- 1Institute of Hydrobiology, Biology Centre of the Czech Academy of Sciences, České Budějovice, Czechia
- 2Faculty of Science, University of South Bohemia, České Budějovice, Czechia
- 3Department of Water Management Planning, Vltava River Authority, State Enterprise, Plzeň, Czechia
- 4Faculty of Fisheries and Protection of Waters, South Bohemian Research Center of Aquaculture and Biodiversity of Hydrocenoses, Vodňany, Czechia
- 5Department of Zoology and Fisheries, Faculty of Agrobiology, Food and Natural Resources, Czech University of Life Sciences, Prague, Czech Republic
- 6Nature Conservation Agency of the Czech Republic, Prague, Czech Republic
Most lotic ecosystems have been heavily modified in recent centuries to serve human needs, for example, by building dams to form reservoirs. However, reservoirs have major impacts on freshwater ecosystem functions and severely affect rheophilic fishes. The aim of this review is to gather evidence that aside from direct habitat size reductions due to reservoir construction, competition for food and space and predation from generalist fishes affect rheophilic community compositions in tributaries (river/stream not directly affected by water retention). River fragmentation by reservoirs enables the establishment of generalist species in altered river sections. The settlement of generalist species, which proliferate in reservoirs and replace most of the native fish species formerly present in pristine river, may cause further diversity loss in tributaries. Generalist migrations in tributaries, spanning from tens of metres to kilometres, affect fish communities that have not been directly impacted by reservoir construction. This causes “edge effects” where two distinct fish communities meet. Such interactions temporarily or permanently reduce the effective sizes of available habitats for many native specialized rheophilic fish species. We identified gaps that need to be considered to understand the mechanistic functioning of distinct fauna at habitat edges. We call for detailed temporal telemetry and trophic interaction studies to clarify the mechanisms that drive community changes upstream of reservoirs. Finally, we demonstrate how such knowledge may be used in conservation to protect the remnants of rheophilic fish populations.
1 Introduction
Riverine ecosystems host much of the world’s biodiversity (Dudgeon et al., 2006; Reid et al., 2019), yet they have been so significantly modified to meet human needs that few free-flowing rivers remain (Grill et al., 2019; Belletti et al., 2020). Rivers have always been considered as essential sources of human wellbeing, which provide food and water for domestic and agricultural use, colonization pathways and transportation corridors, as well as being recognized as sources of energy generation, which range from simple water wheels to sophisticated hydropower plants (Grill et al., 2019). With the increasing demands of growing human populations, rivers are being regulated, impounded, and harnessed to meet electricity, irrigation, water supply, and flood management needs (Nilsson et al., 2005; Lehner et al., 2011; Reid et al., 2019). In addition to their primary purposes, artificial structures such as flumes, sluices, weirs and dams interfere with the natural processes of entire ecosystems. Such impoundments cause river fragmentation, flow regime modifications, sediment deposition, nutrient cycle alterations, and temperature changes, which lead to rapid declines in biodiversity (Belmar et al., 2010; Lehner et al., 2011; Feng et al., 2018; Grill et al., 2019; Jumani et al., 2019). Due to the overall complexity of the impacts of fragmentation on aquatic biodiversity that are caused by damming, the long-term scale of these impacts, and the time lags between causes and consequences, the changes in river functioning are likely underestimated (Fuller et al., 2015; Belletti et al., 2020; Arboleya et al., 2021).
Habitat fragmentation is a paramount topic in biodiversity conservation. One of the most important concepts is called “edge effects”, which in a broad context can be described as the changes in resource availability, physical and biological conditions at ecosystem boundaries or within adjacent ecosystems (Fischer and Lindenmayer, 2007). Its biotic component, on which we focus in this review, includes interactions among generalists occupying human-altered environments and specialized species present in the remaining pristine fragments (Andren and Angelstam, 1988). This primarily terrestrial concept has the potential to be more widely used in fish conservation due to similarities in the interactions among generalist and rheophilic fish fauna living side by side (Tamario et al., 2021). In particular, we review here evidence that reservoir construction may induce similar biotic edge effects between generalists and rheophilic fishes in interconnected rivers and streams (Figure 1), ranging from tens of meters to kilometers depending on the particular system and species.
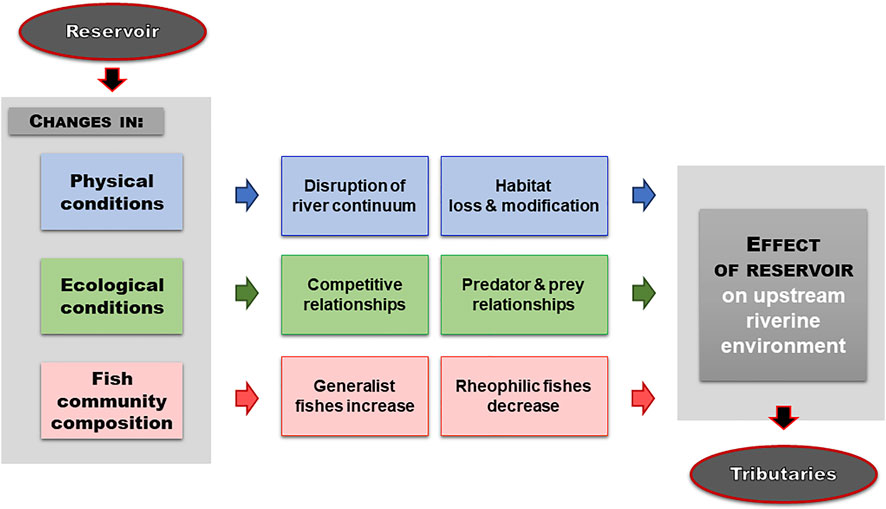
FIGURE 1. Conceptual scheme of synergistic negative effects of reservoir construction on rheophilic fishes in tributaries (river/stream not directly affected by the water retention function of a reservoir). While rheophilic fishes face a range of stressors varying from change in physical conditions to community change, this review focuses on biotic interactions that are modified by reservoir construction (green and pink color). Due to the proliferation of generalists in impounded sections of reservoirs, competitive interactions (e.g., food availability, spawning habitat, spatial niche) and predator-prey relationships (increased mortality of rheophilic fish in various life stages) are compromised, leading to community change in tributaries and negatively impacting rheophilic fishes. Please see main text for details and examples of interactions.
Fish communities along river continua have evolved and are maintained by a series of biotic and continuous longitudinal gradients of abiotic variables through processes known as environmental filtering (Jackson et al., 2001; Kraft et al., 2015), which are reflected by the zonation patterns of fish communities (Aarts and Nienhuis, 2003; Buisson et al., 2008; Troia and Gido, 2014). Increasing riverbed steepness and water discharges and decreasing trophic status, conductivity, and temperature constitute natural barriers to dispersal for generalists and their proliferation in upstream lotic environments inhabited by potamodromous rheophilic species (Vannote et al., 1980; Johnson et al., 1995; Troia and Gido, 2014). These natural processes are seriously damaged by reservoir construction, and the impacts of reservoirs have been monitored with special attention paid to altered habitats below reservoir and newly established fish communities within reservoirs (Bain et al., 1988; Kubečka, 1993; Říha et al., 2009; Ganassin et al., 2021) and to river function alterations downstream and between reservoirs (Preece and Jones, 2002; Perkin et al., 2015; Bartoň et al., 2022). A significant scientific effort has been aimed to discover the effects of limited gene flow due to migration constraints between isolated sites and the way how construction of fish ladders could avert these effects (Roscoe and Hinch, 2010; Kemp, 2016; Tamario et al., 2019; Sun et al., 2022). However, the contact of two distinct fauna that occupy lentic reservoirs and their fluvial tributaries is to the authors’ best knowledge not well covered.
Although edge effects are a critical issue in the global declines of native rheophilic fish species populations in fragmented rivers (Limburg and Waldman, 2009; Deinet et al., 2020), the effect of reservoir fish fauna (which often predominantly consist of locally non-native species) on rheophilic fish has received relatively little attention. To date, most studies have evaluated biodiversity and trait-based metrics in relation to reservoir occurrences and overall river fragmentation and have provided useful insights into the biodiversity declines and functional changes in impounded rivers (Degerman et al., 2007; Wang et al., 2011; Esselman et al., 2013; Van Looy et al., 2014; Cooper et al., 2017; Turgeon et al., 2019). To much less extent were studied generalist fish migrations from reservoirs to riverine sections on habitat edges, which can further degrade river status (Andren and Angelstam, 1988; Tamario et al., 2021). While some fish community changes in rivers can be attributed to abiotic changes in ecosystems, others are likely related to biotic interactions among unnaturally close lentic and lotic fish fauna (Pringle et al., 2000; Jackson et al., 2001).
The aim of this review is to address the topic of edge effects of lentic fish fauna that are composed of generalists in reservoirs and their competitive and predation effects on rheophilic fish communities. We summarize the current state of knowledge, from reservoir settlement of generalist fish species, which is often driven by intentional or unintentional fish transport, to dynamic migrations of generalists into reservoir tributaries. Most importantly, we suggest that predation and the competitive interactions among lotic and lentic fauna are among the causes of the declining biotic integrity of reservoir tributaries. Finally, we show that it is possible to protect riverine communities by intentional fragmentation, where the conservation benefits of such measures exceed their negative impacts. We focus our attention on the impacts of generalist fishes that may come from three sources: 1) local fish species that proliferate after reservoir construction in impounded sections, 2) locally non-native species (species native in the region that were not formerly present in local communities) and 3) regionally non-native species (species not native to the focal region). We mainly focused our attention on the impacts on potamodromous rheophilic fish species, but when we did not find a good example of biotic interactions due to edge effects, we used examples of anadromous rheophilic fish species, which are better studied due to their commercial importance. We have reviewed literature and identified examples of such interactions ranging from temperate to tropical climates (Figure 2). We aim to draw attention to this topic, which is not yet explored in full, since it may help in conservation efforts aimed to preserve declining rheophilic fish species located upstream of reservoirs.
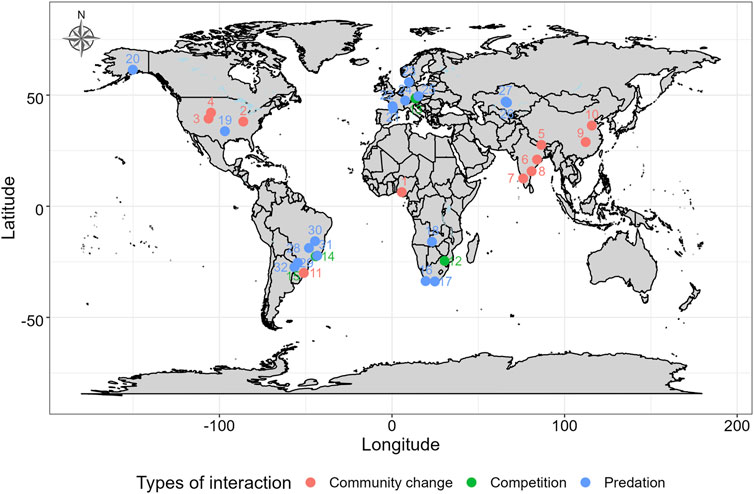
FIGURE 2. Map of evidence for interactions between rheophilic fishes and generalist fishes compiled by the study. The numbers in the map correspond to numerical order of case studies and their supporting references in Table 1.
2 Proliferation of generalists in reservoirs
Dams create lentic or slow-flowing environments that are generally much deeper than the original river channels (Figure 3). Rheophilic fish are able to survive in such environments until they are occupied by other species (Irz et al., 2002; Lenhardt et al., 2009; Knott et al., 2021). However, on a longer time scale, the proportions of rheophilic fish in impounded environments generally become low, and they are replaced by quickly proliferating generalists (Figures 3, 4; Gido et al., 2009; Clavero and Hermoso 2010).
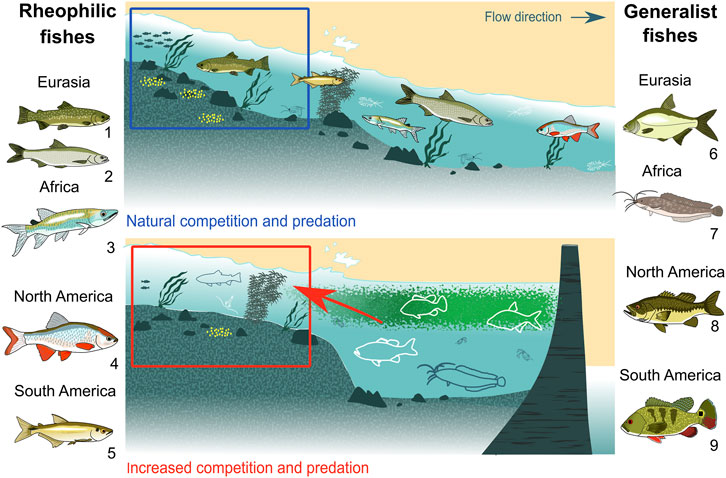
FIGURE 3. Schematic representation of the community changes before (blue rectangle) and after reservoir construction (red rectangle) and the biotic interactions among two distinct faunas of generalist fishes and rheophilic fishes in the tributary at the habitat edge. Rectangle indicates river section that is not directly influenced by increase of water level, yet the community changes due to reservoir construction. The green dots in lower panel indicate shift from littoral primary production to pelagic production and examples of invertebrates indicate the change in the major prey items for omnivorous fish within reservoir driving settlement of generalist fishes. Red arrow indicates enhanced interaction between generalist fishes in reservoir and rheophilic fishes in the tributary. Only subset of fish species reviewed is depicted to maintain clarity—rheophilic fish examples: 1—Salmo trutta, Salmonidae; 2—Leuciscus aspius, Leuciscidae; 3—Hepsetus odoe, Characidae; 4—Cyprinella lutrensis, Leuciscidae; 5—Oligosarcus hepsetus, Characidae; typical generalists: 6—Abramis brama, Cyprinidae; 7—Clarias sp. Clariidae; 8—Micropterus salmoides, Centrarchidae; 9—Cichla ocellaris, Cichlidae. Drawn by Zuzana Sajdlová.
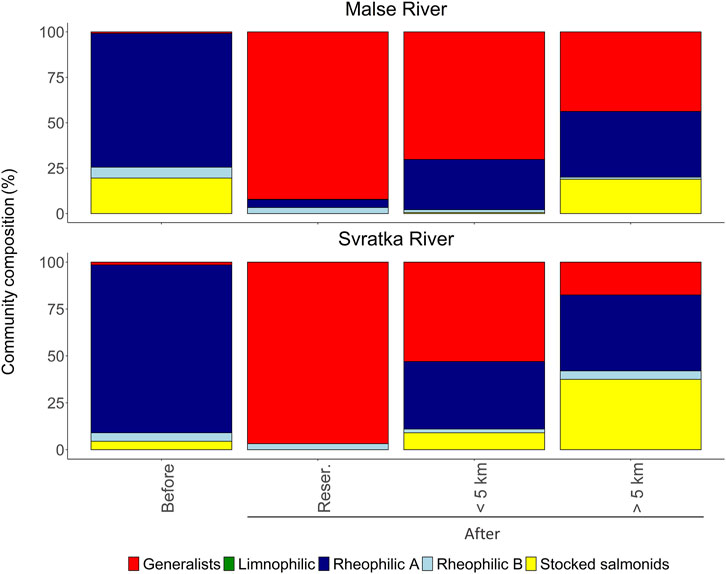
FIGURE 4. Changes in fish guild compositions after the construction of reservoirs in two rivers in the Czech Republic. Four fish faunas are considered: Former river community (Before), reservoir (Reser.), river locations less than 5 km upstream of the reservoirs (<5 km) and more than 5 km upstream of the reservoirs (>5 km). Stocked salmonids refer to brown trout (Salmo trutta) and grayling (Thymallus thymallus) that are artificially supported by fisheries (according to (Peňáz et al., 1968; Hladík et al., 2008)).
The community change has been described e.g. in mainland Europe: a typical reservoir fish community is dominated by bream (Abramis brama), roach (Rutilus rutilus), and bleak (Alburnus alburnus) in the final stage of community succession, although many reservoirs were constructed in rheophile-dominated community zones (Kubečka, 1993; Říha et al., 2009). Two rheophilic fish groups have been classified in Europe: More specialized rheophiles A (e.g., Barbus barbus, Chondrostoma nasus, Leuciscus leuciscus, Squalius cephalus, Alburnoides bipunctaus, and Vimba vimba), which require river conditions at all stages of their life histories, and less specialized rheophiles B (e.g., L. aspius, L. idus, Pelecus cultratus, Gobio gobio, Lota lota, and Cobitis taenia), which are well adapted to lentic conditions during part of the ontogeny (Schiemer and Waidbacher, 1992). This classification appears to be valid in most systems outside Europe, and the rheophiles A formerly abundant before dam construction move upstream from lentic parts of reservoir (Agostinho et al., 1999). Adult rheophiles B can inhabit suitable reservoir habitats but usually become less abundant when reservoirs are occupied by other species. All rheophilic species require flowing water for reproduction, and their early stages have higher dissolved oxygen requirements than generalist and limnophilic species (Balon, 1975). The need for specific water flows and the risk of being displaced from suitable habitats by flow fluctuations (Bartoň et al., 2021; Bartoň et al., 2022) represent a clear disadvantage of rheophilic fishes compared to generalists, which are extremely flexible in their spawning substrate choices and do not rely on narrow ranges of optimal conditions to provide spawning sites (Hladík and Kubečka, 2004).
One of the main factors favoring the replacement of rheophilic fish by generalists in reservoirs is changes in food resources. While the main autochthonous primary production in rivers comes from benthic systems, primary production in reservoirs is mostly pelagic (Ward and Stanford, 1995). Littoral habitats with benthic food chains may be the most productive per unit area, but due to large pelagic spatial proportions of reservoirs, most production occurs in pelagic areas (Fernando, 1994; Moraes et al., 2021). The bulk of production in reservoirs goes from phytoplankton to zooplankton. For rheophilic species, benthic macroinvertebrates and algae are the main autochthonous food sources (Vannote et al., 1980; Aarts and Nienhuis, 2003; Bešta et al., 2015). These resources are generally limited in reservoirs (Agostinho et al., 1999; Moraes et al., 2021) because productive benthic habitats are limited and the benthos are also heavily used by omnivores (Agostinho et al., 1999; Schleuter and Eckmann, 2008). Another important food source for rheophilic fishes is terrestrial insects, which are generally rarely available in reservoirs and are also preyed upon by generalists (Mehner et al., 2005; Vašek et al., 2008). Although specialized, truly planktivorous fishes are often found in small numbers in reservoirs (Fernando and Holčík, 1991), while omnivorous generalists such as cyprinids, characids, percids, and centrarchids are well equipped to prey on zooplankton, which leads to zooplankton size reduction (Seďa and Kubečka, 1997; Hülsmann et al., 1999; Quintana et al., 2015). The main adaptations of omnivorous generalists for zooplankton feeding are dense branchial sieves (Van Den Berg et al., 1994) and sinusoidal swimming to detect dense aggregations of zooplankton (Jarolím et al., 2010). Rheophilic species have no such adaptations and thus cannot compete efficiently for the main food sources in reservoirs. Although rheophiles B may survive as predators or specialized benthivores, rheophiles A slowly disappear from these sites: when the barbel (B. barbus) was locked in the large newly filled Orava Reservoir (Slovakia), its growth rate decreased significantly despite a trophic upsurge in the newly flooded reservoir (Havlena, 1964). Additionally, study conducted in the Medjuvrsje Reservoir (Serbia) shows that the decline of rheophilic fishes gradually increases with reservoir aging (Lenhardt et al., 2009), and the increasing domination of generalists may have further negative impact on the tributaries.
3 Human-driven translocation of lowland species to upstream man-made habitats
Reservoirs often serve as stepping stones for non-native generalists, which facilitates generalists dispersal across the landscape (Havel et al., 2005; Johnson et al., 2008; Liew et al., 2016; Silva-Sene et al., 2022) and thus reservoir communities have usually high similarity with each other, despite their presence in relatively distant watersheds with distinct riverine fish communities. Therefore, reservoirs are one of the sources of biotic homogenization in river ecosystems, which is characterized by a loss of biological uniqueness (Olden and Rooney, 2006; Poff et al., 2007; Clavero and Hermoso, 2010). An example of such homogenization is introduction of Cichla spp. Into reservoirs of Upper Paraná river basin and other Brazilian regions resulting in the biotic homogenizations of native fish assemblages (Franco et al., 2021). This genus has been widely introduced within and outside the Neotropical region and its introduction are mostly occurring in presence of reservoirs (Franco et al., 2022a; Franco et al., 2022b).
In upper-river drainage areas, the colonization mechanisms of newly established reservoirs by non-native generalists act more slowly in the absence of fish transport (Olden et al., 2004). Considering non-human driven reservoir colonization, opportunities would often be limited to external or internal transport of fish eggs by birds, which is a process whose frequency is still unknown in the context of fish dispersal mechanisms (Hirsch et al., 2018; Silva et al., 2019; Lovas-Kiss et al., 2020) and extreme flood events (Taylor and Cooke, 2012). Therefore, the organized breeding and translocation of angler-preferred generalists into novel environments poses a serious threat to native fish communities (Copp et al., 2009; Volta et al., 2013; Early et al., 2016). In addition, many small-sized generalists are introduced accidentally with economically important stocking materials (Lusk et al., 2010) and through the release of ornamental organisms (Patoka et al., 2017) or live baitfish (Drake and Mandrak, 2014). Since these impacts are directly driven by human activities, areas with higher human population densities are more vulnerable to introductions of non-native species (Dawson et al., 2017).
4 Timing of fish migrations from reservoirs to tributaries
Animal migrations, including those of fish, are characterized as regular movements between environments (Northcote, 1984), which are influenced by individual characteristics (Chapman et al., 2011; Skov et al., 2011; Harrison et al., 2015) and can be decomposed into one-way migration events (Lucas and Baras, 2001). In general, fish migrations between lentic and lotic habitats, e.g., between lakes and their tributaries, are species-specific and depend on the food availability in lakes and their tributaries, as well as trade-offs such as predation risk or reproduction (Brönmark et al., 2008; Brodersen et al., 2014; Šmejkal et al., 2018). Some of the generalists that thrive in impounded habitats actively migrate over long distances into tributaries, such as European bream (Hladík and Kubečka, 2003; Pfauserová et al., 2021) or bleak (Šmejkal et al., 2017; Šmejkal et al., 2018) and interacting strongly with rheophilic brown trout (Salmo trutta) and asp (Leuciscus aspius), respectively. Thus, fauna that interact at the edges of habitats can affect fish communities located hundreds of meters to more than one hundred kilometers upstream of impounded reaches (Perkin and Gido, 2011; Pfauserová et al., 2021; Tamario et al., 2021).
Reproductive migrations appear to be the main reason that cause fish to temporarily return from reservoirs to tributaries. In many species, spawning areas and spawning grounds are well defined and persist for many decades (Arnold et al., 2019), For example, lithophils that spawn on gravel and phytophils that spawn on macrophytes or flooded vegetation (Balon, 1975) migrate to tributaries when these substrates are limited in reservoirs (Hladík and Kubečka, 2003). In the case of European fish, spring migrations of rheophilic fish from reservoirs into tributaries are regularly recorded, with lithophilous asp and chub (Squalius cephalus) searching for gravel substrata (Benitez, et al., 2015; Pfauserová et al., 2019; Pfauserová et al., 2021; Šmejkal et al., 2021). Tributaries may also be used by reproducing generalists such as bream that are not strictly dependent on a single spawning substrate and use a wide range of available substrates (Hladík and Kubečka, 2004; Říha et al., 2013) and by phytophilous pike (Esox lucius) searching for submerged vegetation (Sandlund et al., 2016).
Reservoirs contain relatively high abundances of fish, with many generalist species, such as bream and roach, which are food-limited and fail to reach their maximum size due to intra- and interspecific competition and a lack of optimal food sources (benthos) (Šmejkal et al., 2015; Žák et al., 2020). As tributary temperatures drop, food resources become scarcer in the tributary, while predation risks increase (Harvey and Nakamoto, 2013). For this reason, most generalist species occupy tributaries in productive period of the year and return to reservoirs for overwintering (Pfauserová et al., 2021; Pfauserová et al., 2022). Although the mechanisms driving fish migrations from reservoirs to tributaries are not completely understood, it appears that the motivations may be similar to fish migrations from lakes to tributaries, where reproduction plays an important role (Kristiansen and Dølving, 1996; Baril and Magnan, 2002), as well as the predator-prey relationships (Skov et al., 2011).
The migratory activities between shallow lakes and their tributaries and the high species abundances in shallow lake tributaries in winter differ significantly from the dynamics of cyprinid migration between deeper canyon-shaped reservoirs and tributaries, where generalist fishes in tributaries peak in spring and summer and return to reservoirs in winter (Hladík et al., 2008; Pfauserová et al., 2021). For example, common bream, silver bream (Blicca bjoerkna) and roach migrate to small tributaries for overwintering to evade predation when the food availability in lakes is low and predation risks in lakes are relatively higher compared to tributaries (Skov et al., 2008; Skov et al., 2011). Whether the available depths in reservoirs are essential for fish decisions regarding where to overwinter (and thus affecting interactions with rheophilic fish) remains to be investigated.
5 Predation of rheophilic species by generalists in tributaries
One of the key factors that affects fish community structures in freshwater ecosystems is predation (Persson, 1997; Jackson et al., 2001; Temming et al., 2007). Generalist predators that thrive in the human-made lentic parts of river systems interfere with lotic waters, although the magnitude of the edge effects is unknown. In altered ecosystems, adjacent lotic river fauna may be heavily preyed upon (Table 1; Jepsen et al., 2000; Tamario et al., 2021). Introduction of predatory fish can lead to extinction of local species, destruction of unique communities, and severe loss of diversity (Chapleau et al., 1997; Hermoso et al., 2011; Pelicice et al., 2015). For example, the preys for introduced pike consist of native juvenile salmonids or other native rheophilic species where salmonids are less abundant (Sepulveda et al., 2013). Another effect observed in North America is the difference between unfragmented and fragmented streams, where in unfragmented streams more rheophilic specialists persist, and fragmented streams are significantly more dominated by generalist predatory species (Guenther and Spacie, 2006). Reservoir construction on the Laramie River, Wyoming, also caused higher abundances of non-native generalist predatory fish and led to extirpation and population reductions of selected rheophilic fishes (Quist et al., 2005). The authors of this study did not determine the specific mechanisms for the changes in the fish communities upstream of the reservoir, but they expected that non-native predators were the principal cause of community change (Quist et al., 2005). The introduction of peacock bass (Cichla ocellaris) in South America caused considerable negative impacts on fish assemblage composition of upper stream riverine native small-sized fish through predation (Franco et al., 2021). Another example of the influence of generalists on fish species occupying reservoir tributaries is the disappearance of red shiner (Cyprinella lutrensis) from streams directly connected to Lake Texoma, United States, with predation by centrarchids as a likely contributing mechanism (Matthews and Marsh-Matthews, 2011). Similarly, generalist predators threatened native rheophilic fish fauna after the construction of the Kenney Reservoir, USA (Martinez et al., 1994), although a detailed investigation of the modified interactions between species is missing in this study.
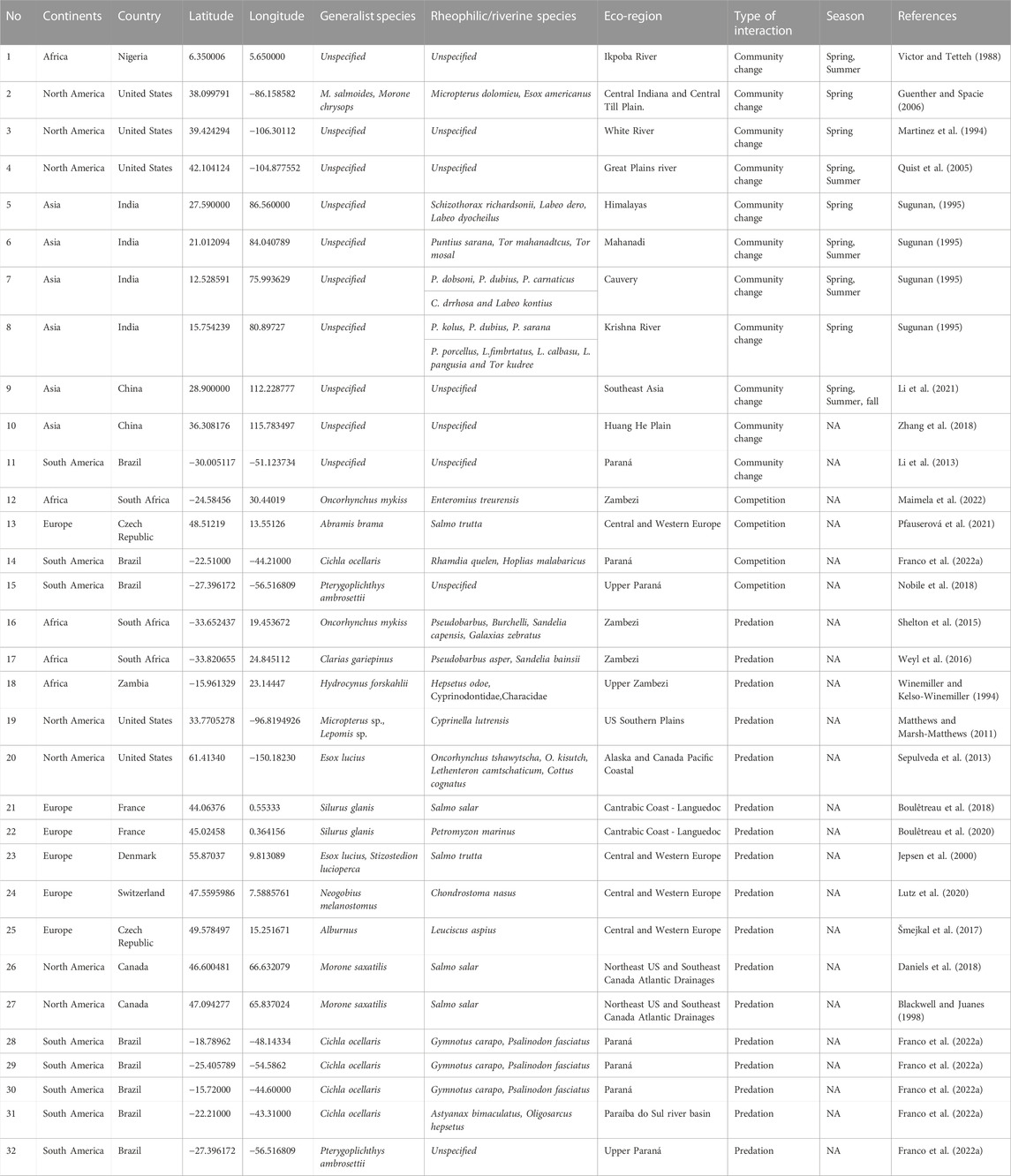
TABLE 1. Examples of field-based evidence of the biotic interactions among generalist fish fauna of reservoir species and rheophilic fish with negative impacts on the remaining rheophilic fish populations based in reservoir tributaries. Number (No) indicates the geographical position of the study in the map (Figure 2).
A notable example in this regard is the largest European freshwater fish, the European catfish (Silurus glanis), which is now regionally non-native in portions of European freshwaters (Copp et al., 2009). Catfish are typically introduced to reservoirs and spread into higher-order rivers (Gago et al., 2016); in some instances, they change fish communities during invasion (Guillerault et al., 2015). Catfish cause high mortality in the critically endangered allis shad (Alosa alosa) during spawning in one of Europe’s most important spawning areas (e.g., Garonne River, Southwest France) (Boulêtreau et al., 2021) but also in the endangered sea lamprey (Petromyzon marinus) (Boulêtreau et al., 2020), Atlantic salmon (Salmo salar) (Boulêtreau et al., 2018) or vimba bream (Vimba vimba), European nase (Chondrostoma nasus) and barbel (Barbus barbus) (Lyach, 2021). Predation on native fishes by African catfish (Clarias gariepinus) caused a drastic reduction in the abundance of endemic and endangered Pseudobarbus asper and Sandelia bainsii in South Africa impoundments (Weyl et al., 2016).
Migratory success or mortality at early life stages can strongly influence population sizes (Larsson, 1985). Most rheophilic fish species must migrate downstream or upstream once or regularly during their lives (Lucas and Baras, 2001). As a result, they inevitably pass through altered sections of rivers with lentic waters where the risk of predation is high (Jepsen et al., 1998; Olsson et al., 2001). An example of how environmental alterations can increase mortality is the massive predation of Atlantic salmon smolts by striped bass (Morone saxatilis) as they pass through reservoirs (Table 1; Blackwell and Juanes, 1998; Daniels et al., 2018). Predation of eggs by non-native species can also be problematic for the recruitment of native fish (Table 1; Schaeffer and Margraf 1987; Silbernagel and Sorensen 2013; Karjalainen et al., 2015). Because of the spawning migrations of generalists in the spring into tributaries (Hladík and Kubečka, 2003), predation on eggs and juveniles may be an important issue. Another example of edge effects is predation of eggs by generalist bleaks, which reduced the reproductive success of rheophilic asp in a tributary of a reservoir (Šmejkal et al., 2017; Šmejkal et al., 2018).
Some rheophilic fish migrate to slower river sections and pools for overwintering (Näslund et al., 1998) and remain nearly inactive during the winter. However, if they use artificial impoundments as overwintering habitats, they may be at higher risk of mortality because many predators are still active when temperatures drop (Brönmark et al., 2008). Although little studied, this may be a very important hidden interaction in altered freshwater ecosystems (Jackson et al., 2001; Hurst, 2007).
6 Temporal habitat displacement of rheophilic species by generalists in tributaries: Competition for food and space
Competitive interactions among fish species may lead to habitat niche displacement or even extirpation of native species (Pfauserová et al., 2021; Tapkir et al., 2022). Strong seasonal migration patterns in generalists such as roach and bleak have been described for fragmented habitats (Slavík et al., 2009; Lothian et al., 2019; Mader et al., 2020). These result in community structure changes with seasonal patterns in tributaries: The ecological quality of fish stocks in the Vltava River, as classified by the European Fish Index (Breine et al. 2005), varied seasonally from good conditions in spring to moderate conditions in autumn, which reflected seasonal colonization by generalist species from the Lipno Reservoir (Pfauserová et al., 2021). Some populations of non-native species, including generalist bream, use this reservoir for overwintering during harsh conditions and tributaries for feeding and/or spawning during the rest of the year (Pfauserová et al., 2021). Such competition for food sources or habitats often results in displacement of native species from tributaries (Hoxmeier and Dieterman, 2016). Accordingly, the presence of non-native fish in tributaries forced native brown trout to relocate to small brook tributaries (Pfauserová et al., 2021). Spatial segregation is a known response of brown trout to increasing competition (Vehanen et al., 1999); however, it might have broader ecological consequences. For example, the critically endangered freshwater pearl mussel (Margaritifera margaritifera) utilizes brown trout as the primary host for its glochidia larvae (Bauer et al., 1991). Forced declines in the usage of the main river by brown trout during the summer when glochidia are released could be considered a threat to reproduction of this mussel, which is considered key to its conservation (Modesto et al., 2018). Seasonal colonization of tributaries by generalist species can thus alter habitats and available resources for native fishes but also affects interactions with other species.
Similar to the evidence from Europe, a study conducted in a reservoir located on the White River, United States showed increased competition of rheophilic fishes with non-native generalists. Generalist species proliferated in the reservoir and comprised up to 90% of the fish community, which also affected the remaining original fish community in the tributary (Martinez et al., 1994). The negative effects of competition with reservoir generalists may also be visible in the significant positive associations of certain species with increasing distances from reservoirs (Falke and Gido, 2006). Another example is the construction of the Three Gorges Reservoir, China, which led to a reduction in rheophilic fishes in the tributaries and increased competition with 18 non-native generalists that proliferated in the modified reservoir environment (Liao et al., 2018; Lin et al., 2018; Liu et al., 2019). Maimela et al. (2022) revealed that adverse impacts on community structure and function were observed due to competition for food and space between O. mykiss and indigenous species in the upper Blyde River Catchment, South Africa. Psedudobarbus verloenii, a tropical rheophilic species, is reported to decline in South Africa due to reservoir construction. This species spawns in clear water, moderate to fast flow throughout the year and rocky substratum. It was widespread throughout the Verlorenvlei and Langvlei River system, South Africa in the past, but the population declined during the last century due to competition with banded Mozambique tilapia (Oreochromis mossambicus), tilapia (Tilapia sparrmanii), and common carp (Cyprinus carpio) (Chakona et al., 2014; Shelton et al., 2015).
Competition for food and space in tributaries is not limited to adult fish. During spring spawning, reservoir generalists can outnumber native rheophilic species and deposit enormous numbers of eggs in tributaries (Hladík and Kubečka, 2004). This deposition of generalist offspring may affect the rheophilic species recruitment as they compete for very similar food sources as juveniles (Specziár and Rezsu, 2009); thus, there is the potential to investigate how rheophilic recruitment is affected by habitat edges with adjacent reservoir fauna. We are not aware of any studies that have quantified this effect.
7 Intentional fragmentation as a tool for reducing the spread of generalist species
Since generalist species usually occupy artificial, degraded habitats (Corbacho and Sánchez, 2001; MacDougall and Turkington, 2005), the simplest strategy to exclude them from uninvaded sites is to protect natural habitats or restore degraded environments and allow them to act as natural barriers against invasions of undesirable species (Rahel, 2007). This restoration measure has been used, e.g., by the removal of the Woolen Mills Dam, United States, and subsequent habitat improvement work, which reduced the dominance of non-native common carp (Cyprinus carpio) in favor of smallmouth bass (Micropterus dolomieu) (Kanehl et al., 1997). Although dam removal (reviewed in e.g. Bednarek, 2001; Tonitto and Riha, 2016; Ding et al., 2019) is optimal solution of strong interaction between generalist fishes and rheophilic fishes, the global trend is to increase proportions of rivers that will be affected by damming (Grill et al., 2015; Zarfl et al., 2015; Winemiller et al., 2016; Cutler et al., 2020). In cases where restoration of degraded environments are not possible, there is a possibility to disrupt the connection between the remaining unoccupied tributary to isolate the habitats that are already occupied by generalist species (Rahel, 2013). This approach, known as intentional fragmentation or isolation management, has been used worldwide and increasingly since the 1950’s (Jones et al., 2021). This management measure significantly increases the eradication success of targeted species in riverine sections, which has otherwise rarely been successful (Simberloff, 2013).
There are several ways to create intentional fragmentation in aquatic systems, and we present the most-often used methods. They consist of mechanical, electrical, chemical or other non-mechanical methods (Table 2). Most commonly, exclusion barriers are used to prevent upstream migration of undesirable species (Rahel 2013). Examples of mechanical barriers include constructing low head dams, gabion barriers, or culvert barriers to restrict common carp and other reservoir generalists from entering the upstream tributaries of the Roaring River, United States (Bulow et al., 1988), limit sea lamprey access to the spawning grounds in the Laurentian Great Lakes basin (McLaughlin et al., 2007; Miehls et al., 2020) and protect native salmonids in western North America (Novinger and Rahel, 2003; Kirk et al., 2018). Although creating new barriers increases fragmentation and can to some extent create new lentic habitats, this impact is exceeded by the benefits of preventing intrusion of unwanted taxa. Moreover, isolation management has been shown for a long time to support native trout populations in headwater streams, when strategically placed barriers isolate catchments that are large enough to allow seasonal movements and maintain metapopulation structures (Harig and Fausch, 2002) and the isolated population is large enough to avoid extinction due to loss of genetic variability or stochastic demographic or environmental events (Cook et al., 2010).
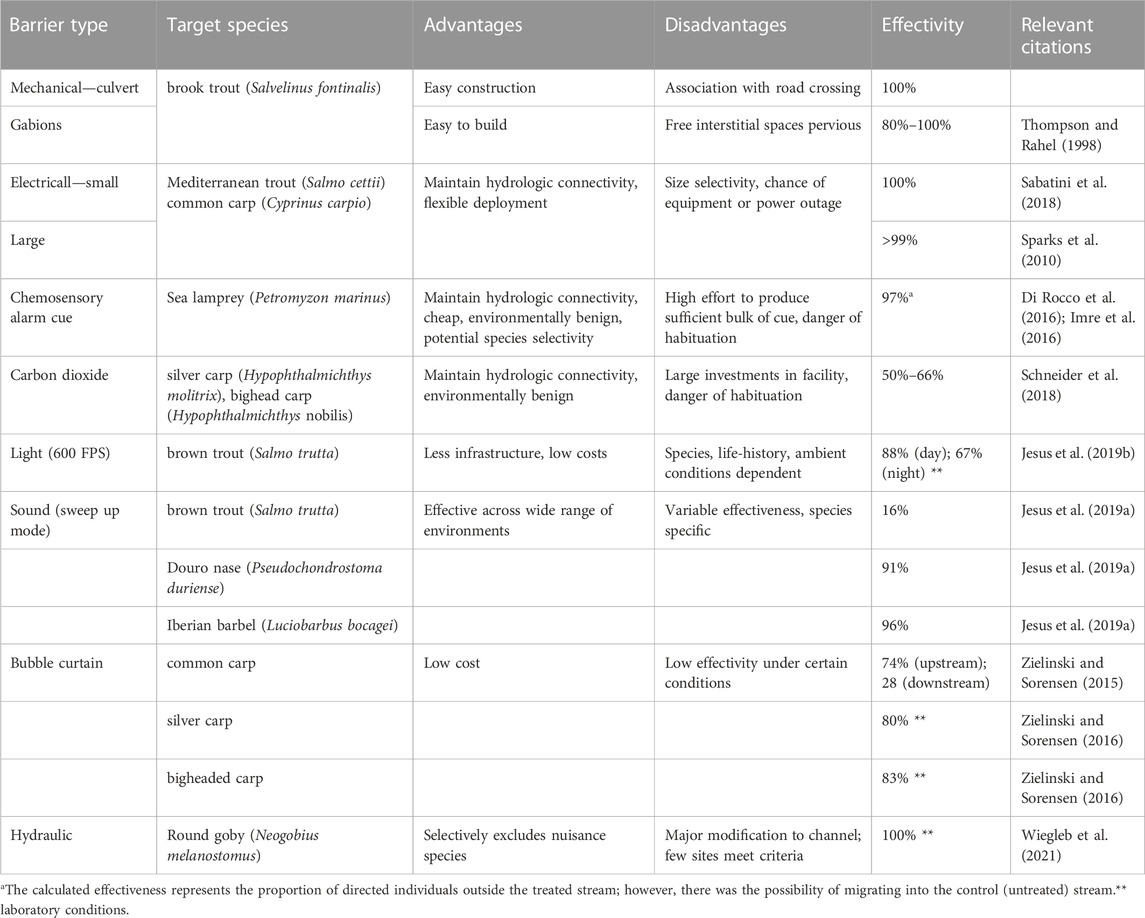
TABLE 2. Examples of different types of barriers used to reduce the spread of generalists that were selected by using the criterion of estimated effectiveness.
Isolation management using electric barriers has been successfully used to block non-native trout and protect reintroduced populations of native Mediterranean brown trout (Salmo cetti) (Sabatini et al., 2018) and a similar device is used seasonally to block the migration of sea lamprey (Petromyzon marinus) and limit its reproduction in the Black Mallard River, Michigan (Johnson et al., 2021). Electric barriers meet the conflicting demands of managers to disrupt biological connectivity while maintaining hydrologic connectivity. Despite this advantage, there is a possibility of power or equipment failure (Clarkson, 2004); these barriers could be overcome by jumping fish, or their effectiveness may decrease during the navigation of metal-hulled barges through these barriers (Parker et al., 2015). The world’s largest and well-known electric barrier system was activated in the Chicago Sanitary and Ship Canal in 2002. Two additional barriers were added in 2009 and still function as the primary barrier to the spread of bigheaded carps (i.e., silver carp Hypophthalmichthys molitrix and bighead carp H. nobilis) between the Mississippi River and the Great Lakes basin (Parker et al., 2016).
Various types of chemical barriers have been used worldwide, but their use is much less widespread compared to mechanical and electric barriers. Application of taxon-specific piscicides can be quite effective in preventing the invasion of non-native species in target areas (Araújo et al., 2018). Although their effects can be effectively neutralized by other substances (Weyl et al., 2014), they are potentially dangerous due to their possible negative effects on entire ecosystems (Birceanu et al., 2014). The use of chemosensory alarm cues (i.e., facilitating early warning for prey fish) could be a cheaper and safer alternative (Frisch, 1938; Ferrari et al., 2010; Imre et al., 2010). The effect of chemical alarm cues was demonstrated primarily under laboratory conditions (Wagner et al., 2011) and showed a significant reduction in the occurrence of non-native species in the field (Di Rocco et al., 2016); however, this effect is not absolute, and combinations with other measures are needed. Carbon dioxide and ozone are other options that can be used to block fish migration and prevent the further spread of non-native species, and they are relatively environmentally benign compared to other chemicals in aquatic systems (Buley et al., 2017; Suski, 2020). While the knowledge of fish behavior with respect to these substances is relatively well known, there are still many data gaps regarding the use of CO2 and O3 in the field, including determining how freshwater river systems will respond to the use of O3 barriers.
Other types of non-mechanical devices that provide barriers to migrations of fish species, which are based on the behavioral responses of fish to physical stimuli (e.g., light, sound, and air bubbles; Bullen and Carlson 2003), are also being used more frequently. Some species are attracted to light (Stamplecoskie et al., 2012), while others are repelled (Hadderingh, 1982), but strobe lights repel most target species and are most effective at pulse rates greater than 300 flashes per minute (Kim and Mandrak, 2017). Experiments showed that acoustic signals influence fish behavior (Vetter et al., 2015), but the observed response was not sufficient to produce a strong deterrent effect in the field (Deleau et al., 2020) and is affected by strong species-specific variability that is likely caused by species differences in auditory sensitivity (Bullen and Carlson, 2003). The efficiency of this type of barrier could be increased in the future by using a sweep or modulated sound (Jesus et al., 2019a) or in combination with air-bubbles (ensonified bubble curtain), which helps to focus and enhance sound fields while often causing its bubbles to resonate (Dennis et al., 2019). The air-bubble curtains that are emitted from air diffusers located along the water bottom achieved over 80% efficiency in reducing passage for bigheaded and common carps (Cyprinus carpio) under laboratory conditions (Zielinski et al., 2014). Although the efficiency was lower in field experiments when fish were more motivated to migrate (Zielinski and Sorensen, 2016), the combination with sound (ensonified bubble curtain) increased its efficiency to 95% or more for bigheaded carps (Taylor et al., 2005). On the other hand, combination of ensonified bubble curtain with strobe light has been show unlikely to block upstream sea lamprey migration in laboratory (Miehls et al., 2017). A new approach to protect pristine upstream areas from invasion by non-native fish consists of hydraulic or velocity barriers (Wiegleb et al., 2021) and these have been tested under laboratory conditions. Based on knowledge of the differences in swimming performance under artificially elevated water flows (Kemp, 2016), environments can be created to prevent passage of the tested non-native species (e.g., round goby Neogobius melanostomus) and allow passage of desired species (e.g., gudgeon Gobio gobio and bullhead Cottus gobio). However, the performance of such barriers has yet to be tested in the field.
In summary, mechanical barriers, when properly operated and maintained, can achieve 100% fish exclusion. Other barrier types are either not 100% effective, are prone to fail in extreme or unexpected events or have not yet been properly tested under field conditions (Table 2). However, the resulting disruption of natural flows by mechanical barriers and blockage of non-target species pose significant challenges. The effectiveness of all behavioral and chemical barriers may continue to decrease over time due to the habituation process of target species (Imre et al., 2016). Nevertheless, they may significantly decrease the number of generalist fishes and potentially reduce the impacts on rheophilic species when combined with other management measures. A systematic review on barrier types and efficiencies along with proper identification of knowledge gaps may be beneficial in this field to achieve progress in the conservation of reservoir tributaries.
8 Conclusion and future directions
Here, we reviewed the impacts on generalists due to damming (which also applies to smaller impoundments) on rheophilic fish fauna based in tributaries and the potential solutions to mitigate their impacts through intentional fragmentation. We suggest that the observed changes in fish diversity and fish functional traits in reservoir tributaries following reservoir construction can be partly attributed to changes in the intensities of interactions among generalists and rheophilic species. We emphasize that the management of edge effects may be critical to maintaining viable populations of rheophilic fishes in lotic ecosystems that are modified to meet human needs. Although many studies have examined the effects of reservoirs on fish diversity (e.g. Esselman et al., 2013; Van Looy et al., 2014; Cooper et al., 2017), detailed data on the intensified interactions among generalists and rheophilic fish and ontogenetic bottlenecks due to competition or predation are generally lacking (Tamario et al., 2021). In particular, we call for detailed temporal telemetry, reproductive migration, and trophic interaction studies to move from descriptive and correlative science to a better understanding of the mechanisms behind the community changes that are caused by artificially enhanced fish fauna interactions. This knowledge may not only provide novel insights into the observed trends but also may lead to novel solutions for rheophilic species conservation efforts, which may be targeted to specific bottlenecks that emerge due to habitat degradation. An example of such conservation measures can consist of blocking the migration of generalists to enable year-round utilization of river channels by brown trout (Pfauserová et al., 2021).
One of the targets of future studies could be determining the habitat proportions that need to be maintained to sustain potamodromous rheophilic fish populations, e.g., (Fausch et al., 2002; Isaak et al., 2007; Perkin et al., 2015). In particular, an important issue is the magnitude of edge effects for protected rheophilic fish species that would prevent their long-term population stability in the face of competition and predation by generalists (e.g. Tamario et al., 2021). Since these patterns will often be species- and site-specific to some extent, considerable conservation efforts will need to be made to minimize local extinctions of rheophilic fishes in fragmented rivers. In case a barrier is important to human needs more than to protecting targeted species, what measures can be implemented to minimize the predation and competition pressures from generalists? Antimigration barriers that prevent generalists and other non-native species from entering tributaries can help preserve the remaining free-flowing segments, and much has been done in recent decades to make progress in this area. However, efficiency evaluations of particular barrier types need more research under comparable field conditions. Migrations of generalists from reservoirs to tributaries provide an opportunity to apply temporarily installed barriers and mitigate edge effects for conservation needs.
Author contributions
MŠ initiated a topical workshop on the manuscript concept and manuscript outline. All authors contributed by drafting manuscript chapters. All authors provided substantial feedback to the first and subsequent drafts.
Funding
MŠ, DB, and JK were supported by the project “The enhancement of rheophilous fish reproduction in the artificial river environment” (No. TJ02000012) and AV 21 strategy support program “Water for Life”. OS, PH, and NP were supported by the European Regional Development Fund Project (No. CZ.02.1.01/0.0/0.0/16_019/0000845). MM was supported by project TAČR “Water systems and water management in the Czech Republic in conditions of climate change” (No. SS02030027).
Acknowledgments
We would like to express thanks to Zuzana Sajdlová for drawing Figure 3.
Conflict of interest
The authors declare that the research was conducted in the absence of any commercial or financial relationships that could be construed as a potential conflict of interest.
Publisher’s note
All claims expressed in this article are solely those of the authors and do not necessarily represent those of their affiliated organizations, or those of the publisher, the editors and the reviewers. Any product that may be evaluated in this article, or claim that may be made by its manufacturer, is not guaranteed or endorsed by the publisher.
References
Aarts, B. G. W., and Nienhuis, P. H. (2003). Fish zonations and guilds as the basis for assessment of ecological [2pt] integrity of large rivers. Hydrobiologia 500, 157–178. doi:10.1023/A:1024638726162
Agostinho, A. A., Miranda, L. E., Bini, L. M., Gomes, L. C., Thomaz, S. M., and Suzuki, H. I. (1999). “Patterns of colonization in neotropical reservoirs, and prognoses on aging,” in Theoretical reservoir ecology and its applications. Editors J. G. Tundisi, and M. Straskraba (Leiden: Blackhuys Publisher), 585.
Andren, H., and Angelstam, P. (1988). Elevated predation rates as an edge effect in habitat islands: Experimental evidence. Ecology 69, 544–547. doi:10.2307/1940455
Araújo, C. V. M., Silva, D. C. V. R., Gomes, L. E. T., Acayaba, R. D., Montagner, C. C., Moreira-Santos, M., et al. (2018). Habitat fragmentation caused by contaminants: Atrazine as a chemical barrier isolating fish populations. Chemosphere 193, 24–31. doi:10.1016/J.CHEMOSPHERE.2017.11.014
Arboleya, E., Fernández, S., Clusa, L., Dopico, E., and Garcia-Vazquez, E. (2021). River connectivity is crucial for safeguarding biodiversity but may be socially overlooked. Insights from Spanish University students. Front. Environ. Sci. 9, 231. doi:10.3389/fenvs.2021.643820
Arnold, G. P., Shipley, O. N., and Siskey, M. R. (2019). Fish migration, horizontal. Encycl. Ocean. Sci., 205–216. doi:10.1016/B978-0-12-409548-9.11339-9
Bain, M. B., Finn, J. T., and Booke, H. E. (1988). Streamflow regulation and fish community structure. Ecology 69, 382–392. doi:10.2307/1940436
Balon, E. K. (1975). Reproductive guilds of fishes: A proposal and definition. J. Fish. Res. Board Can. 32, 821–864. doi:10.1139/f75-110
Baril, M., and Magnan, P. (2002). Seasonal timing and diel activity of lacustrine brook charr, Salvelinus fontinalis, spawning in a lake outlet. Environ. Biol. Fishes 64, 175–181. doi:10.1023/A:1016097704008
Bartoň, D., Blabolil, P., Sajdlová, Z., Vejřík, L., Souza, A. T., Kubečka, J., et al. (2021). Effects of hydropeaking on the attached eggs of a rheophilic cyprinid species. Ecohydrology 14, e2280. doi:10.1002/eco.2280
Bartoň, D., Brabec, M., Sajdlová, Z., Souza, A. T., Duras, J., Kortan, D., et al. (2022). Hydropeaking causes spatial shifts in a reproducing rheophilic fish. Sci. Total Environ. 806, 150649. doi:10.1016/J.SCITOTENV.2021.150649
Bauer, G., Hochwald, S., and Silkenat, W. (1991). Spatial distribution of freshwater mussels: The role of host fish and metabolic rate. Freshw. Biol. 26, 377–386. doi:10.1111/J.1365-2427.1991.TB01405.X
Bednarek, A. T. (2001). Undamming rivers: A review of the ecological impacts of dam removal. Environ. Manage. 27, 803–814. doi:10.1007/s002670010189
Belletti, B., Garcia de Leaniz, C., Jones, J., Bizzi, S., Börger, L., Segura, G., et al. (2020). More than one million barriers fragment Europe’s rivers. Nat 588, 436–441. doi:10.1038/s41586-020-3005-2
Belmar, O., Velasco, J. V., Martínez-Capel, F., and Marín, A. A. (2010). Natural flow regime, degree of alteration and environmental flows in the Mula stream (Segura River basin, SE Spain). Limnetica 29, 353–368. doi:10.23818/limn.29.29
Benitez, J.-P., Matondo, B. N., Dierckx, A., and Ovidio, M. (2015). An overview of potamodromous fish upstream movements in medium-sized rivers, by means of fish passes monitoring. Aquat. Ecol. 49, 481–497. doi:10.1007/s10452-015-9541-4
Bešta, T., Muška, M., Juggins, S., and Těšitel, J. (2015). Comparison of diatom community structure from epilithon and fish guts: Implications for inferring past changes in water quality. Hydrobiologia 742, 233–248. doi:10.1007/s10750-014-1987-1
Birceanu, O., Sorensen, L. A., Henry, M., McClelland, G. B., Wang, Y. S., and Wilkie, M. P. (2014). The effects of the lampricide 3-trifluoromethyl-4-nitrophenol (TFM) on fuel stores and ion balance in a non-target fish, the rainbow trout (Oncorhynchus mykiss). Comp. Biochem. Physiol. C. Toxicol. Pharmacol. 160, 30–41. doi:10.1016/J.CBPC.2013.10.002
Blackwell, B. F., and Juanes, F. (1998). Predation on atlantic salmon smolts by striped bass after dam passage. North Am. J. Fish. Manag. 18, 936–939. doi:10.1577/1548-8675(1998)018<0936:poassb>2.0.co;2
Boulêtreau, S., Carry, L., Meyer, E., Filloux, D., Menchi, O., Mataix, V., et al. (2020). High predation of native sea lamprey during spawning migration. Sci. Rep. 10, 6122–6129. doi:10.1038/s41598-020-62916-w
Boulêtreau, S., Fauvel, T., Laventure, M., Delacour, R., Bouyssonnié, W., Azémar, F., et al. (2021). The giants’ feast”: Predation of the large introduced European catfish on spawning migrating allis shads. Aquat. Ecol. 55, 75–83. doi:10.1007/s10452-020-09811-8
Boulêtreau, S., Gaillagot, A., Carry, L., Tétard, S., De Oliveira, E., and Santoul, F. (2018). Adult Atlantic salmon have a new freshwater predator. PLoS One 13, e0196046. doi:10.1371/JOURNAL.PONE.0196046
Breine, J., Simoens, I., Haidvogl, G., Melcher, A., Pont, D., and Schmutz, S. (2005). Manual for application of the european fish index - EFI: A fish-based method to assess the ecological status of European rivers in support of the water framework directive. FAME, 81.
Brodersen, J., Chapman, B. B., Nilsson, P. A., Skov, C., Hansson, L.-A., and Brönmark, C. (2014). Fixed and flexible: Coexistence of obligate and facultative migratory strategies in a freshwater fish. PLoS One 9, e90294. doi:10.1371/JOURNAL.PONE.0090294
Brönmark, C., Skov, C., Brodersen, J., Nilsson, P. A., and Hansson, L.-A. (2008). Seasonal migration determined by a trade-off between predator avoidance and growth. PLoS One 3, e1957. doi:10.1371/journal.pone.0001957
Buisson, L., Blanc, L., and Grenouillet, G. (2008). Modelling stream fish species distribution in a river network: The relative effects of temperature versus physical factors. Ecol. Freshw. Fish. 17, 244–257. doi:10.1111/J.1600-0633.2007.00276.X
Buley, R. P., Hasler, C. T., Tix, J. A., Suski, C. D., and Hubert, T. D. (2017). Can ozone be used to control the spread of freshwater aquatic invasive species? Manag. Biol. Invasions 8, 13–24. doi:10.3391/MBI.2017.8.1.02
Bullen, C. R., and Carlson, T. J. (2003). Non-physical fish barrier systems: Their development and potential applications to marine ranching. Rev. Fish. Biol. Fish. 13, 201–212. doi:10.1023/B:RFBF.0000019481.10670.94
Bulow, F., Webb, M., Crumby, W., and Quisenberry, S. (1988). Management briefs: Effectiveness of a fish barrier dam in limiting movement of rough fishes from a reservoir into a tributary stream. North Am. J. Fish. Manag. 8, 273–275. doi:10.1577/1548-8675(1988)008<0273:mbeoaf>2.3.co;2
Chakona, A., Swartz, E. R., and Skelton, P. H. (2014). A new species of redfin (teleostei, Cyprinidae, Pseudobarbus) from the Verlorenvlei River system, South Africa. Zookeys 121, 121–137. doi:10.3897/ZOOKEYS.453.8072
Chapleau, F., Findlay, S. C., and Szenasy, E. (1997). Impact of piscivorous fish introductions on fish species richness of small lakes in Gatineau Park, Québec. Écoscience 4, 259–268. doi:10.1080/11956860.1997.11682404
Chapman, B. B., Hulthén, K., Blomqvist, D. R., Hansson, L.-A., Nilsson, J.-Å., Brodersen, J., et al. (2011). To boldly go: Individual differences in boldness influence migratory tendency. Ecol. Lett. 14, 871–876. doi:10.1111/j.1461-0248.2011.01648.x
Clarkson, R. W. (2004). Effectiveness of electrical fish barriers associated with the Central Arizona project. North Am. J. Fish. Manag. 24, 94–105. doi:10.1577/M02-146
Clavero, M., and Hermoso, V. (2010). Reservoirs promote the taxonomic homogenization of fish communities within river basins. Biodivers. Conserv. 20, 41–57. doi:10.1007/S10531-010-9945-3
Cook, N., Rahel, F. J., and Hubert, W. A. (2010). Persistence of Colorado River cutthroat trout populations in isolated headwater streams of Wyoming. Trans. Am. Fish. Soc. 139, 1500–1510. doi:10.1577/T09-133.1
Cooper, A. R., Infante, D. M., Daniel, W. M., Wehrly, K. E., Wang, L., and Brenden, T. O. (2017). Assessment of dam effects on streams and fish assemblages of the conterminous USA. Sci. Total Environ. 586, 879–889. doi:10.1016/J.SCITOTENV.2017.02.067
Copp, G. H., Robert Britton, J., Cucherousset, J., García-Berthou, E., Kirk, R., Peeler, E., et al. (2009). Voracious invader or benign feline? A review of the environmental biology of European catfish Silurus glanis in its native and introduced ranges. Fish. Fish. 10, 252–282. doi:10.1111/j.1467-2979.2008.00321.x
Corbacho, C., and Sánchez, J. M. (2001). Patterns of species richness and introduced species in native freshwater fish faunas of a mediterranean-type basin: The guadiana river (southwest iberian peninsula). Regul. Rivers Res. Manag. 17, 699–707. doi:10.1002/RRR.631
Cutler, J. S., Olivos, J. A., Sidlauskas, B., and Arismendi, I. (2020). Habitat loss due to dam development may affect the distribution of marine-associated fishes in Gabon, Africa. Ecosphere 11. doi:10.1002/ecs2.3024
Daniels, J., Chaput, G., and Carr, J. (2018). Estimating consumption rate of Atlantic salmon smolts (Salmo salar) by striped bass (Morone saxatilis) in the Miramichi River estuary using acoustic telemetry. Can. J. Fish. Aquat. Sci. 75, 1811–1822. doi:10.1139/cjfas-2017-0373
Dawson, W., Moser, D., Van Kleunen, M., Kreft, H., Pergl, J., Pyšek, P., et al. (2017). Global hotspots and correlates of alien species richness across taxonomic groups. Nat. Ecol. Evol. 17 (1), 0186–0187. doi:10.1038/s41559-017-0186
Degerman, E., Beier, U., Breine, J., Melcher, A., Quataert, P., Rogers, C., et al. (2007). Classification and assessment of degradation in European running waters. Fish. Manag. Ecol. 14, 417–426. doi:10.1111/J.1365-2400.2007.00578.X
Deinet, S., Scott-Gatty, K., Rotton, H., Twardek, W. M., Marconi, V., McRae, L., et al. (2020). The living planet index (LPI) for migratory freshwater fish - technical report.
Deleau, M. J. C., White, P. R., Peirson, G., Leighton, T. G., and Kemp, P. S. (2020). The response of anguilliform fish to underwater sound under an experimental setting. River Res. Appl. 36, 441–451. doi:10.1002/RRA.3583
Dennis, C. E., Zielinski, D., and Sorensen, P. W. (2019). A complex sound coupled with an air curtain blocks invasive carp passage without habituation in a laboratory flume. Biol. Invasions 21, 2837–2855. doi:10.1007/s10530-019-02017-6
Di Rocco, R. T., Johnson, N. S., Brege, L., Imre, I., and Brown, G. E. (2016). Sea lamprey avoid areas scented with conspecific tissue extract in Michigan streams. Fish. Manag. Ecol. 23, 548–560. doi:10.1111/FME.12198
Ding, L., Chen, L., Ding, C., and Tao, J. (2019). Global trends in dam removal and related research: A systematic review based on associated datasets and bibliometric analysis. Chin. Geogr. Sci. 29, 1–12. doi:10.1007/s11769-018-1009-8
Drake, D. A. R., and Mandrak, N. E. (2014). Ecological risk of live bait fisheries: A new angle on selective fishing. Fisheries 39, 201–211. doi:10.1080/03632415.2014.903835
Dudgeon, D., Arthington, A. H., Gessner, M. O., Kawabata, Z. I., Knowler, D. J., Lévêque, C., et al. (2006). Freshwater biodiversity: Importance, threats, status and conservation challenges. Biol. Rev. 81, 163–182. doi:10.1017/S1464793105006950
Early, R., Bradley, B. A., Dukes, J. S., Lawler, J. J., Olden, J. D., Blumenthal, D. M., et al. (2016). Global threats from invasive alien species in the twenty-first century and national response capacities. Nat. Commun. 7, 12485. doi:10.1038/ncomms12485
Esselman, P. C., Infante, D. M., Wang, L., Cooper, A. R., Wieferich, D., Tsang, Y. P., et al. (2013). Regional fish community indicators of landscape disturbance to catchments of the conterminous United States. Ecol. Indic. 26, 163–173. doi:10.1016/J.ECOLIND.2012.10.028
Falke, J. A., and Gido, K. B. (2006). Spatial effects of reservoirs on fish assemblages in Great Plains streams in Kansas, USA. River Res. Appl. 22, 55–68. doi:10.1002/RRA.889
Fausch, K. D., Torgesen, C. E., Baxter, C. V., and Li, H. W. (2002). Landscapes to riverscapes: Bridging the gap between research and conservation of stream fishes. Bioscience 52, 483–498. doi:10.1641/0006-3568(2002)052[0483:ltrbtg]2.0.co;2
Feng, M., Zolezzi, G., and Pusch, M. (2018). Effects of thermopeaking on the thermal response of alpine river systems to heatwaves. Sci. Total Environ. 612, 1266–1275. doi:10.1016/J.SCITOTENV.2017.09.042
Fernando, C. H. (1994). Zooplankton, fish and fisheries in tropical freshwaters. Hydrobiol 272, 105–123. doi:10.1007/BF00006516
Fernando, C. H., and Holčík, J. (1991). Fish in reservoirs. Int. Rev. gesamten Hydrobiol. Hydrogr. 76, 149–167. doi:10.1002/iroh.19910760202
Ferrari, M. C. O., Wisenden, B. D., and Chivers, D. P. (2010). Chemical ecology of predator–prey interactions in aquatic ecosystems: A review and prospectusThe present review is one in the special series of reviews on animal–plant interactions. Can. J. Zool. 88, 698–724. doi:10.1139/z10-029
Fischer, J., and Lindenmayer, D. B. (2007). Landscape modification and habitat fragmentation: A synthesis. Glob. Ecol. Biogeogr. 16, 265–280. doi:10.1111/J.1466-8238.2007.00287.X
Franco, A. C. S., García-Berthou, E., and Santosdos, L. N. (2021). Ecological impacts of an invasive top predator fish across South America. Sci. Total Environ. 761, 143296. doi:10.1016/J.SCITOTENV.2020.143296
Franco, A. C. S., Petry, A. C., García-Berthou, E., and dos Santos, L. N. (2022a). Invasive peacock basses (Cichla spp.) and decreased abundance of small native fish in Brazilian reservoirs. Aquat. Conserv. Mar. Freshw. Ecosyst. 32, 1852–1866. doi:10.1002/AQC.3874
Franco, A. C. S., Petry, A. C., Tavares, M. R., de Fátima Ramos Guimarães, T., and dos Santos, L. N. (2022b). Global distribution of the South American peacock basses Cichla spp. follows human interference. Fish. Fish. 23, 407–421. doi:10.1111/FAF.12624
Frisch, K. R. von (1938). Zur psychologie des fisch-schwarmes. Naturwissenschaften 26, 601–606. doi:10.1007/bf01590598
Fuller, M. R., Doyle, M. W., and Strayer, D. L. (2015). Causes and consequences of habitat fragmentation in river networks. Ann. N. Y. Acad. Sci. 1355, 31–51. doi:10.1111/NYAS.12853
Gago, J., Anastácio, P., Gkenas, C., Banha, F., and Ribeiro, F. (2016). Spatial distribution patterns of the non-native European catfish, Silurus glanis, from multiple online sources – A case study for the river tagus (iberian peninsula). Fish. Manag. Ecol. 23, 503–509. doi:10.1111/FME.12189
Ganassin, M. J. M., Muñoz-Mas, R., de Oliveira, F. J. M., Muniz, C. M., dos Santos, N. C. L., García-Berthou, E., et al. (2021). Effects of reservoir cascades on diversity, distribution, and abundance of fish assemblages in three Neotropical basins. Sci. Total Environ. 778, 146246. doi:10.1016/J.SCITOTENV.2021.146246
Gido, K. B., Schaeffer, J. F., and Falke, J. A. (2009). Convergence of fish communities from the littoral zone of reservoirs. Freshw. Biol. 54, 1163–1177. doi:10.1111/J.1365-2427.2008.02162.X
Grill, G., Lehner, B., Lumsdon, A. E., Macdonald, G. K., Zarfl, C., and Reidy Liermann, C. (2015). An index-based framework for assessing patterns and trends in river fragmentation and flow regulation by global dams at multiple scales. Environ. Res. Lett. 10, 015001. doi:10.1088/1748-9326/10/1/015001
Grill, G., Lehner, B., Thieme, M., Geenen, B., Tickner, D., Antonelli, F., et al. (2019). Mapping the world’s free-flowing rivers. Nature 569, 215–221. doi:10.1038/s41586-019-1111-9
Guenther, C. B., and Spacie, A. (2006). Changes in fish assemblage structure upstream of impoundments within the Upper Wabash River basin, Indiana. Trans. Am. Fish. Soc. 135, 570–583. doi:10.1577/T05-031.1
Guillerault, N., Delmotte, S., Boulêtreau, S., Lauzeral, C., Poulet, N., and Santoul, F. (2015). Does the non-native European catfish Silurus glanis threaten French river fish populations? Freshw. Biol. 60, 922–928. doi:10.1111/FWB.12545
Hadderingh, R. (1982). Experimental reduction of fish impingement by artificial illumination at Bergum power station. Int. Rev. gesamten Hydrobiol. 67, 887–900.
Harig, A. L., and Fausch, K. D. (2002). Minimum habitat requirements for establishing translocated cutthroat trout populations. Ecol. Appl. 12, 535–551. doi:10.1890/1051-0761(2002)012[0535:mhrfet]2.0.co;2
Harrison, P. M., Gutowsky, L. F. G., Martins, E. G., Patterson, D. A., Cooke, S. J., and Power, M. (2015). Personality-dependent spatial ecology occurs independently from dispersal in wild burbot (Lota lota). Behav. Ecol. 26, 483–492. doi:10.1093/BEHECO/ARU216
Harvey, B. C., and Nakamoto, R. J. (2013). Seasonal and among-stream variation in predator encounter rates for fish prey. Trans. Am. Fish. Soc. 142, 621–627. doi:10.1080/00028487.2012.760485
Havel, J. E., Lee, C. E., and Zanden, M. J. Vander (2005). Do reservoirs facilitate invasions into landscapes? Bioscience 55, 518–526. doi:10.1641/0006-3568(2005)055[0518:drfiil]2.0.co;2
Havlena, F. (1964). Contribution to the study on age and growth in the barb, Barbus barbus (L.) in the drainage area of the Orava River dam basin. Folia Zool. 13, 321–326.
Hermoso, V., Clavero, M., Blanco-Garrido, F., and Prenda, J. (2011). Invasive species and habitat degradation in Iberian streams: An analysis of their role in freshwater fish diversity loss. Ecol. Appl. 21, 175–188. doi:10.1890/09-2011.1
Hirsch, P. E., N’Guyen, A., Muller, R., Adrian-Kalchhauser, I., and Burkhardt-Holm, P. (2018). Colonizing islands of water on dry land—On the passive dispersal of fish eggs by birds. Fish. Fish. 19, 502–510. doi:10.1111/FAF.12270
Hladík, M., and Kubečka, J. (2003). Fish migration between a temperate reservoir and its main tributary. Hydrobiologia 504, 251–266. doi:10.1023/b:hydr.0000008525.46939.42
Hladík, M., Kubečka, J., Mrkvička, T., Čech, M., Draštík, V., Frouzová, J., et al. (2008). Effects of the construction of a reservoir on the fish assemblage in an inflow river. Czech J. Anim. Sci. 53, 537–547. doi:10.17221/370-cjas
Hladík, M., and Kubečka, J. (2004). The effect of water level fluctuation on tributary spawning migration of reservoir fish. Ecohydrol. Hydrobiol. 4, 449–457.
Hoxmeier, R. J. H., and Dieterman, D. J. (2016). Long-term population demographics of native brook trout following manipulative reduction of an invader. Biol. Invasions 18, 2911–2922. doi:10.1007/s10530-016-1182-6
Hülsmann, S., Mehner, T., Worischka, S., and Plewa, M. (1999). Is the difference in population dynamics of Daphnia galeata in littoral and pelagic areas of a long-term biomanipulated reservoir affected by age-0 fish predation? Hydrobiologia 408–409, 57–63. doi:10.1007/978-94-017-2986-4_6
Hurst, T. P. (2007). Causes and consequences of winter mortality in fishes. J. Fish. Biol. 71, 315–345. doi:10.1111/j.1095-8649.2007.01596.x
Imre, I., Brown, G. E., Bergstedt, R. A., and McDonald, R. (2010). Use of chemosensory cues as repellents for sea lamprey: Potential directions for population management. J. Gt. Lakes. Res. 36, 790–793. doi:10.1016/J.JGLR.2010.07.004
Imre, I., Di Rocco, R. T., Brown, G. E., and Johnson, N. S. (2016). Habituation of adult sea lamprey repeatedly exposed to damage-released alarm and predator cues. Environ. Biol. Fishes 99, 613–620. doi:10.1007/S10641-016-0503-Z
Irz, P., Laurent, A., Messad, S., Pronier, O., and Argillier, C. (2002). Influence of site characteristics on fish community patterns in French reservoirs. Ecol. Freshw. Fish. 11, 123–136. doi:10.1034/J.1600-0633.2002.00004.X
Isaak, D. J., Thurow, R. F., Rieman, B. E., and Dunham, J. B. (2007). Chinook salmon use of spawning patches: Relative roles of habitat quality, size, and connectivity. Ecol. Appl. 17, 352–364. doi:10.1890/05-1949
Jackson, D. A., Peres-Neto, P. R., and Olden, J. D. (2001). What controls who is where in freshwater fish communities - the roles of biotic, abiotic, and spatial factors. Can. J. Fish. Aquat. Sci. 58, 157–170. doi:10.1139/CJFAS-58-1-157
Jarolím, O., Kubečka, J., Čech, M., Vašek, M., Peterka, J., and Matěna, J. (2010). Sinusoidal swimming in fishes: The role of season, density of large zooplankton, fish length, time of the day, weather condition and solar radiation. Hydrobiologia 654, 253–265. doi:10.1007/s10750-010-0398-1
Jepsen, N., Aarestrup, K., Økland, F., and Rasmussen, G. (1998). Survival of radiotagged atlantic salmon (Salmo salar L.) – And trout (Salmo trutta L.) smolts passing a reservoir during seaward migration. Hydrobiologia 371–372, 347–353. doi:10.1023/A:1017047527478
Jepsen, N., Pedersen, S., and Thorstad, E. (2000). Behavioural interactions between prey (trout smolts) and predators (pike and pikeperch) in an impounded river. River Res. Appl. 16, 189–198. doi:10.1002/(sici)1099-1646(200003/04)16:2<189:aid-rrr570>3.0.co;2-n
Jesus, J., Amorim, M. C. P., Fonseca, P. J., Teixeira, A., Natário, S., Carrola, J., et al. (2019a). Acoustic barriers as an acoustic deterrent for native potamodromous migratory fish species. J. Fish. Biol. 95, 247–255. doi:10.1111/JFB.13769
Jesus, J., Teixeira, A., Natário, S., and Cortes, R. (2019b). Repulsive effect of stroboscopic light barriers on native salmonid (Salmo trutta) and cyprinid (Pseudochondrostoma duriense and Luciobarbus bocagei) species of Iberia. Sustain 11, 1332. doi:10.3390/SU11051332
Johnson, B. L., Richardson, W. B., and Naimo, T. J. (1995). Past, present, and future concepts in large river ecology. Bioscience 45, 134–141. doi:10.2307/1312552
Johnson, N. S., Snow, B., Bruning, T., and Jubar, A. (2021). A seasonal electric barrier blocks invasive adult sea lamprey (Petromyzon marinus) and reduces production of larvae. J. Gt. Lakes. Res. 47, S310–S319. doi:10.1016/J.JGLR.2021.09.008
Johnson, P. T. J., Olden, J. D., and Vander Zanden, M. J. (2008). Dam invaders: Impoundments facilitate biological invasions into freshwaters. Front. Ecol. Environ. 6, 357–363. doi:10.1890/070156
Jones, P. E., Tummers, J. S., Galib, S. M., Woodford, D. J., Hume, J. B., Silva, L. G. M., et al. (2021). The use of barriers to limit the spread of aquatic invasive animal species: A global review. Front. Ecol. Evol. 9, 43. doi:10.3389/fevo.2021.611631
Jumani, S., Deitch, M. J., Kaplan, D., Anderson, E. P., Krishnaswamy, J., Lecours, V., et al. (2019). river fragmentation and flow alteration metrics: A review of methods and directions for future research. Environ. Res. Lett. 15, 123009. doi:10.1088/1748-9326/ABCB37
Kanehl, P., Lyons, J., and Nelson, J. (1997). Changes in the habitat and fish community of the milwaukee river, Wisconsin following removal of the woolen Mills dam. North Am. J. Fish. Manag. 17, 387–400. doi:10.1577/1548-8675(1997)017<0387:cithaf>2.3.co;2
Karjalainen, J., Ruokonen, T. J., Marjomäki, T. J., Martikainen, A., Pursiainen, M., Sarvala, J., et al. (2015). Predation by signal crayfish Pacifastacus leniusculus on fish eggs and its consequences for coregonid recruitment. J. Fish. Biol. 86, 651–667. doi:10.1111/jfb.12588
Kemp, P. S. (2016). Meta-analyses, metrics and motivation: Mixed messages in the fish passage debate. River Res. Appl. 32, 2116–2124. doi:10.1002/RRA.3082
Kim, J., and Mandrak, N. E. (2017). Effects of strobe lights on the behaviour of freshwater fishes. Enviromental Biol. Fishes 100, 1427–1434. doi:10.1007/S10641-017-0653-7
Kirk, M. A., Rosswog, A. N., Ressel, K. N., and Wissinger, S. A. (2018). Evaluating the trade-offs between invasion and isolation for native brook trout and nonnative Brown trout in Pennsylvania streams. Trans. Am. Fish. Soc. 147, 806–817. doi:10.1002/TAFS.10078
Knott, J., Nagel, C., and Geist, J. (2021). Wasted effort or promising approach – does it make sense to build an engineered spawning ground for rheophilic fish in reservoir cascades? Ecol. Eng. 173, 106434. doi:10.1016/j.ecoleng.2021.106434
Kraft, N. J. B., Adler, P. B., Godoy, O., James, E. C., Fuller, S., and Levine, J. M. (2015). Community assembly, coexistence and the environmental filtering metaphor. Funct. Ecol. 29, 592–599. doi:10.1111/1365-2435.12345
Kristiansen, H., and Dølving, K. B. (1996). The migration of spawning stocks of grayling Thymallus thymallus, in Lake Mjøsa, Norway. Environ. Biol. Fishes 47, 43–50. doi:10.1007/BF00002378
Kubečka, J. (1993). Succession of fish communities in reservoirs of central and eastern Europe. Comp. Reserv. Limnol. water Qual. Manag. 77, 153–168. doi:10.1007/978-94-017-1096-1_11
Larsson, P. -O. (1985). Predation on migrating smolt as a regulating factor in Baltic salmon, Salmo salar L., populations. J. Fish. Biol. 26, 391–397. doi:10.1111/j.1095-8649.1985.tb04279.x
Lehner, B., Liermann, C. R., Revenga, C., Vörömsmarty, C., Fekete, B., Crouzet, P., et al. (2011). High-resolution mapping of the world’s reservoirs and dams for sustainable river-flow management. Front. Ecol. Environ. 9, 494–502. doi:10.1890/100125
Lenhardt, M., Markovic, G., and Gacic, Z. (2009). Decline in the index of biotic integrity of the fish assemblage as a response to reservoir aging. Water Resour. Manag. 23, 1713–1723. doi:10.1007/S11269-008-9348-3
Li, B., Wang, Y., Tan, W., Saintilan, N., Lei, G., and Wen, L. (2021). Land cover alteration shifts ecological assembly processes in floodplain lakes: Consequences for fish community dynamics. Sci. Total Environ. 782, 146724. doi:10.1016/J.SCITOTENV.2021.146724
Li, J., Dong, S., Peng, M., Yang, Z., Liu, S., Li, X., et al. (2013). Effects of damming on the biological integrity of fish assemblages in the middle Lancang-Mekong River basin. Ecol. Indic. 34, 94–102. doi:10.1016/J.ECOLIND.2013.04.016
Liao, C., Chen, S., De Silva, S. S., Correa, S. B., Yuan, J., Zhang, T., et al. (2018). Spatial changes of fish assemblages in relation to filling stages of the Three Gorges Reservoir, China. J. Appl. Ichthyol. 34, 1293–1303. doi:10.1111/JAI.13798
Liew, J. H., Tan, H. H., and Yeo, D. C. J. (2016). Dammed rivers: Impoundments facilitate fish invasions. Freshw. Biol. 61, 1421–1429. doi:10.1111/FWB.12781
Limburg, K. E., and Waldman, J. R. (2009). Dramatic declines in North Atlantic diadromous fishes. Bioscience 59, 955–965. doi:10.1525/BIO.2009.59.11.7
Lin, P., Xin, G., Liu, F., Li, M., and Liu, H. (2018). Long-term monitoring revealed fish assemblage zonation in the Three Gorges Reservoir. J. Oceanol. Limnol. 37, 1258–1267. doi:10.1007/S00343-019-8165-2
Liu, X., Qin, J., Xu, Y., Ouyang, S., and Wu, X. (2019). Biodiversity decline of fish assemblages after the impoundment of the three Gorges dam in the yangtze river basin, China. Rev. Fish. Biol. Fish. 29, 177–195. doi:10.1007/s11160-019-09548-0
Lothian, A. J., Gardner, C. J., Hull, T., Griffiths, D., Dickinson, E. R., and Lucas, M. C. (2019). Passage performance and behaviour of wild and stocked cyprinid fish at a sloping weir with a Low Cost Baffle fishway. Ecol. Eng. 130, 67–79. doi:10.1016/J.ECOLENG.2019.02.006
Lovas-Kiss, A., Vincze, O., Löki, V., Paller-Kapusi, F., Halasi-Kovács, B., Kovács, G., et al. (2020). Experimental evidence of dispersal of invasive cyprinid eggs inside migratory waterfowl. Proc. Natl. Acad. Sci. U. S. A. 117, 15397–15399. doi:10.1073/pnas.2004805117
Lucas, M. C., and Baras, E. (2001). Migration of freshwater fishes. Editors M. C. Lucas, E. Baras, T. J. Thom, A. Duncan, and O. Slavk (Oxford, UK). doi:10.1002/9780470999653
Lusk, S., Lusková, V., and Hanel, L. (2010). Alien fish species in the Czech Republic and their impact on the native fish fauna. Folia Zool. 59, 57–72. doi:10.25225/fozo.v59.i1.a9.2010
Lutz, E., Hirsch, P. E., Bussmann, K., Wiegleb, J., Jermann, H. P., Muller, R., et al. (2020). Predation on native fish eggs by invasive round goby revealed by species-specific gut content DNA analyses. Aquat. Conserv. Mar. Freshw. Ecosyst. 30, 1566–1577. doi:10.1002/aqc.3409
Lyach, R. (2021). Harvest rates of rheophilic fish Vimba vimba, Chondrostoma nasus, and Barbus barbus have a strong relationship with restocking rates and harvest rates of their predator Silurus glanis in lowland mesotrophic rivers in Central Eu. Sustainability 13, 11379. doi:10.3390/SU132011379
MacDougall, A. S., and Turkington, R. (2005). Are invasive species the drivers or passengers of change in degraded ecosystems? Ecology 86, 42–55. doi:10.1890/04-0669
Mader, H., Brandl, A., and Käfer, S. (2020). Design and function monitoring of an Enature® vertical slot fish pass in a large potamal river in carinthia/Austria. Water 12, 551. doi:10.3390/W12020551
Maimela, L. T., Chimimba, C. T., and Zengeya, T. A. (2022). Niche expansion by indigenous fish species following the introduction of rainbow trout (Oncorhynchus mykiss) in a subtropical river system, upper Blyde River, South Africa. Front. Environ. Sci. 0, 1667. doi:10.3389/FENVS.2022.949353
Martinez, P. J., Chart, T. E., Trammell, M. A., Wullschleger, J. G., and Bergersen, E. P. (1994). Fish species composition before and after construction of a main stem reservoir on the White River, Colorado. Environ. Biol. Fishes 40, 227–239. doi:10.1007/BF00002509
Matthews, W. J., and Marsh-Matthews, E. (2011). Extirpation of red shiner in direct tributaries of Lake Texoma (Oklahoma-Texas): A cautionary case history from a fragmented river-reservoir system. Trans. Am. Fish. Soc. 136, 1041–1062. doi:10.1577/T06-059.1
McLaughlin, R. L., Hallett, A., Pratt, T. C., O’Connor, L. M., and Gordon McDonald, D. (2007). Research to guide use of barriers, traps, and fishways to control sea lamprey. J. Gt. Lakes. Res. 33, 7–19. doi:10.3394/0380-1330(2007)33[7:rtguob]2.0.co;2
Mehner, T., Ihlau, J., Dörner, H., and Hölker, F. (2005). Can feeding of fish on terrestrial insects subsidize the nutrient pool of lakes? Limnol. Oceanogr. 50, 2022–2031. doi:10.4319/LO.2005.50.6.2022
Miehls, S. M., Johnson, N. S., and Hrodey, P. J. (2017). Test of a nonphysical barrier consisting of light, sound, and bubble screen to block upstream movement of sea lampreys in an experimental raceway. North Am. J. Fish. Manag. 37, 660–666. doi:10.1080/02755947.2017.1308892
Miehls, S., Sullivan, P., Twohey, M., Barber, J., and McDonald, R. (2020). The future of barriers and trapping methods in the sea lamprey (Petromyzon marinus) control program in the Laurentian Great Lakes. Rev. Fish. Biol. Fish. 30, 1–24. doi:10.1007/s11160-019-09587-7
Modesto, V., Ilarri, M., Souza, A. T., Lopes-Lima, M., Douda, K., Clavero, M., et al. (2018). Fish and mussels: Importance of fish for freshwater mussel conservation. Fish. Fish. 19, 244–259. doi:10.1111/FAF.12252
Moraes, K., Souza, A. T., Vašek, M., Bartoň, D., Blabolil, P., Čech, M., et al. (2021). Openness of fish habitat matters: Lake pelagic fish community starts very close to the shore. Water 13, 3291. doi:10.3390/W13223291
Näslund, I., Degerman, E., and Nordwall, F. (1998). Brown trout (Salmo trutta) habitat use and life history in Swedish streams: Possible effects of biotic interactions. Can. J. Fish. Aquat. Sci. 55, 1034–1042. doi:10.1139/F97-313
Nilsson, C., Reidy, C. A., Dynesius, M., and Revenga, C. (2005). Fragmentation and flow regulation of the world’s large river systems. Science 308, 405–408. doi:10.1126/science.1107887
Nobile, A. B., Lima, F. P., Freitas-Souza, D., Queiroz, J., Garcia, D. A. Z., Orsi, M. L., et al. (2018). Distribution of non-native suckermouth armoured catfish Pterygoplichthys ambrosettii in the upper Paraná River basin, Brazil. J. Fish. Biol. 92, 1198–1206. doi:10.1111/jfb.13561
Northcote, T. G. (1984). “Mechanisms of fish migration in rivers,” in Mechanisms of migration in fishes. Editors J. McCleave, J. Dodson, and W. Neill (New York, NY, USA: Plenum), 317–355. doi:10.1007/978-1-4613-2763-9_20
Novinger, D. C., and Rahel, F. J. (2003). Isolation management with artificial barriers as a conservation strategy for cutthroat trout in headwater streams. Conserv. Biol. 17, 772–781. doi:10.1046/J.1523-1739.2003.00472.X
Olden, J. D., Poff, N. L. R., Douglas, M. R., Douglas, M. E., and Fausch, K. D. (2004). Ecological and evolutionary consequences of biotic homogenization. Trends Ecol. Evol. 19, 18–24. doi:10.1016/j.tree.2003.09.010
Olden, J. D., and Rooney, T. P. (2006). On defining and quantifying biotic homogenization. Glob. Ecol. Biogeogr. 15, 113–120. doi:10.1111/J.1466-822X.2006.00214.X
Olsson, I. C., Greenberg, L. A., and Eklöv, A. G. (2001). Effect of an artificial pond on migrating Brown trout smolts. North Am. J. Fish. Manag. 21, 498–506. doi:10.1577/1548-8675(2001)021<0498:eoaapo>2.0.co;2
Parker, A. D., Glover, D. C., Finney, S. T., Rogers, P. B., Stewart, J. G., and Simmonds, R. L. (2015). Direct observations of fish incapacitation rates at a large electrical fish barrier in the Chicago Sanitary and Ship Canal. J. Gt. Lakes. Res. 41, 396–404. doi:10.1016/J.JGLR.2015.03.004
Parker, A. D., Glover, D. C., Finney, S. T., Rogers, P. B., Stewart, J. G., and Simmonds, R. L. (2016). Fish distribution, abundance, and behavioral interactions within a large electric dispersal barrier designed to prevent Asian carp movement. Can. J. Fish. Aquat. Sci. 73, 1060–1071. doi:10.1139/CJFAS-2015-0309/SUPPL_FILE/CJFAS-2015-0309SUPPL.DOCX
Patoka, J., Bláha, M., Kalous, L., and Kouba, A. (2017). Irresponsible vendors: Non-native, invasive and threatened animals offered for garden pond stocking. Aquat. Conserv. Mar. Freshw. Ecosyst. 27, 692–697. doi:10.1002/AQC.2719
Pelicice, F. M., Latini, J. D., and Agostinho, A. A. (2015). Fish fauna disassembly after the introduction of a voracious predator: Main drivers and the role of the invader’s demography. Hydrobiologia 746, 271–283. doi:10.1007/s10750-014-1911-8
Peňáz, M., Kubícek, F., Marvan, P., and Zelinka, M. (1968). Influence of the Vir river valley reservoir on the hydrobiological and ichthyological conditions in the river Svratka. Acta Sci. Nat. Acad. Bohemoslov. 2, 1–60.
Perkin, J. S., Gido, K. B., Cooper, A. R., Turner, T. F., Osborne, M. J., Johnson, E. R., et al. (2015). Fragmentation and dewatering transform Great Plains stream fish communities. Ecol. Monogr. 85, 73–92. doi:10.1890/14-0121.1
Perkin, J. S., and Gido, K. B. (2011). Stream fragmentation thresholds for a reproductive guild of Great Plains fishes. Fisheries 36, 371–383. doi:10.1080/03632415.2011.597666
Persson, L. (1997). Competition, predation and environmental factors as structuring forces in freshwater fish communities: Sumari (1971) revisited. Can. J. Fish. Aquat. Sci. 54, 85–88. doi:10.1139/f96-250
Pfauserová, N., Brabec, M., Slavík, O., Horký, P., Žlábek, V., and Hladík, M. (2022). Effects of physical parameters on fish migration between a reservoir and its tributaries. Sci. Rep. 12, 8612. doi:10.1038/s41598-022-12231-3
Pfauserová, N., Slavík, O., Horký, P., Kolářová, J., and Randák, T. (2019). Migration of non-native predator asp (Leuciscus aspius) from a reservoir poses a potential threat to native species in tributaries. Water 11, 1306. doi:10.3390/w11061306
Pfauserová, N., Slavík, O., Horký, P., Turek, J., and Randák, T. (2021). Spatial distribution of native fish species in tributaries is altered by the dispersal of non-native species from reservoirs. Sci. Total Environ. 755, 143108. doi:10.1016/j.scitotenv.2020.143108
Poff, N. L. R., Olden, J. D., Merritt, D. M., and Pepin, D. M. (2007). Homogenization of regional river dynamics by dams and global biodiversity implications. Proc. Natl. Acad. Sci. U. S. A. 104, 5732–5737. doi:10.1073/pnas.0609812104
Preece, R. M., and Jones, H. A. (2002). The effect of keepit dam on the temperature regime of the namoi river, Australia. River Res. Appl. 18, 397–414. doi:10.1002/RRA.686
Pringle, C. M., Freeman, M. C., and Freeman, B. J. (2000). Regional effects of hydrologic alterations on riverine macrobiota in the New World: Tropical-temperate comparisons. Bioscience 50, 807–823. doi:10.1641/0006-3568(2000)050[0807:reohao]2.0.co;2
Quintana, X. D., Arim, M., Badosa, A., María Blanco, J., Boix, D., Brucet, S., et al. (2015). Predation and competition effects on the size diversity of aquatic communities. Aquat. Sci. 77, 45–57. doi:10.1007/s00027-014-0368-1
Quist, M. C., Hubert, A. H., and Rahel, F. J. (2005). Fish assemblage structure following impoundment of a Great plains river | request PDF. West. North Am. Nat. 65, 53–63. Available at: https://www.researchgate.net/publication/286959005_Fish_assemblage_structure_following_Impoundment_of_a_Great_Plains_river (Accessed June 9, 2022).
Rahel, F. J. (2007). Biogeographic barriers, connectivity and homogenization of freshwater faunas: it’s a small world after all. Freshw. Biol. 52, 696–710. doi:10.1111/J.1365-2427.2006.01708.X
Rahel, F. J. (2013). Intentional fragmentation as a management strategy in aquatic systems. Bioscience 63, 362–372. doi:10.1525/BIO.2013.63.5.9
Reid, A. J., Carlson, A. K., Creed, I. F., Eliason, E. J., Gell, P. A., Johnson, P. T. J., et al. (2019). Emerging threats and persistent conservation challenges for freshwater biodiversity. Biol. Rev. 94, 849–873. doi:10.1111/brv.12480
Říha, M., Hladík, M., Mrkvička, T., Prchalová, M., Čech, M., Drastik, V., et al. (2013). Post-spawning dispersal of tributary spawning fish species to a reservoir system. Folia Zool. 62, 1–13. doi:10.25225/fozo.v62.i1.a1.2013
Říha, M., Kubečka, J., Vašek, M., Seďa, J., Mrkvička, T., Prchalová, M., et al. (2009). Long-term development of fish populations in the Římov Reservoir. Fish. Manag. Ecol. 16, 121–129. doi:10.1111/j.1365-2400.2008.00650.x
Roscoe, D. W., and Hinch, S. G. (2010). Effectiveness monitoring of fish passage facilities: Historical trends, geographic patterns and future directions. Fish. Fish. 11, 12–33. doi:10.1111/j.1467-2979.2009.00333.x
Sabatini, A., Podda, C., Frau, G., Cani, M. V., Musu, A., Serra, M., et al. (2018). Restoration of native Mediterranean Brown trout Salmo cettii Rafinesque, 1810 (Actinopterygii: Salmonidae) populations using an electric barrier as a mitigation tool. Eur. Zool. J. 85, 137–149. doi:10.1080/24750263.2018.1453554
Sandlund, O. T., Museth, J., and Øistad, S. (2016). Migration, growth patterns, and diet of pike (Esox lucius) in a river reservoir and its inflowing river. Fish. Res. 173, 53–60. doi:10.1016/J.FISHRES.2015.08.010
Schaeffer, J. S., and Margraf, F. J. (1987). Predation on fish eggs by white perch, Morone americana, in Western Lake Erie. Environ. Biol. Fishes 18, 77–80. doi:10.1007/bf00002331
Schiemer, F., and Waidbacher, H. (1992). “Strategies for conservation of a Danubian fish fauna,” in River conservation and management. Editors P. J. Boon, P. Calow, and G. J. Petts (New York: John Wiley and Sons), 363–382.
Schleuter, D., and Eckmann, R. (2008). Generalist versus specialist: The performances of perch and ruffe in a lake of low productivity. Ecol. Freshw. Fish. 17, 86–99. doi:10.1111/J.1600-0633.2007.00262.X
Schneider, E. V. C., Hasler, C. T., and Suski, C. D. (2018). Fish behavior in elevated co2: Implications for a movement barrier in flowing water. Biol. Invasions 20, 1899–1911. doi:10.1007/s10530-018-1669-4
Seďa, J., and Kubečka, J. (1997). Long-term biomanipulation of rímov reservoir (Czech republic). Hydrobiologia 345, 95–108. doi:10.1023/A:1002911103252
Sepulveda, A. J., Rutz, D. S., Ivey, S. S., Dunker, K. J., and Gross, J. A. (2013). Introduced northern pike predation on salmonids in southcentral Alaska. Ecol. Freshw. Fish. 22, 268–279. doi:10.1111/eff.12024
Shelton, J. M., Samways, M. J., and Day, J. A. (2015). Predatory impact of non-native rainbow trout on endemic fish populations in headwater streams in the Cape Floristic Region of South Africa. Biol. Invasions 17, 365–379. doi:10.1007/s10530-014-0735-9
Silbernagel, J. J., and Sorensen, P. W. (2013). Direct field and laboratory evidence that a combination of egg and larval predation controls recruitment of invasive common carp in many lakes of the upper Mississippi River basin. Trans. Am. Fish. Soc. 142, 1134–1140. doi:10.1080/00028487.2013.788889
Silva, G. G., Weber, V., Green, A. J., Hoffmann, P., Silva, V. S., Volcan, M. V., et al. (2019). Killifish eggs can disperse via gut passage through waterfowl. Ecology 100, e02774. doi:10.1002/ECY.2774
Silva-Sene, A. M., Loures, R. C., de Andrade Neto, F. R., and Pompeu, P. S. (2022). Fish assemblage distribution along free-flowing rivers entering a reservoir cascade. Environ. Biol. Fishes 105, 1535–1547. doi:10.1007/s10641-022-01345-2
Simberloff, D. (2013). Invasive species: What everyone needs to know. 1st ed. New York: Oxford University Press.
Skov, C., Baktoft, H., Brodersen, J., Brönmark, C., Chapman, B. B., Hansson, L.-A., et al. (2011). Sizing up your enemy: Individual predation vulnerability predicts migratory probability. Proc. Biol. Sci. 278, 1414–1418. doi:10.1098/rspb.2010.2035
Skov, C., Brodersen, J., Nilsson, P. A., Hansson, L. A., and Brönmark, C. (2008). Inter- and size-specific patterns of fish seasonal migration between a shallow lake and its streams. Ecol. Freshw. Fish. 17, 406–415. doi:10.1111/j.1600-0633.2008.00291.x
Slavík, O., Horký, P., and Bartoš, L. (2009). Occurrence of cyprinids in fish ladders in relation to flow. Biol. Bratisl. 64, 999–1004. doi:10.2478/s11756-009-0157-9
Šmejkal, M., Baran, R., Blabolil, P., Vejřík, L., Prchalová, M., Bartoň, D., et al. (2017). Early life-history predator-prey reversal in two cyprinid fishes. Sci. Rep. 7, 6924. doi:10.1038/s41598-017-07339-w
Šmejkal, M., Bartoň, D., Brabec, M., Sajdlová, Z., Souza, A. T., Moraes, K. R., et al. (2021). Climbing up the ladder: Male reproductive behaviour changes with age in a long-lived fish. Behav. Ecol. Sociobiol. 75, 22–13. doi:10.1007/s00265-020-02961-7
Šmejkal, M., Ricard, D., Prchalová, M., Říha, M., Muška, M., Blabolil, P., et al. (2015). Biomass and abundance biases in European standard gillnet sampling. PLoS One 10, e0122437. doi:10.1371/journal.pone.0122437
Šmejkal, M., Souza, A. T., Blabolil, P., Bartoň, D., Sajdlová, Z., Vejřík, L., et al. (2018). Nocturnal spawning as a way to avoid egg exposure to diurnal predators. Sci. Rep. 8, 15377. doi:10.1038/s41598-018-33615-4
Sparks, R. E., Barkley, T. L., Creque, S. M., Dettmers, J. M., and Stainbrook, K. M. (2010). Evaluation of an electric fish dispersal barrier in the Chicago Sanitary and ship canal. Am. Fish. Soc. Symp. 74, 139–161.
Specziár, A., and Rezsu, E. T. (2009). Feeding guilds and food resource partitioning in a lake fish assemblage: An ontogenetic approach. J. Fish. Biol. 75, 247–267. doi:10.1111/j.1095-8649.2009.02283.x
Stamplecoskie, K. M., Thomas, R. B., Lower, N., Cottenie, K., Mc Laughlin, R. L., and Mc Donald, D. G. (2012). Response of migratory sea lampreys to artificial lighting in portable traps. North Am. J. Fish. Manag. 32, 563–572. doi:10.1080/02755947.2012.675963
Sugunan, V. V. (1995). Reservoir fisheries of India. Rome: FAO, 423. FAO Fisheries Technical Paper. No. 345.
Sun, J., Tummers, J. S., Galib, S. M., and Lucas, M. C. (2022). Fish community and abundance response to improved connectivity and more natural hydromorphology in a post-industrial subcatchment. Sci. Total Environ. 802, 149720. doi:10.1016/J.SCITOTENV.2021.149720
Suski, C. D. (2020). Development of carbon dioxide barriers to deter invasive fishes: Insights and lessons learned from bigheaded carp. Fishes 5, 25. doi:10.3390/FISHES5030025
Tamario, C., Degerman, E., Polic, D., Tibblin, P., and Forsman, A. (2021). Size, connectivity and edge effects of stream habitats explain spatio-temporal variation in Brown trout (Salmo trutta) density. Proc. R. Soc. B 288, 20211255. doi:10.1098/RSPB.2021.1255
Tamario, C., Sunde, J., Petersson, E., Tibblin, P., and Forsman, A. (2019). Ecological and evolutionary consequences of environmental change and management actions for migrating fish. Front. Ecol. Evol. 7. doi:10.3389/fevo.2019.00271
Tapkir, S., Boukal, D., Kalous, L., Bartoň, D., Souza, A. T., Kolar, V., et al. (2022). Invasive gibel carp (Carassius gibelio) outperforms threatened native crucian carp (Carassius carassius) in growth rate and effectiveness of resource use: Field and experimental evidence. Aquat. Conserv. Mar. Freshw. Ecosyst. 32, 1901–1912. doi:10.1002/aqc.3894
Taylor, M. K., and Cooke, S. J. (2012). Meta-analyses of the effects of river flow on fish movement and activity. Environ. Rev. 20, 211–219. doi:10.1139/A2012-009/SUPPL_FILE/A2012-009SUPPL.DOCX
Taylor, R. M., Pegg, M. A., and Chick, J. H. (2005). Response of bighead carp to a bioacoustic behavioural fish guidance system. Fish. Manag. Ecol. 12, 283–286. doi:10.1111/j.1365-2400.2005.00446.x
Temming, A., Floeter, J., and Ehrich, S. (2007). Predation hot spots: Large scale impact of local aggregations. Ecosystems 10, 865–876. doi:10.1007/s10021-007-9066-3
Thompson, P. D., and Rahel, F. J. (1998). Evaluation of artificial barriers in Small Rocky Mountain streams for preventing the upstream movement of brook trout. North Am. J. Fish. Manag. 18, 206–210. doi:10.1577/1548-8675(1998)018<0206:EOABIS>2.0.CO;2
Tonitto, C., and Riha, S. J. (2016). Planning and implementing small dam removals: Lessons learned from dam removals across the eastern United States. Sustain. Water Resour. Manag. 2, 489–507. doi:10.1007/s40899-016-0062-7
Troia, M. J., and Gido, K. B. (2014). Towards a mechanistic understanding of fish species niche divergence along a river continuum. Ecosphere 5, art41–18. doi:10.1890/ES13-00399.1
Turgeon, K., Turpin, C., and Gregory-Eaves, I. (2019). Dams have varying impacts on fish communities across latitudes: A quantitative synthesis. Ecol. Lett. 22, 1501–1516. doi:10.1111/ELE.13283
Van Den Berg, C., Van Snik, G. J. M., Van Den Boogaart, J. G. M., Sibbing, F. A., and Osse, J. W. M. (1994). Comparative microanatomy of the branchial sieve in three sympatric cyprinid species, related to filter-feeding mechanisms. J. Morphol. 219, 73–87. doi:10.1002/JMOR.1052190109
Van Looy, K., Tormos, T., and Souchon, Y. (2014). Disentangling dam impacts in river networks. Ecol. Indic. 37, 10–20. doi:10.1016/J.ECOLIND.2013.10.006
Vannote, R. L., Wayne Minshall, G., Cummins, K. W., Seddel, J. R., and Cushing, C. E. (1980). The river continuum concept. Can. J. Fish. Aquat. Sci. 37, 130–137. doi:10.1139/f80-017
Vašek, M., Jarolím, O., Čech, M., Kubečka, J., Peterka, J., and Prchalová, M. (2008). The use of pelagic habitat by cyprinids in a deep riverine impoundment: Římov Reservoir, Czech Republic. Folia Zool. 57, 324–336.
Vehanen, T., Mäki-Petäys, A., Aspi, J., and Muotka, T. (1999). Intercohort competition causes spatial segregation in Brown trout in artificial streams. J. Fish. Biol. 55, 35–46. doi:10.1111/j.1095-8649.1999.tb00654.x
Vetter, B. J., Cupp, A. R., Fredricks, K. T., Gaikowski, M. P., and Mensinger, A. F. (2015). Acoustical deterrence of silver carp (Hypophthalmichthys molitrix). Biol. Invasions 17, 3383–3392. doi:10.1007/s10530-015-0964-6
Victor, R., and Tetteh, J. O. (1988). Fish communities of a perturbed stream in Southern Nigeria. J. Trop. Ecol. 4, 49–59. doi:10.1017/S0266467400002492
Volta, P., Jeppesen, E., Leoni, B., Campi, B., Sala, P., Garibaldi, L., et al. (2013). Recent invasion by a non-native cyprinid (common bream Abramis brama) is followed by major changes in the ecological quality of a shallow lake in southern Europe. Biol. Invasions 15, 2065–2079. doi:10.1007/s10530-013-0433-z
Wagner, C. M., Stroud, E. M., and Meckley, T. D. (2011). A deathly odor suggests a new sustainable tool for controlling a costly invasive species. Can. J. Fish. Aquat. Sci. 68, 1157–1160. doi:10.1139/F2011-072/ASSET/IMAGES/LARGE/F2011-072F2.JPEG
Wang, L., Infante, D., Lyons, J., Stewart, J., and Cooper, A. (2011). Effects of dams in river networks on fish assemblages in non-impoundment sections of rivers in Michigan and Wisconsin, USA. River Res. Appl. 27, 473–487. doi:10.1002/RRA.1356
Ward, J. V., and Stanford, J. A. (1995). Ecological connectivity in alluvial river ecosystems and its disruption by flow regulation. Regul. Rivers Res. Manag. 11, 105–119. doi:10.1002/rrr.3450110109
Weyl, O. L. F., Daga, V. S., Ellender, B. R., and Vitule, J. R. S. (2016). A review of Clarias gariepinus invasions in Brazil and South Africa. J. Fish. Biol. 89, 386–402. doi:10.1111/JFB.12958
Weyl, O. L. F., Finlayson, B., Impson, N. D., Woodford, D. J., and Steinkjer, J. (2014). Threatened endemic fishes in SouthSouth Africa’s cape floristic region: A new beginning for the rondegat river. Fisheries 39, 270–279. doi:10.1080/03632415.2014.914924
Wiegleb, J., Hirsch, P. E., Seidel, F., Rauter, G., and Burkhardt-Holm, P. (2021). Flow, force, behaviour: Assessment of a prototype hydraulic barrier for invasive fish. Hydrobiologia 849, 1001–1019. doi:10.1007/s10750-021-04762-z
Winemiller, K. O., and Kelso-Winemiller, L. C. (1994). Comparative ecology of the African pike, Hepsetus odoe, and tigerfish, Hydrocynus forskahlii, in the Zambezi River floodplain. J. Fish. Biol. 45, 211–225. doi:10.1111/J.1095-8649.1994.TB01301.X
Winemiller, K. O., McIntyre, P. B., Castello, L., Fluet-Chouinard, E., Giarrizzo, T., Nam, S., et al. (2016). Balancing hydropower and biodiversity in the amazon, Congo, and mekong. Science 351, 128–129. doi:10.1126/SCIENCE.AAC7082/SUPPL_FILE/WINMEILLER-SM.PDF
Žák, J., Prchalová, M., Šmejkal, M., Blabolil, P., Vašek, M., Matěna, J., et al. (2020). Sexual segregation in European cyprinids: Consequence of response to predation risk influenced by sexual size dimorphism. Hydrobiologia 847, 1439–1451. doi:10.1007/s10750-020-04198-x
Zarfl, C., Lumsdon, A. E., Berlekamp, J., Tydecks, L., and Tockner, K. (2015). A global boom in hydropower dam construction. Aquat. Sci. 77, 161–170. doi:10.1007/s00027-014-0377-0
Zhang, C., Ding, L., Ding, C., Chen, L., Sun, J., and Jiang, X. (2018). Responses of species and phylogenetic diversity of fish communities in the Lancang River to hydropower development and exotic invasions. Ecol. Indic. 90, 261–279. doi:10.1016/J.ECOLIND.2018.03.004
Zielinski, D. P., Hondzo, M., and Voller, V. R. (2014). Mathematical evaluation of behavioral deterrent systems to disrupt fish movement. Ecol. Modell. 272, 150–159. doi:10.1016/J.ECOLMODEL.2013.10.009
Zielinski, D. P., and Sorensen, P. W. (2016). Bubble curtain deflection screen diverts the movement of both Asian and common carp. North Am. J. Fish. Manag. 36, 267–276. doi:10.1080/02755947.2015.1120834
Zielinski, D. P., and Sorensen, P. W. (2015). Field test of a bubble curtain deterrent system for common carp. Fish. Manag. Ecol. 22, 181–184. doi:10.1111/FME.12108
Glossary
Anadromous species fish species spending part of their life cycles in marine environments and reproducing in freshwater
Edge effects interactions among modified and natural environments on their neighboring edges through changes in biotic and abiotic conditions
Generalist species species that can utilize a wide range of ecological conditions and various food sources
Lentic environment a body of standing water
Lotic environment fluvial freshwater environments such as streams and rivers
Potamodromous species fish species spending their whole life cycle in freshwater and typically undergoing only short-distance migration
Rheophilic species fish species that are specialized to live in fast flowing streams and rivers at least for some of their life cycles
River continuum concept continuously integrating series of abiotic and biotic parameters from river spring to its estuary
Keywords: edge effects, habitat fragmentation, non-native species, biodiversity loss, biotic homogenization, freshwater fauna, potamodromous fish, fish migration
Citation: Šmejkal M, Bartoň D, Duras J, Horký P, Muška M, Kubečka J, Pfauserová N, Tesfaye M and Slavík O (2023) Living on the edge: Reservoirs facilitate enhanced interactions among generalist and rheophilic fish species in tributaries. Front. Environ. Sci. 11:1099030. doi: 10.3389/fenvs.2023.1099030
Received: 15 November 2022; Accepted: 09 January 2023;
Published: 19 January 2023.
Edited by:
Stefano Basso, Norwegian Institute for Water Research (NIVA), NorwayReviewed by:
Jason Deboer, Illinois Natural History Survey, United StatesLuca Carraro, Swiss Federal Institute of Aquatic Science and Technology, Switzerland
Copyright © 2023 Šmejkal, Bartoň, Duras, Horký, Muška, Kubečka, Pfauserová, Tesfaye and Slavík. This is an open-access article distributed under the terms of the Creative Commons Attribution License (CC BY). The use, distribution or reproduction in other forums is permitted, provided the original author(s) and the copyright owner(s) are credited and that the original publication in this journal is cited, in accordance with accepted academic practice. No use, distribution or reproduction is permitted which does not comply with these terms.
*Correspondence: Marek Šmejkal, marek.smejkal@hbu.cas.cz