- 1Faculty of Environmental Science and Engineering, Kunming University of Science and Technology, Kunming, China
- 2Xinjiang Institute of Ecology and Geography, Chinese Academy of Sciences, Urumqi, China
- 3Cele National Station of Observation and Research for Desert-Grassland Ecosystems, Chinese Academy of Sciences, Urumqi, China
- 4Center of Research, Faculty of Engineering, Future University in Egypt, New Cairo, Egypt
- 5Department of Agricultural Engineering, Khwaja Fareed University of Engineering and Information Technology, Rahim Yar Khan, Pakistan
- 6Department of Pharmacognosy, Faculty of Pharmacy, Umm Al-Qura University, Makkah, Saudi Arabia
- 7Department of Botany, Government College University, Lahore, Pakistan
- 8Department of Agronomy, Faculty of Agriculture and Environment, The Islamia University of Bahawalpur, Bahawalpur, Pakistan
- 9Department of Biochemistry and Molecular Biology, University of Nevada, Reno, NV, United States
- 10Soil and Water Testing Laboratory for Research, Multan, Pakistan
- 11Research Center on Ecological Sciences, Jiangxi Agricultural University, Nanchang, China
- 12Center for Plant Sciences and Biodiversity, University of Swat, Charbagh, Pakistan
- 13Department of Genetics and Development, Columbia University Irving Medical Center, New York, NY, United States
Biochars provide several agricultural and environmental benefits, such as soil health improvement, better crop growth and yield, carbon sequestration, decreasing greenhouse gas (GHGs) emissions, and regulation of nutrient dynamics. This review highlights the role of biochar in transforming the soil’s physiochemical and biological properties, and their impact on improving seed germination and seedling growth, altering crop physiological attributes, enhancing crop resistance against biotic and abiotic stresses, improving crop productivity, curtailing GHGs, and controlling nutrient leaching losses. However, the type of feedstock used, pyrolysis temperature, application rate and method, soil type and crop species largely influence the biochar performance under different environmental conditions. Application of biochars at low rates help to promote seed germination and seedling growth. Biochar modified the abiotic and microbial processes in the rhizosphere and increased nutrient mineralization and enhanced the nutrient availability for plant uptake. Hence, biochar enhanced the plant resistance against diseases, reduced the availability of heavy metals and improved the plant resilience against environmental stressors. By providing a comprehensive analysis about the variable impacts of biochars on soil physicochemical properties, plant growth, development and productivity and mitigating environmental problems, this review is quite valuable for developing an efficient soil and crop specific biochar with desired functionalities. It could be helpful in improving crop productivity, ensuring food security and better management of environment. Furthermore, this review identifies the knowledge gaps and suggests future outlooks for the commercialization of biochar applications on large-scale.
1 Introduction
Degradation of soil resulting from extensive agricultural practices and changing climatic conditions pose serious threats to global food security (Al-wabel et al., 2015). The exponentially increasing greenhouse gas (GHGs) emissions due to various anthropogenic activities are also detrimental to the environment and sustainable farming systems (Kumar et al., 2018). Additionally, the increasing world population, which is expected to reach 9.8 billion by 2050, will also put the world’s agricultural system under enormous pressure (Ayaz et al., 2021). Hence to feed the burgeoning population, meet the exacerbating food demand, and mitigation of climate change impacts need benign and cost effective strategies which can improve soil health, enhance crop yield and ensure a sustainable farming system and environment (Singh R. et al., 2022). Endowed with unique attributes such as larger specific surface area (SSA), abundant surface functional groups, porous structure, better cation exchange capacity (CEC), embedded minerals, strong adsorption capacity, micronutrients, and high environmental stability, biochar has appeared as a promising material for soil management, soil fertility improvement, reduction in GHGs and environmental management (Abhishek et al., 2022).
Biochar is a carbonaceous material generated from the decomposition of various feedstocks by the pyrolysis process (slow, intermediate, fast pyrolysis, and gasification) under oxygen-restricted conditions (Rady et al., 2016). A wide array of positive impacts is associated with biochar addition, such as increased soil microbial activities, enhanced soil nutrient uptake by plants (Zornoza et al., 2016), improved soil nutrient availability (Ding et al., 2016a), and decreased nutrient leaching (Yin Y. et al., 2021). Furthermore, it improves soil aeration, porosity, bulk density, infiltration rate, aggregate stability, water holding capacity, hydraulic conductivity (Foster et al., 2016), stabilizes heavy metals, and limits their bioavailability to crops growing in hostile or poor quality soils (Gasco et al., 2016a). Biochar also promotes microbial abundance (Zheng et al., 2017) and alleviates heat, drought, and salinity stress effects on crops. It enhances crop growth and productivity (Murtaza et al., 2021a), increases biological N fixation in legumes (Osman et al., 2022), and facilitates carbon sequestration. However, the outcomes mentioned above largely depend on biochar type, the temperature at which biochar is prepared, biochar dose, and soil type.
Due to the heterogeneity of biochar and the complexity of the physio-biochemical characteristics and microbiological processes underlying its effects, biochar manifests different responses under different conditions (Downie et al., 2009; Joseph et al., 2021). Therefore it becomes crucial to elucidate the soil and plant responses against various biochars prepared under different conditions with several diverse properties, particularly under changing climates (Kavitha et al., 2018). It would help to elaborate the underlying mechanisms that govern plant responses related to biochar addition and optimization of biochar preparation method, feedstock type, dose, and method of biochar application for a specific crop grown in a particular soil type. Such optimization would render biochar commercialization on a large scale with multiple benefits such as soil health improvement, yield increase, GHG reduction, and climate change mitigation. Although a variety of recent reviews (Kavitha et al., 2018; El-Naggar et al., 2019; Sakhiya et al., 2020; Bolan et al., 2022; Abhishek et al., 2022; Amalina et al., 2022; Uday et al., 2022) have presented potential benefits of biochar applications across different fields. However, synthesis and current knowledge on biochar-soil-plant interactions is direly needed to elucidate the soil and plant responses to different biochars by considering the type of feedstock, pyrolysis temperature, and biochar application and management practices under different environmental conditions. By providing a comprehensive analysis about the variable impacts of biochars on soil physicochemical properties, plant growth, development and productivity and environmental stress mitigation, this review is quite valuable for developing a soil and crop specific biochar with desired functionalities. It could help to improve crop productivity and sustaining food security under changing climatic conditions.
2 Biochar as a soil ameliorator
Biochar’s physiochemical properties can directly and indirectly affect the soil attributes. After biochar’s addition to soil, its contribution to soil’s physical structure may be significant, as it influences the soil aeration, water holding capacity (WHC), bulk density (BD), and distribution of pore size, porosity, and surface area of soil. Furthermore, several biological and chemical properties of soil can be altered through biochar addition (Figure 1). All these impacts of biochar are discussed in the section given below.
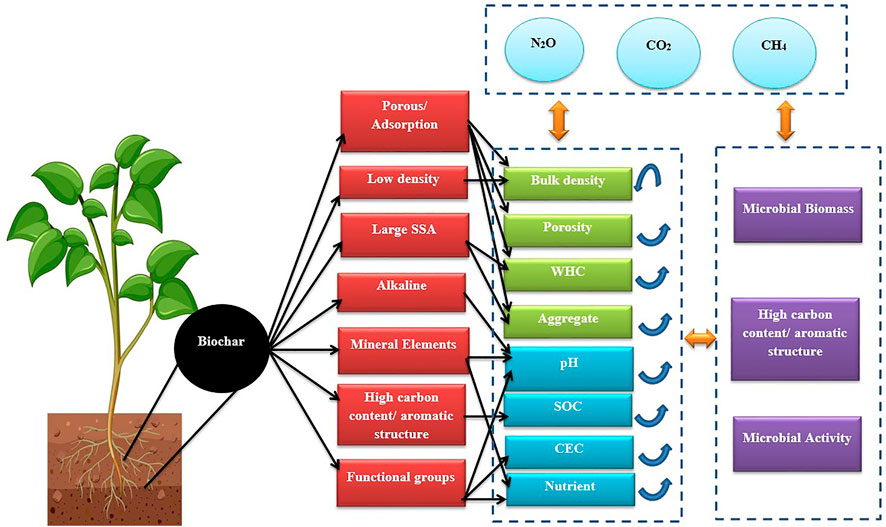
FIGURE 1. Effect of biochar application on soil physicochemical and biological properties; Carbon dioxide (CO2), Cation exchange capacity (CEC), Nitrous oxide (N2O), Methane (CH4), Soil organic carbon (SOC), Specific surface area (SSA), Water holding capacity (WHC).
2.1 Effects on physical attributes of soil
Several studies described that biochar addition improves the physical attributes of soil via reducing bulk density, increasing porosity, and enhancing water retention and aggregation (Baiamonte et al., 2015; Ding et al., 2016b; Carvalho et al., 2020; Seitz et al., 2020). Different kinds of biochars, when added in a sufficient amount into different soils, substantially amend the various soil physical attributes (Table 1). Soil bulk density (BD) is an important indicator of soil physical condition because it indicates the arrangement and packing of soil particles (Singh et al., 2019; Bhat et al., 2022). Low soil BD enhances soil composition, decreases soil compaction, and improves WHC and nutrient release (Tang et al., 2022). Biochar application decreases both the bulk and particle densities of soils (Munoz et al., 2016). This decrease could be attributed to the lower BD (.6 g cm−3) and particle density (1.5–2.0 g cm−3) of biochars as compared to the 1.25 g cm−3 BD and 2.4–2.8 g cm−3 particle density of soils (Yu et al., 2019). Biochar BD varies depending upon the type of feedstock and preparation conditions. For instance, BD of wood biochar is 1.30 g cm−3, woody forest residue biochar is .09 g cm−3, straw biochar is 1.30 g cm−3, maize cob biochar is .29–.36 g cm−3 and rice husk biochar is .37 g cm−3 (Sun and Lu, 2014; Obia et al., 2016; Zhang et al., 2021).
The reduction in soil BD after biochar addition results in an overall increase in soil porosity (Qin et al., 2016). The increase in soil porosity is attributed to the biochar porosity (70%–90%) and further contributed by increased soil aggregation, reduced bulk density, reduction of soil packing and interaction with mineral soil particles (Blanco-Canqui, 2017). Biochar application elevates the total porosity especially the micropores because they help to form porous material (Yang C. et al., 2021). The micropores enhance the water retention, whereas macropores improve the drainage (Baiamonte et al., 2019). The soil porosity was observed to increase in the range of 5–25 µm after biochar amendment (Rasa et al., 2018). Increase in soil porosity can enhance the movement of gases, water, and heat in the soils (Yu et al., 2019). The type of feedstock used for biochar production significantly influences the soil porosity pattern (Yang Q. et al., 2021). Woody biochars contain higher porosity compared to the biochars prepared from crop residues, which causes difference in the biomass cell structure, composition, size, and shape (Edeh et al., 2020). Soil WHC is also affected by the porous structure of the biochar (Bhat et al., 2022). The high porosity and specific surface area (SSA) increase the WHC, reduce the soil water permeability resistance, and change the water flow direction and residence time in soil (Abrol et al., 2016). The improvement in soil WHC after the addition of biochar is attributed to the rise in pore space of soil and biochar mixture. This mixing resulted in increased number of water holding sites due to large number of pores which increased the WHC of soil amended with biochar (Yang C. et al., 2021).
2.2 Effects on chemical attributes of soil
Biochar application may change the soil’s chemical attributes, such as increasing soil organic carbon, CEC, and pH (Abbasi and Anwar, 2015). However, alteration in soil chemical attributes by biochar application is mainly dependent upon biomass, biochar production temperature, types of soils, and biochar application rates (Table 2). It is considered that alkaline biochars (having pH > 7), when added to soil, elevates the pH of soil (Roberts et al., 2015). The biochar pH is largely dependent upon the type of feedstock used, and it may vary from acidic to alkaline. The biochar produced from different agricultural residues, raised the pH of soil from 4.59 to 4.86, 4.8 to 6.3, and 4.3 to 4.6 (Abhishek et al., 2022). Levesque et al. (2021) observed enhanced soil pH by 1 unit after application of Acer saccharum-biochar into clay soil. It was attributed due to the biochar’s high ash content and pH, great liming factors contributing to enhance pH of soil. He et al. (2021) applied rice straw biochar to notice the change in the attributes of acidic paddy soil. They observed that pH buffering capacity and resistance to paddy soil acidification were efficiently improved with biochar application. Biochar application after the wet-dry cycle increased the pH of acidic paddy soil (Hafeez et al., 2021). They proposed that biochar is a dominant solution to remediate acidic soil. The basic mechanism is that the weak acid functional groups on the surface of biochar mainly appear in the form of organic anions under neutral and alkaline soils. However, under soil acidification, these organic anions protonated with H+ and converted into neutral molecules, thus inhibiting the soil acidification and decreasing pH of soil (Wu et al., 2020).
Mostly studies focus on the effect of biochar application in acidic soils due to its potential of increasing pH (Novak et al., 2014; Edenborn et al., 2015), however discussion regarding the effect of biochar on calcareous soils is very limited (Zhang D. et al., 2015). This could be attributed to the buffering capacity of the calcareous soils resisting the alkaline effects of biochar (Usman et al., 2016; Al-Wabel et al., 2017).
CEC is an indirect measure of soil capacity to retain nutrients and water (Alkharabsheh et al., 2021). The biochar CEC is determined as the number of cations adsorbed on the surface of biochar (Zhang et al., 2021). Feedstock material, pyrolysis temperature, and functional groups are the key factors that govern the biochar CEC (Murtaza et al., 2021b). The CEC of biochars reduces with the elevation of pyrolysis temperature owing to the loss of functional negatively charged groups and low pyrolysis temperature (300°C–450°C) (Palansooriya et al., 2019). Sufficient availability of O2-containing functional groups on the surface of biochar leads to anionic surface charge, which increases the CEC of soil after biochar application (Lu et al., 2022). Biochar application significantly increased the CEC by 3%–40% compared to control soil (Laird et al., 2010). Similarly, the CEC of extensively weathered soil was enhanced from 7 to 11 c mol kg−1 after applying biochar from the tamarind plant (Jien and Wang, 2013; Wang et al., 2022). Fang et al. (2016) reported that the CEC of corn straw biochar produced at 450°C was 26.36 c mol kg−1 and decreased to 10.28 c mol kg−1 at 700°C. Munera-Echeverri et al. (2018) revealed that the CEC was low until the temperature surpassed 420°C due to the nutrient content in the feedstock changed with temperature. Abhishek et al. (2022) presented that the high CEC facilitated the heavy metals removal from the polluted soil.
Soil organic matter (SOM) is a crucial factor affecting the soil health (Battaglia et al., 2021). The biochar amount and stability used as a soil improvement play a key role in enhancing the SOM (Alkharabsheh et al., 2021). Adding biochars derived from silver grass, umbrella tree, rice straw, and crop residues caused an increase of SOM content by 42%–72% in sandy soils and 32%–48% in loam soils (El-Naggar et al., 2018). In another investigation (2 years) Adekiya et al. (2020) found that application of hardwood biochar at the rate of 30 Mg t ha−1 increased the SOM content by 77, 18, and 9% compared to control (un-amended soil), 10 and 20 Mg t ha−1 biochar addition. Application of straw biochar and rapeseed stalk biochar also improved the SOM content in a red soil (Yang and Lu, 2020).
Amending soils with biochar also improves the soil organic carbon (SOC) content (Alkharabsheh et al., 2021). However, such increases in SOC are largely dependent on type of feedstock, pyrolysis temperature and soil types (Al-Wabel et al., 2017). Generally, biochars prepared at low pyrolysis temperatures contain a higher labile C compared to biochars fabricated at high pyrolysis temperature (Lévesque et al., 2018). Yin et al. (2014) in an incubation study observed a higher accumulation of SOC with rice-straw biochar prepared at 250°C than biochar generated at 350°C. This could happen due to the contribution of partially pyrolyzed portion of the biomass in biochar (produced at low temperature) to SOC. Furthermore, high temperature treated biochars carry large content of fixed carbon (aromatic C-C bonds) making biochar more stable, whereas low temperature biochars contain more labile substrates (C-H bonds) (Cardinael et al., 2017). Biochar enhances the SOC either by decomposition via soil microbes or through preservation of existing natural SOC (Al-webal et al., 2017). Overall, the effect of biochar addition on soil chemical properties is solely depend upon the type of feedstock, pyrolysis temperature, soil types and biochar application rates. Therefore, to get maximum benefit from biochar amendment, special attention should be paid to the biochar production conditions.
2.3 Effects on soil biological attributes
Biochar applications not only amend the soil physiochemical attributes but also alter soil biological attributes (Table 3) (Manirakiza et al., 2021). Biochar application affects the activity and community structure of soil microbes due to its large SSA, pore space, functional groups, surface volatile organic compounds, minerals and porosity (Gao and Deluca, 2016; Zhu et al., 2017). These alterations could modify the soil structure, reduce nutrient leaching, increase the nutrient cycles, form labile carbon compounds for microbial growth, increase aggregation, enhance nutrient immobilization and retention, and accelerate the plant growth mechanism (Murtaza et al., 2021a; Xu et al., 2021). Biochar particles and pores provide a habitat for the intrusion of filamentous microbes and fungi (Yin D. et al., 2021). Biochars, rich in sugars and yeasts, promote the growth of bacteria (Gram-negative) and fungi (Kocsis et al., 2022). Biochar alkalinity may stimulate the growth of Gram-negative and Gram-positive bacteria (Zimmermann et al., 2012; Osman et al., 2022). Sun et al. (2012) observed the bacterial populations were more dynamic and active in biochar-treated soil than fungal populations under field conditions; this may be attributed to the biochar’s higher surface area and biochar carbon (Liu et al., 2022). Moreover, pyrolysis temperature, biomass type, and carbon content strongly influence dehydrogenase activity, microbial habitats, N immobilization and mineralization (Ameloot et al., 2015).
The high SSA and porous nature of biochar serve as favourable habitats for diverse soil microbes (Ye et al., 2017). The porosity and SSA of biochar largely depend on feedstock type and pyrolysis temperature, high temperatures result in higher porosity and SSA (Palansooriya et al., 2019). The microbes become attached to the biochar micropores by electrostatic forces, hydrophobic attraction, or the precipitate formation, thus resist leaching, and increase their abundance (Abhishek et al., 2022) Moreover, soluble substances such as water, alcohol, sugars, acids and ketone molecules present in micropores and mesopores of biochar alter the microbial composition and abundance in soils (Adnan et al., 2020). An increment in the microbial activity and population of filamentous fungi, Bacillus species and Pseudomonas species have been observed with biochar addition in pepper cultivated soils. An application of maize stalk biochar at the rate of 50, 100, and 200 Mg ha−1 resulted in a 6.6%–31.2% higher fungal abundance compared to un-amended soil (Yao et al., 2017). In another study, Karimi et al. (2020) reported 20%–124% increase in soil microbial biomass in a calcareous soil supplemented with corn residue biochar at 1% and 2% (w/w) compared to control. Domene et al. (2014) found that the microbial abundance could enhance from 366 µgCg−1 (control soil) to 730 µgCg−1 (biochar treated soil). Also, microbial abundance was increased by (5%–50%) with the increase in biochar application rate (from 0%–14%) for various incubation times. Jin et al. (2016) found that litter-derived biochar enhanced the activity of phosphomonoesterase and decreased the activity level of acid phosphomonoesterase in silt loam and clay loam soils, respectively. An elevation in N and P retention in soil was noted due to change in microbial community structure and increased microbial activity in response of biochar application (Palansooriya et al., 2020). Biochar also found to play a crucial role in biological N fixation in legume crops by regulating different mechanisms including, increasing nodule formation, immobilizing N, enhancing P supply and altering the soil pH (Mia et al., 2014; Semida et al., 2015; Partey et al., 2016; Semida et al., 2019). Most probably, changes in resources (C and nutrients), physico-chemical factors, water availability or access to habitat may accelerate the competition among soil microbial communities which causes an alteration in community structure and composition (Semida et al., 2019).
Despite having the positive role of soil microbes in the soil, various soil pathogens can negatively impact the crop growth in the form of diseases (Bass et al., 2019). Biochar showed substantial potential to rectify the problems created by the soil pathogens. Jaiswal et al. (2018) reported that biochar can deactivate and immobilize the enzymes involved in cell wall deterioration and detoxified the metabolites produced by Fusarium oxysporum f. sp. radicis lycopersici and, protected the crop plants against soil pathogens. In another investigation, Gao et al. (2019) reported that the soil of tomato plants infected with Ralstonia solanacearum bacteria was improved with wheat straw biochar and severity of bacterial wilt was reduced with increase in total C, N, C:N ratio, K, P, pH and electrical conductivity. Biochars can potentially inhibit pathogenicity in plants by improving resistance, enhancing nutrient content, and detoxifying and adsorbing harmful chemicals in the polluted soils (Schmidt et al., 2021; Tan et al., 2022).
Biochar’s effects on enzymatic activity in soils depend on the nature of the substrate-enzyme interfaces in the presence of biochar, which are linked with biochar surface area and porosity (Bailey et al., 2011). Biochar with higher porosity and surface area would most likely decrease the extracellular enzymatic activity, given that the functional groups on the biochar would tend to bind the enzymes and substrates and therefore interfere with substrate diffusion on the active sites of the enzyme (Osman et al., 2022). Biochar treatment has both positive and negative effects on soil enzymatic activity. These impacts depend on biochar application rate and soil type (Table 3). Soil enzymes indicate the soil quality because they are directly related to soil microbial activity and biogeochemical cycling of nutrients (Palansooriya et al., 2019). For instance, increase in dehydrogenase activity was observed in different soils amended with different types of biochars (Bhaduri et al., 2016; Irfan et al., 2019). Such increase in dehydrogenase activity could be attributed to the labile organic matter and a high content of volatile matter of biochars (Gasco et al., 2016b). An increase in the activity of extracellular enzymes (β-glucosidase, a-glucosidase, β-xylosidase, and β-d-cellobiosidase) involved in soil sulfur (S) and C cycling was noted in a fluvo-aquic soil amended with biochar (Wang et al., 2015). In another study, elevation in dehydrogenase enzyme activity was observed in a red soil treated with .5% (w/w) bamboo and oak-wood biochar (Demisie et al., 2014). In the same study, β-glucosidase activity was increased in soil supplemented with only bamboo biochar at .5% and 1%. However, increase in urease activity was noted with oak wood biochar (.5% and 2%) and bamboo biochar (.5%) (Demisie et al., 2014; Palansooriya et al., 2020). Several studies (Ameloot et al., 2014; Paz-Ferreiro and Fu, 2016; Singh, 2016) also revealed that biochar manifest differential effects on enzyme activities across different types of soils. A manure-derived biochar decreased the activity of acid-phosphomonoesterase in clay-loam soil whereas activity level of alkaline-phosphomonoesterase was increased in silt-loam soils (Jin et al., 2016). Recently, a global meta-analysis (Pokharel et al., 2020) on biochar application and soil enzyme activities demonstrated an increase in the activities of extracellular enzymes including, phosphatase (25%), urease (23%) and dehydrogenase (20%). On the other hand a decrease of −13%, −7%, and −6% in phenol oxidase, β-glucosidase, and acid phosphatase, respectively was also observed. It is obvious that soils blended with biochar showed augmented proliferation of soil microbes benefitting the soil in several ways. However, more studies are needed to explore the long term effects of biochars on soil microbes and unveil the ecological roles of biochars.
3 Effect of biochar addition on soil nutrients
How biochar addition affects soil nutrient status has been reviewed extensively in different types of soils under various environmental factors (Schmidt et al., 2021). Biochar affects nutrients cycling in soils through retention and sorption, increasing or decreasing their bioavailability by reducing or increasing leaching and emissions (Tisserant and Cherubini, 2019). The transformation of nutrients in biochar-treated soils varies depending on biochar types, carbonization conditions, and soil types (Al-Wabel et al., 2017). Lehmann et al. (2002) advocated two primary methods for stimulating the rates of nutrient retention and decreasing nutrient leachability; (a) biochar addition enhances the adsorption sites, which accelerate the nutrient retention rate, and (b) biochar may perform as a slow releasing nutrient material. The N availability is the most substantial and key nutrient for the plant growth and it is greatly exposed to denitrification, leaching and volatilization (Abhishek et al., 2022). Biochar regulates the soil N through its surface chemistry, by affecting soil pH and influencing the soil microbial communities (Mia et al., 2017; Padhye, 2017). Mandal et al. (2018) reported that the retention of N is influenced by biochar owing to enrichment of O2-enrich functional groups (hydroxyl, aromatic ring carbonyl and aliphatic ether). Many researchers (Cayuela et al., 2014; Mandal et al., 2018; Borchard et al., 2019) highlighted that the maize biochar addition can accelerate the N content in soil through enhancing the net N mineralization, which increases nitrification process, affects denitrification and reduces the NH3 volatilization, and enhances the NH3 and NH4+ adsorption in soil. Marks et al. (2016) reported that adding pinewood-biochar decreased the N mineralization in the form of nitrate ions to amounts equal to ammonium. Likewise, Yao et al. (2017) applied the biochar to loamy sand, where available N contents reduced or remained unaffected due to the adsorption of ammonium, resulting in reduced available N. Furthermore, Jones et al. (2012), investigated the long-term effects of biochar addition on soil N mineralization in mull soils and observed that biochar addition had no substantial impact on nitrate ions, ammonium, and the total N amounts in the soil.
Additionally, biochar produced under different pyrolysis conditions showed variable soil N immobilization and mineralization results. For example, 43% N was immobilized in the soils after biochar addition, which was produced at a higher temperature. In contrast, applying biochar obtained at a low temperature resulted in 7% N mineralization (Bruun et al., 2012). Furthermore, the application rate of biochar also substantially impacted the mineralization of N by decreasing the concentrations of nitrate ions and ammonium in the soil (Bruun et al., 2012). Applying biochar produced at 400°C and 600°C accelerated the uptake of ammonium in the soil, substantially decreasing the soil inorganic N (Bruun et al., 2012). Butnan et al. (2017) compared the biochar applicability produced at 350°C and 500°C. The gross mineralization, labile N fraction and recalcitrant fraction and got enthused after the biochar (350°C) improvement in the soil than biochar produced at 550°C. Additionally, with biochar application, the N-cycle hastened and hence enhanced the gross mineralization of N, nitrification and NH4+ consumption rate by 185%–221%, 10%–69% and 333%–508%, respectively. This was possibly occurred because biochar application increased the soil aeration/porosity, enhanced and stimulated the growth of heterotrophic/aerobic microbial community (Yi et al., 2020). Additionally, nitrates content in soil doubled the concentration of ammonium following the biochar addition, possibly due to the negatively charged surface of biochar and high mineralization of N (Shenbagavalli and Mahimairaja, 2012). Jones et al. (2012) reported that wood-derived biochar did not substantially impact N mineralization or nitrification when applied to agricultural soil. Transformation of inorganic N with higher rates resulting from biochar addition could be explicated through (1) increased soil organic mineralization and net N, (2) denitrification enhanced because of the stimulation of denitrifying bacterial communities, (3) nitrification increased accelerated via a large number of ammonia oxidizers (Jones et al., 2012).
The availability of phosphorous in the soil to plants is affected by the application of biochar, which is regulated by the CEC or anion exchange capacity that leads to phosphorous incorporation (Si et al., 2018). Abhishek et al. (2022) revealed that phosphate complexes form at high pH with Mg2+ and Ca2+ and at low pH with Al3+ and Fe3+. The biochar inhibits the development of phosphate precipitates, and hereafter, the phosphorous availability rises in the plants. Xu et al. (2016) carried out a field study on maize in Indonesia. They exhibited that biochar adding to soil increases the available phosphorous, which elevates the maize yield afterward. They found the biochar application increase the phosphorous for the plants even in less available soil phosphorous conditions. Li and Chan, 2022) reported that sharp decline of P content in clay soil after biochar addition; it was due to the chemical adsorption of P the surfaces clay-mineral and temporary immobilization of P via soil microbes. Kelly et al. (2015) noticed a significant enhancement in P content of clay soil after hardwood-derived biochar application with the rate of 5, 10, 15 g kg−1. An increase of 54% P extractability (∼20 mgkg−1) was achieved in the clay-soil amended with biochars, whereas this increase was 42% (11 mgkg−1) in the control. Wang et al. (2015) conducted a pot experiment to examine the effect of biochar addition (0, 5, 10, and 25 g kg−1 soil) on the soil K dynamics in two types of soil (alfisol and Entisol). Both soil K increased in response to biochar addition, with the impacts more prominent in the Alfisol.
Biochar can improve the availability of soil P by changing the soil microbial communities, as it can provide the suitable growth conditions in the form of porous habitat and C supply for soil microbes (Dai et al., 2021). Zhou et al. (2020) reported an increase in soil P availability and activity of related enzymes by improving the growth of P-solubilizing bacteria (flavobacterium, pseudomonas and thiobacillus) in forest soil with rice-husk biochar application. These alteration could be credited to increased WHC and soil pH (Liu et al., 2017). Biochar enhanced the plant available P in soils by 45% and microbial biomass P by 48% (Gao et al., 2019). The manure and crop residue derived biochars exhibited higher P content than other feedstocks (Gul and Whalen, 2016). Biochar P is less mobile than the P from agricultural residues and it may act as a slow-release P fertilizer. Biochar can be a P-recycling route from agricultural residues. The results of a meta-analysis revealed that biochar amendment significantly improved the P availability in soils for 5 years. Several other investigations (Gao et al., 2019; Glaser and Lehr, 2019; Dai et al., 2021; Schmidt et al., 2021) described that manure and crop residues derived biochars increase availability of P, biochars prepared at high pyrolysis temperature release less P and P availability is reduced in alkaline soils (pH > 7.5) due to the liming effect of biochar. The biochar addition also affects other essential macronutrients such as Ca, Mg, Na, P, and K. For instance, Sigua et al. (2015) reported that biochars of poultry litter and pinewood 50:50 blend considerably improved the soil amounts of Ca, Mg, Na, P and K by 307%, 687%, 2,315%, 669%, 830% respectively, compared to control. Meta-analyses have revealed that biochar addition commonly increases P availability, especially when applied to neutral or acidic soils, and for biochar produced from low C:N feedstocks and at low temperatures (Gao et al., 2019; Glaser and Lehr, 2019). However, biochars prepared from Ca-rich and K-poor feedstocks (e.g., sewage sludge) reduce the P availability because pyrolysis can convert plant-available organic P into inorganic P that is less available in the short term (Rose et al., 2019; Buss et al., 2020). Gunes et al. (2014) demonstrated that the availability of K, P, and N was elevated in alkaline soil with biochar application, with no substantial impact on the macronutrient availability to the plants. Understanding the effects of biochar amendment on soil chemical properties is essential to determine appropriate application regimes under given field conditions. Additionally, a comprehensive comparison of different feedstocks produced at different pyrolysis temperatures is needed to identify and optimize the feedstock effects on nutrient release dynamics and biogeochemical cycling of nutrients in soils treated with biochars.
It can be visualized from the above discussion that biochar application significantly influences soil BD, aeration, porosity, WHC, CEC, pH, nutrient balances, and other parameters of soil quality due to its physicochemical properties and intrinsic structure (Singh et al., 2021; Murtaza et al., 2022a). Higher carbon and mineral content in the biochar are beneficial for improvement of soil health, fertility, and crop growth and yields (Table 4). The application of biochar also increases the microbial biomass, WUE, and NUE when added as soil amendment (Yu et al., 2019). All these modifications induced by biochar in soil physiochemical and biological properties offer great benefits to the agri-systems (Bhat et al., 2022). For instance, biochar enhances the amount of water available to plants, which could help in reducing irrigation frequency and it has great significance in water limited semi-arid regions (Alkharabsheh et al., 2021). Biochar particles with high porosity and large SSA contributed to increased plant available water. Taskin et al. (2019) investigated the effects of poultry manure biochar on chickpea (Cicer arietinum L.), maize (Zea mays L.), soybean (Glycine max L.) and bean (Vigna radiata L.) crops and observed positive effects on growth and other growth related parameters, mainly due to improvement in soil water holding capacity induced by biochar. Tomato height, weight, number of flowers, and fruit yield were also improved after 7.5 t ha−1 rice husk application (Adebajo et al., 2022), and similar results were obtained in faba bean varieties with higher grain yield, fruit protein content, and plant height due to the increased of soil available K and N after biochar addition (Essa et al., 2021). Increased rape shoot biomass (from 2.31 to 4.23 g) and grain yield after rice biochar application was the result of an improvement of soil chemical conditions (soil pH and cation exchange capacity) and nutrient availability (total C and N), together with changes in the associated microbiota (Shahab et al., 2018; Farid et al., 2022). The application of up to 10% (v/v) of wheat straw biochar increased P uptake in barley plants in controlled conditions and in maize plants grown in rhizoboxes (application rate of 15 g kg−1), with an increased shoot biomass and N use efficiency due to a fine root proliferation and an increase in the amount of N and P in soil (Tartaglia et al., 2020). wheat straw biochar application (5–40 t ha−1) promoted the growth and yield of lentil by increasing the organic C content and improving other physicochemical characteristics of the soil, and it was also able to increase maize yield by 23.7% by promoting N uptake (Allohverdi et al., 2021). There are several reports that highlight no or negative effects of biochar application alone or in combination with organic and inorganic fertilizers in soil plant systems. For instance, Rivelli and Libutti, (2022). Applied biochar and other organic amendments (vermicompost from cattle manure and three composts, respectively, from olive pomace, cattle anaerobic digestate with wheat straw, and cattle anaerobic digestate with crop residues and wheat straw), to the soil at two rates (to provide 140 and 280 kg N ha−1, respectively), but biochar did not affect the growth or the qualitative traits of Swiss chard. In another study, Singh et al. (2020) explored the effect of different combinations of chemical fertilizers, rice-husk ash biochar and farm yard manure on agronomic and eco-physiological responses of wheat crop. Sole application of farmyard manure and chemical fertilizer showed better (5%–26% higher) crop eco-physiological responses, whereas sole biochar and biochar plus farmyard manure application manifested poor responses (2%–15% lower) compared to the control. These results revealed that combined application of rice husk ash biochar and farmyard manure limit the crop growth. These were the few examples indicating that how changes in soil physiochemical and biological properties caused by biochar affect crop growth and productivity. A detailed impact of such changes on different plant development phases under different environmental conditions is described in the following section.
4 Interpreting the biochar-soil- plant nexus
4.1 Promote seed germination and early seedling growth
The effects of biochar application on seed germination (Table 5) consisted of inhibition to activation. A high application rate of biochar can have destructive impacts, while a low application rate of biochar can be stimulatory. This section will explain the mechanisms affecting the germination and seedling growth described in the literature.
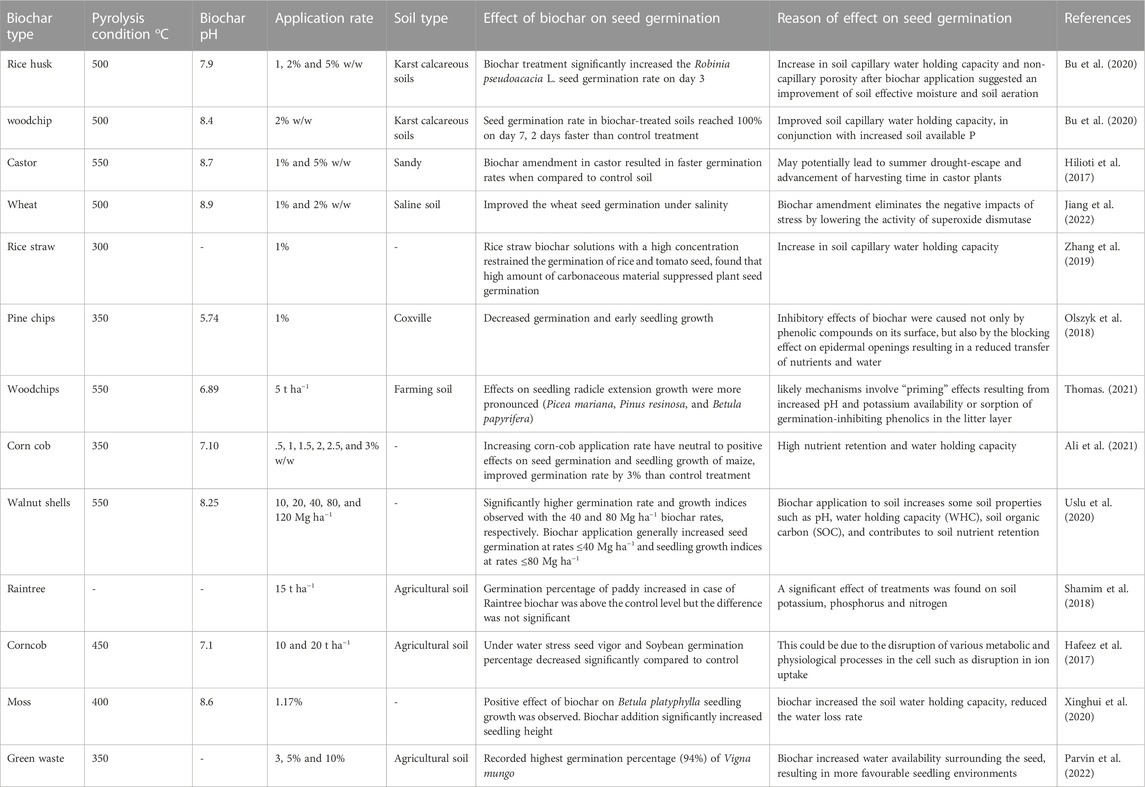
TABLE 5. Effect of biochar application on seed germination and seedling growth under different soils.
Seed germination starts with water absorption and ends when the radicle appears from the seed coat. The four main aspects regulating the effect of biochar treatment on seed germination are 1) release of phytotoxins, 2) salts released from biochar in soil solution, 3) alteration in porosity and WHC of soil, and 4) release of karrikins (germination-regulator hormone) (Joseph et al., 2021). Biochar type, pyrolysis condition, and dose have diverse effects on germination rate and speed. The specific sensitivity of different plant seeds to toxins, water availability, hormones, and salinity may cause variable results (Hasannuzzaman and Fujita, 2022). For instance, in a pot experiment, wood-derived biochar at 80 Mg t ha−1 suppressed the tomatoes’ germination. At the same time, sewage sludge, wheat husk, and paper residue-derived biochar added with the same dose had no impact on lettuce, cucumber, cress, tomatoes, and lentil seed germination (Gasco et al., 2016a). Other investigations that used a range of manure and woody biochars at a rate of 10 Mg t ha−1–40 Mg t ha−1 observed nil or positive impacts on germination (Van Zwieten et al., 2010; Khan et al., 2014; Mete et al., 2015; Gasco et al., 2016b; Das et al., 2020).
Uslu et al. (2020) reported the negative effects (inhibition of seed germination) in different fodder crops at high biochar application rate (120 Mg t ha−1) in a laboratory experiment. Aqueous extracts of various biochars have accelerated seed germination and seedling growth (Zheng et al., 2017). Seed growth and development of early seedlings can be affected due to biochar impacts on soil physical attributes. For example, by enhancing soil aeration and decreasing soil bulk density, biochar can provide oxygen for germination and to improve seedling development through seeding emergence (Obia et al., 2018). Biochar’s chemical impacts on water and soil solution can affect the seed and early seedling growth. For instance, by increasing pH, the alkaline biochars enhance the heavy metals and Al toxicity that can decrease root development in acidic-soils (Shetty et al., 2020). A high dose of biochar with high concentration of soluble salts could suppress seed growth and development by osmotic stress (Sun et al., 2017; Shetty et al., 2020). Kochanek et al. (2016) reported that the biochars comprising organic molecule karrikins, can promote seed growth and seedling development.
French and Iyer-Pascuzzi, (2018) demonstrated that the gibberellin pathway accelerates germination and seedling development through wood-derived biochar in tomato genotypes. Polyphenols and phenols released from biochar can efficiently break the seed dormancy, improve germination, and accelerate the seedling development mechanisms (Reynolds et al., 2018). Biochar comprises organic contaminants, PAHs, and heavy metals generated during the partial combustion process that can suppress germination and seedling development due to a high application rate (Gasco et al., 2016a; Gasco et al., 2016b; Das et al., 2020). Nevertheless, lower application rates, and low concentration of free radicals could be favourable as reactive oxygen can interact with various hormones of plants that stimulate the germination process (Gomes and Garacia, 2013).
It can be summarized from above discussion that mostly biochars and biochar based formulations are helpful in promoting seed germination and growth of seedlings when applied at moderate rates. However, application of biochars at relatively higher rates e.g., more than 40–50 Mg ha−1 could restrict seed germination and early growth due to the release of phytotoxic organic compounds and soluble salts. Furthermore, the mechanisms responsible for positive effects of biochar include water-soluble organic compounds that accelerate the seed germination and growth, chemical reactions that dismiss the inhibitory effects of phytotoxic compounds and heavy metals. The aforementioned effects largely depend upon the temperature, biochars prepared at low temperatures contain higher amounts of organic molecules and promote seed germination and seedling growth at low application rates. Therefore, the abovementioned factors should be considered prior to the application of biochar in the crop fields to get optimum benefits.
4.2 Biochar as a plant growth regulator
Biochar application either decreases or increases plant growth (Deenik et al., 2010). According to previous literature, the changes in plant growth (increase or decrease) depend on soil, biochar type, and biochar preparation temperatures (Figure 2). For example, Kwapinski et al. (2010) reported inhibition of corn growth through soil treatment with biochar derived from silver grass prepared at 400 °C. However, growth was promoted by biochar obtained at 600°C. Moreover, soil treatment with biochar at an application rate of 68 t ha−1 significantly enhanced the growth of cowpea, wheat, and rice (Vaccari et al., 2011; Zheng et al., 2017). In comparison, applying 10% animal manure-derived biochar reduced the sunflower plant height, number of leaves, achenes, and stem diameter (Furtado et al., 2016). Corn-derived biochar pyrolyzed at 400°C added at 20 t ha−1 did not considerably increase the growth of Glycine maxon loam soil (Hafeez et al., 2022). Changes in soil properties have often resulted with biochar addition, leading to increased plant growth (Solaiman et al., 2010). Solaiman et al. (2012) reported that the application rates and biochar type considerably impacted the growth of clover, mug beans, and wheat in the laboratory experiment. In a glasshouse experiment, the growth of radish, soybean, and wheat was enhanced with papermill derived biochar at the application rate of 10 t ha−1 (Van Zwieten et al., 2010). Furthermore, biochar addition to hostile sandy-soil increased the growth of corn by increasing photosynthesis rate, plant-soil water relations, decreased bulk density, and enhanced moisture retention (Haider et al., 2015). Thus, biochar addition may control the poor germination and plant growth induced by poor soil attributes (Furtado et al., 2016).
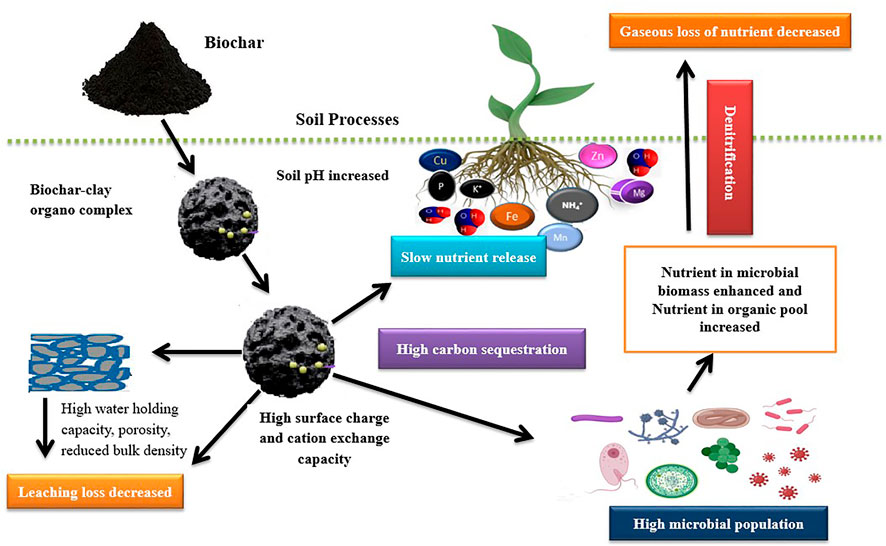
FIGURE 2. Biochar induced modifications in soil properties and its impact on plant growth and development; Copper (Cu), Iron (Fe), Magnesium (Mg), Manganese (Mn), Phosphorus (P), Potassium (K), Ammonium (NH4), Zinc (Zn).
Artiola et al. (2012) reported that pine-derived biochar applied at 2% in a pot study with a sandy-loam and alkaline soil had poorly affected lettuce growth in 2% biochar-treated soil. Moreover, rice plant growth, dry mass weight, and tiller number significantly increased in various varieties grown in treated soil with rice straw biochar at the rate of 15 kg ha−1. While the wheat growth was positively affected by 5% biochar application (Akhtar S. S. et al., 2015). Plant dry and fresh weights of pepper and tomato except romaine were increased by application of poultry manure-based biochar-to loam soil at the rate of 400 kg ha−1 (Vaccari et al., 2015). Burke et al. (2012) reported that the growth of cotton was promoted by hardwood-derived biochar when applied at the rate of 5–10 t ha−1. The growth and yield of Chenopodium quinoa and lettuce were enhanced by 300% in a hostile loam-sandy soil treated with 2% biochar (Trupiano et al., 2017).
Luigi et al. (2022) showed that biochar seems to promote the development of the tomato seedlings, especially at concentrations ranging from 1% to 20% (w/w with peat) without showing any antimicrobial effects on the beneficial soil bacteria at the tomato rhizosphere level and even improving their growth, because the application of biochar enhanced the soil pH as well as the retention of both the soil water and nutrients (Yang et al., 2022). Biochar ameliorated substrate characteristics (available N increase of 17% and total C increase 13%), resulting in a promotion effect on plant root, shoot, and leaf morphology, the biochar-treated plants had a greater number of leaves (38 and 68 at the vegetative and fruit stages, respectively) than the untreated plants (32 and 49, respectively). The biochar also increased leaf area with a rise of 26% and 36% compared with the values measured in the untreated plants. Moreover, the amendment increased twofold root length, root surface area, and root, stem, and leaf biomasses in comparison with untreated plants (Simiele et al., 2022), could have a promoting effect on plant growth as an indirect consequence of its positive effect on growth medium parameters such as water holding capacity and pH enhancement, increased nutrient availability (Malik et al., 2022). Xi et al. (2020) reported that the 2% (w/w) rice biochar application increased soil available N and K, resulting in taller lettuce plants, with longer roots, stronger leaves and stems, as well as greater leaf area. The growth improvement may be related to the impact of biochar on the physicochemical characteristics of the soils. Similarly, Huang et al. (2019) proposed that rice straw biochar contributed to the increase of total soil N content, making it more available to Phragmites communis and promoting its growth. But rice biochar can also stimulate C and N cycling by changing the microbial community. For example, increased rape shoot biomass (from 2.31 to 4.23 g) after rice biochar application was the result of an improvement of soil chemical conditions (soil pH and cation exchange capacity) and nutrient availability (total C and N), together with changes in the associated microbiota (Gomez et al., 2022).
Biochar-extracted liquor [1%–5% (w/w) in water] also promoted plant height and root growth in rice seedlings. The mechanism of action proposed was based on the overexpression of the ABP1 gene and the accumulation of its protein product. Accordingly, molecular modeling showed a molecule on the biochar surface that was able to interact with the ABP1 protein (Gelova et al., 2021). Liu Z. et al. (2021) found that application of 1% rice straw biochar, enhanced the N use efficiency of rice plants and resulted in increased shoot and root biomass (26%–29%), which were attributed to the enhancement of soil microbial biomass after biochar treatment. The application of wheat straw biochar (5–40 t ha−1) promoted the growth of Lens culinaris L. by increasing the organic C content and improving other physicochemical properties of the soil (Khorram et al., 2018). Taken together, biochar application positively augmented the growth and development of plants by increasing the availability of nutrients, water, SOC and improving the soil biological activities.
4.3 Impacts on plant physiological aspects
Various physiological aspects do or do not respond to biochar addition (Table 6) due to factors such as biochar and soil type (Asai et al., 2009; Singh et al., 2020). For example, soil treatment with biochar decreased the content of leaf chlorophyll in rice plants grown in poor-quality soil (Asai et al., 2009). Younis et al. (2015) conducted a pot experiment. They observed that transpiration (42%) and photosynthetic rates (45%), protein content (20%), anthocyanin (60%), lycopene (30%), carotenoids (29%), chlorophyll (40%), and stomatal CO2 level (22%) were improved. The concentration of amino acids and sugars were decreased with the increasing application rate of cotton biochar from 3%–5%. Increased P uptake, availability, and corn growth after biochar application were also observed (Mau and Utami, 2014). Compared with the control, an increase in chlorophyll contents, rate of photosynthesis, and stomatal conductance of the jute plant were found after biochar was mixed into the soil at the rate of 3 kg m−2 (Seehausen et al., 2017).
Haider et al. (2015) described that adding biochar in hostile sandy soils increased plant growth via increasing photosynthesis rate and plant-soil water relation under drought and well-watered conditions. In corn and wheat grown on loamy soil, biochar positively influenced physiological parameters. Where biochar application at the rate of 5% positively impacted xylem Na+ and K+, stomatal conductance, and photosynthesis rate more than the control. However, biochar application did not affect the photochemical ability of the photosystem-II (Akhtar S. S. et al., 2015; Seehausen et al., 2017). Various physiological parameters such as leaf nutrient level, leaf gas exchange, water status, and nutrient recovery of apple plants were positively affected via biochar addition (Eyles et al., 2015). Stomatal conductance, photosynthetic ability, vapor pressure and transpiration rate, and N and P leaf concentration significantly increased compared to control under biochar treatment (Eyles et al., 2015; Hafeez et al., 2017).
A significant rise in P, Mg, K, and N contents in tomato plants was found after biochar addition at the rate of 14 t ha−1 (Vaccari et al., 2015). Moreover, water use efficacy, transpiration and assimilation rate, and leaf water potential were positively influenced in lettuce grown in biochar treated soil (Trupiano et al., 2017). Akhtar S. S. et al. (2015) observed that biochar increased soil sorbing Na+ content and enhanced xylem K+ content, and decreasing N uptake, thereby elevating the potato yield (Akhtar S. S. et al., 2015). The biochar application significantly enhanced biomass and photosynthetic pigments development in plants. The treatments also increased membrane stability index by 45.12% and enhanced water using efficiency by 218.22%, respectively. The increase in antioxidant activities was 76.03%, 29.02%, and 123.27% in superoxide dismutase, peroxidase, and catalase, respectively (Tanveer et al., 2022). It was due to the application of biochar decreases the Pb and As toxicity and enhanced the production of the photosynthetic pigment in Salix viminalis L. They enhanced the production of chlorophyll, biomass and, gas exchange attributes in plants (Visconti et al., 2020) and antioxidants was increased by biochar because of the biochar reduces oxidative stress by the synthesis of ascorbate peroxidase, glutathione reductase, superoxide dismutase, and catalase (Kaya et al., 2020). EL Naggar et al. (2021) used of rice straw biochar (15 t ha−1) and found the enhancement in photosynthetic pigments.
These results were associated with the maintenance of the integrity of cell membranes and the reduction of the oxidative damage of leaf tissues by enhancing catalase (CAT), peroxidase (POX), superoxide dismutase (SOD), and glutathione reductase (GR) activities (Gomez et al., 2022). Biochar significantly increased net photosynthetic rate, transpiration rate, stomatal conductance, and water use efficiency during the plant growth period, relative to control and shown that biochar has great potential in improving chlorophyll fluorescence (Wang S. J. et al., 2021). That’s probably because biochar has the effect of increasing the chlorophyll content of leaves (Feng et al., 2021), which can ensure the synthesis of various enzymes and electron transporters in the process of carbon assimilation, thereby improving the function of leaf photosynthesis (Hou et al., 2021).
4.4 Effects on crop yield/productivity
Biochar application effects on crop productivity are variable due to feedstock composition, pyrolysis conditions, soil properties, and crop and experimental conditions. Various studies show that biochar addition has beneficial impacts on the productivity of different crops (Table 7). For instance, the yield of corn was enhanced by 40% after the addition of salwood-derived biochar (Yamato et al., 2006), 114% by corncob and wood biochar (Cornelissen et al., 2013), and 98% by the treatment of biochar-derived from manure (Uzoma et al., 2011). Compared to controls, the lantana and pine needles-derived biochars enhanced the grain yield of Triticum aestivum by 6%–24%. This is attributed to more efficient enzymatic activities and P and N uptake via grains (Bhattacharjya et al., 2015). Biochar addition at the rate of 40 t ha−1 in sandy loam soil increased rapeseed yield by 36% and potato yield by 53% (Liu S. et al., 2020).
Biochar application also enhances the crop productivity grown in alkaline soil, depending on the pH of applied biochar (Zhang H. et al., 2015). For instance, biochar application to alkaline soil (8.38 pH) at the rate of 20 t ha−1 and 40 t ha−1 enhanced the yield of maize by 18% (Vaccari et al., 2015). Major et al. (2010) presented that the maize yield was significantly increased after wood-derived biochar application (at the rate of 20 t ha−1) to alkaline soil (9.2 pH). The positive impacts of biochar application on alkaline soil depend on the type of biochar. Biochars produced at slow pyrolysis have low pH due to a large amount of aliphatic and volatile compounds (Spokas and Reicosky, 2009). Also, biochar addition has a negative or no impact on crop productivity in alkaline soils. For example, Hansen et al. (2016) conducted a pot study for biochar application at the rate of 1% in sandy loam soil (pH 9.8). Biochar addition exhibited no impact on the growth and yield of the barley crop. In a field experiment, adding wood biochar prepared at fast pyrolysis showed no effects on the corn yield in alkaline nature soil under water stress conditions (Foster et al., 2016). Marks et al. (2014) described that the poplar and pine wood-derived biochar by fast pyrolysis and gasification significantly inhibited the yield of ryegrass and lettuce at the 19 t ha−1 addition rate in calcareous soil (pH 8.9). Generally, biochar effects on crop yield are more prominent in acidic soils, well-weathered and low fertile soils dominated by sesquioxides and kaolinite (Sohi et al., 2010; Xu et al., 2013).
In acidic soils, the positive effects of biochar addition on crop productivity are attributed to increasing soil CEC because of biochar’s large surface area and porous structure. It amends the physical attributes of soil by increasing the soil WHC and decreasing the soil bulk density. Additionally, it enhances nutrient use efficiency and supply of essential nutrients, controls nutrient loss, accelerates microbial activity and their functions, stabilizes phototoxic elements in soil, and reduces the impact of biochar, such as increasing pH (Singh H. et al., 2022). Biochar addition increased the nodulation, biological nitrogen fixation, and yield of various legume species, including soybean, alfalfa, and red clover (Lai et al., 2022). Mete et al. (2015) reported an increase in biological N fixation of bean with the biochar application at the rate of 78 and 100 t ha−1 Mia et al. (2014) observed that biochar addition increased the total biomass, nodule number, and biological N fixation. Possible processes driving the impacts of biochar addition on biological N fixation in legumes such as 1) higher pH of soil improve the Mo availability, an essential nutrient needed in biological N fixation mechanism, 2) Higher concentration of available N immobilization which is associated to biological N fixation enhancement, 3) Strong impact of biochar on nodule production via efficient use of Nod and flavonoids feature, 4) Biochar addition accelerates the concentration of available P to phosphorus-deficient soils (Chen K. et al., 2022; Chen X. et al., 2022).
Various studies have reported that biochar addition had negative or no effects on the productivity of crops. For example, biochar application at the rate of 15 g kg−1 to silty and sandy soils did not enhance the corn yield (Borchard et al., 2014). Nelissen et al. (2015) found no effect of biochar application on the barley crop yield in sandy soil. Lai et al. (2013) observed that the Walnut hull-derived biochar produced at fast pyrolysis did not affect the yield of lettuce, Swiss chard rice, and bell pepper, despite the available K and pH of the soil being considerably higher. Kloss et al. (2014) conducted a greenhouse experiment and observed a decrease in mustard and barely yield after adding the vineyard pruning, wheat straw, and woodchips-derived biochars in chernozem, cambisol, and planosol soils but the yield of red clover remained unaffected. Furthermore, maize-derived biochar application at various rates to fertile soils did not impact the corn yield under field and pot experiments (Guerena et al., 2013).
Simiele et al. (2022) reported that the biochar-treated plants showed a higher number of flowers and fruits, although the mean fruit biomass and morphology remained unchanged. Additionally, higher values of Trans-and cis-lycopene, total soluble solids, and titratable acidity were found in the biochar-treated plants when compared with the untreated ones (Simiele et al., 2022). The promotion of fruit quantity and quality could be attributable to the high total P content in the biochar-treated substrate and the high total N concentration in roots of the biochar-treated plants observed at high level. Indeed, according to other reports, there might be a relationship between the phosphorous and nitrogen contents in both growing substrates and plant tissues and the promotion of fruit production by improving the vegetative and reproductive properties of tomato plants (Hameeda et al., 2019), also attributed to the increased values of lycopene, titratable acidity, and total soluble solids when biochar was used as a soil amendment (Guo et al., 2021). The continuous application of 20 t ha−1 of rice biochar to a rice field resulted in plant growth promotion and an increase of 14%–26% in soil N uptake, 7%–11% in internal N use efficiency, and a 6% in grain yield (Gomez et al., 2022). Bai et al. (2019) showed an enhanced yield (up to 35%) in different rice-wheat rotated soils, probably due to the release of plant macronutrients and micronutrients contained in the rice biochar. Nan et al. (2020) reported a clear improvement in soil bacterial cooperative relationships after treatment with rice biochar in a 4-year field trial. The complexity of the rhizosphere bacterial community was enhanced, most probably due to an increase in total soil C content, alongside with an increased total N content and soil available K and magnesium (Mg), which increased rice yield up to 14.5%. Yin D. et al. (2021) observed an increased rice yield (38%–41%) after the application of N-enriched rice straw and waste wood biochar, due to increased levels of soil C and N contents, as well as iron (Fe) availability. In addition, the application of biochar from wheat straw (20 t ha−1) in rice fields increased yield by 17%, as a consequence of a higher N and P supply, together with an improvement of more than 10% in the N use efficiency (Liu et al., 2021). Overall, improvement in soil physical, chemical and biological properties due to the biochar application caused an increase in crop yield or productivity. However, further studies should focus on optimization of biochar preparation conditions based on soil type, crop species and experimental settings. This could facilitate accuracy of biochar in terms of biochar type, preparation conditions and methods, application time, application rate, and recovery processes and it may help in promoting the application of biochar across diverse environmental conditions at large scale.
4.5 Effect of biochar addition on heavy metals uptake by plants
In plants, absorption of metals occur through the root cortical cells via competitive absorption of essential elements and adopted by symplastic and apoplastic pathways (Tangahu et al., 2011; Haider et al., 2021). Generally, mass flow is responsible for the transport of contaminants to the surface of the roots (Tran and Popova, 2013). These metals may be absorbed via apical portion of the root or the entire surface depending on the nature of the metal. Furthermore, metal uptake also relies on root development and capacity (Begum et al., 2019; Haider et al., 2021). Many investigations have revealed that biochar application is greatly helpful in reducing the absorption of soil contaminants (trace metals) in plants (Palansooriya et al., 2020; Joseph et al., 2021; Murtaza et al., 2022b; Haider et al., 2022). Chen et al. (2018) observed the incorporation of biochar into soils, resulting in an average reduction in plant tissue concentrations of Zn, Cu, Pb, and Cd by 17%, 25%, 39%, and 38%, respectively. Various studies indicated a substantial reduction in heavy metals bioavailability after using biochar at higher rates, such as 10 Mg ha−1 (Wang L. et al., 2020). Biochar surface with oxygenated-functional groups can stimulate the immobilization of heavy metals through different mechanisms (physisorption, electron shuttling, reduction, anion attraction, cation attraction, precipitation, and ion exchange) (Xu et al., 2019). Liming effects of biochar increase the pH of acidic soil, enhancing negatively charged exchange sites on the clay particles and raising cationic metals (Joseph et al., 2021).
Lei et al. (2019) presented that the biochars derived from manure contain higher Ca content than plant-based biochars and, therefore, can immobilize the Cu2+ and Cd2+ via ion exchange. Stable residues generated in the biochars with higher P can immobilize the lead (Pb) by the β-Pb9(PO4)6 formation. In contrast, higher calcite and alkalinity in biochar promote the insoluble Pb3(CO3)2(OH) 2 formation (Li et al., 2016). Biochar surface particles containing C-coated minerals are mainly efficient in reducing heavy metals’ bioavailability (Kumar et al., 2020). Willow-derived biochar at high temperatures facilitate adsorption of heavy metals from sewage sludge via physisorption and chemisorption mechanisms (Bogusz et al., 2019). Khan et al. (2013) reported that various feedstocks that carry high heavy metal contents could decrease the bioavailability of the heavy metals in some soils. For instance, biochar derived from sewage sludge reduced the bioaccumulation of Pb, Ni, Cu, Co, Cr, and As but enhanced Zn and Cd in acidic soil. Biochar can improve the anionic metalloid mobility by reducing the positively charged sites, which reduces the arsenic binding sites with increased soil pH (Vithanage et al., 2017).
Nkoh et al. (2022) reported that the effect of biochar application to polluted soils is the reduction of pollutant uptake by plants, with some exceptions for Fe and Mn. The reductions were estimated at 22.8% (Mn), 33.0% (Ni), 18.3% (Zn), 3.03% (Pb), 41.5% (As), 56.0% (Cr), 25.8% (Cu), and 26.2% (Cd). The underlying mechanisms for this reduction in the bioavailability of heavy metals in soils are diverse with charged metals being fixed via ion exchange, physical entrapment on biochar’s surfaces and changes in soil chemistry (Li et al., 2022). Amending heavy metal polluted soils with biochar reduced the overall daily intake of heavy metals (12.5%), hazard quotient (30.0%), and cancer risk (30.6%). However, these effects can be quite diverse depending on biochar properties, soil properties and the chemistry of concerned heavy metals (Nkoh et al., 2022). For maize plants grown on Pb-polluted soil, biochar treatment reduced the bioavailability of Pb (II) by 71% and the exchangeable Pb(II) by 99%. Compared to the un-amended soil, biochar treatment significantly decreased the associated Pb (II) toxicity to the maize plant (Zhu et al., 2020). Lebrun et al. (2020) examined the growth of Salix viminalis in arsenic and Pb (II)-contaminated soils amended with biochar, iron grit, and compost. They found that biochar-treated soil provided a favourable growing environment for the plant by considerably reducing the toxicity and bioavailability of the contaminants. Also, the phytotoxicity of Cd (II) to rice plants was significantly decreased when biochar was added to polluted soil (Yue et al., 2019). Natasha et al. (2022) observed that biochar application to soils has the potential to decrease the uptake of Zn, Pb, Cu, Cd, Ni and As, by 22%, 28%,38%, 40%, 44% and 48%, respectively in plants. In this study, with more data points, they estimated a 26.2% (Cd), 25.8% (Cu), 56% (Cr), 41.5% (As), 3.03% (Pb), 18.3% (Zn), 33.0% (Ni), and 22.8% (Mn) reduction rate of heavy metals uptake by plants when grown on biochar amended soils.
Biochars containing large surface area, sufficient pore volume and abundant functional groups play crucial role in heavy metal sorption (Ahmad et al., 2018). However, electrostatic interaction and sorption precipitation between heavy metals and biochars are the main mechanisms of heavy metal sorption governed by biochar (Lian and Xing, 2017). Additionally, surface coprecipitation, metal ligand complexation and ion exchange also contribute to the metal sorption on biochars (Ding et al., 2016a). Furthermore, the sorption affinity and capacity of heavy metals largely depend upon surface functional groups rather than pore volume and surface area (Yu et al., 2019). Oxidation of bichar induces carboxlic functional groups on biochar surface which elevated the adsorption capacity of Al3+, as oxygen enriched functional groups acted as coordinated sites for the Al3+. Cd adsorption on biochar was mainly regulated by the ion exchange (Lian and Xing, 2017; Haider et al., 2022). The sorption of Pb3+ on biochar surface was attributed to: 1) interaction of heavy metal with surface functional groups; 2) exchanges of heavy metal with cations (Ca2+, Mg2+) of biochar (Yu et al., 2019). Generally, biochars prepared at middle and low temperatures exhibit the highest adsorption capacity for metal cations (Xiao et al., 2018).
Biochar application appeared as a promising approach for mitigation of heavy metal contamination in plants, which may lead to a higher agricultural productivity and protecting plant community. However, biochar remediation efficiency is largely dependent upon the biochar type, plant species, biogeochemical properties of soil, and specific trace metal. Therefore, future strategies need a comprehensive analysis on determining the optimal methods of biochar production, type of biochar, plant species, popularization, and improving emphasis on suitability, adsorption potential, and sustainability of biochar as an optimum remediation tool against heavy metals while safeguarding the food quality.
5 Role of biochar in resistance to biotic and abiotic stresses in plants
Recently, the beneficial effects of biochar (Figure 3) in reducing plant diseases, including mildew in crops, wheat rust, and other pathosystems and factors, have been examined by various authors (Frenkel et al., 2017; Tian et al., 2021; Wu et al., 2022). More recently, 13 photosystems have analyzed the biochar impacts on plant diseases, and Bonanomi et al. (2015) summarized and reviewed that data. They presented that 85% of these investigations showed positive effects of biochar addition in reducing the severity of plant diseases, around 3% exhibited that addition induced the disease, and about 12% had a neutral impact. During this study, they did not consider that plant resistance/susceptibility to diseases depended on the applied dose of biochar. Frenkel et al. (2017) reviewed the 15 pathogens (such as nematodes, oomycetes, and fungi) data and compared the impacts of different treatments of biochar with control on disease severity and reduction. Biochar application at a high rate did not affect the plant diseases in 60% of the pathogens and 70% of photosystems than control (Jaiswal et al., 2014). In tomato, the use of wheat straw biochar reduced the disease incidence of bacterial wilt caused by Ralstonia solanacearum by up to 75%. This was due to an increase in the diversity and activity of rhizosphere microorganisms, together with alterations of the rhizosphere organic acid and amino acid composition. In addition, this increased microbial rhizosphere activity led to an increased supply of N and P to the plants, resulting in an increased plant biomass and length (Tian et al., 2021). Application of rice hull or rice husk biochar has reported interesting results to enhance the biomass of tomato plants and reduce Meloidogyne incognita infection by triggering defense-related genes such as PR-1b and JERF3 (Arshad et al., 2021). Rice biochar has also been helpful in alleviating the effects of the replanting disease (mainly caused by the accumulation of soil-borne pathogens (Wu et al., 2022). In this respect, an application of 80 g k−1 of rice husk biochar resulted in higher root length, surface area, and volume of apple tree seedlings, reducing the negative effect of the apple replant disease, and actively suppressing Fusarium solani infection (Wang Y. et al., 2019; Wang Y. Y. et al., 2019). In a similar way, the combination of rice hull biochar and plant growth-promoting rhizobacteria led to increased leaf area and biomass of Radix pseudostellariae, stimulated soil beneficial organisms, and suppressed pathogens through the increased production of soil metabolites, thus alleviating the effects of the replanting disease (Wu et al., 2022). Under biotic stress, the use of maize biochar can also improve crop responses. In pepper, the application of biochar from maize stalk reduced the incidence of Phytophthora blight (caused by Phytophthora capsica) by up to 50%, due to an increase in the abundance and diversity of biocontrol fungi within the genus Aspergillus, Chaetomium and Trichoderma. In addition, this biochar also improved soil qualities related to plant growth and development by increasing soil organic matter and N, P, and K content (Wang G. et al., 2020). Moreover, many studies on biochar exhibited that a relatively low application rate of biochar controlled the disease’s severity, but higher application rates did not show positive impacts on eradicating plant diseases (Jaiswal et al., 2015). In recent decades, many studies have reported that biochar addition increases crop yield under normal circumstances and enhances productivity under adverse conditions, including heavy metals, drought, and salinity (Joseph et al., 2021). For example, biochar slightly elevated the permanent wilting point, retaining a greater amount of water at field capacity than water contained at the permanent wilting point (i.e., increased plant-available water) (Hafeez et al., 2017). Thus, enhancement in WHC of the biochar-treated soils can be applied as an agent for increasing plant-available water (Hafeez et al., 2017). In field and pot experiments conducted on sandy clay and sandy loam soils, biochars applied at the rates of 20 t ha−1 enhanced the wheat and soybean germination, seedling growth, and grain yield by reducing the water stress (Hafeez et al., 2017). Haider et al. (2015) presented that adding biochar in hostile sandy soils enhanced plant growth via increasing plant-soil water relations under drought and well-watered conditions. Tammeorg et al. (2014) found that biochar application improved the grain yield under water stress conditions. Adding biochars at high rates can control the adverse impacts of salt stress on the growth and development of plants (Akhtar S. S. et al., 2015). For example, applying 50 t ha−1 of biochar can mitigate the mortality rate induced by salt in Jute and extend the survival rate of P. vulgaris. Moreover, adding biochar at the rate of 5% increased crop yield in salt-induced soils, possibly by transforming the salt stress via Na+ adsorption and enhancing K+ content in the xylem, thereby improving potato yield (Akhtar S. S. et al., 2015). The dual application of maize stalk and rice husk biochar significantly enhanced the growth, physiology, productivity, grain quality, and osmotic stress tolerance of rice plants, as well as nutrient uptake and soil properties, probably due to the activation of the enzymatic antioxidant machinery, for example improved activity of antioxidant enzymes including POX, APX, and CAT (Hafeez et al., 2021). Under abiotic stress situations, wheat straw biochar promotes tolerance of different crops. In this respect, an increased nutrient supply to plants can improve their tolerance against abiotic stresses, e.g., in tomato plants, wheat biochar amendment increased vegetative growth, yield, and quality parameters under saline irrigation, due to the adsorption of Na + ions and the release of Mg+2, Ca+2, and K+ (Zhu et al., 2020). Similarly, the application of wheat straw biochar in soybean plants subjected to salinity and drought increased the N content in the soil, favouring plant growth (Zhang H. et al., 2020). Another mechanism through which wheat straw biochar can increase plant tolerance to drought is the improvement of soil hydrophysical properties (soil water content, bulk density, and water holding capacity) reported in tobacco plants (Liu et al., 2021). Under abiotic stresses, such as drought and salinity, the application of biochar from maize has reported significant increases in plant tolerance. In quinoa plants, maize cob biochar increased the plant antioxidant machinery; reducing the accumulation of reactive oxygen species (ROS) and increasing nutrient uptake under drought and salinity stress (Nehela et al., 2021). However, in licorice plants grown with maize biochar in growth chambers, the increase of plant tolerance under salt stress was a consequence of an increased soil microbial enzymatic activity and nutrient supply to the plant (Egamberdieva et al., 2021). Biochar has also been shown to enhance salinity tolerance, alleviate drought stress, and mitigate the toxicity induced to plants by inorganic and organic soil pollutants. Drought stress alleviation in biochar-amended soils occurs through enhanced water holding capacity thanks to large surface area-to-volume ratio of biochar (Chew et al., 2022). Similarly, decrease in osmotic stress thanks to improved soil water content in addition to reduced Na+ uptake due to Na+’s transient binding on sorption sites on biochar alleviate soil salinity stress for plants in biochar-amended soils (Chew et al., 2022). Kumar et al. (2022) reported a decrease in thermal diffusivity (.6%–21.5%) and thermal conductivity (.3%–32.2%) in sandy loams after biochar addition. Further, there was a decrease in bulk density (24.7%–34.6%) and thermal diffusivity (10.4%–50.8%) of soil, and an improvement in moisture content after biochar addition. These changes decrease soil thermal conductivity (24.7%–59.8%), which ultimately moderates soil temperatures and influences plant growth and biochemical processes in soil. Xiong et al. (2020) re-affirmed that thermal properties are directly correlated with soil moisture and inversely related with soil bulk density and the addition of biochar reduces soil’s thermal properties. Further, soil depth, moisture content, and biochar application rates affect soil temperature and volumetric heat capacity.
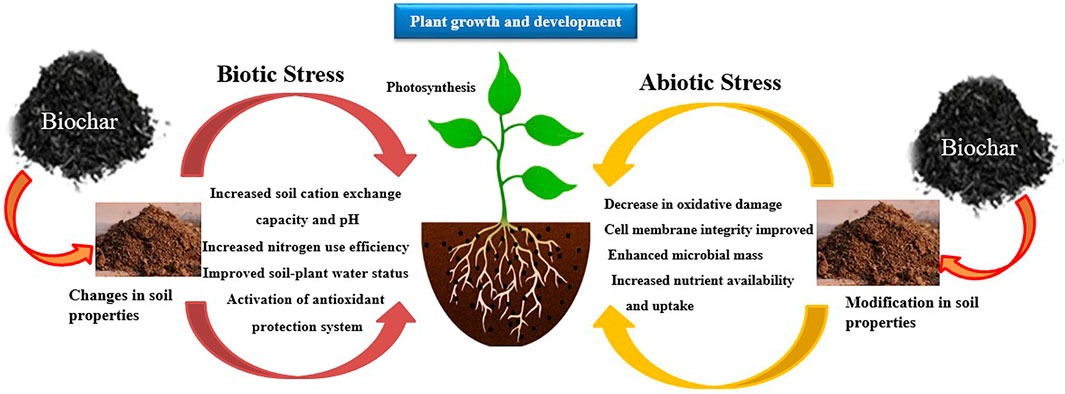
FIGURE 3. Improvement in plant resilience against biotic and abiotic stresses through biochar application.
Biochar modifies the abiotic and microbial processes in the rhizosphere and increases nutrient mineralization and enhances the nutrient availability for plant uptake. Organic matter turnover increases in the soil due to accelerated microbial activity which improves nutrient availability. Hence, biochar enhanced the plant resistance against diseases, reduced the availability of heavy metals and improved the plant resilience against environmental stressors. However, future studies should consider the preparation and formulation of novel treatment methods to prepare modified biochars with improved physicochemical properties enabling a better amelioration of adverse impacts of biotic and abiotic stresses in plants.
6 Application of biochar for soil carbon sequestration/greenhouse gases emission (GHGs)
Applying biochar to various types of soils not only increases the soil fertility but also plays a key role in carbon sequestration/GHGs reduction (Figure 4). Dissolved organic carbon or soil carbon as presented in Table 8, represents carbon sequestration by biochar addition, thus increasing the storage of C in the soil and reducing GHGs emissions (Tang et al., 2022). Carbon sequestration to artificial or natural removal of atmospheric CO2 and its storage in a stable solid form (Song et al., 2022). Naturally, atmospheric CO2 can be directly absorbed through plants by photosynthesis process. Part of the absorbed CO2 is released back into the atmosphere through respiration, and the rest is sequestered first as plant biomass and then as soil organic carbon during the decomposition process of the plant biomass (Zhou et al., 2022). Further decomposition of soil organic carbon discharges the C as CO2 into the atmosphere within a short duration. Thus, the entire mechanism is carbon neutral (Kalu et al., 2022). Biochar is stable and its great content of aromatic C that is harder to decompose than plant biomass is the basis of the C sequestration paybacks of biochar. The pyrolysis of plant biomass breaks the natural C cycle and realizes carbon sequestration through biochar storage in the soil (Cara et al., 2022). It is assessed that around six GtC/yr of carbon biomass is accessible for biochar creation worldwide if 10% of net primary production is utilized, from which 3 GtC/yr of biochar can be created (Pan et al., 2022).
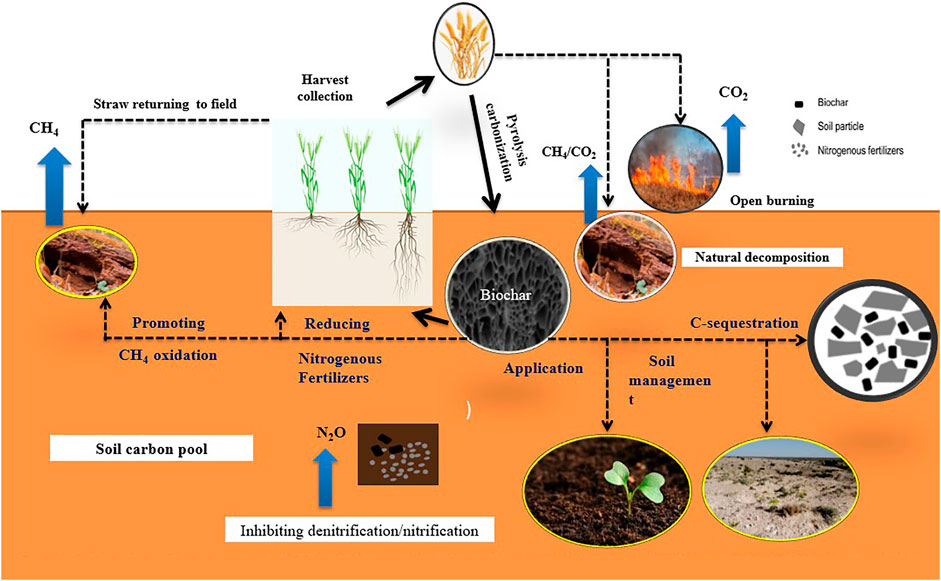
FIGURE 4. Mechanism of greenhouse gas (GHG) reduction and soil carbon (C) sequestration through biochar application; Carbon dioxide (CO2), Methane (CH4), Nitrous oxide (N2O).
Carbonizing agricultural biomass residue (mainly livestock manure and crop straw) and storing the resulting biochar in soils through agricultural soil management have the benefit of solid waste recycling and show pronounced potential for sequestration of C (Nair and Mukherjee, 2022). Rendering to a calculation technique based on a life cycle assessment, 610 Mt/yr of livestock manure and 585 Mt/yr of crop straw are created in China, and converting them into soil biochar can sequester 172 and 264 Mt CO2 e/yr, respectively (Deolikar and Patil, 2022). Yang C. et al. (2021) assessed the C sequestration potential of several crop waste-derived biochars via life cycle assessment at the state level and noticed that the annual C sequestration potential in China could reach around 500 MtCO2e/yr. These findings proved the high C sequestration capability of crop wastes-derived biochars.
Layek et al. (2022) applied biochar derived from corn residue to a maize field under drip irrigation with mulching condition, and study findings exhibited that the sequestration of C enhanced by 16% in the upper 15-cm soil and CH4 emission reduced by 132% after 30 t/ha of biochar addition. Moreover, the biochar treatment enhanced the yield of corn by 7.4% over 2 years. This example suggests that proper application of biochar could contribute to agricultural soil management and climate change mitigation simultaneously. An assessment of the biochar life cycle manifests that reduction in GHGs emissions is mainly associated with changes in feedstock production, biochar production, and storage and stabilization of C in biochar, reduction in emissions of N2O from the agriculture sector (Osman et al., 2022). Generally, biochar treatment decreased the total GHGs (Castaldi et al., 2011). Nevertheless, biochar application impact on various GHGs such as N2O, CH4, and CO2 varies significantly. Biochar treatments usually decrease CO2 production by enhancing carbon stabilization (Castaldi et al., 2011). Non-etheless, no significant impact was observed in the case of soil CO2 respiration during the field study (Hamamoto et al., 2022). The differences created from techniques applied for CO2 calculation, CO2 that originated from the biochar was subtracted from the biochar-soil combination to ascertain the biochar effect on the soil respiration (Zhao et al., 2022). Biochar’s impact on methane emission varied significantly. Feng et al. (2012) reported that the paddy methane emissions substantially reduced after biochar treatments, possibly not due to the suppression of methanogenic growth. It might have resulted from the variable proportion of methanogenic to methanotrophic richness. Methane fluxes did not differ considerably in response to different treatments (Castaldi et al., 2011).
In some cases, total methane emission was found to be increased with biochar addition. It could have happened due to the inhibitory effect of biochar chemicals on the methanotroph’s activity (Zhang A. et al., 2010). In contrast, a net reduction up to 50% in CH4 emission was observed in saturated peat soils after biochar application, which was attributed to the increased activity of methanotrophs in the oxic rhizosphere (Cong et al., 2018; Nguyen et al., 2020). Under well-drained conditions, the CH4 consumption was decreased by ash-rich biochars, probably due to an increased electrical conductivity in the soil solution thus hindring the methanotroph activity (Pascual et al., 2020). Moreover, sorbed hydrocarbon elements of biochar could decompose and emerge as a competitive source of substrates, stimulating CH4 emissions by reducing CH4 oxidation activity (Jandl et al., 2013). Biochar applications have been reported to decrease N2O emissions in laboratory experiments (Case et al., 2012; Lehmann et al., 2021).
In biochar amended field, fluxes of soil N2O ranged from 26%–79% as compared to nitrous oxide fluxes observed in control (Castaldi et al., 2011). The fixed N increased from 50% without biochar addition to 70% with biochar addition at the rate of 90 g kg−1, and N2O fluxes reduced (Rondon et al., 2007). On the contrary, high N-enriched biochars have promoted the emission of N2O (Zhang H. et al., 2010). These results exhibited the efficiency of biochar addition to influence the proportions of N-cycling in the soil via improving ammonia adsorption and nitrification rates and enhancing ammonia storage through increasing the soil CEC (Dawar et al., 2021), hence changing the efficacy of N input into soil system (Osman et al., 2022). Biochar manufactured from woody feedstocks and agricultural waste substantially impacts the NH3, N2O, and CH4 emission mitigation. These emissions can be decreased significantly by applying biochar at the rate of 10% w/w. Biochar pyrolyzed at higher temperatures strongly impacts the mitigation of N2O and CH4 emissions. However, biochar produced at low temperatures is more efficient in decreasing NH3 emissions (Yin X. et al., 2021).
Biochar application provided combined benefits of carbon sequestration and GHG emission reduction which are the key to achieving carbon neutrality goals. However, efficacy of biochars in enhancing C sequestration and reducing GHGs could be improved by preparing specific biochars based on the scientific results demonstrated by different studies. Therefore, attention should be paid to the development of special biochars to get maximum benefit from this commodity to make our environment more sustainable.
7 Biochar application for agricultural sustainability
Biochar is a potentially strong candidate for improving agricultural sustainability by increasing soil health, crop yields and decreasing the use of chemical fertilizers. Various applications of biochar utilization and future research directions are described below.
1. Biochar may comprise toxic elements, including heavy metals, dioxin, and PAHs. It cannot be eliminated once it is added to soils. The toxicity of biochar must be measured before biochar application as a soil conditioner to decrease long-term hazards to crops and soil.
2. Biochar incorporation into alkaline soils is not as productive as in addition to acidic nature soils regarding crop yield. Usually, slow pyrolyzed biochars have low pH values and, therefore can be efficient for amending high pH soil (alkaline soil). Biochars can be added in combination with humic acid and acidic chemical fertilizers. Future investigation should focus on functional biochar addition to alkaline calcareous and sandy soils of arid areas; feedstock selection, preparation temperatures are vital when designing the functional biochars.
3. Biochar’s recommended addition rates to enhance the advantages under specific circumstances are not well-defined yet. Generally, utilizing large amounts of biochar is not conceivable for small-scale farmers. Therefore cost-benefit investigation regarding the use of biochar must be implemented across various cropping systems. Applying biochar in pots while growing nursery plants can be a cost-efficient method for small-scale farmers to follow biochar technology.
4. The impacts of biochar addition on beneficial microbes under field experiments remain largely unclear. However, the co-application of bacteria and biochar can stimulate plant growth and development and increase nutrient use efficiency.
5. The laboratory scale research should be synchronised with field studies. Difference in weather, soil qualities and environmental conditions may render discrepancy between laboratory and field studies. Therefore, long-term and broad scale field investigations are needed to explore the impact of biochar on different soil properties.
6. The long term studies are needed on processes that affect the capture and release of heavy metals in the long term to plan an optimum scheduling of biochar re-application.
7. Studying the effects of biochar properties on microbial nutrient cycling and root membrane potential will facilitate the development of optimal formulations to increase nutrient uptake efficiency.
8. Biochar based carbon trading market could be establish to recognize the GHG reduction and carbon sequestration benefits. The farmers willing to use biochar should be facilitated with incentives.
9. In view of high costs of biochar, more research on biochar modifications (using pre-or post-treatments) are crucial to maximize the advantage of “low dose with high efficiency”.
8 Conclusion
Biochars are broadly diverse and can have several impacts on soil attributes, crops growth and production. The feedstock and the pyrolysis conditions largely influence the properties of biochar and its effects on agricultural ecosystems. Biochar formulations applied at an optimal rate can significantly increase yields under site-specific soil constraints, nutrient, and water-limited conditions. Low temperatures pyrolyzed biochars may improve the availability of nutrients and crop productivity in both types of soils (alkaline and acidic). In contrast, biochars derived at high temperatures may increase soil carbon sequestration for the long-term. The average yield increases of 10%–40% were observed with biochar addition. Biochar reduces the availability of heavy metals, enhances plant resistance potential to various diseases, and increases resilience to different environmental stressors (biotic, abiotic drought, and salt). Biochar accelerates microbial activity, which can enhance the mineralization of nutrients and promote the nutrient uptake mechanism by plants. Biochar selection, its application rates, and compatibility with cropping systems should be considered before biochar addition. Sequestering large amounts of carbon biochar reduces GHGs emissions. A clear understanding of variable effects of biochar on soil and plant systems could facilitate biochar preparation for specific applications through proper feedstock selection by adjusting process conditions and pre- or post-production treatment of biochar that govern the pH, nutrient availability, and adsorption capacity. Guidelines regarding the selection and production of biochar to meet specific soil and environmental constraints should be developed.
Author contributions
Original draft (GM and ZA); writing, review and editing (MU, BA, DN, AU, AK, SB, SME and RI); data collection (DN, MUH, AU and AK); resources and supervision (ZA, GM, IA, MUH and AT); Funding acquisition (SB, IA and SME).
Acknowledgments
The authors would like to thank the Deanship of Scientific Research at Umm Al-Qura University for supporting this work by Grant Code: 22UQU4350073DSR18. This work is also partially funded by the research center of the Future University in Egypt. We are highly thankful to the Kunming University of Science and Technology, Kunming, China for supporting this study.
Conflict of interest
The authors declare that the research was conducted in the absence of any commercial or financial relationships that could be construed as a potential conflict of interest.
Publisher’s note
All claims expressed in this article are solely those of the authors and do not necessarily represent those of their affiliated organizations, or those of the publisher, the editors and the reviewers. Any product that may be evaluated in this article, or claim that may be made by its manufacturer, is not guaranteed or endorsed by the publisher.
References
Abrol, V., Ben-Hur, M., Verheijen, F. G. A., Keizer, J. J., Martins, M. A. S., Tenaw, H., et al. (2016). Biochar effects on soil water infiltration and erosion under seal formation conditions: Rainfall simulation experiment. J. Soils Sed. 16, 2709–2719. doi:10.1007/s11368-016-1448-8
Abakari, G., Luo, G., Meng, H., Yang, Z., Owusu-Afriyie, G., Kombat, E. O., et al. (2020). The use of biochar in the production of tilapia (Oreochromis niloticus) in a biofloc technology system - bft. Aquac. Eng. 102123.
Abbas, T., Rizwan, M., Ali, S., Adrees, M., Mahmood, A., Zia-ur-Rehman, M., et al. (2018). Biochar application increased the growth and yield and reduced cadmium in drought stressed wheat grown in an aged contaminated soil. Ecotoxicol. Environ. Saf. 148, 825–833. doi:10.1016/j.ecoenv.2017.11.063
Abbasi, M. K., and Anwar, A. A. (2015). Ameliorating effects of biocharderived from poultry manure and white clover residues on soil nutrient status and plant growth promotion -greenhouse experiments. PLoS One 10, e0131592. doi:10.1371/journal.pone.0131592
Abhishek, K., Srivastava, A., Vimal, V., Gupta, A. K., Bhujbal, S. K., Biswas, J. K., et al. (2022). Biochar application for greenhouse gas mitigation, contaminants immobilization and soil fertility enhancement: A state-of-the-art review. Sci. Total Environ. 853, 158562. doi:10.1016/j.scitotenv.2022.158562
Abideen, Z., Koyro, H. W., Huchzermeyer, B., Ansari, R., Zulfiqar, F., and Gul, B. (2020). Ameliorating effects of biochar on photosynthetic efficiency and antioxidant defence of Phragmites karka under drought stress. Plant Biol. 22, 259–266. doi:10.1111/plb.13054
Adebajo, S. O., Oluwatobi, F., Akintokun, P. O., Ojo, A. E., Akintokun, A. K., and Gbodope, I. S. (2022). Impacts of rice-husk biochar on soil microbial biomass and agronomic performances of tomato (Solanum lycopersicum L.). Sci. Rep. 12, 1787. doi:10.1038/s41598-022-05757-z
Adekiya, A. O., Agbede, T. M., Olayanju, A., Ejue, W. S., Adekanye, T. A., Adenusi, T. T., et al. (2020). Effect of biochar on soil properties, soil loss, and cocoyam yield on a tropical sandy loam alfisol. Sci. World J. 9391630.
Adnan, M., Fahad, S., Zamin, M., Shah, S., Mian, I. A., Danish, S., et al. (2020). Coupling phosphate-solubilizing bacteria with phosphorus supplements improve maize phosphorus acquisition and growth under lime induced salinity stress. Plants 9, 900. doi:10.3390/plants9070900
Ahmad, Z., Gao, B., Mosa, A., Yu, H. W., Yin, X. Q., Bashir, A., et al. (2018). Removal of Cu(II), Cd(II) and Pb(II) ions from aqueous solutions by biochars derived from potassium-rich biomass. J. Clean. Prod. 180, 437–449. doi:10.1016/j.jclepro.2018.01.133
Akhtar, S. S., Andersen, M. N., and Liu, F. (2015b). Biochar mitigates salinity stress in potato. J. Agron. Crop Sci. 201, 368–378. doi:10.1111/jac.12132
Akhtar, S. S., Andersen, M. N., and Liu, F. (2015a). Residual effects of biochar on improving growth, physiology and yield of wheat under salt stress. Agric. Water Manag. 158, 61–68. doi:10.1016/j.agwat.2015.04.010
Al-Wabel, M. I., Hussain, Q., Usman, A. R. A., Ahmad, M., Abduljabbar, A., Sallam, A. S., et al. (2017). Impact of biochar properties on soil conditions and agricultural sustainability: A review. Land Degrad. Dev. 29, 2124–2161. doi:10.1002/ldr.2829
Al-Wabel, M. I., Usman, A. R. A., El-Naggar, A. H., Aly, A. A., Ibrahim, H. M., Elmaghraby, S., et al. (2015). Conocarpus biochar as a soil amendment for reducing heavy metal availability and uptake by maize plants. Saudi J. Biol. Sci. 22, 503–511. doi:10.1016/j.sjbs.2014.12.003
Ali, L., Xiukang, W., Naveed, M., Ashraf, S., Nadeem, S. M., Haider, F. U., et al. (2021). Impact of biochar application on germination behavior and early growth of maize seedlings: Insights from a growth room experiment. Appl. Sci. 11, 11666. doi:10.3390/app112411666
Ali, S., Rizwan, M., Qayyum, M. F., Ok, Y. S., Ibrahim, M., Riaz, M., et al. (2017). Biochar soil amendment on alleviation of drought and salt stress in plants: A critical review. Environ. Sci. Pollut. Res. 24, 12700–12712. doi:10.1007/s11356-017-8904-x
Alkharabsheh, H. M., Seleiman, M. F., Battaglia, M. L., Shami, A., Jalal, R. S., Alhammad, B. A., et al. (2021). Biochar and its broad impacts in soil quality and fertility, nutrient leaching and crop productivity: A review. Agron 11, 993. doi:10.3390/agronomy11050993
Allohverdi, T., Mohanty, A. K., Roy, P., and Misra, M. (2021). A review on current status of biochar uses in agriculture. Molecules 26, 5584. doi:10.3390/molecules26185584
Almaroai, Y. A., and Eissa, M. A. (2020). Effect of biochar on yield and quality of tomato grown on a metal-contaminated soil. Sci. Hortic. 265, 109210. doi:10.1016/j.scienta.2020.109210
Amalina, F., Abd Razak, A. S., Krishnan, S., Sulaiman, H., Zularisam, A. W., and Nasrullah, M. (2022). Advanced techniques in the production of biochar from lignocellulosic biomass and environmental applications. Clean. Mat. 100137.
Ameloot, N., Sleutel, S., Case, S. D. C., Alberti, G., McNamara, N. P., Zavalloni, C., et al. (2014). C mineralization and microbial activity in four biochar field experiments several years after incorporation. Soil Biol. biochem. 78, 195–203. doi:10.1016/j.soilbio.2014.08.004
Ameloot, N., Sleutel, S., Das, K. C., Kanagaratnam, J., and de Neve, S. (2015). Biochar amendment to soils with contrasting organic matter level: Effects on N mineralization and biological soil properties. GCB Bioenerg. 7, 135–144. doi:10.1111/gcbb.12119
Arif, M., Ali, K., Jan, M. T., Shah, Z., Jones, D. L., and Quilliam, R. S. (2016). Integration of biochar with animal manure and nitrogen for improving maize yields and soil properties in calcareous semi-arid agroecosystems. Field Crops Res. 195, 28–35. doi:10.1016/j.fcr.2016.05.011
Arshad, U., Azeem, F., Mustafa, G., Bakhsh, A., Toktay, H., McGiffen, M., et al. (2021). Combined application of biochar and biocontrol agents enhances plant growth and activates resistance against Meloidogyne incognita in tomato. Gesunde Pflanz. 73, 591–601. doi:10.1007/s10343-021-00580-4
Artiola, J. F., Rasmussen, C., and Freitas, R. (2012). Effects of a biochar-amended alkaline soil on the growth of romaine lettuce and bermudagrass. Soil Sci. 177, 561–570. doi:10.1097/ss.0b013e31826ba908
Asai, H., Samson, B. K., Stephan, H. M., Songyikhangsuthor, K., Homma, K., Kiyono, Y., et al. (2009). Biochar amendment techniques for upland rice production in Northern Laos. Field Crops Res. 111, 81–84. doi:10.1016/j.fcr.2008.10.008
Awasthi, G., Singh, T., Tiwari, Y., Awasthi, A., Tripathi, R. D., Shrivastava, S., et al. (2020). A review on nanotechnological interventions for plant growth and production. Mater. Today Proc. 31, 685–693. doi:10.1016/j.matpr.2020.07.255
Awasthi, M. K., Wang, M., Chen, H., Wang, Q., Zhao, J., Ren, X., et al. (2017). Heterogeneity of biochar amendment to improve the carbon and nitrogen sequestration through reduce the greenhouse gases emissions during sewage sludge composting. Bioresour. Technol. 224, 428–438. doi:10.1016/j.biortech.2016.11.014
Ayaz, M., Feizienė, D., Tilvikiene, V., Akhtar, K., Stulpinaitė, U., and Iqbal, R. (2021). Biochar role in the sustainability of agriculture and environment. Sustainability 13, 1330. doi:10.3390/su13031330
Bai, N., Zhang, H., Li, S., Zheng, X., Zhang, J., Zhang, H., et al. (2019). Long-term effects of straw and straw-derived biochar on soil aggregation and fungal community in a rice-wheat rotation system. Peer J. 6, e6171. doi:10.7717/peerj.6171
Baiamonte, G., Crescimanno, G., Parrino, F., and De Pasquale, C. (2019). Effect of biochar on the physical and structural properties of a sandy soil. Catena 175, 294–303. doi:10.1016/j.catena.2018.12.019
Baiamonte, G., De Pasquale, C., Marsala, V., Cimo, G., Alonzo, G., Crescimanno, G., et al. (2015). Structure alteration of a sandy-clay soil by biochar amendments. J. Soils Sed. 15, 816–824. doi:10.1007/s11368-014-0960-y
Baiga, R., and Rao, B. K. R. (2017). Effects of biochar, urea and their co-application on nitrogen mineralization in soil and growth of Chinese cabbage. Soil Use Manag. 33, 54–61. doi:10.1111/sum.12328
Bailey, V. L., Fansler, S. J., Smith, J. L., and Bolton, H. (2011). Reconciling apparent variability in effects of biochar amendment on soil enzyme activities by assay optimization. Soil Biol. biochem. 43, 296–301. doi:10.1016/j.soilbio.2010.10.014
Bass, D., Stentiford, G. D., Wang, H.-C., Koskella, B., and Tyler, C. R. (2019). The pathobiome in animal and plant diseases. Trends Ecol. Evol. 34, 996–1008. doi:10.1016/j.tree.2019.07.012
Battaglia, M. L., Thomason, W. E., Fike, J. H., Evanylo, G., von Cossel, M., Babur, E., et al. (2021). The broad impacts of corn stover and wheat straw removal for biofuel production on crop productivity, soil health and greenhouse gas emissions: A review. GCB Bioenerg. 13, 45–57. doi:10.1111/gcbb.12774
Begum, N., Hu, Z., Cai, Q., and Lou, L. (2019). Influence of PGPB Inoculation on HSP70 and HMA3 gene expression in Switch grass under cadmium stress. Plants 8, 504. doi:10.3390/plants8110504
Bhaduri, D., Saha, A., Desai, D., and Meena, H. (2016). Restoration of carbon and microbial activity in salt-induced soil by application of peanut shell biochar during short-term incubation study. Chemosphere 148, 86–98. doi:10.1016/j.chemosphere.2015.12.130
Bhat, A., Kuriqi, S., Dar, A., Bhat, M. U. D., Sammen, O., Islam, S. S. T., et al. (2022). Application of biochar for improving physical, chemical, and hydrological soil properties: A systematic review. Sustain 14, 11104. doi:10.3390/su141711104
Bhattachariya, S., Chandra, R., Pareek, N., and Raverkar, K. P. (2015). Biochar and crop residue application to soil: Effect on soil biochemical properties, nutrient availability and yield of rice (oryza sativaL.) and wheat (Triticum aestivum L.). Arch. Agron. Soil Sci., 1–14. doi:10.1080/03650340.2015.1118760
Blanco-Canqui, H. (2017). Biochar and soil physical properties. Soil Sci. Soc. Am. J. 81, 687–711. doi:10.2136/sssaj2017.01.0017
Bogusz, A., Oleszczuk, P., and Dobrowolski, R. (2019). Adsorption and desorption of heavy metals by the sewage sludge and biochar-amended soil. Environ. Geochem. Health 41 (4), 1663–1674. doi:10.1007/s10653-017-0036-1
Bolan, N., Hoang, S. A., Beiyuan, J., Gupta, S., Hou, D., Karakoti, A., et al. (2022). Multifunctional applications of biochar beyond carbon storage. Int. Mat. Rev. 67, 150–200. doi:10.1080/09506608.2021.1922047
Bonanomi, G., Ippolito, F., and Scala, F. (2015). A “black” future for plant pathology? Biochar as a new soil amendment for controlling plant diseases. J. Plant Pathol. 97, 223–234.
Borchard, N., Schirrmann, M., Cayuela, M. L., Kammann, C., Wrage-Monnig, N., Estavillo, J. M., et al. (2019). Biochar, soil and land-use interactions that reduce nitrate leaching and N2O emissions: A meta-analysis. Sci. Total Environ. 651, 2354–2364. doi:10.1016/j.scitotenv.2018.10.060
Borchard, N., Siemens, J., Ladd, B., Moller, A., and Amelung, W. (2014). Application of biochars to sandy and silty soil failed to increase maize yield under common agricultural practice. Soil Tillage Res 144, 184–194. doi:10.1016/j.still.2014.07.016
Bruun, E. W., Ambus, P., Egsgaard, H., and Hauggaard-Nielsen, H. (2012). Effects of slow and fast pyrolysis biochar on soil C and N turnover dynamics. Soil Biol. biochem. 46, 73–79. doi:10.1016/j.soilbio.2011.11.019
Bu, X., Xue, J., Wu, Y., and Ma, W. (2020). Effect of biochar on seed germination and seedling growth of robinia pseudoacacia L. In karst calcareous soils. Commun. Soil Sci. Plant Anal. 1–12.
Burke, J. M., Longer, D. E., Oosterhuis, D. M., Kawakami, E. M., and Loka, D. A. (2012). The effect of source of biochar on cotton seedling growth and development. AAES Res. Ser. 610, 85–88.
Buss, W., Bogush, A., Ignatyev, K., and Masek, O. (2020). Unlocking the fertilizer potential of waste-derived biochar. ACS Sustain. Chem. Engin. 8 (32), 12295–12303. doi:10.1021/acssuschemeng.0c04336
Butnan, S., Deenik, J. L., Toomsan, B., and Vityakon, P. (2017). Biochar properties affecting carbon stability in soils contrasting in texture and mineralogy. Agric. Nat. Resour. 51, 492–498. doi:10.1016/j.anres.2018.03.002
Cara, I. G., Țopa, D., Puiu, I., and Jitareanu, G. (2022). Biochar a promising strategy for pesticide-contaminated soils. Agriculture 12, 1579. doi:10.3390/agriculture12101579
Cardinael, R., Chevallier, T., Cambou, A., Béral, C., Barthès, B. G., Dupraz, C., et al. (2017). Increased soil organic carbon stocks under agroforestry: A survey of six different sites in France. Agric. Ecosyst. Environ. 236, 243–255. doi:10.1016/j.agee.2016.12.011
Carvalho, M. L., Moraes, M. T., Cerri, C. E. P., and Cherubin, M. R. (2020). Biochar amendment enhances water retention in a tropical sandy soil. Agriculture 10, 62. doi:10.3390/agriculture10030062
Case, S. D. C., McNamara, N. P., Reay, D. S., and Whitaker, J. (2012). The effect of biochar addition on N2O and CO2 emissions from a sandy loam soil: The role of soil aeration. Soil Biol. biochem. 51, 125–134. doi:10.1016/j.soilbio.2012.03.017
Castaldi, S., Riondino, M., Baronti, S., Esposito, F. R., Marzaioli, R., Rutigliano, F. A., et al. (2011). Impact of biochar application to a Mediterranean wheat crop on soil microbial activity and greenhouse gas fluxes. Chemosphere 85, 1464–1471. doi:10.1016/j.chemosphere.2011.08.031
Cayuela, M. L., Van Zwieten, L., Singh, B. P., Jeffery, S., Roig, A., and Sánchez-Monedero, M. A. (2014). Biochar’s role in mitigating soil nitrous oxide emissions: A review and meta-analysis. Agric. Ecosyst. Environ. 191, 5–16. doi:10.1016/j.agee.2013.10.009
Chan, K. Y., Van Zwieten, L., Meszaros, I., Downie, A., and Joseph, S. (2007). Agronomic values of green waste biochar as a soil amendment. Aust. J. Soil Res. 45, 629–634. doi:10.1071/sr07109
Chen, D., Liu, X., Bian, R., Cheng, K., Zhang, X., Zheng, J., et al. (2018). Effects of biochar on availability and plant uptake of heavy metals- A meta-analysis. J. Environ. Manag. 222, 76–85. doi:10.1016/j.jenvman.2018.05.004
Chen, K., Li, N., Zhang, S., Liu, N., Yang, J., Zhan, X., et al. (2022a). Biochar-induced changes in the soil diazotroph community abundance and structure in a peanut field trial. Biochar 4, 26. doi:10.1007/s42773-022-00133-6
Chen, X., Duan, M., Zhou, B., and Cui, L. (2022b). Effects of biochar nanoparticles as a soil amendment on the structure and hydraulic characteristics of a sandy loam soil. Soil Use Manage 38, 836–849. doi:10.1111/sum.12740
Chew, J., Joseph, S., Chen, G., Zhang, Y., Zhu, L., Liu, M., et al. (2022). Biochar-based fertiliser enhances nutrient uptake and transport in rice seedlings. Sci. Total Environ. 826, 154174. doi:10.1016/j.scitotenv.2022.154174
Chowdhury, M. A., de Neergaard, A., and Jensen, L. S. (2014). Potential of aeration flow rate and bio-char addition to reduce greenhouse gas and ammonia emissions during manure composting. Chemosphere 97, 16–25. doi:10.1016/j.chemosphere.2013.10.030
Chunyu, T., Fan, Y., and Markus, A. (2022). Carbon materials advancing microorganisms in driving soil organic carbon regulation. Res 2022, 1–12. doi:10.34133/2022/9857374
Cong, W., Meng, J., and Ying, S. C. (2018). Impact of soil properties on the soil methane flux response to biochar addition: A meta-analysis. Environ. Sci. Process. Impacts 20, 1202–1209. doi:10.1039/c8em00278a
Cornelissen, G., Martinsen, V., Shitumbanuma, V., Alling, V., Breedveld, G., Rutherford, D., et al. (2013). Biochar effect on maize yield and soil characteristics in five conservation farming sites in Zambia. Agron 3, 256–274. doi:10.3390/agronomy3020256
Dai, Z., Xiong, X., Zhu, H., Xu, H., Leng, P., Li, J., et al. (2021). Association of biochar properties with changes in soil bacterial, fungal and fauna communities and nutrient cycling processes. Biochar 3, 3239–3254. doi:10.1007/s42773-021-00099-x
Das, S. K., Ghosh, G. K., and Avasthe, R. (2020). Evaluating biomas-derived biochar on seed germination and early seedling growth of maize and black gram. Biomass Convers. biorefin. 1, 14.
Dawar, K., Fahad, S., Alam, S. S., Khan, S. A., Dawar, A., Younis, U., et al. (2021). Influence of variable biochar concentration on yield-scaled nitrous oxide emissions, Wheat yield and nitrogen use efficiency. Sci. Rep. 11, 1–10. doi:10.1038/s41598-021-96309-4
Demisie, W., Liu, Z., and Zhang, M. (2014). Effect of biochar on carbon fractions and enzyme activity of red soil. CATE 121, 214–221. doi:10.1016/j.catena.2014.05.020
Deolikar, R., and Patil, R. (2022). Recent advances in pesticides removal using agroindustry based biochar. Dev. Wastewater Treat. Res. Pro., 265–290.
Ding, Z. H., Hu, X., Wan, Y., Wang, S., and Gao, B. (2016a). Removal of lead, copper, cadmium, zinc, and nickel from aqueous solutions by alkali-modified biochar: Batch and column tests. J. Ind. Eng. Chem. 33, 239–245. doi:10.1016/j.jiec.2015.10.007
Ding, Z. H., Wan, Y. S., Hu, X., Wang, S. S., Zimmerman, A. R., and Gao, B. (2016b). Sorption of lead and methylene blue onto hickory biochars from different pyrolysis temperatures: Importance of physicochemical properties. J. Ind. Eng. Chem. 37, 261–267. doi:10.1016/j.jiec.2016.03.035
Domene, X., Mattana, S., Hanley, K., Enders, A., and Lehmann, J. (2014). Medium-term effects of corn biochar addition on soil biota activities and functions in a temperate soil cropped to corn. Soil Biol. biochem. 72, 152–162. doi:10.1016/j.soilbio.2014.01.035
Downie, A., Crosky, A., and Munroe, P. (2009). “Physical properties of biochar,” in Biochar for environmental management: Science and technology. Editors J. Lehmann, and S. Joseph (London: Earthscan), 13–32.
Edeh, I. G., Masek, O., and Buss, W. (2020). A meta-analysis on biochar's effects on soil water properties - new insights and future research challenges. Sci. Total Environ. 714, 136857. doi:10.1016/j.scitotenv.2020.136857
Edenborn, S. L., Edenborn, H. M., Krynock, R. M., and Haug, K. L. Z. (2015). Influence of biochar application methods on the phytostabilization of a hydrophobic soil contaminated with lead and acid tar. J. Environ. Manage. 150, 226–234. doi:10.1016/j.jenvman.2014.11.023
Egamberdieva, D., Ma, H., Alaylar, B., Zoghi, Z., Kistaubayeva, A., Wirth, S., et al. (2021). Biochar amendments improve licorice (Glycyrrhiza uralensis Fisch.) growth and nutrient uptake under salt stress. Plan. Theory 10, 2135. doi:10.3390/plants10102135
Eger, M., Graz, M., Riede, S., and Breves, G. (2018). Application of MootralTM reduces methane production by altering the archaea community in the rumen simulation technique. Front. Microbiol. 9, 2094. doi:10.3389/fmicb.2018.02094
El-Naggar, A., Awad, Y. M., Tang, X. Y., Liu, C., Niazi, N. K., Jien, S. H., et al. (2018). Biochar influences soil carbon pools and facilitates interactions with soil: A field investigation. Land Degrad. Dev. 29, 2162–2171. doi:10.1002/ldr.2896
El-Naggar, A., Lee, S. S., Awad, Y. M., Yang, X., Ryu, C., Rizwan, M., et al. (2021). Influence of soil properties and feedstocks on biochar potential for carbon mineralization and improvement of infertile soils. Geoderma 332, 100–108. doi:10.1016/j.geoderma.2018.06.017
El-Naggar, A., Lee, S. S., Rinklebe, J., Farooq, M., Song, H., Sarmah, A. K., et al. (2019). Biochar application to low fertility soils: A review of current status, and future prospects. Geoderma 337, 536–554. doi:10.1016/j.geoderma.2018.09.034
Essa, R. E., Afifi, A. A., El-Ashry, S. M., and Ahmed, M. A. (2021). Rice straw biochar application and its impact on yield of some faba bean varieties in sandy soil. Pak. J. Biol. Sci. 24, 1236–1245. doi:10.3923/pjbs.2021.1236.1245
Eyles, A., Bound, S. A., Oliver, G., Corkrey, R., Hardie, M., Green, S., et al. (2015). Impact of biochar amendment on the growth, physiology and fruit of a young commercial apple orchard. Trees 29, 1817–1826. doi:10.1007/s00468-015-1263-7
Fang, B., Lee, X., Zhang, J., Li, Y., Zhang, L., Cheng, J., et al. (2016). Impacts of straw biochar additions on agricultural soil quality and greenhouse gas fluxes in karst area, Southwest China. Soil Sci. Plant Nutr. 62, 526–533. doi:10.1080/00380768.2016.1202734
Farid, I. M., Siam, H. S., Abbas, M. H., Mohamed, I., Mahmoud, S. A., Tolba, M., et al. (2022). Co-Composted biochar derived from rice straw and sugarcane bagasse improved soil properties, carbon balance, and zucchini growth in a sandy soil: A trial for enhancing the health of low fertile arid soils. Chemosphere 292, 133389. doi:10.1016/j.chemosphere.2021.133389
Feng, W. Y., Yang, F., Cen, R., Liu, J., Qu, Z. Y., Miao, Q. F., et al. (2021). Effects of straw biochar application on soil temperature, available nitrogen and growth of corn. J. Environ. Manage. 277, 111331. doi:10.1016/j.jenvman.2020.111331
Feng, Y., Xu, Y., Yu, Y., Xie, Z., and Lin, X. (2012). Mechanisms of biochar decreasing methane emission from Chinese paddy soils. Soil Biol. biochem. 46, 80–88. doi:10.1016/j.soilbio.2011.11.016
Foster, E. J., Hansen, N., Wallenstein, M., and Cotrufo, M. F. (2016). Biochar and manure amendments impact soil nutrients and microbial enzymatic activities in a semi-arid irrigated maize cropping system. Agric. Ecosyst. Environ. 233, 404–414. doi:10.1016/j.agee.2016.09.029
French, E., and Iyer-Pascuzzi, A. S. (2018). A role for the gibberellin pathway in biochar-mediated growth promotion. Sci. Rep. 8 (1), 1–10. doi:10.1038/s41598-018-23677-9
Frenkel, O., Jaiswal, A. K., Elad, Y., Lew, B., Kammann, C., and Graber, E. R. (2017). The effect of biochar on plant diseases: What should we learn while designing biochar substrates? J. Environ. Eng. Landsc. 25, 105–113. doi:10.3846/16486897.2017.1307202
Furtado, G. D. F., Chaves, L. H. G., de Sousa, J. R. M., Arriel, N. H. C., Xavier, D. A., de Lima, G. S., et al. (2016). Soil chemical properties, growth and production of sunflower under fertilization with biochar and NPK. Aust. J. Crop Sci. 10, 418–424. doi:10.21475/ajcs.2016.10.03.p7306
Gao, L., Wang, R., Shen, G., Zhang, J., Meng, G., and Zhang, J. (2017). Effects of biochar on nutrients and the microbial community structure of tobacco-planting soils. J. Soil Sci. Plant Nutr. 17, 884–896. doi:10.4067/s0718-95162017000400004
Gao, S., DeLuca, T. H., and Cleveland, C. C. (2019). Biochar additions alter phosphorus and nitrogen availability in agricultural ecosystems: A meta-analysis. Sci. Total Environ. 654, 463–472. doi:10.1016/j.scitotenv.2018.11.124
Gao, S., and DeLuca, T. H. (2016). Influence of biochar on soil nutrient transformations, nutrient leaching, and crop yield. Adv. Plants Agric. Res. 4, 348–362.
Gasco, G., Cely, P., Paz-Ferreiro, J., Plaza, C., and Mendez, A. (2016a). Relation between biochar properties and effects on seed germination and plant development. Biol. Agric. Hortic. 32, 237–247. doi:10.1080/01448765.2016.1166348
Gasco, G., Paz-Ferreiro, J., Cely, P., Plaza, C., and Mendez, A. (2016b). Influence of pig manure and its biochar on soil CO2 emissions and soil enzymes. Ecol. Eng. 95, 19–24. doi:10.1016/j.ecoleng.2016.06.039
Gaskin, J. W., Speir, R. A., Harris, K., Das, K. C., Lee, R. D., Morris, L. A., et al. (2010). Effect of peanut hull and pine chip biochar on soil nutrients, corn nutrient status, and yield. Agron. J. 102, 623–633. doi:10.2134/agronj2009.0083
Gelova, Z., Gallei, M., Pernisova, M., Brunoud, G., Zhang, X., Glanc, M., et al. (2021). Developmental roles of Auxin binding protein 1 in Arabidopsis thaliana. Plant Sci. 303, 110750. doi:10.1016/j.plantsci.2020.110750
Glaser, B., and Lehr, V. I. (2019). Biochar effects on phosphorus availability in agricultural soils: A metaanalysis. Sci. Rep. 9, 9338. doi:10.1038/s41598-019-45693-z
Goiri, I., Ruiz, R., Atxaerandio, R., Lavin, J. L., Diaz de Otalora, X., and Garcia-Rodriguez, A. (2021). Assessing the potential use of a feed additive based on biochar on broilers feeding upon productive performance, pH of digestive organs, cecum fermentation and bacterial community. Anim. Feed Sci. Technol. 279, 115039. doi:10.1016/j.anifeedsci.2021.115039
Gomes, M., and Garcia, Q. (2013). Reactive oxygen species and seed germination. Biologia 68, 351–357. doi:10.2478/s11756-013-0161-y
Gomez, M. A., Poveda, J., and Escobar, C. (2022). Overview of the use of biochar from main cereals to stimulate plant growth. Front. Plant Sci. 13, 912264. doi:10.3389/fpls.2022.912264
Gonzaga, M. I. S., Mackowiak, C. L., Comerford, N. B., Moline, E. F. da V., Shirley, J. P., and Guimaraes, D. V. (2017). Pyrolysis methods impact biosolids-derived biochar composition, maize growth and nutrition. Soil Tillage Res. 165, 59–65. doi:10.1016/j.still.2016.07.009
Gross, C. D., Edward, W., Cameron, N., Carlyle, S., and Chang, X. (2022). Biochar and its manure-based feedstock have divergent effects on soil organic carbon and greenhouse gas emissions in croplands. Sci. Total Environ. 806, 151337. doi:10.1016/j.scitotenv.2021.151337
Guerena, D., Lehmann, J., Hanley, K., Enders, A., Hyland, C., and Riha, S. (2013). Nitrogen dynamics following field application of biochar in a temperate North American maize-based production system. Plant Soil 365, 239–254. doi:10.1007/s11104-012-1383-4
Gul, S., and Whalen, J. K. (2016). Biochemical cycling of nitrogen and phosphorus in biochar-amended soils. Soil Biol. biochem. 103, 1–15. doi:10.1016/j.soilbio.2016.08.001
Gunes, A., Inal, A., Taskin, M. B., Sahin, O., Kaya, E. C., and Atakol, A. (2014). Effect of phosphorus-enriched biochar and poultry manure on growth and mineral composition of lettuce (Lactuca sativa L. cv.) grown in AL-WABEL ET AL. 31 alkaline soil. Soil Use Manag. 30, 182–188.
Guo, L., Yu, H., Kharbach, M., and Wang, J. (2021). The response of nutrient uptake, photosynthesis and yield of tomato to biochar addition under reduced nitrogen application. Agron 11, 1598. doi:10.3390/agronomy11081598
Hafeez, A., Pan, T., Tian, J., and Cai, K. (2022). Modified biochars and their effects on soil quality: A review. Environments 9, 60. doi:10.3390/environments9050060
Hafeez, E. M., Gowayed, S. M., Nehela, Y., Sakran, R. M., Rady, A., Awadalla, A., et al. (2021). Incorporated biochar-based soil amendment and exogenous glycine betaine foliar application ameliorate rice (Oryza sativa L.) tolerance and resilience to osmotic stress. Plants 10, 1930. doi:10.3390/plants10091930
Hafeez, Y., Iqbal, S., Jabeen, K., Shahzad, S., Jahan, S., and Rasul, F. (2017). Effect of biochar application on seed germination and seedling growth of Glycine max (l.) Merr. Under drought stress. Pak. J. Bot. 49, 7–13.
Haider, F. U., Coulter, J. A., Cheema, S. A., Farooq, M., Wu, J., Zhang, R., et al. (2021). Co-application of biochar and microorganisms improves soybean performance and remediate cadmium-contaminated soil. Ecotoxicol. Environ. Saf. 214, 112112. doi:10.1016/j.ecoenv.2021.112112
Haider, G., Koyro, H. W., Azam, F., Steffens, D., Mculler, C., and Kammann, C. (2015). Biochar but not humic acid product amendment affected maize yields via improving plant soil moisture relations. Plant Soil 395, 141–157. doi:10.1007/s11104-014-2294-3
Haider, I., Raza, M. A. S., Iqbal, R., Aslam, M. U., Habib-ur-Rahman, M., Raja, S., et al. (2020). Potential effects of biochar application on mitigating the drought stress implications on wheat (Triticum aestivum L.) under various growth stages. J. Saudi. Chem. Soc. 24, 974–981. doi:10.1016/j.jscs.2020.10.005
Haider, U. F., Cai, L., Hussain, S., Cheema, S. A., Wu, J., and Zhang, R. (2022). An overview on biochar production, its implications, and mechanisms of biochar-induced amelioration of soil and plant characteristics. Pedosphere 32 (1), 107–130. doi:10.1016/s1002-0160(20)60094-7
Hamamoto, T., Nhamo, N., Chikoye, D., Mukumbuta, I., and Uchida, Y. (2022). Responses of CO2 emissions and soil microbial community structures to organic amendment in two contrasting soils in Zambia. Sci. Rep. 12, 6368. doi:10.1038/s41598-022-10368-9
Hameeda, S. G., Gul, B., Misbah, M., Tasawar, A. C., Adnan, A. A., and Awan, A. A. (2019). Biochar and manure influences tomato fruit yield, heavy metal accumulation and concentration of soil nutrients under wastewater irrigation in arid climatic conditions. Cogent. Food Agric. 5, 1576406. doi:10.1080/23311932.2019.1576406
Hansen, V., Hauggaard-Nielsen, H., Petersen, C. T., Mikkelsen, T. N., and Muller-Stover, D. (2016). Effects of gasification biochar on plant-available water capacity and plant growth in two contrasting soil types. Soil Tillage Res. 161, 1–9. doi:10.1016/j.still.2016.03.002
Hasanuzzaman, M., and Fujita, M. (2022). Plant responses and tolerance to salt stress: Physiological and molecular interventions. Int. J. Mol. Sci. 23, 4810. doi:10.3390/ijms23094810
He, X., Hong, Z. N., Shi, R. Y., Cui, J. Q., Lai, H. W., Lu, H. L., et al. (2021). The effects of H2O2-and HNO3/H2SO4-modified biochars on the resistance of acid paddy soil to acidification. Environ. Pollut. 293, 118588. doi:10.1016/j.envpol.2021.118588
Herath, H. M. S. K., Camps-Arbestain, M., and Hedley, M. (2013). Effect of biochar on soil physical properties in two contrasting soils: An Alfisol and an Andisol. Geoderma 210, 188–197. doi:10.1016/j.geoderma.2013.06.016
Hilioti, Z., Michailof, C. M., Valasiadis, D., Iliopoulou, E. F., Koidou, V., and Lappas, A. A. (2017). Characterization of castor plant-derived biochars and their effects as soil amendments on seedlings. Biomass Bioenerg. 105, 96–106. doi:10.1016/j.biombioe.2017.06.022
Hou, W. H., Zhang, Y. X., Wang, H. J., Zhang, Q. X., Hou, M. L., and Cong, B. M. (2021). Effects of nitrogen application level on leaf photosynthetic characteristics and chlorophyll fluorescence characteristics of Leymus chinensis. Acta Agrestia Sin. 29, 531–536.
Huang, X., Li, S., Li, S., Ye, G., Lu, L., Zhang, L., et al. (2019). The effects of biochar and dredged sediments on soil structure and fertility promote the growth, photosynthetic and rhizosphere microbial diversity of Phragmites communis (Cav.). Trin. Ex. Steud. Sci. Total Environ. 697, 134073. doi:10.1016/j.scitotenv.2019.134073
Ibrahim, M., Li, G., Chan, F. K. S., Kay, P., Liu, X. X., Firbank, L., et al. (2019). Biochars effects potentially toxic elements and antioxidant enzymes in Lactuca sativa L. grown in multi-metals contaminated soil. Environ. Technol. Innov. 15, 100427. doi:10.1016/j.eti.2019.100427
Igalavithana, A. D., Ok, Y. S., Niazi, N. K., Rizwan, M., Al-Wabel, M. I., Usman, A. R. A., et al. (2017). Effect of corn residue biochar on the hydraulic properties of sandy-loam soil. Sustainability 9, 266. doi:10.3390/su9020266
Inal, A., Gunes, A., Sahin, O., Taskin, M. B., and Kaya, E. C. (2015). Impacts of biochar and processed poultry manure applied to a calcareous soil, on the growth of bean and maize. Soil Use Manag. 31, 106–113. doi:10.1111/sum.12162
Ippolito, J. A., Novak, J. M., Busscher, W. J., Ahmedna, M., Rehrah, D., and Watts, D. W. (2012). Switchgrass biochar affects two aridisols. J. Environ. Qual. 41, 1123–1130. doi:10.2134/jeq2011.0100
Irfan, M., Hussain, Q., Khan, K. S., Akmal, M., Ijaz, S. S., Hayat, R., et al. (2019). Response of soil microbial biomass and enzymatic activity to biochar amendment in the organic carbon deficient arid soil: A 2-year field study. Arab. J. Geosci. 12, 95. doi:10.1007/s12517-019-4239-x
Irshad, M. K., Noman, A., Alhaithloul, H. A. S., Adeel, M., Rui, Y., Shah, T., et al. (2020). Goethite-modified biochar ameliorates the growth of rice (Oryza sativa L.) plants by suppressing Cd and As-induced oxidative stress in Cd and as co-contaminated paddy soil. Sci. Total Environ. 717, 137086. doi:10.1016/j.scitotenv.2020.137086
Jay, C. N., Fitzgerald, J. D., Hipps, N. A., and Atkinson, C. J. (2015). Why short- term biochar application has no yield benefits: Evidence from three field-grown crops. Soil Use Manag. 31, 241–250. doi:10.1111/sum.12181
Jaiswal, A. K., Elad, Y., Graber, E. R., and Frenkel, O. (2014). Rhizoctonia solani suppression and plant growth promotion in cucumber as affected by biochar pyrolysis temperature, feedstock and concentration. Soil Biol. biochem. 69, 110–118. doi:10.1016/j.soilbio.2013.10.051
Jaiswal, A. K., Frenkel, O., Elad, Y., Lew, B., and Graber, E. R. (2015). Non-monotonic influence of biochar dose on bean seedling growth and susceptibility to Rhizoctonia solani: The “shifted rmax-effect. Plant Soil 395, 125–140. doi:10.1007/s11104-014-2331-2
Jaiswal, A. K., Frenkel, O., Tsechansky, L., Elad, Y., and Graber, E. R. (2018). Immobilization and deactivation of pathogenic enzymes and toxic metabolites by biochar: A possible mechanism involved in soilborne disease suppression. Soil Biol. biochem. 121, 59–66. doi:10.1016/j.soilbio.2018.03.001
Jandl, G., Eckhardt, K. U., Bargmann, I., Kucke, M., Greef, J. M., Knicker, H., et al. (2013). Hydrothermal carbonization of biomass residues: Mass spectrometric characterization for ecological effects in the soil-plant system. J. Environ. Qual. 42 (1), 199–207. doi:10.2134/jeq2012.0155
Jia, W., Sun, X., Gao, Y., Yang, Y. C., and Yang, L. Y. (2020). Fe-modified biochar enhances microbial nitrogen removal capability of constructed wetland. Sci. Total Environ. 740, 139534. doi:10.1016/j.scitotenv.2020.139534
Jiang, W., Xu, L., Liu, Y., Su, W., Yan, J., and Xu, D. (2022). Effect of biochar on the growth, photosynthesis, antioxidant system and cadmium content of Mentha piperita ‘chocolate’ and Mentha spicata in cadmium-contaminated soil. Agron 12, 2737. doi:10.3390/agronomy12112737
Jiang, Z., Yang, S., Pang, Q., Xu, Y., Chen, X., Sun, X., et al. (2021). Biochar improved soil health and mitigated greenhouse gas emission from controlled irrigation paddy field: Insights into microbial diversity. J. Clean. Prod. 318, 128595. doi:10.1016/j.jclepro.2021.128595
Jien, S. H., and Wang, C. S. (2013). Effects of biochar on soil properties and erosion potential in a highly weathered soil. Catena 110, 225–233. doi:10.1016/j.catena.2013.06.021
Jin, Y., Liang, X., He, M., Liu, Y., Tian, G., and Shi, J. (2016). Manure biochar influence upon soil properties, phosphorus distribution and phosphatase activities: A microcosm incubation study. Chemosphere 142, 128–135. doi:10.1016/j.chemosphere.2015.07.015
Jones, B. E. H., Haynes, R. J., and Phillips, I. R. (2010). Effect of amendment of bauxite processing sand with organic materials on its chemical, physical and microbial properties. J. Environ. Manag. 91, 2281–2288. doi:10.1016/j.jenvman.2010.06.013
Jones, D. L., Rousk, J., Edwards-Jones, G., DeLuca, T. H., and Murphy, D. V. (2012). Biochar-mediated changes in soil quality and plant growth in a three year field trial. Soil Biol. biochem. 45, 113–124. doi:10.1016/j.soilbio.2011.10.012
Joseph, S., Cowie, A. L., Van Zwieten, L., Bolan, N., Budai, A., Buss, W., et al. (2021). How biochar works, and when it doesn’t: A review of mechanisms controlling soil and plant responses to biochar. GCB Bioenerg. 13, 1731–1764.
Kader, S. A., Spalevic, V., and Dudic, B. (2022). Feasibility study for estimating optimal substrate parameters for sustainable green roof in Sri Lanka. Environ. Dev. Sustain., 1–27. doi:10.1007/s10668-022-02837-y
Kalu, S., Kulmala, L., Zrim, J., Peltokangas, K., Tammeorg, P., Rasa, K., et al. (2022). Potential of biochar to reduce greenhouse gas emissions and increase nitrogen use efficiency in boreal arable soils in the long-term. Front. Environ. Sci. 10, 914766. doi:10.3389/fenvs.2022.914766
Kalus, K., Konkol, D., Korczynski, M., Koziel, J. A., and Opalinski, S. (2020). Laying hens biochar diet supplementation—effect on performance, excreta N content, NH3 and VOCs emissions, egg traits and egg consumers acceptance. Agriculture 10, 237. doi:10.3390/agriculture10060237
Karhu, K., Mattila, T., Bergstrom, I., and Regina, K. (2011). Biochar addition to agricultural soil increased CH4 uptake and water holding capacity-results from a short-term pilot field study. Agric. Ecosyst. Environ. 140, 309–313. doi:10.1016/j.agee.2010.12.005
Karimi, A., Moezzi, A., Chorom, M., and Enayatizamir, N. (2020). Application of biochar changed the status of nutrients and biological activity in a calcareous soil. J. Soil Sci. Plant Nutr. 20, 450–459. doi:10.1007/s42729-019-00129-5
Kavitha, B., Reddy, P. V. L., Kim, B., Lee, S. S., Pandey, S. K., and Kim, K. H. (2018). Benefits and limitations of biochar amendment in agricultural soils: A review. J. Environ. Manage. 227, 146–154. doi:10.1016/j.jenvman.2018.08.082
Kaya, C., Akram, N. A., Ashraf, M., Alyemeni, M. N., and Ahmad, P. (2020). Exogenously supplied silicon (Si) improves cadmium tolerance in pepper (Capsicum annuum L.) by up-regulating the synthesis of nitric oxide and hydrogen by Up-Regulating the Synthesis of Nitric Oxide and Hydrogen Sulfide. J. Biotechnol. 316, 35–45. doi:10.1016/j.jbiotec.2020.04.008
Khajavi-Shojaei, S., Moezzi, A., Norouzi Masir, M., and Taghavi, M. (2020). Synthesis modified biochar-based slow-release nitrogen fertilizer increases nitrogen use efficiency and corn (Zea mays L.) growth. Biomass Conv. bioref. 15, 1–9. doi:10.1007/s13399-020-01137-7
Khan, N., Clark, I., Sánchez-Monedero, M. A., Shea, S., Meier, S., and Bolan, N. (2014). Maturity indices in co-composting of chicken manure and sawdust with biochar. Bioresour. Technol. 168, 245–251. doi:10.1016/j.biortech.2014.02.123
Khan, S., Chao, C., Waqas, M., Arp, H. P. H., and Zhu, Y. G. (2013). Sewage sludge biochar influence upon rice (Oryza sativa L) yield, metal bioaccumulation and greenhouse gas emissions from acidic paddy soil. Environ. Sci. Technol. 47, 8624–8632. doi:10.1021/es400554x
Khorram, M., Fatemi, A., Khan, M. A., Kiefer, R., and Jafarnia, S. (2018). Potential risk of weed outbreak by increasing biochar's application rates in slow-growth legume, lentil (Lens culinaris Medik.). J. Sci. Food Agr. 98, 2080–2088. doi:10.1002/jsfa.8689
Kimura, A., Uchida, Y., and Madegwa, Y. M. (2022). Legume species alter the effect of biochar application on microbial diversity and functions in the mixed cropping system—based on a pot experiment. Agriculture 12 (10), 1548. doi:10.3390/agriculture12101548
Kloss, S., Zehetner, F., Wimmer, B., Buecker, J., Rempt, F., and Soja, G. (2014). Biochar application to temperate soils: Effects on soil fertility and crop growth under greenhouse conditions. J. Plant Nutr. Soil Sci. 177, 3–15. doi:10.1002/jpln.201200282
Kochanek, J., Long, R. L., Lisle, A. T., and Flematti, G. R. (2016). Karrikins identified in biochars indicate post-fire chemical cues can influence community diversity and plant development. PLoS One 11, e0161234. doi:10.1371/journal.pone.0161234
Kochanek, J., Soo, R. M., Martinez, C., Dakuidreketi, A., and Mudge, A. M. (2022). Biochar for intensification of plant-related industries to meet productivity, sustainability and economic goals: A review. Resour. Conserv. Recycl. 179, 106109. doi:10.1016/j.resconrec.2021.106109
Kocsis, T., Ringer, M., and Biro, B. (2022). Characteristics and applications of biochar in soil–plant systems: A short review of benefits and potential drawbacks. Appl. Sci. 12, 4051. doi:10.3390/app12084051
Kumar, A., Bhattacharya, T., Mukherjee, S., and Sarkar, B. (2022). A perspective on biochar for repairing damages in the soil–plant system caused by climate change-driven extreme weather events. Biochar 4, 22. doi:10.1007/s42773-022-00148-z
Kumar, A., Joseph, S., Tsechansky, L., Schreiter, I. J., Schuth, C., Taherysoosavi, S., et al. (2020). Mechanistic evaluation of biochar potential for plant growth promotion and alleviation of chromium-induced phytotoxicity in Ficus elastica. Chemosphere 243, 125332. doi:10.1016/j.chemosphere.2019.125332
Kumar, M., Sundaram, S., Gnansounou, E., Larroche, C., and Thakur, I. S. (2018). Carbon dioxide capture, storage and production of biofuel and biomaterials by bacteria: A review. Bioresour. Technol. 247, 1059–1068. doi:10.1016/j.biortech.2017.09.050
Kwapinski, W., Byrne, C. M. P., Kryachko, E., Wolfram, P., Adley, C., Leahy, J. J., et al. (2010). Biochar from biomass and waste. Waste Biomass Valorization 1, 177–189. doi:10.1007/s12649-010-9024-8
Lai, H., Gao, F., Su, H., Zheng, P., Li, Y., and Yao, H. (2022). Nitrogen distribution and soil microbial community characteristics in a legume-cereal intercropping system: A review. Agron 12, 1900. doi:10.3390/agronomy12081900
Lai, W. Y., Lai, C. M., Ke, G. R., Chung, R. S., Chen, C. T., Cheng, C. H., et al. (2013). The effects of woodchip biochar application on crop yield, carbon sequestration and greenhouse gas emissions from soils planted with rice or leaf beet. J. Taiwan Inst. Chem. Eng. 44, 1039–1044. doi:10.1016/j.jtice.2013.06.028
Laird, D. A., Fleming, P., Davis, D. D., Horton, R., Wang, B., and Karlen, D. L. (2010). Impact of biochar amendments on the quality of a typical Midwestern agricultural soil. Geoderma 158, 443–449. doi:10.1016/j.geoderma.2010.05.013
Layek, J., Narzari, R., Hazarika, S., Das, A., Rangappa, K., Devi, S., et al. (2022). Prospects of biochar for sustainable agriculture and carbon sequestration: An overview for eastern himalayas. Sustain 14, 6684. doi:10.3390/su14116684
Lehmann, J., Cowie, A., Masiello, C. A., Kammann, C., Woolf, D., Amonette, J. E., et al. (2021). Biochar in climate change mitigation. Nat. Geoscie 14 (12), 883–892. doi:10.1038/s41561-021-00852-8
Lehmann, J., da Silva, J. P., Rondon, M., Cravo, M. da S., Greenwood, J., Nehls, T., et al. (2002). “Slash and char - a feasible alternative for soil fertility management in the central Amazon?,” in In17th World congress of soil science (Bangkok, Thailand: The International Union of Soil Sciences), 412–449.
Lei, S., Shi, Y., Qiu, Y., Che, L., and Xue, C. (2019). Performance and mechanisms of emerging animal-derived biochars for immobilization of heavy metals. Sci. Total Environ. 646, 1281–1289. doi:10.1016/j.scitotenv.2018.07.374
Levesque, V., Oelbermann, M., and Ziadi, N. (2021). Biochar in temperate soils: Opportunities and challenges. Can. J. Soil Sci. 102 (1), 1–26. doi:10.1139/cjss-2021-0047
Levesque, V., Rochette, P., Ziadi, N., Dorais, M., and Antoun, H. (2018). Mitigation of CO2, CH4 and N2O from a fertigated horticultural growing medium amended with biochars and a compost. Appl. Soil Ecol. 126, 129–139. doi:10.1016/j.apsoil.2018.02.021
Li, H., Liu, Y., Chen, Y., Wang, S., Wang, M., Xie, T., et al. (2016). Biochar amendment immobilizes lead in rice paddy soils and reduces its phytoavailability. Sci. Rep. 6, 31616. doi:10.1038/srep31616
Li, S., and Chan, C. Y. (2022). Will biochar suppress or stimulate greenhouse gas emissions in agricultural fields? Unveiling the dice game through data syntheses. Soil Syst. 6, 73. doi:10.3390/soilsystems6040073
Li, Y., Wang, Z., Jiang, Z., Feng, L., Pan, J., Zhu, M., et al. (2022). Bio-based carbon materials with multiple functional groups and graphene structure to boost methane production from ethanol anaerobic digestion. Bioresour. Technol. 344, 126353. doi:10.1016/j.biortech.2021.126353
Lian, F., and Xing, B. (2017). Black carbon (biochar) in water/soil environments: Molecular structure, sorption, stability, and potential risk. Environ. Sci. Technol. 51, 13517–13532. doi:10.1021/acs.est.7b02528
Liesch, A. M., Weyers, S. L., Gaskin, J. W., and Das, K. C. (2014). Impact of two different biochars on earthworm growth and survival. Ann. Environ. Sci. 4, 1–9.
Lim, T. J., Spokas, K. A., Feyereisen, G., and Novak, J. M. (2016). Predicting the impact of biochar additions on soil hydraulic properties. Chemosphere 142, 136–144. doi:10.1016/j.chemosphere.2015.06.069
Liu, H., Xu, F., Xie, Y., Wang, C., Zhang, A., Li, L., et al. (2018). Effect of modified coconut shell biochar on availability of heavy metals and biochemical characteristics of soil in multiple heavy metals contaminated soil. Sci. Total Environ. 645, 702–709. doi:10.1016/j.scitotenv.2018.07.115
Liu, J., Qiu, H., Wang, C., Shen, J., Zhang, W., Cai, J., et al. (2020b). Effects of biochar amendment on greenhouse gas emission in two paddy soils with different textures. Paddy Water Environ. 19, 87–98. doi:10.1007/s10333-020-00821-8
Liu, M., Linna, C., Ma, S., Ma, Q., Guo, J., Wang, F., et al. (2022). Effects of biochar with inorganic and organic fertilizers on agronomic traits and nutrient absorption of soybean and fertility and microbes in purple soil. Front. Plant Sci. 13, 871021. doi:10.3389/fpls.2022.871021
Liu, S., Kong, F., Li, Y., Jiang, Z., Xi, M., and Wu, J. (2020a). Mineral-ions modified biochars enhance the stability of soil aggregate and soil carbon sequestration in a coastal wetland soil. Cate 193, 104618. doi:10.1016/j.catena.2020.104618
Liu, X., Wei, Z., Ma, Y., Liu, J., and Liu, F. (2021). Effects of biochar amendment and reduced irrigation on growth, physiology, water-use efficiency and nutrients uptake of tobacco (Nicotiana tabacum L.) on two different soil types. Sci. Total Environ. 770, 144769. doi:10.1016/j.scitotenv.2020.144769
Liu, Y., Lu, H., Yang, S., and Wang, Y. (2016). Impacts of biochar addition on rice yield and soil properties in a cold waterlogged paddy for two crop seasons. Field Crops Res. 191, 161–167. doi:10.1016/j.fcr.2016.03.003
Liu, Z., Dugan, B., Masiello, C. A., and Gonnermann, H. M. (2017). Biochar particle size, shape, and porosity act together to influence soil water properties. PLOS ONE 12, e0179079. doi:10.1371/journal.pone.0179079
Lu, H. L., Li, K. W., Nkoh, J. N., He, X., Hong, Z. N., Xu, R. K., et al. (2022). Effects of the increases in soil pH and pH buffering capacity induced by crop residue biochars on available Cd contents in acidic paddy soils. Chemosphere 301, 134674. doi:10.1016/j.chemosphere.2022.134674
Luigi, M., Manglli, A., Dragone, I., Antonelli, M. G., Contarini, M., Speranza, S., et al. (2022). Effects of biochar on the growth and development of tomato seedlings and on the response of tomato plants to the infection of systemic viral agents. Front. Microbiol. 13, 862075. doi:10.3389/fmicb.2022.862075
Mabe, L. T., Su, S., Tang, D., Zhu, W., Wang, S., and Dong, Z. (2017). The effect of dietary bamboo charcoal supplementation on growth and serum biochemical parameters of juvenile common carp (Cyprinus carpio L.). Aquac. Res. 49, 1142–1152. doi:10.1111/are.13564
Mack, M. C., Walker, X. J., Johnstone, J. F., Alexander, H. D., Melvin, A. M., Jean, M., et al. (2021). Carbon loss from boreal forest wildfires offset by increased dominance of deciduous trees. Sci 372, 280–283. doi:10.1126/science.abf3903
Major, J., Rondon, M., Molina, D., Riha, S. J., and Lehmann, J. (2010). Maize yield and nutrition during 4 years after biochar application to a Colombian savanna Oxisol. Plant Soil 333, 117–128. doi:10.1007/s11104-010-0327-0
Malik, L., Sanaullah, M., Mahmood, F., Hussain, S., Siddique, M. H., Anwar, F., et al. (2022). Unlocking the potential of co-applied biochar and plant growth-promoting rhizobacteria (PGPR) for sustainable agriculture under stress conditions. Chem. Biol. Technol. Agric. 9, 58. doi:10.1186/s40538-022-00327-x
Man, K. Y., Chow, K. L., Man, Y. B., Mo, W. Y., and Wong, M. H. (2020). Use of biochar as feed supplements for animal farming. Crit. Rev. Environ. Sci. Technol. 51, 187–217. doi:10.1080/10643389.2020.1721980
Mandal, S., Donner, E., Vasileiadis, S., Skinner, W., Smith, E., and Lombi, E. (2018). The effect of biochar feedstock, pyrolysis temperature, and application rate on the reduction of ammonia volatilisation from biochar-amended soil. Sci. Total Environ. 627, 942–950. doi:10.1016/j.scitotenv.2018.01.312
Manirakiza, E., Ziadi, N., Hamel, C., Lévesque, V., Antoun, H., and Karam, A. (2021). Soil microbial community dynamics after co-application of biochar and paper mill biosolids. Appl. Soil Ecol. 165, 103960. doi:10.1016/j.apsoil.2021.103960
Mannan, M. A., and Shashi, M. A. (2020). Amelioration of drought tolerance in maize using rice husk biochar. London, UK: Maize Prod Use. doi:10.5772/Intechopen.88824
Mannan, M., Halder, E., Karim, M., and Ahmed, J. (2017). Alleviation of adverse effect of drought stress on soybean (Glycine max. L.) by using poultry litter biochar. Bangladesh Agron. J. 19, 61–69. doi:10.3329/baj.v19i2.31854
Mao, J. D., Johnson, R. L., Lehmann, J., Olk, D. C., Neves, E. G., Thompson, M. L., et al. (2012). Abundant and stable char residues in soils: Implications for soil fertility and carbon sequestration. Environ. Sci. Technol. 46, 9571–9576. doi:10.1021/es301107c
Marks, E. A. N., Mattana, S., Alcaniz, J. M., and Domene, X. (2014). Biochars provoke diverse soil mesofauna reproductive responses in laboratory bioassays. Eur. J. Soil Biol. 60, 104–111. doi:10.1016/j.ejsobi.2013.12.002
Marks, E. A. N., Mattana, S., Alcaniz, J. M., Perez-Herrero, E., and Domene, X. (2016). Gasifier biochar effects on nutrient availability, organic matter mineralization, and soil fauna activity in a multi-year Mediterranean trial. Agric. Ecosyst. Environ. 215, 30–39. doi:10.1016/j.agee.2015.09.004
Martinez-Gomez, A., Poveda, J., and Escobar, C. (2022). Overview of the use of biochar from main cereals to stimulate plant growth. Front. Plant Sci. 13, 912264. doi:10.3389/fpls.2022.912264
Martisen, V., Mulder, J., Shitumbanuma, V., Sparrevik, M., Borresen, T., and Cornelissen, G. (2014). Farmer-led maize biochar trials: Effect on crop yield and soil nutrients under conservation farming. J. Plant Nutr. Soil Sci. 177, 681–695. doi:10.1002/jpln.201300590
Mau, A. E. E., and Utami, S. R. (2014). Effects of biochar amendment and arbuscular mycorrhizal fungi inoculation on availability of soil phosphorus and growth of maize. J. Degr. Min. Land Manage. 1, 69–74.
Mehmood, S., Ahmed, W., Ikram, M., Imtiaz, M., Mahmood, S., Tu, S. X., et al. (2020). Chitosan modified biochar increases soybean (Glycine max L.) resistance to salt-stress by augmenting root morphology, antioxidant defense mechanisms and the expression of stress-responsive genes. Plants 9, 1173. doi:10.3390/plants9091173
Mete, F. Z., Mia, S., Dijkstra, F. A., Abuyusuf, M. D., and Hossain, A. S. M. I. (2015). Synergistic effects of biochar and NPK fertilizer on soybean yield in an alkaline soil. Pedosphere 25, 713–719. doi:10.1016/s1002-0160(15)30052-7
Mia, S., Dijkstra, F. A., and Singh, B. (2017). Aging induced changes in biochar’s functionality and adsorption behavior for phosphate and ammonium. Environ. Sci. Technol. 51, 8359–8367. doi:10.1021/acs.est.7b00647
Mia, S., van Groenigen, J. W., van de Voorde, T. F. J., Oram, N. J., Bezemer, T. M., Mommer, L., et al. (2014). Biochar application rate affects biological nitrogen fixation in red clover conditional on potassium availability. Agric. Ecosyst. Environ. 191, 83–91. doi:10.1016/j.agee.2014.03.011
Mirheidari, A., Torbatinejad, N. M., Shakeri, P., and Mokhtarpour, A. (2019). Effects of biochar produced from different biomass sources on digestibility, ruminal fermentation, microbial protein synthesis and growth performance of male lambs. Small Rumin. Res. 183, 106042. doi:10.1016/j.smallrumres.2019.106042
Molina, G., Usmani, Z., Sharma, M., Yasri, A., and Gupta, V. K. (Editors) (2022). Microbes in agri-forestry biotechnology (Florida, United States: CRC Press).
Munera-Echeverri, J. L., Martinsen, V., Strand, L. T., Zivanovic, V., Cornelissen, G., and Mulder, J. (2018). Cation exchange capacity of biochar: An urgent method modification. Sci. total Environ. 642, 190–197. doi:10.1016/j.scitotenv.2018.06.017
Munoz, C., Gongora, S., and Zagal, E. (2016). Use of biochar as a soil amendment: A brief review. Chil. J. Agric. Anim. Sci. 32, 37–47.
Murtaza, G., Ahmed, Z., Dai, D. Q., Iqbal, R., Bawazeer, S., Usman, M., et al. (2022b). A review of mechanism and adsorption capacities of biochar based engineered composites for removing aquatic pollutants from contaminated water. Front. Environ. Sci. 10, 1035865. doi:10.3389/fenvs.2022.1035865
Murtaza, G., Ahmed, Z., and Usman, M. (2022a). Feedstock type, pyrolysis temperature and acid modification effects on physiochemical attributes of biochar and soil quality. Arab. J. Geosci. 15, 305. doi:10.1007/s12517-022-09539-9
Murtaza, G., Ahmed, Z., Usman, M., Tariq, W., Ullah, Z., Shareef, M., et al. (2021a). Biochar induced modifications in soil properties and its impacts on crop growth and production. J. Plant Nutr. 44, 1–15. doi:10.1080/01904167.2021.1871746
Murtaza, G., Ditta, A., Ullah, N., Usman, M., and Ahmed, Z. (2021b). Biochar for the management of nutrient impoverished and metal contaminated soils: Preparation, applications, and prospects. J. Soil Sci. Plant Nutr. 21, 2191–2213. doi:10.1007/s42729-021-00514-z
Nair, V. D., and Mukherjee, A. (2022). The use of biochar for reducing carbon footprints in land-use systems: Prospects and problems. Carb. Footprints 1, 12. doi:10.20517/cf.2022.2.07
Nan, Q., Wang, C., Wang, H., Yi, Q., Liang, B., Xu, J., et al. (2020). Biochar drives microbially-mediated rice production by increasing soil carbon. J. Hazard. Mat. 387, 121680. doi:10.1016/j.jhazmat.2019.121680
Natasha, N., Shahid, M., Khalid, S., Bibi, I., Naeem, M. A., Niazi, N. K., et al. (2022). Influence of biochar on trace element uptake, toxicity and detoxification in plants and associated health risks: A critical review. Crit. Rev. Environ. Sci. Technol. 52, 2803–2843. doi:10.1080/10643389.2021.1894064
Nehela, Y., Mazrou, Y. S., Alshaal, T., Rady, A., El-Sherif, A., Omara, A. E. D., et al. (2021). The integrated amendment of sodic-saline soils using biochar and plant growth-promoting rhizobacteria enhances maize (Zea mays L.) resilience to water salinity. Plants 10, 1960. doi:10.3390/plants10091960
Nelissen, V., Ruysschaert, G., Manka Abusi, D., Dhose, T., De Beuf, K., Al-Barri, B., et al. (2015). Impact of a woody biochar on properties of a sandy loam soil and spring barley during a two-year field experiment. Eur. J. Agron. 62, 65–78. doi:10.1016/j.eja.2014.09.006
Nguyen, B. T., Trinh, N. N., and Bach, Q. V. (2020). Methane emissions and associated microbial activities from paddy salt-affected soil as influenced by biochar and cow manure addition. Appl. Soil Ecol. 152, 103531. doi:10.1016/j.apsoil.2020.103531
Nguyen, D. H., Scheer, C., Rowlings, D. W., and Grace, P. R. (2016). Rice husk biochar and crop residue amendment in subtropical cropping soils: Effect on biomass production, nitrogen use efficiency and greenhouse gas emissions. Biol. Fertil. Soils 52, 261–270. doi:10.1007/s00374-015-1074-4
Nkoh, J. N., Ajibade, F. O., Atakpa, E. O., Abdulaha-Al Baquy, M., Mia, S., Odii, E. C., et al. (2022). Reduction of heavy metal uptake from polluted soils and associated health risks through biochar amendment: A critical synthesis. J. Hazard. Mat. Adv. 6, 100086. doi:10.1016/j.hazadv.2022.100086
Novak, J. M., Spokas, K. A., Cantrell, K. B., Ro, K. S., Watts, D. W., Glaz, B., et al. (2014). Effects of biochars and hydrochars produced from lignocellulosic and animal manure on fertility of a mollisol and entisol. Soil Use Manage 30, 175–181.
Obia, A., Mulder, J., Hale, S. E., Nurida, N. L., and Cornelissen, G. (2018). The potential of biochar in improving drainage, aeration and maize yields in heavy clay soils. PLoS One 13, e0196794. doi:10.1371/journal.pone.0196794
Obia, A., Mulder, J., Martinsen, V., Cornelissen, G., and Borresen, T. (2016). In situ effects of biochar on aggregation, water retention and porosity in light-textured tropical soils. Soil Tillage Res. 155, 35–44. doi:10.1016/j.still.2015.08.002
Olszyk, D. M., Shiroyama, T., Novak, J. M., and Johnson, M. G. (2018). A rapid-test for screening biochar effects on seed germination. Commun. Soil Sci. Plant Anal. 49, 2025–2041. doi:10.1080/00103624.2018.1495726
Osman, A. I., Fawzy, S., Farghali, M., El-Azazy, M., Elgarahy, A. M., Fahim, R. A., et al. (2022). Biochar for agronomy, animal farming, anaerobic digestion, composting, water treatment, soil remediation, construction, energy storage, and carbon sequestration: A review. Environ. Chem. Lett. 20, 2385–2485. doi:10.1007/s10311-022-01424-x
Ouyang, L., Tang, Q., Yu, L., and Zhang, R. (2014). Effects of amendment of different biochars on soil enzyme activities related to carbon mineralisation. Soil Res. 52, 706–716. doi:10.1071/sr14075
Padhye, L. P. (2017). Influence of surface chemistry of carbon materials on their interactions with inorganic nitrogen contaminants in soil and water. Chemosphere 184, 532–547. doi:10.1016/j.chemosphere.2017.06.021
Palansooriya, K. N., Shaheen, S. M., Chen, S. S., Tsang, D. C., Hashimoto, Y., Hou, D., et al. (2020). Soil amendments for immobilization of potentially toxic elements in contaminated soils: A critical review. Environ. Int. 134, 105046. doi:10.1016/j.envint.2019.105046
Palansooriya, K. N., Wong, J. T. F., Hashimoto, Y., Huang, L., Rinklebe, J., Chang, S. X., et al. (2019). Response of microbial communities to biochar-amended soils: A critical review. Biochar 1, 3–22. doi:10.1007/s42773-019-00009-2
Pan, L., Mao, L., Zhang, H., Wang, P., Wu, C., Xie, J., et al. (2022). Modified biochar as a more promising amendment agent for remediation of pesticide-contaminated soils: Modification methods, mechanisms, applications, and future perspectives. Appl. Sci. 12, 11544. doi:10.3390/app122211544
Pankaj, U., and Pandey, V. C. (Editors) (2022). “Microbial based land restoration handbook,” Plant-microbial interaction and soil remediation (Florida, United States: CRC Press), 1.
Partey, S. T., Saito, K., Preziosi, R. F., and Robson, G. D. (2016). Biochar use in a legume–rice rotation system: Effects on soil fertility and crop performance. Arch. Agron. Soil Sci. 62, 199–215. doi:10.1080/03650340.2015.1040399
Parvin, B. A., Sujatha, K., Geetha, R., and Kannan, P. (2022). Biochar coating and its effect on seed quality and biochemical parameters in black gram (Vigna mungo L.) var. VBN 11. Pharma Innov. 11, 2836–2840.
Pascual, M. B., Sanchez-Monedero, M. A., Chacon, F. J., Sanchez-Garcia, M., and Cayuela, M. L. (2020). Linking biochars properties to their capacity to modify aerobic CH4 oxidation in an upland agricultural soil. Geoderma 363, 114179. doi:10.1016/j.geoderma.2020.114179
Paz-Ferreiro, J., Lu, H., Fu, S., Mendez, A., and Gasco, G. (2014). Use of phytoremediation and biochar to remediate heavy metal polluted soils: A review. Solid earth. 5, 65–75. doi:10.5194/se-5-65-2014
Paz-Ferreiro, J., and Fu, S. (2016). Biological indices for soil quality evaluation: Perspectives and limitations. Land Degrad. Dev. 27, 14–25. doi:10.1002/ldr.2262
Pokharel, P., Ma, Z., and Chang, S. X. (2020). Biochar increases soil microbial biomass with changes in extra-and intracellular enzyme activities: A global meta-analysis. Biochar 2, 65–79. doi:10.1007/s42773-020-00039-1
Pratwi, E. P. A., and Shinogi, Y. (2016). Rice husk biochar application to paddy soil and its effects on soil physical properties, plant growth, and methane emission. Paddy Water Environ 14, 521–532. doi:10.1007/s10333-015-0521-z
Pressler, Y., Foster, E. J., Moore, J. C., and Cotrufo, M. F. (2017). Coupled biochar amendment and limited irrigation strategies do not affect a degraded soil food web in a maize agroecosystem, compared to the native grassland. GCB Bioenerg. 9, 1344–1355. doi:10.1111/gcbb.12429
Qin, X., Li, Y., Wang, H., Liu, C., Li, J., Wan, Y., et al. (2016). Long-term effect of biochar application on yield-scaled greenhouse gas emissions in a rice paddy cropping system: A four-year case study in south China. Sci. Total Environ. 569, 1390–1401. doi:10.1016/j.scitotenv.2016.06.222
Rady, M. M., Semida, W. M., Hemida, K. A., and Abdelhamid, M. T. (2016). The effect of compost on growth and yield of Phaseolus vulgaris plants grown under saline soil. Int. J. Recycl. Org. Waste Agric. 5, 311–321. doi:10.1007/s40093-016-0141-7
Rasa, K., Heikkinen, J., Hannula, M., Arstila, K., Kulju, S., and Hyvaluoma, J. (2018). How and why does willow biochar increase a clay soil water retention capacity? Biomass Bioenerg. 119, 346–353. doi:10.1016/j.biombioe.2018.10.004
Reynolds, A., Joseph, S. D., Verheyen, T. V., Chinu, K., Taherymoosavi, S., Munroe, P. R., et al. (2018). Effect of clay and iron sulphate on volatile and water-extractable organic compounds in bamboo biochars. J. Anal. Appl. Pyrol 133, 22–29. doi:10.1016/j.jaap.2018.05.007
Rivelli, A. R., and Libutti, A. (2022). Effect of biochar and inorganic or organic fertilizer Co-application on soil properties, plant growth and nutrient content in Swiss chard. Agron 12, 2089. doi:10.3390/agronomy12092089
Roberts, D. A., Paul, N. A., Dworjanyn, S. A., Bird, M. I., and de Nys, R. (2015). Biochar from commercially cultivated seaweed for soil amelioration. Sci. Rep. 5, 9665–9666. doi:10.1038/srep09665
Rondon, M. A., Lehmann, J., Ramırez, J., and Hurtado, M. (2007). Biological nitrogen fixation by common beans (Phaseolus vulgaris L.) increases with bio-char additions. Biol. Fertil. Soils 43, 699–708. doi:10.1007/s00374-006-0152-z
Rondon-Quintana, H. A., Reyes-Lizcano, F. A., Chaves-Pabon, S. B., Bastidas-Martinez, J. G., and Zafra-Mejia, C. A. (2022). Use of biochar in asphalts. Sustain 14 (8), 4745.
Rose, T. J., Schefe, C., Weng, Z. H., Rose, M. T., van Zwieten, L., Liu, L., et al. (2019). Phosphorus speciation and bioavailability in diverse biochars. Plant soil 443 (1-2), 233–244. doi:10.1007/s11104-019-04219-2
Sakhiya, A. K., Anand, A., Aier, I., Vijay, V. K., and Kaushal, P. (2020). Suitability of rice straw for biochar production through slow pyrolysis: Product characterization and thermodynamic analysis. Bioresour. Technol. Rep. 15, 100818. doi:10.1016/j.biteb.2021.100818
Sangeetha, J., Soytong, K., Thangadurai, D., and Al-Tawaha, A. R. M. (Editors) (2022). Organic farming for sustainable development (Florida, United States: CRC Press).
Sanger, A., Reibe, K., Mumme, J., Kaupenjohann, M., Ellmer, F., Roβ, C. L., et al. (2017). Biochar application to sandy soil: Effects of different biochars and N fertilization on crop yields in a 3-year field experiment. Arch. Agron. Soil Sci. 63, 213–229. doi:10.1080/03650340.2016.1223289
Sattar, A., Sher, A., Ijaz, M., Irfan, M., Butt, M., Abbas, T., et al. (2019). Biochar application improves the drought tolerance in maize seedlings. Phyt. B. Aires. 88, 379–388. doi:10.32604/phyton.2019.04784
Schmidt, H., Kammann, C., Hagemann, N., Leifeld, J., Bucheli, T. D., Sanchez Monedero, M. A., et al. (2021). Biochar in agriculture – a systematic review of 26 global meta-analyses. GCB Bioenerg. 13, 1708–1730. doi:10.1111/gcbb.12889
Schubert, D. C., Chuppava, B., Witte, F., Terjung, N., and Visscher, C. (2021). Effect of two different biochars as a component of compound feed on nutrient digestibility and performance parameters in growing pigs. Front. Anim. Sci. 2, 633958. doi:10.3389/fanim.2021.633958
Seehausen, M., Gale, N., Dranga, S., Hudson, V., Liu, N., Michener, J., et al. (2017). Is there a positive synergistic effect of biochar and compost soil amendments on plant growth and physiological performance? Agron 7, 13. doi:10.3390/agronomy7010013
Seitz, S., Teuber, S., Geißler, C., Goebes, P., and Scholten, T. (2020). How do newly-amended biochar particles affect erodibility and soil water movement?-a small-scale experimental approach. Soil Syst. 4, 60. doi:10.3390/soilsystems4040060
Semida, W. M., Abd El-Mageed, T. A., Howladar, S. M., Mohamed, G. F., and Rady, M. M. (2015). Response of Solanum melongena L. seedlings grown under saline calcareous soil conditions to a new organo-mineral fertilizer. J. Anim. Plant Sci. 25, 485–493.
Semida, W. M., Beheiry, H. R., Setamou, M., Simpson, C. R., Abd El-Mageed, T. A., Rady, M. M., et al. (2019). Biochar implications for sustainable agriculture and environment: A review. South Afr. J. Bot. 127, 333–347. doi:10.1016/j.sajb.2019.11.015
Shahab, A., Qi, S., Zaheer, M., Rashid, A., Talib, M. A., and Ashraf, U. (2018). Hydrochemical characteristic and water quality assessment for drinking and agricultural purposes in District Jacobabad, Lower Indus Plain, Pakistan. Int. J. Agric. Biol Engin 11 (2), 115–121.
Shamim, M., Saha, N., and Hye, F. B.Department of Forestry and Environmental Science, School of Agriculture and Mineral Sciences, Shahjalal University of Science and Technology, Sylhet-3114, BangladeshDepartment of Forestry and Environmental Science, School of Agriculture and Mineral Sciences, Shahjalal University of Science and Technology, Sylhet-3114, Bangladesh (2018). Effect of biochar on seed germination, early growth of Oryza sativa L. and soil nutrients. Trop. Plant Res. 5, 336–342. doi:10.22271/tpr.2018.v5.i3.042
Shenbagavalli, S., and Mahimairaja, S. (2012). Characterization and effect of biochar on nitrogen and carbon dynamics in soil. Int. J. Adv. Res. 2, 249–255.
Shetty, R., Vidya, C. S. N., Prakash, N. B., Lux, A., and Vaculík, M. (2020). Aluminum toxicity in plants and its possible mitigation in acid soils by biochar: A review. Sci. Total Environ. 765, 142744. doi:10.1016/j.scitotenv.2020.142744
Si, L., Xie, Y., Ma, Q., and Wu, L. (2018). The short-term effects of rice straw biochar, nitrogen and phosphorus fertilizer on rice yield and soil properties in a cold waterlogged paddy field. Sustain 10, 537. doi:10.3390/su10020537
Sigua, G. C., Novak, J. M., Watts, D. W., Johnson, M. G., and Spokas, K. (2015). Efficacies of designer biochars in improving biomass and nutrient uptake of winter wheat grown in a hard setting subsoil layer. Chemosphere 142, 176–183. doi:10.1016/j.chemosphere.2015.06.015
Simiele, M., Argentino, O., Baronti, S., Scippa, G. S., Chiatante, D., Terzaghi, M., et al. (2022). Biochar enhances plant growth, fruit yield, and antioxidant content of cherry tomato (Solanum lycopersicum L.) in a soilless substrate. Agriculture 12, 1135. doi:10.3390/agriculture12081135
Singh, H., Northup, B. K., Rice, C. W., and Prasad, P. V. V. (2022b). Biochar applications influence soil physical and chemical properties, microbial diversity, and crop productivity: A meta-analysis. Biochar 4, 8. doi:10.1007/s42773-022-00138-1
Singh, K. (2016). Microbial and enzyme activities of saline and sodic Soils. Land Degrad. Dev. 27, 706–718. doi:10.1002/ldr.2385
Singh, R., Kumari, T., Verma, P., Singh, B. P., and Raghubanshi, A. S. (2022a). Compatible package-based agriculture systems: An urgent need for agro-ecological balance and climate change adaptation. Soil Ecol. Lett. 4 (3), 187–212. doi:10.1007/s42832-021-0087-1
Singh, R., Singh, P., Singh, H., and Raghubanshi, A. S. (2019). Impact of sole and combined application of biochar, organic and chemical fertilizers on wheat crop yield and water productivity in a dry tropical agro-ecosystem. Biochar 1 (2), 229–235. doi:10.1007/s42773-019-00013-6
Singh, R., Srivastava, P., Bhadouria, R., Yadav, A., Singh, H., and Raghubanshi, A. S. (2020). Combined application of biochar and farmyard manure reduces wheat crop eco-physiological performance in a tropical dryland agro-ecosystem. Energy Ecol. Environ. 5, 171–183. doi:10.1007/s40974-020-00159-1
Sohi, S. P., Krull, E., Lopez-Capel, E., and Bol, R. (2010). A review of biochar and its use and function in soil. Adv. Agron. 105, 47–82.
Solaiman, Z. M., Blackwell, P., Abbott, L. K., and Storer, P. (2010). Direct and residual effect of biochar application on mycorrhizal root colonisation, growth and nutrition of wheat. Soil Res. 48, 546–554. doi:10.1071/sr10002
Solaiman, Z. M., Murphy, D. V., and Abbott, L. K. (2012). Biochars influence seed germination and early growth of seedlings. Plant Soil 353, 273–287. doi:10.1007/s11104-011-1031-4
Song, B., Almatrafi, E., Tan, X., Luo, S., Xiong, W., Zhou, C., et al. (2022). Biochar-based agricultural soil management: An application-dependent strategy for contributing to carbon neutrality. Renew. Sustain. Energy Rev. 164, 112529. doi:10.1016/j.rser.2022.112529
Spokas, K. A., and Reicosky, D. C. (2009). Impacts of sixteen different biochars on soil greenhouse gas production. Ann. Environ. Sci. 3, 179–193.
Subedi, R., Taupe, N., Ikoyi, I., Bertora, C., Zavattaro, L., Schmalenberger, A., et al. (2016). Chemically and biologically-mediated fertilizing value of manure-derived biochar. Sci. Total Environ. 550, 924–933. doi:10.1016/j.scitotenv.2016.01.160
Sun, F., and Lu, S. (2014). Biochars improve aggregate stability, water retention, and pore-space properties of clayey soil. J. Plant Nutr. Soil Sci. 177, 26–33. doi:10.1002/jpln.201200639
Sun, J., Drosos, M., Mazzei, P., Savy, D., Todisco, D., Vinci, G., et al. (2017). The molecular properties of biochar carbon released in dilute acidic solution and its effects on maize seed germination. Sci. Total Environ. 576, 858–867. doi:10.1016/j.scitotenv.2016.10.095
Tammeorg, P., Simojoki, A., Meakelea, P., Stoddard, F. L., Alakukku, L., and Helenius, J. (2014). Biochar application to a fertile sandy clay loam in boreal conditions: Effects on soil properties and yield formation of wheat, turnip rape and faba bean. Plant Soil 374, 89–107. doi:10.1007/s11104-013-1851-5
Tan, S., Narayanan, M., Huong, D. T. T., Ito, N., Unpaprom, Y., Pugazhendhi, A., et al. (2022). A perspective on the interaction between biochar and soil microbes: A way to regain soil eminence. Environ. Res. 214, 113832. doi:10.1016/j.envres.2022.113832
Tang, H., Chen, M., Wu, P., Faheem, M., Feng, Q., Lee, X., et al. (2022). Engineered biochar effects on soil physicochemical properties and biota communities: A critical review. Chemosphere 311, 137025. doi:10.1016/j.chemosphere.2022.137025
Tangahu, B. V., Sheikh-Abdullah, S. R., Basri, H., Idris, M., Anuar, N., and Mukhlisin, M. (2011). A review on heavy metals (As, Pb, and Hg) uptake by plants through phytoremediation. Int. J. Chem. Eng. 2011, 1–31. doi:10.1155/2011/939161
Tanveer, K., Ilyas, N., Akhtar, N., Yasmin, H., Hefft, D. I., El-Sheikh, M. A., et al. (2022). Role of biochar and compost in cadmium immobilization and on the growth of Spinacia. PLoS ONE 17, e0263289. doi:10.1371/journal.pone.0263289
Tartaglia, M., Arena, S., Scaloni, A., Marra, M., and Rocco, M. (2020). Biochar administration to san Marzano tomato plants cultivated under low-input farming increases growth, fruit yield, and affects gene expression. Front. Plant Sci. 11, 1281. doi:10.3389/fpls.2020.01281
Taskin, M. B., Kadioglu, Y. K., Sahin, O., Inal, A., and Gunes, A. (2019). Effect of acid modified biochar on the growth and essential and non-essential element content of bean, chickpea, soybean, and maize grown in calcareous soil. Communi Soil Sci. Plant Analy 50 (13), 1604–1613. doi:10.1080/00103624.2019.1631326
Thomas, S. C. (2021). Biochar effects on germination and radicle extension in temperate tree seedlings under field conditions. Can. J. For. Res. 51, 10–17. doi:10.1139/cjfr-2019-0386
Tian, J. H., Shuang, R. A. O., Yang, G. A. O., Yang, L. U., and Cai, K. Z. (2021). Wheat straw biochar amendment suppresses tomato bacterial wilt caused by Ralstonia solanacearum: Potential effects of rhizosphere organic acids and amino acids. J. Integr. Agric. 20, 2450–2462. doi:10.1016/s2095-3119(20)63455-4
Timmis, K., and Ramos, J. L. (2021). The soil crisis: The need to treat as a global health problem and the pivotal role of microbes in prophylaxis and therapy. Microb. Biotechnol. 14 (3), 769–797. doi:10.1111/1751-7915.13771
Tisserant, A., and Cherubini, F. (2019). Potentials, limitations, Co-benefits, and trade-offs of biochar applications to soils for climate change mitigation. Land 8, 179. doi:10.3390/land8120179
Tomczyk, A., Sokolowska, Z., and Boguta, P. (2020). Biochar physicochemical properties: Pyrolysis temperature and feedstock kind effects. Rev. Env. Sci. Biotechnol. 19, 191–215. doi:10.1007/s11157-020-09523-3
Tran, T. A., and Popova, L. P. (2013). Functions and toxicity of cadmium in plants: Recent advances and future prospects. Turk. J. Bot. 37, 1–13.
Trupiano, D., Cocozza, C., Baronti, S., Amendola, C., Vaccari, F. P., Lustrato, G., et al. (2017). The effects of biochar and its combination with compost on lettuce (Lactuca sativa L.) growth, soil properties, and soil microbial activity and abundance. Int. J. Agron. 2017, 1–12. doi:10.1155/2017/3158207
Uday, V., Harikrishnan, P. S., Deoli, K., Mahlknecht, J., and Kumar, M. (2022). Current trends in production, morphology, and real-world environmental applications of biochar for the promotion of sustainability. Bioresour. Technol. 127467, 127467. doi:10.1016/j.biortech.2022.127467
Uslu, O. S., Babur, E., Alma, M. H., and Solaiman, Z. M. (2020). Walnut shell biochar increases seed germination and early growth of seedlings of fodder crops. Agriculture 10, 427. doi:10.3390/agriculture10100427
Usman, A. R. A., Al-Wabel, M. I., Ok, Y. S., Al-Harbi, A., Wahb-Allah, M., El- Naggar, A. H., et al. (2016). Conocarpus biochar induces changes in soil nutrient availability and tomato growth under saline irrigation. Pedosphere 26, 27–38. doi:10.1016/s1002-0160(15)60019-4
Uzoma, K. C., Inoue, M., Andry, H., Fujimaki, H., Zahoor, A., and Nishihara, E. (2011). Effect of cow manure biochar on maize productivity under sandy soil condition. Soil Use Manag. 27, 205–212. doi:10.1111/j.1475-2743.2011.00340.x
Vaccari, F. P., Baronti, S., Lugato, E., Genesio, L., Castaldi, S., Fornasier, F., et al. (2011). Biochar as a strategy to sequester carbon and increase yield in durum wheat. Eur. J. Agron. 34, 231–238. doi:10.1016/j.eja.2011.01.006
Vaccari, F. P., Maienza, A., Miglietta, F., Baronti, S., Di Lonardo, S., Giagnoni, L., et al. (2015). Biochar stimulates plant growth but not fruit yield of processing tomato in a fertile soil. Agric. Ecosyst. Environ. 207, 163–170. doi:10.1016/j.agee.2015.04.015
Van Zwieten, L., Kimber, S., Morris, S., Chan, K., Downie, A., Rust, J., et al. (2010). Effects of biochar from slow pyrolysis of papermill waste on agronomic performance and soil fertility. Plant Soil 327, 235–246. doi:10.1007/s11104-009-0050-x
Visconti, D., Álvarez-Robles, M. J., Fiorentino, N., Fagnano, M., and Clemente, R. (2020). Use of Brassica juncea and Dactylis glomerata for the phytostabilization of mine soils amended with compost or phytostabilization of mine soils amended with compost or biochar. Chemosphere 260, 127661. doi:10.1016/j.chemosphere.2020.127661
Vithanage, M., Herath, I., Joseph, S., Bundschuh, J., Bolan, N., Ok, Y. S., et al. (2017). Interaction of arsenic with biochar in soil and water: A critical review. Carbon 113, 219–230. doi:10.1016/j.carbon.2016.11.032
Wang, G., Ma, Y., Chenia, H. Y., Govinden, R., Luo, J., and Ren, G. (2020b). Biochar-mediated control of phytophthora blight of pepper is closely related to the improvement of the rhizosphere fungal community. Front. Microbiol. 11, 1427. doi:10.3389/fmicb.2020.01427
Wang, L., Huang, J., Li, G., Luo, J., Bolan, N. S., and Hou, D. (2022). Long-term immobilization of soil metalloids under simulated aging: Experimental and modeling approach. Sci. Total Environ. 806, 150501. doi:10.1016/j.scitotenv.2021.150501
Wang, L., O'Connor, D., Rinklebe, J., Ok, Y. S., Tsang, D. C. W., Shen, Z., et al. (2020a). Biochar aging: Mechanisms, physicochemical changes, assessment, and implications for field applications. Environ. Sci. Technol. 54, 14797–14814. doi:10.1021/acs.est.0c04033
Wang, S. J., Zheng, J. L., Wang, Y. J., Yang, Q. F., Chen, T. T., Chen, Y. L., et al. (2021a). Photosynthesis, chlorophyll fluorescence, and yield of peanut in response to biochar application. Front. Plant Sci. 12, 650432. doi:10.3389/fpls.2021.650432
Wang, X., Song, D., Liang, G., Zhang, Q., Ai, C., and Zhou, W. (2015). Maize biochar addition rate influences soil enzyme activity and microbial community composition in a fluvo-aquic soil. Appl. Soil Ecol. 96, 265–272. doi:10.1016/j.apsoil.2015.08.018
Wang, Y., Ma, Z., Wang, X., Sun, Q., Dong, H., Wang, G., et al. (2019a). Effects of biochar on the growth of apple seedlings, soil enzyme activities and fungal communities in replant disease soil. Sci. Hortic. 256, 108641. doi:10.1016/j.scienta.2019.108641
Wang, Y., Ren, Q., Li, T., Zhan, W., Zheng, K., Liu, Y., et al. (2021b). Influences of modified biochar on metal bioavailability, metal uptake by wheat seedlings (Triticum aestivum L.) and the soil bacterial community. Environ. Saf. 220, 112370. doi:10.1016/j.ecoenv.2021.112370
Wang, Y. Y., Ji, H. Y., Lyu, H. H., Liu, Y. X., He, L. L., You, L. C., et al. (2019b). Simultaneous alleviation of Sb and Cd availability in contaminated soil and accumulation in Lolium multiflorum Lam. After amendment with Fe–Mn-Modified biochar. J. Clean. Prod. 231, 556–564. doi:10.1016/j.jclepro.2019.04.407
Whitman, T., Whitman, E., Woolet, J., Flannigan, M. D., Thompson, D. K., and Parisien, M. A. (2019). Soil bacterial and fungal response to wildfires in the Canadian boreal forest across a burn severity gradient. Soil Biol. biochem. 138, 107571. doi:10.1016/j.soilbio.2019.107571
Willson, N. L., Van, T. T. H., Bhattarai, S. P., Courtice, J. M., McIntyre, J. R., Prasai, T. P., et al. (2019). Feed supplementation with biochar may reduce poultry pathogens, including Campylobacter hepaticus, the causative agent of spotty liver disease. PLoS ONE 14, e0214471. doi:10.1371/journal.pone.0214471
Wu, C., Shi, L., Xue, S., Li, W., Jiang, X., Rajendran, M., et al. (2019). Effect of sulfur-iron modified biochar on the available cadmium and bacterial community structure in contaminated soils. Sci. Total Environ. 647, 1158–1168. doi:10.1016/j.scitotenv.2018.08.087
Wu, H., Wu, H., Jiao, Y., Zhang, Z., Rensing, C., and Lin, W. (2022). The combination of biochar and PGPBs stimulates the differentiation in rhizosphere soil microbiome and metabolites to suppress soil-borne pathogens under consecutive monoculture regimes. GCB Bioenerg. 14, 84–103. doi:10.1111/gcbb.12906
Wu, J., Li, Z., Huang, D., Liu, X., Tang, C., Parikh, S. J., et al. (2020). A novel calcium-based magnetic biochar is effective in stabilization of arsenic and cadmium co-contamination in aerobic soils. J. Hazard. Mat. 387, 122010. doi:10.1016/j.jhazmat.2019.122010
Xi, J., Li, H., Xi, J., Tan, S., Zheng, J., and Tan, Z. (2020). Effect of returning biochar from different pyrolysis temperatures and atmospheres on the growth of leaf-used lettuce. Environ. Sci. Pollut. Res. 27, 35802–35813. doi:10.1007/s11356-020-09840-8
Xiao, X., Chen, B., Chen, Z., Zhu, L., and Schnoor, J. L. (2018). Insight into multiple and multilevel structures of biochars and their potential environmental applications: A critical review. Environ. Sci. Technol. 52 (9), 5027–5047. doi:10.1021/acs.est.7b06487
Xinghui, W. U., Baoling, S. U., Lin, Q. I., Wang, Y., Ma, Y., and Zhou, L. (2020). Effects of biochar on seed germination and seedling growth of Betula platyphylla. For. Resour. Manage. 6, 128–134.
Xiong, J., Yu, R., Islam, E., Zhu, F., Zha, J., and Sohail, M. I. (2020). Effect of biochar on soil temperature under high soil surface temperature in coal mined arid and semiarid regions. Sustain 12, 8238. doi:10.3390/su12198238
Xu, G., Wei, L. L., Sun, J. N., Shao, H. B., and Chang, S. X. (2013). What is more important for enhancing nutrient bioavailability with biochar application into a sandy soil: Direct or indirect mechanism? Ecol. Eng. 52, 119–124. doi:10.1016/j.ecoleng.2012.12.091
Xu, G., Zhang, Y., Sun, J., and Shao, H. (2016). Negative interactive effects between biochar and phosphorus fertilization on phosphorus availability and plant yield in saline sodic soil. Sci. Total Environ. 568, 910–915. doi:10.1016/j.scitotenv.2016.06.079
Xu, W., Whitman, W. B., Gundale, M. J., Chien, C., and Chiu, C. (2021). Functional response of the soil microbial community to biochar applications. GCB Bioenerg. 13, 269–281. doi:10.1111/gcbb.12773
Xu, Z., Xu, X., Tao, X., Yao, C., Tsang, D. C., and Cao, X. (2019). Interaction with low molecular weight organic acids affects the electron shuttling of biochar for Cr (VI) reduction. J. Hazard. Mat. 378, 120705. doi:10.1016/j.jhazmat.2019.05.098
Yamato, M., Okimori, Y., Wibowo, I. F., Anshori, S., and Ogawa, M. (2006). Effects of the application of charred bark of Acacia mangium on the yield of maize, cowpea and peanut, and soil chemical properties in South Sumatra, Indonesia. Soil Sci. Plant Nutr. 52, 489–495. doi:10.1111/j.1747-0765.2006.00065.x
Yang, C. D., and Lu, S. G. (2020). Dynamic effects of direct returning of straw and corresponding biochar on acidity, nutrients, and exchangeable properties of red soil. Huan Jing Ke Xue 41, 4246–4252. doi:10.13227/j.hjkx.202002213
Yang, C., Liu, J., and Lu, S. (2021a). Pyrolysis temperature affects pore characteristics of rice straw and canola stalk biochars and biochar-amended soils. Geoderma 397, 115097. doi:10.1016/j.geoderma.2021.115097
Yang, Q., Masek, O., Zhao, L., Nan, H., Yu, S., Yin, J., et al. (2021b). Country-level potential of carbon sequestration and environmental benefits by utilizing crop residues for biochar implementation. Appl. Energy 282, 116275. doi:10.1016/j.apenergy.2020.116275
Yang, Y., Chen, T., Xiao, R., Chen, X., and Zhang, T. (2022). A quantitative evaluation of the biochar’s influence on plant disease suppress: A global meta-analysis. Biochar 4, 43. doi:10.1007/s42773-022-00164-z
Yao, Q., Liu, J., Yu, Z., Li, Y., Jin, J., Liu, X., et al. (2017). Three years of biochar amendment alters soil physiochemical properties and fungal community composition in a black soil of Northeast China. Soil Biol. biochem. 110, 56–67. doi:10.1016/j.soilbio.2017.03.005
Ye, S., Zeng, G., Wu, H., Zhang, C., Dai, J., Liang, J., et al. (2017). Biological technologies for the remediation of co-contaminated soil. Crit. Rev. Biotechnol. 37, 1062–1076. doi:10.1080/07388551.2017.1304357
Yi, Y., Huang, Z., Lu, B., Xian, J., Tsang, E. P., Cheng, W., et al. (2020). Magnetic biochar for environmental remediation: A review. Bioresour. Technol. 298, 122468. doi:10.1016/j.biortech.2019.122468
Yin, D., Li, H., Wang, H., Guo, X., Wang, Z., Lv, Y., et al. (2021a). Impact of different biochars on microbial community structure in the rhizospheric soil of rice grown in albic soil. Molecules 26, 4783. doi:10.3390/molecules26164783
Yin, X., Penuelas, J., Sardans, J., Xu, X., Chen, Y., Fang, Y., et al. (2021c). Effects of nitrogen-enriched biochar on rice growth and yield, iron dynamics, and soil carbon storage and emissions: A tool to improve sustainable rice cultivation. Environ. Pollut. 287, 117565. doi:10.1016/j.envpol.2021.117565
Yin, Y. F., He, X. H., Gao, R., Ma, H. L., and Yang, Y. S. (2014). Effects of rice straw and its biochar addition on soil labile carbon and soil organic carbon. J. Int. Agric. 13, 491–498. doi:10.1016/s2095-3119(13)60704-2
Yin, Y., Yang, C., Li, M., Zheng, Y., Ge, C., Gu, J., et al. (2021b). Research progress and prospects for using biochar to mitigate greenhouse gas emissions during composting: A review. Sci. Total Environ. 798, 149294. doi:10.1016/j.scitotenv.2021.149294
Younis, U., Athar, M., Malik, S. A., Shah, M. H. R., and Mahmood, S. (2015). Biochar impact on physiological and biochemical attributes of spinach (Spinacia oleracea L.) in nickel contaminated soil. Glob. J. Environ. Sci. Manag. 1, 245–254.
Yu, H., Zou, W., Chen, J., Chen, H., Yu, Z., Huang, J., et al. (2019). Biochar amendment improves crop production in problem soils: A review. J. Environ. Manage. 232, 8–21. doi:10.1016/j.jenvman.2018.10.117
Yue, L., Lian, F., Han, Y., Bao, Q., Wang, Z., and Xing, B. (2019). The effect of biochar nanoparticles on rice plant growth and the uptake of heavy metals: Implications for agronomic benefits and potential risk. Sci. Total Environ. 656, 9–18. doi:10.1016/j.scitotenv.2018.11.364
Yue, Y., Cui, L., Lin, Q., Li, G., and Zhao, X. (2017). Efficiency of sewage sludge biochar in improving urban soil properties and promoting grass growth. Chemosphere 173, 551–556. doi:10.1016/j.chemosphere.2017.01.096
Zhai, L., CaiJi, Z., Liu, J., Wang, H., Ren, T., Gai, X., et al. (2015). Short-term effects of maize residue biochar on phosphorus availability in two soils with different phosphorus sorption capacities. Biol. Fertil. Soils 51, 113–122. doi:10.1007/s00374-014-0954-3
Zhang, A., Cui, L., Pan, G., Li, L., Hussain, Q., Zhang, X., et al. (2010a). Effect of biochar amendment on yield and methane and nitrous oxide emissions from a rice paddy from Tai Lake plain, China. Agric. Ecosyst. Environ. 139, 469–475. doi:10.1016/j.agee.2010.09.003
Zhang, D., Pan, G., Wu, G., Kibue, G. W., Li, L., Zhang, X., et al. (2015a). Biochar helps enhance maize productivity and reduce greenhouse gas emissions under balanced fertilization in a rainfed low fertility inceptisol. Chemosphere 142, 106–113. doi:10.1016/j.chemosphere.2015.04.088
Zhang, H., Lin, K., Wang, H., and Gan, J. (2010b). Effect of Pinus radiata derived biochars on soil sorption and desorption of phenanthrene. Environ. Pollut. 158, 2821–2825. doi:10.1016/j.envpol.2010.06.025
Zhang, H., Marchant-Forde, J. N., Zhang, X., and Wang, Y. (2020a). Effect of cornstalk biochar immobilized bacteria on ammonia reduction in laying hen manure composting. Molecules 25, 1560. doi:10.3390/molecules25071560
Zhang, H., Voroney, R. P., and Price, G. W. (2015b). Effects of temperature and processing conditions on biochar chemical properties and their influence on soil C and N transformations. Soil Biol. biochem. 83, 19–28. doi:10.1016/j.soilbio.2015.01.006
Zhang, K., Wang, Y., Mao, J., and Chen, B. (2019). Effects of biochar nanoparticles on seed germination and seedling growth. Environ. Pollut. 256, 113409. doi:10.1016/j.envpol.2019.113409
Zhang, M., Liu, Y., Wei, Q., Liu, L., Gu, X., and Gou, J. (2022). Biochar-based fertilizer enhances the production capacity and economic benefit of open-field eggplant in the karst region of southwest China. Agriculture 12 (9), 1388. doi:10.3390/agriculture12091388
Zhang, Y., Chen, Z., Chen, C., Li, F., and Shen, K. (2021). Effects of UV-modified biochar derived from phytoremediation residue on Cd bioavailability and uptake in Coriandrum sativum L. in a Cd-contaminated soil. Environ. Sci. Pollut. Res. 28, 17395–17404. doi:10.1007/s11356-020-11931-5
Zhang, Z., Dong, X., Wang, S., and Pu, X. (2020b). Benefits of organic manure combined with biochar amendments to cotton root growth and yield under continuous cropping systems in Xinjiang, China. Sci. Rep. 10, 1–10. doi:10.1038/s41598-020-61118-8
Zhao, Z., Shi, F., and Guan, F. (2022). Effects of plastic mulching on soil CO2 efflux in a cotton field in northwestern China. Sci. Rep. 12, 4969. doi:10.1038/s41598-022-08793-x
Zheng, J., Han, J., Liu, Z., Xia, W., Zhang, X., Li, L., et al. (2017). Biochar compound fertilizer increases nitrogen productivity and economic benefits but decreases carbon emission of maize production. Agric. Ecosyst. Environ. 241, 70–78. doi:10.1016/j.agee.2017.02.034
Zhou, C., Heal, K., Tigabu, M., Xia, L., Hu, H., Yin, D., et al. (2020). Biochar addition to forest plantation soil enhances phosphorus availability and soil bacterial community diversity. For. Ecol. Manage. 455, 117635. doi:10.1016/j.foreco.2019.117635
Zhou, W., Zhang, Y., Zhong, K., Xiong, R., Long, P., Xu, Y., et al. (2022). Ferrate-modified biochar for greenhouse gas mitigation: First-principles calculation and paddy field trails. Agron 12, 2661. doi:10.3390/agronomy12112661
Zhu, X., Chen, B., Zhu, L., and Xing, B. (2017). Effects and mechanisms of biochar-microbe interactions in soil improvement and pollution remediation: A review. Environ. Pollut. 227, 98–115. doi:10.1016/j.envpol.2017.04.032
Zhu, Y., Wang, H., Lv, X., Zhang, Y., and Wang, W. (2020). Effects of biochar and biofertilizer on cadmium-contaminated cotton growth and the antioxidative defense system. Sci. Rep. 10, 20112–12. doi:10.1038/s41598-020-77142-7
Zimmermann, M., Bird, M. I., Wurster, C., Saiz, G., Goodrick, I., Barta, J., et al. (2012). Rapid degradation of pyrogenic carbon. Glob. Chang. Biol. 18, 3306–3316. doi:10.1111/j.1365-2486.2012.02796.x
Zornoza, R., Moreno-Barriga, F., Acosta, J. A., Munoz, M. A., and Faz, A. (2016). Stability, nutrient availability and hydrophobicity of biochars derived from manure, crop residues, and municipal solid waste for their use as soil amendments. Chemosphere 144, 122–130. doi:10.1016/j.chemosphere.2015.08.046
Zou, Q., An, W., Wu, C., Li, W., Fu, A., Xiao, R., et al. (2018). Red mud-modified biochar reduces soil arsenic availability and changes bacterial composition. Environ. Chem. Lett. 16, 615–622. doi:10.1007/s10311-017-0688-1
Keywords: agricultural sustainability, biochar, crop yield, food security, soil ameliorator, carbon sequestration
Citation: Murtaza G, Ahmed Z, Eldin SM, Ali B, Bawazeer S, Usman M, Iqbal R, Neupane D, Ullah A, Khan A, Hassan MU, Ali I and Tariq A (2023) Biochar-Soil-Plant interactions: A cross talk for sustainable agriculture under changing climate. Front. Environ. Sci. 11:1059449. doi: 10.3389/fenvs.2023.1059449
Received: 07 October 2022; Accepted: 04 January 2023;
Published: 14 February 2023.
Edited by:
Lanfang Han, Guangdong University of Technology, ChinaReviewed by:
Jie Jin, North China Electric Power University, ChinaRishikesh Singh, Panjab University, India
Copyright © 2023 Murtaza, Ahmed, Eldin, Ali, Bawazeer, Usman, Iqbal, Neupane, Ullah, Khan, Hassan, Ali and Tariq. This is an open-access article distributed under the terms of the Creative Commons Attribution License (CC BY). The use, distribution or reproduction in other forums is permitted, provided the original author(s) and the copyright owner(s) are credited and that the original publication in this journal is cited, in accordance with accepted academic practice. No use, distribution or reproduction is permitted which does not comply with these terms.
*Correspondence: Zeeshan Ahmed, emVlc2hhbmFncm9ub21pc3RAeWFob28uY29t; Rashid Iqbal, cmFzaGlkLmlxYmFsQGl1Yi5lZHUucGs=; Iftikhar Ali, SWZ0aWtoYXJAZ2VuZXRpY3MuYWMuY24=