- 1College of Marine Sciences, Shanghai Ocean University, Shanghai, China
- 2Key Laboratory of Sustainable Exploitation of Oceanic Fisheries Resources, Ministry of Education, Shanghai, China
- 3National Engineering Research Centre for Oceanic Fisheries, Shanghai Ocean University, Shanghai, China
- 4Key Laboratory of Oceanic Fisheries Exploration, Ministry of Agriculture and Rural Affairs, Shanghai, China
Despite concerns about the environmental impact of microplastics (MPs), knowledge of MP contamination levels in pelagic squid is lacking. This study investigated the stomach of an ecologically and commercially important but poorly known squid species, the jumbo squid Dosidicus gigas, found in the tropical and Southern temperate Pacific. We examined the characteristics and spatial distribution of MPs in fifty D. gigas (16.0–37.8 cm mantle length) and assessed potential MP pollution risks. MPs were detected in 50.0% of specimens and had an average abundance of 0.88 ± 1.12 items/individual and 0.24 ± 0.36 items/g stomach weight, and were sized 58.42–2,944.85 μm. The MPs were dominated by polyethylene terephthalate (31.82%) in polymer type, fragments (54.55%) and fibers (43.18%) in shape, and blue (59.59%) in color. All specimens could be evaluated as low risk. No statistically significant correlations were found between the MP abundances and D. gigas stomach weight, stomach fullness index, distance from the coast, and mantle length. While regression analyses showed that fuller stomachs contained larger MPs. Spatial variations in shape and polymer composition suggest that low-density fibrous MPs could be easily transported offshore by upwelling and/or ocean currents. These results indicate intraspecific variation in D. gigas MP contaminants and underscore the need to assess the potential site-specific ecotoxicological consequences of increased plastic pollution to pelagic squid.
1 Introduction
Since its emergence in the early 20th century, plastic products have become a widely used material based on their characteristics of durability, cheapness, and great convenience (Dehaut et al., 2019). However, the difficulties in degrading plastic have led to plastic waste causing serious environmental problems (Barnes et al., 2009). Recently, there has been growing concern about microplastics (MPs), defined as plastic particles smaller than 5 mm (Arthur et al., 2009). MPs can be divided into primary and secondary MPs. Primary MPs are formed on the microscale, such as microbeads in personal hygiene products, while secondary MPs result from the degradation of large-sized plastics through physical, chemical, biological and UV radiation (Gewert et al., 2015). Compared to large plastics, MPs are smaller, making them easier to disperse in different environments (Zhang T et al., 2021). As a result, MPs are ubiquitous in the marine environment and are readily ingested by marine organisms (Lusher et al., 2013), either through direct capture or by feeding on contaminated prey (Nelms et al., 2018). Previous studies showed that exposure of MPs in aquatic species around the world, such as zooplankton (Collignon et al., 2014; Botterell et al., 2019), crustaceans (Cau et al., 2019; Hara et al., 2020), cephalopods (Oliveira et al., 2020; Gong et al., 2021), and fishes (Lusher et al., 2013; Zhang et al., 2019; Huang et al., 2022). Consumption of MPs can cause not only physical harm (Cole et al., 2015) but also physiological harm (Lusher et al., 2013). In addition, the high hydrophobicity of MPs can result in toxic chemicals, such as persistent organic pollutants (POPs), and trace metals, and serve as carriers for pollutant diffusion (Brennecke et al., 2016).
Because most studies have focused on crustaceans and fish, the potential presence of MPs in cephalopods (particularly in pelagic squid) has not been extensively explored (Gong et al., 2021). In the present study, we focused on a commercially important species of pelagic squid, jumbo squid Dosidicus gigas. The commercial annual catches of this species varying between 0.8 and 1.2 million tonnes in 2014–2018 (FAO yearbook. Fishery and aquaculture statistics). D. gigas is considered a voracious and adaptable pelagic predator of a wide range of prey including crustaceans, fish, and cephalopods (including cannibalism) (Nigmatullin et al., 2001; Alegre et al., 2014). Given the high detection rate of MP ingestion reported in crustaceans (Watts et al., 2014; Zhang Z et al., 2021) and fish (Boerger et al., 2010; Gassel and Rochman, 2019), there is no doubt that the digestive system of D. gigas contain MPs. In addition, differences in dietary sources could result in spatial variability in the abundance and characteristics of MPs in D. gigas. This species is also the main prey items of marine top predators, such as tunas, sharks, and whales (Ruiz-Cooley et al., 2004). They can thus act as vectors for the transfer of MPs to high-trophic-level marine predators.
The aim of the present study was to determine the characteristics and spatial distribution of MPs in the D. gigas, and to conduct a risk assessment for the D. gigas in the tropical and southern temperate Pacific. Identifying the intraspecific variation of MP pollution is essential for establishing methods to assess the potential ecological impacts of MPs in highly migratory pelagic predators. The information obtained in this study will hopefully improve the understanding of MP accumulates in pelagic squid and its availability for incorporation into the food chain is fundamental for understanding threats to high-trophic-level marine predators around the world.
2 Materials and methods
2.1 Sample collection and preparation
Dosidicus gigas specimens were collected by scientific observers during nocturnal jigging operations between February and May 2019 (Figure 1). A total of 50 individuals were collected from the main fishing grounds in the tropical and Southern temperate Pacific (see Supplementary Table S1 for detailed sampling information). Specimens were immediately frozen on board and kept stored at −20°C until further analysis. Dorsal mantle length (ML) was recorded in the laboratory. The stomach was dissected and weighed for further analysis of MP. The stomach fullness index is assigned by visual observation (Breiby and Jobling, 1985): 0 (empty), 1 (few residues), 2 (half full), 3 (almost full), and 4 (full). The outer areas of the stomachs were rinsed with ultrapure water (Milli-Q water) to remove any adhered particles.
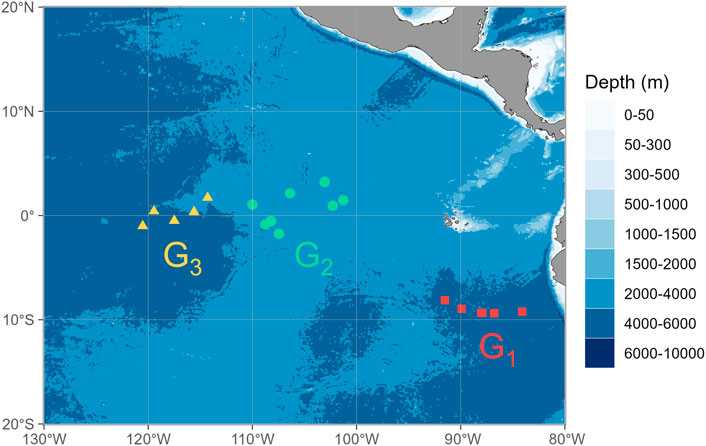
FIGURE 1. Locations where jumbo squid Dosidicus gigas specimens were sampled in the eastern tropical Pacific Ocean. Sampling locations are merged into three groups and indicated with squares, circles, and triangles for Group Ⅰ (G1), Group Ⅱ (G2), and Group Ⅲ (G3), respectively.
2.2 Extraction, observation, and identification of microplastics
Microplastic extraction was performed using the modified method of our previous study (Gong et al., 2021). The stomachs were transferred to Erlenmeyer flasks and treated with 10% KOH (20 mL per Gram of wet tissue), then covered with aluminum foil to avoid contamination. All Erlenmeyer flasks were placed in a constant temperature oscillator (70°C, 150 rpm) until the stomachs were completely digested. Thereafter, about 6 g NaCl per 20 mL mixture was added overnight for density separation. The suspension was vacuum filtered through a glass fiber filter (2.7 μm pore size, 47 mm diameter, Whatman Inc.).
Each filter was placed in a clean Petri dish pending analysis. The stereomicroscope (SZX2-FOF) and a U-TV0.63XC digital camera (both from Olympus, Tokyo, Japan) were used to observe the filters. All suspect MPs (>50 μm) were photographed and measured using ImageJ version 1.50 software. Shape and color were identified based on a visual assessment of the morphometric characteristics.
To confirm polymer composition, each suspected MP was scanned using a Micro-Fourier Transform Infrared Spectroscope (Micro-FTIR, Spotlight 400, PerkinElmer) in Attenuated Total Reflection mode. Spectra were collected over a broad spectral range (650–4,000 cm−1) at a resolution of 4 cm−1 from an average of 32 sample scans (D’Souza et al., 2020; Huang et al., 2022). The resulting spectra were compared to libraries of standard spectra. The polymer composition was only identified when the confidence level of at least a 70% match or reliable spectral match (after visual inspection) was accepted.
2.3 Quality control
Some measures to avoid potential contamination were implemented throughout: all the solvents (including Milli-Q water) used for sample processing and analysis were filtered over a glass fiber filter (2.7 μm pore size, 47 mm diameter, Whatman Inc.), all devices such as tweezers and Erlenmeyer flasks were thoroughly rinsed three times with filtered ultrapure water and dried; nitrile gloves and cotton laboratory clothing were always worn; experiments were conducted in a closed, ultra-clean laboratory to prevent potential airborne MPs. Clean Petri dishes were placed next to the working zone and checked after experiments. Reference materials such as vessel coatings and fishing gear were also collected. In the case of potential airborne contamination, the same characteristics of microparticles, according to size, shape, and color, were not included in this the results.
2.4 Risk assessment of microplastics
To the best of our knowledge, there is currently no standardized protocol for systematically evaluating the potential risks of MPs in pelagic squid. As a consequence, we applied MP abundance into consideration to assess the MP pollution risk in the D. gigas.
Risk assessment was expressed as pollution load index (Tomlinson et al., 1980). The formulas employed for PLI were as follows:
where PLIi is the pollution load index (Tomlinson et al., 1980) for individual i, Ci is the MP abundance for individual i and C0 are defined as the baseline MP abundance, which is theoretically a reference value of the minimum average MP abundance in squid species. Given the absence of a reference value for our study area and species, we followed the approach adopted in previous studies (Kabir et al., 2021; Zhang et al., 2022) and assigned C0 to the lowest MPs abundance of contaminated D. gigas obtained in this study. Variation in the selected constant does not affect the relative relationships of PLI among individuals, only absolute values, and therefore does not influence the comparison results.
2.5 Statistic analysis
To assess the potential spatial variations in MP characteristics of D. gigas, the specimens were merged into three main groups according to the sampling sites, i.e., Group Ⅰ (G1, 84°–92°W, N = 17), Group Ⅱ (G2, 100°–110°W, N = 22), and Group Ⅲ (G3, 114°–121°W, N = 11) (Figure 1). Comparisons among sampling groups were performed using the Kruskal-Wallis test since the assumptions of normality and homogeneity of the variances were not met. In addition, Spearman’s rank correlation was used to analyze the relationships between ML, stomach weight and fullness index, and distance from coast with MP characteristics. Regarding the relationship between the stomach weight and the number of MPs per Gram of stomach weight, this correlation test was considered eliminated in the analysis. Statistically significant results were indicated with p < 0.05. All results are presented as mean ± standard deviation (SD).
3 Results and discussion
D gigas is widely distributed in the eastern Pacific and its population structure is complex. Several studies have reported spatial variations of foraging strategies among population units within of D. gigas (Gong et al., 2020; Gao et al., 2022). For example, fishes were the main prey items of D. gigas collected off the coast of Chile (Pardo-Gandarillas et al., 2014) while cephalopods (including cannibalism) and fishes were the main prey items of D. gigas from the Ecuadorian waters (Rosas- Luis and Chompoy-Salazar, 2016) and the waters off Peru (Alegre et al., 2014). Although the stomach content of G2 and G3 individuals were not reported, previous studies performed on trophic ecology of D. gigas using stable isotope and fatty acid analyses indicate they might have specific dietary sources (Gong et al., 2020). It is generally accepted that MPs could be ingested by pelagic predators (e.g., D. gigas), either by direct capture or by feeding contaminated prey (Gong et al., 2021; Huang et al., 2022). In this study, stomachs from 50 individuals (16.0–37.8 cm ML) were used to test the hypothesis that the D. gigas from different areas have spatial variability in MP contamination. No difference in ML was observed between D. gigas collected from three sampling groups (G1: 22.5 ± 5.3 cm, G2: 22.2 ± 4.4 cm, G3: 23.3 ± 2.5 cm, Kruskal-Wallis, χ2 = 1.45, p = 0.49).
3.1 Microplastic abundance
The visual sorting initially identified a total of 197 suspected MPs. Of these, 44 MPs (22.34%) were confirmed to be plastic polymers by Micro-FTIR. Of all D. gigas analyzed, 25/50 (50.00%) specimens contained MPs and had an average abundance of 0.88 ± 1.12 items/individual and 0.24 ± 0.36 items/g tissue wet weight. Three sampling groups had a similar detection rate of MPs, 47.06% (G1), 50.00% (G2), and 54.55% (G3), respectively. The MP abundances for sampling groups were, G1: 1.00 ± 1.32 items/individual (0.16 ± 0.23 items/g), G2: 0.68 ± 0.84 items/individual (0.25 ± 0.34 items/g), G3: 1.09 ± 1.30 items/individual (0.35 ± 0.53 items/g) (Figure 2). Although MP abundances varied inconsistently across the G1, G2 and G3, no difference in MP abundance was observed among sampling groups (Kruskal-Wallis, items/individual: χ2 = 0.55, p = 0.76; items/g: χ2 = 0.57, p = 0.75). For all individuals, no significant Spearman correlations (0.19 < p < 0.95) were found between the MP abundances and the stomach weight, stomach fullness index, distance from coast, and ML of D. gigas.
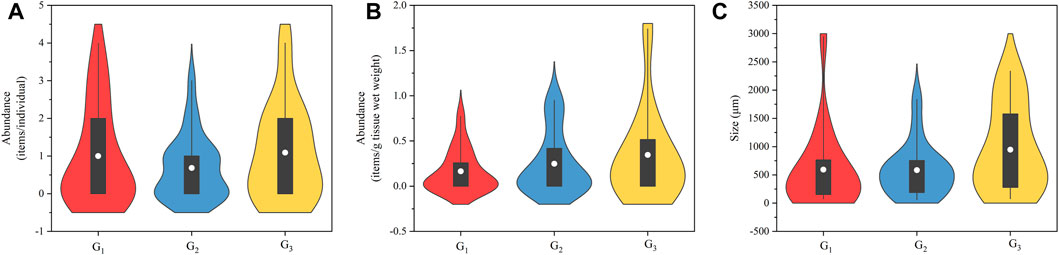
FIGURE 2. Violin plots of the abundance (A, B) and size (C) of microplastics in jumbo squid Dosidicus gigas collected from three areas (abbreviations as in Figure 1). The violin plot starts with a standard boxplot (with minimum, interquartile range, mean, and maximum values) and then adds rotating kernel density plots on each side of the boxplot.
Overall, these findings suggest that all sampling groups were influenced by similar level of MPs pollution. This phenomenon possibly due to MPs could be rapidly egested by D. gigas, which has a fast digestion rate (Gao et al., 2022). Until now, no evidence of MP accumulation in large predator has been documented in other studies (Chagnon et al., 2018). Therefore, the similar MP abundance in each group maybe driven by continuous ingestion and egestion of MPs rather than prey items or distance from coast.
3.2 Morphology, color, and chemical composition of microplastics
The size range of MPs in all individuals was 58.42–2,944.85 μm (685.86 ± 669.87 μm, Figure 2), with the smallest MPs found in the G2 and the largest found in the G1. The average size of MPs in each group was 592.37 ± 725.68 μm, 584.24 ± 475.07 μm, and 945.34 ± 774.49 μm, respectively. No difference in the size of MP was observed among sampling groups (Kruskal-Wallis, χ2 = 2.54, p = 0.28), possibly due to the significant positive Spearman correlations between MP size and stomach fullness index (0.41 ≤ rs ≤ 0.42, p < 0.04). In our study, three groups had similar body size as mentioned before and stomach fullness (Kruskal-Wallis, χ2 = 0.55, p = 0.76) which could result in the similar size of MP. In contrast, no significant Spearman correlations (0.21 < p < 0.62) were found between the MP size and the stomach weight and distance from coast of D. gigas.
Three shapes of MPs were recorded in all individuals, including fiber, fragment, and film (Figure 3). The most common MP type was fragment (54.55%), which differs from previous studies performed on D.gigas collected from the Southern waters of G1 (Gong et al., 2021). This inconsistency among studies may be linked to the D.gigas collected in this study had a relatively small body size. The fragments detected maybe came from the fragmentation of large-sized plastics (Yu et al., 2022; Zhang et al., 2022). The percentage of fibers reached 43.18% in all samples and a single piece of film was found in a specimen from G2. These fibrous MPs maybe sourced from the degradation of fishing gears and ropes, which would be consistent with previous results reported for marine animals from fishing grounds (Zhang et al., 2019; Yu et al., 2022). Fibrous MPs have larger aspect ratios with the rough surface, are easier to embed in tissues, and persist longer, which can cause mechanical damage and more severe toxicological effects on organisms compared to other types of MPs (Rebelein et al., 2021; Zheng et al., 2022). However, the prevalence of fibers in the samples may often be overestimated when the collection and analysis of the samples are not conducted under strictly controlled clean air (Hermsen et al., 2017).
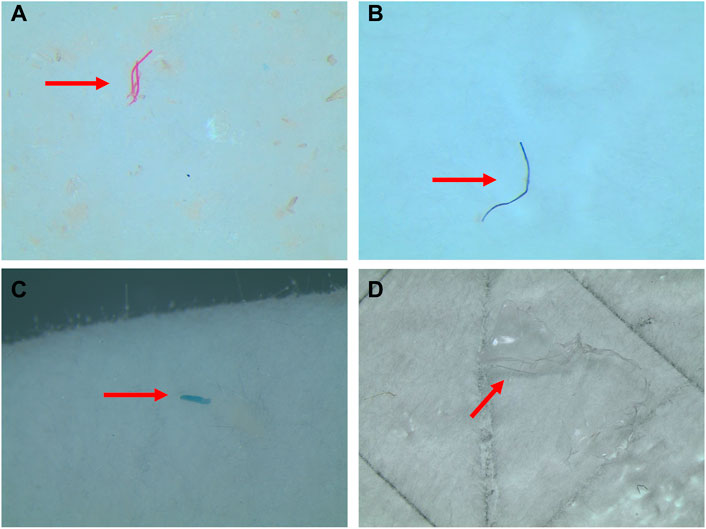
FIGURE 3. Examples of microplastics found in the stomach of jumbo squid Dosidicus gigas: fiber in red (A) and blue (B), fragment in blue(C), and film in transparent (D).
Different compositions of shapes were found among the sampling groups. MPs in G1 specimens had a higher proportion of fragments (76.47%), with the remaining fibers (23.53%). For G2 and G3 specimens, however, the MPs were dominated by the fibrous form (58.33% and 53.33%), with 41.67% and 40.00% MP fragments, respectively. This pattern might be due to fibrous MPs could easily be transported by ocean currents to offshore areas, which accounts for the higher detection rate of fibers that we observed in G2 and G3; however, the exact mechanism of transfer of fibrous MPs in this case remains to be explored.
Although color distributions varied inconsistently in each group, blue was the most dominant color (59.59% of all MPs), scoring 64.71%, 46.67%, and 66.67% in G1, G2, and G3, respectively. These findings match the high detection frequency and high amount of this MP color in the seawater and other squids (Zhang et al., 2019; Daniel et al., 2021; Zhang et al., 2022). The other colors of identified MPs were black, red, yellow, and MP in transparent film form (Figure 4).
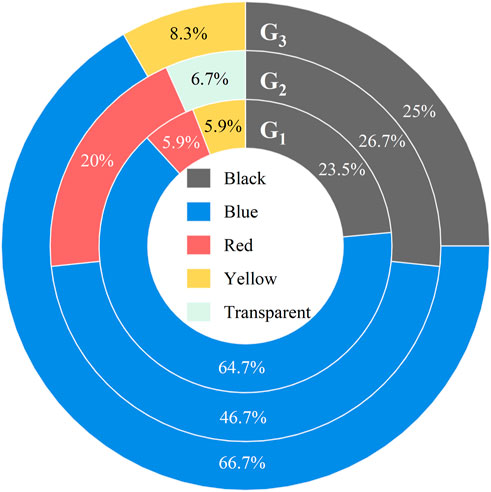
FIGURE 4. Microplastic color distribution of jumbo squid Dosidicus gigas from three sampling groups (abbreviations as in Figure 1) in the Eastern tropical Pacific Ocean.
Up to eleven polymer types were determined (Supplementary Figure S1). In all samples, polyethylene terephthalate (PET) was identified most frequently at 31.82% and reached 35.29%, 40.00%, and 16.67% in G1, G2, and G3, respectively. PET is employed primarily as a textile, with applications in clothing, ropes, and fishing gears (Šaravanja et al., 2022). The other frequently polymers were cellophane (CP, 18.18%) and polystyrene (PS, 11.36%), and the remaining polymer accounted for less than 10.00% of the MPs detected (Figure 5). These polymers are ubiquitous in the marine environment (Alomar and Deudero, 2017; Li et al., 2019; Liu et al., 2020). PET (1.34 g/cm3), CP (1.42 g/cm3), and PS (1.05 g/cm3) have a higher density than seawater (1.025 g/cm3) and tend to sink into deeper water layers (Woodall et al., 2014), making themselves available to organisms living in these areas. D. gigas spends the vast majority of its time in the deep-sea hypoxic environment may be the cause for the high proportion of these high-density MPs in our study (Stewart et al., 2013). D. gigas is also considered highly migratory, undertake ontogenetic migration between the continental shelf and the open ocean (Gilly et al., 2006; Stewart et al., 2013). They can thus act as important significant bioindicators of MP contamination in a vast three-dimensional space. However, low-density polymers (e.g., polypropylene (PP), 0.90–0.92 g/cm3; polydimethylsiloxane (PDMS), 0.98 g/cm3; and styrene-butadiene rubber (SBR), 0.94 g/cm3), were also found in the D. gigas (Figure 5), possibly because of biofouling and subsequent vertical transport (Kooi et al., 2017; Hipfner et al., 2018; Liu et al., 2020). Interestingly, the low-density polymers were only detected in D. gigas from G2 and G3, which were far from the coast than G1 (Figure 1). Possibly, offshore Ekman transport off Peru could move low-density polymer MPs to relatively farther offshore, and D. gigas feeding in the nearshore waters (i.e., G1) might be exposed to more high-density polymers than in offshore waters (i.e., G2 and G2) (Thiel et al., 2007; Ory et al., 2018). It is noteworthy that the distribution of polymers in seawater of our study area has not yet been published, further efforts are needed to conclude the potential relationship between microplastic transport and Ekman dynamics.
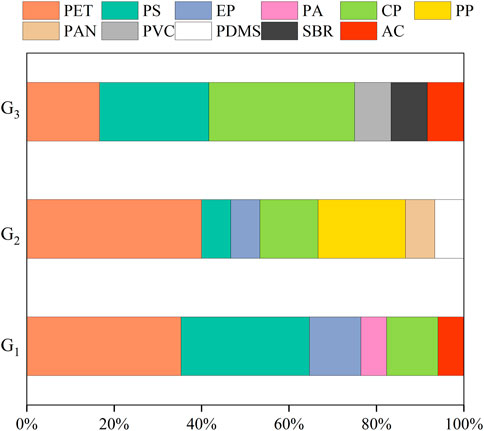
FIGURE 5. Proportions of microplastic polymers among jumbo squid Dosidicus gigas specimens from three sampling groups (abbreviations as in Figure 1 in the Eastern tropical Pacific Ocean. PET: polyethylene terephthalate, PS: polystyrene, EP: epoxy resin, PA: polyamide, CP: cellophane, PP: polypropylene, PAN: polyacrylonitrile, PVC: polyvinyl chloride, PDMS: polydimethylsiloxane, SBR: styrene butadiene rubber, AC: Acrylates copolymer.
3.3 Risk assessment of microplastics
Regarding wild marine organisms, there is currently no standardized method to monitor the risk of MPs, while MP abundance is a useful indicator to assess the potential risks of MPs (Xu et al., 2018; Kabir et al., 2021).
Based on the number of MPs per individual, the PLI values for sampling groups were 0.66 ± 0.77, 0.57 ± 0.61, and 0.75 ± 0.76, respectively. For the number of MPs per Gram of stomach weight, the PLI values were 1.04 ± 1.24, 1.31 ± 1.49, and 1.57 ± 1.77, respectively. No difference in PLI values was found among the sampling groups (Kruskal-Wallis, items/individual: χ2 = 0.54, p = 0.76; items/g: χ2 = 0.57, p = 0.75). These values indicated that all individuals had a very low risk in the study area (Xu et al., 2018).
Overall, this study is the first record of the intraspecific variation in MP contamination levels in pelagic squid from oceanic habitats and also provides an preliminary examination of the risk assessment of MPs. Spatial diversity of MP shape and polymer type was found. The pollution risk assessment showed that all D. gigas were at low risk of pollution. The site-specific MP contaminants may be used to facilitate priority pollution monitoring. The pollution risk assessment provided insights into pollution and a basis for future comprehensive environmental and human health risk assessments in the context of increased plastic pollution from pelagic squid.
Data availability statement
The raw data supporting the conclusion of this article will be made available by the authors, without undue reservation.
Ethics statement
The animal study was reviewed and approved by Institutional animal care and use Committee of Shanghai Ocean University.
Author contributions
YW: Conceptualization, methodology, formal analysis, writing—original draft. XC: Methodology, validation, formal analysis, resources, supervision, funding acquisition.
Funding
This work was supported by the project on the survey and monitor-evaluation of global fishery resources sponsored by the Ministry of Agriculture and Rural Affairs.
Acknowledgments
The authors thank all the staff at Shanghai Ocean University for their assistance with sample pretreatment. We also thank Yi Gong for assistance with data analysis and interpretation.
Conflict of interest
The authors declare that the research was conducted in the absence of any commercial or financial relationships that could be construed as a potential conflict of interest.
Publisher’s note
All claims expressed in this article are solely those of the authors and do not necessarily represent those of their affiliated organizations, or those of the publisher, the editors and the reviewers. Any product that may be evaluated in this article, or claim that may be made by its manufacturer, is not guaranteed or endorsed by the publisher.
Supplementary material
The Supplementary Material for this article can be found online at: https://www.frontiersin.org/articles/10.3389/fenvs.2023.1069124/full#supplementary-material
Supplementary Figure S1 | Photographs of the spectra of the FTIR analyses. A, polyvinyl chloride; B, polypropylene; C, polyacrylonitrile; D, acrylates copolymer; E, polydimethylsiloxane; F, epoxy resin.
Supplementary Table S1 | Summary information of jumbo squid Dosidicus gigas specimens sampled in the Eastern tropical Pacific Ocean.
References
Alegre, A., M´enard, F., Tafur, R., Espinoza, P., Argüelles, J., Maehara, V., et al. (2014). Comprehensive model of jumbo squid Dosidicus gigas trophic ecology in the northern Humboldt current system. PLoS One 9, e85919. doi:10.1371/journal.pone.0085919
Alomar, C., and Deudero, S. (2017). Evidence of microplastic ingestion in the shark Galeus melastomus Rafinesque, 1810 in the continental shelf off the Western Mediterranean Sea. Environ. Pollut. 223, 223–229. doi:10.1016/j.envpol.2017.01.015
Arthur, C., Baker, J., and Bamford, H. (2009). Proceedings of the international research workshop on the occurrence, effects, and fate of microplastic marine debris. Group 530.
Barnes, D. K., Galgani, F., Thompson, R. C., and Barlaz, M. (2009). Accumulation and fragmentation of plastic debris in global environments. Philosophical Trans. R. Soc. B Biol. Sci. 364 (1526), 1985–1998. doi:10.1098/rstb.2008.0205
Boerger, C. M., Lattin, G. L., Moore, S. L., and Moore, C. J. (2010). Plastic ingestion by planktivorous fishes in the north pacific central gyre. Mar. Pollut. Bull. 60 (12), 2275–2278. doi:10.1016/j.marpolbul.2010.08.007
Botterell, Z. L. R., Beaumont, N., Dorrington, T., Steinke, M., Thompson, R. C., and Lindeque, P. K. (2019). Bioavailability and effects of microplastics on marine zooplankton: A review. Environ. Pollut. 245, 98–110. doi:10.1016/j.envpol.2018.10.065
Breiby, A., and Jobling, M. (1985). Predatory role of the flying squid Todarodes sagittatus in North Norwegian waters. NAFO Sci. Counc. Stud. 9, 125–132.
Brennecke, D., Duarte, B., Paiva, F., Caçador, I., and Canning-Clode, J. (2016). Microplastics as vector for heavy metal contamination from the marine environment. Estuar. Coast. Shelf Sci. 178, 189–195. doi:10.1016/j.ecss.2015.12.003
Cau, A., Avio, C. G., Dessì, C., Follesa, M. C., Moccia, D., Regoli, F., et al. (2019). Microplastics in the crustaceans Nephrops norvegicus and Aristeus antennatus: Flagship species for deep-sea environments? Environ. Pollut. 255, 113107. doi:10.1016/j.envpol.2019.113107
Chagnon, C., Thiel, M., Antunes, J., Ferreira, J. L., Sobral, P., and Ory, N. C. (2018). Plastic ingestion and trophic transfer between Easter Island flying fish (Cheilopogon rapanouiensis) and yellowfin tuna (Thunnus albacares) from Rapa Nui (Easter Island). Environ. Pollut. 243, 127–133. doi:10.1016/j.envpol.2018.08.042
Cole, M., Lindeque, P., Fileman, E., Halsband, C., and Galloway, T. S. (2015). The impact of polystyrene microplastics on feeding, function and fecundity in the marine copepod Calanus helgolandicus. Environ. Sci. Technol. 49 (2), 1130–1137. doi:10.1021/es504525u
Collignon, A., Hecq, J. H., Galgani, F., Collard, F., and Goffart, A. (2014). Annual variation in neustonic micro- and meso-plastic particles and zooplankton in the Bay of Calvi (Mediterranean-Corsica). Mar. Pollut. Bull. 79 (1-2), 293–298. doi:10.1016/j.marpolbul.2013.11.023
Daniel, D. B., Ashraf, P. M., Thomas, S. N., and Thomson, K. T. (2021). Microplastics in the edible tissues of shellfishes sold for human consumption. Chemosphere 264, 128554. doi:10.1016/j.chemosphere.2020.128554
Dehaut, A., Hermabessiere, L., and Duflos, G. (2019). Current frontiers and recommendations for the study of microplastics in seafood. Trends Anal. Chem. 116, 346–359. doi:10.1016/j.trac.2018.11.011
D'Souza, J. M., Windsor, F. M., Santillo, D., and Ormerod, S. J. (2020). Food web transfer of plastics to an apex riverine predator. Glob. Chang. Biol. 26, 3846–3857. doi:10.1111/gcb.15139
Gao, X., Gong, Y., Chen, X., and Li, Y. (2022). Dietary shifts and niche partitioning throughout ontogeny reduce intraspecific competition in a pelagic generalist predator. Mar. Ecol. Prog. Ser. 692, 81–97. doi:10.3354/meps14079
Gassel, M., and Rochman, C. M. (2019). The complex issue of chemicals and microplastic pollution: A case study in north pacific lanternfish. Environ. Pollut. 248, 1000–1009. doi:10.1016/j.envpol.2019.03.002
Gewert, B., Plassmann, M. M., and MacLeod, M. (2015). Pathways for degradation of plastic polymers floating in the marine environment. Environ. Sci. Process. impacts 17, 1513–1521. doi:10.1039/c5em00207a
Gong, Y., Li, Y., Chen, X., and Yu, W. (2020). Trophic niche and diversity of a pelagic squid (Dosidicus gigas): A comparative study using stable isotope, fatty acid, and feeding apparatuses morphology. Front. Mar. Sci. 7, 642. doi:10.3389/fmars.2020.00642
Gong, Y., Wang, Y., Chen, L., Li, Y., Chen, X., and Liu, B. (2021). Microplastics in different tissues of a pelagic squid (Dosidicus gigas) in the northern Humboldt Current ecosystem. Mar. Pollut. Bull. 169, 112509. doi:10.1016/j.marpolbul.2021.112509
Gilly, W. F., Markaida, U., Baxter, C. H., Block, B. A., Boustany, A., Zeidberg, L., et al. (2006). Vertical and horizontal migrations by the jumbo squid Dosidicus gigas revealed by electronic tagging. Mar. Ecol. Prog. Ser. 324, 1–17. doi:10.3354/meps324001
Hara, J., Frias, J., and Nash, R. (2020). Quantification of microplastic ingestion by the decapod crustacean Nephrops norvegicus from Irish waters. Mar. Pollut. Bull. 152, 110905. doi:10.1016/j.marpolbul.2020.110905
Hermsen, E., Pompe, R., Besseling, E., and Koelmans, A. (2017). Detection of low numbers of microplastics in North Sea fish using strict quality assurance criteria. Mar. Pollut. Bull. 122, 253–258. doi:10.1016/j.marpolbul.2017.06.051
Hipfner, J. M., Galbraith, M., Tucker, S., Studholme, K. R., Domalik, A. D., Pearson, S. F., et al. (2018). Two forage fishes as potential conduits for the vertical transfer of microfibres in Northeastern Pacific Ocean food webs. Environ. Pollut. 239, 215–222. doi:10.1016/j.envpol.2018.04.009
Huang, X., Gao, H., Li, Z., Wu, F., Gong, Y., and Li, Y. (2022). Microplastic contamination and risk assessment in blue shark (Prionace glauca) from the eastern tropical Pacific ocean. Mar. Pollut. Bull. 184, 114138. doi:10.1016/j.marpolbul.2022.114138
Kabir, A. H. M. E., Sekine, M., Imai, T., Yamamoto, K., Kanno, A., and Higuchi, T. (2021). Assessing small-scale freshwater microplastics pollution, land-use, source-to-sink conduits, and pollution risks: Perspectives from Japanese rivers polluted with microplastics. Sci. Total Environ. 768, 144655. doi:10.1016/j.scitotenv.2020.144655
Kooi, M., Van Nes, E. H., Scheffer, M., and Koelmans, A. A. (2017). Ups and downs in the Ocean: Effects of biofouling on vertical transport of microplastics. Environ. Sci. Technol. 51, 7963–7971. doi:10.1021/acs.est.6b04702
Li, J., Lusher, A. L., Rotchell, J. M., Deudero, S., Turra, A., Bråte, I. L. N., et al. (2019). Using mussel as a global bioindicator of coastal microplastic pollution. Environ. Pollut. 244, 522–533. doi:10.1016/j.envpol.2018.10.032
Liu, K., Courtene-Jones, W., Wang, X., Song, Z., Wei, N., and Li, D. (2020). Elucidating the vertical transport of microplastics in the water column: A review of sampling methodologies and distributions. Water Res. 186, 116403. doi:10.1016/j.watres.2020.116403
Lusher, A. L., McHugh, M., and Thompson, R. C. (2013). Occurrence of microplastics in the gastrointestinal tract of pelagic and demersal fish from the English Channel. Mar. Pollut. Bull. 67 (1-2), 94–99. doi:10.1016/j.marpolbul.2012.11.028
Nelms, S. E., Galloway, T. S., Godley, B. J., Jarvis, D. S., and Lindeque, P. K. (2018). Investigating microplastic trophic transfer in marine top predators. Environ. Pollut. 238, 999–1007. doi:10.1016/j.envpol.2018.02.016
Nigmatullin, C. M., Nesis, K. N., and Arkhipkin, A. I. (2001). A review of the biology of the jumbo squid Dosidicus gigas (Cephalopoda: Ommastrephidae). Fish. Res. 54, 9–19. doi:10.1016/S0165-7836(01)00371-X
Oliveira, A. R., Sardinha-silva, A., Andrews, P. L. R., Green, D., Cooke, G. M., Hall, S., et al. (2020). Microplastics presence in cultured and wild-caught cuttlefish, Sepia officinalis. Mar. Pollut. Bull. 160, 111553. doi:10.1016/j.marpolbul.2020.111553
Ory, N., Chagnon, C., Felix, F., Fernández, C., Ferreira, J. L., Gallardo, C., et al. (2018). Low prevalence of microplastic contamination in planktivorous fish species from the southeast Pacific Ocean. Mar. Pollut. Bull. 127, 211–216. doi:10.1016/j.marpolbul.2017.12.016
Pardo-Gandarillas, M. C., Lohrmann, K. B., George-Nascimento, M., and Ibáñez, C. M. (2014). Diet and parasites of the jumbo squid Dosidicus gigas in the humboldt current system. Molluscan Res. 34 (1), 10–19. doi:10.1080/13235818.2013.860870
Rebelein, A., Int-veen, I., Kammann, U., and Scharsack, J. P. (2021). Microplastic fibers-Underestimated threat to aquatic organisms? Sci. Total Environ. 777, 146045. doi:10.1016/j.scitotenv.2021.146045
Rosas-Luis, R., and Chompoy-Salazar, L. (2016). Description of food sources used by jumbo squid Dosidicus gigas (D’Orbigny, 1835) in Ecuadorian waters during 2014. Fish. Res. 173, 139–144. doi:10.1016/j.fishres.2015.08.006
Ruiz-Cooley, R. I., Gendron, D., Aguíñiga, S., Mesnick, S., and Carriquiry, J. D. (2004). Trophic relationships between sperm whales and jumbo squid using stable isotopes of C and N. Mar. Ecol. Prog. Ser. 277, 275–283. doi:10.3354/meps277275
Šaravanja, A., Pušić, T., and Dekanić, T. (2022). Microplastics in wastewater by washing polyester fabrics. Materials 15 (7), 2683. doi:10.3390/ma15072683
Stewart, J. S., Field, J. C., Markaida, U., and Gilly, W. F. (2013). Behavioral ecology of jumbo squid (Dosidicus gigas) in relation to oxygen minimum zones. Deep Sea Res. Part II Top. Stud. Oceanogr. 95, 197–208. doi:10.1016/j.dsr2.2012.06.005
Thiel, M., Macaya, E., Acuña, E., Arntz, W., Bastias, H., Brokordt, K., et al. (2007). The Humboldt current system of northern and Central Chile. Oceanogr. Mar. Biol. 45, 195–344. doi:10.1201/9781420050943.ch6
Tomlinson, D. L., Wilson, J. G., Harris, C. R., and Jeffrey, D. W. (1980). Problems in the assessment of heavy-metal levels in estuaries and the formation of a pollution index. Helgoländer Meeresun 33 (1-4), 566–575. doi:10.1007/bf02414780
Watts, A. J. R., Lewis, C., Goodhead, R. M., Beckett, S. J., Moger, J., Tyler, C. R., et al. (2014). Uptake and retention of microplastics by the shore crab Carcinus maenas. Environ. Sci. Technol. 48, 8823–8830. doi:10.1021/es501090e
Woodall, L. C., Sanchez-Vidal, A., Canals, M., Paterson, G. L. J., Coppock, R., Sleight, V., et al. (2014). The deep sea is a major sink for microplastic debris. R. Soc. open Sci. 1 (4), 140317. doi:10.1098/rsos.140317
Xu, P., Peng, G., Su, L., Gao, Y., Gao, L., and Li, D. (2018). Microplastic risk assessment in surface waters: A case study in the changjiang estuary, China. Mar. Pollut. Bull. 133, 647–654. doi:10.1016/j.marpolbul.2018.06.020
Yu, W., Chen, J., Zhang, S., Zhao, Y., Fang, M., Deng, Y., et al. (2022). Extraction of biodegradable microplastics from tissues of aquatic organisms. Sci. Total Environ. 838, 156396. doi:10.1016/j.scitotenv.2022.156396
Zhang, F., Wang, X., Xu, J., Zhu, L., Peng, G., Xu, P., et al. (2019). Food-web transfer of microplastics between wild caught fish and crustaceans in East China Sea. Mar. Pollut. Bull. 146, 173–182. doi:10.1016/j.marpolbul.2019.05.061
Zhang, T., Sun, Y., Song, K., Du, W., Huang, W., Gu, Z., et al. (2021). Microplastics in different tissues of wild crabs at three important fishing grounds in China. Chemosphere 271, 129479. doi:10.1016/j.chemosphere.2020.129479
Zhang, Z., Gao, S. H., Luo, G., Kang, Y., Zhang, L., Pan, Y., et al. (2021). The contamination of microplastics in China's aquatic environment: Occurrence, detection and implications for ecological risk. Environ. Pollut. 296, 118737. doi:10.1016/j.envpol.2021.118737
Zhang, F., Peng, G., Xu, P., Zhu, L., Li, C., Wei, N., et al. (2022). Ecological risk assessment of marine microplastics using the analytic hierarchy process: A case study in the yangtze river estuary and adjacent marine areas. J. Hazard. Mater. 425, 127960. doi:10.1016/j.jhazmat.2021.127960
Keywords: dosidicus gigas, pelagic squid, spatial variation, stomach, risk assessment, eastern tropical pacific ocean
Citation: Wang Y and Chen X (2023) Microplastics in a pelagic squid (Dosidicus gigas) from the Eastern tropical Pacific Ocean: Characteristics, spatial variation, and preliminary risk assessment. Front. Environ. Sci. 11:1069124. doi: 10.3389/fenvs.2023.1069124
Received: 13 October 2022; Accepted: 16 January 2023;
Published: 03 February 2023.
Edited by:
Nsikak U. Benson, Covenant University, NigeriaReviewed by:
David Chelazzi, University of Florence, ItalyGabriel Enrique De-la-Torre, Saint Ignatius of Loyola University, Peru
Atsuhiko Isobe, Kyushu University, Japan
Marta Gouveia Martins, New University of Lisboa, Portugal
Copyright © 2023 Wang and Chen. This is an open-access article distributed under the terms of the Creative Commons Attribution License (CC BY). The use, distribution or reproduction in other forums is permitted, provided the original author(s) and the copyright owner(s) are credited and that the original publication in this journal is cited, in accordance with accepted academic practice. No use, distribution or reproduction is permitted which does not comply with these terms.
*Correspondence: Yaxin Wang, eXh3YW5nQHNob3UuZWR1LmNu