- 1Escuela Superior Politécnica del Litoral, Escuela Superior Politécnica del Litoral, Centro del Agua y Desarrollo Sustentable, Campus Gustavo Galindo, Guayaquil, Ecuador
- 2Departamento de Biología, Facultad Experimental de Ciencias, Universidad del Zulia, Maracaibo, Venezuela
- 3Grupo de Investigación en Biodiversidad y Ecología de Sistemas Acuáticos, Departamento de Acuicultura, Pesca y Recursos Naturales Renovables, Facultad de Acuicultura y Ciencias del Mar (FACM), Universidad Técnica de Manabí, Bahíade Caráquez, Ecuador
- 4Grupo de Investigación en Sanidad Acuícola, Inocuidad y Salud Ambiental, Departamento de Acuicultura, Pesca y Recursos Naturales Renovables, Facultad de Acuicultura y Ciencias del Mar (FACM), Universidad Técnica de Manabí, Bahíade Caráquez, Ecuador
- 5Laboratory of Limnology, Center for Agrarian Sciences, Plant Technology and Environmental Sciences Department, Federal University of Paraíba, Paraíba, Brazil
- 6Department of Zoology, School of Biology, Aristotle University, Thessaloniki, Greece
- 7Department of Hydrobiology, University of Białystok, Bialystok, Poland
- 8Department of Biological Sciences, Northern Kentucky University, Highland Heights, KY, United States
Introduction: La Segua is an important wetland in Ecuador, recognized as a Ramsar site and of great ecological relevance. Currently, La Segua is threatened by human pressures and unsustainable landscape use associated with agriculture and aquaculture. This study is the first approach to the occurrence of suspended microplastics (SMPs) in an Ecuadorian lentic waterbody and the estimated potential threat of these on the filter and suspension feeders in aquatic food webs.
Methods: Surface water samples were collected at 16 sampling points in the wetland to analyze the occurrence, concentration, and horizontal distribution of Suspected Suspended Microplastics (SSMPs) and zooplankton.
Results and discussion: SSMPs were found in all sampling sites, and concentrations were between 313 and 490 particles/liter. Fragments were the most abundant category, followed by fibers, films, and pellets. The highest concentration of SSMPs was found in sampled sites on the east coast and in the middle of the wetland. The frequency and concentration of categories of SSMps found in surface water largely reflect the reality of local human activity around the La Segua wetland. Only rotifers and copepod nauplii were found in the zooplankton. The SSMMPs to zooplankton ratio varied between 0.3 and 441 particles/individuals.The SSMPs/zooplankton ratio indicates that fish and other filter and suspension feeders of aquatic food webs could be more likely to feed on suspended microplastics than natural food items around the wetland. SSMPs indicate that microplastics may represent.
Introduction
Most studies dealing with microplastic pollution in aquatic ecosystems are focused on the world’s oceans (Wu et al., 2019; Talbot & Chang, 2022), and little is known about freshwater ecosystems (Niaounakis, 2017; Blettler et al., 2018; Lambert & Wagner, 2018). Οnly about 18% of microplastic-related studies are associated with freshwaters (Blettler et al., 2018; Li and Liu, 2018), mainly reporting on large surface water bodies (Wagner et al., 2014). According to Li et al. (2020), the knowledge of the impact of microplastic pollution in freshwater environments is still in its infancy when compared to that of marine environments. Freshwater studies are biased toward rivers (Talbot & Chang, 2022).
The relatively few existing studies demonstrate the magnitude of this emerging environmental pollution (Wagner et al., 2014; Horton et al., 2017; Eerkes-Medrano, 2018; Li and Liu, 2018) and draw attention to the problem that is now closer to people (Niaounakis, 2017) and has a more global character (Free et al., 2014; Zhang et al., 2016; Shahul Hamid et al., 2018).
Particularly in Neotropical regions, the knowledge of microplastic pollution in inland water bodies is incipient (Alfonso et al., 2020; Li et al., 2020; Orona-Návar et al., 2022). The studies in this region barely represent 11.8% of the total published papers on the pollution by microplastics in inland water bodies, and these are concentrated only on Argentina, Brazil, Colombia, and Chile (Blettler et al., 2018). Only one study on this issue in Ecuadorian freshwater bodies is known (Donoso & Ríos-Touma, 2020).
Due to their small size, suspended microplastics (SMPs) are potentially bioavailable, via ingestion, to a wide range of filter-feeding consumers and suspended particulate matter feeders, whose food size range overlaps with the suspended microplastic size (Scherer et al., 2018; Botterell et al., 2019). Microplastic ingestion has been observed in freshwater invertebrates and fish (Wagner et al., 2014; Jeong et al., 2016; Ziajahromi et al., 2017; Sarijan et al., 2018, Sherer et al., 2018). The existing studies suggest that the ingestion of microplastics by fish can be harmful and produce detrimental effects (Silva-Cavalcanti et al., 2017; Raza, 2018). Also, microplastics can adsorb bacteria, viruses, or other pollutants (e.g., heavy metals and organic pollutants) (Rodrigues et al., 2019; Fred-Ahmadu et al., 2020; Santos-Echeandía et al., 2020). Therefore, microplastic pollution may represent a potential risk for human consumption (Rochman et al., 2015; Wright & Kelly, 2017; Joon, 2019), the commercial value of fisheries (Vázquez-Rowe et al., 2021; Masiá et al., 2022), aquaculture activities (Chen et al., 2021; Zhou et al., 2021), and other ecosystem services of water bodies (Prata et al., 2021; Sridharan et al., 2021).
This paper presents an empirical assessment of SMPs and the threat that represents to filter and suspended particulate matter feeders in a freshwater wetland in a dry coastal region of Ecuador. For this assessment, we used only visual examination of microplastics by microscopy and the ratio between microplastics and zooplankton.
Visual examination of microplastics by microscopy is commonly used to quantify and characterize microplastics. It has been used as either a stand-alone tool or as a first step for analysis (Lusher et al., 2020; Kotar et al., 2022). Limitations related to the size and nature of the particles may affect the identification of microplastics, and extra steps for chemical characterization have been included for more accuracy. Additional techniques include Fourier transform infrared (FTIR) spectroscopy, Raman spectroscopy, and pyrolysis–gas chromatography with mass spectrometry (Py-GCMS) (Cowger et al., 2020; Primpke et al., 2020). Some studies skip microscopy entirely and fully automate spectroscopy via scanning and mapping (Primpke et al., 2020).
These additional protocols make laboratory procedures more complicated. They are an important limitation for most tropical countries, where research funds, availability of trained technicians, and lack of modern equipment can result in great obstacles to be overcome. This is particularly true in African (Robarts & Zohary, 2018) and Latin American countries (Orova-Návar et al., 2022). Throughout this paper, we will refer to the suspended microplastic particles examined as suspected suspended microplastics (SSMPs) because chemical determination analyses nor the “hot needle test” was performed to confirm the nature of the particles.
On the other hand, the ratio of suspended microplastics/zooplankton indicates the degree of interaction between microplastics and zooplankton. This ratio permits the estimation of how microplastic contamination levels may affect organisms that feed on suspended particles in aquatic food webs (Collignon et al., 2014). A high ratio of microplastics to zooplankton implicates a relatively high number of microplastics that may cause visual confusion with natural food for filter and suspension consumers (Rose, 2019).
This empirical approach has been frequently used in marine environments (Moore et al., 2001; 2002; Collignon et al., 2012; 2014; Frias et al., 2014; Gorokhova, 2015; Panti et al., 2015; Di Mauro et al., 2017; Vasilopoulou et al., 2021), but, to the best of our knowledge, it never has been used in freshwater environments.
Considering the gaps in the knowledge of microplastics in Neotropical freshwater environments (Orona-Návar et al., 2022) and only visual examination as the first step to assess microplastic pollution (Kotar et al., 2022), we try to address the following questions:
1. What leads to the occurrence of SSMPs and how are these associated with zooplankton abundances throughout the wetland?
2. What are the categories of SSMPs and can these give information on the origin/sources of the material?
3. Can microplastic pollution threaten the wetland’s food webs and ecosystem services?
Study site
La Segua is a freshwater wetland located in the coastal region of Ecuador, northeast of Manabí Province (0° 42.5′–0° 44.3′S and 80° 09′–80° 12.2′W) (Figure 1). It has a total area of 18.36 km2 and an altitude of 5 m.a.s.l. La Segua consists of a central swamp, which forms a large floodplain covered by water in the rainy months (December–April) (IUCN, 2000). Its terrestrial and aquatic fauna has become a regional economic engine with commercial fisheries and aquaculture activities (Montilla-Pacheco, 2017). The types of soil found in this region are sandy, silty, and/or clay-silty, with deep sedimentary deposits. A total of 164 bird species are temporary or permanent residents of this wetland, representing a high potential for the development of tourist activities, for e.g., bird watching (Montilla-Pacheco, 2017).
This freshwater body is the fifth most important wetland in Ecuador, and since 2000, it has been designated as a Ramsar site for its environmental and ecological importance. Even though the wetland is covered by the Ramsar Convention (www.ramsar.org), it has complex problems related to eutrophication and the use of agrochemicals, soil pollution, increased sedimentation, reduced water levels, and changes in biodiversity, especially in the ichthyofauna which seems to be suffering a marked decrease in their diversity (Montilla-Pacheco, 2017; Noles et al., 2017). At present, 5–12 fish species have been identified in this area, and the native Dormitator latifrons (Richardson) and invaders Oreochromis niloticus L. and O. mossambicus (Peters) constitute the base of artisanal fisheries and aquaculture activities, which are the means of livelihood for the inhabitants of the surrounding area (Montilla-Pacheco, 2017; Noles et al., 2017). Both species are highly vulnerable to microplastic pollution because they are considered omnivorous and filter-feeding (Basto-Rosales et al., 2020; Vega-Villasante et al., 2021).
Methods
Sampling procedure
Surface water samples to analyze both zooplankton and SSMPs were collected at 16 sampling points from the wetland on 23 April 2019 (Figure 1). For quantitative plankton samples, 40 L of surface water collected using a metal bucket at a depth of about 20 cm was filtered through a 65-μm plankton net. All the samples were preserved in glass bottles with metal lips filled with Lugol’s solution.
In the laboratory, the samples were fixed with a formalin solution (4% final concentration) and filtered through a 5-mm metal sieve to remove larger particles. The contamination risks were avoided as far as possible during the laboratory procedures following suggestions from Vasilopoulou et al. (2021), such as cleaning all the materials used with bi-distilled water (i.e., glass pipettes, glass–metal counting cameras, and steel needles) and by working under controlled conditions while wearing cotton lab coats and nitrile gloves. The samples were covered under a glass camera (inverted glass aquariums), except during microscopic investigation. Airborne contamination was not estimated.
Temperature (T, oC), pH, total suspended solids (TSSs, mg l−1), and conductivity (Cond, μS.cm−1) of water collected using a bucket were measured simultaneously with plankton collection using a multiparameter portable probe (PCSTestr 35) without cables. Site sampling depth (D, m) was measured using a marked rod. Water transparency (t, m) was estimated using a Secchi disk (Cole, 1994).
Identification and counting
To separate the SSMPs from zooplankton, samples in a formalin solution with 4% final concentration were sedimented for 24 h in 1-L graduated glass cylinders and washed out to prevent particles from sticking to the side and top of the cylinder under a glass camera to pervert air contamination before microscopic analysis. The top and bottom portions were observed in a Sedwick–Rafter chamber under an Olympus CX photo-microscope at 40 × magnification with a light background. These represent particles with less densities.
SSMPs were picked using a glass pipette and were categorized into fragments, fibers (monofilament line), films, and pellets, according to Li et al. (2014). SSMPs with slender and greatly elongated shapes were defined as fibers; SSMPs with a spherical shape were defined as pellets; SSMPs with a small and very thin layer were defined as films; and an isolated or incomplete part of a large plastic was defined as a fragment. No color or size analyses were performed.
Zooplankton individuals were picked up from the bottom portion using a glass pipette and were identified under the microscope only to group into levels (i.e., rotifers and copepod nauplius), while the other particles were not counted. SSMPs and zooplankton were counted separately. In both cases, as many cameras as possible were used to reach a sufficient total count of 300 or more SSMPs or zooplanktons.
Data analysis
For each sample, the occurrence of zooplankton and SSMPs, concentration (particles/liter) of SSMP categories and abundance of zooplankton groups (individuals/liter), and the ratio of SSMPs to zooplankton were estimated using the principle of the ratio SMPs/zooplankton (Moore et al., 2001; 2002; Collignon et al., 2014). Pearson’s correlation analysis (r) was performed to assess the relations between zooplankton abundance, rotifer abundance, nauplius abundance, total concentration of SSMPs, concentration of each category of SSMPs, and each environmental variable. The analyses were carried out in R version 3.5.1 (R Core Team, 2018).
Results
At the sampling time, the water depth varied from 1.0 to 2.1 m. Higher conductivity and total suspended solids were found in sites located in the middle of the wetland. The water transparency remained below 0.8 m in all sampled points (Table 1).
SSMPs were found in all sites (Figure 2). The concentration of these particles ranged from 313 to 490 particles/liter, showing small variations throughout the wetland (Figure 2) with an average value of 384.3 ± 52.0 particles/liter. The highest concentration of SSMPs was recorded at points located near the west coast and in the middle of the wetland (Figure 2).
The categories of SSMPs in surface water are shown in Figure 3. These categories vary between 1 and 4, with an average value of 2.6 ± 0.9 categories of SSMPs per sample. Fragments were the most abundant and frequent category, followed by fibers and films, while pellets were the least represented. Selected photographs of fragments and fibers are shown in Supplementary Material S1.
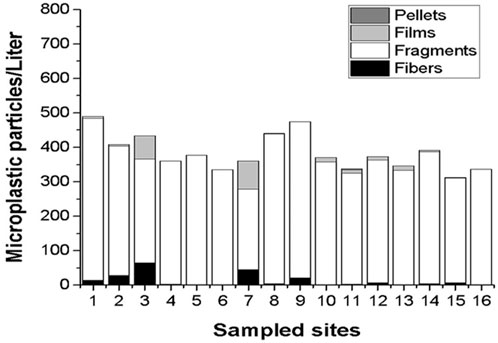
FIGURE 3. Horizontal variations of pellets, films, fragments, and fibers in sampling sites of the La Segua wetland.
Zooplankton was present in 68.8% of the samples (Figure 2) and was represented only by rotifers and copepod nauplii. Zooplankton abundance ranged from 1 to 1,182 individuals/liter, and the number of taxa was between 1 and 8. The highest abundance and number of taxa were observed toward the east coast and in the middle zone of the wetland (Figure 2).
The SSMP-to-zooplankton ratio varied between 0.3 and 441 particles/individuals (Figure 2), except in one sampling site, where the concentration of SSMPs markedly exceeded the abundance of zooplankton. The correlation between SSMP concentration, the concentration of each category of SSMPs, zooplankton abundance and rotifers and nauplius abundance, and each environmental variable was not significant (p>0.44).
Discussion
Although the procedures used included accuracy improvements related to the use of volumetric samples and not nets, where the determination of the filtered volume is usually inaccurate (Moore et al., 2001; Collignon et al., 2012; 2014; Frias et al., 2014; Gorokhova, 2015; Panti et al., 2015; Di Mauro et al., 2017; Vasilopoulou et al., 2021), the results found can be considered an underestimate of the concentrations of SSMPs in the wetland. This is because we did not use filtration, which would have allowed the identification of smaller particles not detectable by optical microscopy (Lindeque et al., 2020), and only less-dense particles were counted. However, we also did not use acid digestion or “hot needle test,” to avoid confusion between plastic particles and other types of particles, and other analytical techniques that would identify microplastics (for example, SEM/EDS) such as infrared (IR), Raman spectroscopy, and pyrolysis–gas chromatography with mass spectrometry (Py-GCMS) (Cowger et al., 2020; Primpke et al., 2020). Therefore, overestimation values should also be expected by using only visual examination by microscopy (Kotar et al., 2022).
Taking these biases into account, our results showed the occurrence of SSMPs in a range of concentrations (>300 particles/liter) and indicated that SSMPs may highly pollute La Segua. The recorded concentrations of microplastics in freshwater systems worldwide are less than 1–270 particles/liter (e.g., Horton et al., 2017; Wu et al., 2019; Ríos Mendoza, 2020). Because one of the greatest difficulties that currently exist is the heterogeneity of the methods used for the collection and identification of microplastics, the results of the concentration of microplastics must be compared with great care.
The results showing the predominance of fragments suggested that urban population activities are the main source of SSMP pollution in this water body (Kumar et al., 2021). Fragments are secondary particles derived from the degradation of larger plastic products (Wu et al., 2019) by photodegradation (UV radiation) and physical abrasion, which produces small particles that fall into the micro- or even nanoparticle size ranges (Ríos Mendoza, 2020). Origins of fragments include the breakdown of plastic bags, bottles, cups, food wraps, plastic films used to cover agricultural soil and crops, foam insulation materials, and automobile tires (Duis & Coors, 2016; Lambert & Wagner, 2018; Ríos Mendoza, 2020). This microplastic category has been linked to waste from regions with high urban population densities (Faure et al., 2012; Tang et al., 2021) and low urban population densities, where waste management is incipient or non-existent (Free et al., 2014; Tang et al., 2021). In the case of the towns of Chone and Tosagua, located upriver of La Segua wetland, populations are relatively low, but there are only sanitary landfills and open dumps as solid waste management treatments. One of the environmental problems threatening the La Segua wetland ecosystem is the dumping of solid waste (Smith & Maltby, 2003). Government reports indicate that 70% of the houses of the four communities that reside on the margins of the wetland burn or bury the garbage, while the remaining 30% deposit it in landfills. According to He et al. (2019) and Golwala and Zhang (2021), landfills are the major repository and disseminators of microplastics, including primary and secondary microplastics. Therefore, around La Segua, sources of plastic contamination can be exacerbated. Plastics may be dragged into the wetland mainly by runoff waters and tributary rivers and wind and are deposited and degraded in the wetland.
Fishery activities in the area may also produce some significant sources of plastic debris, such as polystyrene buoys, nylon ropes, and fragments of lines or gill nets (Cardozo et al., 2018; Xue et al., 2020; Zhan et al., 2021). Similarly, in La Segua, nylon ropes and buoys are used extensively in aquaculture activities and can be lost, inappropriately discarded, or damaged during use. Therefore, categories of SSMPs found in the water surface largely reflect the reality of local human activity around La Segua wetland.
These results of the occurrence and identification of sources of microplastics demonstrated the attributes of the present empirical assessment as a suitable and relatively simple approach and a first step to assess microplastics floating in shallow water bodies, especially in regions with poor research funds, no modern equipment, and limited specialized personnel, as in most tropical countries (Robarts & Zohary, 2018; Orova-Návar et al., 2022). Recent studies by Kotar et al. (2022) indicated that despite the limitations in characterizing microplastics of smaller sizes (less than 20 µm), the visualization by microscopy alone can result in relatively good quantification and characterization of larger-sized microplastics. The microscopical characterization of microplastics in combination with a ratio of zooplankton/SSMPs is a relatively inexpensive, reliable, and widely accessible method. In addition, the method can provide estimates of SSMP concentration, size, color, and morphology, indicate about the risk to wildlife (Bucci & Rochman, 2022), and provide information on source apportionment (Zhu et al., 2021).
The high SSMPs/zooplankton ratio of only SSMP particles in some sampled sites suggested that fishes and other filter or suspension feeders in the food web could be more likely to feed on suspended microplastics than natural planktonic prey around the whole wetland. The small size of microplastic particles and their appealing coloration and buoyancy allow for easy picking and ingestion by fish (Jovanović, 2017; Kumar et al., 2020). Fish are visual predators and are more likely to confuse particles and prey items. Laboratory experiments indicate that fish larvae feed preferentially on plastic particles when exposed to microplastics and natural food (De Sá et al., 2015).
Aquatic invertebrates such as protozoans, rotifers, cladocerans, and mussels, being suspension and filter feeders, are assumed to be especially prone to microplastic ingestion, as shown in numerous studies (Scherer et al., 2018; Wong et al., 2020). Moreover, both fish and invertebrates may unintentionally ingest microplastics if microplastics are already present inside or adhered to their prey (Jovanović, 2017; Wong et al., 2020). Particularly, D. latifrons and tilapia species, found in La Segua are considered omnivorous and filter-feeding (Basto-Rosales et al., 2020; Vega-Villasante et al., 2021). These conditions do not allow them to have optical selectivity on their prey, suggesting that microplastic ingestion is predominantly accidental. Filter-feeding fishes are generally believed to be more susceptible to microplastic ingestion due to their unselective feeding strategy (Wesch et al., 2016; Mizraji et al., 2017). Additionally, omnivorous fish, because they consume a wide variety of resources, have increased chances of intaking microplastics actively or accidentally (Mizraji et al., 2017). Therefore, the SSMP ingestion would be expected in these fish populations, as recently found by Isea et al. (2022). These authors reported the stomach contents of D. latifrons specimens collected in La Segua and found a frequency of occurrence (FO) of plastics of 50% and a plastic load (PL) between 5 and 55 particles per individual. They also reported that 97% of plastic particles were microplastics (less than 5.0 mm) and 3% were larger. Similarly, high values of FO and PL have been found in tilapia specimens (Acosta, unpublished data.)
According to Parker et al. (2021), controlled microplastic exposure studies highlight various effects on fish physiology, biochemistry, and behavior that are often complex, unpredictable, species-specific, and non-linear concerning dose–response relationships. Egestion is typically rapid and effective, although particles of a particular shape and/or size may remain or translocate across the intestinal wall to other organs via blood.
In the La Segua wetland, microplastic pollution may be an evident threat to the stability and efficiency of food webs involving filter and suspension feeders as trophic components. It may negatively affect fisheries and aquaculture activities and other ecosystem services. According to Markic et al. (2019), the impact of the ingestion of plastics is due to physical effects, toxicological effects, and the combination of these with other local stressors. Various types of pollutants in water, such as antibiotics (Quan et al., 2019), polycyclic aromatic hydrocarbons (Zhang S. et al., 2019; Zhang P. et al., 2019; Zhang W. et al., 2019), heavy metals (Tang N. et al., 2020; Tang S. et al., 2020; Dong et al., 2020; Guo et al., 2020) and organic compounds (Tang et al., 2021), and bacteria and viruses (Rodrigues et al., 2019; Fred-Ahmadu et al., 2020; Santos-Echeandía et al., 2020), are adsorbed by microplastics in water. The La Segua wetland faces complex environmental problems related to multiple local stressors, such as the use of pesticides, fertilizers, and the absence of solid and water waste management (Montilla-Pacheco, 2017; Noles et al., 2017). Microplastics add to the potential health risk posed by these environmental conditions to human consumers and to other components of the aquatic food web (Huang et al., 2021; Rahman et al., 2021).
The results, as a first step to understanding microplastic pollution and its threat to food webs in La Segua, indicated that high microplastic pollution associated with anthropogenic activities may be affecting food webs. However, additional and more complete studies are needed: a. Visual examination of microplastics must be complemented with other protocols to increase data accuracy, such as chemical analyses (Kotar et al., 2022) and other procedures that allow the inclusion of particles smaller than 65 μm, microplastic particles of higher density, and their distinction from other types of particles. b. Size and color must be examined to understand their origins and pathways. c. Spatial variation must be analyzed because they may be affected by physical watershed characteristics such as slope and elevation (Talbot & Chang, 2022; Talbot et al., 2022). d. Temporal variables of influence include wind, precipitation, and stormwater runoff, and water flow/discharge must be estimated (Talbot & Chang, 2022; Talbot et al., 2022). Both c and d would allow us to explain the variations in the present study’s horizontal distribution and identify the pathways in the wetland. e. The presence of plastics in sediments and different components of the aquatic biota must be assessed (Bhutto & You, 2022; Yıldız et al., 2022).
Data availability statement
The original contributions presented in the study are included in the article/Supplementary Materials; further inquiries can be directed to the corresponding author.
Author contributions
Conception or design of the work: CL, AS-P, YC-Q, LS, and LD-G. Data collection: CL, VA, LS, AS-P, and YC-Q. Data analysis and interpretation: CL, VA, LG-B, LD-G, GS, EM, and MS-K. Drafting of the article: CL, VA, LS, LG-B, LD-G, GS, EM, and MS-K. Critical revision of the article: CL, EM, MK, GS, LG-B, and MS-K. Final approval of the version to be published: CL, VA, LS, AS-P, YC-Q, GS, LG-B, MK, EM, LD, and MS-K.
Funding
This study was funded by the contribution of the Universidad Técnica de Manabí (UTM), who provided financial support to the project “Aspectos biológicos del chame Dormitator latifrons en ambientes naturales y de producción”. A permit collection (No. 004-2019-DP-DPAM-MAE) was issued by the Ministry of Environment (Ministerio de Agua, Ambiente y Transición, Ecuador) of Ecuador. This paper is a product of the project CADS-11-2021 supported by the Escuela Superior Politécnica del Litoral (ESPOL) to CL. This work was carried out within the framework of the Interinstitutional General Agreement for Technical and Scientific Cooperation between the ESPOL and the UTM on 09-19-2020.
Acknowledgments
The authors thank two reviewers whose comments on the first draft of the manuscript helped improve it considerably. The authors also thank W. Lafuente, K. Mendieta, and J. Vidal for their help in the field and laboratory work.
Conflict of interest
The authors declare that the research was conducted in the absence of any commercial or financial relationships that could be construed as a potential conflict of interest.
Publisher’s note
All claims expressed in this article are solely those of the authors and do not necessarily represent those of their affiliated organizations, or those of the publisher, the editors, and the reviewers. Any product that may be evaluated in this article, or claim that may be made by its manufacturer, is not guaranteed or endorsed by the publisher.
Supplementary material
The Supplementary Material for this article can be found online at: https://www.frontiersin.org/articles/10.3389/fenvs.2023.1028970/full#supplementary-material
SUPPLEMENTARY MATERIAL S1 | Selected photographs of fibers and fragments in SSMPs collected in La Segua.(A) Transparent fiber, (B) Short blue fiber (C) Long blue Fiber, and (D) fragment.
References
Alfonso, M. B., Scordo, F., Seitz, C., Manstretta, G. M., Ronda, A. C., Arias, A. H., et al. (2020). First evidence of microplastics in nine lakes across Patagonia (South America). Sci. Total Environ. 1, 139385. doi:10.1016/j.scitotenv.2020.139385
Basto-Rosales, M. E., Carrillo-Farnés, O., Montoya-Martínez, C. E., Badillo-Zapata, D., de Oca, G. G., Álvarez-González, C. A., et al. (2020). Meat protein quality of dormitator latifrons (pisces: Eleotridae): Arguments for use by rural communities. Ecosistemas Recur. Agropecu. 7 (1), e2172. doi:10.19136/era.a7n1.2172
Bhutto, S., and You, X. (2022). Spatial distribution of microplastics in Chinese freshwater ecosystem and impacts on food webs. Environ. Pollut. 293, 118494. doi:10.1016/j.envpol.2021.118494
Blettler, M. C., Abrial, E., Khan, F. R., Sivri, N., and Espinola, L. A. (2018). Freshwater plastic pollution: Recognizing research biases and identifying knowledge gaps. Water Res. 143 (15), 416–424. doi:10.1016/j.watres.2018.06.015
Blettler, M. C., Garello, N., Ginon, L., Abrial, E., Espinola, L. A., and Wantzen, K. M. (2019). Massive plastic pollution in a mega-river of a developing country: Sediment deposition and ingestion by fish (Prochilodus lineatus). Environ. Pollut. 1, 113348. doi:10.1016/j.envpol.2019.113348
Botterell, Z. L., Beaumont, N., Dorrington, T., Steinke, M., Thompson, R. C., and Lindeque, P. K. (2019). Bioavailability and effects of microplastics on marine zooplankton: A review. Environ. Pollut. 245, 98–110. doi:10.1016/j.envpol.2018.10.065
Bucci, K., and Rochman, C. M. (2022). Microplastics: A multidimensional contaminant requires a multidimensional framework for assessing risk. Microplastics Nanoplastics 2, 7. doi:10.1186/s43591-022-00028-0
Cardozo, A. L., Farias, E. G., Rodrigues-Filho, J. L., Moteiro, I. B., Scandolo, T. M., and Dantas, D. V. (2018). Feeding ecology and ingestion of plastic fragments by Priacanthus arenatus: What's the fisheries contribution to the problem? Mar. Pollut. Bull. 130, 19–27. doi:10.1016/j.marpolbul.2018.03.010
Chen, G., Li, Y., and Wang, J. (2021). Occurrence and ecological impact of microplastics in aquaculture ecosystems. Chemosphere 274, 129989. doi:10.1016/j.chemosphere.2021.129989
Coello, S., Vinueza, D., Echeverría, M. F., Cisneros, F., Astudillo, Herrera, J., Cervantes, E., et al. (2009). Diagnóstico ambiental de las cuencas de los ríos Chone y Portoviejo. Inf. Prep. el Minist. del Ambiente. Ecobiotec del Ecuador.
Collignon, A., Hecq, J. H., Galgani, F., Collard, F., and Goffart, A. (2014). Annual variation in neustonic micro-and meso-plastic particles and zooplankton in the Bay of Calvi (Mediterranean – corsica). Mar. Pollut. Bull. 79, 293–298. doi:10.1016/j.marpolbul.2013.11.023
Collignon, A., Hecq, J. H., Glagani, F., Voisin, P., Collard, F., and Goffart, A. (2012). Neustonic microplastic and zooplankton in the north western mediterranean sea. Mar. Pollut. Bull. 64, 861–864. doi:10.1016/j.marpolbul.2012.01.011
Cowger, W., Booth, A. M., Hamilton, B. M., Thaysen, C., Primpke, S., Munno, K., et al. (2020). Reporting guidelines to increase the reproducibility and comparability of research on microplastics. Appl. Spectrosc. 74, 1066–1077. doi:10.1177/0003702820930292
de Sá, L. C., Luís, L. G., and Guilhermino, L. (2015). Effects of microplastics on juveniles of the common goby (pomatoschistus microps): Confusion with prey, reduction of the predatory performance and efficiency, and possible influence of developmental conditions. Environ. Pollut. 196, 359–362. doi:10.1016/j.envpol.2014.10.026
Di Mauro, R., Kupchik, M. J., and Benfield, M. C. (2017). Abundant plankton-sized microplastic particles in shelf waters of the northern Gulf of Mexico. Environ. Pollut. 230, 798–809. doi:10.1016/j.envpol.2017.07.030
Dong, Y., Gao, M., Song, Z., and Qiu, W. (2020). As (III) adsorption onto different-sized polystyrene microplastic particles and its mechanism. Chemosphere 239, 124792. doi:10.1016/j.chemosphere.2019.124792
Donoso, J. M., and Rios-Touma, B. (2020). Microplastics in tropical andean rivers: A perspective from a highly populated Ecuadorian basin without wastewater treatment. Heliyon 6, e04302. doi:10.1016/j.heliyon.2020.e04302
Duis, K., and Coors, A. (2016). Microplastics in the aquatic and terrestrial environment: Sources (with a specific focus on personal care products), fate and effects. Environ. Sci. Eur. 28, 2. doi:10.1186/s12302-015-0069-y
Eerkes-Medrano, D. (2018). Thompson R. ¨Occurrence, fate, and effect of microplastics in freshwater systems,¨ in Microplastic contamination in aquatic environments in Zeng E Y. Elsevier, 95–132. doi:10.1016/B978-0-12-813747-5.00004-7
Faure, F., Corbaz, M., Baecher, H., and De Alencastro, L. (2012). Pollution due to plastics and microplastics in lake geneva and in the mediterranean. Sea Arch. Sci. 65, 157–164.
Fred-Ahmadu, O. H., Bhagwat, G., Oluyoye, I., Benson, N. U., Ayejuyo, O. O., and Palanisami, T. (2020). Interaction of chemical contaminants with microplastics: Principles and perspectives. Sci. Total Environ. 706, 135978. doi:10.1016/j.scitotenv.2019.135978
Free, C. M., Jensen, O. P., Mason, S. A., Eriksen, M., Williamson, N. J., and Boldgiv, B. (2014). High-levels of microplastic pollution in a large, remote, mountain lake. Mar. Pollut. Bull. 85, 156–163. doi:10.1016/j.marpolbul.2014.06.001
Frias, J., Gago, J., Otero, V., and Sobral, P. (2016). Microplastics in coastal sediments from Southern Portuguese shelf waters. Environ, 114. doi:10.1016/j.marenvres.2015.12.006
Frias, J. P., Otero, V., and Sobral, P. (2014). Evidence of microplastics in samples of zooplankton from Portuguese coastal waters. Mar. Environ. Res. 95, 89–95. doi:10.1016/j.marenvres.2014.01.001
Golwala, H., Zhang, X., Iskander, S. M., and Smith, A. L. (2021). Solid waste: An overlooked source of microplastics to the environment. Sci. Total Environ. 769, 144581. doi:10.1016/j.scitotenv.2020.144581
Gorokhova, E. (2015). Screening for microplastic particles in plankton samples: How to integrate marine litter assessment into existing monitoring programs? Mar. Pollut. Bull. 99, 271–275. doi:10.1016/j.marpolbul.2015.07.056
Guo, X., Hu, G., Fan, X., and Jia, H. (2020). Sorption properties of cadmium on microplastics: The common practice experiment and a two-dimensional correlation spectroscopic study. Ecotox Environ. Saf. 190, 110118. doi:10.1016/j.ecoenv.2019.110118
He, P., Chen, L., Shao, L., Zhang, H., and Lü, F. (2019). Municipal solid waste (msw) landfill: A source of microplastics? Evidence of microplastics in landfill leachate. Water Res. 159, 38–45. doi:10.1016/j.watres.2019.04.060
Horton, A. A., Svendsen, C., Williams, R. J., Spurgeon, D. J., and Lahive, E. (2017). Large microplastic particles in sediments of tributaries of the River Thames, UK–Abundance, sources and methods for effective quantification. Mar. Pollut. Bull. 114, 218–226. doi:10.1016/j.marpolbul.2016.09.004
Huang, W., Song, B., Liang, J., Niu, Q., Zeng, G., Shen, M., et al. (2021). Microplastics and associated contaminants in the aquatic environment: A review on their ecotoxicological effects, trophic transfer, and potential impacts to human health. J. Hazard Mater 405, 124187. doi:10.1016/j.jhazmat.2020.124187
Isea, F., Quispe, J. D. F., Bermúdez-Medranda, A., Acosta, V., Santana-Piñeros, A., Cruz-Quintana, Y., et al. (2022). Plastics ingestion in a wild population of the Pacific fat sleeper (Dormitator latifrons). Pac Sci. 76 (4) (Acccepted.
Jeong, C. B., Won, E. J., Kang, H. M., Lee, M. C., Hwang, D. S., Hwang, U. K., et al. (2016). Microplastic size-dependent toxicity, oxidative stress induction, and p-JNK and p-p38 activation in the monogonont rotifer (Brachionus koreanus). Environ. Sci. Technol. 50, 8849–8857. doi:10.1021/acs.est.6b01441
Joon, M. (2019). Trophic transfer of microplastics in zooplanktons towards its speculations on human health: A review. J. Biomed. Ther. Sci. 6, 814.
Jovanović, B. (2017). Ingestion of microplastics by fish and its potential consequences from a physical perspective. Integr. Environ. Assess. Manag. 13, 510–515. doi:10.1002/ieam.1913
Kotar, S., McNeish, R., Murphy-Hagan, C., Renick, V., Lee, C. F., Steele, C., et al. (2022). Quantitative assessment of visual microscopy as a tool for microplastic research: Recommendations for improving methods and reporting. Chemosphere 14, 136449. doi:10.1016/j.chemosphere.2022.136449
Kumar, P., Gore, P. M., Magisetty, R., Kandasubramanian, B., and Shunmugam, R. (2020). Poly (1, 6-heptadiyne)/ABS functionalized microfibers for hydrophobic applications. J. Polym. Res. 27, 14. doi:10.1007/s10965-019-1981-4
Kumar, R., Sharma, P., and Bandyopadhyay, S. (2021). Evidence of microplastics in wetlands: Extraction and quantification in Freshwater and coastal ecosystems. J. Water Process Eng, 40. doi:10.1016/j.jwpe.2021.101966
Lambert, S., and Wagner, M. (2018). “Microplastics are contaminants of emerging concern in freshwater environments: An overview,” in Freshwater microplastics. The handbook of environmental chemistry. Editors M. Wagner, and S. Lambert 58 Series Editors (Damià Barceló and Andrey G. Kostianoy. Springer Open). doi:10.1007/978-3-319-61615-5
Li, C., Busquets, R., and Campos, L. C. (2020). Assessment of microplastics in freshwater systems: A review. Sci. Total Environ. 707, 135578. doi:10.1016/j.scitotenv.2019.135578
Li, J., Liu, H., Liu, S., Huang, G., and Yang, H. H. (2018). Chen, jp microplastics in freshwater systems: A review on occurrence, environmental effects, and methods for microplastics detection. Water Res. 120, 137–143. doi:10.1016/j.bios.2018.08.046
Lindeque, P. K., Cole, M., Coppock, R. L., Lewis, C. N., Miller, R. Z., Watts, A. J., et al. (2020). Are we underestimating microplastic abundance in the marine environment? A Comp. microplastic capture nets Differ. mesh-size Environ Pollut 265, 114721. doi:10.1016/j.envpol.2020.114721
Lusher, A. L., Bråte, I. L., Munno, K., Hurley, R. R., and Welden, N. A. (2020). Is it or isn't it: The importance of visual classification in microplastic characterization. Appl. Spectrosc. 74, 1139–1153. doi:10.1177/0003702820930733
Markic, A., Gaertner, J. C., Gaertner-Mazouni, N., and Koelmans, A. A. (2019). Plastic ingestion by marine fish in the wild. Crit. Rev. Env. Sci. Tec. 50, 657–697. doi:10.1080/10643389.2019.1631990
Masiá, P., Mateo, J. L., Arias, A., Bartolomé, M., Blanco, C., Erzini, K., et al. (2022). Potential microplastics impacts on African fishing resources. Sci. Total Environ. 806, 150671. doi:10.1016/j.scitotenv.2021.150671
Mizraji, R., Ahrendt, C., Perez-Venegas, D., Vargas, J., Pulgar, J., Aldana, M., et al. (2017). Is the feeding type related with the content of microplastics in intertidal fish gut. Mar. Pollut. Bull. 116, 498–500. doi:10.1016/j.marpolbul.2017.01.008
Montilla-Pacheco, A. M. (2017). Vera MN, Palma CR. Análisis de las condiciones geográficas y ecológicas del humedal La Segua, provincia de Manabí. Ecuad. La Técnica, 18.
Moore, C. J., Moore, S. L., Leecaster, M. K., and Weisberg, S. B. (2001). A comparison of plastic and plankton in the North Pacific central gyre. Mar. Pollut. Bull. 42, 1297–1300. doi:10.1016/s0025-326x(01)00114-x
Moore, C. J., Moore, S. L., Weisberg, S. B., Lattin, G. L., and Zellers, A. F. (2002). A comparison of neustonic plastic and zooplankton abundance in southern California’s coastal waters. Mar. Pollut. Bull. 44, 1035–1038. doi:10.1016/s0025-326x(02)00150-9
Noles, P., Philco, C., Delgado, C., Eudaldo, L., and López, M. (2017). Calidad de agua del Humedal La Segua-Chone mediante su ictiofauna como bioindicador. Rev. Investig. Talent. 4, 69.
Orona-Návar, C., García-Morales, R., Loge, F. J., Mahlknecht, J., Aguilar-Hernández, I., and Ornelas-Soto, N. (2022). Microplastics in Latin America and the caribbean: A review on current status and perspectives. J. Environ. Manage 309, 114698. doi:10.1016/j.jenvman.2022.114698
Panti, C., Giannetti, M., Baini, M., Rubegni, F., Minutoli, R., and Fossi, M. C. (2015). Occurrence, relative abundance and spatial distribution of microplastics and zooplankton NW of sardinia in the pelagos sanctuary protected area, mediterranean sea. Chem 12, 618. doi:10.1071/en14234
Parker, B., Andreou, D., Green, I. D., and Britton, J. R. (2021). Microplastics in freshwater fishes: Occurrence, impacts and future perspectives. Fish Fish. 22, 467–488. doi:10.1111/faf.12528
Prata, J. C., da Costa, J. P., Lopes, I., Andrady, A. L., Duarte, A. C., and Rocha-Santos, T. (2021). A One Health perspective of the impacts of microplastics on animal, human and environmental health. Sci. Total Env. 777, 146094. doi:10.1016/j.scitotenv.2021.146094
Primpke, S., Cross, R. K., Mintenig, S. M., Simon, M., Vianello, A., Gerdts, G., et al. (2020). Toward the systematic identification of microplastics in the environment: Evaluation of a new independent software tool (siMPle) for spectroscopic analysis. Appl. Spectrosc. 74, 1127–1138. doi:10.1177/0003702820917760
Quan, B., Li, X., Zhang, H., Zhang, C., Ming, Y., Huang, Y., et al. (2019). Technology and principle of removing triclosan from aqueous media: A review. Chem. Eng. J. 15, 122185. doi:10.1016/j.cej.2019.122185
Rahman, A., Sarkar, A., Yadav, O. P., Achari, G., and Slobodnik, J. (2021). Potential human health risks due to environmental exposure to nano- and microplastics and knowledge gaps: A scoping review. Sci. Total Environ. 757, 143872. doi:10.1016/j.scitotenv.2020.143872
Raza, A. (2018). Microplastics in freshwater systems: A review on its accumulation and effects on fishes. Preprints. doi:10.20944/preprints201810.0696.v1
Ríos-Mendoza, L. R., and Balcer, M. (2020). Microplastics in freshwater environments. Encyclopedia of the World's Biomes Elsevier, .325–353. doi:10.1016/B978-0-12-409548-9.12394-2
Robarts, R. D., and Zohary, T. (2018). Limnology and the future of African inland waters. Inland waters 8, 399–412. doi:10.1080/20442041.2018.1481729
Rochman, C. M., Tahir, A., Williams, S. L., Baxa, D. V., Lam, R., Miller, J. T., et al. (2015). Anthropogenic debris in seafood: Plastic debris and fibers from textiles in fish and bivalves sold for human consumption. Sci. Rep. 5, 14340. doi:10.1038/srep14340
Rodrigues, J. P., Duarte, A. C., Santos-Echeandía, J., and Rocha-Santos, T. (2019). Significance of interactions between microplastics and POPs in the marine environment: A critical overview. TrAC 111, 252–260. doi:10.1016/j.trac.2018.11.038
Rose, D. (2019). Characterization of microplastics in the surface waters of Kingston Harbour. Sci. Total Environ. 664, 753–760. doi:10.1016/j.scitotenv.2019.01.319
Santos-Echeandia, J., Rivera-Hernández, J. R., Rodrigues, J. P., and Molto, V. (2020). Interact. mercury beached plastics special Atten. zonation, Degrad. status Polym. type.Mar Chem 222, 10103788. doi:10.1016/j.marchem.2020.103788
Sarijan, S., Azman, S., Said, M. I., Andu, Y., and Zon, N. F. (2018). Microplastics in sediment from skudai and tebrau river, Malaysia: A preliminary study. InMATEC Web Conf. 250, 06012. doi:10.1051/matecconf/201825006012
Scherer, C., Weber, A., Lambert, S., and Wagner, M. (2018). Interactions of microplastics with freshwater biota. in Freshwater microplastics (Cham: Springer), 153–180. doi:10.1007/978-3-319-61615-5_8
Shahul Hamid, F., Bhatti, M. S., Anuar, N., Anuar, N., Mohan, P., and Periathamby, A. (2018). Worldwide distribution and abundance of microplastic: How dire is the situation? Waste Manag. Res. 36, 873–897. doi:10.1177/0734242x18785730
Silva-Cavalcanti, J. S., Silva, J. D., de França, E. J., de Araújo, M. C., and Gusmao, F. (2017). Microplastics ingestion by a common tropical freshwater fishing resource. Environ. Pollut. 221, 218–226. doi:10.1016/j.envpol.2016.11.068
Smith, R. D., and Maltby, E. (2003). Using Ecosyst. approach implement convention Biol. Divers. key issues case Stud. IUCN.
Sridharan, S., Kumar, M., Bolan, N. S., Singh, L., Kumar, S., Kumar, R., et al. (2021). Are microplastics destabilizing the global network of terrestrial and aquatic ecosystem services? Environ. Res. 198, 111243. doi:10.1016/j.envres.2021.111243
Tahimic, C. G. T., Wang, Y., and Bikle, D. D. (2013). Anabolic effects of IGF-1 signaling on the skeleton. Front. Endocrinol. 4, 6. doi:10.3389/fendo.2013.00006
Talbot, R., and Chang, H. (2022). Microplastics in freshwater: A global review of factors affecting spatial and temporal variations. Environ. Pollut. 292, 118393. doi:10.1016/j.envpol.2021.118393
Talbot, R., Granek, E., Chang, H., Wood, R., and Brander, S. (2022). Spatial and temporal variations of microplastic concentrations in Portland's freshwater ecosystems. Sci. Total Environ. 833, 155143. doi:10.1016/j.scitotenv.2022.155143
Tang, N., Liu, X., and Xing, W. (2020a). Microplastics in wastewater treatment plants of Wuhan, Central China: Abundance, removal, and potential source in household wastewater. Sci. Total Environ. 745, 141026. doi:10.1016/j.scitotenv.2020.141026
Tang, S., Lin, L., Wang, X., Feng, A., and Yu, A. (2020b). Pb (II) uptake onto nylon microplastics: Interaction mechanism and adsorption performance. J. Hazard Mater 386, 121960. doi:10.1016/j.jhazmat.2019.121960
Tang, Y., Liu, Y., Chen, Y., Zhang, W., Zhao, J., He, S., et al. (2021). A review: Research progress on microplastic pollutants in aquatic environments. Total Environ. 766, 142572. doi:10.1016/j.scitotenv.2020.142572
Vasilopoulou, G., Kehayias, G., Kletou, D., Kleitou, P., Triantafyllidis, V., Zotos, A., et al. (2021). Microplastics investigation using zooplankton samples from the coasts of Cyprus (eastern mediterranean). Water 13, 2272. doi:10.3390/w13162272
Vázquez-Rowe, I., Ita-Nagy, D., and Kahhat, R. (2021). Microplastics in fisheries and aquaculture: Implications to food sustainability and safety. Curr. Opin. Green Sustain Chem. 29, 100464. doi:10.1016/j.cogsc.2021.100464
Vega-Villasante, F., Ruiz-González, L. E., Chong-Carrillo, O., Basto-Rosales, M. E., Palma-Cancino, D. J., Tintos-Gómez, A., et al. (2021). Biology and use of the pacific fat sleeper dormitator latifrons (Richardson, 1844): State of the art review. Biol. use Pac. fat sleeper Dormitator latifrons (Richardson, 1844) state art review.Lat. Am. J. Aquat. Res. 49, 391–403. doi:10.3856/vol49-issue3-fulltext-2637
Wagner, M., Scherer, C., Alvarez-Muñoz, D., Brennholt, N., Bourrain, X., Buchinger, S., et al. (2014). Microplastics in freshwater ecosystems: What we know and what we need to know. Environ. Sci. Eur. 26, 12. doi:10.1186/s12302-014-0012-7
Wesch, C., Bredimus, K., Paulus, M., and Klein, R. (2016). Towards the suitable monitoring of ingestion of microplastics by marine biota: A review. Environ. Pollut. 218, 1200–1208. doi:10.1016/j.envpol.2016.08.076
Wong, J. K., Lee, K. K., Tang, K. H., and Yap, P. S. (2020). Microplastics in the freshwater and terrestrial environments: Prevalence, fates, impacts and sustainable solutions. Sci. Total Environ. 719, 137512. doi:10.1016/j.scitotenv.2020.137512
Wright, S. L., and Kelly, F. J. (2017). Plastic and human health: A micro issue? Environ. Sci. Technol. 5, 6634–6647. doi:10.1021/acs.est.7b00423
Wu, P., Huang, J., Zheng, Y., Yang, Y., Zhang, Y., He, F., et al. (2019). Environmental occurrences, fate, and impacts of microplastics. Ecotoxicol. Environ. Saf. 184, 109612. doi:10.1016/j.ecoenv.2019.109612
Xue, B., Zhang, L., Li, R., Wang, Y., Guo, J., Yu, K., et al. (2020). Underestimated microplastic pollution derived from fishery activities and “hidden” in deep sediment. Environ. Sci. Technol. 54 (4), 2210–2217. doi:10.1021/acs.est.9b04850
Yıldız, D., Yalçın, G., Jovanović, B., Boukal, D. S., Vebrová, L., Riha, D., et al. (2022). Eff. a microplastic Mix. differ across trophic levels taxa a Freshw. food web situ mesocosm Exp. Sci. Total Environ 836, 155407. doi:10.1016/j.scitotenv.2022.155407
Zhang, K., Su, J., Xiong, X., Wu, X., Wu, C., and Liu, J. (2016). Microplastic pollution of lakeshore sediments from remote lakes in Tibet plateau, China. Environ. Pollut. 219, 450–455. doi:10.1016/j.envpol.2016.05.048
Zhang, P., Liu, S., Tan, X., Liu, Y., Zeng, G., Yin, Z., et al. (2019b). Microwave-assisted chemical modification method for surface regulation of biochar and its application for estrogen removal. Process Saf. Environ. 128, 329–341. doi:10.1016/j.psep.2019.06.009
Zhang, S., Wang, J., Liu, X., Qu, F., Wang, X., Wang, X., et al. (2019a). Microplastics in the environment: A review of analytical methods, distribution, and biological effects. TrAC 111, 62–72. doi:10.1016/j.trac.2018.12.002
Zhang, W., Liu, Y. G., Tan, X. F., Zeng, G. M., Gong, J. L., Lai, C., et al. (2019c). Enhancement of detoxification of petroleum hydrocarbons and heavy metals in oil-contaminated soil by using glycine-β-cyclodextrin. J. Environ. Res. Public Health 16, 1155. doi:10.3390/ijerph16071155
Zhang, X., Li, S., LiuYu, Y. K., Zhang, H., Yu, H., Jiang, J., et al. (2021). Neglected microplastics pollution in the nearshore surface waters derived from coastal fishery activities in Weihai, China. Sci. Total Environ. 768, 144484. doi:10.1016/j.scitotenv.2020.144484
Zhou, A., Zhang, Y., Xie, S., Chen, Y., Li, X., Wang, J., et al. (2021). Microplastics and their potential effects on the aquaculture systems: A critical review. Rev. Aquac. 13, 719–733. doi:10.1111/raq.12496
Zhu, X., Munno, K., Grbic, J., Werbowski, L. M., Bikker, J., Ho, A., et al. (2021). Holistic assessment of microplastics and other anthropogenic microdebris in an urban bay sheds light on their sources and fate. ACS ES&T Water 1, 1401–1410. doi:10.1021/acsestwater.0c00292
Keywords: Ramsar site, pollution, plastics, rotifers, Neotropics, food web
Citation: López C, Soto LM, Acosta V, Santana-Piñeros AM, Cruz-Quintana Y, Gomes-Barbosa L, Stamou G, Karpowicz M, Michaloudi E, Domínguez-Granda L and Steinitz-Kannan M (2023) A first step to assess suspended microplastics in a freshwater wetland from the coastal region of Ecuador. Front. Environ. Sci. 11:1028970. doi: 10.3389/fenvs.2023.1028970
Received: 26 August 2022; Accepted: 10 March 2023;
Published: 27 March 2023.
Edited by:
Eugenia López-López, National Polytechnic Institute, MexicoReviewed by:
Carlos Gravato, University of Lisbon, PortugalAlessio Gomiero, Norwegian Research Institute (NORCE), Norway
Copyright © 2023 López, Soto, Acosta, Santana-Piñeros, Cruz-Quintana, Gomes-Barbosa, Stamou, Karpowicz, Michaloudi, Domínguez-Granda and Steinitz-Kannan. This is an open-access article distributed under the terms of the Creative Commons Attribution License (CC BY). The use, distribution or reproduction in other forums is permitted, provided the original author(s) and the copyright owner(s) are credited and that the original publication in this journal is cited, in accordance with accepted academic practice. No use, distribution or reproduction is permitted which does not comply with these terms.
*Correspondence: Carlos López, Y2xsb3BlekBlc3BvbC5lZHUuZWM=