- 1Key Laboratory of Eco-Hydrology of Inland River Basin, Northwest Institute of Eco-Environment and Resources, Chinese Academy of Sciences, Lanzhou, China
- 2University of Chinese Academy of Sciences, Beijing, China
- 3Faculty of Resources and Environment, Baotou Teachers’ College, Inner Mongolia University of Science and Technology, Baotou, China
Afforestation is an important and effective way to curb wind-sand hazards in the Alxa Desert. However, over-afforestation makes soil drying occur frequently. The formation of a soil drying layer is seriously restricting the effectiveness of vegetation construction and regional ecological stability. To clarify the process of soil desiccation in Haloxylon ammodendron plantations and determine the suitable planting years, a typical H. ammodendron plantation in this area was selected as the research object, and the soil moisture variation characteristics of this sand-fixing vegetation region with vegetation age were analyzed. The analysis results on soil water supply, consumption, and balance showed that the soil water storage in 0–400 cm soil layer of H. ammodendron plantation varies significantly in different ages. The soil water storage in 0–11 years old was the largest, and the soil water supply was greater than the soil water consumption. The soil water storage in profile increased with the increase of precipitation, the soil water storage of H. ammodendron at 11–22 years old increased first and then decreased with precipitation, and the inflexion existed in 16.5 years old. The soil water consumption of H. ammodendron plantation at 22–46 years old was greater than that of soil water supplement, and the soil moisture appeared negative balance continuously. Therefore, to prevent further deterioration of soil moisture ecological environment in the H. ammodendron plantation and to promote the sustainable development of afforestation in desert areas, thinning measures are suggested when the growth period reaches 16.5 years. The research results could provide scientific basis for afforestation and soil dry layer regulation in the Alxa desert.
1 Introduction
The hazards associated with sandstorms caused by desertification are a global eco-environmental problem. China has launched the world’s largest afforestation programme, which has had remarkable success in combating Northwest China desertification (Suo and Cao, 2021). The area of sand damage in China is up to 1.64 million km2, accounting for 17% of the total area. With an area of 2,70,000 km2, the Alxa desert area is among the areas experiencing the most severe desertification and windblown sand in China and is the main control area of windblown sand. It is also the key area for the construction of non-irrigated vegetation and ecological barriers in northern China. It has been nearly 60 years since artificial vegetation was used to control sand damage in China, and it is also one of the most effective methods for ecological reconstruction and sand damage control in sand areas (Kebin and Kaiguo, 1989; Wang et al., 2017). It has been proven that sand fixation by plants can effectively restrain the development of desertification, reduce the harm of aeolian sand and promote local habitat restoration (Xue and Fang, 2002; Singh et al., 2012). However, whether in eastern China, where rainfall is heavy, or in northwestern China, where rainfall is low, large-scale afforestation can lead to a decline in groundwater levels, soil moisture loss, vegetation degradation and even new desertification, seriously hindering the sustainability of afforestation (Gao and Shao, 2012; Wang et al., 2021). For sustainable development of afforestation, scholars have introduced the concept of vegetation carrying capacity, that is, under a given water condition, the maximum soil moisture that can be carried by the vegetation (Guo, 2021). She et al. (2014) developed a physical process-based model of vegetation carrying capacity to explain the interaction between transpiration and precipitation replenishment of soil water. Xia and Shao (2008) studied the dynamic effects of plant density on soil water in Loess Plateau and found that the optimum planting density is suitable for the vegetation there, providing a theoretical basis for exploring the vegetation carrying capacity in arid areas. Jia et al. (2019) based on the relationship among leaf area index, evapotranspiration and net primary productivity of three kinds of artificial vegetation, the Biome-BGC model was used to study the vegetation carrying capacity of the region, which provided a theoretical basis for afforestation in the Loess Plateau of China. However, the water consumption of vegetation is different in different stages of forest age (Becknell and Powers, 2014), which obviously interferes with the estimation of the vegetation carrying capacity. There are five growth stages of H. ammodendron: young forest, middle-aged forest, near-mature forest, mature forest, and over-mature forest.
H.ammodendron is a shrub or small tree of Chenopodiaceae. It can survive on the desert soil with annual precipitation of 25–200 mm, and needs little water at the early stage of growth. The root length of H. ammodendron is 5–7 times that of the aboveground part. The young H. ammodendron is only 140 mm, but its roots are well developed, which can be more than 10 m at 40 years old (Zhou et al., 2017). It can grow well in soil with salt content of 1%–2%, and the critical range of salt tolerance is 4%–6% (Lue et al., 2022). These characteristics make it a pioneer species of Afforestation, known as the guardian of the desert, which is decisive in the sustainable development of regional ecosystems. However, water is the main limiting factor for its growth and development. Because the afforestation area is usually large and widely distributed, which can’t be irrigated, so only precipitation and groundwater can be used by H. ammodendron, and the large-scale decline of H. ammodendron plantation has occurred in arid areas (Song et al., 2021). Deng et al. (2019) studied the physiological characteristics of H. ammodendron under drought stress and found that it could cope with drought stress by regulating its internal water absorption mechanism, and its strong water retention ability was the key to its survival in drought environment. H. ammodendron shows strong osmotic adjustment ability and high water use efficiency (Gao et al., 2010). Both the scarcity of soil moisture and the increase of groundwater depth are important reasons for the mortality of H. ammodendron trees and overall decline in the vegetation (Hu et al., 2021).
At present, studies on the growth and decay of H. ammodendron mainly focus on plant density, photosynthetic water physiology, and root distribution pattern, and few studies concern on the income-expenditure balance and dynamic of soil water factors of H. ammodendron plantation in arid area. What are the effects of afforestation on soil moisture in desert areas? What is the optimal growth period of sand-fixing vegetation under given water conditions? These are major scientific problems that must be faced when combating desertification. Determining the carrying capacity of vegetation is an important prerequisite for answering these questions. H. ammodendron is an excellent pioneer plant species for windbreaks and sand fixation and has been planted at a large scale in the desert areas of northwest China. The Alxa desert region is an important area for vegetation reconstruction. Artificial vegetation has greatly changed the land use patterns and vegetation characteristics of the region, and the soil hydrological effects that will be produced by artificial vegetation of different ages remains to be studied. Therefore, in this study, we selected the Yabu-lai forest farm in the Alxa desert as our experimental site and analyzed the temporal variation in soil moisture and its profile distribution in H. ammodendron plantations at different ages by using fixed-point and systematic observations for five consecutive age plots in 2021. The effect of forest age on the soil moisture of H. ammodendron plantations was studied to provide a theoretical basis for water resource management and vegetation construction in this region.
2 Materials and methods
2.1 Study sites
Alxa desert (Figure 1) is located in the hinterland of the Asian continent. The region has a large temperature difference between winter and summer and has ample sunshine. Evaporation is intense in this study area, with an average annual evaporation of 3,100 mm. The precipitation decreases from the southeast to the northwest, and evaporation increases from the southeast to the northwest. The annual average temperature is 7.9°C–10°C, and the annual precipitation is 39.3–224.2 mm. The annual frost-free period is 142–174 days. This area receives 2,993–3,345 h of sunshine. The underground water level is approximately 80–120 m. H. ammodendron has developed root system, high survival rate, among which 11-year-old H. ammodendron root system up to 8.4 m (Lu et al., 2021). As a typical desert control area in China, the afforestation of this area has exceeded 65,000 km2, of which H. ammodendron is the main vegetation type of afforestation.
2.2 Field sampling
According to the requirement of consistency between forest age gradient and space environment, using the method of space-time substitution, 5-, 11-, 22-, 34-, and 46-year-old H. ammodendron plantations were selected as representative plots, three 50 m × 50 m plots were designated in each H. ammodendron plantation. In May and September of 2021, samples were taken from 0 to 400-cm soil profiles at the center of the sample plot in layers of 20 cm. Three duplicate samples were collected from each sample point. The samples were placed in aluminum containers and taken back to the laboratory for soil moisture measurement by the gravimetric method. Three undisturbed soil samples were collected near the sampling sites with circular knives to determine soil bulk density, field water holding capacity and other soil hydraulic characteristics. The soil particle size was measured by Mastersizer 2000 laser particle size analyzer (Malvern Instruments, Malvern, UK). The vegetation growth indexes measured in this experiment mainly included tree height and tree density which was measured using the tape measurement. The selected plots were all planted in a standardized “two-row and one-belt” pattern, so the initial tree density was 230 tree/ha, and the existing tree density was calculated by square measurement. At the same time, the sample plots were investigated (Table 1), and there was no other vegetation around, so planting density and other vegetation did not affect the experimental results.
2.3 Statistical analysis
Soil water storage is the amount of soil water stored in a certain unit volume. In this study, based on the observed 0–400-cm soil depth, the soil water storage values per unit volume at these soil depths were calculated as follows:
where SWSi is the soil water restorage of the 0–400-cm layer from point i (i = 1,…,n) mm, SVWCi is the soil volumetric moisture content in cm3cm-3, di is the soil depth in mm, and
The groundwater level in the study area is relatively deep (over 80 m), and precipitation is the only source of soil water. The main factors affecting the soil water supply in H. ammodendron forestland are natural precipitation, followed by canopy interception loss and surface runoff. The canopy interception of H. ammodendron is very small and negligible. The empirical formula of the relationship between precipitation and soil water supply, which is widely used in this research area, was adopted in this study (Guo and Shao. 2007):
where
Due to the negative balance of deep water storage caused by forest and grass vegetation over-consuming, the dry soil layer that below the rainfall infiltration recharge depth is long-standing desiccated. This process is a special hydrological phenomenon in arid and semi-arid areas. (Zhao et al., 2020). The soil desiccation can be judged and evaluated with different methods. Most researchers think that the soil moisture in dry layer should be within the upper limit of field steady water holding capacity or soil steady humidity and the lower limit of wilting point (Jia et al., 2019). In order to quantitatively describe the desiccation intensity, Chen, et al. (2005) defined the desiccation index, which is: the ratio of the actual effective soil water content of a certain soil layer to the stable effective water content of this layer.
where SDI is soil desiccation index, WM is wilting point, SSM refers to the field water holding capacity, and SM is soil moisture.
3 Results
3.1 Soil moisture distribution in different age H.ammodendron plantations
Pearson correlation analysis showed that forest age and soil depth were significantly negatively correlated with soil moisture. The correlation coefficient between forest age and soil moisture was −0.795, which was significantly correlated at 0.01 level, while that between soil depth and soil moisture was −0.255, which was significantly correlated at the level of 0.05 (Table 2). The age of forest determines the soil moisture, and the older the age of forest, the more significant the response of the soil moisture in the deeper layer for the age of forest. The average soil moisture of the 0–100 cm layer is 7.5%, and the soil moisture decreases from the shallow layer to the deep layer. The soil moisture of the shallow layer (0–100 cm) is obviously higher than that of the deep layer (100–400 cm); the deep soil moisture is relatively stable, and the soil moisture has no obvious stratification change. After sand-fixing vegetation had been established for 11 years, the pattern of soil moisture in the sand-fixing area changed. When the sand-fixing vegetation was established for 30 years, the soil moisture in the deep layer decreased greatly, and the soil moisture in the deep layer was between 1% and 3%. The soil moisture presented a decreasing trend, and the variation range of soil moisture content in the deep layer with planting years was larger than that in the shallow layer. The soil moisture is highly heterogeneous in spatial distribution due to the effects of precipitation, evapotranspiration, topography and land use patterns. The range of variation in soil moisture in different soil layers under different land use patterns is quite different, and the coefficient of variation (CV) reflects this characteristic. Figure 2 showed that the CV increased with the increase of forest age, which was mainly reflected in the deep soil layer, suggesting the vertical distribution of soil moisture was affected by the old-age H. ammodendron plantation partially.
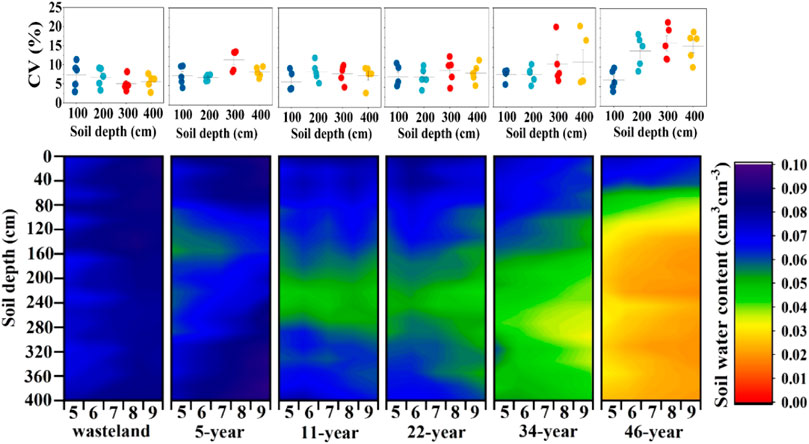
FIGURE 2. Vertical distribution of soil moisture and CV in H. ammodendron plantations of different ages.
The soil water consumption depth and supplement depth refer to the profile depth of soil water consumed by plants in the current year and the depth to which precipitation can infiltrate in the current year under a specific land use mode, which are the result of the joint action of precipitation infiltration supplement and system water consumption. Based on the analysis of storage water consumption and replenishing depth of H. ammodendron soil layers at ages 5, 11, 22, 34 and 46, it can be seen that the different forest ages significantly affected soil water consumption, and the recharge depth of different plots were affected by soil moisture, canopy interception, evapotranspiration and land use patterns. The depth of soil water consumption of the five-year-old H. ammodendron forest was 120–160 cm, and for the 11–22-year-old forest was 160–280 cm, but that of the 34–46-year-old H. ammodendron forest was higher than the measured depth of 400 cm because the soil water was depleted by the growth of the old-age H. ammodendron forest, and the soil water supply was less than the soil water consumption. Without artificial intervention, the soil desiccation in the deep layer would persist for a long period of time.
3.2 The soil water supply and consumption of H. ammodendron plantations
Atmospheric precipitation is the main source of soil water in the study area, and the dynamic changes in soil under different land use patterns are greatly affected by precipitation. Precipitation over time during the 2021 study period is shown in Figure 3. The precipitation was mainly concentrated in May–October and peaked in June. The soil water storage in 2021 of H. ammodendron 0–400 cm at the ages of 5, 11, 22, 34 and 46 years was monitored from May to September in the study area (Figure 3). The soil water storage of H. ammodendron at 0–400 cm at 5 years old was the highest, and that of H. ammodendron at 46 years old was the lowest. During the observation period, H. ammodendron began to grow from May to June, and the soil water storage in the sample plots was relatively high due to the rainfall supplement in the previous year. It is worth noting that the soil water storage of H. ammodendron at 5–11 years old increased gradually with the months; in 11–46-year-old plots, H. ammodendron showed the opposite trend. Although there was more precipitation in June, the air temperature rose quickly, the evaporation of surface soil was larger, the water consumption intensity of H. ammodendron roots gradually increased, the soil water consumption was larger than that of replenishment, the soil water storage of old H. ammodendron decreased gradually, the soil water storage of old H. ammodendron reached the lowest value in the following September, and the soil water consumption was much greater than that caused by soil water supplementation.
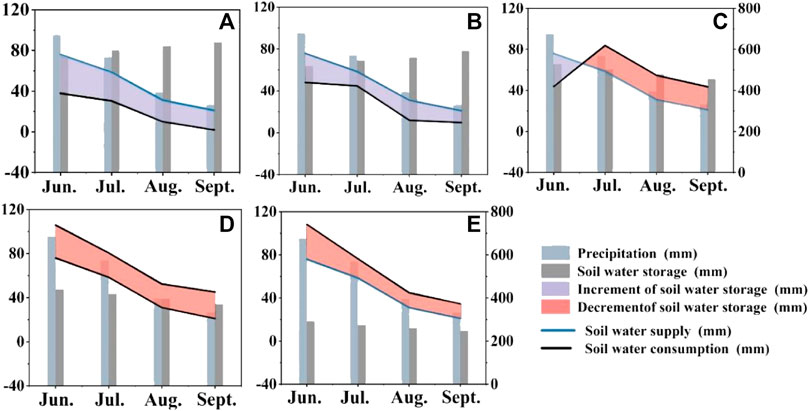
FIGURE 3. Soil water supply, consumption and water balance diagram of H. ammodendron plantations at different ages: (A) 5, (B) 11, (C) 22, (D) 34, and (E) 46 years, the blue line represents soil water supply, the black line represents soil water consumption, and the area between the two lines represents the increment of soil water storage. Where increment of soil water storage and decrement of soil water storage are denoted by different colors.
According to the model of soil water supplement-consumption, the threshold of the balance between soil water supplement by precipitation and transpiration consumption of vegetation is 16.5 years (Figure 4). In May–June, H. ammodendron began to grow, and the vegetation transpiration was smaller than the amount of soil water supply by precipitation. In addition, the amount of precipitation in June was high, so the soil water storage showed a positive trend in the early stage of the growing season. After June, as the temperature rose, the evapotranspiration gradually increased, and the precipitation decreased in the later stage. The soil water supply was less than the soil water consumption, which made the soil water storage decrease. In general, the soil water storage capacity of the 0–400 cm soil layer of H. ammodendron stands of different ages was significantly different, among which the soil water storage capacity of 5–11-year-old stands was the highest and that of 5–11-year-old stands was higher than that of soil water consumption because of soil water supplementation. The soil water storage profile increased with increasing precipitation, indicating that precipitation can partly replenish soil water. At 16.5 years old, the supply and consumption of soil water reached equilibrium, and then the consumption of soil water was greater after that. The continuous negative balance of soil moisture in the H. ammodendron forest from May to September indicated that the soil moisture consumed by strong transpiration during the growth period could not be replenished by precipitation for the aged H. ammodendron forest.
3.3 Soil desiccation at H. ammodendron plantations of different ages
Figure 5 shows the soil desiccation index (SDI) of the 0–400-cm soil layer in H. ammodendron stands of different ages. The results show that the soil SDI of the 0–400-cm soil layer in H. ammodendron stands of different ages first decreases and then increases with the deepening of the soil layer with the following order: 46a > 34a > 22a > 11a > 5a> Wasteland. The results showed that with the growth of H. ammodendron and the increase in tree age, the degree of soil desiccation increased and reached the maximum at 46 years of age. However, it is worth noting that the SDI of different soil layers is quite different, and the SDI of the 0–100 cm soil layer is higher in different tree ages, indicating that the soil layer is most supplemented by precipitation, and soil desiccation does not occur in the soil layer. Compared with the upper layer, the other stand ages obviously decreased and exhibited serious desiccation. The SDI of the deeper 300–400-cm soil layer was quite different. The roots of H. ammodendron in the 5- and 11-year-old stands did not reach this layer, so the soil moisture of this layer was higher and the desiccation phenomenon did not appear. The soil moisture was high, but the H. ammodendron forests at 22, 34 and 46 years of age showed serious desiccation. In general, the soil moisture trend observed in the vertical profile of H. ammodendron forests was consistent and changed regularly with the growth years of H. Ammodendron. The range of soil moisture change was small in the 0–100-cm soil layerand the soil moisture of the 100–200-cm soil layer decreased rapidly. The soil moisture of H. ammodendron forests decreased to its lowest point in this layer in the 5-year-old stand. Because the soil layer below 100 cm is less affected by environmental factors, the soil layer with the moisture absorbed by plant roots for plant growth and transpiration is the low moisture layer. Figure 5 shows that the low moisture layer mainly lies in the 100–400-cm soil layer, but the range of the low moisture layer is different in different tree ages. In the 5-year-old H. ammodendron plantation, the low soil moisture layer was mainly located in the 100–160-cm rang. But in the 11- and 22-year-old H. ammodendron plantations, the low soil moisture layers were mainly located in the 160–280-cm range. With increasing tree age, the water consumption depth of the root system of fruit trees increased, which caused the water consumption layer of fruit trees to gradually move down, and the low moisture layer of H. ammodendron soil was more than 400 cm at 46 years old.
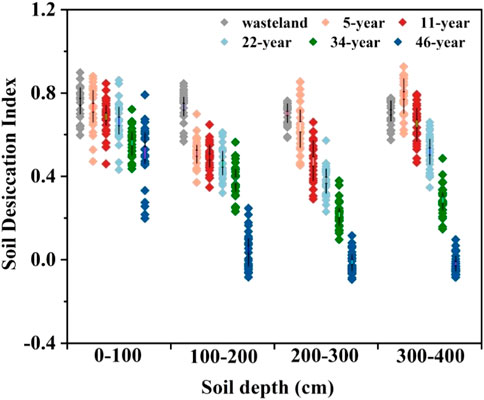
FIGURE 5. Evaluation of soil water deficit of H. ammodendron plantations at 0–400 cm in different ages.
4 Discussion
4.1 Effect of vegetation on soil moisture
The influence of vegetation on soil moisture mainly includes the water absorbed (root) and transpiration (leaf, twig and canopy) by individual plants to maintain their normal growth, survival and photosynthesis and their indirect effects on soil moisture, such as the interception of precipitation by the plant canopy (Lei et al., 2010; Wang et al., 2012). In the desert region of Alxa, where the groundwater is more than 80 m deep, rainfall is the main source of the soil water (Pei et al., 2008). Groundwater does not participate in the local SPAC water cycle, so the vertical distribution of soil water in the desert area of Alxa only shows the profile water distribution curve formed by precipitation or the infiltration from the run-on and redistribution into the soil. This kind of water profile distribution structure easily causes deep soil water storage to be overutilized by plants and is difficult to compensate for (Chen et al., 2013). The soil moisture of H. ammodendron forestland in the Alxa desert has similar distribution characteristics in the vertical direction (Figure 2). Generally, in the soil layer that is deeper than 100 cm, the water retention effect of the surface fine sand layer has little volatility, while in the soil layer that is shallower than 100 cm, the soil moisture basically shows a decreasing trend with increasing forest age.
Hu et al. (2010) found a similar result in his study of soil moisture on the Loess Plateau, while Gao and Shao (2012) came to the opposite conclusion. The reason for the above differences is that Hu et al. (2010) research object was a single deep root shrub, which is similar to this study, while Gao and Shao (2012) research included not only shrubs but also many annual herbs, which have a greater impact on shallow soil moisture, resulting in the change in shallow soil moisture being greater than that of deep soil moisture. In addition Figure 2 shows that the trend of change of soil moisture in the 0–100 cm soil layer with forest age is small, but the deep soil moisture (100–300 cm) shows a decreasing trend with increasing forest age, and Wang et al. (2015) found a similar trend in the Heihe River Basin. There are two main causes of this phenomenon. First, the study area is located in the Alxa desert, with sparse precipitation and strong evaporation, and because of its unique soil texture, precipitation is difficult to supplement below the 100-cm soil layer. In addition, with the continuous increase in forest age, precipitation cannot meet the water consumption of H. ammodendron transpiration, and the excessive consumption of soil water by plants exhausts the soil water. The excessive water consumption by vegetation roots results in the soil dry layer, which hinders the vertical movement of soil water, thus leading to vegetation decline (Jia et al., 2020). Investigation in this study found that when the H. ammodendron has been planted for more than 30 years, many branches and leaves fall off, stems become dry and broken, and the entire plant even dies (Figure 6). The variation of soil moisture is the result of the interactions of many factors, such as climate, vegetation type, soil texture, and topographic conditions. The consistency of soil texture, topographic conditions, and meteorological conditions in the study area ensures that the results are not affected by the above factors. It is worth noting that due to the lack of long-term continuous soil moisture data in this study, the forest age sequence was constructed by the “spatio-temporal substitution” method, which resulted in the inability to quantify the long-term potential soil moisture recovery. Therefore, future research should pay attention to the amount of potential soil moisture recovery.
4.2 Supply and consumption of soil moisture
Soil water consumption is affected by the characteristics of vegetation water consumption and the environment (Prechsl et al., 2015; Wu et al., 2019). In general, plants tend to use shallow soil, mainly because it takes less energy to consume shallow soil water (Schenk, 2008), and when the shallow soil water is insufficient, plants use more of the deep soil water (Jian et al., 2017; Tang and Chen, 2018). In this study we found that older H. ammodendron preferentially consumed more deep soil water than younger H. ammodendron. This shows that H. ammodendron forests do not consume shallow soil water first and then use deep soil water when shallow soil water is insufficient, but as forests age, H. ammodendron consumes shallow and deep soil water simultaneously. The consumption of soil water is affected by the soil plant water potential gradient. Consuming deep soil water does not always mean that more energy is needed. Climate drought leads to the reduction of shallow soil water potential. In this case, the deep soil water potential may be higher than that of the shallow soil (Bucci et al., 2004). Therefore, the consumption process of H. ammodendron can be characterized as follows: in the young age stage, H. ammodendron mainly consumes shallow water, while in the old age stage, when the water in the low wet layer decreases to a certain value, H. ammodendron takes its roots downward and consumes more deep soil water. The soil water conversion process of H. ammodendron at different forest ages was obtained using a soil water balance of 0–400 cm. In the early stage of H. ammodendron growth (11 years), the soil water storage increases with increasing precipitation, indicating that precipitation can meet the water requirement for the normal growth of H. ammodendron, while there is an inflection point in the middle age (16.5 years). Then, with increasing forest age, the supply of rainfall to soil water has difficulty meeting the consumption of soil water by H. ammodendron, and there is a negative balance of soil moisture.
The process of deep soil water consumption reveals the relationship between the water demand of H. ammodendron and the supply and demand of deep soil water. Older H. ammodendron need to grow more leaves for photosynthesis to synthesize more organic matter for vegetation growth, but this also leads to more deep soil water consumption. At the same time, the drying of soil moisture will lead to the reduction of cell swelling pressure, cell contraction and the weakening of leaf growth (Jaleel and Llorente, 2009). This vicious cycle is the main reason for the formation of a dry soil layer and the large-scale decline of H. ammodendron. Therefore, for aging orchards, reducing leaf growth and deep soil water consumption can reduce the drying of deep soil water to a certain extent (Jaleel and Llorente, 2009) to avoid the excessive drying of deep soil affecting plant survival. The water balance of vegetation is dynamic. On the one hand, the water consumption of vegetation is less at the beginning of afforestation. With the increase of afforestation years, the more water consumption, the more biomass. On the other hand, when the age of forest exceeds the threshold of vegetation carrying capacity, the soil moisture in turn restricts the growth of vegetation and eventually leads to the death. Therefore, determining the vegetation carrying capacity is the key for the success of afforestation efforts. Due to the lack of the actual transpiration water consumption data of H. ammodendron forest age, this study mainly discussed the vegetation carrying capacity through the water balance based on the relationship between soil moisture and forest age. Future studies should be combined with the transpiration water consumption data of H, ammodendron and other comprehensive assessment results.
4.3 Vegetation carrying capacity of soil moisture in desert areas
The vegetation carrying capacity of soil moisture is a key measure of afforestation rationality, and Chinese afforestation measures enacted over the past 60 years have made outstanding contributions to the region’s wind and sand control; however, the excessive water consumption of artificial vegetation has also broken the dynamic equilibrium between precipitation and native vegetation, and a new ecological problem, represented by the dry soil layer, has generally emerged (Gao and Shao, 2012; Jia et al., 2015; Bai et al., 2021). In recent years, a large number of studies have found that artificial vegetation consumption of soil water storage leads to soil moisture reduction (Chang et al., 2007). Almost all plantations can make the soil form desiccation layer, and the phenomenon of a soil desiccation layer is widely distributed (Kebin and Kaiguo, 1989). The average annual precipitation in the desert region of Alxa is approximately 150 mm, and the infiltration depth is usually less than 100 cm. During the continuous growth period of H. ammodendron, the root system consumes water intensively, and the precipitation in the rainy season is quickly consumed by evaporation and vegetation. The desiccation of soil below 100 cm is difficult to replenish and restore by precipitation infiltration, and the soil is in a state of desiccation for a long time (Zhang, et al., 2022). The results showed that the average soil moisture and soil water storage in the 0–400-cm soil layer decreased with each year, and the corresponding soil water consumption increased with each year with the increase in the growth years of H. ammodendron. Soil desiccation of H. ammodendron forests occurred to different degrees in the other years except the 5-year-old soil stand (Figure 5). These results indicate that the dry soil layer below the infiltration depth of rainfall in H. ammodendron forestland in the Alxa desert area is the result of the long-term accumulation of soil water consumption during the continuous growth of H. ammodendron, and the desiccation degree increases with the extension of planting years.
Haloxylon ammodendron is a perennial and deep-rooted plant. Its water consumption in the early growth period depends on the double supply of natural precipitation and deep soil water storage when the water content is high and the growth of H. ammodendron is fierce. However, with the increase in H. ammodendron’s age, the excessive water consumption in deep soil is intense, the water storage of the soil reservoir decreases each year, the water consumption depth of the root system gradually deepens, and the dry soil layer gradually forms and continuously thickens. The growth of H. ammodendron mainly depends on the precipitation supply in the year, and the lack of water supply leads to the decline and death of H. ammodendron. The present study suggested that in the desert region of Alxa, where the precipitation does not exceed 200 mm and the groundwater cannot be utilized by plants, the threshold of soil water and vegetation carrying capacity of H. ammodendron should not exceed 16.5 years. When the afforestation areas exceeds the threshold, the vegetation density can be reduced, which can not only prevent the soil moisture ecological environment from further deterioration but also play wind-proofing and sand-fixing roles and promote the sustainable development of afforestation in desert areas. Although the quantitative description and modeling of soil water carrying capacity are still at the stage of discussion, it is of great significance to define it scientifically in the future practice of national sand control. Based on the perspective of soil water balance, the optimal growth period of H. ammodendron plantations should not exceed 16.5 years. However, the economic maturity period may vary from this. Hence, future studies should combine soil water balance and input-output ratio to comprehensively determine the optimal growth years of plantations.
5 Conclusion
Afforestation (of H. ammodendron) in the Alxa desert has disrupted the original soil water balance and the distribution pattern of soil moisture. These changes have also driven a decline in vegetation, so the vegetation carrying capacity of soil moisture must be considered when studying afforestation in desert areas. The stability and persistence of vegetation and soil water balance are the basis for determining the carrying capacity. Under the specific precipitation and soil conditions in the Alxa desert area of China, based on the perspective of soil water balance, the optimal growth period of H. ammodendron plantations should not exceed 16.5 years. Therefore, the growth period of H. ammodendron plantations should be below this threshold in the future, and this is the premise by which the stability of vegetation and sustainable sand-fixing benefits can be ensured.
Data availability statement
The raw data supporting the conclusion of this article will be made available by the authors, without undue reservation.
Author contributions
DZ: Conceptualization, Data curation, Investigation, Methodology, Formal analysis, Roles/Writing—original draft, Writing—review and editing; JS: Conceptualization, Data curation, Formal analysis, Funding acquisition, Methodology; BJ: Investigation; CZ: Conceptualization, Data curation, Formal analysis, Investigation; JQ: Investigation; XH: Investigation; CW: Investigation; XZ: Investigation; ZL: Investigation. All authors read and approved the final manuscript. All authors have read and agreed to the published version of the manuscript. All authors contributed to the article and approved the submitted version.
Funding
This study was supported by the Major Science and Technology Project in Inner Mongolia Autonomous region of China (No. Zdzx2018057), the Innovation Cross Team Project of Chinese Academy of Sciences, CAS (No. JCTD-2019–19), Transformation Projects of Scientific and Technological Achievements in Inner Mongolia Autonomous region of China (No. 2021CG0046), and the National Natural Science Foundation of China (No. 42001038).
Conflict of interest
The authors declare that the research was conducted in the absence of any commercial or financial relationships that could be construed as a potential conflict of interest.
Publisher’s note
All claims expressed in this article are solely those of the authors and do not necessarily represent those of their affiliated organizations, or those of the publisher, the editors and the reviewers. Any product that may be evaluated in this article, or claim that may be made by its manufacturer, is not guaranteed or endorsed by the publisher.
Supplementary materials
The Supplementary Material for this article can be found online at: https://www.frontiersin.org/articles/10.3389/fenvs.2023.1054195/full#supplementary-material
References
Bai, Y., Liu, M., Yi, J., and Zhang, H. (2021). Temporal stability analysis of soil moisture along a coniferous forest hillslope with subtropical monsoon climate in southwest China. J. Mt. Sci-Engl 18 (11), 2900–2914. doi:10.1007/s11629-021-6679-5
Becknell, J. M., and Powers, J. S. (2014). Stand age and soils as drivers of plant functional traits and aboveground biomass in secondary tropical dry forest. Can. J. For. Res. 44 (6), 604–613. doi:10.1139/cjfr-2013-0331
Bucci, S. J., Scholz, F. G., Guillermo, G., Meinzer, F. C., Hinojosa, J. A., Hoffmann, W. A., et al. (2004). Processes preventing nocturnal equilibration between leaf and soil water potential in tropical savanna woody species. Tree Physiol. 10, 1119–1127. doi:10.1093/treephys/24.10.1119
Chang, X., Zhao, W., and Zhang, Z. (2007). Water consumption characteristic of Haloxylon ammodendron for sand binding in desert area. Acta Ecol. Sin. 27 (5), 1826–1837.
Chen, H., Wang, K., and Shao, M. (2005). A review on the effect of vegetation rehabilitation on the desiccation of deep soil layer on the loess plateau. Sci. Silvae Sin. 41 (4), 155–161.
Chen, L. J., Station, Q. F. A. D., Institute, E. E., and Sciences, C. L. (2013). Soil water and salt distribution under furrow irrigation of saline water with plastic mulch on ridge. J. Arid. Land 5, 60–70. doi:10.1007/s40333-013-0142-6
Deng, L. F., Zhang, F., Zhang, H. W., Zhang, X. L., and Yuan, J. (2019). Analysis of the spectral characteristics of Haloxylon ammodendron under water stress. Spectrosc. Spect. Anal. 39 (1), 210–215.
Gao, L., and Shao, M. (2012). Temporal stability of soil water storage in diverse soil layers. Catena 95, 24–32. doi:10.1016/j.catena.2012.02.020
Gao, S., Su, P. X., Yan, Q. D., and Ding, S. S. (2010). Canopy and leaf gas exchange of Haloxylon ammodendron under different soil moisture regimes. Sci. China Life Sci. 53 (6), 718–728. doi:10.1007/s11427-010-4013-5
Guo, Z. S., and Shao, M. A. (2007). Dynamics of soil water supply and consumption in artificial caragana shrub land. J. Soil Water Conservation 21 (2), 5. (in Chinese).
Guo, Z. S. (2021). Soil water carrying capacity for vegetation. Land Degrad. Dev. 32 (14), 3801–3811. doi:10.1002/ldr.3950
Hu, D., Lv, G. H., Qie, Y. D., Wang, H. F., Yang, F., and Jiang, L. M. (2021). Response of morphological characters and photosynthetic characteristics of Haloxylon ammodendron to water and salt stress. Sustainability-Basel 13 (1), 388. doi:10.3390/su13010388
Hu, W., Shao, M., and Reichardt, K. (2010). Using a new criterion to identify sites for mean soil water storage evaluation. Soil Sci. Soc. Am. J. 74 (3), 762–773. doi:10.2136/sssaj2009.0235
Jaleel, C. A., and Llorente, B. E. (2009). Drought stress in plants: A review on water relations. Biosci. Res. 6 (1), 20–27.
Jia, X., Shao, M., Yu, D., Zhang, Y., and Binley, A. (2019). Spatial variations in soil-water carrying capacity of three typical revegetation species on the loess plateau, China. Agric. Ecosyst. Environ. 273, 25–35. doi:10.1016/j.agee.2018.12.008
Jia, X., Shao, M., Zhang, C., and Zhao, C. (2015). Regional temporal persistence of dried soil layer along south-north transect of the Loess Plateau, China. J. Hydrol. 528, 152–160. doi:10.1016/j.jhydrol.2015.06.025
Jia, X. X., Zhao, C. L., Wang, Y. Q., Zhu, Y. J., Wei, X. R., and Shao, M. A. (2020). Traditional dry soil layer index method overestimates soil desiccation severity following conversion of cropland into forest and grassland on China's Loess Plateau. Agr. Ecosyst. Environ. 291, 106794. doi:10.1016/j.agee.2019.106794
Jia, Y. H., Li, T. C., Shao, M. A., Hao, J. H., Wang, Y. Q., Jia, X. X., et al. (2019). Disentangling the formation and evolvement mechanism of plants-induced dried soil layers on China's Loess Plateau. Agr. For. Meteorol. 269, 57–70. doi:10.1016/j.agrformet.2019.02.011
Jian, W., Fu, B., Nan, L., and Li, Z. (2017). Seasonal variation in water uptake patterns of three plant species based on stable isotopes in the semi-arid Loess Plateau. Sci. Total Environ. 609, 27–37. doi:10.1016/j.scitotenv.2017.07.133
Kebin, Z., and Kaiguo, Z. (1989). Afforestation for sand fixation in China. J. Arid. Environ. 16 (1), 3–10. doi:10.1016/s0140-1963(18)31041-3
Lei, H., Zhang, Z., and Li, X. (2010). Sap flow of Artemisia ordosica and the influence of environmental factors in a revegetated desert area: Tengger Desert, China. Hydrol. Process 24 (10), 1248–1253.
Lu, F., Shayiban·WubuliLiu, S., and Xu, G. (2021). Rooting depth determined physiological response of Haloxylon ammodendron to summer drought. Acta Ecol. Sin. 41 (8), 3178–3189.
Lue, X. P., Shao, K. Z., Xu, J. Y., Li, J. L., Ren, W., Chen, J., et al. (2022). A heat shock transcription factor gene (HaHSFA1) from a desert shrub, Haloxylon ammodendron, elevates salt tolerance in Arabidopsis thaliana. Environ. Exp. Bot. 201, 104954. doi:10.1016/j.envexpbot.2022.104954
Pei, S., Hua, F., and Wan, C. (2008). Changes in soil properties and vegetation following exclosure and grazing in degraded Alxa desert steppe of Inner Mongolia, China. Agr. Ecosyst. Environ. 124 (1), 33–39. doi:10.1016/j.agee.2007.08.008
Prechsl, U. E., Burri, S., Gilgen, A. K., Kahmen, A., and Buchmann, N. (2015). No shift to a deeper water uptake depth in response to summer drought of two lowland and sub-alpine C 3 -grasslands in Switzerland. Oecologia 177 (1), 97–111. doi:10.1007/s00442-014-3092-6
Schenk, H. J. (2008). The shallowest possible water extraction profile: A null model for global root distributions. Vadose Zone J. 7 (3), 1119–1124. doi:10.2136/vzj2007.0119
She, D. L., Liu, D. D., Xia, Y. Q., and Shao, M. A. (2014). Modeling effects of land use and vegetation density on soil water dynamics: Implications on water resource management. Water Resour. Manag. 28 (7), 2063–2076. doi:10.1007/s11269-014-0599-x
Singh, K., Pandey, V. C., Singh, B., and Singh, R. R. (2012). Ecological restoration of degraded sodic lands through afforestation and cropping. Ecol. Eng. 43, 70–80. doi:10.1016/j.ecoleng.2012.02.029
Song, C. W., Li, C. J., Halik, U., Xu, X. W., Lei, J. Q., Zhou, Z. B., et al. (2021). Spatial distribution and structural characteristics for Haloxylon ammodendron plantation on the southwestern edge of the gurbantunggut desert. Forests 12 (5), 633. doi:10.3390/f12050633
Suo, X. H., and Cao, S. X. (2021). China's three north shelter forest program: Cost-benefit analysis and policy implications. Environ. Dev. Sustain 23 (10), 14605–14618. doi:10.1007/s10668-021-01260-z
Tang, Y., Chen, Y., Wen, J., Xie, Y., and Lu, S. (2018). Water use strategies for two dominant tree species in pure and mixed plantations of the semiarid Chinese Loess Plateau. Ecohydrology 11 (2), e1943. doi:10.1002/eco.1943
Wang, G., Chen, Z., Shen, Y., and Yang, X. (2021). Efficient prediction of profile mean soil water content for hillslope-scale Caragana korshinskii plantation using temporal stability analysis. Catena 206, 105491. doi:10.1016/j.catena.2021.105491
Wang, G., Zhao, W., Liu, H., Zhang, G., and Li, F. (2015). Changes in soil and vegetation with stabilization of dunes in a desert–oasis ecotone. Ecol. Res. 30 (4), 639–650. doi:10.1007/s11284-015-1267-1
Wang, W., Li, B. Y., and Ren, Z. Y. (2017). Ecosystem service function evaluation: A case study of the yinchuan basin in China. Ecol. Eng. 106, 333–339. doi:10.1016/j.ecoleng.2017.06.014
Wang, X. P., Zhang, Y. F., Hu, R., Pan, Y. X., and Berndtsson, R. (2012). Canopy storage capacity of xerophytic shrubs in Northwestern China. J. Hydrol. 454-455, 152–159. doi:10.1016/j.jhydrol.2012.06.003
Wu, H., Zhao, G., Li, X. Y., Wang, Y., Sun, W., Jiang, Z., et al. (2019). Identifying water sources used by alpine riparian plants in a restoration zone on the Qinghai-Tibet Plateau: Evidence from stable isotopes. ence Total Environ. 697, 134092. doi:10.1016/j.scitotenv.2019.134092
Xia, Y. Q., and Shao, M. A. (2008). Soil water carrying capacity for vegetation: A hydrologic and biogeochemical process model solution. Ecol. Model 214 (2-4), 112–124. doi:10.1016/j.ecolmodel.2008.01.024
Xue, J. H., and Fang, S. Z. (2002). Afforestation techniques and vegetation restoration profits analysis of degraded ecosystems in karst mountain areas. J. Nanjing For. Unversity 26 (2), 1–6.
Zhang, P., Wang, R., Dai, Y., Li, Q., Ma, Y., and Zhao, J. (2022). Soil moisture availability and soil desiccation under different vegetation conditions in yuci,shanxi. Res. Soil Water Conservation 29 (5), 192–198.
Zhao, C. L., Jia, X. X., Shao, M. A., and Zhang, X. B. (2020). Using pedo-transfer functions to estimate dry soil layers along an 860-km long transect on China's Loess Plateau. Geoderma 369, 114320. doi:10.1016/j.geoderma.2020.114320
Keywords: Haloxylon ammodendron, plantations, soil desiccation, soil water content, water balance
Citation: Zhou D, Si J, He X, Jia B, Zhao C, Wang C, Qin J, Zhu X and Liu Z (2023) The relationship between vegetation and soil moisture reveals the vegetation carrying capacity threshold—A case study of a Haloxylon ammodendron plantation in the Alxa desert, China. Front. Environ. Sci. 11:1054195. doi: 10.3389/fenvs.2023.1054195
Received: 26 September 2022; Accepted: 05 May 2023;
Published: 16 May 2023.
Edited by:
Birhanu Biazin, International Potato Center, GhanaReviewed by:
Hailong Wang, Sun Yat-sen University, ChinaYu Wang, Lanzhou University of Technology, China
Xu Cundong, North China University of Water Conservancy and Electric Power, China
Copyright © 2023 Zhou, Si, He, Jia, Zhao, Wang, Qin, Zhu and Liu. This is an open-access article distributed under the terms of the Creative Commons Attribution License (CC BY). The use, distribution or reproduction in other forums is permitted, provided the original author(s) and the copyright owner(s) are credited and that the original publication in this journal is cited, in accordance with accepted academic practice. No use, distribution or reproduction is permitted which does not comply with these terms.
*Correspondence: Jianhua Si, amlhbmh1YXNAbHpiLmFjLmNu