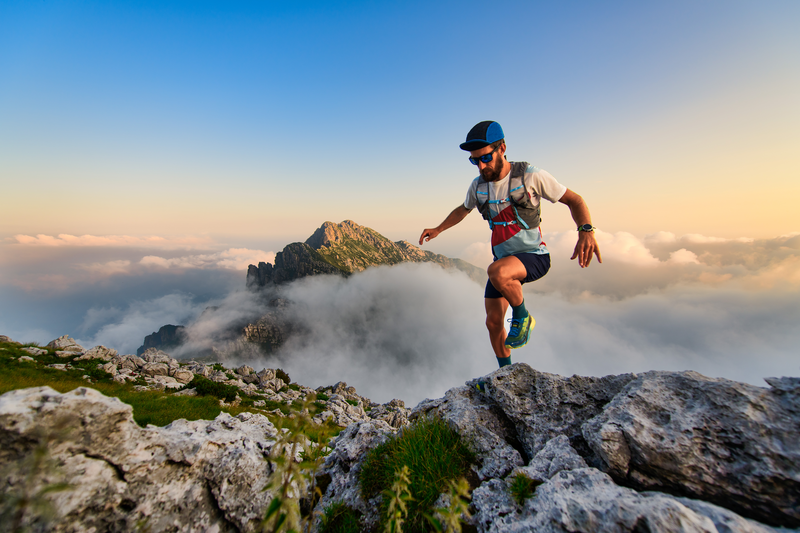
94% of researchers rate our articles as excellent or good
Learn more about the work of our research integrity team to safeguard the quality of each article we publish.
Find out more
ORIGINAL RESEARCH article
Front. Environ. Sci. , 09 February 2023
Sec. Freshwater Science
Volume 11 - 2023 | https://doi.org/10.3389/fenvs.2023.1099942
Vegetation restoration has always been the focus of ecological research, but the synergistic effect of the soil carbon cycle and vegetation restoration succession process in karst area is still unclear. In this study, the complete succession zones of non-karst, karst, and karst vegetation restoration landforms in a National Nature Reserve, Caohai (Guizhou Province, China), were compared. The content distribution characteristics of SOC, ROC and DOC were investigated, as well as the geomorphology, spatial location and interaction were studied by using GLMM. The results show that the soil types and vegetation coverage of different landforms lead to a decrease in SOC in karst area with an increase in depth. The soil content in karst area was low, and in order to maintain the normal growth of vegetation, the proportion of soil ROC and DOC in SOC must be high, which leads to the order of soil ROC content in unrepaired area > vegetation restoration area > non-karst area. In addition, the content of SOC in the surface layer of the vegetation restoration area was lower than that of the unrepaired area and the non-karst area. The soil microbial activity in the vegetation restoration area is stronger, and more organic carbon was fixed in the plant. The vegetation coverage and diversity increased obviously and markedly as a result of dominant plants changing (from Gramineae to Gramineae + Compositae) in the karst vegetation restoration area, but the soil in the karst area could not provide sufficient nutrition for plants, ultimately resulting in an inverse ratio between surface SOC and vegetation diversity. This study aims to enhance understandings of ecological functions and vegetation restoration in karst areas, as well as responses to regional carbon cycling.
Karst area is typical of fragile ecology in China, with a domestic area of up to 3.44 million km2 (Yuan, 2001; Wang, 2018). Due to special karst geographical and chemical properties, in-situ soils are characterized as having a low formation rate, weak water retention capacity, and imbalanced self-restoration (Lan et al., 2022; Yang et al., 2022). Up to 85% mountain coverage with steep terrain and thin soil layer, Guizhou province is regarded as an important karst areas in Southwest China (Zhao et al., 2017). Particularly, artificial disturbances aggravate soil erosion and rocky desertification as well as cause a significant reduction in soil coverage in the karst area (Zhou et al., 2020).
Soil organic carbon (SOC) serves as an indicator for soil properties, which improve nutrient cycling capacity and play an important role in soil and water conservation. In general, regional soil conditions (e.g., fertility and buffer capacity) are largely regulated by in-situ SOC (Ma et al., 2020). Active SOC can be directly utilized and transformed by plants and microorganisms, responding to regional carbon cycling and thus organic carbon balance (Cui et al., 2021; Wu et al., 2020). Readily oxidizable carbon (ROC), in particular, shows potential for capturing early SOC changes with high turnover rates (Zhang et al., 2019c). Dissolved organic carbon (DOC) is a carrier for element migration and transformation in soils, which highly affects nutrient cycles, microbial activities, and SOC transformation (Guo et al., 2020). Thus, it is necessary to unravel distinct organic carbon dynamics and influencing factors in soil environments (Bai et al., 2020; Gu et al., 2023).
Vegetation restoration is a common measure for soil and water conservation, which can also control rocky desertification in karst areas (Bastin et al., 2019; Jiang et al., 2014). Studies showed that vegetation restoration improved the ecological environment under the soil-plant complex system, promoting soil nutrient accumulation and improving rocky desertification (Gerhardt et al., 2017; Yadav et al., 2018; Huang et al., 2018). At present, some achievements made and widespread attentions on vegetation restoration in karst areas, however, researches were mostly focused on the corresponding restoration models and land use patterns. For example, previous reports examined the differences between natural and artificial restorations (Hu et al., 2018; Hu et al., 2020), the relationships between vegetation restoration measures and soil quality via model calculations (Zhang et al., 2019b), and the effects of different vegetation types on soil nutrients (Guan and Fan, 2020). Nevertheless, the mechanisms of vegetation restoration on soil property variability and the synergistic carbon cycle in karst areas have yet to be revealed (Rubino et al., 2010; Wu et al., 2022b). Soil SOC participates in the succession of vegetation restoration, linking soil and vegetation systems during the material cycle and accumulation. In this context, the heterogeneous organic carbon components generally trace the SOC pool and reflect its dynamics. As a result, plant communities stimulated organic carbon sequestration into soils via photosynthesis, contributing large amounts of SOC to terrestrial ecosystems (Wang et al., 2022). Therefore, studies on the variability of SOC components in response to vegetation restoration as well as their inherent links to soil nutrients are merited.
In this study, spatiotemporal substitution method (Sun et al., 2021) was used to compare variability of characteristic in the karst (Jiangjiawan) regional after vegetation restoration soil, unrepaired soil in the same region and non-karst soil (Yangguanshan) in a National Nature Reserve Caohai, Southwest China (the selected sample belt has spatial differences and can reflect the state of plant succession in different periods.). We explored SOC variety characteristics constrained by vegetation restoration during distinct landforms. The purposes are to provide theoretical support for further understandings of the regional carbon cycle, ecological functions, and vegetation restoration in karst areas, which hope to provide a scientific basis for vegetation selection after ecological destruction.
The study area was a National Nature Reserve Caohai (26°49′-26°53′N, 104°12′-104°18′E), situating in Guizhou Province, Southwest China (Figure 1). The Caohai was adjacent to the hinterland of the Wumeng Mountains in the central part of the Yunnan-Guizhou Plateau. It was a specific plateau wetland formed by geomorphologically stagnant water in the karst basin, which was also regarded as the largest karst artificial lake in China. Caohai was located in a subtropical monsoon area with discernible dry and wet seasons. Rainfall was concentrated in the wet season (summer and autumn) with sufficient light. The annual average temperature was 10.5°C. The soil types of the reserve mainly include yellow soil and calcareous soil with few plant species, which is typical of a plateau wetland ecosystem.
The study area was within the scope of the ‘ecological isolation corridor project of Caohai North Slope Mountain in Weining County’, Guizhou Province. Through the vegetation restoration carried out by artificially planting Yunnan poplar (broad-leaved deciduous forest) on the upper-slope, the purpose of reducing soil and water loss was achieved. The project improves the regional ecological environment and increases the ecological carrying capacity of the region while increasing the diversity of biological species.
Soil samples were collected in August 2021. Considering that the vegetation succession in the non-karst area was relatively perfect and the vegetation types are different in different slope positions. A sample belt was selected in a non-karst area locating on the upper-slope, middle-slope and lower-slope (three sample squares are arranged in each slope). Soil samples were randomly selected for analysis from five sampling points. In the karst area (Jiangjiawan), two sample zones before and after vegetation restoration were selected, all of which were in the same habitat, half of which were restored by vegetation and the other half as a comparative study. According to the present situation of vegetation succession, each sample zone is divided into two parts (uphill and downhill), and samples are taken according to different dominant communities of plants. Three sample squares were selected in each area, and five sample points were randomly selected for sampling and analysis (as shown in Figure 1). The age of the vegetation restoration transect was 3–4 years, and the natural vegetation was mainly herbaceous. The soil type in the Jiangjiawan area was calcareous, and the vegetation community was relatively uniform before restoration: including elm seedlings and herbaceous plant communities such as Elymus, pennisetum, and sage millet (Zhang et al., 2013; Wu et al., 2013). The basic situation was shown in Table 1.
The SOC content in three sample zones was analyzed using “spatio-temporal substitution method”. Soil samples were collected, and indeed, the community composition characteristics at each sample site were documented. The sampling profile was 50 cm deep with 10 cm intervals. Before laboratory analysis, soil samples were cryopreserved in a self-sealed bag. According to the experimental requirements, the collected samples were treated by air drying, crushing, and sieving.
SOC was determined by the potassium dichromate oxidation-external thermal method (Nelson and Sommers, 1982). ROC was determined by 333 mol/L KMnO4 oxidation-colorimetry (Blair et al., 1995). DOC was determined by 0.2 mol/L FeSO4 titration (Raber et al., 1998; Walkley and Black, 1934).
The total phosphorus (TP) content of soil was determined using NaOH. melting-molybdenum-antimony anti-chromogenic-ultraviolet spectrophotometry. Soil available phosphorus (AP) was determined by 0.5 mol/L NaHCO3 extraction-molybdenum-antimony anti-chromogenic-UV spectrophotometry. Ultraviolet spectrophotometry was employed to assess nitrate nitrogen and ammonium nitrogen. And the 2 mol/L potassium chloride-indophenol blue colorimetric method, respectively. All determinations used deionized water to measure soil pH at a ratio of 1 wt/vol (2.5) (Wu et al., 2022a). Samples for soil bulk density analysis were collected using steel cylinders (5 cm diameter and 5 cm height) with a volume of 100 cm3. Soil moisture content was obtained from weight loss by drying moist soil in the field at 105°C to a constant weight for at least 6 h. Determination of soil aggregates by the wet sieve method. All superior purity reagents were purchased from Sinopharm Chemical Reagent Co., Ltd (Shanghai, China) including sulfuric acid, hydrochloric acid, sodium hydroxide, etc. Soil physical and chemical properties are listed in Table 2.
The data were analyzed by Excel 2021, and SPSS 26.0 software was used for generalized linear mixed model (GLMM) analysis. The principal component analysis (PCA) of all kinds of SOC as variables was tested by Canoco Software 5.0 software. The drawing was prepared using GraphPad Prism 9.0 software, and the correlation analysis of the two matrices was analyzed by R 4.1.0.
The particle size distribution of soil aggregates exhibited notable differences in distinct geomorphological types (Table 3). Except for Pinus Yunnanensis (upper-slope), the proportion of macroaggregates in non-karst areas was larger than that in karst areas, while the aggregates >5 mm in Elymus dahuricus (lower-slope) and Pennisetum alopecuroides (lower-slope) were significantly higher than those of Artemisia argyi- Setaria viridis (lower-slope) and Pennisetum alopecuroides (lower-slope) restored by vegetation. However, vegetation restoration failed to cause obvious effects on the gap in microaggregates.
The distribution of SOC content in three sample zones is shown in Figure 2. In non-karst area, SOC content of Pinus Yunnanensis (upper-slope) was significantly higher than that of other depths, while SOC content of Phyllostachys sulphurea (middle-slope) fluctuated in different depths. In comparison, the SOC content of Plantago asiatica (middle-slope) decreased gradually. In karst areas, the distributions of SOC of E. dahuricus (upper-slope), Pennisetum alopecuroides (lower-slope), and E. dahuricus (lower-slope) were more uniform, and SOC of Sporobolus fertilis (upper-slope) decreased gradually. After vegetation restoration, SOC content of S. viridis (upper-slope) increased gradually with the increased depth, whereas SOC content of Pennisetum alopecuroides (lower-slope) exhibited insignificant changes.
FIGURE 2. Content distribution characteristics of SOC in different bands. Capital letters represent significant differences between different depths in the same region, and lowercase letters represent significant differences between different regions at the same depth. PSY:Pinus Yunnanensis; PSA:Phyllostachys sulphurea; POA:Plantago asiatica; ESD:Elymus dahuricus; SSF:Sporobolus fertilis; PMA:Pennisetum alopecuroides; AAA:Artemisia argyi; SAV: Setaria viridis; AAA- SAV: Artemisia argyi- Setaria viridis; US: Upper-slope; MS: Middle-slope; LS: Lower-slope (Similarly hereinafter).
The generalized linear mixed model (GLMM) was performed in the non-normal analysis of SOC, as well as to evaluate geomorphology, spatial location, and their interactions (the dominant vegetation community as a random factor) in the conditions of spatial location, deep soil geomorphology, and the corresponding interaction in non-karst area, karst area, and vegetation-restored karst area (Table 4). We observed significant differences in SOC content between karst and non-karst topography (0–20 cm). There is a very significant difference in topsoil between karst vegetation restoration area and non-karst area, and the spatial location of deep soil has a significant effect on SOC content.
The distribution of soil ROC content in the three sample zones is shown in Figure 3. Soil ROC content in non-karst areas basically conformed to the law of decreasing with increased depth. Before vegetation restoration, S. fertilis (upper-slope) and Pennisetum alopecuroides (lower-slope) decreased with the increased soil depth in karst area, while the distribution of E. dahuricus (upper-slope) and E. dahuricus (lower-slope) showed the opposite observations. However, after vegetation restoration, the soil ROC content of A. argyi- S. viridis (lower-slope) increased at first and then decreased with depth. The soil ROC of S. viridis (upper-slope) decreased with the depth, while Pennisetum alopecuroides (lower-slope) showed a contrary trend.
The GLMM showed that topsoil geomorphology, spatial location, and their interaction had no significant effect on soil ROC content in karst and non-karst areas, while deep soil spatial location had significant effects on soil ROC content in karst and non-karst areas (Table 5). The interaction of topsoil geomorphology and spatial location between karst vegetation restoration area and non-karst area has a significant impact on soil ROC, while the deep soil spatial location has a very significant impact on soil ROC. The influence of upper-slope sample points on soil ROC in karst vegetation restoration area was significantly higher than that in non-karst area, and the geomorphology and spatial location of deep soil in karst area and karst vegetation restoration area had a significant influence on soil ROC content.
Figure 4 demonstrates the distribution of soil DOC content across three sample zones. The DOC content of the topsoil of Pinus Yunnanensis (upper-slope) in non-karst area was higher than that of other depths. Plantago asiatica (lower-slope) increased as the depth increased. The SOC content of E. dahuricus (upper-slope), S. fertilis (upper-slope), and Pennisetum alopecuroides (lower-slope) gradually decreased with the depth, while that of E. dahuricus (lower-slope) gradually increased. After vegetation restoration, the soil DOC content of A. argyi (upper-slope), S. viridis (upper-slope), and A. argyi- S. viridis (lower-slope) increased at first and then decreased, while that of Pennisetum alopecuroides (lower-slope) decreased at first and then increased.
The GLMM revealed that the spatial location of the soil profile in karst and non-karst areas has a major impact on the soil DOC content. Meanwhile, the interaction in the karst area (upper-slope) the effect of the interaction of geomorphology and spatial location on soil DOC content was also significantly higher than that of non-karst landforms. The spatial location and interaction of deep soil in karst vegetation restoration area and non-karst area have a significant impact on soil DOC, and the influence of non-karst landform (lower-slope) on soil DOC is greater than that of restored karst landform as shown in Table 6.
Figure 5 depicts the PCA analysis of the physical and chemical properties of topsoil based on three samples. The findings revealed that the chemical and physical characteristics of the soil surface layer were primarily driven by two principal components (PCs) in different regions, with a cumulative total variance of 96.087%.Among them, the PC1 spindle exhibited the greatest influence, explaining 87.291% of the data variation. PC2 spindle explained 8.796% of the data variation. The non-karst area is mainly located at the negative end of the PC1 and PC2 axis, and the karst area is mainly located at the positive end of the PC1 and PC2 axis. The karst vegetation restoration area had both positive and negative PC1 axis and negative PC2 axis. Physical and chemical properties differed significantly between non-karst and karst areas. However, soil physical and chemical properties were closer to non-karst areas with no significant differences after vegetation restoration.
FIGURE 5. Principal coordinate analysis of soil physical and chemical properties. PC1: major coordinates of the largest possible explain data changes; PC2: the rest of the degree of change in the proportion of the largest main coordinate components.
In this study, two matrix Spearman correlation analyses and average clustering (class average method) were used to examine the correlations between soil environmental factors and organic carbon (SOC, DOC, and ROC), as shown in Figure 6. In non-karst areas, ROC and TN, ROC and AP, pH and TP were respectively positively correlated. Cluster analysis could be divided into four groups: 1) pH; 2) SWC; 3) BD, EC, NH4+-N and TN; 4) SOC, DOC, ROC and NO3−-N, AP and TP were clustered together. For unrepaired karst areas, SWC was positively correlated with NH4+-N and negatively correlated with BD. Cluster analysis could be divided into three groups: 1) pH; 2) SWC, BD, EC, NH4+-N and TN; 3) SOC, DOC, ROC and NO3−-N, AP and TP. For karst restoration areas, there was a significant positive correlation between NH4+-N and NO3−-N and a significant negative correlation between TN and AP. Cluster analysis could be divided into three groups: 1) pH; 2) SWC, BD and EC; 3) SOC, DOC, ROC, NH4+-N, TN, NO3−-N, AP and TP.
FIGURE 6. Correlation analysis of soil physical and chemical properties by two matrices. *p < 0.1; **p < 0.05; ***p < 0.01.
The ecological environment of karst soils was more fragile than that in non-karst areas due to the limitations of the soil formation mechanism and its special three-dimensional structure above ground and underground (Xiong and Chi, 2015). The study showed that top soil organic carbon content in karst area was significantly lower than that in non-karst area, while deep soil organic carbon content was higher than that in non-karst area, SOC generally exhibits the spatial distribution characteristics of up-slope < down-slope. First, the top soil organic carbon content was related to the accumulation of litter and root biomass on the soil surface and SOC degradation. Soil surface accumulation of litter and root biomass were the main sources of SOC input (Long et al., 2022). The soil in karst area was limestone, and the weathered soil dissolves in weak acid, most of which was washed away by rainwater, The development of the soil parent material layer was slow, which was not conducive to plant growth, and the plant coverage was low (Wang et al., 2020). The soil in the non-karst area was yellow soil, and the soil parent material layer was well developed. In addition to herbs, there were a large number of shrubs and trees covered by vegetation in non-karst areas, and thick plant litter was accumulated on the soil surface. Higher plant coverage and species richness maintain a high level of root biomass, and the decomposition of surface vegetation litter, plant roots, and litter releases a large number of nutrient elements needed for plant growth. Accumulate a large amount of nutrients, such as organic matter and humus in the surface layer (Chen et al., 2022a). Secondly, calcareous soil was developed from carbonate rock, resulting in a weakly alkaline pH and a high dissolution rate for supplementing lost calcium and related SOC (Li et al., 2015). In comparison, yellow soil belongs to zonal soil (acidic pH) with large leached calcium and magnesium; acid hydrolysis removes the components rich in proteins, nucleic acids, and polysaccharides from soil organic matter, which makes soil organic matter low (Wang et al., 2013; Zhu et al., 2007). Bacteria, actinomycetes, and other microorganisms were extremely active in calcium-rich calcareous soil, causing organic matter to decompose to form humus and rapid turnover, so that the SOC content of yellow soil in deep soil was significantly lower than that of calcareous soil (He et al., 2019; Yang et al., 2019); In addition, serious soil erosion also leads to the loss of SOC, and the SOC lost on the upper-slope accumulates on the down-slope, so that the SOC on the downslope is larger than that on the upper-slope (Liu et al., 2020).
Some studies reported a significant positive correlation between soil ROC, DOC, and SOC contents in brick red soil environments (Huang et al., 2015; Guan and Fan, 2020). However, we observed a higher ROC content in the karst area than the non-karst area with extremely significant effects of geomorphology and spatial location on soil ROC. This was due to low soil content in karst landforms (rock was exposed and the rock fragment content was high) with high sensibility in response to the surrounding environments. Soil must maintain a high proportion of soil ROC and DOC in order to maintain the normal growth of vegetation (Huang et al., 2013). This was supported by the results of the GLMM model. In this study, the results show that in karst areas, the DOC/SOC proportion was much higher than in non-karst areas. Due to the significant positive correlation between the content of large soil aggregates and the content of iron and aluminum compounds in the soil, the content of large aggregates (>5 mm) in karst areas was substantially lower than that in non-karst areas, according to Table 3. (Xue et al., 2019). The increase in the adsorption of iron-aluminum compounds will affect the soil DOC/SOC ratio, and the two are inversely proportional (Ren et al., 2018). SOC in karst area was more active and easier to be leached and eroded by wind than that in non-karst area (Chen et al., 2022b), which indirectly explained the distribution characteristics of active organic carbon in karst area.
As an important measure to control soil erosion, vegetation restoration plays a vital role in the process of ecological restoration, which is also helpful to promote the development of eroded soil, improve soil quality and fertility (Liang et al., 2021). In this study, the vegetation types in karst area were mainly perennial herbaceous plants, while the roots of herbaceous plants were mainly concentrated in the soil surface layer. Thus, effects on SOC and TN were mainly concentrated in surface soil (Lei et al., 2021). Surface SOC in the karst vegetation restoration area was lower than that in the same spatial position in the karst unrepaired area, which might be attributed to the early stage of vegetation restoration in this area. Microbial activity was relatively strong, causing less SOC to accumulate due to its fixation in the vegetation (Xiao et al., 2022). Soil ROC in the restored area was lower than that in the karst area with an insignificant change in soil DOC, whereas surface soil ROC/SOC and DOC/SOC in the restored area were higher than those in the karst area. The proportion of surface soil aggregates (>5 mm) decreased significantly after vegetation restoration. The GLMM model showed that vegetation restoration had a significant effect on surface soil ROC. This indicated that rhizosphere activity weakened SOC stability and increased its activity despite vegetation restoration having significant carbon sequestration benefits (Zhang et al., 2019a; Hu et al., 2021b). In addition, we also found that the SOC content of the surface soil on the upper-slope was higher than on the lower-slope in both the karst area and the karst vegetation restoration area. Understandably, the erosion degree of surface runoff in the karst area was weaker than that in other areas, and the loss of SOC in surface runoff was relatively less. Coupled with specific karst landforms, the bare degree of rock on the upper-slope was higher than that on the lower-slope, suggesting a certain interception effect on SOC (Liang et al., 2018).
After vegetation restoration, the vegetation coverage was significantly improved. For instance, vegetation types developed from a single Gramineae to enriched Gramineae and Compositae. Soil surface TP increased and N:P decreased greatly after vegetation restoration (yet plant growth exhibited nitrogen limitation) (Li et al., 2015). Compositae plants with dicotyledons were more adaptable to environments, and Compositae plants spread rapidly with strong reproductive ability, which preempted the growth space of originally Gramineae and increased plant diversity (Jin et al., 2022; Gao et al., 2022). Studies reported that species diversity and richness could cause SOC sequestration by affecting soil microbial biomass and activity (Dawud et al., 2016; Hu et al., 2021a). However, here we showed that vegetation diversity was inversely proportional to soil surface carbon content, which might be related to the role of “plant-soil feedback (PSF)". Insufficient soil fertility in karst areas led to decreased soil microbial activity and failed to provide enough nutrition for plants. Strong competition occurred among plant populations in the condition of soil nutrients fixed or depleted, leading negative feedback from soil to plants. Current researches on the process and mechanism of “PSF”, especially at the community level, remain limited (Wang, 2020; Conrad et al., 2016). Further works for the corresponding knowledge were thus merited.
Vegetation restoration was regarded as an important measure for soil and water conservation. Here we investigated spatial patterns of SOC and its influencing factors in a karst area at the initial stage of vegetation restoration. Significant differences in SOC were found among different landforms. Surface SOC was substantially lower in karst areas than in non-karst areas, and ROC/SOC and DOC/SOC in surface soil were higher than that in non-karst area. Vegetation restoration changed soil active organic carbon pool and enhanced soil carbon sequestration capacity. However, SOC was unstable without upward trends due to the influence of soil stability and dominant plant communities in the early stage of vegetation restoration. Spatial location, plant richness, ROC/SOC and DOC/SOC in surface soil were larger than those in karst area attributing to influence of topography. Soil physical and chemical indexes such as soil activity, plant coverage, and soil bulk density were improved compared with those before restoration, which evidenced a significant improvement of vegetation restoration on soil quality in karst area.
The original contributions presented in the study are included in the article/supplementary material, further inquiries can be directed to the corresponding author.
YW conceived, designed, and conducted the experiment; RW. analyzed the data and wrote the manuscript; MZ, PH, and XT performed the experiments and sorted out data; YW and JZ. assisted in the modification. All authors have revised the manuscript and approved the version submitted.
This study was funded by the Project funding of Guizhou Provincial Science and Technology Projects (No. [2021] 099, QKHJC [2021]1421).
The authors declare that the research was conducted in the absence of any commercial or financial relationships that could be construed as a potential conflict of interest.
All claims expressed in this article are solely those of the authors and do not necessarily represent those of their affiliated organizations, or those of the publisher, the editors and the reviewers. Any product that may be evaluated in this article, or claim that may be made by its manufacturer, is not guaranteed or endorsed by the publisher.
BAI, Y. X., SHENG, M. Y., HU, Q. J., ZHAO, C., WU, J., and ZHANG, M. S. (2020). Effects of land use change on soil organic carbon and its components in karst rocky desertification of southwest China. Chinese Journal of Applied Ecology 31, 1607–1616. doi:10.13287/j.1001-9332.202005.016
BASTIN, J. F., FINEGOLD, Y., GARCIA, C., MOLLICONE, D., REZENDE, M., ROUTH, D., ZOHNER, C. M., and CROWTHER, T. W. (2019). The global tree restoration potential. Science 365, 76–79. doi:10.1126/science.aax0848
BLAIR, G., LEFROY, R., and LISLE, L. (1995). Soil carbon fractions based on their degree of oxidation, and the development of a carbon management index for agricultural systems. Australian Journal of Agricultural Research 46, 1459–1466. doi:10.1071/ar9951459
CHEN, M. L., ZHAO, Y. P., ZHANG, J. C., WANG, Y. H., MENG, M. J., LIU, X., LI, C., and XIE, D. J. (2022a). Soil Organic Carbon Characteristics of Different Forest Types in Fengyang Mountain. Journal of Northeast Forestry University 50, 69–75.
CHEN, M. L., CHEN, Z. Z., LEI, J. R., WU, T. T., and LI, Y. L. (2022b). Distribution characteristics of active organic carbon components in sediments of typical community types of mangrove wetland in Qinglan Port. Acta Ecologica Sinica 42, 4572–4581.
CONRAD, S., CHRISTIAN, R., MAREK, S., SASCHA, W., and SUSANNE, W. (2016). Digging deeper – how soil biota drive and respond to plant invasions: No evidence that plant–soil feedback effects of native and invasive plant species under glasshouse conditions are reflected in the field. Journal of Ecology 104.
CUI, L., SUN, H. M., DU, X. H., FENG, W. T., WANG, Y. G., ZHANG, J. C., and JIANG, J. (2021). Dynamics of labile soil organic carbon during the development of mangrove and salt marsh ecosystems. Ecological indicators 129, 107875. doi:10.1016/j.ecolind.2021.107875
DAWUD, S. M., RAULUND-RASMUSSEN, K., DOMISCH, T., FINéR, L., JAROSZEWICZ, B., and VESTERDAL, L. (2016). Is Tree Species Diversity or Species Identity the More Important Driver of Soil Carbon Stocks, C/N Ratio, and pH? Ecosystems 19, 645–660. doi:10.1007/s10021-016-9958-1
GAO, R., AI, N., LIU, G. Q., LIU, C. H., and QIANG, F. F. (2022). Characteristics of understory herb communities across time during restoration in coal mine reclamation areas and their coupling with soil properties. Acta Prataculturae Sinica 31, 61–68.
GERHARDT, K. E., GERWING, P. D., and GREENBERG, B. M. (2017). Opinion: Taking phytoremediation from proven technology to accepted practice. Plant Science 256, 170–185. doi:10.1016/j.plantsci.2016.11.016
GU, J., BOL, R., ZHANG, H. C., and WANG, Y. (2023). Controls on soil dissolved organic carbon along the 4000 km North-South forest transect in Eastern China. Catena 220, 106691. doi:10.1016/j.catena.2022.106691
GUAN, H. L., and FAN, J. W. (2020). Effects of vegetation restoration on soil quality in fragile karst ecosystems of southwest China. PeerJ 8, e9456. doi:10.7717/peerj.9456
GUO, Z. Y., WANG, Y. H., WAN, Z. M., ZUO, Y. J., HE, L. Y., LI, D., YUAN, F. H., WANG, N. N., LIU, J. Z., SONG, Y. Y., SONG, C. C., and XU, X. F. (2020). Soil dissolved organic carbon in terrestrial ecosystems: Global budget, spatial distribution and controls. Global ecology and biogeography 29, 2159–2175. doi:10.1111/geb.13186
HE, T. G., YU, Y. F., MENG, Y. C., SU, T. M., HU, J. M., DU, H., WANG, J., LI, Z. Y., ZHANG, Y., WEI, C. H., and FAN, S. (2019). Profile Distribution Characteristics of Organic Carbon and Nutrients in Different Degraded Lime Soils in Karst Area, Northwest Guangxi Province. Research of Soil and Water Conservation 26, 13–18.
HU, H. Y., ZHANG, Y. L., ZHU, Z. P., HUANG, C. F., WANG, S. Z., and ZHOU, C. F. (2021a). Changes in soil enzyme activity and microbial diversity at different vegetation restoration stages in eroded red soil. Chin J Appl Environ Biol 27, 734–741.
HU, P. L., LIU, S. J., YE, Y. Y., ZHANG, W., WANG, K. L., and SU, Y. R. (2018). Effects of environmental factors on soil organic carbon under natural or managed vegetation restoration. Land Degradation and Development 29, 387–397. doi:10.1002/ldr.2876
HU, P. L., XIAO, J., ZHANG, W., XIAO, L. M., YANG, R., XIAO, D., ZHAO, J., and WANG, K. L. (2020). Response of soil microbial communities to natural and managed vegetation restoration in a subtropical karst region. Catena 195, 104849. doi:10.1016/j.catena.2020.104849
HU, H. Y., FANG, H. Y., XIAO, S. S., DUAN, J., ZHANG, J., LIU, H. G., and TANG, C. J. (2021b). Soil carbon sink effect of main management models in typical granite erosion area of red soil in south China. Ecology and Environmental Sciences 30, 1617–1626.
HUANG, Q., WU, J. T., CHEN, J., WU, C., WANG, H., CHEN, W., and HE, Y. F. (2015). Adsorption of Dissolved Organic Carbon (DOC) on Soil: A Review. Soils 47, 446–452.
HUANG, X. F., WANG, S. J., and ZHOU, Y. C. (2018). Soil organic carbon change relating to the prevention and control of rocky desertification in Guizhou Province, SW China. Int. J. of Global Warming 15, 315. doi:10.1504/ijgw.2018.10014247
HUANG, Z. S., FU, Y. H., and YU, L. F. (2013). Characteristic evolution of soil organic carbon pool with the process of natural restoration of karst forest vegetation. Acta pedologica sinica 50, 306–314.
JIANG, Z. C., LIAN, Y. Q., and QIN, X. Q. (2014). Rocky desertification in Southwest China: Impacts, causes, and restoration. Earth-Science Reviews 132, 1–12. doi:10.1016/j.earscirev.2014.01.005
JIN, X. L., ZHAO, W., LI, M. D., LIU, D. D., and JU, W. L. (2022). Effects of Soil Moisture on the Stoichiometric Characteristics of Aboveground Plants Following Conversion of Farmland to Grassland on the Loess Plateau. Research of Soil and Water Conservation 29, 57–63.
LAN, X., DING, G. J., DAI, Q. H., and YAN, Y. J. (2022). Assessing the degree of soil erosion in karst mountainous areas by extenics. Catena 209, 105800. doi:10.1016/j.catena.2021.105800
LEI, S. Y., GUO, J. W., HE, L., HE, J., XU, X. M., LV, D., and ZHANG, X. P. (2021). Spatial Differentiation Characteristics of Grassland Restoration and Soil Physical Properties of Slope in Hilly-gully Region of Loess Plateau. Journal of Soil and Water Conservation 35, 251–258.
LI, X., ZENG, Q. C., AN, S. S., DONG, Y. H., and LI, Y. Y. (2015). Ecological Stoichiometric Characteristics in Leaf and Litter Under Different Vegetation Types of Zhifanggou Watershed on the Loess Plateau. Environmental Science 36, 1084–1091.
LIANG, Y. M., SU, Y. R., HE, X. Y., and CHEN, X. B. (2018). Distribution characteristics of soil nutrient and pH in rhizosphere versus non-rhizosphere from dominant shrub along different slope position in a karst region. Carsologica Sinica 37, 53–58.
LIANG, Y. P., LI, X., ZHA, T. G., and ZHANG, X. X. (2021). Vegetation Restoration Alleviated the Soil Surface Organic Carbon Redistribution in the Hillslope Scale on the Loess Plateau, China. Frontiers in Environmental Science 8. doi:10.3389/fenvs.2020.614761
LIU, R. H., PAN, Y. F., BAO, H., LIANG, S. C., JIANG, Y., TU, H. R., NONG, J. L., and HUANG, W. Q. (2020). Variations in Soil Physico-Chemical Properties along Slope Position Gradient in Secondary Vegetation of the Hilly Region, Guilin, Southwest China. Sustainability 12, 1303. doi:10.3390/su12041303
LONG, Q. X., LAN, J. C., and JIANG, Y. X. (2022). Effects of soil organic carbon fractions on aggregates under ecological restoration in rocky desertification region. Acta Ecologica Sinica 42, 7390–7402.
MA, T. S., DENG, X. W., CHEN, L., and XIANG, W. H. (2020). The soil properties and their effects on plant diversity in different degrees of rocky desertification. United States: Science of the Total Environment, 736.
NELSON, D. W., and SOMMERS, L. E. (1982). “Total carbon, organic carbon, and organic matter,” in Methods of Soil Analysis, Part 2.Chemical and Microbial Properties. Editors A.L. Page, R.H. Miller, and D.R. Keeney (Madison, Wisconsin: Agronomy Society of America), 539–552.
RABER, B., KöGEL-KNABNER, I., STEIN, C., and KLEM, D. (1998). Partitioning of polycyclic aromatic hydrocarbons to dissolved organic matter from different soils. Chemosphere 36, 79–97. doi:10.1016/s0045-6535(97)00352-4
REN, Y. B., Lü, M. K., and XIE, J. S. (2018). Effects of Dicranopteris dichotoma on soil dissolved organic carbon in severely eroded red soil. Acta Ecologica Sinica 38, 2288–2298.
RUBINO, M., DUNGAIT, J. A. J., EVERSHED, R. P., BERTOLINI, T., ANGELIS, P. D., D’ONOFRIO, A., LAGOMARSINO, A., LUBRITTO, C., MEROLA, A., TERRASI, F., and COTRUFO, M. F. (2010). Carbon input belowground is the major C flux contributing to leaf litter mass loss: Evidences from a 13 C labelled-leaf litter experiment. Soil Biology and Biochemistry 42, 1009–1016. doi:10.1016/j.soilbio.2010.02.018
SUN, C. L., WANG, Y. W., WANG, C. J., LI, Q. J., WU, Z. H., YUAN, D. S., and ZHANG, J. L. (2021). Effects of land use conversion on soil extracellular enzyme activity and its stoichiometric characteristics in karst mountainous areas. Acta Ecologica Sinica 41, 4140–4149.
WALKLEY, A., and BLACK, I. A. (1934). An Examination of the Degtjareff Method for Determining Soil Organic Matter, and a Proposed Modification of the Chromic Acid Titration Method. Soil Science 37, 29–38. doi:10.1097/00010694-193401000-00003
WANG, L. J., Wang, P., Sheng, M., and Tian, J. (2018). Ecological stoichiometry and environmental influencing factors of soil nutrients in the karst rocky desertification ecosystem, southwest China. Global ecology and conservation 16, e00449. doi:10.1016/j.gecco.2018.e00449
WANG, M. Y., SHI, X. Z., YU, D. S., XU, S. X., TAN, M. Z., SUN, W. X., and ZHAO, Y. C. (2013). Regional Differences in the Effect of Climate and Soil Texture on Soil Organic Carbon. Pedosphere 23, 799–807. doi:10.1016/s1002-0160(13)60071-5
WANG, R. Y., MATTOX, C. M., PHILLIPS, C. L., and KOWALEWSKI, A. R. (2022). Carbon Sequestration in Turfgrass-Soil Systems. Plants-Basel 11, 2478. doi:10.3390/plants11192478
WANG, S. J. (2020). Key ecological issues in plant-soil feedback: pattern, process and mechanism. Journal of Nanjing Forestry University (Natural Sciences Edition) 44, 1–9.
WANG, X. F., HUANG, X. F., HU, J. W., and ZHANG, Z. M. (2020). Relationship Among Soil Organic Carbon and Small Environment and Lithology in the Rocky Desertification Process in Different Karst Landforms. ournal of Soil and Water Conservation 34, 295–303.
WU, H., ZHANG, J. L., FAN, Y. W., YU, L. F., YAN, L. B., and YUAN, C. J. (2013). Numerical classification and ordination of forest communities in Caohai basin. J. Nanjing For. Univ. (Nat. Sci. Ed.) 37, 47–52.
WU, J. Q., MA, W. W., LI, G., ALHASSAN, A.-R. M., WANG, H. Y., and CHEN, G. P. (2020). Vegetation degradation along water gradient leads to soil active organic carbon loss in Gahai wetland. Ecological Engineering 145, 105666. doi:10.1016/j.ecoleng.2019.105666
WU, Y. J., TIAN, X., ZHANG, M. Y., WANG, R. Z., and WANG, S. (2022a). A Case Study of Initial Vegetation Restoration Affecting the Occurrence Characteristics of Phosphorus in Karst Geomorphology in Southwest China. Sustainability 14, 12277. doi:10.3390/su141912277
WU, Y. J., TIAN, X., ZHANG, M. Y., and WANG, S. (2022b). Influence of vegetation restoration on carbon in the lakeside zone of Karst Wetland in Guizhou Plateau. Acta Ecologica Sinica 42, 7785–7795.
XIAO, S. S., FANG, H. Y., XU, J. W., CHANG, M. Q., QIN, X. L., PENG, W. J., LIU, H. G., and SHEN, F. X. (2022). Advances of the Soil Organic Carbon Stability Under Vegetation Restoration in Eroded Area. Journal of Soil and Water Conservation 36, 1–8.
XIONG, K. N., and CHI, Y. K. (2015). The Problems in Southern China Karst Ecosystem in Southern of China and Its Countermeasures. Ecological Economy 31, 23–30.
XUE, B., HUANG, L., HUANG, Y. A., YIN, Z. Y., LI, X. K., and LU, J. W. (2019). Effects of organic carbon and iron oxides on soil aggregate stability under different tillage systems in a rice–rape cropping system. Catena 177, 1–12. doi:10.1016/j.catena.2019.01.035
YADAV, K. K., GUPTA, N., KUMAR, A., REECE, L. M., SINGH, N., REZANIA, S., and KHAN, S. A. (2018). Mechanistic understanding and holistic approach of phytoremediation: A review on application and future prospects. Ecological Engineering 120, 274–298. doi:10.1016/j.ecoleng.2018.05.039
YANG, H., PRELOVSEIC, M., HUANG, F., ZHANG, C. L., CAO, J. H., and NATAŠA, R. (2019). Quantification and evaluation of soil organic carbon and its fractions: case study from the classical karst, sw slovenia. Acta Carsologica 48, 295–311. doi:10.3986/ac.v48i3.7305
YANG, P. P., LI, R., GU, Z. K., QIN, L., SONG, T., LIU, Z. X., GAO, J. Y., and YUAN, J. (2022). Runoff sediment characteristics affected by erosive rainfall patterns in a small watershed in karst areas of southwest China. Catena 219, 106591. doi:10.1016/j.catena.2022.106591
YUAN, D. X. (2001). Comparison of global karst ecosystems: scientific objectives and implementation plans. Advances in Earth Science 2001, 461–466.
ZHANG, H. L., DENG, Q., HUI, D. F., WU, J. P., XIONG, X., ZHAO, J. Q., ZHAO, M. D., CHU, G. W., ZHOU, G. Y., and ZHANG, D. Q. (2019a). Recovery in soil carbon stock but reduction in carbon stabilization after 56-year forest restoration in degraded tropical lands. Forest Ecology and Management 441, 1–8. doi:10.1016/j.foreco.2019.03.037
ZHANG, J. L., WU, H., YU, L. F., YAN, L. B., YUAN, C. J., and CAI, G. J. (2013). Grass sea wetland basin is a typical karst forest community α, β diversity analysis. For. Sci. Technol 2013, 7–11.
ZHANG, H. L., XU, X. L., LI, Z. W., LIU, M. X., XU, C. H., ZHANG, R. F., and LUO, W. (2019b). Effects of vegetation restoration on soil quality in degraded karst landscapes of southwest China. United States: Science of the Total Environment, 650.
ZHANG, H. L., WANG, S. J., LI, J. H., CAO, R., CHEN, M. K., and LI, S. H. (2019c). Response of soil readily oxidizable carbon to community succession of Xishuangbanna tropical forests. Acta Ecologica Sinica 39, 6257–6263.
ZHAO, Y. L., LI, X. B., and ZHANG, Y. (2017). Technology and application of mountainous area divisions in Qian-Gui Karst areas. Journal of Geo-information Science 19, 934–940.
ZHOU, L. G., WANG, X. D., WANG, Z. Y., ZHANG, X. M., CHEN, C., and LIU, H. F. (2020). The challenge of soil loss control and vegetation restoration in the karst area of southwestern China. International Soil and Water Conservation Research 8, 26–34. doi:10.1016/j.iswcr.2019.12.001
Keywords: vegetation restoration, karst, soil organic carbon, succession, vegetation community
Citation: Wu Y, Wang R, Zhang M, He P, Wu Y, Tian X and Zhang J (2023) Spatial patterns and influencing factors of soil SOC、DOC、ROC at initial stage of vegetation restoration in a karst area. Front. Environ. Sci. 11:1099942. doi: 10.3389/fenvs.2023.1099942
Received: 16 November 2022; Accepted: 30 January 2023;
Published: 09 February 2023.
Edited by:
Lishan Ran, The University of Hong Kong, SAR ChinaReviewed by:
Binghui He, Southwest University, ChinaCopyright © 2023 Wu, Wang, Zhang, He, Wu, Tian and Zhang. This is an open-access article distributed under the terms of the Creative Commons Attribution License (CC BY). The use, distribution or reproduction in other forums is permitted, provided the original author(s) and the copyright owner(s) are credited and that the original publication in this journal is cited, in accordance with accepted academic practice. No use, distribution or reproduction is permitted which does not comply with these terms.
*Correspondence: Yunjie Wu, d3lqenhjdmJuQDE2My5jb20=
Disclaimer: All claims expressed in this article are solely those of the authors and do not necessarily represent those of their affiliated organizations, or those of the publisher, the editors and the reviewers. Any product that may be evaluated in this article or claim that may be made by its manufacturer is not guaranteed or endorsed by the publisher.
Research integrity at Frontiers
Learn more about the work of our research integrity team to safeguard the quality of each article we publish.