- 1Regional Water and Environmental Sanitation Centre Kumasi, Department of Civil Engineering, Kwame Nkrumah University of Science and Technology, Kumasi, Ghana
- 2Department of Environmental Health and Sanitation Education, Akenten Appiah-Menka University of Skills Training and Entrepreneurial Development, Kumasi, Ghana
- 3Department of Desalination and Water Treatment, Albert Katz International School for Desert Studies, Zuckerberg Institute for Water Research, The Jacob Blaustein Institutes for Desert Research, Ben-Gurion University of the Negev, Beersheba, Israel
Reclaimed water from wastewater has become a prominent water source option to manage water scarcity. This study explores the potential of coconut husk biomass, a common waste material in Ghana, as a valuable low-cost resource for greywater treatment. Engineered column treatment was applied to investigate the influence of pyrolysis and biochar properties of coconut husk biomass waste on greywater treatment. Coconut husk biomass waste was pyrolyzed at 600°C and characterized using SEM, FTIR, and XRD. Three engineered columns with 1) raw coconut husk powder (RCHP), 2) charred coconut husk powder (CCHP), and 3) sand-gravel filters (control setup) were used. A hydrostatic head of greywater with a throughput of 8.0 ml/min and a hydraulic retention time of 45 min was maintained for engineered columns. The SEM image suggested an increased surface area and pores due to the pyrolysis of the husk biomass. RCHP and CCHP contributed to 63% and 95% turbidity removal, respectively. Experimental results showed high removal efficiencies of 71% COD for CCHP. The nitrate removal efficiency of 78.93%, 88.38%, and 28.65% was observed for RCHP, CCHP, and control respectively. The log removal of faecal coliform by CCHP was two orders of magnitude higher than RCHP. Faecal and total coliform removal was 2.87 log units for CCHP. Significant differences were observed between CCHP and RCHP, p < 0.05 for electrical conductivity and total dissolved solids of effluents. CCHP showed a promising potential for greywater treatment. Pyrolyzed coconut husk powder is a promising adsorbent applicable to greywater treatment.
1 Introduction
One of the very pressing global barriers to socioeconomic development is water scarcity (Liu et al., 2017). Available water resources such as groundwater and open-bodied water are further degraded and constantly under stress due to natural and anthropogenic occurrences (Ahuti, 2015; IWMI, 2019). In view of this global scarcity, wastewater (including greywater) reclamation is thus an emerging water management technique that is fast becoming a significant water source option (Singhal and Perez-Garcia, 2016; Yoshikawa et al., 2019). With an annual global wastewater production of approximately 1,500 billion cubic meters and daily domestic wastewater generation of 700–960 million cubic meters, it is estimated that greywater accounts for 70%–80% of household wastewater, and its reuse can cut urban demand for potable water by 30%–70%.
Several physical, chemical, biological, and a combination of techniques ranging from conventional to non-conventional systems (Maimon and Gross, 2018) have been applied to wastewater reclamation aimed at reducing treatment costs and improving treatment efficiency (Gwenzi et al., 2017). Physical techniques such as screening, gravity separation, dissolved air flotation, filtration, adsorption, desorption, membrane filtration, coagulation, flocculation, de-emulsification, and chemical techniques such as pH adjustment, precipitation, ion exchange, oxidation, reduction, electrolysis, resin-based processes, and disinfection have all been applied (Meena et al., 2021). Biological treatment options include aerobic and anaerobic treatment and ion-exchange membrane bioreactors. The treatment method usually depends on the end-use, composition, and characteristics of the influent greywater, pollutant or pathogen of interest, treatment costs, treatment efficiency, energy, operations, and maintenance costs, among others (Abdel-Shafy and Al-Sulaiman, 2014). Of all treatment options, adsorption has proven to be a highly favorable and much cheaper treatment option. Profound importance of adsorption include high pollutant removal efficiency, ease of operation and manipulation, tendency to eliminate multiple pollutants concurrently as well as cost effectiveness (Egbosiuba et al., 2020). Detoxification through adsorption is facilitated by the presence of reactive functional groups in adsorbates as well as the bonds formed between the adsorbate and adsorbent (Rashed, 2013). Adsorption has been used to remove many contaminants from wastewater including textile dyes and heavy metals (Egbosiuba et al., 2021). Depending on the contaminant of focus, the type and dosage of adsorbent used and removal conditions vary, i.e. pyrolysis conditions (time, temperature), pH, contact time (Egbosiuba et al., 2021).
Coconut husk, also known as coco peat or coconut coir, is the fine spongy fiber left behind when coconut husk fibers are separated from the shell during processing (Ifelebuegu and Momoh, 2015; Suman and Gautam, 2017). It is also one material that has many useful applications in water treatment (Parsons, 2014). It has been used extensively to remove different pollutants from water and wastewater streams (Marathe et al., 2021). It is also one of the emerging adsorbents used for heavy metal adsorption and nitrogen removal (Ifelebuegu and Momoh 2015; Clarence, 2016). When converted to biochar, pyrogenic black carbon derived from incomplete biomass combustion, the coconut husk is an effective media for biofiltration in wastewater treatment (de Oliveira Cruz et al., 2019; Halfhide et al., 2019) with a solid sorption capacity to pesticides and natural contaminants. Its physicochemical properties like surface area, particle size distribution, functional groups, pore spaces, or volume contribute to its removal efficiency (Suman and Gautam, 2017). Pyrolysis of biochar influences the surface functional groups by rearranging structural bonds. An FTIR spectrum of the biochar would reveal a disappearance of certain bands characteristic of the carbonized raw materials (Tomczyk et al., 2020). Findings from batch experiments suggested that the biochar’s maximum phosphorus sorption capacity could reach >100 mg g−1 (Yao, 2013). Biochar at a pH of 6 has also shown complete phosphate removal from fertilizer wastewater. Sugar beet biochar pyrolyzed at 600°C shows the adsorption of phosphate ions on the biochar surface (Chen et al., 2011). Similarly, orange peels produced at temperatures between 250 and 700°C gave 80%–85% phosphate removal. Nitrates are adsorbed by bamboo biochar with a concentration range of 0–10 mg/L. Biochar produced above 400°C was effective for organic and inorganic pollutant sorption, whereas porous carbonized fractions are dominant at high temperatures (Uchimiya et al., 2010). Food waste biochar is also known to enhance Copper coagulation and remove Chromium, Nickel, and organic dyes from wastewater (Yang et al., 2021).
Meanwhile, coconut waste contributes significantly to the waste management problem in developing economies like Ghana and other coconut-rich countries. A large volume of waste is generated daily, and in Ghana, the husks are not disposed of properly, becoming an aesthetically objectionable breeding habitat for mosquitoes and other disease-causing vectors. Although other studies have shown that coconut husk biomass is suitable for water and wastewater treatment, its application to wastewater in Ghana has not been thoroughly investigated. With urban wastewater generation expected to increase in countries like Ghana from about 530,346 m3/day (36%) in 2000 to about 1,452,383 m3/day (45%) in 2020, it is worthwhile to explore the use of locally available coconut husk biomass for greywater treatment (Gyampo, 2012).
Therefore, this study will characterize locally available coconut husk biomass waste, convert it to biochar through pyrolysis, establish the influence of pyrolysis on its physical and chemical properties and further investigate its potential for greywater treatment. The study will contribute to the sustainable development goal (SDG) six on clean water and sanitation by 1) complementing ongoing scientific studies on low-cost wastewater treatment 2) improving the body of knowledge on using coconut husk biomass for wastewater treatment 3) reducing the burden of coconut husk biomass waste by re-using the husk, towards a circular economy.
2 Materials and method
2.1 Coconut husk powder (CHP) production and characterization
Coconut husk was cut into chunks and dried at 105°C for 24 h until complete drying as shown in Figures 1A,B. The dried husk was milled to obtain Raw Coconut Husk Powder (RCHP). A fraction was also charred at 600°C (Egbosiuba et al., 2020) for 2 h in a Nabertherm 2,345 muffle furnace and milled to obtain Charred Coconut Husk Powder (CCHP). Optimum temperature range for pyrolysis is between 500 and 800°C but 600°C in particular yields very high microsurface area, pore volumes and a tremendous adsorption capacity (Chartterjee et al., 2020). Both RCHP and CCHP were sieved to obtain a particle size of 0.2–0.4 mm (Figures 1C,D). A known volume of distilled water was mixed with the two fractions separately in a ratio of 1:10, after which the pH of each mixture was determined using a pH meter. The Fourier-Transform Infrared (FTIR) Spectroscopy used Bruker Alpha II Platinum ATR-FTIR to study the chemical composition and surface functional groups. The morphology of the two forms of CHP was observed using Scanning Electron Microscopy (SEM) under various voltages, beam sizes, and magnifications ranging from ×50 to 2000X using a Nano-SEM 230 FEG. The SEM was run in low vacuum mode using the variable contrast detector.
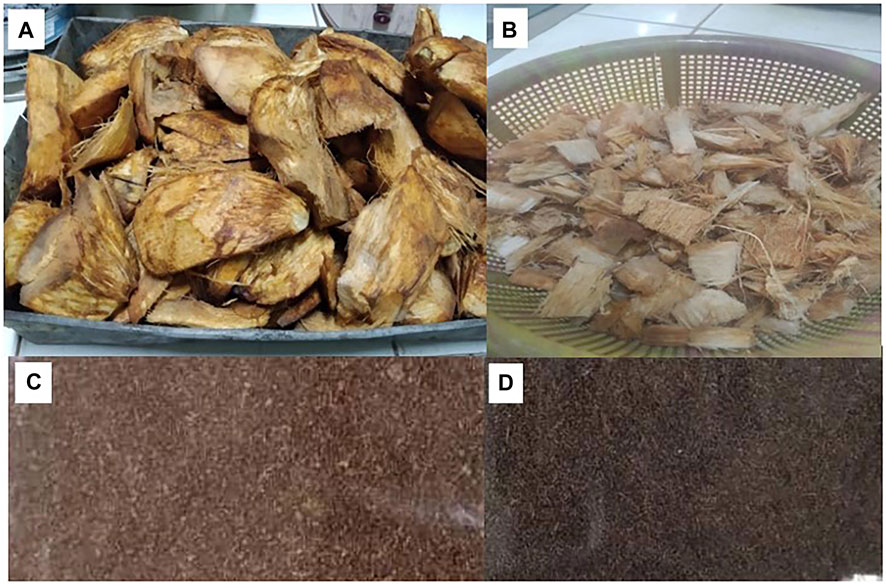
FIGURE 1. (A) Fresh coconut husk (B) Dried coconut husk (C) Raw coconut husk powder (D) Charred coconut husk powder.
2.2 Filter design and greywater sampling
The column filter bed consisted of a column of 40.0 cm in length, a fixed bed depth of 14.0 cm, and a 7.0 cm internal diameter. Three beds were designed for the Control, RCHP, and CCHP beds, each in duplicates (Figure 2). A weight of 110.0 g of the CCHP corresponded to a bed depth of 14.0 cm whilst 80.0 g of RCHP corresponded to the same bed depth, RCHP was denser than CCHP. Each filter bed was supported by 2.0 cm sand and gravel layers at the bottom and a 1.0 cm sand layer at the top to prevent channelling. The influent flow rate was set at 8.0 ml/min at a retention time of 45 min.
The influent feed was domestic greywater collected from students’ residential facilities at the Kwame Nkrumah University of Science and Technology campus in Kumasi, Ghana. The effluents were collected from the filters over time and analyzed for physicochemical and biological properties using standard methods for the examination for water and wastewater (APHA 2005) where applicable. pH, electric conductivity, and TDS were measured by immersing the Palintest Micro 800 MULTI electrode in a known volume of solution. Colour was determined using the Lovibond Comparator. Equal volumes of sample and distilled water were pipetted into separate test tubes and inserted into the comparator and measurements taken. The HANNA Turbidimeter HI 93414 was used to measure the turbidity of samples. Empty vials were filled with a known sample volume and placed in the vial holder for measurements. DO and BOD5 were analyzed by mixing a known volume of sample with standard nutrient pillows and subsequently measuring the DO using HACH HQ 30d meter. COD was analyzed by the digestion method. Nitrates were measured using HACH DR3900 spectro photometer at a wavelength of 500 nm. Microbial analysis was done by the Serial Dilution Method using chromocult agar, membrane filtration and plate counting.
3 Results and discussion
3.1 Physical characteristics of RCHP and CCHP
The physical properties of CCHP are presented in Table 1. The pH of the RCHP was determined to be 4.67, an indication of the acidic nature of the adsorbent. Conversely, the pH of the CCHP was 10.61, being basic. The basic pH of the CCHP may be due to the pyrolysis of the CCHP, which modified the structural morphology and increased the ash content. Carbonization also favors the formation of carbonates and inorganic alkalis while decreasing acidic functional groups (Tomczyk et al., 2020). The pH values are within ranges found in similar studies of coconut husks (Samaniego and Tanchuling, 2019). The bulk density and porosity of the RCHP were higher than the CCHP, suggesting that the RCHP has relatively better throughput. Kalaivani and Jawaharlal (2019) reported bulk density slightly higher than that obtained in this study. The RCHP recorded a moisture content of 5.7% and the CCHP, 5.6%. The relatively lower moisture content of the CCHP could be attributed to the temperature effect on the CCHP, which contributes to the loss of water and volatile organic compounds.
3.2 SEM imaging
The SEM images in Figure 3 reflect differences between the morphology and pore structure of the RCHP and CCHP. The RCHP shows a distinct fibrous structure with heterogeneous cylindrical pores. After carbonization, it is observed that the linkages of the carbon-bearing adsorbent in the microstructure are split. This follows observations by Suman and Gautam (2017). The CCHP shows relatively higher pores and increased surface area due to the breakdown of lignin and cellulose compounds at elevated temperatures under pyrolysis. Maximum surface area have been reported to occur at 600°C (Egbosiuba et al., 2020). Higher pores provide adequate receptacles within the interstices of the adsorbents for adsorbates. Increased surface area is equally necessary for increased sites for the adsorption of contaminants. The SEM images demonstrate that the properties of the RCHP are enhanced after charring and based on the pore sizes and surface area, the potential for contaminant removal of the CCHP is relatively higher as compared to the RCHP.
3.3 Functional group characteristics of CHP
The infrared spectra of the RCHP and CCHP are presented in Figures 4, 5 respectively. The peak observed at 3,340 cm−1 (Figure 4) is characteristic of an O-H stretch of carboxylic acids (Egbosiuba et al., 2020) or hydroxyl vibrations of phenolic groups of lignin within the coconut husk powder, similarly observed by Etim et al., 2016 and Mishra and Basu, 2020. At 2,913 cm−1 the medium peak indicates a C-H stretching motion of alkane groups (Malik et al., 2017; Suman and Gautam, 2017). The C=O intensity stretch at 1722 cm−1 is characteristic of carboxylic acids, and 1,605.4 cm−1 represents the C=C bonds observed for RCHP (Wang and Sarkar, 2018; Bello et al., 2019). The C-C bonds in aromatic compounds in the lignin of coconut husks are observed at 1,515 cm−1 in the RCHP spectrum. Lignin itself shows bands at 1,242 to 1,514 cm−1 resulting from aromatic C=C and C=O stretches of carboxylic acids (Qambrani et al., 2017). The N-O symmetric bond shows the presence of the nitro group at 1,368 cm−1. At 1,440 cm−1, the broad intensity at 1,032.45 cm-1 indicates symmetric C-O stretching movements in cellulose (Chen et al., 2011).
The spectrum observed for the CCHP (Figure 5) does not align with the bands of known functional groups. This may be due to pyrolysis that modified the structural integrity of the CHP. The decomposition of cellulose and lignin polymers during carbonization facilitates the disintegration of bonds. The characteristic O-H band observed for the RCHP was absent in the spectrum of the charred sample because temperatures above 400°C have been known to decompose lignin present in coconut husk (Wang and Sarkar, 2018) hence, the absence of the characteristic O-H vibrations in the IR spectrum of CHP calcined at 600°C (Suman and Gautam, 2017). The C=O peak is also not observed in the spectrum of the charred CHP because, during the carbonization of coconut husk, carboxyl and carbonyl groups are broken down to yield carbon monoxide and dioxides (Wang and Sarkar, 2018). The peaks in the spectrum are due to incomplete degradation that occurs at 600°C of which higher temperature carbonization would produce relatively smoother IR curves.
3.4 Influent greywater quality
Table 2 shows the result of the physicochemical and biological parameters of the influent greywater. The pH range of the greywater sampled in Kumasi was determined to be 6.5 to 7.4. The mean pH and BOD are comparable to similar studies of grey water in Kumasi (Dwumfour-Asare et al., 2020). The total suspended solids concentration (TSS) range corroborates the values reported by Chaillou et al. (2011). The influent Chemical Oxygen Demand (COD) concentration was lower than domestic greywater characteristics in Kumasi (Dwumfour-Asare et al., 2020). However, the concentrations are comparable to studies conducted by Pillai and Vijayan (2012). Empirical data from Casanova et al. (2001) also support the turbidity value of the influent observed in this study. However, Anim et al. (2014) reported a relatively higher turbidity of 90 NTU. The difference in turbidity values of the greywater influent may be attributed to the variations in water use of consumers. Microbial cultures of Salmonella spp and Escherichia coli were identified in the influent greywater.
3.5 Effect of charred husk powder on effluent quality
3.5.1 pH
From an influent pH of 6.73, the greywater effluent from the RCHP column bed dropped to 5.27, and the effluent through the CCHP column bed increased to 8.8, a reflection of the acidity and alkaline nature of the RCHP and CCHP, respectively.
Biochar is generally alkaline as carbonization has been found to increase the pH of the biomass feedstock due to the formation of carbonates and inorganic alkalis. Carbonization also decreases acidic functional groups (Belhachemi et al., 2019). Thus, releasing OH ions from biochar when in contact with water and increase pH. The carbonization process favors the formation of carbonates and inorganic alkalis while decreasing the acidic functional groups of the feedstock (Tomczyk et al., 2020).
Depending on the intended use, pH adjustment may be required for effluents from both RCHP and CCHP. Considering the adsorbent pH of 4.67 for the RCHP, 10.61 for the CCHP, and the effluent pH, it can be concluded that the adsorbents adjusted the acidity and alkalinity during the treatment of the effluents based on their prevailing pH. Thus, the pH of effluents from biochar treatments tends to have increased pH compared to non/biochar treatments (Emslie, 2019).
3.5.2 Dissolved oxygen (DO)
Both RCHP and CCHP improved the dissolved oxygen concentration of the greywater exponentially. Influent DO was 0.86 mg/L, effluent DO from the CCHP was 7.37 mg/L, the RCHP was 5.21 mg/L and the Control was 4.84 mg/L. The DO concentration of the effluents establishes a possible reduction in the organic matter content of the influent as greywater was filtered. Biochar is also rich in oxygen-containing compounds, which may serve as oxidizing agents for water flowing through the adsorbents (Qambrani et al., 2017). The effluent flowing through the Control recorded an appreciable level of DO. This is consistent with studies by Yaseen et al. (2019), where a 30%–50% increase in the DO of laundry greywater through sand-gravel filters was observed. The effluent DO’s were within guideline values for discharge and reuse by the World Health Organization (WHO), which is >1 mg/L. However, stricter limit of >2 mg/L is recommended in some countries (World Health Organization, 2006).
3.5.3 Electrical conductivity (EC) and total dissolved solids (TDS)
Significant differences were observed between CCHP and RCHP (p < 0.05) in terms of electrical conductivity (EC) and Total Dissolved Solids (TDS) of effluent. Generally, effluents from the CCHP showed an increase in EC and TDS concentrations from 298.19 μS/cm to 411.74 μS/cm and 145.36 to 200.76 mg/L respectively. The RCHP effluents recorded a reduction in EC and TDS concentrations from 298.19 to 207.81 μS/cm and 145.36 to 101.18 mg/L respectively. The relatively higher EC of the CCHP effluent suggests the release of water-soluble ions from the biochar into the greywater as suggested by Belhachemi et al., 2019. It has been asserted that pyrolysis increases the EC of biochar (Hoffman et al., 2019) probably as a result of the breaking of bonds which further enhances the release of free ions in the CCHP into the effluent. The findings compare positively with other studies that have reported an increase in EC after carbonization (Suman and Gautam, 2017). Effluents from the Control showed a decrease in both TDS and EC like Yaseen et al. (2019), consistent with the fact that sand filters are known to reduce the EC of water due to ion exchanges between the finer particles of sand and the water.
3.5.4 Turbidity and colour
Effluents were generally less turbid after filtration. Turbidity values declined from an influent value of 67.30 NTU to 3.62 NTU, 24.61 NTU, and 41.27 NTU for CCHP, RCHP, and Control respectively. This corresponds to 95% removal for CCHP, 63% for RCHP, and 39% for the Control. The increased surface area and porosity of the biochar due to pyrolysis enhances the removal of solids and adsorption of molecules that impart color and turbidity (Enaime et al., 2020; Fu et al., 2020). Turbidity values for the RCHP were relatively lower than CCHP due to the adsorbent’s low porosity and surface area. Turbidity removal by the Control is consistent with the available literature on turbidity removal by sand filters (Saad et al., 2016) in that sand and gravel grains are known to remove suspended matter in greywater, thereby making wastewater less turbid (Samayamanthula et al., 2019). From an influent of 58 TCU, results obtained for color in the treated effluent were 10 TCU, 69 TCU, and 63 TCU for CCHP, RCHP, and Control. The Control and RCHP showed an increase in the color of greywater after filtration. This could be attributed to microbial activities on both the greywater and the adsorbent, which will impart some turbidity and color.
3.5.5 BOD, COD, and TSS removal
The removal efficiency of organic matter by CCHP was relatively higher than by RCHP (Figure 6). The percentage removal of COD and BOD was 71% and 16%, respectively, for CCHP. COD removal efficiency for RCHP was 1.7% but was relatively poor for BOD removal. CCHP showed significant differences (p < 0.05) in COD removal compared to RCHP and Control. Though RCHP successfully removed suspended solids, it was not effective for BOD and COD removal. The negative BOD recorded for the RCHP could be attributed to nitrogenous oxygen demand in the presence of nitrifying bacteria over the incubation period. Cocopeat supports microbial communities’ growth (Thomson, 2014) and over time, microbial breakdown of lignocellulose in cocopeat and pine barks occurs as reported by (Dalahmeh, 2016) significantly reducing their ability for BOD and COD removal. In the case of CCHP, there was no observed microbial breakdown of the biochar because pyrolysis alters biochar carbons’ chemical nature, making them relatively stable and resistant to biological decomposition (Qambrani et al., 2017).
3.5.6 Nitrate removal
Nitrate removal was 78.93%, 88.38%, and 28.65% for RCHP, CCHP, and Control respectively (Figure 7). The percentage removal of the CCHP was 10% higher than the RCHP and 60% higher than the Control. The differences could be attributed to the carbonization of the husk which possibly increased the adsorptive sites and capacity of the husk, improved the C: N ratio, and the activity of denitrifying bacteria (Halfhide et al., 2019). The removal rate was higher than predicted by Yao (2013) biochar carbonized at 600°C. However, the removal rate was consistent with studies by Halfhide et al. (2019) who reported nitrate removal from stock solution and wastewater between wastewater 74%–90%.
3.5.7 Microbial populations
The biochars effectively reduced microbial loads in the wastewater stream (Figure 8). The log removal of Faecal Coliform (FC) by the RCHP was two orders of magnitude less than that of the CCHP. Faecal and total coliform removal was 2.87 log units for CCHP compared to 0.31 log units for Total Coliform (TC) removal recorded for the control filter. This supports the assertion that biochar filters increase the removal of coliforms (E. coli) from water (Maurya et al., 2020). Previous studies reported log removal between 0.1 and 1.0-log units for E. coli removal depending on initial counts. Though the findings from this study agree with the reported values, the log removal observed is relatively higher. The higher removal observed for this study may be attributed to biochar properties and the varying nature of biochar feedstocks (Afrooz and Boehm, 2016). The particle sizes of the biochar also facilitate microbial removal by adsorption due to the larger surface area and increased contact with the wastewater (Guan et al., 2020). A significant difference was observed between the CCHP and the Control (p < 0.05). The log removal recorded in the control filter was 0.31-log units for Total coliforms. The reduction in the microbial population in the control filter could be due to straining aided by biofilm formation on the sand and gravel surfaces.
4 Conclusion
Coconut husk biomass showed great potential as a filter material for greywater treatment. Pyrolysis was successfully applied to the coconut husk, which significantly improved greywater treatment. CCHP contributed to 95% of turbidity removal. High removal efficiencies of 71% COD by CCHP were observed. The CCHP had a relatively higher nitrate removal efficiency of 88.38%, as compared to 78.93% and 28.65% for RCHP and Control respectively. Empirical evidence demonstrates that CCHP was more effective in removing color, turbidity, COD, BOD, and nitrates. The SEM image suggests that the carbonization of the raw coconut husk increased the surface area and number of pores of the adsorbent. The log removal of faecal coliform by CCHP was two orders of magnitude higher than the RCHP. The CCHP was also efficient in the treatment of microbial loads. The results demonstrate that pyrolyzed coconut husk biomass waste is a promising adsorbent applicable to the treatment of greywater.
Data availability statement
The original contributions presented in the study are included in the article/supplementary material, further inquiries can be directed to the corresponding author.
Author contributions
All authors listed have made a substantial, direct, and intellectual contribution to the work and approved it for publication.
Funding
This study was funded by the Regional Water and Environmental Sanitation Centre Kumasi (RWESCK) at the Kwame Nkrumah University of Science and Technology (KNUST), with funding from the Ghana Government through the World Bank, under the Africa Centres of Excellence project. The views, however, expressed in this paper do not reflect those of the World Bank, Ghana Government, or KNUST.
Acknowledgments
ETH Zurich for Development (ETH4D) and Eawag is duly acknowledged for providing financial and technical support.
Conflict of interest
The authors declare that the research was conducted in the absence of any commercial or financial relationships that could be construed as a potential conflict of interest.
The handling editor OG declared a shared affiliation with the author KA-B at the time of review.
Publisher’s note
All claims expressed in this article are solely those of the authors and do not necessarily represent those of their affiliated organizations, or those of the publisher, the editors and the reviewers. Any product that may be evaluated in this article, or claim that may be made by its manufacturer, is not guaranteed or endorsed by the publisher.
References
Abdel-Shafy, H. I., and Al-Sulaiman, A. M. (2014). Assessment of physico-chemical processes for treatment and reuse of greywater. Egyptian J. Chem. 57 (3), 215–231. doi:10.21608/ejchem.2014.1042
Afrooz, A. R. M. N., and Boehm, A. B. (2016). Escherichia coli removal in biochar-modified biofilters: Effects of biofilm. PLoS ONE 11 (12), 1–17. doi:10.1371/journal.pone.0167489
Anim, S., Agyemang, E., and Wiafe, S. (2014). The use of a model sand filtration system for greywater treatment: A case study of hotels and hostels in eastern region, Ghana. Health, Saf. Environ. 2 (8), 159–166. doi:10.14196/hse.v2i8.151
APHA (2005). Standard methods for the examination of water and wastewater. Washington, DC, USA: American Public Health Association APHA, 21.
Belhachemi, M., Khiari, B., Jegurium, M., and Sepilveda-Escribano, A. (2019). “Characterization of biomass-derived chars,” in Chars and carbon materials derived from biomass, 70–107.
Bello, O. S., Adegoke, K. A., Fagbenro, S. O., and Lameed, O. S. (2019). Functionalized coconut husks for rhodamine-B dye sequestration. Appl. Water Sci. 9 (8), 1–15. doi:10.1007/s13201-019-1051-4
Chaillou, K., Gérente, C., Andres, Y., and Wolbert, D. (2011). Bathroom greywater characterization and potential treatments for reuse. Water, Air, and Soil Pollution 215 (1–4), 31–42. doi:10.1007/s11270-010-0454-5
Chatterjee, R., Sajjadi, B., Chen, W-Y., Mattern, D. L., Hammer, N., Raman, V., et al. (2020). Effect of pyrolysis temperature on PhysicoChemical properties and acoustic-based amination of biochar for efficient CO2 adsorption. Front. Energy Res. 8, 85. doi:10.3389/fenrg.2020.00085
Chen, B., and Lv, s. (2011). A novel magnetic biochar efficiently sorbs organic pollutants and phosphate. Bioresour. Tech. 102 (2), 716–723. doi:10.1007/s11270-010-0454-5
Clarence, O. A. (2016). Assessment of quantity of coconut waste generated and management in the Kumasi metropolis, Ghana, BSc dissertation. Kumasi: Kwame Nkrumah University of Science and Technology.
Dalahmeh, S. S. (2016). “Capacity of biochar filters for wastewater treatment in onsite systems ‒ technical report (No. 2016:090)” in Capacity of biochar filters for wastewater treatment in onsite systems. Uppsala, Sweden: Swedish University of Agricultural Sciences.
de Oliveira Cruz, L. M., Gomes, B. G. L. A., Tonetti, A. L., and Figueiredo, I. C. S. (2019). Using coconut husks in a full-scale decentralized wastewater treatment system: The influence of an anaerobic filter on maintenance and operational conditions of a sand filter. Ecol. Eng. 127, 454–459. doi:10.1016/j.ecoleng.2018.12.021
Dwumfour-Asare, B., Nyarko, K. B., Essandoh, H. M. K., and Awuah, E. (2020e00489). DomesticGreywater flows and pollutant loads: A neighbourhood study within a university campus in Ghana: Characteristics of greywater in a residential neighbourhood. Sci. Afr. 9, 1–12. doi:10.1016/j.sciaf.2020.e00489
Egbosiuba, T. C., Abdulkareem, A. S., Kovo, A. S., Afolabi, E. A., Tijani, J. O., Auta, M., et al. (2020). Ultrasonic enhanced adsorption of methylene blue onto the optimized surface area of activated carbon: Adsorption isotherm, kinetics and thermodynamics. Chem. Eng. Res. Des. 153, 315–336. doi:10.1016/j.cherd.2019.10.016
Egbosiuba, T. C., Abdulkareem, A. S., Kovo, A. S., Afolabi, E. A., Tijani, J. O., Bankole, M. T., et al. (2021). Adsorption of Cr(VI), Ni(II), Fe(II) and Cd(II) ions by KIAgNPs decorated MWCNTs in a batch and fixed bed process. Sci. Rep. 11 (1), 75–20. doi:10.1038/s41598-020-79857-z
Emslie, D. K. (2019). Analysis of sand filtration syst with common filter media and innovative materials. Vancouver, Canada: University of British Columbia. doi:10.14288/1.0377280
Enaime, G., Baçaoui, A., Yaacoubi, A., and Lübken, M. (2020). Biochar for wastewater treatment-conversion technologies and applications. Appl. Sci. Switz. 10 (10), 3492. doi:10.3390/app10103492
Etim, U. J., Umoren, S. A., and Eduok, U. M. (2016). Coconut coir dust as a low-cost adsorbent for the removal of cationic dye from aqueous solution. J. Saudi Chem. Soc. 20, S67–S76. doi:10.1016/j.jscs.2012.09.014
Fu, J., Zhang, J., Jin, C., Wang, Z., Wang, T., Cheng, X., et al. (2020). Effects of temperature, oxygen, and steam on pore structure characteristics of coconut husk activated carbon powders prepared by one-step rapid pyrolysis activation process. Bioresour. Technol. 310, 123413. doi:10.1016/j.biortech.2020.123413
Guan, P., Prasher, S. O., Afzal, M. T., George, S., Ronholm, J., Dhiman, J., et al. (2020). Removal of Escherichia coli from lake water in a biochar-amended biosand filtering system. Ecol. Eng. 150 (2019), 105819. doi:10.1016/j.ecoleng.2020.105819
Gwenzi, W., Chaukura, N., Noubactep, C., and Mukome, F. N. D. (2017). Biochar-based water treatment systems as a potential low-cost and sustainable Technology for clean water provision. J. Environ. Manag. 197, 732–749. doi:10.1016/j.jenvman.2017.03.087
Gyampo, M. A. (2012). “Wastewater production, treatment, and use in Ghana,” in Third regional workshop of the project ‘safe use of wastewater in agriculture, 26–28.
Halfhide, T., Lalgee, L. J., Singh, K. S., Williams, J., Sealy, M., Manoo, A., et al. (2019). Nutrient removal using spent coconut husks. H2Open J. 2 (1), 125–136. doi:10.2166/h2oj.2019.011
Hanandeh, A. El, Albalasmeh, A. A., and Gharaibeh, M. (2017). Phosphorus removal from wastewater in biofilters with biochar augmented geomedium: Effect of biochar particle size. Clean Soil Air Water 45 (9999), 1600123–1600127. doi:10.1002/clen.201600123
Hoffman, V., Rodriguez Correa, C., Sautter, D., Maringolo, E., and Kruse, A. (2019). Study of the electrical conductivity of biobased carbonaceous powder materials under moderate pressure for the application as electrode materials in energy storage technologies. GCB Bioenergy 11 (1), 230–248. doi:10.1111/gcbb.12545
Ifelebuegu, O. A., and Momoh, Z. (2015). An evaluation of the adsorptive properties of coconut husk for oil spill cleanup. Int. Conf. Adv. Appl. Sci. Environ. Technol. 1, 33–37. doi:10.15224/978-1-63248-040-8-38
International Water Management Institute (2019). Nnovative water solutions for sustainable development. Colombo: IWMI Strategy 2019-2023. doi:10.5337/2019.208I
Kalaivani, K., and Jawaharlal, M. (2019). Study on physical characterisation of cocopeat with different proportions of organic amendments for soilless cultivation J. Pharmacognosy Phytochem., 8 (3), 2283–2286.
Liu, J., Yang, H., Gosling, S. N., Kummu, M., Flörke, M., Pfister, S., et al. (2017). Water scarcity assessments in the past, present, and future. Earth's future 5 (6), 545–559. doi:10.1002/2016ef000518
Maimon, A., and Gross, A. (2018). Greywater: Limitations and perspective. Curr. Opin. Environ. Sci. Health 2, 1–6. doi:10.1016/j.coesh.2017.11.005
Malik, R., Dahiya, S., and Lata, S. (2017). An experimental and quantum chemical study of removal of utmostly quantified heavy metals in wastewater using coconut husk: A novel approach to mechanism. Int. J. Biol. Macromol. 98, 139–149. doi:10.1016/j.ijbiomac.2017.01.100
Marathe, D., Raghunathan, K., Singh, A., Thawale, P., and Kumari, K. (2021). A modified lysimeter study for phyto-treatment of moderately saline wastewater using plant-derived filter bedding materials. Front. Microbiol. 12, 767132. doi:10.3389/fmicb.2021.767132
Maurya, A., Singh, M. K., and Kumar, S. (2020). “Biofiltration technique for removal of waterborne pathogens,” in Waterborne pathogens (New Delhi: Elsevier), 123–141. doi:10.1016/b978-0-12-818783-8.00007-4
Meena, M., Sonigra, P., Yadav, G., and Barupal, T. (2021). “Wastewater treatment techniques: An introduction,” in Removal of emerging contaminants through microbial processes. Editor M. P. Shah (Singapore: Springer). doi:10.1007/978-981-15-5901-3_8
Mishra, L., and Basu, G. (2020). “Coconut fiber,” in Handbook of natural fibres (Kolkata, West Bengal: Elsevier), 8.1, 231–255. doi:10.1016/b978-0-12-818398-4.00010-4
Parsons, S. (2014). Cocopeat effluent water filtration systems in the Philippines: A comparative evaluation of alternative implementation models. Master’s Paper. North Carolina: Duke University.
Pillai, J. S., and Vijayan, N. (2012). Decentralized greywater treatment for nonpotable reuse in a vertical flow constructed wetland. 2012 international conference on green technologies. ICGT 978 (1), 58–63. doi:10.1109/ICGT.2012.6477948
Qambrani, N. A., Rahman, M., Won, S., Shim, S., and Ra, C. S. (2017). Biochar properties and eco-friendly applications for climate change mitigation, waste management, and wastewater treatment: A review. Renewable and Sustainable Energy Reviews 79, 255–273. doi:10.1016/j.rser.2017.05.057
Rashed, M. N. (2013). “Adsorption technique for the removal of organic pollutants from water and wastewater,” in Organic pollutants - monitoring, risk and treatment, 167–194.
Saad, F. N. M., Jamil, M. N., Odli, Z. S. M., and Izhar, T. N. T. (2016). Study on modified sand filtration towards water quality of wet market wastewater. MATEC Web Conf. 78, 01104–01107. doi:10.1051/matecconf/20167801104
Samaniego, J., and Tanchuling, M. A. (2019). Predicting useful life of cocopeat in a filter bed treating wastewater with heavy metals using HYDRUS-1D. ASEAN Eng. J. 9 (2), 44–56. doi:10.11113/aej.v9.15512
Samayamanthula, D. R., Sabarathinam, C., and Bhandary, H. (2019). Treatment and effective utilization of greywater. Appl. Water Sci. 9 (4), 90–12. doi:10.1007/s13201-019-0966-0
Singhal, N., and Perez-Garcia, O. (2016). Degrading organic micropollutants: The next challenge in the evolution of biological wastewater treatment processes. Front. Environ. Sci. 4, 36. doi:10.3389/fenvs.2016.00036
Suman, S., and Gautam, S. (2017). Pyrolysis of coconut husk biomass: Analysis of its biochar properties. Energy Sources, Part A Recovery, Util. Environ. Eff. 39 (8), 761–767. doi:10.1080/15567036.2016.1263252
Thomson, A. A. (2014). Development of water and wastewater biofiltration technologies for the developing World using locally available packing media: Case studies in vietnam and Haiti. PhD dissertation. North Carolina: Duke University.
Tomczyk, A., Sokołowska, Z., and Boguta, P. (2020). Biochar physicochemical properties: Pyrolysis temperature and feedstock kind effects. Rev. Environ. Sci. Biotechnol. 19 (1), 191–215. doi:10.1007/s11157-020-09523-3
Uchimiya, M., Wartelle, L. H., Lima, I. M., and Klasson, K. T. (2010). Sorption of deisopropylatrazine on broiler litter biochars. J. Agric. Food Chem. 58 (23), 12350–12356. doi:10.1021/jf102152q
Wang, Q., and Sarkar, J. (2018). Pyrolysis behaviors of waste coconut shell and husk biomasses. Int. J. Energy Prod. Manag. 3 (1), 34–43. doi:10.2495/EQ-V3-N1-34-43
World Health Organization (2006). A compendium of standards for wastewater reuse in the eastern mediterranean region World Health organization regional office for the eastern mediterranean regional Centre for environmental Health activities CEHA, 19. Cairo: World Health Organization. Retrieved from http://applications.emro.who.int/dsaf/dsa1184.pdf.
Yang, H., Kang, J. K., Park, S. J., and Lee, C. G. (2021). Effect of pyrolysis conditions on food waste conversion to biochar as a coagulant aid for wastewater treatment. J. Water Process Eng. 41, 102081. doi:10.1016/j.jwpe.2021.102081
Yao, Y. (2013). Sorption of phosphate and other contaminants on biochar and its environmental implications. PhD dissertation. Gainesville: University of Florida.
Yaseen, Z. M., Zigale, T. T., Tiyasha, D, R. K., Salih, S. Q., Awasthi, S., Bhagat, S. K., et al. (2019). Laundry wastewater treatment using a combination of sand filter, bio-char and teff straw media. Nat. Res. Sci. Rep. 9 (1), 18709–18711. doi:10.1038/s41598-019-54888-3
Keywords: biochar, coconut husk biomass, engineered column treatment, greywater, pyrolysis, waste
Citation: Taylor TS, Appiah-Effah E, Akodwaa-Boadi K, Obeng E and Ofei-Quartey MNL (2023) Engineered column treatment of greywater using raw and pyrolyzed coconut husk powder. Front. Environ. Sci. 11:1077379. doi: 10.3389/fenvs.2023.1077379
Received: 22 October 2022; Accepted: 09 January 2023;
Published: 23 January 2023.
Edited by:
Osnat Gillor, Ben-Gurion University of the Negev, IsraelReviewed by:
Adewale George Adeniyi, University of Ilorin, NigeriaTitus Egbosiuba, Chukwuemeka Odumegwu Ojukwu University, Nigeria
Copyright © 2023 Taylor, Appiah-Effah, Akodwaa-Boadi, Obeng and Ofei-Quartey. This is an open-access article distributed under the terms of the Creative Commons Attribution License (CC BY). The use, distribution or reproduction in other forums is permitted, provided the original author(s) and the copyright owner(s) are credited and that the original publication in this journal is cited, in accordance with accepted academic practice. No use, distribution or reproduction is permitted which does not comply with these terms.
*Correspondence: Theodora Sophia Taylor, dGhlb2RvcmFzdGF5bG9yQGdtYWlsLmNvbQ==