- State Key Laboratory of Hydrology-Water Resources and Hydraulic Engineering, Nanjing Hydraulic Research Institute, Nanjing, China
Due to the impact of climate change and rapid urbanization, issues around global urban flood control and water environment security have emerged as major global concerns. As a practical way to address these issues, exploitation of urban rainwater resources has become a worldwide hotspot for research and application. This paper 1) briefly examined the evolution of rainwater utilization management modes in advanced countries, 2) classified urban rainwater utilization measures from the utilization stages into three categories—source control, medium transmission, and terminal treatment, 3) summarized the advantages, disadvantages, and scope of the application of these measures, 4) reviewed the benefits, drawbacks, and application areas of these measures, and 5) conducted a quantitative analysis of their impact on rainwater pollution and flood control.
1 Introduction
According to the statistics from the United Nations, in 2018, 55.3% of the world’s population resided in cities, and by 2030, 60% of all housing will be found in urban areas, with one in three people residing in a city with a population of at least 500,000. (UN, 2019). Rapid urbanization has given rise to an increase in impermeable urban surfaces, which has significantly altered the natural hydrological cycle in urban areas (Damien et al., 2016), making urban flooding a worldwide problem (Hussain et al., 2019). On the one hand, for instance, during the rainy season in China, cities like Beijing and Zhengzhou in the north and Guangzhou and Shenzhen in the south suffered from substantial storm water flooding issues, resulting in significant financial and human losses (Haghighatafshar et al., 2019; Liu et al., 2021; Zhao et al., 2021). On the other hand, as a joint result of population growth, low per capita water resources, and water quality contamination, more than 400 Chinese cities are currently struggling with acute shortages of water resources and water quality deterioration. Utilization of urban rainwater resources has become critical and urgent as it is an important means and method for alleviating urban waterlogging, reducing peak flow, and addressing urban water shortages.
The concept of urban rainwater resource utilization has been proposed for a long time. Initially focusing more on the safe management of urban rainwater flooding, it is now given new and different connotations by different countries based on their specific conditions (Fletcher et al., 2015).
Developed countries like the Netherlands and the United States are at the forefront regarding utilization of urban rainwater resources. In the United States, bills have been passed by the Congress to assure the collection and storage of rainwater. Federal laws also mandate “in situ flood storage” for all new development zones (Cheng et al., 2007). In light of federal laws, each state has created “rainwater utilization regulations.” For the same purposes, the United States has also provided economic incentives, such as general tax controls, issuance of obligation bonds, and federal and state loans (Steffen et al., 2013). In the Netherlands, people also have attached great importance to utilization of rainwater resources. Large buildings there have rainwater collection systems, and the country has very advanced real-time simulation technologies and utilization models for rainwater resources. Not only mechanical methods are used to purify rainwater, but also biochemical methods are applied to obtain high-quality rainwater treatment, allowing rainwater to be reused (Schets et al., 2010).
Concerning the issues of urban rainwater resource utilization, this article sets three objectives: 1) to summarize the histories of relevant modes adopted by different developed countries; 2) to outline, classify, and analyze various relevant methods; and 3) to provide a reference for the application and popularization of relevant measures in the future.
2 Urban rainwater management modes
The practice of rainwater management dates back to 3000 BC (Fletcher et al., 2015). Since the 1970s, developed countries have experienced 40 years of development and practice in urban rainwater and flood management and gradually formed a relatively complete and mature index system. The purpose of rainwater management in urban areas is to reduce the risk of urban flooding, alleviate the shortage of water, and ensure the safety of water resources. Urban rainwater management has recently developed quickly across the globe, and it now has an increasing number of modes and measures (Chen et al., 2016; Qi et al., 2020). Urban rainwater management requires the integrated management of the water cycle within a catchment area, combines the management of urban water supply, groundwater, wastewater, and rainwater (Eyni et al., 2021), and considers the various facilities and institutions involved in the urban water cycle roles and interactions involved in the urban water cycle (Fletcher and Deletic, 2007). It also plays an important role in legislation, concerning urban water management (Mitchell, 2006). Different rainwater utilization modes adopted by different countries are surveyed in the following sections.
2.1 America: Best management practices (BMPs), low impact development (LID), and green infrastructure (GI)
In the early 1970s, the United States realized that the traditional “drainage-based” approach was insufficient to address the problems of urban rainwater pollution and flooding (Haghighatafshar et al., 2019). In order to protect the water environment more actively, the term best management practices (BMPs) was first introduced in the Clean Water Act enacted in 1972. At first, the primary goal of BMPs was to control non-point source pollution. Now, BMPs are committed to taking comprehensive measures to solve the problems regarding water quality, water volume, and ecology, which significantly reduces peak flow by using centralized facilities (Ice, 2004). BMPs can generally be divided into two categories: structured measures and non-structural measures. Structured measures mainly include terminal treatment measures, such as rainwater pond, rainwater wetland, and infiltration facilities, while non-structural measures refer to various management measures (Che et al., 2014). From 1979 to 1983, the United States Environmental Protection Agency implemented the national urban runoff plan, which identified four types of urban rainwater BMPs: detention devices, recharge devices, housekeeping practices, and others (United States, 1972; United States, 1983). In the United States and Canada, BMPs were promoted in the cities through the national urban runoff plan in the early 1990s. Later, the concept of BMPs occurred in rainwater design manuals all over the United States, and the measures described in BMPs have been implemented and widely developed there.
The term low impact development (LID) was first coined in a land-use planning report in Vermont in 1977 (Liu et al., 2016; Mi et al., 2018). LID is a concept of urban rainwater management based on BMPs that serves as a supplement to macro-scale BMPs. LID aims to minimize the negative impact of urbanization or site development on the water environment from the very beginning, focusing on the use of small, decentralized eco-technical measures to maintain or restore the hydrological cycle before site development (Gregoire and Clausen, 2011; Rodak et al., 2019). LID minimizes the cost of rainwater management and solves the comprehensive problems of rainwater systems more efficiently and stably (Dietz et al., 2007; Xie et al., 2017). Specific measures include bio-retention facilities, green roofs, and grass ditches, which are near the source of runoff. The LID Research Center was established in 1998, which substantially promoted the development of the national urban runoff plan. The use of LID became mainstream in the early 21st century when it was incorporated into legislation throughout North America (TRCA, 2010).
The concept of green infrastructure (GI) was first introduced in the 1990s. GI originated from landscape design and landscape ecology, focusing on the ecological service function of urban greening. Since the 20th century, GI has been widely used by governments all over the world because of its rainwater management benefits and urban comfort improvements (Walmsley, 1995). GI is widely used in decentralized rainwater management networks to reduce rainwater runoff, improve water quality, and provide ecosystem sustainability (Fu et al., 2019). Compared with LID, GI involves some larger facilities, such as landscape water bodies, green corridors, and large wetlands (Koc et al., 2018), and emphasizes collaboration with urban planning, landscape design, ecology, and biological protection. GI is generally used in multi-scale regional planning or design to replace traditional drainage or gray regulation and storage facilities. It is more effective in achieving the dual objectives of rainwater control and protection or restoration of natural hydrological conditions and ecosystems.
2.2 Britain: Sustainable urban drainage systems (SUDS)
The drainage network in Britain that discharged sewage into the sea was built in the 18th century (Brown and Farrelly, 2009). Later, because the rainwater of the buildings and pavement areas on the catchment surface of the basin greatly exceeded the capacity of the drainage pipe network, floods caused by rainstorm and pollution problems caused by runoff became more and more salient, making it difficult to meet the national requirements for drainage and ecological environment protection (Perales-Momparler, 2015). The concept of sustainable urban drainage systems (SUDS) was first proposed by Jim Conlin of Scottish Water in October 1997 (Butler and Parkinson, 1997). SUDS consist of a range of technologies and methods used for drainage, and the structural measures are essentially the same as that of LID. The concept of SUDS is based on simulating natural processes, such as infiltration, evaporation, and filtration, to deal with possible flood events (Perales-Momparler et al., 2015). At present, the development of SUDS focuses more on water quality management to mitigate the adverse effects of climate change (Gimenez-Maranges et al., 2020).
2.3 Australia: Water-sensitive urban design (WSUD)
The concept of water-sensitive urban design (WSUD) was first introduced in Australia in the 1990s (Cook et al., 2019). It was further refined in the early 21st century when the Australian Joint Committee on Urban Drainage established a WSUD task force in 2004 to promote the construction of an integrated water system design in the urban design process, which was an effort that involved many different industries. The early concept of WSUD centered around rainwater management, and afterwards it aimed to minimize the impact of urban development on the surrounding environment and covered the management of the integrated urban water cycle, including water supply, pollutant discharge reduction, water conservation, and rainwater management (Demuzere et al., 2014; Locatelli et al., 2020). WSUD has many subsets, and one of the most important subsets is rainwater management, aiming at flood prevention, flow management, water quality improvement, rainwater harvest, and non-potable water supplement (Fletcher et al., 2015).
2.4 China: Sponge city (SPC)
The concept of the sponge city (SPC) was first introduced in China in 2014. A sponge city has good “elasticity” in adapting to environmental changes and responding to natural disasters like a sponge. It absorbs, stores, seeps, and purifies water on rainy days and releases and uses the stored water when needed. A sponge city has the flexibility to deal with various disasters. It takes LID as the main design means and strives to keep the hydrological conditions untouched after the completion of the site development (Zhu et al., 2019; Yang et al., 2020). It satisfies the sustainable management idea of protecting the urban water source ecological environment. The sponge city requires all cities to establish a new urban rainwater treatment system that integrates infiltration, storage, stagnation, use, and drainage to retain about 80% of the rainwater, so as to conserve water and improve urban ecological benefits (Fletcher et al., 2015; Sang and Yang, 2017). China has selected 30 cities as pilot projects to promote sponge city construction (Hu et al., 2019; Eyni et al., 2021). However, the development of sponge cities in China is still in the preliminary stage, and there are still some problems, such as crude utilization methods, insufficient universality, lack of official standard for analyzing and assessing the benefits of rainwater utilization, blind construction of facilities, and lack of adequate planning.
2.5 New Zealand: Low impact urban design and development (LIUDD)
After about 30 years of research and practice, modern rainwater management in New Zealand has also formed a relatively complete system, which is at the forefront in the world and has achieved remarkable results. Based on BMPs and LID in the United States and WSUD in Australia, New Zealand puts a special emphasis on ecology and on respect for nature and coined the term “low impact urban design and development (LIUDD)” in “sustainable urban investment and development project” implemented by the New Zealand Foundation for Research, Science and Technology (FRST) in 2003. LIUDD combines national laws and plans and bases itself on the cultural heritage and legislative system. LIUDD emphasizes the application of local plant communities in urban low impact design to protect biodiversity, which uses interdisciplinary rainwater system design methods to simulate the process of natural ecosystems and highlights the combination of ecological functions and regional characteristics (Che et al., 2012).
2.6 Scope of application and features of the rainwater utilization modes
The modes of urban rainwater resource utilization and their histories in different countries are shown in Figure 1. Moreover, several typical rainwater utilization modes are compared and summarized in Table 1. As demonstrated in Table 1, current rainwater management modes are all improvements based on traditional drainage systems. BMPs, LID, and SUDS measures complement the existing drainage infrastructure. GI, WSUD, LIUDD, and SPC are more prominent in top-level design and integrated planning and cross-fertilizing and are widely used in urban planning, landscape design, and other multi-disciplines. Compared with the traditional rainwater measures, the methods and measures adopted in the new modes tend to be smaller, more decentralized, more integrated with the landscape, more protective of natural resources, and more cost-effective.
3 Construction of urban rainwater utilization measure systems
3.1 Classification of measures
Urban rainwater utilization measures can be classified along two dimensions: utilization ways and utilization stages. In terms of utilization ways, urban rainwater utilization measures can be divided into collection, transmission, infiltration, saving, and purification measures.
Collection measures are usually used to collect rainwater from roofs, roads, and green spaces, occupy small areas, and are easy to place. Collection measures include green roofs and rainwater tanks. Transmission measures can transfer the collected rainwater to other rainwater utilization facilities and municipal drainage systems and also have a certain collection capacity. Transmission measures mainly include grass ditches, permeation tubes, and permeation ditches. Infiltration measures infiltrate and supply groundwater, reduce surface runoff and the drainage pressure of the municipal pipe network, and alleviate urban waterlogging by direct rainwater infiltration. Infiltration measures mainly include permeable pavements, rainwater gardens, and infiltration wells. Saving measures use the naturally formed or artificially constructed water-storage space to retain or store rainwater and then slowly discharge it after the flood peak, so as to provide a basis for the reuse of rainwater resources in time and space, and achieve the role of peak cutting and peak shifting. The stored rainwater resources can be directly used for urban greening and irrigation. Saving measures mainly include impounding reservoirs and wet swales. Purification measures remove the polluted impurities in rainwater through the filtration of vegetation and the soil planted with vegetation and improve and control the water quality, so as to achieve water purification and environmental beautification. Purification measures mainly include vegetation buffer strands and bio-retention facilities.
With respect to the stages of rainwater utilization, such measures can be divided into source control, medium transmission, and terminal treatment measures. Source control measures are set before the rainwater enters the municipal pipe network, river ditches, and other drainage systems, with the purpose of controlling the water quantity and quality and increasing the infiltration and storage reuse. The main technical measures include green roofs, rainwater tanks, permeable pavements, and vegetation buffer strands. Medium transmission measures are generally set in the process of runoff confluence, and the overflowing rainwater is discharged into the municipal ditches and pipe networks after the rainwater exceeds the processing capacity of the source control measures. Medium transmission measures aim to intercept, regulate, and store rainwater, and the treated rainwater will be discharged or reused. The main technical measures include grass ditches, permeation tubes, permeation ditches, infiltration wells, and rainwater gardens. Terminal treatment measures are subjected to centralized physical, chemical, and biological treatments to remove pollutants in the rainwater and improve the rainwater quality and finally directly discharged into the receiving water body or reused after the rainwater is collected at the end of the drainage system. The main technical measures include impounding reservoirs, wet swales, and bio-retention facilities.
3.2 Application analysis of rainwater utilization measures
Current urban rainwater utilization measures include green roofs, rainwater tanks, grass ditches, permeation tubes/ditches, permeable pavements, rainwater gardens, infiltration wells, impounding reservoirs, wet swales, vegetation buffer strands, and bio-retention facilities (Li et al., 2014). A diagram of the distribution of the rainwater measures in cities is displayed in Figure 2.
3.2.1 Source control measures
3.2.1.1 Green roof
Green roofs as an important rainwater utilization measure have emerged in order to bring the inner urban cycle more harmonious with nature because modern urban rooftops account for approximately 20%–25% of the total urban area (Besir and Cuce, 2018). Green roofs, also known as vegetated roofs, eco-roofs, or natural roofs, are considered a new rainwater utilization technology integrating architectural art and greening technology, having the vegetation and the growth medium required for green vegetation (Vijayaraghavan, 2016). A green roof is composed of a vegetation, growth substrate, filter fabric, drainage element, protection layer, root barrier, insulation layer, water-proofing membrane, and roof deck from the top to bottom (He et al., 2016; Mao et al., 2021). A specific configuration of the green roofs is shown in Figure 3. Green roofs can fit in various types of buildings, such as office buildings, hotels, residences, schools, and gymnasiums (Shafique et al., 2018). Green roofs can reduce rainwater runoff, reduce greenhouse gas emissions to mitigate the urban heat island effect, reduce energy consumption, improve air and water quality, lower the pH of acid rainwater, provide better ecological urban living and wildlife habitat, and absorb noise (Coma et al., 2016; Tang and Qu, 2016; Abu-Zreig et al., 2019).
Wang et al. (2011) showed that the green roofs can effectively retain the roof rainwater runoff. The water retention rate is between 55%–88% during different seasons and different rainfalls, and the roof planted with different plants has the best reduction effect on storm runoff of 62%–80% under the same condition of the building substrate. Ayub et al. (2015) showed that planting suitable plants can reduce flood peak flow by 30.5%–67%. Simmons et al. (2008) showed that the green roofs can delay the peak runoff for 10 min, and when the rainfall is less than 10 mm, it will be absorbed by the green roofs. In addition, Wang et al. (2014) showed that the removal rates of TSS (total suspended solids), TP (total phosphorus), and TN (total nitrogen) pollution loads by green roofs can reach 40%, 31.6%, and 29.8%, respectively.
3.2.1.2 Permeable pavement
Permeable pavements are a form of pavement coverage that uses gravel and sand with good permeability and high porosity in the surface layer, subgrade, and lowest soil foundation, making rainwater enter the interior of the pavement structure smoothly and penetrate into the soil base through the drainage pipe inside the pavement, so as to reduce surface runoff and ground recharge (Qin, 2015). In terms of surface materials, permeable pavements can be classified into permeable bricks, permeable asphalt concrete, and permeable cement concrete pavements. An exact structure of permeable pavements is shown in Figure 4. The hydrological performance of permeable pavements depends on the water-storage capacity of the foundation and the saturated hydraulic conductivity of the foundation soil (Mullaney and Lucke, 2014; Kuruppu et al., 2019). The major advantages of permeable pavements lie in their abilities to purify water, restore natural hydrology, reduce runoff, mitigate urban heat island effects, and reduce road noise (Al-Rubaei et al., 2014; Yu et al., 2015; Gülbaz and Kazezyılmaz-Alhan, 2016).
Wang et al. (2019) showed that the total surface runoff of permeable pavements decreased by 1%–40%, and that the peak flow decreased by 7%–42%. Liu et al. (2020) found that, in the case of heavy rainfall, permeable pavements can effectively retain rainwater runoff, with an average runoff retention of 42.5%–52.5%. Regarding different materials of permeable pavements, Zhao et al. (2020) showed that the average peak delay time of structural permeable bricks and that of ordinary permeable bricks is 6.3 and 16.3 min, respectively. Li et al. (2018), summarizing several cases, concluded that the removal rates of TSS, TP, and TN pollution loads by permeable pavements can reach 56%–96%, 24%–63.4%, and 42%, respectively.
3.2.1.3 Bio-retention facility
Bio-retention facilities are similar to vegetated shallow trenches, planted in areas with a low terrain, making full utilization of the urban open space and improving rainwater quality through plant retention and soil infiltration. It is generally composed of a surface rainwater retention layer, vegetation planting layer, planting soil layer, sand-filter layer, and rainwater collection part (Nguyen et al., 2019).
Based on the KOSIM model, Tian et al. (2019) showed the environmental benefits of bio-retention facilities. The KOSIM model is a hydrological assessment model developed by Hanover Water Co., Ltd. As a Windows-based program for analyzing flood storage and detention areas of the urban drainage system, the KOSIM model can be used to calculate the performance of combined sewage overflow, sponge facilities, and rainwater storage tanks. In the case of large- and medium-sized rainstorms, the runoff retention rate of bio-retention facilities is 53%–79%. Pollutant removal is the main function of bio-retention facilities, while bio-retention facilities with different structures generally have different pollutant removal rates.
3.2.1.4 Vegetation buffer strand
A vegetation buffer strand, which consists of a horizontal water distributor and vegetation, uses surface plants and soil to intercept runoff pollutants. It disperses rainwater evenly across the vegetated area of the slope to promote infiltration and to diffuse runoff in the slope. Vegetation buffer strand plants are divided into two types: grass and woodland. The plants with better treatment effects are selected according to the specific situation. The infiltration and retention of vegetation are influenced by the slope, length, and plant species of the strip (Otto et al., 2008). Vegetation buffer strands are suitable for small areas, around impervious paved areas, parcel boundaries, and both sides of the roads (Gavrić et al., 2019). A specific structure of vegetation buffer strands is shown in Figure 5.
Zhang et al. (2022) showed that vegetation buffer strands can play an important role in preventing and controlling sedimentation, as their interception rate of sedimentation can reach more than 0.9 based on VFSMOD (vegetative filter strip modelling). At the same time, vegetation buffer strands also possess an important function of runoff regulation as they can completely intercept the runoff generated by small- and medium-sized rainfalls. Developed by Munoz-Carpena, VFSMOD estimated the flow and pollutant load in the runoff catchment area and the reduction of runoff flow and pollutant load in the buffer zone based on the model of storm and site conditions.
3.2.2 Medium transmission measures
3.2.2.1 Grass ditch
Grass ditches refer to surface ditches planted with vegetation that are used for collecting, transmitting, purifying rainwater, and connecting to other low-impact development facilities and municipal drainage systems. They can be generally divided into three types: transfer grass ditches, dry grass ditches, and wet grass ditches (Khadka et al., 2020). A specific configuration of grass ditches is shown in Figure 6.
Zhang et al. (2017) showed the control effect of different catchment methods of grass ditches on rainwater runoff flow. The experimental results showed that the rainwater runoff flow was reduced by 7.06%–9.51%, the peak reduction rate reached 2.67%–6.44%, and the peak appeared 9.6–12.2 min later. Wang et al. (2011) showed that the removal rates of TP and TN in grass ditches are generally 20%–40% and 20%–60%, respectively. Li et al. (2016) showed the removal ability of suspended solids and COD (chemical oxygen demand) in rainwater runoff by typical grass ditches. The experimental results showed that the removal rates of suspended solids and COD in rainwater runoff by grass ditches can reach 92.1%–99.3% and 51.72%–86.35%, respectively. Guo et al. (2015) showed that when the vegetation coverage of grass ditches reached about 60%, the removal rates of ammonia nitrogen and total phosphorus in rainwater runoff could be increased by about 10% and 20%, respectively.
3.2.2.2 Permeation tube/ditch
Permeation tubes/ditches refer to rainwater pipes/drains with an infiltration function, which can be made of a combination of perforated plastic pipes, sand-free concrete pipes/drains, and gravels (Bhagu et al., 2012). Permeation ditches, which are used to collect and store rainwater from a single area, can be set on the ground to intercept rainwater runoff or supply the phreatic layer underground as part of the rainwater pipeline. During the construction of permeation ditches, the influence of the soil infiltration rate and pollution on aquifers should be mainly considered.
3.2.2.3 Rainwater garden
Rainwater gardens are effective LID practices, usually constructed in green areas with low terrain. They supply groundwater through infiltration, remove pollutants before the rainwater enters the local rivers (Virginia Department of Forestry, 2019), and have landscape effects and ecological values. The advantages of rainwater gardens include purifying rainwater quality, delaying rainwater peaks, having low construction costs, having easy maintenance and management, and having high popularity (Amur et al., 2020). Although rainwater gardens retain a significant portion of rainwater and reduce the risk of flooding, the amount of water reduced during short periods of intense rainstorms is very small. Therefore, when designing rainwater gardens, it should be considered that the ability of rainwater gardens to reduce urban flood is limited (Martine et al., 2014; Guo et al., 2015). A specific structure of a rainwater garden is shown in Figure 7.
Tang et al. (2016) showed that the rainwater gardens with different fillers reduce the amount of rainfall by 9.8%–85.9% and the peak runoff by 11.2%–93.3%, and the flood peak delay time is 10–40 min. The urban storm flood process model built based on SWMM (storm water management model) has a runoff control rate of 50.9%–84.7% under different design rainfall return periods. SWMM is a rainstorm flood management model developed and studied by the United States Environmental Protection Agency (EPA) in 1971. It is applied to urban rainfall runoff simulation and is the most widely used urban rainstorm runoff model at present. For the removal of pollutants, Du et al. (2021) showed that the reduction rates of TSS, COD, TN, and TP are 20.8%–93.3%, 28.6%–92.6%, 11.6%–54.8%, and 9.8%–47.3%, respectively. The longer the recurrence period is, the lower the runoff control and pollutant reduction rates are.
3.2.2.4 Infiltration well
Infiltration wells are rainwater infiltration devices that can increase the infiltration effect through rainwater infiltration facilities on the well wall and on the bottom of the well. Infiltration wells are buried deeply under the ground, and horizontal infiltration drainage pipes are set around them to supplement the groundwater.
3.2.3 Terminal treatment measures
3.2.3.1 Rainwater tank
Rainwater tanks are normally connected to seepage places, such as rainwater gardens or gravel-filled dry wells, and installed in small rooms near buildings to collect rainwater flowing down from roof downpipes for non-potable water utilization, such as cleaning toilets. The advantages of rainwater tanks include low-cost rainwater collection and use facilities, high water collection efficiency, simple setup, and easy maintenance management.
Rainwater tanks, with respect to the installation location, can be divided into above-ground rainwater tanks and underground rainwater tanks. A specific structure of rainwater tanks is shown in Figure 8. Rainwater tanks have a weak purification effect on rainwater quality, so it is necessary that they are cleaned regularly inside to avoid pollution, which affects their normal functional effects (Petrucci et al., 2012; Litofsky et al., 2014). The payback period of rainwater tank investment is influenced by a combination of tank size, water consumption, local water prices, location, and lifetime costs (Nachshon et al., 2016; Sharma and Gardner, 2020).
3.2.3.2 Impounding reservoir
Impounding reservoirs are commonly used facilities for collection and storage of rainwater. They are also a way to control the total amount of runoff and reduce the peak flow. Impounding reservoirs can be divided into reinforced concrete, masonry, and plastic module impounding reservoirs according to different materials. Urban areas with limited land utilization mostly use underground impounding reservoirs, which are built by reinforced concrete, masonry, or other materials, have good adaptability and save space but require a lot of excavation, and are more difficult to clean and maintain (Wu et al., 2020).
3.2.3.3 Wet swale
Most urban wet swales are artificial wet swales that simulate the structure and function of natural wet swales. Wet swales can efficiently control the pollution content of surface runoff, integrate ecological restoration and landscape aesthetic values, have low investment and simple operation and management, facilitate sedimentation, and have stable effects. In particular, they can operate reliably under unfavorable conditions, and their effects do not depend on soil properties.
Koon (1995) showed that the removal efficiency of TSS in the wet swales was between 67% and 81%, and the reduction range of TP was between 17% and 39%. Winston et al. (2012) showed that wet swales reduced TN in runoff by 40%.
3.3 Comparison of the technical measures of rainwater utilization
This article summarized the advantages, disadvantages, and application scope of the aforementioned technical measures, as shown in Table 2. In addition, this article also selected five typical measures and quantified their environmental benefits. The comparison results are shown in Table 3. Considering the control and reduction efficiency of the measures for runoff and rainfall runoff pollutants, eight indicators were selected to reflect the environmental benefits of the measures: the runoff reduction rate, peak reduction rate, rainwater retention rate, flood peak delay time, total suspended solids (TSS) reduction rate, total nitrogen (TN) reduction rate, total phosphorus (TP) reduction rate, and chemical oxygen demand (COD) reduction rate. The results showed that source control measures, including green roofs and permeable pavements, perform better regarding runoff reduction, peak reduction, rainwater retention, and flood peak delay, while medium transmission and terminal treatment measures, including grass ditches, rainwater gardens, and wet swales, perform better in pollutant removal. Green roofs can reduce runoff by 80%, reduce flood peak by 67%, retain rainwater by 88%, and delay flood peak time by 10 min. Permeable pavements can reduce runoff by 90%, reduce flood peak by 80%, retain rainwater by 52.5%, and delay flood peak time by 16.3 min. Grass ditches, rainwater gardens, and wet swales remove TSS by over 80%, TP by over 39%, and TN by over 40%.
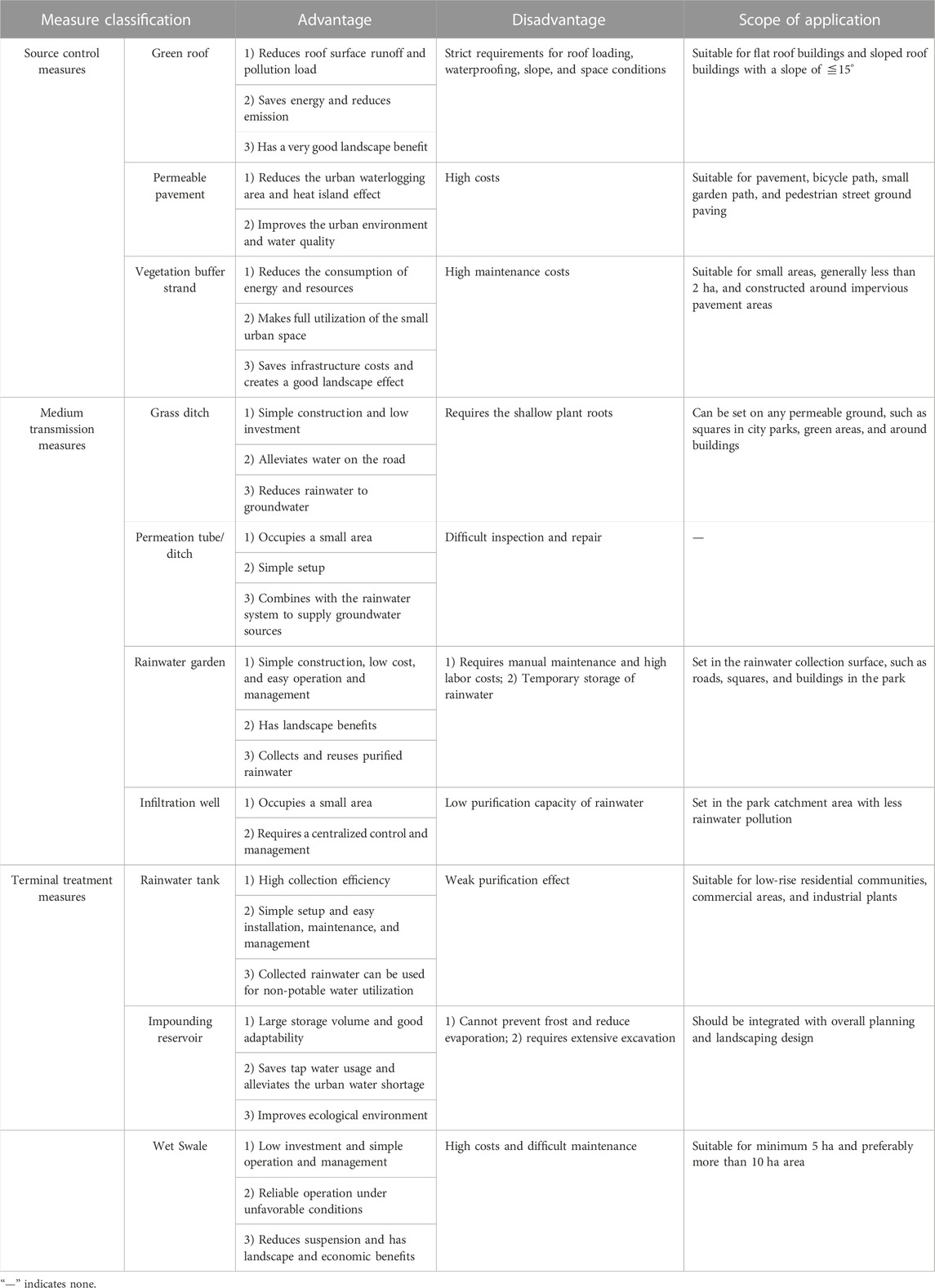
TABLE 2. Advantages, disadvantages, and the scope of application for the technical measures of rainwater utilization.
For other benefits that are not easy to quantify, this article made a qualitative comparative analysis, and the comparison results are shown in Table 4. In terms of economic benefits, land areas of green roofs, permeable pavements, and infiltration wells are small, and construction costs of grass ditches, permeable pavements, and infiltration wells are low, and maintenance costs of grass ditches, permeable pavements, rainwater gardens, and vegetation buffer strands are low. With respect to the ecological benefits, pollution control, peak retardation, and total storage of rainwater gardens, wet swales, and bio-retention facilities show a relatively good performance. Regarding aesthetic benefits, green roofs stand out to some extent. Overall, different measures have different advantages and should be set in different locations in urban design planning, considering the specific local conditions.
4 Prospects
The article provided an outlook on each of the two blocks: rainwater management modes and rainwater utilization measures.
4.1 Rainwater management modes
In the past few decades, the urban rainwater management model has undergone significant changes, from mainly relying on the traditional gray drainage system to reduce urban waterlogging to green facilities that combine environmental, health, social, and economic factors. Countries that have just started rainwater utilization can learn from rainwater management modes of leading countries as well as their experience and develop more rainwater utilization modes.
4.1.1 Establish and improve the relevant law and regulation system and incentive mechanism of rainwater management
The implementation of a system cannot be separated from the support of the legal force. The mature rainwater management mode has a sound system of laws and regulations related to rainwater management as well as an effective incentive mechanism. Countries should strengthen policy guidance, formulate appropriate discharge standards and charging systems, and ensure the acceleration of the city’s rainwater management.
4.1.2 Popularize awareness of water conservation
While vigorously developing the economy, countries should also make people aware of the importance of environmental protection. Countries should vigorously publicize the importance of rainwater management and environmental protection. The public should understand and manifest the understanding in various activities, change public awareness through various channels, and actively participate in the construction of a new mode.
4.1.3 Strengthen interdisciplinary and multi-sectoral cooperation
Urban rainwater management is an extremely complex issue, involving architecture, planning, landscape, municipal engineering, environmental engineering, hydraulics, urban water supply and drainage, social resource management, and other disciplines. Although the importance of cooperation between various departments has been repeatedly emphasized when formulating relevant policies, more adjustments are needed in practice. Rainwater management personnel should have the basic knowledge of various disciplines involved in urban rainwater management and should be able to communicate and cooperate closely in the future design work, so as to make the drainage system design more scientific. When necessary, they can also collaborate with leading foreign design teams to learn advanced experience.
4.1.4 Adapt to national conditions
Each country has different geographical conditions and cultural customs. A mature rainwater utilization mode is often only suitable for certain specific conditions, such as abundant water or drought in the country. For example, the sponge cities in China have developed rapidly in recent years, and many rainwater management indicators have been set up, but most of them have not been implemented well because many indicators are simply copied from the technical indicators of developed countries in rainwater utilization, having the problem of lacking rationality. Therefore, each country should adjust its measures according to the local conditions, base its relevant works on national conditions, and establish a mode suitable for its own country.
4.1.5 Establish management systems with clear division of powers and responsibilities
To solve the urban storm flood problem, not only is it necessary to build drainage facilities, but also they should be maintained by relevant departments and developers in accordance with the rights and obligations specified in the relevant system. Establishing and improving the accountability system for rainwater management are helpful for finding ways to solve problems as soon as possible, which is beneficial to efficient working of the storm flood management facilities.
4.2 Rainwater utilization measures
There are many specific measures for rainwater utilization, which have ecological and sustainable characteristics and broad application prospects. In reality, rainwater utilization measures are basically sporadic, and comprehensive planning is needed to give full play to reap the maximum benefits. The government should carry out planning at different levels to form a rainwater management system with master planning, large watershed planning, small watershed planning, and environmental management planning and retain and reduce rainwater at all levels to achieve “zero growth” of regional development before and after runoff. At present, rainwater utilization measures and technologies are still in the exploratory stage, lacking extensive engineering applications, and their control and treatment effects on surface runoff are not stable. Countries should learn from each other’s successful cases and choose appropriate rainwater utilization measures according to the local conditions.
Author contributions
All authors listed have made a substantial, direct, and intellectual contribution to the work and approved it for publication.
Funding
This research was jointly funded by the National Key R&D Program of China (2018YFE0206200), the Projects of National Natural Science Foundation of China (52179073), and the Natural Science Foundation of Jiangsu Province, China (Grant No. BK20200160).
Conflict of interest
The authors declare that the research was conducted in the absence of any commercial or financial relationships that could be construed as a potential conflict of interest.
Publisher’s note
All claims expressed in this article are solely those of the authors and do not necessarily represent those of their affiliated organizations, or those of the publisher, the editors, and the reviewers. Any product that may be evaluated in this article, or claim that may be made by its manufacturer, is not guaranteed or endorsed by the publisher.
References
Abu-Zreig, M., Ababneh, F., and Abdullah, F. (2019). Assessment of rooftop rainwater harvesting in northern Jordan. Phys. Chem. Earth 114. doi:10.1016/j.pce.2019.08.002doi:102794
Al-Rubaei, A. M., Viklander, M., and Blecken, G. T. (2014). Long-term hydraulic performance of stormwater infiltration systems. Urban Water J. 12 (8), 660–671. doi:10.1080/1573062x.2014.949796
Amur, A., Wadzuk, B. M., and Traver, R. (2020). Analyzing the performance of a rain garden over 15 Years: How predictable is the rain garden's response. Proceedings of the International Low Impact Development Conference, July 2020, Bethesda, Maryland.
Ayub, K. R., Ghani, A. A., and Zakaria, N. A., (2015). Green roof: Vegetation response towards lead and potassium. Proceedings of the 1st Young Scientist International 469 Conference of Water Resources Development and Environmental Protection, 5–7.
Besir, A. B., and Cuce, E. (2018). Green roofs and facades: A comprehensive review. J. Renew. Sustain. Ener. 82, 915–939. doi:10.1016/j.rser.2017.09.106
Brown, R. R., and Farrelly, M. A. (2009). Challenges ahead: Social and institutional factors influencing sustainable urban stormwater management in Australia. Water Sci. Technol. 59, 653–660. doi:10.2166/wst.2009.022
Butler, D., and Parkinson, J. (1997). Towards sustainable urban drainage. Water Sci. Technol. 35, 53–63. doi:10.2166/wst.1997.0330
Chahar, B., Graillot, D., and Gaur, S. (2012). Storm-water management through infiltration trenches. J. Irrigation Drainage Eng. 138 (3), 274–281. doi:10.1061/(asce)ir.1943-4774.0000408
Che, W., Frank, T., Li, J. Q., and Zhang, Y. J. (2012). Introduction to auckland modern stormwater management (Ⅰ): Relevant legislation and plans. Water and Wastewater Eng. 38 (3), 28–32. (In Chinese).
Che, W., Yan, P., Zhao, Y., and Tian, F. (2014). Development and analysis of International updated stormwater management systems. China Water and Wastewater 30, 45–51.
Chen, Y., Samuelson, H. W., and Tong, Z. (2016). Integrated design workflow and a new tool for urban rainwater management. J. Environ. Manage. 180, 45–51. doi:10.1016/j.jenvman.2016.04.059
Cheng, J., Xu, Q. X., Yang, K., Liu, L. L., and Fan, Q. J. (2007). Comparison of foreign urban rainwater resource utilization management systemsand some inspirations. China Water and Wastewater 12, 68–72. (In Chinese).
Coma, J., Pérez, G., Solé, C., Castell, A., and Cabeza, L. F. (2016). Thermal assessment of extensive green roofs as passive tool for energy savings in buildings. Renew. Energy 85, 1106–1115. doi:10.1016/j.renene.2015.07.074
Cook, S., van Roon, M., Ehrenfried, L., LaGro, J., and Yu, Q. (2019). “WSUD ‘best in class’ - case studies from Australia, New Zealand, United States, europe, and asia,” in Approaches to water sensitive urban design (Amsterdam, Netherlands: Elsevier), 561–585.
Damien, T., Ghassan, C., Daniel, P., Yves, K., and Marie-Christine, G. (2016). Impact of runoff infiltration on contaminant accumulation and transport in the soil/filter media of sustainable urban drainage systems: A literature review. Sci. Total Environ. 569, 904–926. doi:10.1016/j.scitotenv.2016.04.215
Demuzere, M., Orru, K., Heidrich, O., Olazabal, E., Geneletti, D., Orru, H., et al. (2014). Mitigating and adapting to climate change: Multi-functional and multi-scale assessment of green urban infrastructure. J. Environ. Manage. 146, 107–115. doi:10.1016/j.jenvman.2014.07.025
Du, Y. E., Hou, J. M., Ma, H. L., Liu, Q. C., Wang, X. H., Zhang, Z. A., et al. (2021). Spatial pattern optimization of LID facility based on SWMM. China Water and Wastewater 37 (19), 120–125. (In Chinese).
Eyni, A., Skardi, M. J. E., and Kerachian, R. (2021). A regret-based behavioral model for shared water resources management: Application of the correlated equilibrium concept. Sci. Total Environ. 759, 143892. doi:10.1016/j.scitotenv.2020.143892
Fletcher, T. D., and Deletic, A. (2007). Data requirements for integrated urban water management. Netherlands: Taylor and Francis.
Fletcher, T. D., Shuster, W., Hunt, W. F., Ashley, R., Butler, D., Arthur, S., et al. (2015). SUDS, LID, BMPs, WSUD and more - the evolution and application of terminology surrounding urban drainage. Urban. Water J. 12, 525–542. doi:10.1080/1573062x.2014.916314
Fu, X., Hopton, M. E., Wang, X. H., Goddard, H., and Liu, H. Q. (2019). A runoff trading system to meet watershed-level stormwater reduction goals with parcel-level green infrastructure installation. Sci. Total Environ. 689, 1149–1159. doi:10.1016/j.scitotenv.2019.06.439
Gavric, S., Leonhardt, G., Mar Sa Lek, J., and Viklander, M. (2019). Processes improving urban stormwater quality in grass swales and filter strips: A review of research findings. Sci. Total Environ. 669, 431–447. doi:10.1016/j.scitotenv.2019.03.072
Gimenez, M., Breuste, J., and Hof, A. (2020). Sustainable drainage systems for transitioning to sustainable urban flood management in the European union: A review. J. Clean. Prod. 255, 120191. doi:10.1016/j.jclepro.2020.120191
Gregoire, B. G., and Clausen, J. C. (2011). Effect of a modular extensive green roof on stormwater runoff and water quality. Ecol. Eng. 37, 963–969. doi:10.1016/j.ecoleng.2011.02.004
Gülbaz, S., and Kazezyılmaz-Alhan, C. M. (2016). Experimental investigation on hydrologic performance of lid with rainfall-watershed-bioretention system. J. Hydrol. Eng. 22, D4016003. doi:10.1061/(asce)he.1943-5584.0001450
Guo, F., Chen, J. G., Yang, J., Meng, Y. Y., and Gong, Y. A. (2015). Regulatory effect of grassed swales on road surface runoff in beijing city. Bull. Soil Water Conservation 35 (3), 176–181.
Guo, J. C. Y., and Lu, T. M. (2015). Operation of cap orifice in a rain garden. J. Hydrol. Eng. 20, 6015002. doi:10.1061/(asce)he.1943-5584.0001184
Haghighatafshar, S., Yamanee-Nolin, M., Klinting, A., Roldin, M., Gustafsson, L. G., Aspegren, H., et al. (2019). Hydroeconomic optimization of mesoscale blue-green stormwater systems at the city level. J. Hydrol. 578, 124125. doi:10.1016/j.jhydrol.2019.124125
He, Y., Yu, H., Dong, N. N., and Hang, Y. (2016). Thermal and energy performance assessment of extensive green roof in summer: A case study of a lightweight building in shanghai. Energy Build. 127, 762–773. doi:10.1016/j.enbuild.2016.06.016
Hu, M., Zhang, X. Q., Li, Y., Yang, H., and Tanaka, K. (2019). Flood mitigation performance of low impact development technologies under different storms for retrofitting an urbanized area. J. Clean. Prod. 222, 373–380. doi:10.1016/j.jclepro.2019.03.044
Hussain, F., Hussain, R., Wu, R. S., and Abbas, T. (2019). Rainwater harvesting potential and utilization for artificial recharge of groundwater using recharge wells. Processes 7, 623. doi:10.3390/pr7090623
Ice, G. (2004). History of innovative best management practice development and its role in addressing water quality limited waterbodies. J. Environ. Eng. 130 (6), 684–689. doi:10.1061/(asce)0733-9372(2004)130:6(684)
Khadka, A., Kokkonen, T., Niemi, T. J., Lhde, E., Sillanpää, N., and Koivusalo, H. (2020). Towards natural water cycle in urban areas: Modelling stormwater management designs. Urban Water J. 17, 587–597. doi:10.1080/1573062x.2019.1700285
Koc, C. B., Osmond, P., and Peters, A. (2018). Mapping and classifying green infrastructure typologies for climate-related studies based on remote sensing data. Urban For. Urban Gree. 37, 154–167.
Koon, J. (1995). Evaluation of water quality ponds and swales in the issaquah/east lake sammamish basins. Washington, D C, USA: King County Surface Water Management and Washington Department of Ecology.
Kuruppu, U., Rahman, A., and Rahman, M. A. (2019). Permeable pavement as a stormwater best management practice: A review and discussion. Environ. Earth Sci. 78, 327. doi:10.1007/s12665-019-8312-2
Li, H., Li, K., and Zhang, X. (2016). Performance evaluation of grassed swales for stormwater pollution control. Procedia Eng. 154, 898–910. doi:10.1016/j.proeng.2016.07.481
Li, M. Y., Zhang, S. H., Wang, Y. J., and Wang, Y. Q. (2018). Runoff management performances of permeable pavements: A review. Environ. Sci. Technol. 41 (12), 105–112.
Li, T., Zhang, W., and Huang, J. J. (2014). Integrated rainwater management planning, evaluation and implementation: Take Shanghai as an example. J. Southeast Univ. 30, 206–211.
Litofsky, A. L. E., and Jennings, A. J. (2014). Evaluating rain barrel storm water management effectiveness across climatography zones of the United States. J. Environ. Eng. 7, 1943–7870. doi:10.1061/(asce)ee.1943-7870.0000815
Liu, B. J., Li, N., and Xiong, K. Y. (2016). Study on construction of measures-system for low impact development and its effect. Water Resour. Hydropower Eng. 47 (8), 19–22.
Liu, S., Yin, Y., Xiao, H., Jiang, H., and Shi, R. (2021). The effects of ice nucleation on the microphysical processes and precipitation for a heavy rainfall event in Beijing. Atmos. Res. 253, 105476. doi:10.1016/j.atmosres.2021.105476
Liu, W., Feng, Q., Chen, W., and Deo, R. C. (2020). Stormwater runoff and pollution retention performances of permeable pavements and the effects of structural factors. Environ. Sci. Pollut. Res. 27, 30831–30843. doi:10.1007/s11356-020-09220-2
Locatelli, L., Guerrero, M., Russo, B., Martínez-Gomariz, E., Sunyer, D., and Martínez, M. (2020). Socio-economic assessment of green infrastructure for climate change adaptation in the context of urban drainage planning. Sustainability 12, 3792. doi:10.3390/su12093792
Mao, J., Xia, B. Y., Zhou, Y., Bi, F., Zhang, X. D., Zhang, W., et al. (2021). Effect of roof materials and weather patterns on the quality of harvested rainwater in Shanghai, China. J. Clean. Prod. 279, 123419. doi:10.1016/j.jclepro.2020.123419
Martine, Galarneau, Michèle, Prévost, Laurène, Autixier, Madoux-Humery, A. S., Prevost, M., et al. (2014). Evaluating rain gardens as a method to reduce the impact of sewer overflows in sources of drinking water. Sci. Total Environ. 499, 238–247. doi:10.1016/j.scitotenv.2014.08.030
Mi, W. J., Zhang, A. J., and Ren, W. Y. (2018). Oversea utilization and development of urban rainwater resources with low impact and its implications for construction of sponge city in China. Bull. Soil Water Conserv. 3, 345–352.
Mitchell, V. G. (2006). Applying integrated urban water management concepts: A review of Australian experience. Environ. Manage. 37, 589–605. doi:10.1007/s00267-004-0252-1
Mullaney, J., and Lucke, T. (2014). Practical review of pervious pavement designs. Clean-Soil Air Water 42, 111–124. doi:10.1002/clen.201300118
Nachshon, U., Netzer, L., and Livshitz, Y. (2016). Land cover properties and rain water harvesting in urban environments. Sustain. Cities Soc. 27, 398–406. doi:10.1016/j.scs.2016.08.008
Nguyen, T. T., Ngo, H. H., Guo, W., Wang, X. C., Ren, N., Li, G., et al. (2019). Implementation of a specific urban water management-Sponge City. Sci. Total Environ. 652, 147–162. doi:10.1016/j.scitotenv.2018.10.168
Otto, S., Vianello, M., Infantino, A., Zanin, G., and Guardo, A. D. (2008). Effect of a full-grown vegetative filter strip on herbicide runoff: Maintaining of filter capacity over time. Chemosphere 71, 74–82. doi:10.1016/j.chemosphere.2007.10.029
Perales-Momparler, C. S. (2015). A regenerative urban stormwater management methodology: The role of SUDS construction and monitoring in the transition of a mediterranean city. Valencia, Spain: Universitat Politecnica de Valencia.
Perales-Momparler, S., Andres-Domenech, I., Andreu, J., and Escuder-Bueno, I. (2015). A regenerative urban stormwater management methodology: The journey of a mediterranean city. J. Clean. Prod. 109, 174–189. doi:10.1016/j.jclepro.2015.02.039
Petrucci, G., Deroubaix, J. F., Gouvello, B. D., Deutsch, J. C., Bompard, P., and Tassin, B. (2012). Rainwater harvesting to control stormwater runoff in suburban areas. An experimental case-study. Urban Water J. 9, 45–55. doi:10.1080/1573062x.2011.633610
Qi, Y., Chan, F. K. S., Thorne, C., O’Donnell, E., Quagliolo, C., Comino, E., et al. (2020). Addressing challenges of urban water management in Chinese sponge cities via nature-based solutions. Water 12 (10), 2788. doi:10.3390/w12102788
Qin, Y. H. (2015). A review on the development of cool pavements to mitigate urban heat island effect. Renew. Sustain. Energy Rev. 52, 445–459. doi:10.1016/j.rser.2015.07.177
Rodak, C. M., Moore, T. L., David, R., Jayakaran, A. D., and Vogel, J. R. (2019). Urban stormwater characterization, control, and treatment. Water Environ. Res. 91, 1034–1060. doi:10.1002/wer.1173
Sang, Y. F., and Yang, M. (2017). Urban waterlogs control in China: More effective strategies and actions are needed. Nat. Hazards 85, 1291–1294. doi:10.1007/s11069-016-2614-4
Schets, F. M., Italiaander, R., Van Den Berg, H. H. J. L., and de Roda Husman, A. M. (2010). Rainwater harvesting: Quality assessment and utilization in The Netherlands. J. Water Health 8 (2), 224–235. doi:10.2166/wh.2009.037
Shafique, M., Kim, R., and Rafiq, M. (2018). Green roof benefits, opportunities and challenges – a review. Renew. Sustain. Energy Rev. 90, 757–773. doi:10.1016/j.rser.2018.04.006
Sharma, A., and Gardner, T. (2020). Comprehensive assessment methodology for urban residential rainwater tank implementation. Water 12 (2), 315. doi:10.3390/w12020315
Simmons, M. T., Gardiner, B., Windhager, S., and Tinsley, J. (2008). Green roofs are not created equal: The hydrologic and thermal performance of six different extensive green roofs and reflective and non-reflective roofs in a sub-tropical climate. Urban Ecosyst. 11, 339–348. doi:10.1007/s11252-008-0069-4
Steffen, J., Jensen, M., Pomeroy, C. A., and Burian, S. J. (2013). Water supply and stormwater management benefits of residential rainwater harvesting in US cities. J. Am. Water Resour. As. 49 (4), 810–824. doi:10.1111/jawr.12038
Tang, S. C., Luo, W., Jia, Z. H., Li, S., Wu, Y., and Zhou, M. (2016). Effects of filler and rainfall characteristics on runoff reduction of rain garden and achieving the goal of sponge city construction. J. Soil Water Conservation 30 (01), 73–78. (In Chinese).
Tang, X., and Qu, M. (2016). Phase change and thermal performance analysis for green roofs in cold climates. Energy Build. 121, 165–175. doi:10.1016/j.enbuild.2016.03.069
Tian, Y., Zhang, Q. W., Li, D., Liu, G. H., Huang, L. L., Li, H. H., et al. (2019). Bio-retention effectiveness and parameter evaluation based on model simulation. Environ. Eng. 37 (7), 52–56. (In Chinese).
Toronto Region Conservation Authority, (2010). Low impact development stormwater management; plannign and desing guide. Toronto, Canada: TRCA.
United Nations, (2018). United nations population division world urbanization prospects: The 2018 revision. https://population.un.org/wup/.
United States of America, (1983). Clean water Act (1983). Washington, DC, USA: United States Government.
Vijayaraghavan, K. (2016). Green roofs: A critical review on the role of components, benefits, limitations and trends. Renew. Sustain. Energy Rev. 57, 740–752. doi:10.1016/j.rser.2015.12.119
Virginia Department of Forestry, Virginia Department of Forestry Rain gardens. http://www.dof.virginia.gov/manage/riparian/rain-gardens.html.
Walmsley, A. (1995). Greenways and the making of urban form. Landsc. Urban Plan. 33, 81–127. doi:10.1016/0169-2046(95)02015-l
Wang, H. Y., Wu, H., Ma, H. W., Wang, P., and Ling, L. (2019). Study on waterlogging reduction effect of three types of permeable pavements in sponge city construction. China Water Wastewater 35 (12), 44–48.
Wang, J., Yin, W., Ye, M., Lei, A. L., and Li, S. M. (2011). Advance on grassed swales technology in non-point source pollution control. Environ. Sci. Technol. 34 (5), 90–94.
Wang, S. M., Li, X. Y., Zhang, J. H., Yu, H., Hao, Y. Z., and Yang, W. Y. (2014). Influence of green roof application on water quantity and quality in urban region. Chin. J. Appl. Ecol. 25 (7), 2026–2032.
Wang, S. M., Yu, H., Zhang, B., and Shao, L. (2011). Review of green roof in controlling urban non point source pollution. J. Chongqing Univ. Arts Sci. Nat. Sci. Ed. 30 (04), 59–64.
Winston, R. J., Hunt, W. F., Kennedy, S. G., Wright, J. D., and Lauffer, M. S. (2012). Field evaluation of storm-water control measures for highway runoff treatment. J. Environ. Eng. 138 (1), 101–111. doi:10.1061/(asce)ee.1943-7870.0000454
Wu, H. T., Yan, A. P., Zeng, X. G., Wang, Z. X., and Yang, T. (2020). Design and optimization of combined initial rainwater storage tank in separate drainage system. China Water Wastewater 36 (12), 106–110.
Yang, M. Y., Liu, C. M., Pan, X. Y., and Liang, K. (2020). Analysis of sponge city system and research points from the perspective of urban water cycle. Acta Geogr. Sin. 75 (09), 1831–1844.
Yu, B., Jiao, L., Ni, F., and Yang, J. (2015). Long-term field performance of porous asphalt pavement in China. Road. Mat. Pavement 16, 214–226. doi:10.1080/14680629.2014.944205
Zhang, X. Y., Zhang, W., Shi, Z. N., and Li, S. M. (2017). Effect on grassed swales for hydrological regulation of urban stormwater runoff by different influent pattern. Sci. Technol. Eng. 17 (25), 290–294.
Zhang, Y. Y., Nie, S. H., Cai, G. Q., Sun, Z., and Zong, Q. L. (2022). Investigation and simulation on the effect of vegetative filter strip on surface runoff. Res. Soil Water Conservation 29 (02), 36–42. (In Chinese).
Zhao, D., Zha, J., and Wu, J. (2021). Changes in rainfall of different intensities due to urbanization-induced land-use changes in Shenzhen, China. Clim. Dynam. 56 (7-8), 2509–2530. doi:10.1007/s00382-020-05601-y
Zhao, Y. L., Wang, J. L., Li, L. H., et al. (2020). Effect of different types of permeable brick on the stormwater runoff quantity control. Chin. J. Environ. Eng. 14 (3), 835–841. (In Chinese).
Keywords: urban rainwater resources, management modes, utilization measures, pollution control, measure benefits
Citation: Xu J, Dai J, Wu X, Wu S, Zhang Y, Wang F, Gao A and Tan Y (2023) Urban rainwater utilization: A review of management modes and harvesting systems. Front. Environ. Sci. 11:1025665. doi: 10.3389/fenvs.2023.1025665
Received: 23 August 2022; Accepted: 20 January 2023;
Published: 07 February 2023.
Edited by:
Xiaohong Chen, Sun Yat-sen University, ChinaReviewed by:
Chidozie Charles Nnaji, University of Nigeria, Nsukka, NigeriaYuan Li, Hohai University, China
Peipeng Wu, Zhengzhou University, China
Copyright © 2023 Xu, Dai, Wu, Wu, Zhang, Wang, Gao and Tan. This is an open-access article distributed under the terms of the Creative Commons Attribution License (CC BY). The use, distribution or reproduction in other forums is permitted, provided the original author(s) and the copyright owner(s) are credited and that the original publication in this journal is cited, in accordance with accepted academic practice. No use, distribution or reproduction is permitted which does not comply with these terms.
*Correspondence: Jiangyu Dai, anlkYWlAbmhyaS5jbg==; Xiufeng Wu, eGZ3dUBuaHJpLmNu; Shiqiang Wu, c3F3dUBuaHJpLmNu; Yu Zhang, eXV6aGFuZ0BuaHJpLmNu