- Key Laboratory of Geographical Processes and Ecological Security in Changbai Mountains, Ministry of Education, School of Geographical Sciences, Northeast Normal University, Changchun, China
Promoting cropland carbon (C) sequestration through straw return has always been the focus of numerous studies. However, there is still a lack of comprehensive understanding of the straw return effects on soil organic carbon (SOC) and soil inorganic carbon (SIC) sequestration. Therefore, the present study aims to investigate the effects of long-term straw return on SOC and SIC sequestration across the 0–100 cm soil profile in the maize planting cropland in Northeastern China. The results showed an increasing trend in SOC contents in the 0–100 cm soil profile following long-term straw return, while significant decreases in SIC contents were observed in the surface (0–20 cm) and subsoil (20–60 cm) layers, respectively. In addition, the SOC stock increased significantly in the subsoil layer following long-term straw return, by an average value of 44%, which is higher than those observed in other soil layers. On the other hand, the SIC stock in the subsoil layer increased by an average value of 24% and decreased in the surface and under-subsoil layers by average values of 53% and 33%, respectively. Moreover, the exchangeable calcium contents were positively correlated with SOC and SIC stock, demonstrating the soil calcium contributes to SOC and SIC sequestration. The present study highlighted the importance of the subsoil layer for effective straw return strategies in cropland to promote SOC and SIC sequestration in croplands.
1 Introduction
Cropland soil is one of the major contributors to carbon (C) sequestration in the terrestrial ecosystem (Amelung et al., 2020; Rodrigues et al., 2021), which may potentially increase the soil organic C (SOC) sequestration by 0.4–1.2 billion tons C/year (Lal, 2011). Since most C in cropland soils is accumulated in the SOC form, providing numerous benefits and partially offsetting anthropogenic C (Guo and Gifford, 2002). Previous studies have mainly focused on promoting SOC sequestration, suggesting deeper soils may have great potential than surface soil layer (Rumpel and Koegel-Knabner, 2011; Button et al., 2022). However, soil inorganic C (SIC) sequestration in cropland has attracted less attention from researchers.
Deeper soil layers (>20 cm) generally contain more than 50% of the global SOC reserves (Rumpel and Koegel-Knabner, 2011). Indeed, the SOC dynamics between deeper and surface soil layers are controlled by different mechanisms (Mulder et al., 2015). Compared to surface soils, deeper soils are characterized by reduced plant residue inputs, decreased substrate availability, lower microbial biomass and activities, a greater abundance of available minerals, and prevailing oligotrophic conditions (Button et al., 2022). The SOC sequestration is controlled by the balance between C inputs and outputs from plant residues under constant long-term environmental and management conditions (Feng et al., 2022). In addition, previous studies have confirmed that secondary clay minerals and short-range-ordered iron (Fe) and aluminum (Al) phases are critical contributors to SOC accumulation (Chen et al., 2020). Moreover, exchangeable calcium (Caex) is prevalent in soils and has been considered an important factor in the mechanism of SOC stabilization in neutral and calcareous soils (He et al., 2022). On the other hand, the SIC accounts for nearly 40% of the C reserves worldwide and is considered also an important cropland soil C pool (Lal, 2004; Rowley et al., 2018; Kim et al., 2020). Indeed, recent studies have confirmed that intensive agricultural activities have accelerated the weathering and dissolution processes of SIC (Kim et al., 2020). The soil acidification, excessive nitrogen (N) fertilization, and water balance are the main factors leading to dramatic losses of SIC in cropland soil (Tao et al., 2022). According to previous studies, 7% of the SIC reserves in the 0–40 cm soil layer were lost, corresponding to 1.1 Mg/ha, and 7 million ha of cropland has become carbonate free in China over the 1980–2020 period (Raza et al., 2020). The SIC accumulation and distribution vary considerably with soil depth, depending on soil parent material, the partial pressure of CO2 in soil, soil pH, soil moisture, and cation contents (such as Ca2+ or Mg2+) (Kim et al., 2020; Tao et al., 2022).. Calcium carbonate (CaCO3) is the main existing form of SIC, hence the calcium source plays an important role and determines whether carbonate can be formed (Sun et al., 2022; Wang et al., 2014). SIC in the surface soil layer may be susceptible to leaching and precipitation into deeper soil layers under high soil water fluxes, CO2 degassing, and acidic conditions (Zhao X. et al., 2020). According to the report that the SIC density have decreased by 38% and 27% at depths of 0–20 cm and 20–40 cm, respectively during the past 30 years (Tao et al., 2022). Therefore, determining the differences in SOC and SIC sequestration between surface and deeper soil layers and providing a basis for evaluating the effectiveness of practices to promote C sequestration in the soil profile.
Straw return is one of the agricultural practices which maintains or enhances the fertility and productivity of soils (Yang et al., 2022b). However, the contribution of the straw return practice to SOC formation and accumulation remains uncertain. Long-term experimental field studies demonstrated the benefits of the straw return practice in SOC sequestration, showing significant increases in SOC contents in the surface soil layer by an average of 12% following straw return (Liu C. et al., 2014). The fragmentation of plant detritus from straw return results in the formation of the particulate SOC, enhancing soil carbon retention (Witzgall et al., 2021). Moreover, the straw return could provide favorable water, nutrients, and thermal conditions, promoting microbial growth and thus enhancing SOC accumulation in cropland (Turmel et al., 2015). Indeed, previous studies have demonstrated the important role of straw return in maintaining SOC storage without regard to the straw residue amounts (Yang et al., 2022a), depending on soil type, environmental factors (i.e., temperature and moisture), physicochemical characteristics (i.e., texture, pH, and total N), and biological characteristics (i.e., microbial community composition and abundance) (Berhane et al., 2020). There are many differences in environmental factors, physicochemical, and biological characteristics between surface and deeper soil layers. Therefore, the contribution of the straw return to SOC sequestration in the deeper soil layer cannot be directly determined based on the characteristics of the surface soil layer. There are limited studies on the assessment of SIC contribution to soil C pool, and the impacts of straw return in promoting SOC and SIC sequestration between surface and deeper soil layers remains unclear.
In this study, pairwise sampling was carried out to compare SOC and SIC sequestration across the 0–100 cm soil profile in the continuous more than ten years straw return fields in northeastern China. The main objectives of this study were to: 1) assess the distribution characteristics of SOC and SIC contents in the soil profiles; 2) determine the difference in SOC and SIC sequestration in the soil profiles; (iii) identify the environmental factors that promote SOC and SIC sequestration in long-term straw return cropland.
2 Materials and methods
2.1 Study sites
This study was based on spring maize cropping region with straw return at the Lishu county in Jilin Province, northeastern China. This region is a typical spring maize-growing area in the Golden Maize Belt of China (Lv et al., 2015). The mean annual precipitation and temperature of about 614 mm and 6.9°C, respectively. In this study, four pairs of study sites were selected for investigating the straw return effects on SOC and SIC sequestration, the basic information as shown in Table 1. There are two pairs of locations at each site, we chose one conventional fertilization cropland as control treatment (CT) and one conventional fertilization plus long-term straw return as straw return treatment (SR). In study area, spring maize is typically planted in early May and harvested early October. The conventional fertilizers amount about were: N 240 kg/ha, P2O5 110 kg/ha, and K2O 110 kg/ha. The total amount of maize straw return that remained at harvest every year was about 7.5 t/ha, maize straw was directly mulched on cropland after harvest for every year. All agronomic practices were consistent with local farmer practices.
2.2 Soil sampling
In April 2021, we sampled one 100-cm deep soil core from each quadrat using a soil auger with a 38 mm diameter. Each quadrat was repeated three times for every treatment. Stratified soil samples were collected from three intervals taken from the soil horizon at 0–20 cm (surface layer), 20–60 cm (subsoil layer), and 60–100 cm (under-subsoil layer). These intervals of soil horizon were divided according to the degree of agricultural disturbance. In order to avoid cross-contamination between different sampling plots, the soil auger was cleaned at least three times before each sampling until completely removing the attached residues to the soil auger using purified water (neutral).
2.3. Soil physicochemical properties
The collected soil samples were first homogenized and air-dried immediately after sampling, then sieved through a 2 mm sieve to remove roots and impurities. Soil pH was measured in a 1:2.5 soil-deionized water ratio after equilibration for 30 min. Total C and total N contents in the soil samples were determined using an elemental analyzer (Euro Vector 3000, Italy), following drying at 60°C to a constant weight and homogenization with a ball mill (Retsch MM301, Germany). The SOC contents were determined using the potassium dichromate oxidation method, while SIC was calculated as the difference between total C and SOC. Soil exchangeable calcium (Caex) was extracted with 1 mol/L ammonium acetate (pH 7.0), and measured by an inductively coupled plasma-optical emission spectrometer (ICP-OES, Avio200, PerkinElmer, United States). In addition, soil texture was measured using a laser diffraction particle size analyzer (Microtrac S3500, United States). On the other hand, edaphic parameters, namely soil temperature, moisture, and salinity, were measured using a calibrated Frequency Domain Reflectometry (FDR) (WITU Agricultural Technology, Shenyang, China). To estimate soil bulk density (BD) in the study sites, according to (Song et al., 2005):
where SOC denote SOC contents (g/kg).
2.4 SOC and SIC stock calculation
The SOC and SIC stock were estimated based on SOC and SIC contents using the following formulas (You et al., 2020):
where SOC and SIC denote SOC and SIC contents (g/kg); BD denotes the soil bulk density (g/cm3); D is the soil depths (cm).
2.5 Statistical analysis
The collected data were first assessed for normality and homogeneity using the Shapiro–Wilk test, then the independent t-test was performed to assess statistical differences in SOC and SIC contents (stock) between the long-term straw return and the control treatment. In addition, the relationships between SOC and SIC contents in different soil layers were assessed using the Pearson correlation. Differences and correlations were considered significant at p < 0.05. All statistical tests were performed using SPSS 22.0 (SPSS Inc. Chicago, IL, United States), while the obtained results were plotted using OriginPro 2019 (OriginLab, Northampton, MA, United States). Redundancy analysis (RDA, Canoco five software) was performed to determine the correlations between environmental parameters, SOC stock, and SIC stock.
3 Results
3.1 Distribution of SOC and SIC contents
The obtained results revealed increasing trends in SOC contents in cropland following long-term straw return (Figures 1A–1). The SOC contents in surface, subsoil, and under-subsoil layers increased by average values of 35%, 45%, and 33% over control treatment. In long-term straw return, the SOC ranged from 4.89 g/kg to 17.47 g/kg with average value of 9.74 ± 3.81 g/kg in the surface layer, 0.94 g/kg to 11.77 g/kg with average value of 5.01 ± 2.72 g/kg in the subsoil layer, and 0.70 g/kg to 6.06 g/kg with average value of 2.50 ± 1.24 g/kg in the under-subsoil layer. In control treatment, the SOC contents ranged from 3.48 g/kg to 12.46 g/kg with average value of 7.24 ± 2.99 g/kg in surface layer, 0.92 g/kg to 8.35 g/kg with average value of 3.45 ± 2.01 g/kg in subsoil layer, and 0.23 g/kg to 4.69 g/kg with average value of 1.89 ± 1.32 g/kg in sunder-subsoil layer. The results revealed differences in the increase in SOC contents between soil layers, showing the greatest increase in the subsoil layer and minimum increase in the under-subsoil layer.
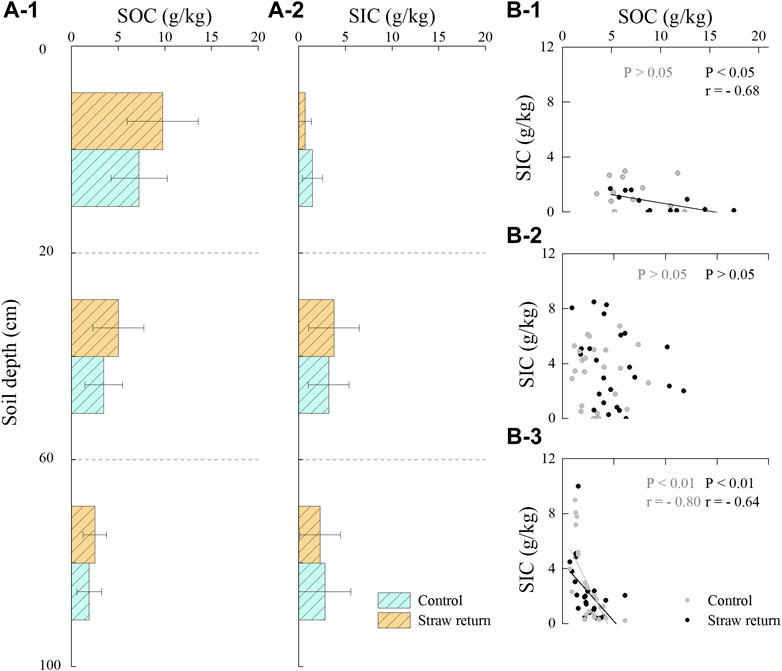
FIGURE 1. Vertical distribution of SOC (a-1) and SIC contents (a-2); Relationships between SOC and SIC contents in the surface (b-1), subsoil (b-2), and under-subsoil (b-3) layers. Bars indicate mean ± SD values (n = 3); * denotes p < 0.05.
On the other hand, the SIC contents exhibited decreasing/increasing trends in cropland after long-term straw return (Figures 1A, 2). The results showed decreases in the SIC contents in the surface soil and under-subsoil layers by average values of 53% and 19%, but increases in the subsoil layers by average values of 17% over control treatment. In long-term straw return, the SIC contents ranged from 0 g/kg to 1.71 g/kg with average value of 0.69 ± 0.67 g/kg in surface layer, 0 g/kg to 8.50 g/kg with average value of 3.76 ± 2.71 g/kg in subsoil layer, and 0.33 g/kg to 10.01 g/kg with average value of 2.28 ± 2.16 g/kg in under-subsoil layer. In control treatment, the SIC contents ranged from 0 g/kg to 2.99 g/kg with average value of 0 g/kg to 1.47 ± 1.08 g/kg in surface layer, 0.22 g/kg to 8.99 g/kg with average value of 3.21 ± 2.19 g/kg in subsoil layer, and 0.33 g/kg to 10.01 g/kg with average value of 2.80 ± 2.79 g/kg in under-subsoil layer. In addition, the results revealed an increase in SIC contents in long-term straw return mainly in the subsoil layer.
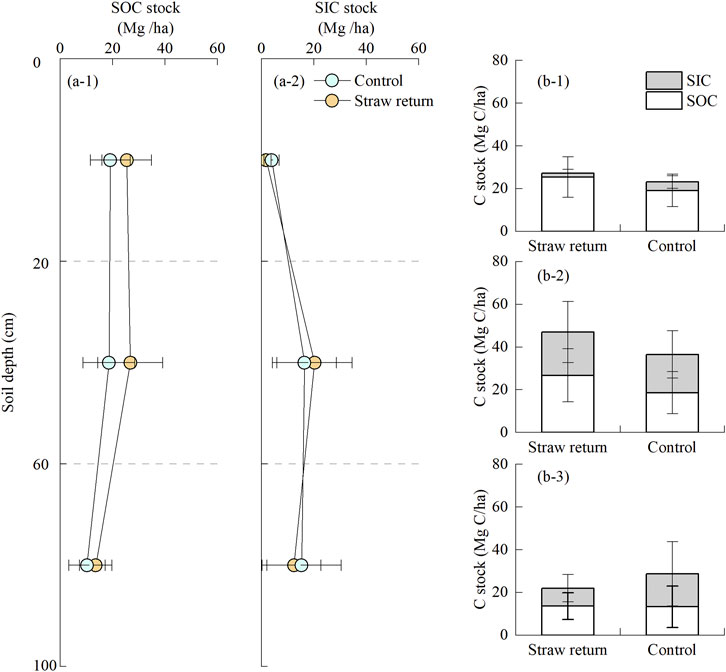
FIGURE 2. Vertical distribution of SOC and SIC stock in straw return (a-1) and control (a-2) scenarios; SOC and SIC stock in the surface (b-1), subsoil (b-2), and under-subsoil (b-3) layers. Bars indicate mean ± SD values (n = 3).
The correlation results revealed differences in correlation coefficients between SOC and SIC contents between soil layers (Figures 1B–1, B–2, B–3). Indeed, SOC and SIC contents showed a significant negative correlation coefficient in surface (at p < 0.05, r = −0.68 in the long-term straw return scenarios) and under-subsoil layers (at p < 0.01, r = −0.64 in the long-term straw return scenarios, r = −0.80 in the control scenarios). Whereas no significant correlation between SOC and SIC contents was observed in the subsoil layer, however, it suggests some intriguing possibility of the co-sequestration relationship between SOC and SIC.
3.2 Promotion of SOC and SIC sequestration in different soil profiles
The results of SOC stock in the 0–100 cm soil profiles suggested greater sequestration after long-term straw return (Figure 2). In the surface soil layer, the SOC stock increased by an average value of 33% and the SIC stock was decreased by an average value of 53% after long-term straw return in comparison to the control treatment. In the subsoil, the SOC stock increased by an average value of 44% and SIC stock increased by an average value of 24% over control treatment. While in the subsoil layer, the SOC stock increased by an average value of 32% and the SIC stock was decreased by an average value of 19% after long-term straw return.
The results revealed significantly higher total C, SOC, and SIC stock in the subsoil layer than those revealed in the surface and under subsoil layers (Figure 3). In the long-term straw return, the total C, SOC, and SIC stock of the subsoil layer accounted for 48%, 27%, and 21% to the total C stock in 0–100 cm soil profile (Figure 3A). In the control treatment, the total C, SOC, and SIC stock in the subsoil layer accounted for 41%, 22%, and 19% to the total C stock in 0–100 cm soil profile (Figure 3B).
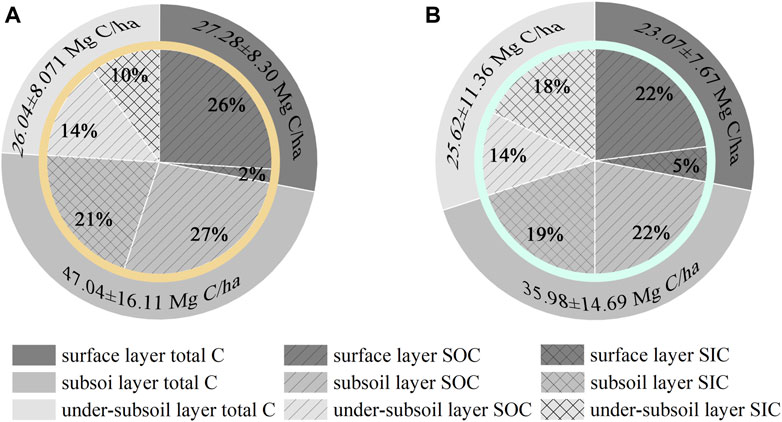
FIGURE 3. Contribution of total C, SOC, and SIC in each layer to the total C stock in the entire soil profile (0–100 cm) in long-term straw return (A) and control treatment(B).
3.3 Factors influencing SOC and SIC sequestration
The RDA analysis demonstrated variations in the influences of factors on SOC and SIC stock with soil depths (Figure 4). The results showed increases in the SOC stock in the 0–100 cm soil profiles with the increasing straw return, clay, total N, salinity, and Caex contents, while decreases in the SOC stock was observed with increasing pH and temperature. On the other hand, SIC stock increased with increasing pH, temperature, sand, and Caex contents and decreased with increasing the straw return, clay, total N, and salinity of the soil. For difference soil layers, the results showed increases in SIC stock with straw return in the subsoil layer, while decreases in SIC stock was observed in surface and under-subsoil layers. In addition, SIC stock in deeper soil layers (subsoil and under-subsoil layers) were strongly and positively influenced by Caex and pH compared to those in the surface layer, while SOC stock was strongly and positively influenced mainly by Caex, particularly in deeper soil layers.
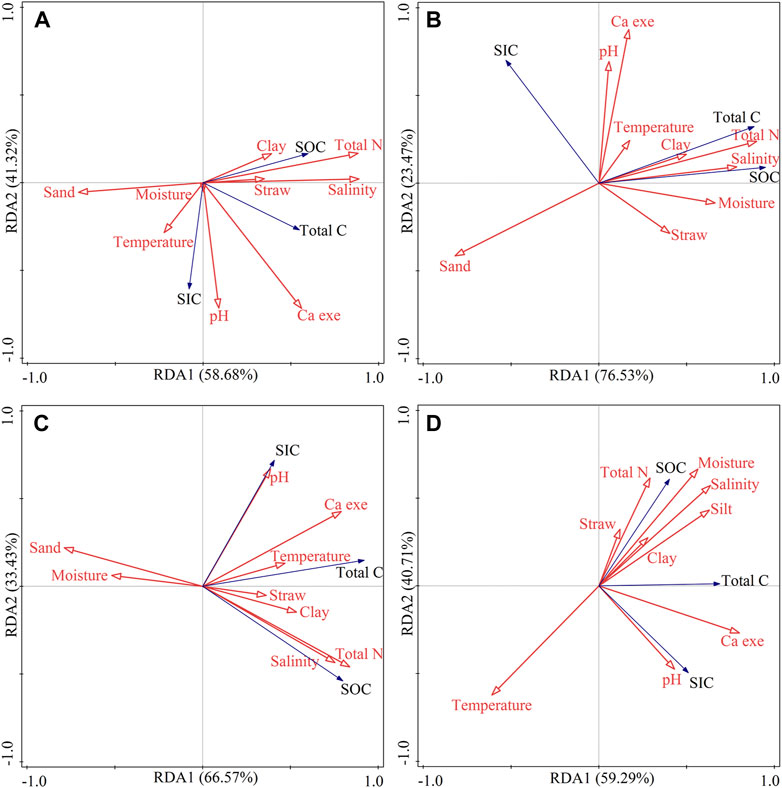
FIGURE 4. Redundancy analysis (RDA) results of the effects of environmental factors on SOC and SIC stock in the entire soil profile (0–100 cm) (A), surface layer (B), subsoil layer (C), and under-subsoil layer (D).
The Pearson’s correlation result showed that Caex contents were positively correlated with SOC (at p < 0.05) and SIC (at p < 0.001) stock (Figure 5). The SOC stock increased with increasing total N, silt, and salinity (at p < 0.001), while the SIC stock gradually increased with increasing soil pH (at p < 0.001).
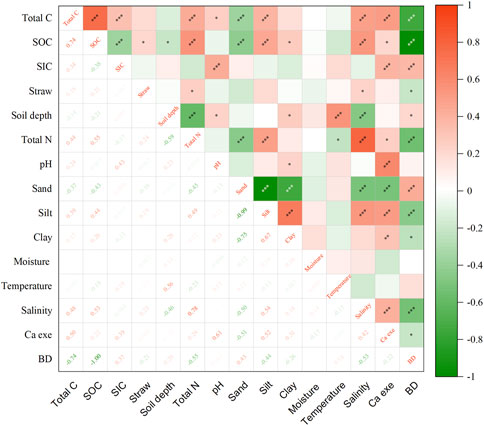
FIGURE 5. Pairwise comparisons of environmental factors, SOC stock, and SIC stock; Color gradients represent Pearson’s correlation coefficients. *, **, and *** denote significant correlation coefficient at p < 0.05, p < 0.01, and p < 0.001, respectively.
4 Discussion
Our results demonstrated that long-term straw return could promote SOC sequestration. The results revealed increases in SOC contents in the 0–100 cm soil profiles by an average value range of 33%–45% (Figure 1). The increases in SOC contents were slightly higher than those reported in previous studies (Berhane et al., 2020; Liu C. et al., 2014). Indeed, previous studies have shown increases in SOC contents in the surface soil layer by more than 12% following straw return in the rice paddy and upland fields through a global scale meta-analysis, taking into account different durations and cropping systems (Berhane et al., 2020). Their results revealed increases in SOC contents in upland and rice paddy fields following straw return by 17.0% and 11.4%, respectively (Xia et al., 2018). The long-term straw return (over 4 years) resulted in increases in the SOC contents by a range value of 15.89%–43.19%, with an average value of 27.7%, which is significantly higher than those observed in a short-term return field (<4 years), showing increases by a range value of 11.2%–15.67%, with an average value of 13.4% (Xia et al., 2018). By comparing SOC stock in different soil layers, it was observed greatest increases in SOC stock in the subsoil layer by an average value of 44% following long-term straw return compared to control scenarios (Figure 2). The gradual decrease in the rate of plant C inputs along the soil profile, moreover, reduced soil disturbance, aeration, and microbial activity in deeper soil layers (Button et al., 2022), that might also result in longer SOC residence times in deeper soil layers than in the surface layer. On the other hand, previous empirical studies have demonstrated strong effects of mineral protection on SOC in deeper soil layers (He et al., 2022), where Caex and Fe/Al oxide contents were higher than in the surface layer (Chen et al., 2021). Moreover, it has been reported that clay content was positively correlate with bacterial diversity (Xia et al., 2020) and soil texture could affect both the decomposition of plant litter and the retention SOC in the soil (Angst et al., 2021). The soil salinity could alter bacterial network modularity and leads to spatial distribution in cropland soils (Zhao S. et al., 2020). In support of above speculation, the results of the present study revealed consistent findings, showing significant effects of soil clay, silt, total N, and salinity on SOC stock (Figure 4, 5).
In addition, our results revealed different effects of long-term straw return along the soil profile. The SIC contents decreased in surface by average values of 53% and increased in subsoil layers by average values of 17% (Figure 1). Previous studies have shown significant decreases in SIC contents in the extensive cropland soils in the eastern part of Northern China (Wu et al., 2009). The loss of carbonates from surface soil layers is one of the serious consequences of acidification (Zamanian and Kuzyakov, 2019). Indeed, water balance and soil acidification could be the main factors causing dramatic losses in the SIC stock in cropland (Tao et al., 2022). It has been reported that SIC contents were negatively correlated with groundwater recharge in the Chinese Loess Plateau (Liu W. et al., 2014). In addition, the results of previous studies revealed losses in the SIC storage in the 0–7.3 m soil profile under rainfed and irrigated cropping systems estimated at 328 Mg/ha and 730 Mg/ha, respectively, compared to the SIC storage in native vegetation fields (Kim et al., 2020). On the other hand, a global assessment showed that N fertilization-induced soil acidification increased the released CO2 amount, reaching 7.48 Tg/year (Zamanian et al., 2018). Contrary to cropland soils that showed pH values greater than 7, Deng et al. (2022) was demonstrated that straw return could significantly decrease soil pH (Deng et al., 2022). These findings are consistent with those revealed in the present study, showing positive correlations between the between the SIC stock and pH values, respectively (Figure 4, 5). The comparison of the long-term straw return effects on the SIC stock in different soil layers indicated that the subsoil layer has great potential for SIC sequestration. Indeed, the SIC stock was increased 24% in the subsoil layer, while decreased by average values of 53% in surface layer, and decreased by average values of 33% in under-subsoil layer over control treatment (Figure 2). Previous studies have shown that calcium sources in deeper soil layers can determine whether carbonate formation results in SIC sequestration (Schlesinger, 2017; Kim et al., 2020). The dissolved calcium ions in the soil may be leached to groundwater or precipitate as carbonate if the calcite solubility constant is reached, decreasing SIC contents in the surface soil layer (Li et al., 2017; Tao et al., 2022), which is consistent with the results of the present study, showing higher Caex contents in the subsoil layer than those in the surface and under-subsoil layers following long-term straw return (Supplementary Table S1).
The effects of straw return on SOC and SIC sequestration are regulated by the combination of many environmental variables. The results showed that Caex contents were positively correlated with SOC and SIC stock, more particularly in the deeper soil layer. Comparable evidence was highlighted, showing a strong positive association of calcium with SOC and SIC in soils (Rowley et al., 2021). Soil calcium can indirectly promote soil aggregation, leading to the accumulation of occluded SOC and forming cationic bridges to mineral-associated SOC (C. Chen et al., 2014; Kalinichev and Kirkpatrick, 2007). Soil calcium constitutes an equally important fraction of SOC in all soil types and under various environmental conditions (Wang et al., 2021). On the other hand, CaCO3 is the most common calcium form of SIC. Indeed, SIC sequestration can be promoted by applying Ca2+ or using feedstocks with a high proportion of Ca2+ 4. Under natural conditions, higher Ca2+ contents are often observed in 30–50 cm soil depth than those in 0–10 cm soil depth (He et al., 2022). Dissolved Ca2+ in the under-subsoil layer can be easily leached to groundwater (Li et al., 2017), which is consistent with the results revealed in the present study, showing significant increases in Caex contents in the subsoil layer compared to those in surface and under-subsoil layers (Supplementary Table S1).
The findings of the current study highlighted the importance of the subsoil layer for effective straw return strategies to promote SOC and SIC sequestration in cropland soils. In addition, Ca2+ contents in the subsoil layer may contributes to prevent SIC depletion and SIC leaching in surface and under-subsoil layers, respectively. Studies on the mechanisms leading to greater SOC and SIC sequestration potentials under long-term straw return are required. Moreover, particular attention should be devoted to the role of soil calcium in promoting SOC and SIC sequestration in future studies.
5 Conclusion
In summary, this study comprehensively considered the effect of long-term straw return on SOC and SIC sequestration, and found the subsoil layer is worth considered. In surface and under-subsoil layer, straw return increased SOC stock by 33% and 32%, but reduced SIC stock by 53% and 19%. In subsoil layer, long-term straw return resulted in a coordinated increase of SOC and SIC stock, that were increased by average values of 44% and 24%, respectively. In addition, Caex contents in the deeper soil layer exhibited higher positive correlations with SOC (p < 0.05) and SIC stock (p < 0.001) than those revealed in the soil surface layer suggesting calcium was beneficial to the increase of SOC and SIC sequestration by long-term straw return. The present study provides a comprehensive understanding of the straw return effects on SOC and SIC sequestration in soil profile to develop effective strategies for enhancing C sequestration in cropland.
Data availability statement
The raw data supporting the conclusion of this article will be made available by the authors, without undue reservation.
Author contributions
MX: Methodology, investigation, laboratory analysis, data curation, formal analysis, and writing-original draft. TZ: Management of long-term field experiment, investigation, writing-review. SL: Laboratory analysis, data curation, writing-review. ZL: Laboratory analysis, data curation, writing-review. ZW: Methodology, investigation, conceptualization, supervision, funding acquisition, project administration, writing-review, and editing. All authors read and approved the final manuscript.
Funding information
This research was funded by the Jilin Province Science and technology development plan project (Grant number YDZJ202201ZYTS611) and the Jilin Province Science and Technology Development Plan (Grant number 20190301057NY).
Conflict of interest
The authors declare that the research was conducted in the absence of any commercial or financial relationships that could be construed as a potential conflict of interest.
Publisher’s note
All claims expressed in this article are solely those of the authors and do not necessarily represent those of their affiliated organizations, or those of the publisher, the editors and the reviewers. Any product that may be evaluated in this article, or claim that may be made by its manufacturer, is not guaranteed or endorsed by the publisher.
Supplementary material
The Supplementary Material for this article can be found online at: https://www.frontiersin.org/articles/10.3389/fenvs.2023.1095401/full#supplementary-material
References
Amelung, W., Bossio, D., de Vries, W., Kogel-Knabner, I., Lehmann, J., Amundson, R., et al. (2020). Towards a global-scale soil climate mitigation strategy. Nat. Commun. 11 (1), 5427. doi:10.1038/s41467-020-18887-7
Angst, G., Pokorný, J., Mueller, C. W., Prater, I., Preusser, S., Kandeler, E., et al. (2021). Soil texture affects the coupling of litter decomposition and soil organic matter formation. Soil Biol. Biochem. 159, 108302. doi:10.1016/j.soilbio.2021.108302
Berhane, M., Xu, M., Liang, Z., Shi, J., Wei, G., and Tian, X. (2020). Effects of long-term straw return on soil organic carbon storage and sequestration rate in north China upland crops: A meta-analysis. Glob. Change Biol. 26 (4), 2686–2701. doi:10.1111/gcb.15018
Button, E. S., Pett-Ridge, J., Murphy, D. V., Kuzyakov, Y., Chadwick, D. R., and Jones, D. L. (2022). Deep-C storage: Biological, chemical and physical strategies to enhance carbon stocks in agricultural subsoils. Soil Biol. Biochem. 170, 108697. doi:10.1016/j.soilbio.2022.108697
Chen, C., Dynes, J. J., Wang, J., Karunakaran, C., and Sparks, D. L. (2014). Soft X-ray spectromicroscopy study of mineral-organic matter associations in pasture soil clay fractions. Environ. Sci. Technol. 48 (12), 6678–6686. doi:10.1021/es405485a
Chen, C., Hall, S. J., Coward, E., and Thompson, A. (2020). Iron-mediated organic matter decomposition in humid soils can counteract protection. Nat. Commun. 11 (1), 2255. doi:10.1038/s41467-020-16071-5
Chen, L., Fang, K., Wei, B., Qin, S., Feng, X., Hu, T., et al. (2021). Soil carbon persistence governed by plant input and mineral protection at regional and global scales. Ecol. Lett. 24, 1018–1028. doi:10.1111/ele.13723
Deng, F., Wang, H., Xie, H., Bao, X., He, H., Zhang, X., et al. (2022). Low-disturbance farming regenerates healthy deep soil toward sustainable agriculture - evidence from long-term no-tillage with stover mulching in Mollisols. Sci. Total Environ. 825, 153929. doi:10.1016/j.scitotenv.2022.153929
Feng, J., He, K., Zhang, Q., Han, M., and Zhu, B. (2022). Changes in plant inputs alter soil carbon and microbial communities in forest ecosystems. Glob. Change Biol. 28, 3426–3440. doi:10.1111/gcb.16107
Guo, L. B., and Gifford, R. M. (2002). Soil carbon stocks and land use change: A meta analysis. Glob. Change Biol. 8 (4), 345–360. doi:10.1046/j.1354-1013.2002.00486.x
He, M., Fang, K., Chen, L., Feng, X., Qin, S., Kou, D., et al. (2022). Depth-dependent drivers of soil microbial necromass carbon across Tibetan alpine grasslands. Glob. Change Biol. 28 (3), 936–949. doi:10.1111/gcb.15969
Kalinichev, A. G., and Kirkpatrick, R. J. (2007). Molecular dynamics simulation of cationic complexation with natural organic matter. Eur. J. Soil Sci. 58 (4), 909–917. doi:10.1111/j.1365-2389.2007.00929.x
Kim, J. H., Jobbagy, E. G., Richter, D. D., Trumbore, S. E., and Jackson, R. B. (2020). Agricultural acceleration of soil carbonate weathering. Glob. Change Biol. 26 (10), 5988–6002. doi:10.1111/gcb.15207
Lal, R. (2011). Sequestering carbon in soils of agro-ecosystems. Food Policy 36, S33–S39. doi:10.1016/j.foodpol.2010.12.001
Lal, R. (2004). Soil carbon sequestration impacts on global climate change and food security. Science 304 (5677), 1623–1627. doi:10.1126/science.1097396
Li, Y., Zhang, C., Wang, N., Han, Q., Zhang, X., Liu, Y., et al. (2017). Substantial inorganic carbon sink in closed drainage basins globally. Nat. Geosci. 10, 501–506. doi:10.1038/NGEO2972
Liu, C., Lu, M., Cui, J., Li, B., and Fang, C. (2014). Effects of straw carbon input on carbon dynamics in agricultural soils: A meta-analysis. Glob. Change Biol. 20 (5), 1366–1381. doi:10.1111/gcb.12517
Liu, W., Wei, J., Cheng, J., and Li, W. (2014). Profile distribution of soil inorganic carbon along a chronosequence of grassland restoration on a 22-year scale in the Chinese Loess Plateau. Catena 121, 321–329. doi:10.1016/j.catena.2014.05.019
Lv, S., Yang, X., Lin, X., Liu, Z., Zhao, J., Li, K., et al. (2015). Yield gap simulations using ten maize cultivars commonly planted in Northeast China during the past five decades. Agric. For. Meteorology 205, 1–10. doi:10.1016/j.agrformet.2015.02.008
Mulder, V. L., Lacoste, M., Martin, M. P., Richer-de-Forges, A., and Arrouays, D. (2015). Understanding large-extent controls of soil organic carbon storage in relation to soil depth and soil-landscape systems. Glob. Biogeochem. Cycles 29 (8), 1210–1229. doi:10.1002/2015GB005178
Raza, S., Miao, N., Wang, P., Ju, X., Chen, Z., Zhou, J., et al. (2020). Dramatic loss of inorganic carbon by nitrogen-induced soil acidification in Chinese croplands. Glob. Change Biol. 26 (6), 3738–3751. doi:10.1111/gcb.15101
Rodrigues, L., Hardy, B., Huyghebeart, B., Fohrafellner, J., Fornara, D., Barančíková, G., et al. (2021). Achievable agricultural soil carbon sequestration across Europe from country-specific estimates. Glob. Change Biol. 27 (24), 6363–6380. doi:10.1111/gcb.15897
Rowley, M. C., Grand, S., Spangenberg, J. E., and Verrecchia, E. P. (2021). Evidence linking calcium to increased organo-mineral association in soils. Biogeochemistry 153 (3), 223–241. doi:10.1007/s10533-021-00779-7
Rowley, M. C., Grand, S., and Verrecchia, E. P. (2018). Calcium-mediated stabilisation of soil organic carbon. Biogeochemistry 137 (1-2), 27–49. doi:10.1007/s10533-017-0410-1
Rumpel, C., and Koegel-Knabner, I. (2011). Deep soil organic matter-a key but poorly understood component of terrestrial C cycle. Plant Soil 338 (1-2), 143–158. doi:10.1007/s11104-010-0391-5
Schlesinger, W. H. (2017). An evaluation of abiotic carbon sinks in deserts. Glob. Change Biol. 23 (1), 25–27. doi:10.1111/gcb.13336
Song, G. H., Li, L. Q., Pan, G. X., and Zhang, Q. (2005). Topsoil organic carbon storage of China and its loss by cultivation. Biogeochemistry 74 (1), 47–62. doi:10.1007/s10533-004-2222-3
Sun, Z., Meng, F., and Zhu, B. (2022). Influencing factors and partitioning methods of carbonate contribution to CO2 emissions from calcareous soils. Soil Ecol. Lett. 5, 6–20. doi:10.1007/s42832-022-0139-1
Tao, J., Raza, S., Zhao, M., Cui, J., Wang, P., Sui, Y., et al. (2022). Vulnerability and driving factors of soil inorganic carbon stocks in Chinese croplands. Sci. Total Environ. 825, 154087. doi:10.1016/j.scitotenv.2022.154087
Turmel, M.-S., Speratti, A., Baudron, F., Verhulst, N., and Govaerts, B. (2015). Crop residue management and soil health: A systems analysis. Agric. Syst. 134, 6–16. doi:10.1016/j.agsy.2014.05.009
Wang, S., Jia, Y., Liu, T., Wang, Y., Liu, Z., and Feng, X. (2021). Delineating the role of calcium in the large-scale distribution of metal-bound organic carbon in soils. Geophys. Res. Lett. 48 (10), e2021GL092391. doi:10.1029/2021gl092391
Wang, X. J., Xu, M. G., Wang, J. P., Zhang, W. J., Yang, X. Y., Huang, S. M., et al. (2014). Fertilization enhancing carbon sequestration as carbonate in arid cropland: Assessments of long-term experiments in northern China. Plant Soil 380 (1-2), 89–100. doi:10.1007/s11104-014-2077-x
Witzgall, K., Vidal, A., Schubert, D. I., Hoeschen, C., Schweizer, S. A., Buegger, F., et al. (2021). Particulate organic matter as a functional soil component for persistent soil organic carbon. Nat. Commun. 12 (1), 4115. doi:10.1038/s41467-021-24192-8
Wu, H., Guo, Z., Gao, Q., and Peng, C. (2009). Distribution of soil inorganic carbon storage and its changes due to agricultural land use activity in China. Agric. Ecosyst. Environ. 129 (4), 413–421. doi:10.1016/j.agee.2008.10.020
Xia, L., Lam, S., Wolf, B., Kiese, R., Chen, D., and Butterbach-Bahl, K. (2018). Trade-offs between soil carbon sequestration and reactive nitrogen losses under straw return in global agroecosystems. Glob. Change Biol. 24, 5919–5932. doi:10.1111/gcb.14466
Xia, Q., Rufty, T., and Shi, W. (2020). Soil microbial diversity and composition: Links to soil texture and associated properties. Soil Biol. Biochem. 149, 107953. doi:10.1016/j.soilbio.2020.107953
Yang, Y., Bao, X., Xie, H., He, H., Zhang, X., Shao, P., et al. (2022a). Frequent stover mulching builds healthy soil and sustainable agriculture in Mollisols. Agric. Ecosyst. Environ. 326, 107815. doi:10.1016/j.agee.2021.107815
Yang, Y., Xie, H., Mao, Z., Bao, X., He, H., Zhang, X., et al. (2022b). Fungi determine increased soil organic carbon more than bacteria through their necromass inputs in conservation tillage croplands. Soil Biol. Biochem. 167, 108587. doi:10.1016/j.soilbio.2022.108587
You, M., Han, X., Hu, N., Du, S., Doane, T. A., and Li, L.-J. (2020). Profile storage and vertical distribution (0-150 cm) of soil inorganic carbon in croplands in northeast China. Catena 185, 104302. doi:10.1016/j.catena.2019.104302
Zamanian, K., and Kuzyakov, Y. (2019). Contribution of soil inorganic carbon to atmospheric CO2: More important than previously thought. Glob. Change Biol. 25 (1), E1–E3. doi:10.1111/gcb.14463
Zamanian, K., Zarebanadkouki, M., and Kuzyakov, Y. (2018). Nitrogen fertilization raises CO2 efflux from inorganic carbon: A global assessment. Glob. Change Biol. 24 (7), 2810–2817. doi:10.1111/gcb.14148
Zhao, X., Zhao, C., Stahr, K., Kuzyakov, Y., and Wei, X. (2020). The effect of microorganisms on soil carbonate recrystallization and abiotic CO2 uptake of soil. Catena 192, 104592. doi:10.1016/j.catena.2020.104592
Keywords: straw return, soil organic carbon, soil inorganic carbon, soil profile, cropland
Citation: Xie M, Zhang T, Liu S, Liu Z and Wang Z (2023) Profile soil organic and inorganic carbon sequestration in maize cropland after long-term straw return. Front. Environ. Sci. 11:1095401. doi: 10.3389/fenvs.2023.1095401
Received: 11 November 2022; Accepted: 19 January 2023;
Published: 01 February 2023.
Edited by:
Wakene Negassa, University of Copenhagen, DenmarkReviewed by:
Sileshi Gudeta Weldesemayat, University of KwaZulu-Natal, South AfricaVikas Sharma, Sher-e-Kashmir University of Agricultural Sciences and Technology, India
Copyright © 2023 Xie, Zhang, Liu, Liu and Wang. This is an open-access article distributed under the terms of the Creative Commons Attribution License (CC BY). The use, distribution or reproduction in other forums is permitted, provided the original author(s) and the copyright owner(s) are credited and that the original publication in this journal is cited, in accordance with accepted academic practice. No use, distribution or reproduction is permitted which does not comply with these terms.
*Correspondence: Zhongqiang Wang, d2FuZ3pxMDI3QG5lbnUuZWR1LmNu