yields, soil TN, and TC contents were opposite to those of soil pH (Table 1). With the exception of Gemmatimonadetes and Firmicutes, the other five phyla were not significantly influenced by crop yield (Table 1).
4 Discussion
4.1 The effect of chemical N on soil acidification
Nitrogen application notably impacted soil chemical properties. In our trial, N fertilization decreased soil pH significantly. Results of other studies were found to be in line with ours. For example, significant soil acidification was found in a 6-year switchgrass (Panicum virgatum L.) after the application of chemical N fertilizer, and adding 196 kg N ha-1 decreased the soil pH from 5.74 to 6.35 (no-N control) to 4.50–4.93 (Li et al., 2021). In general, repeated application of ammonium-based fertilizers is known to decrease soil pH over time (Sadeghpour et al., 2017). Similarly, Hui et al. (2022) report that soil pH in a maize-wheat rotation system decreased from 7.93 (no-N control) to 6.53 at 300 kg N ha-1 urea in north China over 15 years. Similar to urea, controlled-release N fertilizer has been shown to notably decrease soil pH over time compared to CK. Our results support previous findings that chemical N fertilizer accelerates soil acidification (Mei et al., 2021).
Soil acidity is a major limiting factor for crop production. Our correlation analysis indicated a negative relationship between soil pH and crop yield (Table 1). Petersen (2015) reports that soil acidity costs Western Australian grain farmers about A$1.6 billion per year in lost production. In that region, the application of lime is insufficient to reverse acidification (Gazey et al., 2014). Moreover, soil acidity can stimulate an increase in soil-available heavy metals such as lead, cadmium, mercury, and arsenic (Deng et al., 2020). In the south of China, soil heavy metals pollution is exacerbated by soil acidification. In our study, soil pH decreased linearly with increasing N fertilization rates; for each kg of N applied, soil pH decreased by 0.0004–0.007 units (Figure 2A). These results suggest a need to periodically assess soil pH and adjust it to avoid reductions in crop yield. Consideration of the above findings calls for chemical N fertilizers to be replaced with organic or bio carbon-based products.
4.2 The effect of chemical N on soil nutrition imbalance
Nitrogen fertilizer application increased soil TN and TC contents (Figures 3B, C). Similarly, another study also found that soil TN and soil TC were increased with an increase in chemical N application rate (Li et al., 2019; Hui et al., 2022). This increase in soil TC and TN could be attributed to that N fertilizer application promoted an increase in plant root biomass and fallen leaves residues (Khan et al., 2007; Li et al., 2019). Compared to wheat, maize generally has a much higher root biomass. Consequently, soil TN and TC in the maize harvest season was higher than that of the wheat season (Figures 2B, C). The significantly lower TC in wheat_19_20 could have been influenced by the lower urea application rate (83 kg N ha-1) and much lower wheat biomass than that of wheat_18_19. Regarding soil TN, chemical N input can also increase the soil TN pool. However, Zeng et al. (2016) report that N addition (0–240 kg N ha-1 y-1) had fewer effect on soil TC and TN throughout 6 years of grassland management. This could be explained by the higher initial soil N 0.21% and soil C 1.86% in grasslands. For our research, the highest level of soil N and soil C were only 0.12% and 0.9%, respectively, and these did not continue to increase when N application reached high levels. The latter results suggested that soil N and C reached a saturated state when N was applied at a certain high level (Viljoen et al., 2020). Before reaching saturation, soil TN and TC pools can increase.
According to Hui et al. (2022), nitrogen fertilization could decrease the C/N ratio and they reported that the soil C/N ratio was decrease two-fold by the addition of 300 kg N ha-1 y-1 compared to a no-N control in a maize-wheat rotation. Similarly, our data suggested that adding N fertilizer linearly decreased the C/N ratio. An explanation for this decrease could be that N application did not increase C and N in the same ratio, leading to a higher increase of N than C and thus, a decrease in the C/N ratio.
4.3 The effect of fertilization on soil microbial activities
Soil bacterial composition is very sensitive to management practices including N fertilizer inputs (Guo et al., 2020; Dang et al., 2022; Rieke et al., 2022). In this study, with increasing N rates, the microbial communities showed a decrease in richness, diversity, and evenness, which is consistent with previous studies (Zeng et al., 2016; Mei et al., 2021). High urea application rates have been reported to inhibit the development of ammonium oxidizing bacteria and archaea owing to the release of ammonium which acts as an electron donor for nitrification (Geisseler and Scow, 2014). Meanwhile, lower ammonium concentration can enhance nitrification rates (Koper et al., 2010). For our study, application rates as high as 350 and 500 kg ha-1 strongly inhibited microbial community development. Microbial structure also changed significantly with N application. These results indicated that some microbial communities were promoted, and some were inhibited by N application. In detail, the richness of Actinobacteria, Gemmatimonadetes, Firmicutes and Patescibacteria increased with the increased N rates. However, increasing N application rates decreased the richness of Acidobacteria, Chloroflexi and Nitrospirae. Similarly, Actinobacteria and Patescibacteria were decreased when N application rates increased (Yu et al., 2019; Mei et al., 2021).
The above-mentioned changes in microbial communities may have been caused by soil properties. In our study, we found that decreased soil pH, and increased soil TN and TC contents stimulated the richness of Actinobacteria, Gemmatimonadetes, Firmicutes and Patescibacteria, but inhibited the growth of Acidobacteria, Chloroflexi and Nitrospirae. Similarly, other studies also found that these bacteria were positively or negatively correlated with soil pH, SOM, TN, and TC (Yu et al., 2019; Guo et al., 2020). Acidobacteria is acidophilic and is positively correlated with soil pH and negatively correlated with soil organic C (Dai et al., 2018). Deng et al. (2020) also report that Nitrospirae declined under mineral N addition, which may be because it decreased nitrification activity. Therefore, the impacts of N application on soil bacterial composition maybe regulated by soil properties.
4.4 The effect of N fertilizer on crop yield and mitigation solutions
As expected, N fertilization notably increased crop yields. The results indicated that wheat yield was maximized at 150 kg N ha-1 and maize yield was maximized at 200 kg N ha-1. At these N application rates, we also found notably higher crop N use efficiency for wheat and maize. These results indicated that any rates above those given could lead to economic losses, excess N balances, and potential N losses (Sadeghpour et al., 2017). In a 14-year trial, Wang et al. (2022) reported that maize yield reached 5,000–7,000 kg ha-1 at 240 kg N ha-1 y-1 which is similar to our findings. In rural China, farmers apply about 300 kg N ha-1 y-1 for wheat and about 250 kg N ha-1 y-1 for maize (Guo et al., 2022). These levels are significantly higher than our reported N application rates for a maize-wheat rotation. Italian farmers applied around 170 kg N ha-1 chemical fertilizer with a yield of 5,800 kg ha-1 for wheat and about 230 kg N ha-1 urea with a yield about 9,000 kg ha-1 for maize (Fantin et al., 2017). However, a survey in Minnesota, United States, showed that in a corn crop only given 142–169 kg N ha-1, the corn yield reached 7.78 × 103–10.61 × 103 kg ha-1 (Bierman et al., 2012). Therefore, an excessive application of chemical N will not increase crop yields. According to the above-mentioned reports, the N application rate could be no higher than 150 kg N ha-1 for wheat and about 200 kg N ha-1 for maize; this recommendation is consistent with the wheat-maize rotation research findings in the north of China: Beijing, Hebei, Henan, and Shandong province (Khashnevisan et al., 2020).
Regarding NUE, our study showed the first-year wheat_17_18 ranged 91.0%–120.5% and the maize 40.9%–66.0%. These rates were much higher than in previous studies and Yu summarizes that global NUE is about 42% for wheat and 36% for maize (Yu et al., 2022). Khashnevisan et al. (2020) report that the NUE of wheat and maize respectively ranged 27%–61% and 34%–53%. However, similar to previous studies, the following rotation also showed much lower NUE and, with rates of 28.6%–79.7% for wheat and 23.5%–46.3% for maize. The higher NUE of the preceding rotation may have been affected by the previous 5 years of vegetable crops, which involved high inputs of organic and inorganic fertilizer. Furthermore, high levels of nutrient residues in soil can improve crop growth. Similarly, maize rotation with sorghum and soybeans can significantly increase maize yield and soil nitrogen stocks (Silva et al., 2020). Therefore, varying the crops in a rotation may be an efficient means of increasing NUE and reducing N environmental pollution.
Furthermore, chemical N application increased soil health risks such as soil acidification and imbalances in soil N and C content, and decreased soil microbial diversity. These properties may be improved by returning crop straw to the land, the addition of manure, intercropping, or a fixed N crop rotation (Liptzin et al., 2022). Manure and straw addition were found to significantly increase SOC, TC, and TN (Sadeghpour et al., 2016), and consequently promoted the growth of Firmicutes and Proteobacteria (Sun et al., 2015; Dang et al., 2022). Furthermore, compared to chemical N, straw, and manure addition have been found to mitigate the decline of Nitrospirae (Dang et al., 2022). Soil pH can be increased by the addition of manure, especially if it contains high amounts of carbonate (Sadeghpour et al., 2016). However, pH can be exacerbated by straw in corporation (Sun et al., 2015; Dang et al., 2022). Intercropping maize and soybeans can notably increase soil SOC storage (Glaze-Corcoran et al., 2020; Wang et al., 2021). Intercropping with peanuts and soybeans (Glycine max L.) can significantly increase crop yields, especially for the intercropping of soybeans, but with no obvious changes in soil N (Wang et al., 2022). Hence, manure application and intercropping with legume crops may be effective practices for maize and wheat production by improving soil acidification, SOM, and soil microbial growth.
5 Conclusion
This study revealed that long-term chemical N fertilizer application in a maize/wheat system that was previously vegetable farmland can cause significant soil health problems by accelerating soil acidification, increasing soil N and C imbalance, decreasing soil microbial diversity and changing bacterial communities. We concluded that soil acidification rate was 0.0004–0.0012 pH equivalent if chemical N fertilizer was applied at 1 kg N ha-1. The soil TN and TC content became more stable at certain cumulative N application rates. However, the C/N ratio linearly decreased as N rate increased. The correct rate for N application to wheat (150 kg N ha-1) and maize (200 kg N ha-1) is critical to maximize crop yield and N use efficiency. N chemical fertilizer application significantly improved the growth of Actinobacteria, Gemmatimonadetes, Firmicutes and Patescibacteria, but inhibited the growth of Acidobacteria, Chloroflexi and Nitrospirae. Compared with urea, the controlled-release fertilizer did not notably change crop yields, soil TN or TC content, and soil bacterial diversity. We recommend that growers should apply N at the correct rate, and that performing rotations with different crops can notably increase crop yield and NUE. Integrated manure and legume intercropping could also reduce the chemical N lost in a maize-wheat system, reduce the chance of soil acidification, and increase SOM and soil microbial growth, all critical for sustaining crop production and global food security.
Data availability statement
The original contributions presented in the study are included in the article/Supplementary Material, further inquiries can be directed to the corresponding authors.
Author contributions
MD: Conceptualization, methodology, and writing; HM and DY: Data analysis; AS: Reviewing and editing; GY and YH: Conceptualization, methodology, and supervision.
Funding
The financial support of the Natural Science Foundation of Zhejiang Province (LGJ21D030001) and the Key Project of Zhejiang Province (2021C04020 and 2022C02046) is gratefully acknowledged.
Conflict of interest
The authors declare that the research was conducted in the absence of any commercial or financial relationships that could be construed as a potential conflict of interest.
Publisher’s note
All claims expressed in this article are solely those of the authors and do not necessarily represent those of their affiliated organizations, or those of the publisher, the editors and the reviewers. Any product that may be evaluated in this article, or claim that may be made by its manufacturer, is not guaranteed or endorsed by the publisher.
Supplementary material
The Supplementary Material for this article can be found online at: https://www.frontiersin.org/articles/10.3389/fenvs.2023.1108288/full#supplementary-material
References
Allison, S. D., Hanson, C. A., and Treseder, K. K. (2007). Nitrogen fertilization reduces diversity and alters community structure of active fungi in boreal ecosystems. Soil Biol. Biochem. 39, 1878–1887. doi:10.1016/j.soilbio.2007.02.001
Bierman, P. M., Rosen, C. J., Venterea, R. T., and Lamb, J. A. (2012). Survey of nitrogen fertilizer use on corn in Minnesota. Agric. Syst. 109, 43–52. doi:10.1016/j.agsy.2012.02.004
Caires, E. F., Corrêa, J. C. L., Churka, S., Barth, G., and Garbuio, F. J. (2006). Surface application of lime ameliorates subsoil acidity and improves root growth and yield of wheat in an acid soil under no-till system. Sci. Agric. 63, 502–509. doi:10.1590/s0103-90162006000500013
Caporaso, J. G., Lauber, C. L., Walters, W. A., Berg-Lyons, D., Lozupone, C. A., Turnbaugh, P. J., et al. (2011). Global patterns of 16S rRNA diversity at a depth of millions of sequences per sample. Proc. Natl. Acad. Sci. 108, 4516–4522. doi:10.1073/pnas.1000080107
Dai, Z., Su, W., Chen, H., Barberan, A., Zhao, H., Yu, M., et al. (2018). Long-term nitrogen fertilization decreases bacterial diversity and favors the growth of Actinobacteria and Proteobacteria in agro-ecosystems across the globe. Glob. Change Biol. 24, 3452–3461. doi:10.1111/gcb.14163
Dang, P., Li, C., Lu, C., Zhang, M., Huang, T., Wan, C., et al. (2022). Effect of fertilizer management on the soil bacterial community in agroecosystems across the globe. Agric. Ecosyst. Environ. 326, 107795. doi:10.1016/j.agee.2021.107795
Davison, E. A. (2009). The contribution of manure and fertilizer nitrogen to atmospheric nitrous oxide since 1860. Nat. Geosci. 2, 659–662. doi:10.1038/ngeo608
Deng, M., Zhu, Y., Shao, K., Zhang, Q., Ye, G., and Shen, J. (2020). Metals source apportionment in farmland soil and the prediction of metal transfer in the soil-rice-human chain. J. Environ. Manag. 260, 110092. doi:10.1016/j.jenvman.2020.110092
Fantin, V., Righi, S., Rondini, I., and Masoni, P. (2017). Environmental assessment of wheat and maize production in an Italian farmers' cooperative. J. Clean. Prod. 140, 631–643. doi:10.1016/j.jclepro.2016.06.136
Gazey, C., Oliver, Y., and Fisher, J. (2014). 20 Years of soil acidity RD andE in western Australia—what have we learned? Grain industry association of western Australia 2014 perth crop updates. Available at: http://www.giwa.org.au/2014-cropupdates.
Geisseler, D., and Scow, K. M. (2014). Long-term effects of mineral fertilizers on soil microorganisms—a review. Soil Biol. Biochem. 75, 54–63. doi:10.1016/j.soilbio.2014.03.023
Glaze-Corcoran, S., Hashemi, M., Sadeghpour, A., Jahanzad, E., Afshar, R. K., Liu, X., et al. (2020). Understanding intercropping to improve agricultural resiliency and environmental sustainability. Adv. Agron. 162, 199–256. doi:10.1016/bs.agron.2020.02.004
Guo, J., Li, C., Xu, X., Sun, M., and Zhang, L. (2022). Farmland scale and chemical fertilizer use in rural China: New evidence from the perspective of nutrient elements. J. Clean. Prod. 376, 134278. doi:10.1016/j.jclepro.2022.134278
Guo, Z., Wan, S., Hua, K., Yin, Y., Chu, H., Wang, D., et al. (2020). Fertilization regime has a greater effect on soil microbial community structure than crop rotation and growth stage in an agroecosystem. Appl. Soil Ecol. 149, 103510. doi:10.1016/j.apsoil.2020.103510
Hajkowicz, S., and Young, M. (2005). Costing yield loss from acidity, sodicity and dryland salinity to Australian agriculture. Land Degrad. Dev. 16, 417–433. doi:10.1002/ldr.670
Hati, K. M., Swarup, A., Mishra, B., Manna, M., Wanjari, R., Mandal, K., et al. (2008). Impact of long-term application of fertilizer, manure and lime under intensive cropping on physical properties and organic carbon content of an Alfisol. Geoderma 148, 173–179. doi:10.1016/j.geoderma.2008.09.015
Hui, K., Xi, B., Tan, W., and Song, Q. (2022). Long-term application of nitrogen fertilizer alters the properties of dissolved soil organic matter and increases the accumulation of polycyclic aromatic hydrocarbons. Environ. Res. 215, 114267. doi:10.1016/j.envres.2022.114267
Kalkhoran, S. S., Pannell, J. D., Thamo, T., White, B., and Polyakov, M. (2019). Soil acidity, lime application, nitrogen fertility, and greenhouse gas emissions: Optimizing their joint economic management. Agric. Syst. 176, 102684. doi:10.1016/j.agsy.2019.102684
Khan, S. A., Mulvaney, R. L., Ellsworth, T. R., and Boast, C. W. (2007). The myth of nitrogen fertilization for soil carbon sequestration. J. Environ. Qual. 36, 1821–1832. doi:10.2134/jeq2007.0099
Khashnevisan, B., Rafiee, S., Pan, J., Zhang, Y., and Liu, H. (2020). A multi-criteria evolutionary-based algorithm as a regional scale decision support system to optimize nitrogen consumption rate; A case study in North China plain. J. Clean. Prod. 256, 120213. doi:10.1016/j.jclepro.2020.120213
Kong, X., Lai, R., Li, B., Liu, H., Li, K., Feng, G., et al. (2014). Chapter four - fertilizer intensification and its impacts in China's HHH plains. Adv. Agron. 125, 135–169. doi:10.1016/B978-0-12-800137-0.00004-2
Koper, T. E., Stark, J. M., Habteselassie, M. Y., and Norton, J. M. (2010). Nitrification exhibits Haldane kinetics in an agricultural soil treated with ammonium sulfate or dairy-waste compost. FEMS Microbiol. Ecol. 74, 316–322. doi:10.1111/j.1574-6941.2010.00960.x
Li, B., Roley, S. S., Duncan, D. S., Guo, J., Quensen, J. F., Yu, H. Q., et al. (2021). Long-term excess nitrogen fertilizer increases sensitivity of soil microbial community to seasonal change revealed by ecological network and metagenome analyses. Soil Biol. Biochem. 160, 108349. doi:10.1016/j.soilbio.2021.108349
Li, M., Hu, H., He, X., Jia, J., Drosos, M., Wang, G., et al. (2019). Organic carbon sequestration in soil humic substances as affected by application of different nitrogen fertilizers in a vegetable-rotation cropping system. J. Agric. food Chem. 67 (11), 3106–3113. doi:10.1021/acs.jafc.8b07114
Li, W., Xie, L., Zhao, C., Hu, X., and Yin, C. (2022). Nitrogen fertilization increases soil microbial biomass and alters microbial composition especially under low soil water availability. Microb. Ecol. 8, 1–13. doi:10.1007/s00248-022-02103-8
Liptzin, D., Norris, C. E., Cappellazzi, S. B., et al. (2022). An evaluation of carbon indicators of soil health in long-term agricultural experiments. Soil Biol. Biochem. 172, 108708.
Lucas, R. W., Klaminder, J., Futter, M. N., Bishop, K., Egnell, G., Laudon, H., et al. (2011). A meta-analysis of the effects of nitrogen additions on base cations: Implications for plants, soils, and streams. For. Ecol. Manag. 262, 95–104. doi:10.1016/j.foreco.2011.03.018
Mack, M. C., Schuur, E. A. G., Bret-Harte, M. S., Shaver, G. R., and Chapin, F. S. (2004). Ecosystem carbon storage in arctic tundra reduced by long-term nutrient fertilization. Nature 431, 440–443. doi:10.1038/nature02887
Mei, N., Zhang, X., Wang, X., Peng, C., Gao, H., Zhu, P., et al. (2021). Effects of 40 years applications of inorganic and organic fertilization on soil bacterial community in a maize agroecosystem in northeast China. Eur. J. Agron. 130, 126332. doi:10.1016/j.eja.2021.126332
Petersen, E. (2015). Economic analysis of the impacts and management of SubsoilConstraints. Report for the grains research and development corporation FundedProject: DAW00242 subsoil constraints-understanding and management. Western Australia: Department of Primary Industries and Regional Development.
Ramirez, K. S., Craine, J. M., and Fierer, N. (2012). Consistent effects of nitrogen amendments on soil microbial communities and Processes across biomes. Glob. Change Biol. 18, 1918–1927. doi:10.1111/j.1365-2486.2012.02639.x
Rieke, E. L., Cappellazzi, S. B., Cope, M., et al. (2022). Linking soil microbial community structure to potential carbon mineralization: A continental scale assessment of reduced tillage. Soil Biol. Biochem. 168, 108618.
Sadeghpour, A., Ketterings, Q. M., Godwin, G. S., Czymmek, K. J., et al. (2017). Under-or over-application of nitrogen impact corn yield, quality, soil, and environment. Agron. J. 109 (1), 343–353. doi:10.2134/agronj2016.06.0355
Sadeghpour, A., Ketterings, Q. M., Vermeylen, F., Godwin, G. S., and Czymmek, K. J. (2016). Soil properties under nitrogen vs. phosphorus based manure and compost management of corn. Soil Sci. Soc. Am. J. 80 (5), 1272–1282. doi:10.2136/sssaj2016.03.0086
Savci, S. (2012). An agricultural pollutant: Chemical fertilizer. Int. J. Environ. Sci. Dev. 3, 73–80. doi:10.7763/ijesd.2012.v3.191
Silva, P. C. G., Tiritan, C. S., Echer, F. R., Cordeiro, C. F. d. S., Rebonatti, M. D., and Santos, C. H. d. (2020). No-tillage and crop rotation increase crop yields and nitrogen stocks in sandy soils under agroclimatic risk. Field Crops Res. 258, 107947. doi:10.1016/j.fcr.2020.107947
Sun, R., Zhang, X., Guo, X., Wang, D., and Chu, H. (2015). Bacterial diversity in soils subjected to long-term chemical fertilization can be more stably maintained with the addition of livestock manure than wheat straw. Soil Biol. Biochem. 88, 9–18. doi:10.1016/j.soilbio.2015.05.007
Viljoen, C., van der Colf, J., and Swanepoel, P. A. (2020). Benefits are limited with high nitrogen fertiliser rates in kikuyu-ryegrass pasture systems. Land 9 (6), 173. doi:10.3390/land9060173
Wang, X., Chen, Y., Yang, K., Duan, F., Liu, P., Wang, Z., et al. (2021). Effects of legume intercropping and nitrogen input on net greenhouse gas balances, intensity, carbon footprint and crop productivity in sweet maize cropland in South China. J. Clean. Prod. 314, 127997. doi:10.1016/j.jclepro.2021.127997
Wang, Y., Zhang, Y., Zhang, H., Yang, Z., Zhu, Q., Yan, B., et al. (2022). Intercropping-driven nitrogen trade-off enhances maize productivity in a long-term experiment. Field crops Res. 287, 108671. doi:10.1016/j.fcr.2022.108671
Wessén, E., Hallin, S., and Philippot, L. (2010). Differential responses of bacterial and archaeal groups at high taxonomical ranks to soil management. Soil Biol. andBiochemistry 42, 1759–1765. doi:10.1016/j.soilbio.2010.06.013
Whitten, M. G., Wong, M. T. F., and Rate, A. W. (2000). Amelioration of subsurface acidity in the south-west of western Australia: Downward movement and mass balance of surface-incorporated lime after 2-15 years. Soil Res. 38, 711–728. doi:10.1071/sr99054
Wrage, N., Velthof, G. L., Van Beusichem, M. L., and Oenema, O. (2001). Role of nitrifier denitrification in the production of nitrous oxide. Soil Biol. Biochem. 33, 1723–1732. doi:10.1016/s0038-0717(01)00096-7
Yu, H., Ling, N., Wang, T., Zhu, C., Wang, Y., Wang, S., et al. (2019). Responses of soil biological traits and bacterial communities to nitrogen fertilization mediate maize yields across three soil types. Soil and Tillage Res. 185, 61–69. doi:10.1016/j.still.2018.08.017
Yu, X., Keitel, C., Zhang, Y., Wangeci, A. N., and Dijkstra, F. A. (2022). Global meta-analysis of nitrogen fertilizer use efficiency in rice, wheat and maize. Agric. Ecosyst. Environ. 338, 108089. doi:10.1016/j.agee.2022.108089
Keywords: chemical fertilizer, nitrogen, maize-wheat rotation, soil acidification, soil bacterial community, soil health
Citation: Deng M, Ma H, Sadeghpour A, Yang G, Hu Y and Yang D (2023) Responses of crop production and soil health to chemical nitrogen fertilization in a maize-wheat rotation system. Front. Environ. Sci. 11:1108288. doi: 10.3389/fenvs.2023.1108288
Received: 25 November 2022; Accepted: 03 February 2023;
Published: 13 February 2023.
Edited by:
Ryusuke Hatano, Hokkaido University, JapanReviewed by:
Ahmad Ali, Jiangsu University, ChinaMuhammad Shaaban, Bahauddin Zakariya University, Pakistan
Copyright © 2023 Deng, Ma, Sadeghpour, Yang, Hu and Yang. This is an open-access article distributed under the terms of the Creative Commons Attribution License (CC BY). The use, distribution or reproduction in other forums is permitted, provided the original author(s) and the copyright owner(s) are credited and that the original publication in this journal is cited, in accordance with accepted academic practice. No use, distribution or reproduction is permitted which does not comply with these terms.
*Correspondence: Guiling Yang, Z3VpbGluZ2NoaW5hMjAwOEAxNjMuY29t; Yan Hu, aHV5YW5AYWdyaS5nb3YuY24=