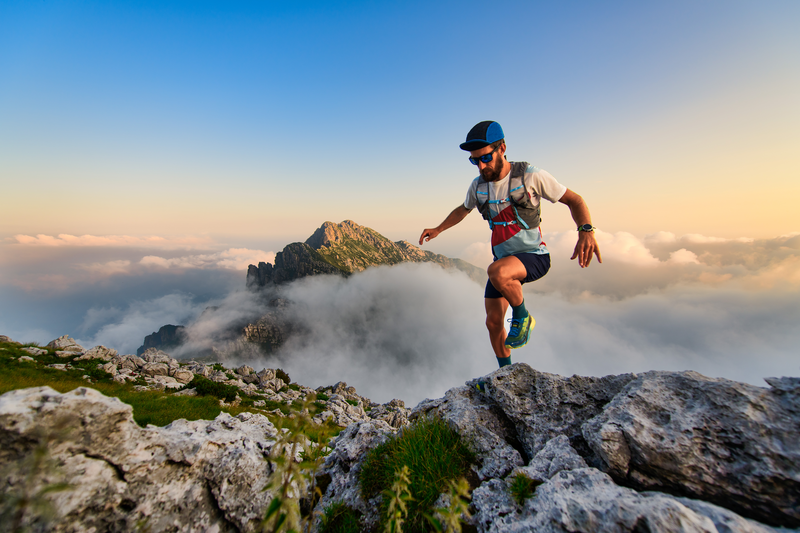
94% of researchers rate our articles as excellent or good
Learn more about the work of our research integrity team to safeguard the quality of each article we publish.
Find out more
ORIGINAL RESEARCH article
Front. Environ. Sci. , 13 February 2023
Sec. Toxicology, Pollution and the Environment
Volume 11 - 2023 | https://doi.org/10.3389/fenvs.2023.1031770
In micro-densitometry of wood it is standard procedure to extract resin and other soluble compounds before X-ray analysis to eliminate the influence of these extractives on wood-density. Dendrochemical studies using X-ray fluorescence analysis on the other hand are commonly conducted without previous extraction. However, it is well known that translocation processes of elements during heartwood formation in trees or (temporal) differences in sap content of wood samples can influence dendrochemical element profiles. This might bias environmental signals stored in time series of element concentrations in wood proxies. We hypothesize that metals tightly bound to cell walls show a more robust proxy potential for environmental conditions than easily translocated ones. To eliminate the noise of these soluble substances in wood elemental time series, their extraction prior to analysis might be necessary. In our study we tested the effect of different solvents (water, alcohol, and acetone) and different extraction times on elemental time series of three tree species with differing wood structure (Pinus sylvestris; Quercus robur and Populus tremula). Micro-XRF analysis was conducted on nine replicates per species using an ITRAX-Multiscanner. A set of elements commonly detected in wood (S, Cl, K, Ca, Ti, Mn, Fe, and Ni) was analysed at high resolution before and after several extraction runs. Besides lowering their levels, extraction did not significantly change the temporal trends for most elements. However, for some elements, e.g., Potassium, Chlorine or Manganese, especially the water extraction led to significant decreases in concentrations and altered temporal trends. Apparently the dipole effect of water produced the strongest extraction power of all three solvents. In addition we observed a dependency of extraction intensity from wood density which differed between wood types. Our results help in interpreting and evaluating element profiles and mark a step forward in establishing dendrochemistry as a robust proxy in dendro-environmental research.
Dendrochemistry, i.e., the chemical analysis of tree-rings is increasingly used as a natural archive of historical changes in the chemistry of the environment of trees. A wide variety of analytical techniques (destructive and non-destructive) is applied to analyse wood samples in the contexts of environmental pollution, changes in soil chemistry, dating of volcanic eruptions and forest fires or provenancing of wood (Pearson et al., 2005; Kuang et al., 2008; Locosselli et al., 2018; Muñoz et al., 2019; Rocha et al., 2020; Boeschoten et al., 2022). Temporal trends of elemental concentrations are used as proxies for historical changes of these diverse environmental factors. However, fundamental methodological issues concerning preferential uptake and translocation of elements as well as other physiologically regulated growth processes in woody plants often complicate the interpretation of the proxy–environment relationship in dendrochemistry (Watmough, 1999; Binda et al., 2021; Ballikaya et al., 2022). It is for example, still unclear to what extend sap contents of the vessels and elements bound to the cell walls contribute to the overall chemical profiles of wood samples.
While interpreting the presence or absence of elements in time series of wood many factors and processes need to be taken into account: climatic and other environmental conditions, season of sampling, nutrient availability, tree species, species capacity to uptake or actively block elements by roots, ionic solubility, charge, ionic radius ratio and the function of the element in the tree (essential or not to its development) or the binding to the xylem matrix, to name only a few (Balouet et al., 2009; Binda et al., 2021; Ballikaya et al., 2022). Some of these factors affect processes outside the tree and during uptake, others are more internal and occur in the tree-stems during wood formation (Locosselli et al., 2018).
Wood consists of different vessels/tracheids, fibres and parenchymatic tissues. Elements taken up by a tree are transported by the sap- and water stream in the phloem and xylem and can either be incorporated in any of these fibres/cell walls or stored in non-structural forms or xylem sap solutes. A significant influence of the xylem sap on dendrochemical time series was for example, shown by Hagemeyer and Schäfer. (1995) in a study that compared trace metal concentrations in samples taken at different times of the vegetation season which revealed considerable seasonal variations. Similarly, in an analysis of xylem sap in spruce, changes in sap element concentration with season were found for some elements (K, Ca, Mg, Mn, and Al) whereas variations of concentration in P, Cu, Zn, and Fe could not be attributed to time of the year (Smith and Shortle, 2001).
Depending on the element and the binding strength of the different atoms, their relative position in the wood or tree ring is more or less stable in time. Active and passive translocation processes during the trees lifetime may blur or destroy any chronological recording of environmental trends (Nabais et al., 2001; Smith et al., 2014; Scharnweber et al., 2016; Kohler et al., 2019). For example, heartwood formation in many trees includes a relocation of essential elements from the inner to the outer sapwood (Meerts, 2002; Smith et al., 2014). Opposite to this, many non-essential elements can be found in higher concentration in the trees heartwood due to an increase in cation binding capacity towards the inner stem-parts (Herbauts et al., 2002). Furthermore also the storage conditions of wood after tree-death can affect dendrochemical profiles. Subfossil wood which was preserved over centuries in sediments, peat or water is often highly subjected to leaching, concretion or other processes of elemental exchange with its surrounding which might affect dendrochemical profiles (Fors et al., 2015; Ghavidel et al., 2020).
Therefore an extraction of easily translocated elements and sap solutes or elements added to the wood after tree-death prior to any dendrochemical analysis might help to reveal “true” environmental trends archived in tree rings which are otherwise obscured. It can be assumed that trends in elemental concentrations which persist after an extraction pretreatment might be less influenced by translocation processes during the trees lifetime as well as by leaching or accumulation of trace elements after tree-death and during wood storage.
Following this reasoning an extraction of soluble contents in wood samples before dendrochemical analysis was recommended by Cutter and Guyette. (1993) to reduce the biasing influence of translocation processes of elements in dendrochemical time series. If applied, it could be shown that this chemical pre-treatment of wood samples increased correlations between element profiles in different conifer species (Gavrikov et al., 2022). In another study Belt et al. (2017) could visualize the influence of an extraction on the concentration of extractives present in pine heartwood, so called pinosylvins. However, Soxhlet extraction did not completely remove all pinosylvins in their study. A notable effect of extraction on radial time series of nitrogen in tree rings was also presented by Sheppard and Thompson. (2000). They recommend to extract all samples prior to any nitrogen measurement in wood to eliminate the influence of N present in sap solutes. Similarly, a chemical pre-treatment is recommended for dendrochronological analyses of carbon or nitrogen isotopes (Hart and Classen, 2003; Au and Tardif, 2009). In contrast, most dendrochemical studies analyse untreated wood samples (e.g., Scharnweber et al., 2016; Hevia et al., 2018; Muñoz et al., 2019; Rocha et al., 2020).
Like in isotopic studies an extraction pre-treatment is standard procedure in microdensitometrical analyses of coniferous and subfossil wood in order to remove the biasing influence of especially resin and other soluble compounds on wood density (Björklund et al., 2019). Sample preparation of the tree cores for microdensitometry follows the same protocol as for X-ray fluorescence (XRF) analysis. Therefore it is important to know the extent a possible later XRF-measurement of the same samples is influenced by this extraction.
Micro-XRF (µXRF) offers a non-destructive, sensitive, spatially highly resolved and comparably quick method to simultaneously measure a pre-defined set of elements in one analysis (Balouet et al., 2009; Binda et al., 2021). Different extractives and extraction times can be tested on one sample which is repeatedly measured. This allows for a direct quantification of the extraction effect. However, without calibration µXRF provides only relative concentrations measured in countrates which nevertheless enable for a detailed comparison of elemental trends.
Here we applied µXRF in order to test different extraction pre-treatments and their influence on a set of elements usually found in wood. The idea for this research was born at a workshop on dendrochemistry in 2019 in Stockholm. Three participating labs, namely, from University of Greifswald, Germany, from Stockholm University, Sweden and from the Research Institute Cetemas, Spain, all of them running an ITRAX-Multiscanner (Cox Analytical Systems, Sweden) for µXRF analysis of wood samples, designed the experiment and conducted the analyses. Different wood types are studied to explore a possible influence of wood structure on the extraction success. The goal of the study is to identify methods to reduce extraneous variation by extractives and sap solutes in dendrochemical time series of elements firmly incorporated in cell walls of wood. This might help in sharpening the environmental signal in dendrochemical time series and ease an attribution of detected trends to environmental factors in future studies.
In order to test if wood properties have an influence on the extraction process we selected three different tree species with differing wood anatomy for our analyses: Pedunculate oak (Quercus robur L.) as representative for ring-porous tree species with clear distinction between the large vessels of the earlywood and the denser latewood with smaller vessels; poplar (Populus tremula L.) as representative for diffuse porous tree-species with more homogeneous wood, smaller vessels and unclear distinction between early- and latewood and Scots pine (Pinus sylvestris L.) representing typical conifer wood with tracheids and clear density differences between early- and latewood. Oak and pine additionally form a typical heartwood which is distinctively different in colour and element content compared to the outer sapwood area. Sample trees were growing in managed forests on locally typical forest sites without any manipulation or specific pollution history. One tree per species was chosen for analysis to avoid inter-tree variations in dendrochemical profiles which would complicate the direct comparison of the extraction treatments. Oak was sampled in Northern Spain at Cerredo forest (Redes Natural-Park) in Degaña Council, an Atlantic ombrophilous forest with low intensity management and dominance of oak. The poplar samples origin from National Royal City Park near Stockholm, a mixed forest of Scots pine, Norway spruce, pedunculate oak, common birch, and trembling aspen. Pine was sampled in a planted, monospecific age-class forest south of Greifswald, NE-Germany. Trees were felled in 2018 and stem discs of 4–5 cm thickness from the three species were cut in breast height. Using a chop saw, three blocks representing three radii were cut from the discs. From each of the three resulting blocks three wooden laths of ∼1.2 mm thickness were cut using a twinblade saw (Dendrocut, Walesch, Switzerland) resulting in a total of nine samples from each tree and species (Figure 1). The samples differ in age and mean growth rates, oak shows 133 years at breast height and a mean ring width of 0.9 mm, pine is 33 years old with a mean growth rate of 3.2 mm and poplar trees are 46 years at breast height with a mean RW of 3.6 mm (Supplementary Figure S1).
FIGURE 1. Sample preparation and analysis steps: Three wood blocks from three different radii of one stem disc were prepared and three 1.2 mm thick sample stripes were cut, resulting in a total of nine samples per tree species/wood-type. Itrax measurements were done prior to extraction and after each extraction round in the three solvents alcohol, water and acetone.
Prior to any extraction exercise all nine samples per species were measured in an ITRAX Multiscanner at each respective laboratory. The scanners at Greifswald University (pine), Stockholm University (poplar) and CETEMAS (oak) were equipped with a 1.9 kW copper X-ray tube. Standard parameters of 30kV and 50 mA of tube voltage and current were used. Due to the copper X-ray tube used for analysis, Cu could not be analysed. Exposure time was set to 10 s per spot with a spatial resolution of 100 micron. Measurements were conducted under acclimatized conditions of 20°C and 50% relative humidity.
After this first XRF measurement of the untreated samples, different extraction rounds in three different solvents were carried out at all three labs. Samples 1-3, originating from the same radius (see Figure 1) were boiled in a Soxhlet apparatus using 98% ethyl-alcohol (ethanol) as extractive over 12 h. This resembles a standard procedure in microdensitometry for extracting resin and other soluble compounds in order to minimize their biasing influence on annual wood densities (Björklund et al., 2019). For samples 4-6 mineral water (Evian) was chosen as an extractive, again using hot extraction in a Soxhlet apparatus over 12 h. Temperature in the Soxhlet ranges between 50°C and −60°C measured in the actual extraction cylinder. Our decision to prefer mineral water over distilled water was based on the assumption that subfossil or archaeological wood is often stored in wet environments. Here, the ambient water can interact with the wood in two ways: Elements can be leached from the wood or minerals from the water can bind to the wood structures, which cannot be tested with distilled water. Nevertheless, since the water repeatedly evaporates during Soxhlet extraction and the samples only get in contact with the distillation product of the mineral water, in our experiment contamination of the samples with minerals from the water can be expected to be low although not entirely be excluded. Direct contact of the wood samples with the original mineral water only takes place at the very beginning of the extraction process, before the water begins to evaporate. Finally, samples 6-9 were extracted with acetone, this time at 20°C, a so called “cold extraction”. After extraction all samples were air dried over 3 days and scanned again in the ITRAX using the same device settings. A second extraction round over additional 12 h using the same solvents, drying and final XRF-measurements completes the experiment (Figure 1). Finally raw data of elemental countrates from three replicates for each treatment (untreated, 12 h, 12 h + 12 h extraction) and each solvent (ethanol, water, and acetone) were available for further analyses. The sampling, sample preparation, extractions and XRF-scannings were done at each respective laboratory separately, so each lab analysed nine samples from one species.
Data were analysed separately per species. Elements selected for analysis include the macronutrients sulfur (S), potassium (K) and calcium (Ca), and the micronutrient or trace elements chlorine (Cl), titanium (Ti), manganese (Mn), iron (Fe) and nickel (Ni). This set of elements is typically found in wood and can be detected by XRF with standard measurement parameters. To avoid the influence of edge effects (partly due to refraction of the X-rays when the focussed beam starts hitting the sample surface), the bark and the first ring(s) with extremely high countrates for some elements were excluded from all analyses. Countrates of the specific elements were divided by the coherent scatter to standardize in order to avoid the influence of changing matrix conditions of the wood on elemental countrates (Weltje and Tjallingii, 2008; Scharnweber et al., 2016).
In order to align and average all three replications per treatment, a common x-axis needs to be introduced. Therefore, for all samples the ring boundaries were defined on the radiographic image using WinDendro (Regent Instruments, Canada) and the pixel-based output of this measurement was used to transfer these boundaries to the elemental countrates which were finally averaged within years. Due to very narrow and sometimes unclear ring boundaries (see Figure 2C for an example) this could not be performed for the oak samples. In this case, the analysis has been performed applying a “macro tool” that allows to process the data obtained by the QSpec software (Cox Analytical Systems, Sweden) by previously defined length intervals, from bark to pith. This enables for a correct alignment of the three replicates per treatment before and after extraction in the analyses without averaging the countrates within ring boundaries. In general, the common signal between the three replicates per species and treatment was high, justifying an averaging of the results between the three replicates in elemental chronologies (Supplementary Table S2).
FIGURE 2. Radiographic images of (A) a pine (B) a poplar and (C) an oak sample overlaid with the trends for manganese, chlorine and potassium respectively for the control measurement (blue curve) and after 24 h of Soxhlet extraction in water (red curve). The lower panels show the differences between both curves (i.e., the extracted part) as a heatmap on the same radiograph. For pine the extraction success is higher at the inner heartwood part, for poplar chlorine peaks are more or less completely extracted and oak shows a relatively complete extraction over the whole sample. Darker colours of the radiographs correspond to the denser latewood, lighter colours to the earlywood.
Elemental countrates of the three species were compared between treatments and differences tested for significance using an ANOVA with Tukeys post hoc test. In order to test for potential changes in temporal trends of all analysed elements we computed the raw differences between control and 24 h extraction for each data point. Then the data is plotted for control and linear models are fitted and tested for significance of the slope. For selected elements of the pine and poplar samples with pronounced effects of the extraction we additionally tested if wood density has a significant influence on the extraction success. For this purpose the differences between control and 24 h extraction were related to wood-density values obtained from the radiographic images after grey scale calibration with a standard plexi-ladder. Data analysis was performed in R (R Development Core Team, 2022).
Extraction success differs between element and extractive, results are summarised in Figure 3; Table 1. In pine alcohol extraction had a slight effect on countrates of Cl and K which was already noticeable after 12 h of extraction. S, Ca, Ti, Mn, and Fe concentrations were not significantly affected by alcohol extraction. Water extraction removed significant amounts of Cl, K, and Mn from the pine samples, S, Ca, Ti, and Fe stayed rather constant. Finally, cold extraction using acetone as an extractive did not have a pronounced effect on the elements studied, with the exception of Ni (Supplementary Figure S2). For this element all three methods indicate a significant decrease after 24 h of extraction time in pine wood (Figure 3, upper panels).
FIGURE 3. Boxplots showing the countrates for all selected elements for pine, poplar and oak and the three solvents used (ethanol, water, and acetone). All countrates were divided by the coherent scatter to account for changing matrix conditions. y-Axes are log transformed to ease comparability between elements with very different medians. Results of the ANOVA post hoc test for group comparison are reported in Table 1. Blue: control; orange: 12 hrs extraction time; red: 24 hrs extraction time.
TABLE 1. Summary of observed changes in mean countrates and temporal trends of analysed elements for three different extractives. The small letters represent the results of the ANOVA-post hoc test between the three groups “control”, “12 h extraction” and “24 h extraction” (see Figure 3 for boxplots of the data). Significant changes in temporal trends are identified by fitting a linear model to the difference curves between control and 24 h extraction and testing for significance of the slope (see Supplementary Figures S5–S7 for details).
In the diffuse porous wood of poplar, alcohol extraction had an effect on countrates of Cl and K. Water extraction effectively removed Cl and K from the samples. Interestingly, countrates of Ca, Ti and Fe increased after the extraction, for Ca possibly because the mineral water used as an extractive contained Calcium ions (82 mg/L, see Supplementary Table S1) which got attached to the wood structures during the Soxhlet extraction. Similarly, the acetone cold extraction led to increases in Ti and Fe countrates already after 12 h of extraction, whereas Cl concentration decreased significantly.
Finally in the ring-porous oak, water effectively removed K from the samples, similar to the other wood types. All three extractives led to an increase in Fe, and to a lesser extend also Mn-countrates after extractions in oak. High values of Mn in the sapwood were nevertheless effectively removed by the water extraction (Supplementary Figure S4). Also the light elements S and Cl show an increase in countrates after extraction in water and alcohol. Likely this noticeable increase in countrates in some elements and species represents an artefact from the compositional data structure of the XRF-data, with concentration changes in one element possibly also influencing the countrates of other elements (Beckhoff et al., 2007; Weltje and Tjallingii, 2008).
In general for all three species the Soxhlet water extraction had the strongest effect on elemental countrates.
Taking a deeper look at the temporal trends of the elements it seems that wood density had a significant impact on the extraction success. For pine the concentration differences after extraction of the elements K, Cl and Mn which were affected most from the extraction, are depending on wood density, with a tendency of decreasing extraction effectiveness with higher wood density (Figure 4, upper panels). Although the variance in the data is high and the relationship is not very tight, the linear model is significant (p < 0.01) for all three elements. Contrary to this, in the diffuse porous wood of poplar, extraction tends to be more effective in wood of higher density thus the latewood part of the annual ring. Scatterplots show a significant positive relationship between wood density and extraction efficiency for the water-Soxhlet treatment for the three most affected elements K, Cl, and Ca (Figure 4, lower panels). As Ca concentrations increased with extraction i.e., Ca-ions from the water possibly got attached to the wood, it can be assumed that Ca-ions bind more effectively to wood of higher densities with a higher share of cell walls compared to cell lumen. As this change in Ca-countrates in poplar wood only becomes significant after 24 h extraction time, a contamination likely occurred during the second extraction round (see Figure 3; Supplementary Figure S3). Again, like in pine, values are very scattered and the relationship is far from tight. Because of small deviations of the measurement path between the different measurements of each sample, we abstained from comparing extraction amounts to wood densities for oak with its narrow rings. This analysis would be prone to large biases as an unequivocal assignment of each pixel of the control measurement to the repeated measurements after treatment is very difficult.
FIGURE 4. Relationship between wood density and extraction intensity for selected elements in the pine (upper row) and poplar samples (lower row); plotted are the differences values in countrates between control and the 24 h water extraction for K, Mn, and Cl (pine) and K, Cl, and Ca (poplar). Linear models (red lines) are significant (p < 0.01, Supplementary Table S3), a smoothing line is also plotted (blue line). Note the opposite slopes with decreasing extraction success with increasing densities for pine samples (negative slopes) and increasing extraction intensity with increasing densities for poplar (positive slopes).
From the time series curves of Mn it can be seen that the older parts of the pine samples showed bigger differences between control and treatment, likely due to heartwood/sapwood differences, with the heartwood containing a higher amount of soluble Mn (Figure 2A; Supplementary Figure S2). This is corroborated by lowered densities of the older wood parts after the extraction treatment (see Supplementary Figure S2). Therefore for this element a clear change in temporal trend after extraction can be observed (Supplementary Figure S5). For poplar no such patterns of higher extraction effectiveness in the older wood parts emerge-here also the densities show only marginal differences after extraction with the exception of water-Soxhlet extraction, where wood density was slightly lower after 24 h of extraction time over the whole sample (Supplementary Figure S3). Clear trend differences after extraction with all three reagents can be observed in poplar only for Ca (Supplementary Figure S6). Due to the high number of rings and their very narrow ring widths and unclear ring boundaries (see example in Figure 2C), we could not crossdate and average the elemental countrates within years for the oak samples. A comparison of the pixel based oak time series however revealed similar trend changes for K and Mn like in pine (Supplementary Figures S4, S7). Although not very evident from the time series plots it turned out that for oak also Ca (all three extractives), Cl (water), Fe (water) and Ni (water and alcohol) displayed significant trend changes after extraction (Supplementary Figure S7). Due to the much higher number of data points (for oak countrates are not averaged within ring boundaries) the linear models are tested significant already for flat slopes compared to the other two species.
Changes in countrates and temporal trends are summarized for all three species and treatments in Table 1. Except for Ca after alcohol extraction in pine, Ti after acetone extraction in pine and Cr after water extraction in poplar, ANOVA testing was significant for all elements, even if the differences in means were not very pronounced (Figure 3). This has to do with the high resolution XRF-measurements in 100 micron steps resulting in several hundred of data points (degrees of freedom) per sample. So already small differences led to significant results when testing the global null-hypotheses of equal means between groups.
Our results show a heterogeneous picture of changes in elemental composition after extraction with the three solvents ethanol, water and acetone for the three different wood structures analysed in this study. Ethanol had a marginal effect on most elements studied in pine and poplar wood. Only Cl and K concentrations and in pine also Ni changed markedly after 24 h of extraction. In the ring porous oak the elements S, Cl, Ti, Mn, and Fe showed even higher countrates after alcohol extraction, the others remained stable, similar to acetone. The strongest effect we observed for all species after Soxhlet-extraction using water. Likely the dipole structure of water effectively dissolved especially potassium (K) from all three wood types. This high mobility and easy solubility of potassium points at a rather limited proxy potential of this element concerning reconstructions of environmental changes. In addition Mn in pine and oak is affected by water extraction. Mn has been used in different dendrochemical studies and concentration changes are interpreted as driven by changes in soil chemistry and their effect on Mn-availability (Augustin et al., 2005; Kuang et al., 2008). In case of pine wood we could show that not only the amount of Mn decreased after extraction but also the temporal trend was affected, resulting in a much flatter slope over time (see Figure 2; Supplementary Figure S2). We interpret this with Mn being part of substances involved in heartwood formation-an active physiological process that needs to be separated from environmental trends. Water extraction seems promising in this regard but further studies specifically focused on heartwood-sapwood differences are needed to confirm this. New techniques like Raman spectroscopy imaging or micro X-ray absorption near edge structure (µ-XANES) offer adequate tools for high resolution visualization of wood extractives, cell wall polymers, compounds inserted into the cell walls and chemical speciation of elements in wood which can help in disentangling heartwood formation processes from other dendrochemical signals (Belt et al., 2017; Alves et al., 2021). Calcium for example, has been shown to occur in very different chemical speciations in the sapwood (calcium-oxalate, carbonate) and heartwood (calcium-sulfate) of mangrove trees (Alves et al., 2021).
As already shown by Hagemeyer and Schäfer. (1995) and pointed out by others (Smith and Shortle, 2001; Smith et al., 2014), calcium concentrations in wood vary over the vegetation season and this element is generally very mobile (Scharnweber et al., 2016). Our results show that water treatment led to a significant increase in Ca in poplar and oak wood. We expect this to be partly caused by Ca-contamination of the samples during Soxhlet extraction with the mineral water used in our experiment. Ca-ions from the water might have bound to the wood structure during the beginning of the experiment when the samples were in direct contact not only with the distillation product but with the original mineral water. This hints at a possible strong influence of wet storage conditions of subfossil and archaeological wood on Ca-concentrations which needs to be accounted for in dendrochemical analyses of this material.
Besides actual enrichments by ions from the solvent, an increase in countrates of certain elements like for example, Fe in poplar and oak can have methodological reasons. With X-ray fluorescence analysis just countrates i.e., the number of photons of a given energy which hit the detector in a given time will be determined. So it will just give relative concentrations and would require specific calibrations for relating the countrates to actual concentrations. Nevertheless, as the relationship between countrates and actual concentrations is mostly linear (Huang et al., 2016; Rodriguez et al., 2020), a comparison of countrates between different XRF-measurements like in our study is generally possible without calibration. During XRF-scanning for each measurement spot a continuous energy spectrum is generated. Peaks in the spectra are assigned to elements according to their specific emitted electron energy (element lines), taking into consideration also sum peaks or certain interferences between elements (Beckhoff et al., 2007). Peak areas are transformed to elemental countrates by means of a complex model fitting procedure, including the subtraction of background radiation intensity. For the ITRAX multiscanner data analysed in this study the Qspec software was used for this purpose. The multi element set used in this analysis is preselected by the user. A small change in model parameters or the selection or non-selection of elements in the model can influence the model fit and consequently element specific countrates. Also, effects related to compositional data structures with concentration changes in one element influencing the countrates of one or more other elements can occur. This is because countrates of a specific element are not only proportional to its concentration but also a distinct function of the matrix, i.e., the elements accompanying the element in the sample (Beckhoff et al., 2007). For example, the increase in countrates of Fe and Ti in poplar and oak very likely are not an actual increase but related to methodological issues in this peak fitting procedure. Therefore, we would like to urge a cautious interpretation especially for elements with low concentrations or close to their detection limits in dendrochemical studies based on µXRF-data. If possible, a complementation of µXRF analyses with different analytical techniques like inductively coupled mass spectography (ICP-MS) or atomic absorption spectography (AAS) should be applied to reach a quantification for lower concentrations and a general validation of XRF-data.
Except for oak, chlorinated compounds are dissolved by most extraction treatments, including water. Studies interpreting trends and peaks in Cl-concentrations with pollution events (Balouet et al., 2007; Balouet et al., 2012) therefore need to be interpreted with caution. However, it has to be noted that the Cl-concentrations are often close to the detection limit of the measurement procedure applied in this study. In XRF the detection limits vary as a function of the atomic number of the element. Therefore very light elements like Cl have a rather high detection limit which is close to the concentrations usually found in wood. Nevertheless the mostly stable concentrations of S after extraction in our study point to a rather good proxy potential of this element. Studies using X-ray synchrotron analyses could show that S in different oxidation stages is mainly present in the inner cell walls of wood (Fairchild et al., 2009). In general, the form in which an element is contained in the wood can have an effect on the extraction efficiency. Due to its polar form with partial positive charges on the hydrogens, a partial negative charge on the oxygen and a bent overall structure, water for example, mainly dissolves ions and polar molecules like salts. Therefore pectinates and oxalates of various metals present in the intercellular space of wood (Gavrikov et al., 2022) as well as other crystals might be more efficiently extracted by water compared to metals present in different atomic form in the cell walls.
Besides lowering their levels, extraction did not strongly change the temporal trends for many elements (see Supplementary Figures S2–S4)—so possibly metals that are more effectively bound to cell walls correlate to the metal concentrations in the sap and cell lumen leading to lowered means but unchanged temporal trends if sap contents are extracted. Also here high resolution visualization techniques like Raman spectroscopy imaging or particle induced X-ray emission analysis (PIXE) are needed to further elaborate this relationship.
For the elements Cl, K, and Mn we found a significant influence of wood density on the extraction intensity in pine wood. Lower density earlywood showed higher differences in Cl, K and Mn between control and treatment compared to the denser latewood. This could be interpreted with the higher share of cell lumen in the lighter earlywood containing soluble Cl, K, and Mn compared to the denser latewood where the elements possibly are more tightly bound to the cell walls. In addition heartwood formation processes seem to play a role as Mn-extraction was more effective in the inner wood parts of pine which also showed lower wood-densities after extraction. Likely resin and other organic compounds containing Mn were dissolved from the heartwood. In contrast poplar showed higher extraction effectiveness with increasing wood density. Cell sizes are more heterogeneously distributed over the annual ring in diffuse porous wood of poplar with no clear differences between early-and latewood. Also heartwood formation processes are negligible in this species. This might explain the differing behaviour compared to coniferous pine wood. However it does not fully explain the observed relationship. Further studies are thus needed.
To conclude, we could show that, depending on the element, considerable concentration changes and altered temporal trends can occur when wood samples are subjected to an extraction before XRF analysis. This mainly affects macronutrients like K and Ca which are known to be heavily translocated and therefore not very well suited for dendrochemical analysis of environmental changes (Binda et al., 2021) and easily soluble elements like chlorine. Besides that, heartwood/sapwood related concentration changes with substances involved in heartwood formation resolved by extraction lead to changed temporal trends in our study. This might be beneficial when identifying “true” environmental signals in temporal trends of metal concentrations in wood. From the solvents tested water seems to have the strongest effect when used in a Soxhlet apparatus. Contamination during the extraction process can be avoided by using distilled water instead of mineral water. For those elements that were affected by the extraction treatments a duration of 24 h can be recommended as effectiveness increased with extraction time.
The raw data supporting the conclusion of this article will be made available by the authors, without undue reservation.
TS, ER, AGA, BG, and SH developed the research idea and outlined and conducted the study including lab work and data analysis. SA and MW helped with data analysis and data illustration. TS wrote the manuscript with the help of ER, AGA, BG, SH, SA, and MW. All authors approved the submitted version.
This paper and the research behind it would not have been possible without the exceptional support of laboratory staff. CETEMAS and DendroGreif would especially like to thank Laura Glez and Ulrich Möbius for their contribution to this work in terms of sample preparation, extraction development, and XRF analysis, as well as Marko Smiljanić for his assistance in the analysis of the results. We thank the Bolin Centre for Climate Research for funding of the workshop on Dendrochemistry that preceded this paper. Three reviewers are acknowledged for their valuable comments which helped to improve an earlier version of the manuscript.
The authors declare that the research was conducted in the absence of any commercial or financial relationships that could be construed as a potential conflict of interest.
All claims expressed in this article are solely those of the authors and do not necessarily represent those of their affiliated organizations, or those of the publisher, the editors and the reviewers. Any product that may be evaluated in this article, or claim that may be made by its manufacturer, is not guaranteed or endorsed by the publisher.
The Supplementary Material for this article can be found online at: https://www.frontiersin.org/articles/10.3389/fenvs.2023.1031770/full#supplementary-material
Alves, E. E. N., Rodriguez, D. R. O., de Azevedo Rocha, P., Vergütz, L., Junior, L. S., Hesterberg, D., et al. (2021). Synchrotron-based X-ray microscopy for assessing elements distribution and speciation in mangrove tree-rings. Results Chem. 3, 100121. doi:10.1016/j.rechem.2021.100121
Au, R., and Tardif, J. C. (2009). Chemical pretreatment of thuja occidentalis tree rings: Implications for dendroisotopic studies. Can J. Res 39, 1777–1784. doi:10.1139/x09-091
Augustin, S., Stephanowitz, H., Wolff, B., Schröder, J., and Hoffmann, E. (2005). Manganese in tree rings of Norway spruce as an indicator for soil chemical changes in the past. Eur J. Res 124, 313–318. doi:10.1007/s10342-005-0084-4
Ballikaya, P., Marshall, J., and Cherubini, P. (2022). Can tree-ring chemistry be used to monitor atmospheric nanoparticle contamination over time? Atmos. Environ. 268, 118781. doi:10.1016/j.atmosenv.2021.118781
Balouet, J-C., Oudijk, G., Smith, K. T., Petrisor, I., Grudd, H., and Stocklassa, B. (2007). Applied dendroecology and environmental forensics. Characterizing and age dating environmental releases: Fundamentals and case studies. Environ. Forensics 8, 1–17. doi:10.1080/15275920601180487
Balouet, J. C., Burken, J. G., Karg, F., Vroblesky, D., Smith, K. T., Grudd, H., et al. (2012). Dendrochemistry of multiple releases of chlorinated solvents at a former industrial site. Environ. Sci. Technol. 46, 9541–9547. doi:10.1021/es300318v
Balouet, J. C., Smith, K. T., Vroblesky, D., and Oudijk, G. (2009). Use of dendrochronology and dendrochemistry in environmental forensics: Does it meet the daubert criteria? Environ. Forensics 10, 268–276. doi:10.1080/15275920903347545
Beckhoff, B., Kanngießer, B., Langhoff, N., Wedell, R., and Wolff, H. (2007). Handbook of practical X-ray fluorescence analysis. Berlin, Germany: Springer Science & Business Media.
Belt, T., Hänninen, T., and Rautkari, L. (2017). Cellular level distributions of Scots pine heartwood and knot heartwood extractives revealed by Raman spectroscopy imaging. Ind. Crops Prod. 108, 327–335. doi:10.1016/j.indcrop.2017.06.056
Binda, G., Di Iorio, A., and Monticelli, D. (2021). The what, how, why, and when of dendrochemistry:(paleo) environmental information from the chemical analysis of tree rings. Sci. Total Environ. 758, 143672. doi:10.1016/j.scitotenv.2020.143672
Björklund, J., von Arx, G., Nievergelt, D., Wilson, R., Van den Bulcke, J., Günther, B., et al. (2019). Scientific merits and analytical challenges of tree-ring densitometry. Rev. Geophys 57, 1224–1264. doi:10.1029/2019rg000642
Boeschoten, L. E., Sass-Klaassen, U., Vlam, M., Comans, R. N. J., Koopmans, G. F., Rocha, V., et al. (2022). Clay and soil organic matter drive wood multi-elemental composition of a tropical tree species: Implications for timber tracing. Sci. Total Environ. 849, 157877. doi:10.1016/j.scitotenv.2022.157877
Cutter, B. E., and Guyette, R. P. (1993). Anatomical, chemical, and ecological factors affecting tree species choice in dendrochemistry studies. J. Environ. Qual. 22, 611–619. doi:10.2134/jeq1993.00472425002200030028x
Fairchild, I. J., Loader, N. J., Wynn, P. M., Frisia, S., Thomas, P. A., Lageard, J. G. A., et al. (2009). Sulfur fixation in wood mapped by synchrotron X-ray studies: Implications for environmental archives. Environ. Sci. Technol. 43, 1310–1315. doi:10.1021/es8029297
Fors, Y., Grudd, H., Rindby, A., and Bornmalm, L. (2015). X-Ray fluorescence for cultural heritage: Scanning biochemical fingerprints in archaeological shipwrecks. Spectrosc. Eur. 27, 11–13.
Gavrikov, V., Fertikov, A., Sharafutdinov, R., and Vaganov, E. (2022). Elemental relationships in the wood of four siberian conifers: Whether elements are an occasional mixture. Int. J. Plant Biol. 13, 142–150. doi:10.3390/ijpb13020014
Ghavidel, A., Hofmann, T., Bak, M., Sandu, I., and Vasilache, V. (2020). Comparative archaeometric characterization of recent and historical oak (Quercus spp.) wood. Wood Sci. Technol. 54, 1121–1137. doi:10.1007/s00226-020-01202-4
Hagemeyer, J., and Schäfer, H. (1995). Seasonal variations in concentrations and radial distribution patterns of Cd, Pb and Zn in stem wood of beech trees (Fagus sylvatica L.). Sci. Total Environ. 166, 77–87. doi:10.1016/0048-9697(95)04476-h
Hart, S. C., and Classen, A. T. (2003). Potential for assessing long-term dynamics in soil nitrogen availability from variations in δ 15N of tree rings. Isot. Environ. Health Stud. 39, 15–28. doi:10.1080/1025601031000102206
Herbauts, J., Penninckx, V., Gruber, W., and Meerts, P. (2002). Radial variations in cation exchange capacity and base saturation rate in the wood of pedunculate oak and European beech. Can J. Res 32, 1829–1837. doi:10.1139/x02-097
Hevia, A., Sánchez-Salguero, R., Camarero, J. J., Buras, A., Sangüesa-Barreda, G., Galván, J. D., et al. (2018). Towards a better understanding of long-term wood-chemistry variations in old-growth forests: A case study on ancient pinus uncinata trees from the pyrenees. Sci. Total Environ. 625, 220–232. doi:10.1016/j.scitotenv.2017.12.229
Huang, J., Löwemark, L., Chang, Q., Lin, T., Chen, H., Song, S., et al. (2016). Choosing optimal exposure times for XRF core-scanning: Suggestions based on the analysis of geological reference materials. Geochem. Geophys Geosystems 17, 1558–1566. doi:10.1002/2016gc006256
Kohler, M., Niederberger, J., Wichser, A., Bierbaß, P., Rötzer, T., Spiecker, H., et al. (2019). Using tree rings to reconstruct changes in soil P availability–Results from forest fertilization trials. Dendrochronologia 54, 11–19. doi:10.1016/j.dendro.2019.01.001
Kuang, Y. W., Zhou, G. Y., Chu, G. W., Sun, F. F., and Li, J. (2008). Reconstruction of soil pH by dendrochemistry of Masson pine at two forested sites in the Pearl River Delta, South China. Ann. Sci 65, 804. doi:10.1051/forest:2008070
Locosselli, G. M., Chacón-Madrid, K., Arruda, M. A. Z., de Camargo, E. P., Moreira, T. C. L., de André, C. D. S., et al. (2018). Tree rings reveal the reduction of Cd, Cu, Ni and Pb pollution in the central region of São Paulo, Brazil. Environ. Pollut. 242, 320–328. doi:10.1016/j.envpol.2018.06.098
Meerts, P. (2002). Mineral nutrient concentrations in sapwood and heartwood: A literature review. Ann. Sci 59, 713–722. doi:10.1051/forest:2002059
Muñoz, A. A., Klock-Barría, K., Sheppard, P. R., Aguilera-Betti, I., Toledo-Guerrero, I., Christie, D. A., et al. (2019). Multidecadal environmental pollution in a mega-industrial area in central Chile registered by tree rings. Sci. Total Environ. 696, 133915. doi:10.1016/j.scitotenv.2019.133915
Nabais, C., Freitas, H., and Hagemeyer, J. (2001). Tree rings and dendroanalysis. New York: Met Environ Anal biodiversity Marcel Dekker, 367–400.
Pearson, C., Manning, S. W., Coleman, M., and Jarvis, K. (2005). Can tree-ring chemistry reveal absolute dates for past volcanic eruptions? J. Archaeol. Sci. 32, 1265–1274. doi:10.1016/j.jas.2005.03.007
R Development Core Team (2022). R: A language and environment for statistical computing. Avaliable At: http://www.r-project.org/.
Rocha, E., Gunnarson, B., Kylander, M. E., Augustsson, A., Rindby, A., and Holzkämper, S. (2020). Testing the applicability of dendrochemistry using X-ray fluorescence to trace environmental contamination at a glassworks site. Sci. Total Environ. 720, 137429. doi:10.1016/j.scitotenv.2020.137429
Rodriguez, D. R. O., de Almeida, E., Tomazello-Filho, M., and de Carvalho, H. W. P. (2020). Space-resolved determination of the mineral nutrient content in tree-rings by X-ray fluorescence. Sci. Total Environ. 708, 134537. doi:10.1016/j.scitotenv.2019.134537
Scharnweber, T., Hevia, A., Buras, A., van der Maaten, E., and Wilmking, M. (2016). Common trends in elements? Within- and between-tree variations of wood-chemistry measured by X-ray fluorescence — a dendrochemical study. Sci. Total Environ. 566-567, 1245–1253. doi:10.1016/j.scitotenv.2016.05.182
Sheppard, P. R., and Thompson, T. L. (2000). Effect of extraction pretreatment on radial variation of nitrogen concentration in tree rings. J. Environ. Qual. 29, 2037–2042. doi:10.2134/jeq2000.00472425002900060042x
Smith, K. T., Balouet, J. C., Shortle, W. C., Chalot, M., Beaujard, F., Grudd, H., et al. (2014). Dendrochemical patterns of calcium, zinc, and potassium related to internal factors detected by energy dispersive X-ray fluorescence (EDXRF). Chemosphere 95, 58–62. doi:10.1016/j.chemosphere.2013.08.017
Smith, K. T., and Shortle, W. C. (2001). Conservation of element concentration in xylem sap of red spruce. Trees 15, 148–153. doi:10.1007/s004680000085
Watmough, S. A. (1999). Monitoring historical changes in soil and atmospheric trace metal levels by dendrochemical analysis. Environ. Pollut. 106, 391–403. doi:10.1016/s0269-7491(99)00102-5
Keywords: dendrochemistry, extraction, tree-rings, elements, XRF
Citation: Scharnweber T, Rocha E, González Arrojo A, Ahlgrimm S, Gunnarson BE, Holzkämper S and Wilmking M (2023) To extract or not to extract? Influence of chemical extraction treatment of wood samples on element concentrations in tree-rings measured by X-ray fluorescence. Front. Environ. Sci. 11:1031770. doi: 10.3389/fenvs.2023.1031770
Received: 02 September 2022; Accepted: 31 January 2023;
Published: 13 February 2023.
Edited by:
Oladele Ogunseitan, University of California, Irvine, United StatesReviewed by:
Constantin Nechita, National Institute for research and Development in Forestry Marin Dracea (INCDS), RomaniaCopyright © 2023 Scharnweber, Rocha, González Arrojo, Ahlgrimm, Gunnarson, Holzkämper and Wilmking. This is an open-access article distributed under the terms of the Creative Commons Attribution License (CC BY). The use, distribution or reproduction in other forums is permitted, provided the original author(s) and the copyright owner(s) are credited and that the original publication in this journal is cited, in accordance with accepted academic practice. No use, distribution or reproduction is permitted which does not comply with these terms.
*Correspondence: T. Scharnweber, dG9iaWFzLnNjaGFybndlYmVyQHVuaS1ncmVpZnN3YWxkLmRl
Disclaimer: All claims expressed in this article are solely those of the authors and do not necessarily represent those of their affiliated organizations, or those of the publisher, the editors and the reviewers. Any product that may be evaluated in this article or claim that may be made by its manufacturer is not guaranteed or endorsed by the publisher.
Research integrity at Frontiers
Learn more about the work of our research integrity team to safeguard the quality of each article we publish.