- 1Harrow Research and Development Centre, Agriculture and Agri-Food Canada, Harrow, ON, Canada
- 2Research Center for Scientific Development in Fenher River Valley, Taiyuan Normal University, Jinzhong, Shanxi, China
- 3Ottawa Research and Development Centre, Agriculture and Agri-Food Canada, Ottawa, ON, Canada
Evaluating the nitrogen (N) use efficiency of animal manure is essential to optimize its application for profitable crop production without impairing the environment. A four-year field study was conducted under corn (Zea mays L.)–soybean (Glycine max L.) rotation in a Brookston clay loam soil using the yield control approach. Treatments included inorganic fertilizer (IN), and liquid (LM), solid (SM), and composted (CM) swine manure applied at the rate equivalent to an available N of 200 kg ha−1 and a non-fertilization control (CT). Seven N use indices were employed to evaluate N use efficiency. LM obtained comparable corn yields relative to IN. Corn yield in SM was inconsistent from one year to another, and CM had minimal agronomical value in our study. Soybeans with IN posed the highest grain yields of 3,468 and 3,761 kg ha−1 in 2005 and 2007, respectively. In contrast to grain yield and total N uptake, the gain N removal of either corn or soybeans was comparable between the two alternative years. The distinctions between N supply dynamics of manures and their influences on yield, grain N removal, and total above-ground plant N uptake of corn were well discriminated by N use efficiency (NUE), N uptake efficiency (NUpE), N utilization efficiency (NUtE), N agronomic efficiency (NAE), and N recovery efficiency (NRE), but not by N physiological efficiency (NPE) and N harvest index (NHI). Legacy effects on soybean yield from the preceding corn were detected by NAE and NRE. Based on grain yield in conjunction with N use efficiency parameters, the IN performed the best, followed by LM. The NUE, NUpE, NUtE, NAE, and NRE parameters used to evaluate chemical fertilizer N were also applicable to evaluate manure N efficiency.
Introduction
Proper use of animal manure as a soil amendment can be an agronomically and economically feasible management practice to treat livestock waste and support sustainable crop production. Despite the distinct nature and composition of manures (e.g., solid vs. liquid and cattle vs. swine), manure applications often have positive effects on crop production (Edmeades, 2003; Lithourgidis et al., 2007; Hua et al., 2020), soil physical structure (Wong and Ho, 1991; Butler and Muir, 2006), and soil nutrient levels (Sutton et al., 1986; Eghball et al., 2004; Hao et al., 2018; Zhang et al., 2021). However, the diversity of manure types (Webb et al., 2011) and sources also posed difficulties for evaluating its agronomic effectiveness and environmental risks. Over-application of animal manure is a commonplace around the agricultural area with intensive livestock production (Beegle and Lanyon, 1994; Saam et al., 2005; Qin et al., 2020). Excess nitrogen (N) supply from manure can cause N loss from the agricultural field and consequently impair the quality of groundwater (Allen et al., 2006) and surface water (Ribbe et al., 2008; Cui et al., 2020) and subsequently affect negatively the aquatic ecosystems. Therefore, evaluating the N use efficiencies of manures is the essential step to optimize manure application for profitable crop production without impairing the environment (Li et al., 2019).
As a predominantly agricultural region in the province, southern Ontario has been receiving much concern about its non-point source pollution contributing to the Lake Eire. The excess nutrients from agriculture have a detrimental impact on the water quality of the lake, which can lead to toxic algae blooms (Hopkins et al., 2016). Ontario has several of the most intensive manure-producing sub-sub-drainage regions across the country. For example, of the five sub-sub-drainages across the country of Canada with manure production levels over 6,000 kg ha−1, four are located in Ontario (EnrioStats, 2015). Since data obtainable in 1986, the number of hogs raised in Ontario, Canada, increased to 3.8 million in 2007 (Statistics Canada, 2008). Ontario is the second-largest hog-producing province in Canada, with 24.4% of all hogs, and the majority is in southern Ontario (Khan et al., 2018). On average, a feeder hog excretes 11 kg N head−1 year−1 (Fraser, 1985). With an equivalency of over 90 thousand metric tons of urea fertilizer, improving the agronomical value of hog manure as a nutrient source is increasingly of interest for Ontario farmers. In 2006, Canadian livestock produced over 180 million tons of manure. Of this total, 9% was produced by swine (EnrioStats, 2015).
Of the factors that influence the N use efficiency of manures, the C:N ratio is the most important one that controls many important processes, like mineralization and immobilization (Qian and Schoenau, 2002). When manure is applied to the soil, either N mineralization or immobilization will occur, depending on the C:N ratio (Beauchamp, 1986; Qian and Schoenau, 2002; de Freitas et al., 2003). Liquid hog manure contains a greater amount of N relative to organic C, and the organic matter in liquid hog manure is easily decomposable compared to that produced by ruminant livestock (Sommer et al., 2007). The microbial biomass activity often results in a fast release of inorganic N (de Freitas et al., 2003) after applying manures with a low C:N ratio. On the contrary, manure application might cause N immobilization at a high manure C:N ratio up to 15–16:1 (Beauchamp, 1986; Qian and Schoenau, 2002). Solid hog manure mixed with straw bedding increases its C:N ratio; thus, there are chances for N immobilization once applied. Manure composting changes organic matter characteristics, and the dynamics of available N release from composted manure would be different from those from uncomposted ones (García et al., 1991; Gale et al., 2006). The decomposition-recalcitrant compound leftovers after composting are much more stable than the humic acids in uncomposted organic materials and are only slowly available to microbial biomass (Deiana et al., 1990; García et al., 1991).
Other factors such as local climate, soil characteristics, and crop management also play important roles in affecting manure N use efficiency through mineralization and immobilization, which affect the supply of available N in opposite ways (Trehan and Wild, 1993; Sørensen and Jensen, 1995; Norvan et al., 1997; Tate, 2020). Many approaches have been developed to evaluate N use efficiency on chemical fertilizer N in mono-cropping systems (Sowers et al., 1994; Delogu et al., 1998; LÓpez-Bellido and LÓpez-Bellido, 2001). For the crop rotation system, however, there is a lack of proper methodology and suitable parameters to evaluate the N use efficiency of manures (Schmitt et al., 1999; Mooleki et al., 2002; Wen et al., 2003; Mooleki et al., 2004; Yang et al., 2020).
Traditionally, fertilizer N is applied in the corn phase as it is a N-demanding crop, followed by soybeans without N fertilization (Badaruddin and Meyer, 1994; Yamoah et al., 1998). When manure is applied to meet the corn N requirement, its residual effects on the subsequent soybean have rarely been taken into consideration. Although N in liquid hog manure was supposed to behave similarly to that in soluble fertilizer N, a portion of N was found to be immobilized after application (Sørensen and Amato, 2002; Azeez and Van Averbeke, 2010). A sizable amount of N in hog slurry remained in a relatively stable organic form in the soil years after application, along with enhanced microbial biomass activities (Peu et al., 2007; Lyyemperumal et al., 2008). In addition, N use efficiency by crop varies with the application rate and increasing the rate of N application usually decreases its use efficiency and vice versa (Latiri-Souki et al., 1998). A full characterization of N use efficiency usually requires multiple application rates, which is expensive and time-consuming. An yield control approach, a simplified experiment applying a single rate of N designed for agronomical yield, would obtain essential information at a low cost and allow fair evaluation of the phyto-availability of nutrients from different organic materials (Wen et al., 2002a; Wen et al., 2002b).
The objectives of this study were, therefore, to apply the yield control approach and to gain insights into the N use efficiency of liquid, solid, and composted hog manures in a corn-soybean rotation system using the multi-N use efficiency parameters derived from the evaluation of chemical fertilizer N. The findings were expected to be helpful in adjusting hog manure N use strategies in southern Ontario, as well as other areas in the world with similar soil, crop, and climate conditions.
Materials and methods
Site description and experimental design
The study was established in spring 2004 on a Brookston clay loam (fine loamy, mixed, mesic Typic Argiaquoll) at the Hon. Eugene F. Whelan Experimental Farm of the Harrow Research and Development Center, Agriculture and Agri-Food Canada, Woodslee, Ontario, Canada (42°13′N, 82°44′W). The climate is humid and cool-temperate with a long-term mean annual air temperature of 8.7°C and average annual precipitation of 875.5 mm. The precipitation in the growing season, May to October, ranged from 232.8 to 535.4 mm, and temperature ranged from 17.3 to 19.3°C, during the study period from 2004 to 2007 (Table 1). Before the experiment, grain corn was grown for 3 years with an annual application of 150 kg N ha−1 to homogenize soil fertility. The soil organic C and total N were considered typical of this area of Ontario. Selected physical and chemical properties of the soil are listed in Table 2.
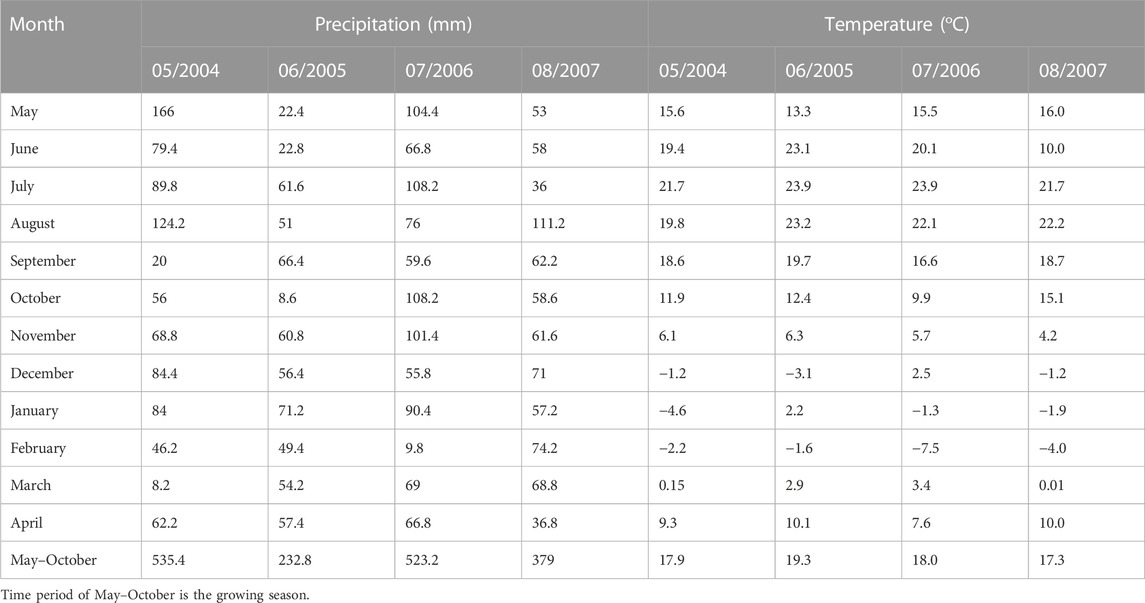
TABLE 1. Monthly and growing season’s means of precipitation and temperature at the study site, Woodslee, ON, Canada.
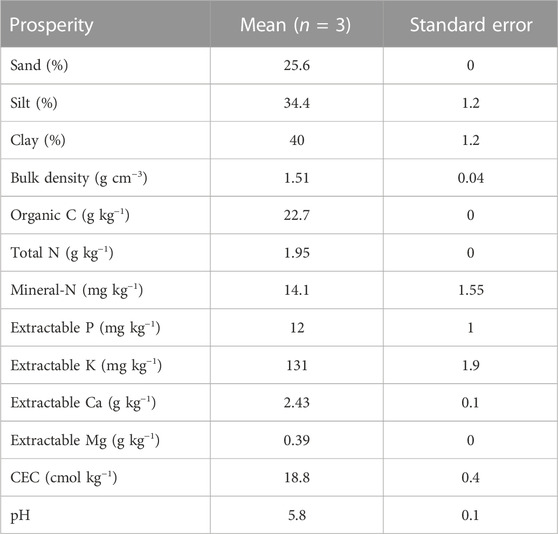
TABLE 2. Selected chemical and physical properties of a Brookston clay loam soil (0–15 cm) in this study.
The experiment consisted of 19 treatments derived from three forms of swine manures (i.e., liquid, solid, and composted liquid), two application approaches (i.e., P-based vs. N-based), combinations of swine manure/compost with chemical fertilizers, and two controls, respectively with zero-nutrient and chemical fertilizers. The treatments had three replicates, totaling 57 plots, each in size of 9 m × 25 m. The plots were laid out as a randomized complete block design. Corn was grown in the year of initiation, followed by soybeans to form a 4-year corn-soybean rotation. There were five treatments selected for the current study, including three forms of swine manures: liquid (LM), solid (SM), and composted (CM), two controls with one for zero-nutrient (CT), and the other for inorganic fertilizer (IN) with the application of 200 kg N ha−1 as ammonium nitrate, the most recommended profitable N rate for corn in southern Ontario, in combination with 50 kg phosphorus (P) ha−1 as triple superphosphate and 100 kg potassium (K) ha−1 as marine K for an expected yield of 10,000 kg ha−1 (OMAFRA, 2009). Analyses of manure and compost for total N, P, and K, as well as the moisture contents were conducted two to 3 days prior to the application. Swine manures and compost were applied based on the rates that supplied an amount of total P of 50 kg ha−1. The designed N rate of 200 kg available N ha−1 was determined by considering 56, 38, and 40% of total N that were available for crops during the growing season in liquid and solid swine manures and swine manure compost (OMAFRA, 2002), respectively, with the balances of the designed N and K rates made up with ammonium nitrate (NH4NO3) and potassium chloride (KCL). All the K in manure was assumed to be available to crops (Table 3). All manures, compost, and chemical fertilizers were pre-planted, broadcasted, and incorporated immediately. The fertilizers and manures/compost were applied to the corn phase of the rotation in 2004 and 2006 but not to the soybean phase in 2005 and 2007.
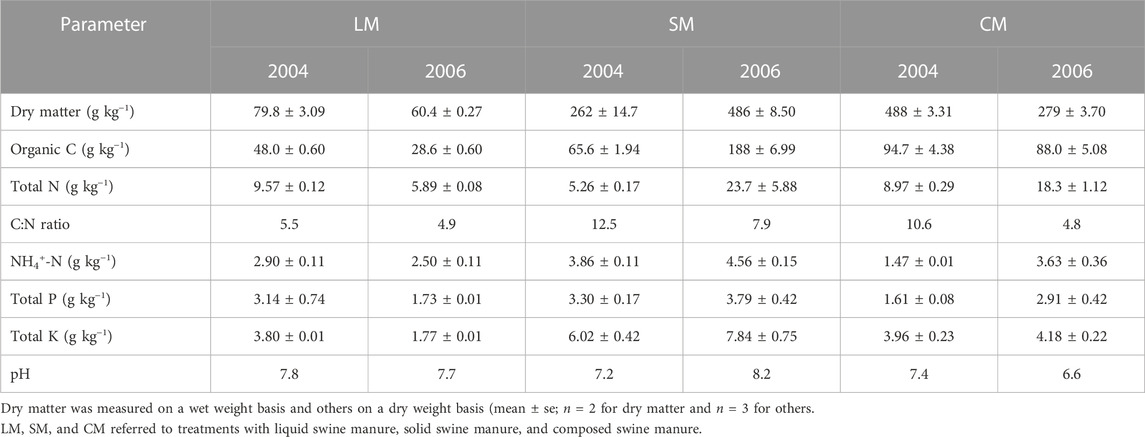
TABLE 3. Chemical and physical compositions of liquid, solid, and composted hog manure applied in the study.
Corn was seeded using a Kinze four-row planter (Kinze Manufacturing Co., Williamsburg, IA) with a row spacing of 75 cm (12 rows plot−1) at the rate of 76,852 seeds ha−1 on 24 June 2004 and 2 June 2006. Delayed planting in 2004 required an early-maturing variety (cv. N29A2, Syngenta Seeds Canada), whereas a normal variety (cv. N45A6, Syngenta Seeds Canada) was used in 2006. To ensure pest control, Roundup [glyphosate: (N-(phosphonomethyl) glycine), 1.4 kg a.i. ha−1] was applied on June 4, then, a mixture of Dual Magnum [s-metolachlor: (2-Chloro-N-(2-ethyl-6-methylphenyl)-N-((1S)-2-methoxy-1-methylethyl) acetamide, 1.4 kg a.i. ha−1], and Atrazine (6-Chloro-N2-ethyl-N4-isopropyl-1,3,5-triazine-2,4-diamine, 1.0 kg a.i. ha−1) were sprayed to all plots on 25 June 2004. In 2006, a mixture of Roundup (1.4 kg a.i. ha−1), Dual Magnum (1.2 kg a.i. ha−1), and Atrazine (1.0 kg a.i. ha−1) was sprayed to all plots on June 2.
Soybean was seeded on 19 May 2005 (cv. S24-K4 RR, Syngenta Seeds Canada) and on 24 May 2007 (cv. 92M52-RR, Pioneer Hi-Bred Ltd.), using a Kearney eight-row planter (Kearney Planters, Thamesville, ON) with a row spacing of 38.1 cm and a rate of 3,73,132 seeds ha−1. On 16 May 2005, Roundup (1.4 kg a.i. ha−1) was sprayed, and on May 18, a mixture of PPI-Dual II Magnum (1.4 kg a.i. ha−1) and Sencor (metribuzin: 4-Amino-6-(1,1-Dimethylethyl)-3-(methylthio)-1,2,4-triazin-5(4H)-one, 0.5 kg a.i. ha−1) was pre-planted and incorporated into the soil on the plots. In 2007, a mixture of Dual II Magnum (1.4 kg a.i. ha−1) and Sencor (0.5 kg a.i. ha−1) was sprayed in the seeding day, and again, Roundup (0.9 kg a.i. ha−1) was sprayed on June 25.
Manure preparation and application
The liquid hog manure applied in 2004 and 2006 was from a finishing pig producer located in Essex County, approximately 50 km east of Woodslee. The farm raised 550 animal units annually with premixed and blended corn and soybeans. A concrete tank built 3-m deep below the ground was used to collect the slurry. The manure samples were collected, chilled to 4°C, and analyzed before application. The storage tank was agitated slightly before pumping slurry into the spreader tank and was partially emptied every spring. A 4-manifold system applicator attached to the spreader tank, covering 3 m width of spread, was used for field operation. The amount of slurry required for the treatment was pre-calculated, as previously described. The rate of spreading by the applicator was pre-adjusted using running water. Three passes were used to cover a plot. In 2004, the manure was spread on the soil surface on June 16, and the plots were disked (5–8 cm), triple K-ed (6–8 cm), and packed on June 22. The delayed spread incorporation in June was due to rainfall in May and between spreading and incorporation. In 2006, the manure was spread on May 29 and incorporated into the soil on May 30.
In 2004, solid hog manure was obtained from the aforementioned farm. In 2006, solid manure was obtained from a similar finishing hog producer in Kent County, approximately 125 km northeast of Woodslee, with 300–600 animal units on average. In both farms, the manure mixed with wheat-straw beddings was cleaned weekly from the pen and was continuously piled up till use. After delivery to the experimental site, a composite of eight samples was taken from the pile and mixed. The samples were analyzed three to four days prior to the application. On the day of application, manure was put into the spreader, passed through double beaters into a pile, pitchforked into tarred garbage pails, and manually weighed to determine the required rates, and it was then loaded back into the manure spreader and spread on the soil surface. Incorporation was done on the day of application by disk, triple-K, and pack.
Hog manure composts were prepared at the Ridgetown Campus, University of Guelph at Kent County (east of Woodslee). Composting started in May, using a composter of 15.24 m × 2.13 m × 1.82 m. Liquid hog manure was sprayed onto shredded wheat-straw bales. The materials were turned over on days 1, 4, and 8, following the application. The initial moisture after the slurry application was 70%. After reaching 55°C and stabilized for the initial 24 h, the materials were kept at 55°C–70°C for 2 weeks and then removed from the composter and stockpiled to a height of 3.6–4.2 m. The sampling, mixing, calibration, and application of the compost in both years were the same as the solid manure. The compositions of manures used are shown in Table 3.
Sampling and data collection
Soil from each plot was sampled at pre-planting and post-harvest. Composite samples of 12 cores from 0–15 cm depths were hand-collected using a 2.54 cm diameter auger. Fresh samples were used for inorganic N and bulk density measurements. The samples were then air-dried, passed through a 2 mm sieve, and stored at 4°C before other analyses. The pipette method was used for analyzing the soil texture (Gee and Bauder, 1986). The organic C and total N were analyzed by dry-combustion (LECO CNS-1000 Analyzer, LECO Corp., St-Joseph, MI). Soil NH4- and NO3-N were extracted with 2 M KCl and determined colorimetrically (Keeney and Nelson, 1982). Soil test P was estimated as Olsen P (Olsen and Sommers, 1982). Soil test K was determined using the ammonium acetate (NH4OAc) procedure (Knudsen et al., 1982). Soil pH was determined in a soil/water ratio of 1:2. Bulk density was measured as described by Grossman and Reinsch (2002).
Manure samples were dried under a fume hood with forced air at 40°C for determining moisture and then manually ground to pass through a 0.5 mm sieve for chemical analysis. Carbon and total N were measured using the LECO Analyzer. The samples were digested with H2SO4-H2O2. The concentration of total P was determined using a flow injection auto-analyzer (QuikChemFIA+8000 series, Lachat Instruments, Loveland, CO) with the ammonium molybdate ascorbic acid reduction method by Murphy and Riley (1962). The concentration of total K was measured using a flame photometer connected to the flow injection auto-analyzer (QuikChemFIA+8000 series, Lachat Instruments, Loveland, CO). A 10 g aliquot of hog manure was diluted with 100 mL of 2 M KCl and shaken for an hour. The mixture was filtered with vacuum suction, and NH4- and NO3-N were determined colorimetrically (Keeney and Nelson, 1982).
After crops reached the maturity level, 12 and 16 corn plants per plot in 2004 and 2006, respectively, and 20 soybean plants per plot were randomly chosen from the rows. Plants were hand-cut right from above the ground. Fallen leaves were collected. Dry weights of biomass in 2005 and 2007 were calculated based on plant density. Grain and straw sub-samples were taken for N analysis using the LECO analyzer. A combine was used to determine corn grain yield on the central four rows (3.048 m × 25 m) and soybean yield on the central 12 rows (4.57 m × 25 m). The grain moisture was determined using a grain analysis computer (GAC200, Dickey–John Corp., Cornwall, ON). The yields reported were converted to contain 15% and 13% of the moisture for corn and soybeans, respectively.
Parameters for N use efficiency evaluation
The collected plant and soil data were used to performed N use efficiency analyses with seven parameters (Table 4): N use efficiency (NUE) (Eq. 1), N uptake efficiency (NUpE) (Eq. 2), N utilization efficiency (NUtE) (Eq. 3), N agronomical efficiency (NAE) (Eq. 4), N recovery efficiency (NRE) (Eq. 5), N physiological efficiency (NPE) (Eq. 6), and N harvest index (NHI) (Eq. 7).
where Nx and N0 denote the amount of N applied at a rate with designated treatment and at zero rate (CT), respectively, (the same definitions apply to the other N parameters thereafter).
Data analysis
Analysis of variance on crop yield, seed N uptake (N removal), plant N uptake and N-use efficiency parameters were conducted by PROC GLM (SAS Institute, 1999). Fisher’s protected LSD was employed for the mean separation of treatments. A paired t-test across treatments was used to compare the difference between years within the same crop. All statistical analyses were performed at the significance level of p ≤ 0.05. Figures were plotted using SigmaPlot (v12.5, SY Software, San Jose, CA).
Results and discussion
Corn and soybean yields
While both liquid (LM) and solid manure (SM) with adjusted total N produced comparable corn grain yields with IN in 2004 (Figure 1, 2004), only LM did in 2006. SM yielded significantly (p < 0.05) lower corn grain than IN and LM in 2006 (Figure 1, 2006). The grain yields with CM were significantly (p < 0.05) lower than those with LM and SM in both corn phases in the 4-year rotation. Averaged across the treatments, corn grain yield was significantly (p < 0.05) higher in 2006 (6,650 ± 471 kg ha−1) than in 2004 (5,300 ± 440 kg ha−1). In addition to the short season variety used in 2004, severe drought in September (Table 1) (precipitation was 25% of the 47-year average) might have also influenced the yield; even the corn was approaching the end of kernel filling in September. Corn is most susceptible to drought from the flowering stage to the kernel filling stage (Grant et al., 1989; Chapman et al., 1996). Corn kernels are particularly sensitive to the negative effects of drought stress during grain filling (Bruce et al., 2002; OMAFRA, 2022). Moisture stress at this stage could result in grain abortion and decreased productivity (Luo et al., 2010). Corn grain yield with IN in 2006 (9,000 ± 274 kg ha−1) was close to the expected agronomical yield (10,000 kg ha−1). As LM produced comparable yields relative to IN in both years, it suggested that the proposed N calibration coefficient was suitable for LM fertilization. The variable yield with SM implied that more variables governed the N availability in SM fertilization than in LM, such as pH, which might have altered the effects of solid manure application in acidic soils (pH = 5.8 in this study). Adjusting pH to near neutrality by manure application, the crop production in acidic soils can be improved greatly by increasing nutrient solubility (Whalen et al., 2000). While the total N in SM in 2006 was much higher, the proportion of inorganic N over total N and the resultant input of inorganic N was much lower than that in 2004 (Table 3). The fact that the grain yield did not benefit from CM application indicated that this composted hog manure was not a suitable N source for crop production under the current calibration system.
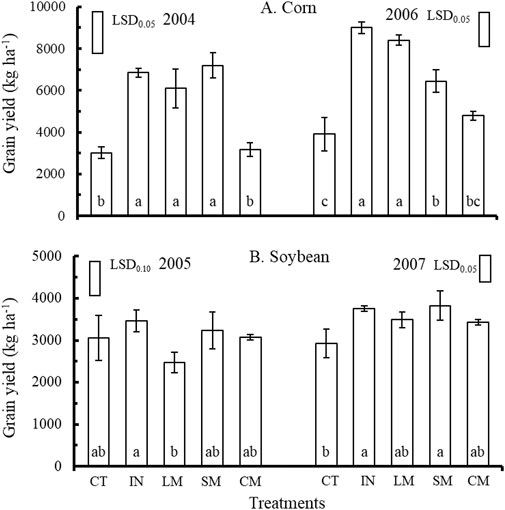
FIGURE 1. Grain yields of corn and soybean in rotation from 2004 to 2007. The abbreviations of CT, IN, LM, SM, and CM referred to the control without fertilization, inorganic fertilizer, liquid swine manure, solid swine manure and composed swine manure, respectively. There was no significant difference between the bars labeled with the same letter on it within the same year under Fisher’s protected LSD test at significance level of p ≤ 0.05.
No significant (p > 0.05) differences in soybean grain yield were found among the fertilized treatments and CT in 2005, except for a marginally lower yield in LM plots (Figure 1B). However, soybean grain yield was significantly (p < 0.05) higher in the fertilized plots than in the non-fertilized CT in 2007, probably attributable to the synthetic effects of residual N from preceding fertilization and symbiotic N2 fixation by soybean. On average, the yield was significantly (p < 0.05) higher in 2007 (3,380 ± 122 kg ha−1) than in 2005 (2,950 ± 164 kg ha−1), probably due to the severe drought in 2005 (Table 1) where the total precipitation was 56% of the 47-year average.
Grain N removal and total plant N uptake
The manifestation of grain N uptake as influenced by treatments with either corn or soybeans in any given year was similar to that of grain yields (Figure 2). The grain N removal by corn or soybeans was consistent between the two growing seasons. The pattern of total plant N uptake in corn as influenced by treatments in 2004 and 2006 resembled to that of corresponding grain N removal (Figure 2A; Figure 3A), while the total N uptake pattern in 2007 corresponded to grain N removal (Figures 2B, 3B), and no significant difference was observed among treatments in 2005. In contrast to the consistent grain N removal by corn or soybean between the two growing seasons, the averaged total N uptake was significantly (p < 0.05) lower in 2004 (77.1 ± 7.8 kg ha−1) than that in 2006 (88.2 ± 7.6 kg ha−1) for corn, while it was significantly (p < 0.05) higher in 2007 (210 ± 12.5 kg ha−1) than that in 2005 (190 ± 6.9 kg ha−1) for soybeans.
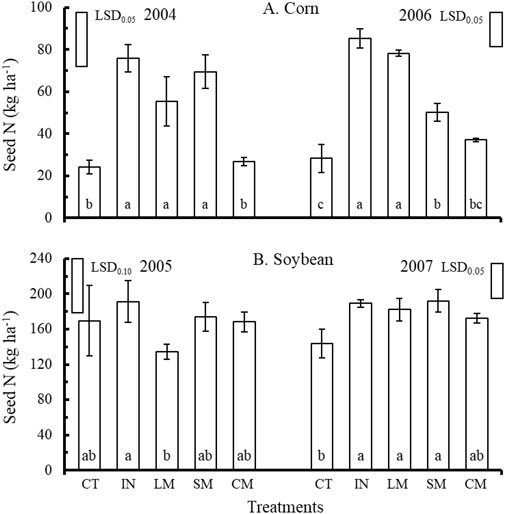
FIGURE 2. Grain N removal (seed N) of corn and soybeans in rotation from 2004 to 2007. The abbreviations of CT, IN, LM, SM, and CM referred to the control without fertilization, inorganic fertilizer, liquid swine manure, solid swine manure, and composed swine manure, respectively. There was no significant difference between the bars labeled with the same letter on it within the same year under Fisher’s protected LSD test at a significant level of p ≤ 0.05.
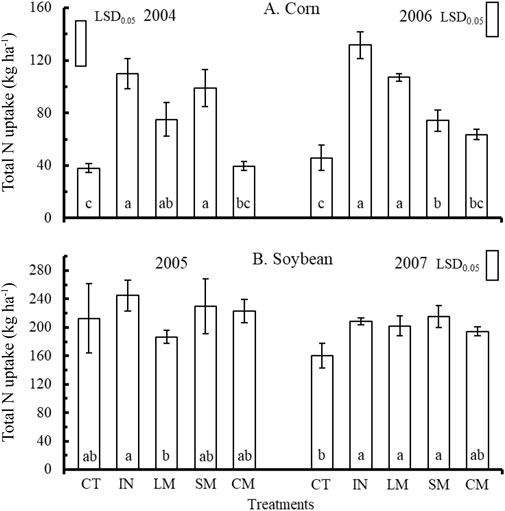
FIGURE 3. Total above-ground plant N uptake of corn and soybean in rotation from 2004 to 2007. The abbreviations of CT, IN, LM, SM, and CM referred to the control without fertilization, inorganic fertilizer, liquid swine manure, solid swine manure, and composed swine manure, respectively. There was no significant difference between the bars labeled with the same letter on it within the same year under Fisher’s protected LSD test at a significant level of p ≤ 0.05.
N use efficiency parameters with corn
The seven parameters involved in this study to evaluate the N use efficiency were summarized in Table 4. Each of them showed their validity to assess fertilizer N use on different aspects. While, NUE, NUpE, and NUtE were concerned about the total N supply or uptake that included soil residual N from the previous cropping system, NAE and NRE focused on the applied fertilizer N amount in farming practices (Table 4). However, NPE and NHI emphasized the N uptake difference between fertilization and zero-fertilization treatments (Table 4). Furthermore, the NUE and NUtE focused on the grain yield as the evaluation objective, but the rest regarded N uptake in either grain, above-ground, or N uptake increase as the target (Table 4).
Nitrogen use efficiency (NUE)
The index defines the efficiency of N from N supply to produce grain yield (Huggins and Pan, 1993; Li et al., 2019). In 2004 and 2006, per unit of total N supply in IN produced the highest grain yield among treatments as indicated by NUE (Figure 4). The CM produced the lowest yields, suggesting the N added with CM was inefficient in producing grain, presumably due to the N immobilization with the composted hog manure application. Although the C:N ratio (about 10) in CM was smaller than the reported critical ratio (15–16), triggering N immobilization in cattle manure (Beauchamp, 1986; Qian and Schoenau, 2002), N immobilization still apparently happened along with the composted hog manure application, as in addition to the C:N ratio, organic matter’s chemical structure, and composition of manure, and soil microbial biomass activity also affect N biological turnover (Kirchmann and Lundvall, 1993; de Freitas et al., 2003). It was found that lignin:N and polyphenol:N ratios in crop residues affected N mineralization negatively but promoted N immobilization (Trinsoutrot et al., 2000). Composting changed organic matter characteristics, and the N turnover from composted manure would be different from the un-composted ones (García et al., 1991; Yang et al., 2019). The organic matter in composted manure has a high molecular weight, polycondensed structure, and multiple bonding sites (Deiana et al., 1990; García et al., 1991; Yuan et al., 2019). They probably contain more energy per unit of weight to microbial biomass compared to the organic matter in un-composted organic materials. The relatively weak soil microbial biomass activity after the application of composted manure compared to low-C:N liquid manure (de Freitas et al., 2003; Lyyemperumal et al., 2008) might also not be conducive to the available N release from composted manure in this study. Further studies are needed to investigate if N immobilization in CM in our study was associated with high concentrations of these compounds.
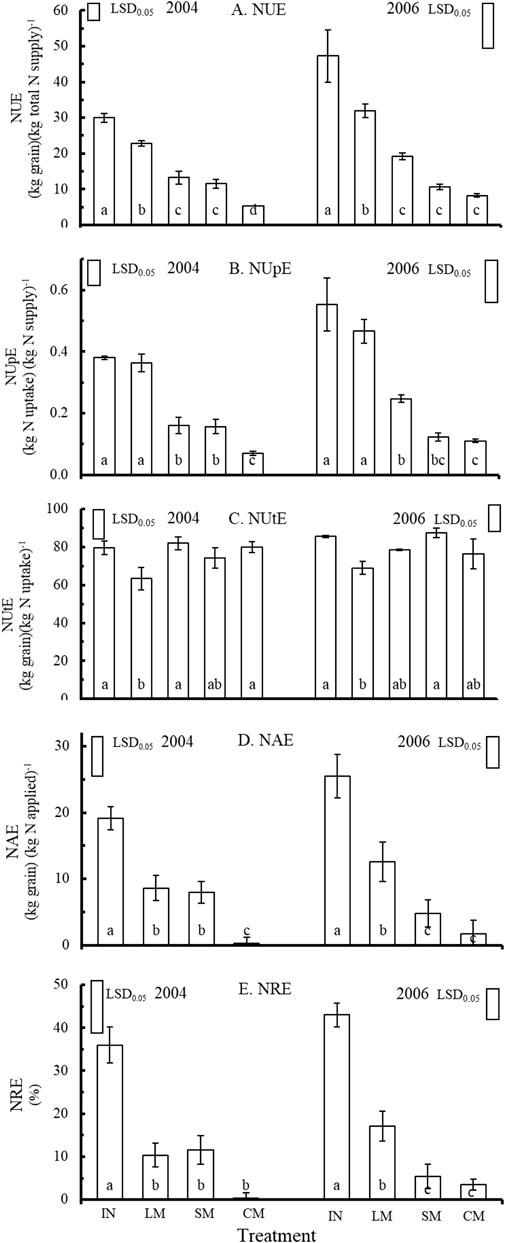
FIGURE 4. Changes in the selected N use efficiency parameters for corn as affected by nutrient sources. (A) NUE, N use efficiency; (B) NUpE, N uptake efficiency; (C) NUtE, N utilization efficiency; (D) NAE, N agronomical efficiency; and (E) NRE, N recovery efficiency. The bars under a designated year and followed by different letters are significantly different as determined by the protected LSD. The abbreviations of IN, LM, SM, and CM referred to the treatments with inorganic fertilizer, liquid swine manure, solid swine manure, and composed swine manure, respectively.
Corresponding to grain yields, the averaged NUE overall treatments were significantly (p < 0.05) higher in 2006 compared to those in 2004. The N supply in NUE included soil inorganic N at seeding, mineralized soil N (Mmin) during the growing season, and the N applied. The Nmin was estimated as plant N uptake minus the difference between pre-planting and post-harvest soil inorganic N in CT (Huggins and Pan, 1993). The estimated Nmin (11.2 ± 8.4 and 33.5 ± 12.2 kg ha−1 in 2004 and 2006, respectively) appeared to be smaller as compared with in situ measurements (ranging from 96 to 120 kg N ha−1 during the corn growing season, equivalent to 2%–3% of the soil total N) from two other soils in eastern Ontario (Wu et al., 2008), as the Nmin in this study presented the net mineralization with the immobilized N and N loss through leaching and denitrification excluded.
Nitrogen uptake efficiency (NUpE)
The NUpE represented the efficiency of total N supply to crop N uptake (López-Bellido and López-Bellido, 2001). In this study, the higher NUpE with IN compared with the manure treatments was plausibly explained by the dynamics of available N supply. In contrast to that, a portion of organic N was not immediately available in manure treatments; the N in CT and IN was readily absorbed by plants any time. The lowest NUpE value with CM indicated that the highest amount of N unavailable for crop uptake during the growing seasons. As with NUE, NUpE was higher in 2006 compared to that in 2004, resulting in higher plant N uptake in 2006 than in 2004.
Nitrogen utilization efficiency (NUtE)
This parameter described the efficiency of assimilated N by the plant in grain production (Barraclough et al., 2010; Gouis et al., 2010). CM had higher NUtE compared with other fertilization treatments because N was a limited factor in the plots with the composed hog manure. With the N deficit, crops use the N assimilated more efficiently to maximize grain production (Ottman, et al., 2000). The result was also observed in a study with controlled releasing chemical fertilizer. The controlled releasing chemical fertilizer at the later growing stage was more efficiently used for grain production compared to the chemical N applied at seeding (Wen et al., 2001). A portion of absorbed N from LM and SM stemmed from the decomposition of the organic manure matter; it was released slowly and absorbed at a later stage of crop production; thus, it could be used more efficiently to grain production, reflected by higher NUtE with LM and SM. In contrast to the yield and total N uptake, the averaged NUtE was comparable between the growing seasons as the index reflects the dynamics of available N supply solely.
Nitrogen-agronomical efficiency (NAE)
The NAE measures the efficiency of applied N in producing grain yield (Craswell and Godwin, 1984). Per unit of N applied with IN produced more than double yields compared to that with LM and SM in 2004 and 2006 (Figure 4), as the chemical fertilizer N was the most effective N source. Nitrogen from CM had minor contribution to yield, thus, had minor agronomical value in this study. No difference in NAEs was found between the 2 years, as the index reflects the nature of a fertilizing material in the ability to supply available N for grain production.
Nitrogen recovery efficiency (NRE)
About 36% and 43% of applied N with IN were recovered in 2004 and 2006, respectively, tripling that measured with LM and SM in 2004, and more than doubling that with LM and five folding that with SM in 2006 (Figure 4). Recovery from CM was very small in both the years, suggesting very limited N released from CM during the corn growing season.
Nitrogen physiological efficiency (NPE)
The NPE represented the yield associated with N from the applied N (Craswell and Godwin, 1984). The denominator representing the N uptake associated with the applied N. NPE is a further development of NUtE, defining the efficiency of assimilating N from the specified source to grain production. By introducing two more variables, the index increases the variability. In 2004, 54.9 ± 9.1, 85.3 ± 4.6, and 74.0 ± 12.9 (kg grain kg−1 N applied) were measured with IN, LM, and SM, respectively. In 2006, only IN and LM were measurable [59.4 ± 7.0 and 72.1 ± 6.0 (kg grain kg−1 N applied), respectively]. In general, the slightly higher NPEs with manures than with IN in both the years supported the assumption that the absorbed N from the manure sources at a later stage of crop growth was more efficiently used to produce grain (Ottman et al., 2000; Wen et al., 2001).
Nitrogen harvest index (NHI)
The NHI described the transfer efficiency of assimilated N from a specified N source to the grain associated with that source (Daba, 2017). In 2004, the NHI was 72.6 ± 5.0, 83.5 ± 2.8, and 76.8 ± 5.4 (%), and in 2006, 66.4 ± 3.8, 83.5 ± 4.6, and 89.9 ± 10.0 (%) for IN, LM, and SM, respectively. The higher NHIs with LM and SM than with IN indicated that the higher portion of N in grain stemmed from the manure sources at a later stage of crop production.
N use efficiency parameters with soybeans
The N uptake of soybeans either by grain or by the total plant was much higher than that of corn (Figure 2; Figure 3). Available N supply in the subsequent year was often much less than that in the year of application, regardless of chemical N fertilizer or manure (Anderson et al., 1997; Sørensen and Amato, 2002; Wen et al., 2003; Anderson, 2021). The majority of N in soybeans was from its symbiotic N2-fixation. Addition of N to soybean grown in fertile southern Ontario soils is not recommended, as it may delay root nodulation (OMAFRA, 2009). In addition to N, other nutrients applied, particularly for P, would affect soybean growth by influencing root nodule development and, subsequently, fixing N2 (Wen et al., 2008). Although the varied yield and the N uptake of soybeans were influenced by the overall residual effects left by corn, the determination of N use efficiency would help identify the best growing environment for soybeans.
The NAE and NRE were higher with IN than with others in most cases (Figure 5). On the contrary, the NAE and NRE were negative with LM in 2005, reflecting a deteriorated growth environment by the previous LM application for soybeans. Better growth conditions in 2007 resulted in significantly higher NAE and NRE in 2007 compared to those in in 2005.
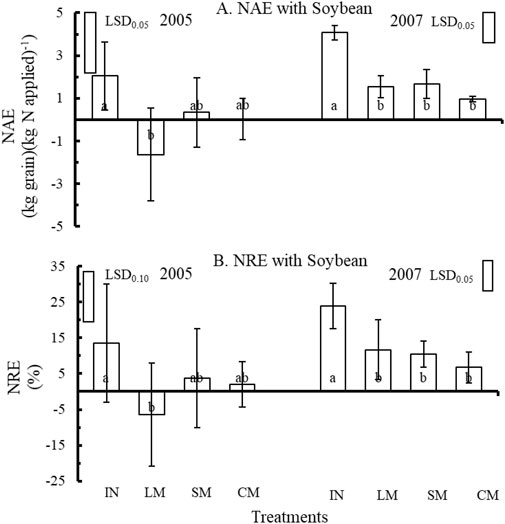
FIGURE 5. Changes in the selected N use efficiency parameters for soybeans as affected by nutrient sources applied in preceding corn. NAE, N agronomical efficiency, and NRE, N recovery efficiency. The bars under a designated year and followed by different letters are significantly different as determined by the protected LSD. The abbreviations of IN, LM, SM, and CM referred to the treatments with inorganic fertilizer, liquid swine manure, solid swine manure, and composed swine manure, respectively.
The effect of treatments on NUtE, NHI, and NPE faded out in both 2005 and 2007; this was attributed to the fact that most of the absorbed N for all the treatments was from the same source of self N2-fixation. Averagely, the values were higher in 2007 than in 2005, specifically, 17.8 ± 0.1 vs. 14.8 ± 0.3 (kg grain kg−1 total N uptake) for NUtE, 0.95 ± 0.04 vs. 0.77 ± 0 (%) for NHI, and 16.2 ± 0.7 vs. 13.1 ± 1.6 (kg grain kg−1 N applied) for NPE. Again, better growth conditions in 2007 resulted in higher N uptake, higher transfer of uptake N to grain, and subsequently higher grain yield compared to that in 2005. The legacy effects of treatments were plausibly reflected by NAE and NRE but not by NUtE, NHI, and NPE. This is because the former two indices focus on the N applied only, whereas NUtE, NHI, and NPE emphasize total N supply or uptake that cannot discriminate N sources of external applied and soil residual.
Conclusion
Judged by yield in conjunction with NUE, NUpE, NAE, and NRE, the corn with IN performed the best in both 2004 and 2006, followed by LM. Interestedly, CM had little agronomic value in our study. Comparable yields between IN and LM treatments for the rotation corn in two alternative years proved that N in liquid manure can be used as a predictable N source for the agronomic production of corn. However, this study demonstrated distinctions in the dynamics of N supply between chemical fertilizer and liquid hog manure by measuring seven N use efficiency parameters. While the higher NUE, NUpE, NAE, and NRE with IN quantified its advantages over manure N in multi-aspects, the higher NUtE with LM revealed that the crop absorbed N from its slowly released organic N was more efficiently used to produce grain for corn. The corn yield promotion by the N in solid hog manure was inconsistent in the 2 years. Care should be taken to use the proposed N calibration to project the available N in solid hog manure. For the subsequent soybean in the rotation, higher NAE and NRE with IN than with the manure sources indicated that inorganic N applied to the preceding corn had left better production potentials for the subsequent soybean from a plant nutrition point of view.
Data availability statement
The original contributions presented in the study are included in the article/Supplementary Material; further inquiries can be directed to the corresponding author.
Author contributions
JN: data validation, analysis participation, and manuscript preparation. TZ: conceptualization, funding acquisition, project administration, methodology, and writing—review and editing. GW, ZZ, and YJ: data analysis or participation, manuscript preparation participation, and review and editing. CT and TW: investigation, collaboration, and writing—review and editing.
Funding
This study was funded by the Agriculture and Agri-Food Canada A-Base Research Program.
Acknowledgments
Analytical and other technical assistances from Mary-Anne Reeb and Brian Hohner are acknowledged. The senior author acknowledges the finacial support from the Planning Fund of the Ministry of Educatiion, Humanities and Social Sciences (19YJAZH066), the Philosophy and Social Sciences Key Research Base of Higher Education Institutions of Shanxi (20200133), and the Research Project Supported by Shanxi Scholarship Council of China (2017-105).
Conflict of interest
The authors declare that the research was conducted in the absence of any commercial or financial relationships that could be construed as a potential conflict of interest.
Publisher’s note
All claims expressed in this article are solely those of the authors and do not necessarily represent those of their affiliated organizations, or those of the publisher, the editors, and the reviewers. Any product that may be evaluated in this article, or claim that may be made by its manufacturer, is not guaranteed or endorsed by the publisher.
References
Allen, S. C., Nair, V. D., Graetz, D. A., Jose, S., and Nair, P. R. (2006). Phosphorus loss from organic versus inorganic fertilizers used in alleycropping on a Florida ultisol. Agric. Ecosyst. Environ. 117, 290–298. doi:10.1016/j.agee.2006.04.010
Anderson, D. (2021). Manure nitrogen availability from manures and N application recommendations around the midwest. Ames: The Manure Scoop. Available at: http://themanurescoop.blogspot.com/2021/01/manure-nitrogen-availability-from.html (Accessed on Jan. 13, 2023).
Anderson, I. C., Buxton, D. R., Karlen, D. L., and Cambardella, C. (1997). Cropping system effects on nitrogen removal, soil nitrogen, aggregate stability, and subsequent corn grain yield. Agron. J. 89, 881–886. doi:10.2134/agronj1997.00021962008900060006x
Azeez, J. O., and Van Averbeke, W. (2010). Nitrogen mineralization potential of three animal manures applied on a sandy clay loam soil. Bioresour. Technol. 101 (14), 5645–5651. doi:10.1016/j.biortech.2010.01.119
Badaruddin, M., and Meyer, D. W. (1994). Grain legume effects on soil nitrogen, grain yield, and nitrogen nutrition of wheat. Crop Sci. 34, 1304–1309. doi:10.2135/cropsci1994.0011183x003400050030x
Barraclough, P. B., Howarth, J. R., Jones, J., Lopez-Bellido, R., Parmar, S., Shepherd, C. E., et al. (2010). Nitrogen efficiency of wheat: Genotypic and environ-mental variation and prospects for improvement. Eur. J. Agron. 33, 1–11. doi:10.1016/j.eja.2010.01.005
Beauchamp, E. G. (1986). Availability of nitrogen from three manures to corn in the field. Can. J. Soil Sci. 66, 713–720. doi:10.4141/cjss86-070
Beegle, D. B., and Lanyon, L. E. (1994). Understanding the nutrient management process. J. Soil Water Conserv. 49 (2), S23.
Bruce, W. B., Edmeades, G. O., and Barker, T. C. (2002). Molecular and physiological approaches to maize improvement for drought tolerance. J. Exp. Bot. 53, 13–25. doi:10.1093/jexbot/53.366.13
Butler, T. J., and Muir, J. P. (2006). Dairy manure compost improves soil and increases tall wheatgrass yield. Agron. J. 98, 1090–1096. doi:10.2134/agronj2005.0348
Chapman, S. C., Edmeades, G. O., and Crossa, J. (1996). “Pattern analysis of grains from selection for drought tolerance in tropical maize population,” in Plant adaptation and crop improvement. Editors M. Cooper,, and G. L. Hammer (UK: CAB INTERNATIONAL: Walling Ford), 513–527.
Craswell, E. T., and Godwin, D. C. (1984). The efficiency of nitrogen fertilizers applied to cereals grown in different climates. (No. REP-3326. CIMMYT.).
Cui, N., Cai, M., Zhang, X., Abdelhafez, A. A., Zhou, L., Sun, H., et al. (2020). Runoff loss of nitrogen and phosphorus from a rice paddy field in the east of China: Effects of long-term chemical N fertilizer and organic manure applications. Glo. Eco. Conser. 22, e01011. doi:10.1016/j.gecco.2020.e01011
Daba, N. A. (2017). Influence of nitrogen fertilizer application on grain yield, nitrogen uptake efficiency and nitrogen use efficiency of bread wheat (Triticum aestivum L.) cultivars in Eastern Ethiopia. J. Agric. Sci. 9 (7), 202–217. doi:10.5539/jas.v9n7p202
de Freitas, J. R., Schoenau, J. J., Boyetchko, S. M., and Cyrenne, S. A. (2003). Soil microbial populations, community composition, and activity as affected by repeated applications of hog and cattle manure in eastern Saskatchewan. Can. J. Microbiol. 49, 538–548. doi:10.1139/w03-069
Deiana, E., Gessa, C., Manunza, B., Rausa, R., and Seeber, R. (1990). Analytical and spectroscopic characterization of humic acid extracted from sewage sludge, manure and worm compost. Soil Sci. 150, 419–424. doi:10.1097/00010694-199007000-00003
Delogu, G., Cattivelli, L., Pecchioni, N., De Falcis, D., Maggiore, T., and Stanca, A. M. (1998). Uptake and agronomic efficiency of nitrogen in winter barley and winter wheat. Eur. J. Agron. 9, 11–20. doi:10.1016/s1161-0301(98)00019-7
Edmeades, D. C. (2003). The long-term effects of manures and fertilisers on soil productivity and quality: A review. Nutr. Cycl. Agroecosys. 66, 165–180. doi:10.1023/a:1023999816690
Eghball, B., Ginting, D., and Gilley, J. E. (2004). Residual effects of manure and compost applications on corn production and soil properties. Agron. J. 96, 442–444. doi:10.2134/agronj2004.0442
EnrioStats (2015). A geographical profile of livestock manure production in Canada, 2006. Available at: https://www150.statcan.gc.ca/n1/pub/16-002-x/2008004/article/10751-eng.htm#a6 (Accessed on July 5, 2022).
Fraser, H. (1985). Manure characteristics. Factsheet order No. 85-109. Guelph, ON, Canada: Ontario Ministry of Agriculture and Food.
Gale, E. S., Sullivan, D. M., Cogger, C. G., Bary, A. I., Hemphill, D. D., and Myhre, E. A. (2006). Estimating plant-available nitrogen release from manures, composts, and specialty products. J. Environ. Qual. 35, 2321–2332. doi:10.2134/jeq2006.0062
García, C., Hernades, T., Costa, F., and Polo, A. (1991). Humic substances in composted sewage sludge. Waster Manage. Res. 9, 189–194. doi:10.1016/0734-242x(91)90004-q
Gee, G. W., and Bauder, J. W. (1986). “Particle-size analysis,” in Methods of soil analysis. Part 1. Physical and mineralogical methods. Agron. Monogr. Editor A. Klute (USA: SSSA Madison WI), 383–411.
Gouis, J. L., Gaju, O., Hubbart, S., Allard, V., Orford, S., Heumez, H., et al. (2010). Genetic improvement for increased nitrogen use efficiency in wheat. Asp. Appl. Bio. 105, 151–158.
Grant, R. F., Jakson, B. S., Kiniry, J. R., and Arkin, G. F. (1989). Water deficit timing effects on yield components in maize. Agron. J. 81, 61–65. doi:10.2134/agronj1989.00021962008100010011x
Grossman, R. B., and Reinsch, T. G. (2002). “Chapter 2. The solid phase. 2.1.2 core method,” in Methods of soil analysis. Part 4. Physical methods. Editor W. A. Dick (USA: SSSA Madison WI), 207–210.
Hao, X,J., Zhang, T. Q., Wang, Y. T., Tan, C. S., Qi, Z. M., Welacky, T., et al. (2018). Soil test phosphorus and phosphorus availability of swine manures with long-term application. Agron. J. 110, 1943–1950. doi:10.2134/agronj2017.07.0412
Hopkins, M., Beck, M., Rossi, E., Luh, N., Allen-King, R. M., and Lowry, C. (2016). “Quantifying the urban and rural nutrient fluxes to lake erie using a paired watershed approach,” in AGU fall meeting abstracts.
Hua, W., Luo, P., An, N., Cai, F., Zhang, S., Chen, K., et al. (2020). Manure application increased crop yields by promoting nitrogen use efficiency in the soils of 40-year soybean-maize rotation. Sci. Rep. 10, 14882. doi:10.1038/s41598-020-71932-9
Huggins, D. R., and Pan, W. L. (1993). Nitrogen efficiency component analysis: An evaluation of cropping system differences in productivity. Agron. J. 85, 898–905. doi:10.2134/AGRONJ1993.00021962008500040022X
Keeney, D. R., and Nelson, D. W. (1982). “Nitrogen-Inorganic forms,” in Methods of soil analysis. Part 2. Chemical and microbiological properties. Editor Pageet al. (USA: SSSA Madison WI), 539–579.
Khan, S. U., O’Sullivan, T. L., Poljak, Z., Alsop, J., and Greer, A. L. (2018). Modeling livestock population structure: A geospatial database for Ontario swine farms. BMC veterinary Res. 14 (1), 31–11. doi:10.1186/s12917-018-1362-y
Kirchmann, H., and Lundvall, A. (1993). Relationship between N immobilization and volatile fatty acids in soil after application of pig and cattle slurry. Biol. Fert. Soils 15, 161–164. doi:10.1007/bf00361605
Knudsen, D., Peterson, G. A., and Fratt, P. F. (1982). “Lithium, sodium, and potassium,” in Methods of soil analysis. Part 2. Chemical and microbiological properties. Editor Pageet al. (USA: SSSA Madison WI), 225–245.
Latiri-Souki, K., Nortcliff, S., and Lawlor, D. W. (1998). Nitrogen fertilizer can increase dry matter, grain production and radiation and water use efficiencies for durum wheat under semi-arid conditions. Eur. J. Agron. 9, 21–34. doi:10.1016/s1161-0301(98)00022-7
Li, Y., Li, Z., Cui, S., Chang, S. X., Jia, C., and Zhang, Q. (2019). A global synthesis of the effect of water and nitrogen input on maize (Zea mays) yield, water productivity and nitrogen use efficiency. Agric. For. Meteorol. 268, 136–145. doi:10.1016/j.agrformet.2019.01.018
Lithourgidis, A. S., Matsi, T., Barbayiannis, N., and Dordas, C. A. (2007). Effect of liquid cattle manure on corn yield, composition, and soil properties. Agron. J. 99, 1041–1047. doi:10.2134/agronj2006.0332
López-Bellido, R. J., and López-Bellido, L. (2001). Efficiency of nitrogen in wheat under mediterranean conditions: Effect of tillage, crop rotation and N fertilization. Field Crops Res. 71 (1), 31–46. doi:10.1016/s0378-4290(01)00146-0
Luo, M., Liu, J., Lee, D. R., Scully, B. T., and Guo, B. Z. (2010). Monitoring the expression of maize genes in developing kernels under drought stress using oligo-microarray. J. Integr. Plant Biol. 52, 1059–1074. doi:10.1111/j.1744-7909.2010.01000.x
Lyyemperumal, K., Green, J., Israel, D. W., Ranells, N. N., and Wei, S. (2008). Soil chemical and microbiological properties in hay production systems: Residual effects of contrasting N fertilization of swine lagoon effluent versus ammonium nitrate. Biol. Fertil. Soils. 44, 425–434. doi:10.1007/s00374-007-0221-y
Mooleki, S. P., Schoenau, J. J., Charles, J. L., and Wen, G. (2004). Effect of rate, frequency and incorporation of feedlot cattle manure on soil nitrogen availability, crop performance and nitrogen use efficiency in east-central Saskatchewan. Can. J. Soil Sci. 84, 199–210. doi:10.4141/s02-045
Murphy, J., and Riley, J. P. (1962). A modified single solution method for the determination of phosphate in natural waters. Anal. Chim. Acta. 27, 31–36. doi:10.1016/s0003-2670(00)88444-5
Norvan, T., Leterme, P., Arsene, G. G., and Mary, B. (1997). Nitrogen transformation after the spreading of pig slurry on bare soil and ryegrass using 15N-labeled ammonium. Eur. J. Agron. 7, 181–188.
Olsen, S. R., and Sommers, L. E. (1982). “Phosphorus,” in Methods of soil analysis. Part 2. Chemical and microbiological properties. (USA: SSSA Madison WI), 403–427.
OMAFRA (Ontario Ministry of Agriculture, Food and Rural Affaires) (2002). Agronomy guide for field crops. Toronto, ON, Canada: Pub 811; Queen’s Printer for Ontario.
OMAFRA (Ontario Ministry of Agriculture, Food and Rural Affaires) (2009). Agronomy guide for field crops. Guelph, ON, Canada: Pub 811. Ontario Ministry of Agriculture, Food and Rural Affaires.
OMAFRA (Ontario Ministry of Agriculture, Food and Rural Affaires) (2022). Drought in field corn. Accessed on Jan. 13, 2023. Available at: http://omafra.gov.on.ca/english/crops/weather/droughtinfieldcorn.htm.
Ottman, M. J., Doerge, T. A., and Martin, E. C. (2000). Durum grain quality as affected by nitrogen fertilization near anthesis and irrigation during grain fill. Agron. J. 92 (5), 1035–1041. doi:10.2134/agronj2000.9251035x
Peu, P., Birgand, F., and Martinez, J. (2007). Long term fate of slurry derived nitrogen in soil: A case study with a macro-lysimeter experiment having received high loads of pig slurry (solepur). Bioresour. Technol. 98, 3228–3234. doi:10.1016/j.biortech.2006.07.019
Qian, P., and Schoenau, J. J. (2002). Availability of nitrogen in solid manure amendments with different C:N ratios. Can. J. Soil Sci. 82, 219–225. doi:10.4141/s01-018
Qin, X., Guo, S., Zhai, L., Pan, J., Khoshnevisan, B., Wu, S., et al. (2020). How long-term excessive manure application affects soil phosphorous species and risk of phosphorous loss in fluvo-aquic soil. Environ. Pollut. 266, 115304. doi:10.1016/j.envpol.2020.115304
Rao, A. C. S., Smith, J. L., Parr, J. F., and Papendick, R. I. (1992). Considerations in estimating nitrogen recovery efficiency by the difference and isotopic dilution methods. Fertil. Res. 33, 209–217. doi:10.1007/BF01050876
Ribbe, L., Delgado, P., Salgado, E., and Flügel, W. A. (2008). Nitrate pollution of surface water induced by agricultural non-point pollution in the Pocochay watershed, Chile. Desalination 226 (1-3), 13–20. doi:10.1016/j.desal.2007.01.232
Saam, H., Powell, J. M., Jackson-Smith, D. B., Bland, W. L., and Posner, J. L. (2005). Use of animal density to estimate manure nutrient recycling ability of Wisconsin dairy farms. Agr. Sys. 84 (3), 343–357. doi:10.1016/j.agsy.2004.06.020
Schmitt, M. A., Russelle, M. P., Randall, G. W., and Lory, J. A. (1999). Manure nitrogen crediting and management in the USA: Survey of University faculty. J. Prod. Agric. 12, 419–422. doi:10.2134/jpa1999.0419
Sommer, S. G., Petersen, S. O., Sørensen, P., Poulsen, H. D., and Møller, H. B. (2007). Methane and carbon dioxide emissions and nitrogen turnover during liquid manure storage. Nutr. Cycl. Agroecosyst. 78, 27–36. doi:10.1007/s10705-006-9072-4
Sørensen, P., and Amato, M. (2002). Remineralisation and residual effects of N after application of pig slurry to soil. Eur. J. Agron. 16, 81–95. doi:10.1016/s1161-0301(01)00119-8
Sørensen, P., and Jensen, E. S. (1995). Mineralization-immobilization and plant uptake of nitrogen as influenced by the spatial distribution of cattle slurry in soils of different texture. Plant Soil 173, 283–291. doi:10.1007/bf00011466
Sowers, K. E., Pan, W. L., Miller, B. C., and Smith, J. L. (1994). Nitrogen use efficiency of split nitrogen applications in soft white winter wheat. Agron. J. 86, 942–948. doi:10.2134/agronj1994.00021962008600060004x
Statistics Canada (2008). Catalogue no. 23-010-XIE. Hog statistics, 7. Ottawa, ON, Canada: Ministry of Industry.
Sutton, A. L., Nelson, D. W., Kelly, D. T., and Hill, D. L. (1986). Comparison of solid vs. liquid dairy manure applications on corn yield and soil composition. J. Environ. Qual. 15, 370–375. doi:10.2134/jeq1986.00472425001500040010x
Tate, R. L. (2020). “The nitrogen cycle: Mineralization, immobilization, and nitrification,” in Soil microbiology (John Wiley and Sons), 355–388.
Trehan, S. P., and Wild, A. (1993). Effects of an organic manure on the transformations of ammonium nitrogen in planted and unplanted soil. Plant Soil 151, 287–294. doi:10.1007/bf00016295
Trinsoutrot, I., Recous, S., Bentz, B., Lineres, M., Cheneby, D., and Nicolardot, B. (2000). Biochemical quality of crop residues and carbon and nitrogen mineralization kinetics under nonlimiting nitrogen conditions. Soil Sci. Soc. Am. J. 64, 918–926. doi:10.2136/sssaj2000.643918x
Webb, J., Sorensen, P., Velthof, G. L., Amon, B., Pinto, M., Rodhe, L., et al. (2011). Study on variation of manure N efficiency throughout Europe. Luxembourg: AEA Technology plc.
Wen, G., Mori, T., Yamamoto, T., Chikushi, J., and Inoue, M. (2001). Nitrogen recovery of coated fertilizers and influence on peanut seed quality for peanut plants grown in sandy soil. Comm. Soil Sci. Plant Anal. 32 (19-20), 3121–3140. doi:10.1081/css-120001111
Wen, G., Bates, T. E., Voroney, R. P., Yamamoto, T., Chikushi, J., and Curtin, D. (2002a). A yield control approach to assess phytoavailability of Zn and Cu in irradiated, composted sewage sludges and composted manure in field experiments: 1. Zinc. Plant Soil 246, 231–240. doi:10.1023/a:1020661225434
Wen, G., Bates, T. E., Inanaga, S., Voroney, R. P., Hamamura, K., and Curtin, D. (2002b). A yield control approach to assess phytoavailability of Zn and Cu in irradiated, composted sewage sludges and composted manure in field experiments: 2. Copper. Plant Soil 246, 241–248. doi:10.1023/a:1020665309504
Wen, G., Schoenau, J. J., Charles, J. L., and Inanaga, S. (2003). Efficiency parameters of nitrogen in hog and cattle manure in the second year following application. J. Plant Nutri. Soil Sci. 166, 490–498. doi:10.1002/jpln.200321135
Wen, G., Chen, C., Neill, K., Wichman, D., and Jackson, G. (2008). Yield response of pea, lentil and chickpea to phosphorus addition in a clay loam soil of central Montana. Arch. Agron. Soil Sci. 54, 69–82. doi:10.1080/03650340701614239
Whalen, J. K., Chang, C., Clayton, G. W., and Carefoot, J. P. (2000). Cattle manure amendments can increase the pH of acid soils. Soil Sci. Soc. Am. J. 64, 962–966. doi:10.2136/sssaj2000.643962x
Wong, J. W. C., and Ho, G. E. (1991). Effects of gypsum and sewage sludge amendment on physical properties of fine bauxite refining residue. Soil Sci. 152, 326–332. doi:10.1097/00010694-199111000-00003
Wu, T. Y., Ma, B. L., and Liang, B. C. (2008). Quantification of seasonal soil nitrogen mineralization for corn production in eastern Canada. Nutri. Cycl. Agroecosys. 81, 279–290. doi:10.1007/s107
Yamoah, C. F., Varvel, G. E., Waltman, W. J., and Francis, C. A. (1998). Long-term nitrogen use and nitrogen-removal index in continuous crops and rotations. Field Crop Res. 57, 15–27. doi:10.1016/s0378-4290(97)00109-3
Yang, X., Liu, E., Zhu, X., Wang, H., Liu, H., Liu, X., et al. (2019). Impact of composting methods on nitrogen retention and losses during dairy manure composting. Int. J. Environ. Res. Public Health. 16 (18), 3324. doi:10.3390/ijerph16183324
Yang, Y. J., Lei, T., Du, W., Liang, C. L., Li, H. D., and Lv, J. L. (2020). Substituting chemical fertilizer nitrogen with organic manure and comparing their nitrogen use efficiency and winter wheat yield. J. Agri. Sci. 158, 262–268. doi:10.1017/s0021859620000544
Yuan, Y., Xi, B., He, X. S., Tan, W., Zhang, H., Li, D., et al. (2019). Polarity and molecular weight of compost-derived humic acids impact bio-dechlorination of pentachlorophenol. J. Agric. Food Chem. 67 (17), 4726–4733. doi:10.1021/acs.jafc.8b05864
Keywords: swine manure, nitrogen use efficiency, liquid manure, solid manure, compost, nitrogen fertilizer
Citation: Niu J, Zhang T, Wen G, Zheng Z, Jia Y, Tan CS and Welackey T (2023) Agronomic approach to evaluate the nitrogen use efficiency of liquid, solid, and composted swine manures in corn–soybean rotation. Front. Environ. Sci. 11:1021890. doi: 10.3389/fenvs.2023.1021890
Received: 17 August 2022; Accepted: 16 January 2023;
Published: 09 February 2023.
Edited by:
Lucy Louise Burkitt, Massey University, New ZealandCopyright © 2023 Niu, Zhang, Wen, Zheng, Jia, Tan and Welackey. This is an open-access article distributed under the terms of the Creative Commons Attribution License (CC BY). The use, distribution or reproduction in other forums is permitted, provided the original author(s) and the copyright owner(s) are credited and that the original publication in this journal is cited, in accordance with accepted academic practice. No use, distribution or reproduction is permitted which does not comply with these terms.
*Correspondence: Tiequan Zhang, VGllcXVhbi5aaGFuZ0BhZ3IuZ2MuY2E=