- 1Institute for Wetland and Waterfowl Research, Ducks Unlimited Canada, Stonewall, MB, Canada
- 2Biological Sciences, University of Manitoba, Winnipeg, MB, Canada
Once introduced to shallow aquatic ecosystems common carp (Cyprinus carpio) often degrade habitat, negatively impacting the native organisms that rely on these systems. Detrimental effects often observed following the introduction of carp include a reduction in water clarity as bottom sediments become disturbed and resuspended and phytoplankton blooms become more severe and frequent. This results in a reduction of submersed aquatic vegetation (SAV), the effects of which are felt across multiple trophic levels. We sought to limit large carp (>70 mm maximum body width) access to a culturally and biologically significant 18,500 ha freshwater coastal wetland located in Manitoba, Canada to restore pre-carp conditions which were characterized by clear water and abundant SAV. In winter 2012–2013, exclusion structures were built to limit access by large carp to Delta Marsh during the spring and summer. A monitoring program (2009–2018) compared marsh conditions before and after carp exclusion. Water clarity improved following carp exclusion, largely driven by a reduction of inorganic suspended solids (ISS) rather than phytoplankton biomass, indicating that maintaining clear water conditions might be supplemented by reductions in nutrient export from agricultural areas adjacent to the marsh. The decrease in ISS and phytoplankton varied spatially, with the greatest change observed in the westernmost area of the marsh which is more sheltered compared to the large open bays characterizing eastern areas of the marsh. SAV doubled in percent cover through the 6 years of monitoring post-carp exclusion and SAV cover and species richness in the marsh was comparable to what was present in the early 1970s when there was also partial carp exclusion. Similar to water clarity, the increase in SAV cover was most significant in sheltered areas of the marsh. Our results suggest that excluding large carp can improve water clarity, SAV cover, and SAV species richness in large freshwater wetlands, benefiting waterfowl and other species.
Introduction
Water clarity and submersed aquatic vegetation (SAV) play important roles in aquatic ecosystems. High water clarity promotes SAV growth by allowing increased light penetration through the water column (Robel 1961; Hanson and Butler 1994). Areas with healthy SAV communities have greater fish species richness and diversity and serve as spawning, refuge, and feeding habitat (Randall et al., 1996; Weaver et al., 1997; Cvetkovic et al., 2010; Miller et al., 2018). Associated invertebrates are food sources for both fish and waterfowl (Bartonek and Hickey 1969; Keast 1984; DuBowy 1985; Hann 1995), and the vegetative parts are consumed by some waterfowl species (Collias and Collias 1963; DuBowy 1985). SAV in turn helps maintain a clear water state through several pathways: by reducing sediment and nutrient resuspension by wave action (Hamilton and Mitchell 1996; Horppila and Nurminen 2005), acting as refuge for zooplankton that consume phytoplankton (Scheffer 1999; Celewicz-Goldyn and Kuczynska-Kippen 2017), competing with phytoplankton for nutrients (van Donk et al., 1990), and potentially limiting algae growth through allelopathy (Körner and Nicklish 2002; Hilt and Gross 2008).
In many parts of the world, and particularly in North American shallow aquatic ecosystems, invasive common carp (Cyprinus carpio; hereafter, carp) negatively impact water clarity and SAV assemblages by resuspending bottom sediments and lowering light penetration (Badiou and Goldsborough 2010; Kaemingk et al., 2016), and through physical disturbance of SAV during feeding and spawning (Tryon 1954; Crivelli 1983). Carp absence-presence studies in shallow aquatic ecosystems have demonstrated a switch from a clear water, SAV dominated phase to a turbid, phytoplankton dominated phase (Lougheed et al., 1998; Zambrano and Hinojosa 1999; Bajer et al., 2009). Other studies have documented an opposite shift from a turbid to a clear water state after benthivorous fish (especially carp) have been removed or partially excluded, triggering increased growth, extent, and diversity of SAV species (Meijer et al., 1990; Ivey et al., 1998; Schrage and Downing 2004; Kaemingk et al., 2016). The first recorded appearance of carp in the wild in Manitoba, Canada was in the Red River at Lockport in 1938 (Hinks 1943). Carp are believed to have first appeared at Lake Manitoba and Delta Marsh in the late 1940s (Atton 1959; Swain 1979). By the early 1960s, large spring runs of carp were observed at the channels connecting Delta Marsh to Lake Manitoba.
Delta Marsh in western Canada (50.19875, -98.20478), is one of North America’s largest freshwater coastal wetlands covering 18,500 ha (Watchorn et al., 2012). A forested beach ridge separates Delta Marsh and Lake Manitoba. Four channels transect the ridge, connecting the marsh to the lake, and water flows between marsh and lake are dynamic and driven by the prevailing winds (Aminian 2015). Marsh water quality is influenced by lake water inputs and runoff from the surrounding watershed, with less mixing and dilution of marsh water during periods of low water levels and runoff (Bortoluzzi 2013). Water clarity is generally low, with an average turbidity range of 12–45 nephelometric turbidity units (NTU) and total suspended solids range from 10 to 100 mg/L (Hnatiuk 2006; Bortoluzzi 2013). The marsh water is brackish, with a conductivity range of 1,600–2,640 μS/cm (Bortoluzzi 2013). As with Lake Manitoba, Delta Marsh water is typically alkaline (Bortoluzzi 2013).
Historically, Delta Marsh had abundant and diverse emergent and SAV assemblages (Hinks and Fryer 1936) which helped support populations of breeding and migrating waterbirds. However, over the last 50+ years the marsh had become turbid and SAV cover decreased by > 50% (Goldsborough and Wrubleski 2001). Concerns over decreasing SAV and the effect on higher trophic levels prompted several SAV mapping surveys at the marsh, the first occurring in 1974 (Anderson and Jones 1976). Further decreases in SAV areal extent documented in surveys in 1997 and 2009–2010, decreasing water clarity, and a series of studies examining the impacts of carp on Delta Marsh using a combination of mesocosm and whole ecosystem manipulation experiments (Evelsizer and Turner 2006; Hnatiuk 2006; Badiou and Goldsborough 2010, 2015; Hertam 2010) led researchers to conclude carp were largely responsible for the deteriorating conditions observed at the marsh. Numerous other studies have found that carp are particularly detrimental to coastal wetlands and shallow lakes (Lougheed et al., 2004; Schrage and Downing 2004; Bajer et al., 2009). Additionally, other stressors such as the regulation of Lake Manitoba water levels beginning in 1961, the increasing enrichment of the marsh by nutrients from domestic sewage, agricultural manures, and fertilizers (through direct runoff and diversion of flood waters from the Assiniboine River through operation of the Portage Diversion), and expansion of hybrid cattail (Typha x glauca) have also contributed to the declining health of the marsh (Wrubleski et al., 2016). There may also be synergistic effects between carp and these stressors. For example, the presence of carp is believed to amplify eutrophication in aquatic ecosystems (Drenner et al., 1998) and biomanipulation has been proposed as an effective way to mitigate eutrophication in wetland systems (Angeler et al., 2003).
To address the adverse effects caused by carp we initiated a project to exclude large carp (>70 mm maximum body width) from Delta Marsh with the objective of improving waterfowl habitat. In winter 2012–2013, exclusion structures were built on channels connecting Lake Manitoba to Delta Marsh. Fish, including carp, overwinter in the lake and migrate into the marsh each spring (Lapointe 1986). The exclusion structures were fitted with removable screens with 70 mm spacing between vertical steel bars. Screens are positioned several weeks after the start of fish migration to allow early arriving native fish access to the marsh, but prior to the peak of carp migration. The screens are removed in mid-July. We monitored water clarity and SAV cover at Delta Marsh pre-(2009, 2010, 2012) and post-(2013–2018) carp exclusion. We hypothesized that water clarity, SAV cover and species richness would increase following the exclusion of large carp from the marsh.
Methods
Site description
Delta Marsh is divided into three main units by anthropogenic structures (Figure 1). The Portage Diversion isolates the west marsh from the other units. This 29 km long channel was completed in 1970 and is used to divert flood waters from the Assiniboine River north into Lake Manitoba. Five kilometers east of the diversion is Provincial Road 240 which divides the center and east marsh. The east marsh makes up the largest portion of Delta Marsh and from Provincial Road 240 it stretches approximately 19 km northeastward along the south shore of Lake Manitoba (Figure 1). For increased spatial resolution the east marsh was analyzed as two separate units (east 1 and east 2). The division between these two units occurs at a natural and nearly mid-way point of the east marsh where water movement is constricted through several small channels (Figure 1). Carp were excluded from all but the most northeasterly large bay of the marsh (Figure 1).
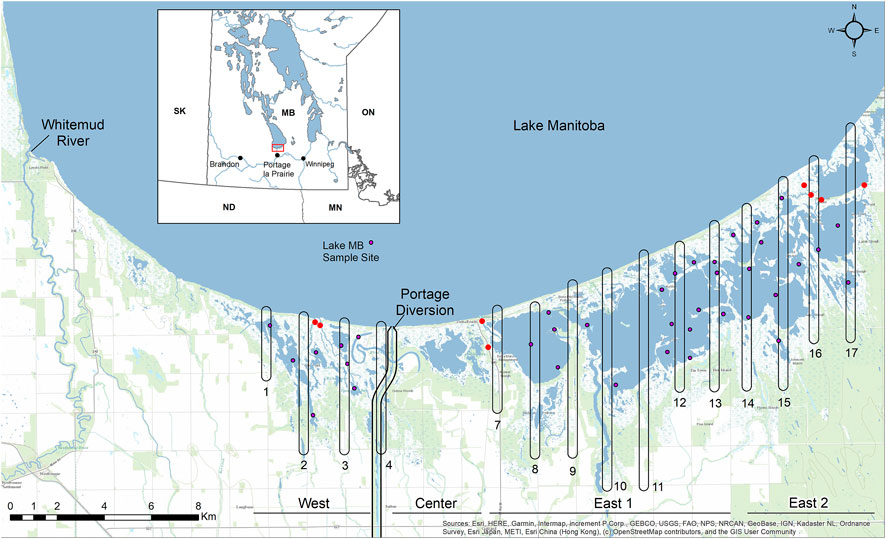
FIGURE 1. Location of water clarity sampling sites (purple dots) in the west, east 1, and east 2 units at Delta Marsh (37), and at Lake Manitoba (1). Red circles are locations of carp exclusion structures; numbered rounded rectangles are submersed aquatic vegetation mapping transects. The inset figure shows the location of Delta Marsh (red bounding box) within Manitoba.
Delta Marsh water clarity data
Water sampling occurred at 37 sites (Figure 1) during May to July 2009, 2010, and 2012–2018, the period corresponding to when carp exclusion screens would have been in place post-carp exclusion. Each site was sampled approximately every two to three weeks, with three to seven sample rounds completed each year. Sampling was avoided when wind speed exceeded 30 km/h. Measurements included water depth (cm) and surface water temperature (°C), and integrated water samples of the uppermost 1 m of the water column were collected using an acrylic tube inserted vertically into the water column. Samples were kept in a cooler until they could be processed.
Water samples were processed the same day at a field laboratory and analyzed for specific conductance (µS/cm; YSI 85 probe and meter [YSI Inc., Yellow Springs, OH]), total (TSS) and inorganic (ISS) suspended solids (mg/L), and total chlorophyll and chlorophyll-a (µg/L). Known volumes of the water samples were filtered through a vacuum pump and pre-weighed Whatman GF/C 42.5 mm filter paper then dried at 100°C in a drying oven for 24 h and weighed for TSS; filters were then placed in a furnace at 600°C for 1 h and weighed again for ISS. As a proxy for phytoplankton biomass, total chlorophyll (chlorophyll-a + phaeophytin; µg/L) pigment absorbance was measured after filtering a second portion of the water samples through the vacuum pump and a Whatman GF/C 42.5 mm filter. Each filter was then placed in a 7 ml glass vial and frozen for 24 h before 5 ml of 90% methanol was added, after which the vials were placed in a dark drawer for another 24 h; the liquid was pipetted from the vials to a cuvette and analyzed for chlorophyll-a in a spectrophotometer at 665nm and 750nm. The cuvettes were then acidified with one drop of 0.01N hydrochloric acid; after an hour the cuvettes were analyzed for phaeophytin in the spectrophotometer at wavelengths of 665nm and 750nm. Chlorophyll-a, phaeophytin, and total chlorophyll concentrations were calculated based on absorbance readings at wavelength values of 665 nm and 750 nm (Marker et al., 1980).
Lake Manitoba data
Lake Manitoba water chemistry data (conductivity [µS/cm], ammonia [NH3, mg/L], total nitrogen [TN, mg/L], and total phosphorous [TP, mg/L]) were obtained from Manitoba Environment, Climate and Parks. Water quality samples were collected at a sampling station (MB05LLS013; Manitoba Environment, Climate and Parks) located in the south basin of the lake, approximately 3.5 km north of Delta Marsh (Figure 1).
We summed daily volumes (m3/day) of water discharging from two main tributaries to the Lake Manitoba south basin: the Whitemud River and the Portage Diversion (Figure 1). Daily volumes were downloaded from the Water Survey of Canada (gauges 05LL002 and 05LL019, respectively). Daily volumes (January 1st–December 31st; 2009–2018) were summed to obtain total annual water volume discharge (m3).
Water clarity data analysis
Delta Marsh water clarity was compared pre- and post-carp exclusion, and between the west, east 1, and east 2 units in the marsh. We used ISS and total chlorophyll as separate water clarity response variables in two modelling efforts to separate the inorganic and algal contributors to suspended solids. Generalized linear mixed models (log link function and gamma distribution; SAS Institute, Inc., Cary, NC) were used to model ISS and total chlorophyll as a function of period (pre- or post-carp exclusion), unit (east 1, east 2, west; Figure 1), unit*period, and a number of covariates known to affect water clarity (Meijer et al., 1990; Chow-Fraser 1999, 2006; Beaulieu et al., 2013), including: year-varying annual Lake Manitoba total discharge loading (Portage Diversion + Whitemud River); year- and sample round-varying Lake Manitoba conductivity, total chlorophyll, NH3, TN, TP, and water level; site-varying relative exposure index (REI); site- and year-varying Julian date of sampling; Delta Marsh water depth, water temperature, total chlorophyll, chlorophyll-a, windspeed effects, resuspension potential, and conductivity.
Random effects of site, year, and site*year were also included to account for repeated sampling both within and across years. The covariate REI was calculated to characterize the exposure of each site to wind generated waves (Keddy 1982):
REI =
where i is ith compass heading (X = 1 to 36; every 10° starting at 0°); V is mean wind speed from the ith direction; P is proportion with which wind occurred from the ith direction; and F is effective fetch distance from the ith direction. We summarized mean wind speed and wind direction frequency from data collected every 15 min during May to July over a 5-year period, 2014–2018, at a weather station located at the SE corner of the marsh (50.19946, -98.08638). We used geospatial models (see Rohweder et al., 2012) to calculate effective fetch distances.
To model increases or decreases in ISS and in total chlorophyll that might lag behind rising or falling wind speeds we included lagged wind speed and resuspension potential covariates over nine-time intervals: at the time of sampling, and averaged over the preceding 1, 3, 6, 9, 12, 18, 24, and 36 hourly intervals. We used hourly wind speed and wind direction data from Environment and Climate Change Canada’s (ECCC) Portage Southport weather station (Station ID: 5012324; station located 30.1 km south of the center of Delta Marsh). When wind speed and wind direction values were missing, we substituted data from ECCC’s Portage Southport A or Delta Marsh CS stations. Several sources (Carper and Bachmann 1984; Booth et al., 2000; Bajkiewicz-Grabowska et al., 2016) have validated shallow water wave theory that when water depth is less than half the wavelength, the wave orbital motion beneath the water surface interacts with the bottom sediments, at which point sediment resuspension is likely. Resuspension potential is described as:
Modeling subsets were run to identify the optimum time interval at which to model lagged wind speed and resuspension potential. REI was also considered as a candidate to represent windspeed effects. Fixed effects of unit, period, unit*period, REI, and random effects of site, year, and site*year were included in all of these competing models and the effects of windspeed and resuspension potential were added one-at-a-time. Best approximating models (identified as minimizing AIC) were used for identifying a best time interval scale for representing windspeed and resuspension potential.
Since resuspension potential incorporates wind speed and water depth, two full model simplification paths were explored including either the best time interval effects of (i) wind speed and water depth or (ii) resuspension potential, with all the other variables described previously. To facilitate model convergence independent variables were scaled by orders of magnitude. REI was retained in all model runs as it characterizes the wind exposure of each water sampling site, which influences water clarity at varying degrees spatially across Delta Marsh. The effects of unit, period, and unit*period were retained as these were the effects of primary interest. Full models were sequentially simplified by removing the effects with the smallest Wald-statistics (Wood et al., 2008) and all models were ranked by AIC to identify the best approximating and competing models (identified as those within 2 ΔAIC units and structurally simpler than the top model [Arnold 2010]). Ratios of estimated marginal means (EMMs) were used to determine if there were overall or unit specific differences in ISS and total chlorophyll between pre- and post-carp exclusion.
Submersed aquatic vegetation data
SAV beds at Delta Marsh were mapped between July and September 1974, 1997, 2009–2010 (this survey spanned two field seasons), 2014, and 2016–2018. Watercraft were used in all years to ground-truth vegetation bed perimeters. The extent of SAV beds were mapped, and SAV species were recorded and assigned to either single or mixed species assemblages. Survey methods used in 1974 (Anderson and Jones 1976) and 1997 (D. Wrubleski and M. Anderson, Ducks Unlimited Canada, unpublished report) combined the use of aerial photographs and ground truthing. Due to turbid conditions a garden rake was dragged along the marsh bottom to delineate vegetation bed perimeters in 1997 and 2009–2010 (M. Baschuk and D. Wrubleski, unpublished report). Aerial photographs were not used in the 2009–2010 and 2014 SAV surveys due to turbid conditions which limited vegetation bed interpretation. Vegetation beds were drawn on paper maps in the field. A handheld data collector (Trimble Juno; Trimble Navigation Ltd., Sunnyvale, CA) was also used in 2014. All paper maps were later digitized using ArcGIS (ESRI, Inc., Redland, CA). In 2016–2018 polygons and points representing SAV beds were recorded using a tablet running ESRI’s Collector for ArcGIS software connected to a GPS receiver. Points were created for small SAV beds measuring approximately 4 m2. During data processing, point locations were buffered 1 m on all sides resulting in polygons 4 m2. SAV beds smaller than 4 m2 were not mapped.
In 1974, 1997, 2009–2010, and 2014 SAV distribution was mapped across the entire east marsh; in 2010 the west marsh was also included. In 2016–2018 only areas falling under 400 m wide survey transects (hereafter, SAV mapping transects; Figure 1) were mapped in both the east and west marsh. In order to standardize the comparison of SAV among years, we only included survey data within SAV mapping transects 1–4 and 7–17 within the carp exclusion zone (Figure 1).
Submersed aquatic vegetation data analysis
We assigned each SAV record to one of ten treatments based on marsh unit and SAV survey year(s): 1) west, late pre-carp exclusion (2010), 2) west, late post-carp exclusion (2016–2018), 3) east 1, early pre-carp exclusion (1974), 4) east 1, late pre-carp exclusion (1997 and 2009–2010), 5) east 1, early post-carp exclusion (2014), 6) east 1, late post-carp exclusion (2016–2018), 7) east 2, early pre-carp exclusion (1974), 8) east 2, late pre-carp exclusion (1997 and 2009–2010), 9) east 2, early post-carp exclusion (2014), and 10) east 2, late post-carp exclusion (2016–2018). We specify pre- and post-carp exclusion here in the context of the current carp exclusion project, as in the 1970s there was also carp exclusion. For each treatment we calculated the estimated percent areal cover of total SAV and of the three most common SAV species found at Delta Marsh (Ceratophyllum demersum [coontail], Myriophyllum sibiricum [northern watermilfoil], and Stuckenia pectinata [sago pondweed]) as the proportion of SAV areal extent to open water area. As mixed species bed assemblage classifications were not consistent among years we summed, by species, the areal extent of vegetation beds with any occurrence of that species to facilitate within-species comparisons. The data were treated as pseudo-binomial, and a generalized linear model (SAS PROC GLIMMIX; using a logit link function, binomial distribution, and allowing for overdispersion) was used to obtain log-odds estimates of SAV cover as a function of treatment. Odds ratio contrasts of estimated marginal means (EMMs) were used to identify whether the differences in total and species-specific SAV percent areal coverage were significant among treatments.
Results
Water clarity
The effects of average wind speed and resuspension potential over the preceding 18 h advanced to the ISS full model reduction sets. The best approximating reduced model for ISS included effects of unit, period, unit*period, Lake Manitoba (LKMB) NH3, Delta Marsh (DM) chlorophyll-a, total chlorophyll, REI, and average wind speed over the preceding 18 h. A competing model (ΔAIC = 1.74) included all effects in the best approximating model except DM chlorophyll-a. ISS was positively correlated with the effects (β, ± SE), in descending order of strength, of average windspeed over the preceding 18 h (0.301, ± 0.025), REI (0.128, ± 0.027), LKMB NH3 (12.4, ± 3.40), DM total chlorophyll (0.063, ± 0.032), and DM chlorophyll-a (0.121, ± 0.063; Figure 2).
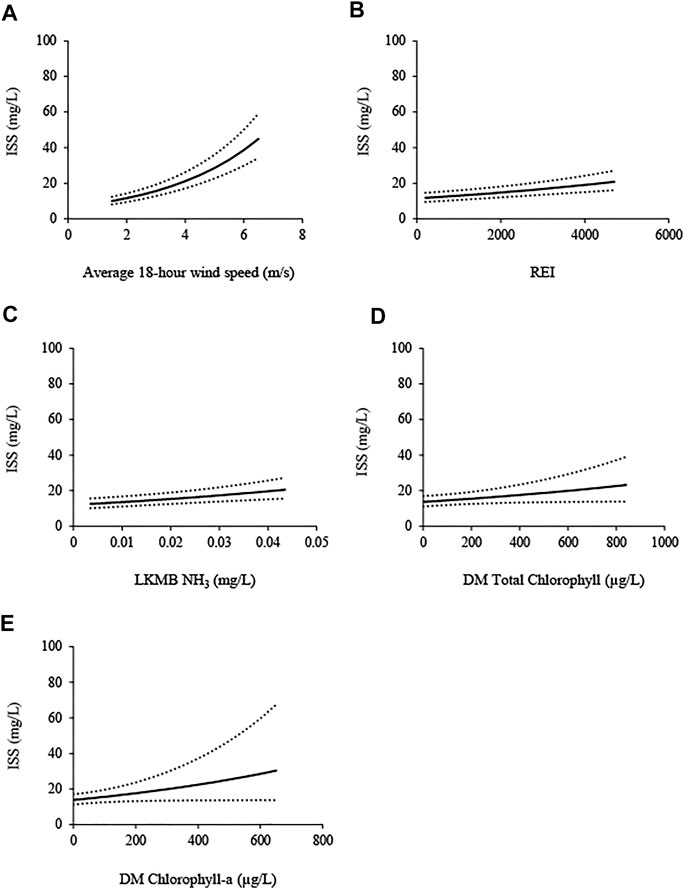
FIGURE 2. Inorganic suspended solids (ISS; mg/L) in the Delta Marsh (DM) carp exclusion zone (west, east 1, and east 2 units), 2009, 2010, and 2012–2018, modeled in relation to: (A) average wind speed over the preceding 18 hours (average 18-h wind speed; m/s), (B) relative exposure index (REI), (C) Lake Manitoba (LKMB) ammonia (NH3; mg/L), (D) DM total chlorophyll (μg/L), and (E) DM chlorophyll-a (μg/L). 95% confidence intervals (±; dotted lines) are shown. Each covariate effect was isolated by setting all other covariates to their mean values, averaging over unit*period effects and setting random effects of site, year, and site*year to zero.
The effects of average windspeed in the preceding 36 h and resuspension potential over the preceding 24 h advanced to the full model reduction sets for total chlorophyll. The best approximating model included effects of unit, period, unit*period, DM water temperature, Julian date, ISS, REI, and conductivity, and LKMB conductivity, NH3, TN, TP, and total discharge. There were no competing models. As REI was imprecisely estimated (i.e., the ratio of the estimated effect to standard error was < ∼2) we did not plot or interpret this effect. Effects (β, ± SE) that were positively correlated with total chlorophyll, in descending order of strength, were: DM water temperature (0. 743, ± 0. 075), Julian date (1.029, ± 0.107), and ISS (0.922, ± 0.133), LKMB conductivity (0.203, ± 0.033), NH3 (14.6, ± 3.54), and TN (0.289, ± 0.079). Effects that were negatively correlated with total chlorophyll, in descending order of strength, were: DM conductivity (-0.091, ± 0.022), LKMB total discharge (-0.023, ± 0.008) and TP (-2.51, ± 0.911; Figure 3).
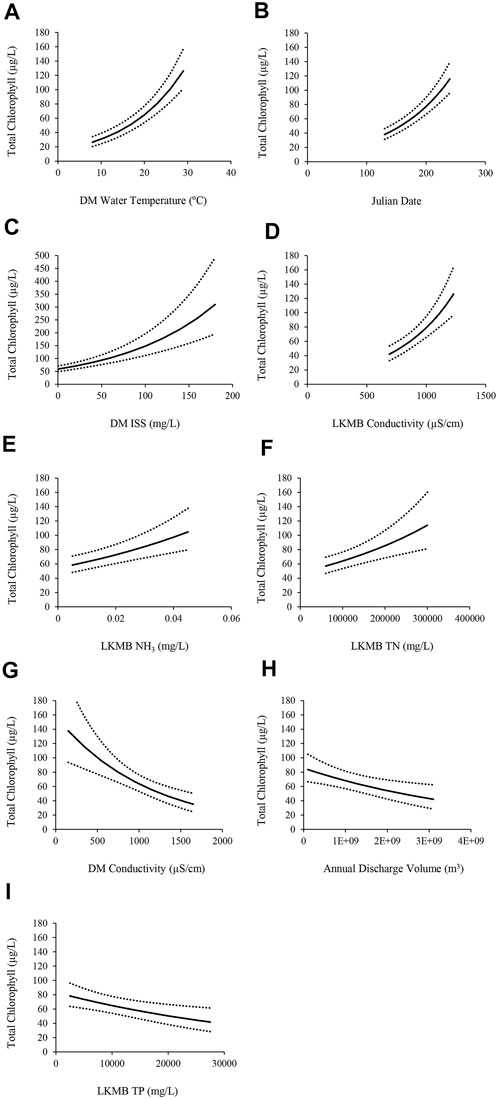
FIGURE 3. Total chlorophyll (μg/L) in the Delta Marsh (DM) carp exclusion zone (west, east 1, and east 2 units), 2009, 2010, and 2012–2018, modeled in relation to: (A) DM water temperature (°C), (B) Julian date (days), (C) DM inorganic suspended solids (ISS; mg/L), (D) Lake Manitoba (LKMB) conductivity (μS/cm), (E) LKMB ammonia (NH3; mg/L), (F) LKMB total nitrogen (TN; mg/L), (G) DM conductivity (μS/cm), (H) Total annual discharge volume (m3) into LKMB, and (I) LKMB total phosphorus (TP; mg/L). 95% confidence intervals (± dotted lines) are shown. Each covariate effect was isolated by setting all other covariates to their mean values, averaging over unit*period effects and setting random effects of site, year, and site*year to zero.
Estimated means ratios indicate there was an overall marginal decline (-32%) in ISS pre-to post-carp exclusion (Table 1). The magnitude of decline in ISS was spatially dependent, with a significantly larger decrease in the west marsh unit (-59%) relative to inconclusive changes in the east 1 (-10%) and east 2 units (-14%; Table 1 and Figure 4). Tests of statistical significance of changes in total chlorophyll were inconclusive, although there was evidence of an overall decline (-22%) pre-to post-carp exclusion, but no evidence of a difference between marsh units (Table 1; Figure 4).
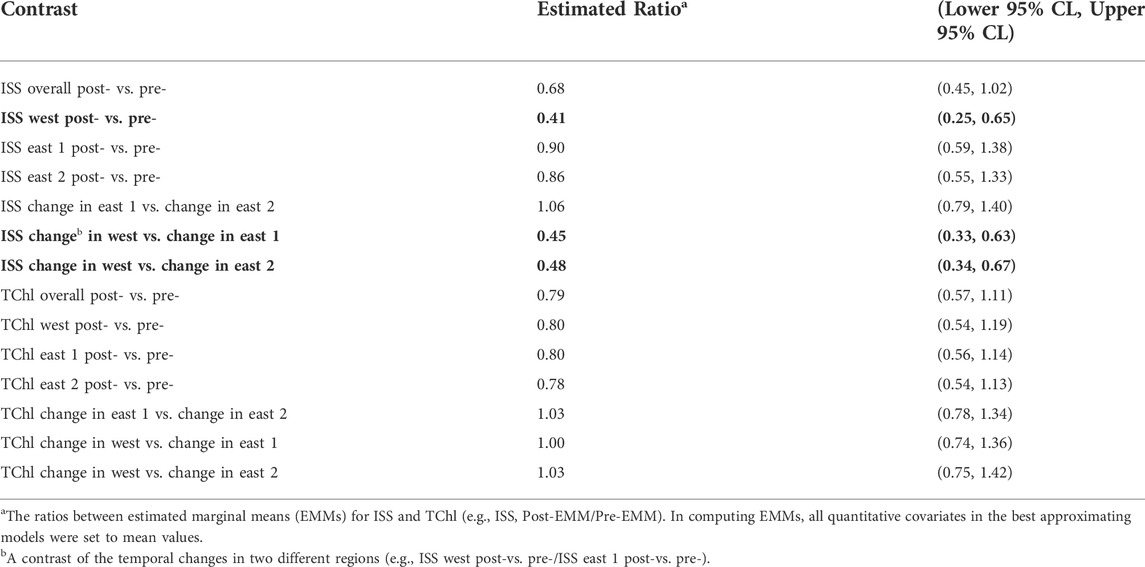
TABLE 1. Tests of contrasts in inorganic suspended solids (ISS) and total chlorophyll (TChl) within the carp exclusion zone during May to July by treatment period [pre- (2009, 2010, and 2012) and post- (2013–2018) carp exclusion] and marsh unit (west, east 1, east 2), Delta Marsh, Manitoba. Contrasts are statistically significant (bold) when 95% confidence intervals exclude 1.00 (CL = confidence limit).
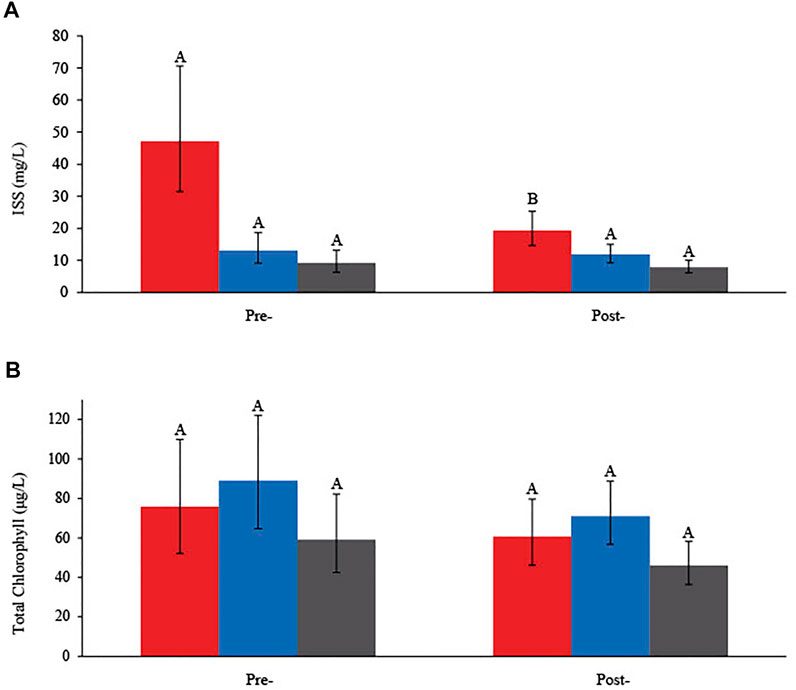
FIGURE 4. (A) Estimated marginal means (EMMs) of (A) inorganic suspended solids (ISS; mg/L) and (B) total chlorophyll (μg/L), with 95% confidence intervals, in the west (red), east 1 (blue), and east 2 (grey) units pre- (2009, 2010, and 2012) and post- (2013–2018) carp exclusion, Delta Marsh, Manitoba. Different letters (A and B) indicate a statistical difference between periods (pre- or post-carp exclusion) within the same marsh unit.
Submersed aquatic vegetation
Overall in the east marsh carp exclusion zone the areal extent of SAV declined between mapping surveys conducted in 1974 and 1997, and increased with each survey between 2014 and 2018 (Figure 5). In the west marsh, SAV areal extent increased since the pre-carp exclusion survey in 2010 (Figure 5).
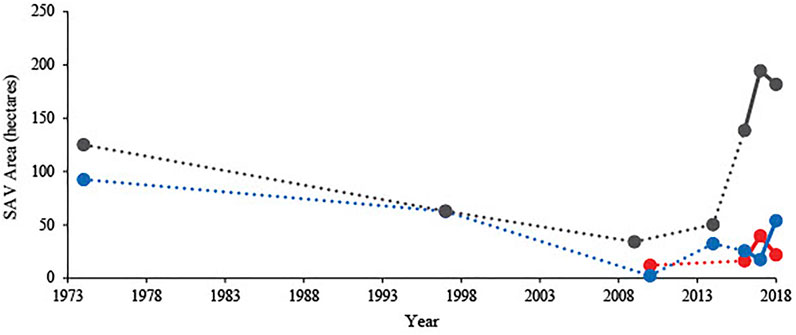
FIGURE 5. Total areal extent (ha) of submersed aquatic vegetation (SAV) in SAV mapping transects 1–4 in the west (red), 7–13 in the east 1 (blue), and 14–17 in the east 2 (grey) carp-exclusion zones, 1974, 1997, 2009, 2010, 2014, and 2016–2018, Delta Marsh, Manitoba. Dotted lines connect non-consecutive survey years; solid lines connect consecutive survey years.
There were no significant differences in total SAV percent cover (hereafter, SAV cover) between treatment periods in the west and east 1 marsh units (Table 2; Figure 6A). Total SAV cover in the late post-treatment period was significantly greater in the west and east 2 units versus the east 1 unit, and in the east 2 unit declined significantly by one third between the early pre- and late pre-treatment periods. SAV cover in the east 2 marsh unit significantly tripled between the late pre- and late post-treatment periods, and significantly increased between early post- and late post-treatment periods (Table 2; Figure 6A).
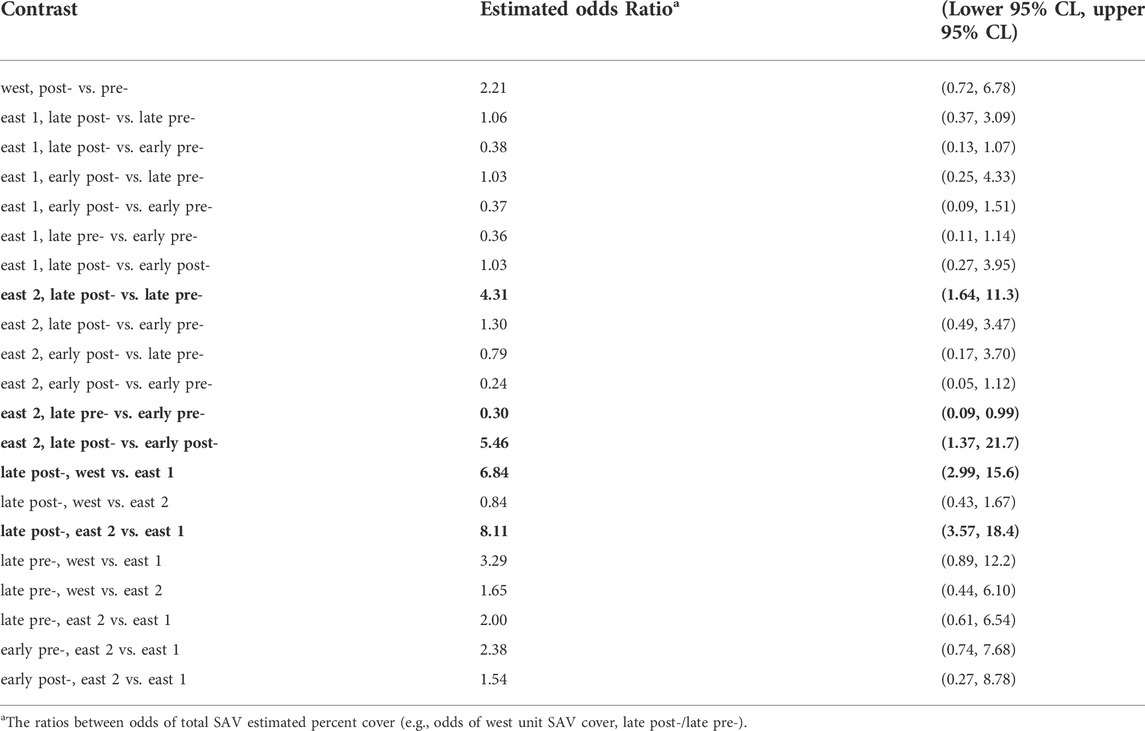
TABLE 2. Tests of contrasts in total submersed aquatic vegetation (SAV) estimated percent cover between treatment period: early pre-carp exclusion [1974]; late pre-carp exclusion [1997 and 2009–2010]; early post-carp exclusion [2014]; late post-carp exclusion [2016–2018], and marsh unit (west, east 1, east 2), Delta Marsh, Manitoba. Contrasts are statistically significant (bold) when 95% confidence intervals exclude 1.00 (CL = confidence limit).
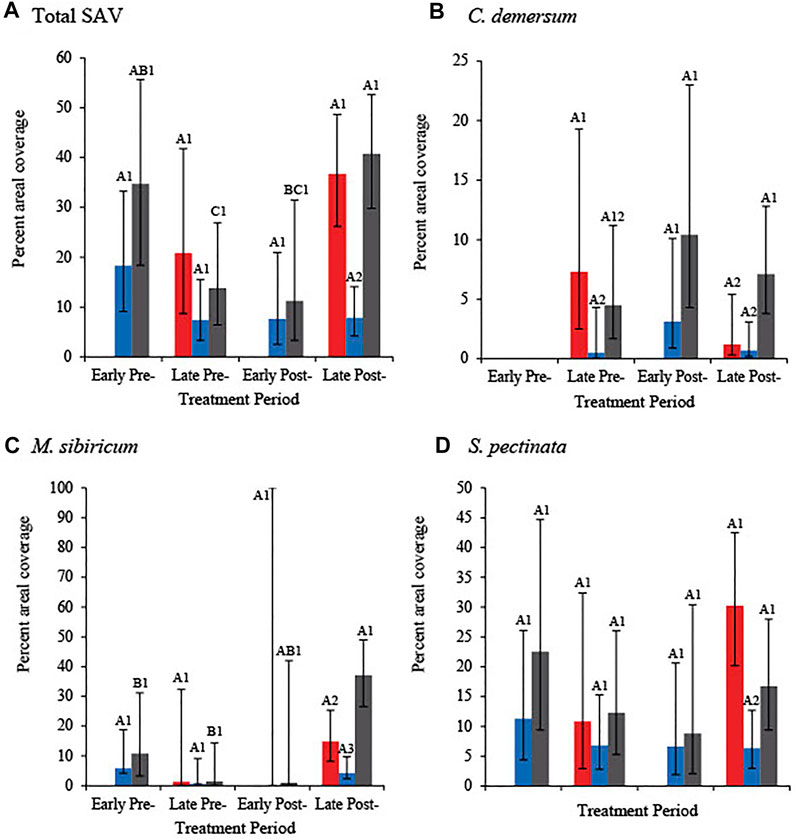
FIGURE 6. Percent cover and 95% confidence intervals of (A) total submersed aquatic vegetation (SAV) and the three most common SAV species [(B) Ceratophyllum demersum, (C) Myriophyllum sibiricum, and (D) Stuckenia pectinata] in SAV mapping transects and 1–4 in the west (red), 7–13 in the east 1 (blue), and 14–17 in the east 2 (grey) carp exclusion units, Delta Marsh, Manitoba. Treatment periods are defined as: early pre-carp exclusion (1974), late pre-carp exclusion (1997, 2009–2010), early post-carp exclusion (2014), and late post-carp exclusion (2016–2018). Percent cover included homogenous vegetation beds and mixed species bed assemblages where each species occurred. Different letters (A–C) indicate a statistical difference between treatment periods within the same species and unit. Different numbers (1–3) indicate a statistical difference between units within each SAV group (total SAV or species) and treatment period.
The same contrasts of odds ratios for total SAV cover (see Table 2) were assessed for each of the three dominant SAV species. There were no significant changes in C. demersum cover between treatment periods; however, there were significant cover differences between marsh units in the late pre-treatment period; cover was nearly 15 times greater in the west versus the east 1 unit (Figure 6B). In the late post-treatment period, C. demersum cover was greatest in the east 2 versus the west and east 1 units (Figure 6B). In the late pre-treatment period M. sibiricum cover was low in all marsh units (Figure 6C). M. sibiricum cover increased in all units after carp were excluded. In the late post-treatment period, M. sibiricum cover was greatest in the east 2 unit, followed in descending order by the west and east 1 units (Figure 6C). S. pectinata was the dominant SAV species, making up the majority of the total SAV cover in almost all treatment periods and marsh units (Figure 6D). While S. pectinata cover in the east 2 unit declined between the early pre- and early post-treatment periods, before increasing in the late post-treatment period, the changes were not statistically significant (Figure 6D). The increase in S. pectinata cover in the west unit was also not statistically significant. S. pectinata cover in east 1 remained consistently lower after the early pre-treatment period. In the late post-treatment period, S. pectinata cover was significantly greater in the west and east 2 units as compared to the east 1 unit (Figure 6D). Other species-specific contrasts were not statistically significant, possibly due in some cases to extremely low cover values, high relative variability in cover among SAV mapping transects and treatment periods, or possibly as a result of how we summed mixed species bed areal extents.
While the increase in SAV percent cover was significant in the west unit post-carp exclusion, we observed a decrease in S. pectinata areal extent in the west unit in 2018 (Figure 5). In late July and early August 2018, extensive beds of SAV, primarily composed of S. pectinata, were observed in the west unit (P. Kowal, personal observation). However, many vegetation beds had disappeared when the SAV was mapped 15–17 August.
We recorded changes in the dominance and number of SAV species within the marsh between treatment periods. During the pre- and early post-treatment periods the dominant SAV species in the east marsh was S. pectinata; M. sibiricum was the dominant species in the east marsh in the late post-treatment period (Figure 6). In the west marsh, S. pectinata was the dominant SAV species across pre- and post-treatment periods (Figure 6). Six SAV species were identified at Delta Marsh in 2014, four in 2016, and eight in 2017 and 2018. Records of sparse occurrences of some species in 2016 were lost, and therefore our estimates of species richness are conservative for that year. Potamogeton pusillus (small pondweed) and Sparganium eurycarpum (giant bur-reed) were detected in 2017 and 2018 after last being observed in 1997. In 2017, Chara, a genus of charophyte green algae characteristic of clear water habitats, was observed for the first time in recent years, in the east 2 unit.
Discussion
Biomanipulation, through removal or exclusion, has been widely used to successfully reduce carp biomass in small lakes (Sorensen Bajer, 2020) and shown to result in significant water quality improvements such as reduced turbidity, decreases in chlorophyll-a, and increased SAV (Schrage and Downing 2004; Kaemingk et al., 2016; Jůza 2019; Huser et al., 2022). However, most attempts to regulate invasive carp populations have been largely applied to smaller (<250 ha), isolated water bodies with limited hydrological connections (Lougheed et al., 2004). For the most part, carp biomanipulation has been achieved through physical removal via harvest fishing (Bajer et al., 2011) and specialized traps (Stuart et al., 2011), water level drawdowns (Verrill and Berry 1995), chemical/biological removal mechanisms such as rotenone (Schrage and Downing 2004; Bonneau and Scarnecchia 2015), use of the koi herpes virus (McColl et al., 2007), and daughterless carp genetic manipulation (Brown and Gilligan 2014). However, physical removal is often only successful in the short term with carp populations usually rebounding quickly (Tempero et al., 2019) and chemical/biological removal is not feasible and is challenging within existing regulatory frameworks on large interconnected systems with important commercial and indigenous fisheries such as the Delta Marsh-Lake Manitoba system. Our research demonstrates that limiting access of large carp using exclusion structures, specifically during the spawning season, can improve water clarity and SAV cover in a large freshwater coastal wetland.
Post-carp exclusion we measured increases in water clarity, and SAV cover and species richness. We saw spatially variable increases in SAV cover post-carp exclusion which were accompanied by spatially variable decreases in ISS and to a lesser extent total chlorophyll, similar to results of other carp removal studies (Hanson and Butler 1994; Schrage and Downing 2004). Increased water clarity and SAV cover can arise through several synergistic mechanisms. Increased water clarity allows deeper light penetration which enhances SAV growth (Robel 1961; Lougheed et al., 1998). Increased SAV cover results in less wind and wave action; thus lowering sediment disturbance and resuspension, further increasing water clarity and SAV growth (Bachmann et al., 2000; Cho 2007; Van Zuidam and Peeters 2015). Hanson and Butler (1994) found that an initial increase in water clarity after fish removal was caused by increased zooplankton grazing during the growing season which allowed for the establishment of SAV; subsequent water clarity improvements were maintained by SAV lowering sediment resuspension and algal biomass. The changes in SAV cover and ISS following carp exclusion at Delta Marsh were significantly greater in the west unit compared to the east 1 and 2 units. The east 1 unit has larger, more exposed bays than the east 2 unit, and both units are more open and exposed than the west unit and this may explain why the effects of carp exclusion on ISS were muted in the east units relative to the west unit. This is supported by average 18 h wind speeds and REI being the two strongest environmental predictors of ISS at Delta Marsh. The simultaneous existence of both turbid and clear water states within one shallow aquatic system is possible depending on the degree of exposure and presence of SAV (Scheffer et al., 1994), especially in a system as large and complex as Delta Marsh. The exclusion of carp appears to have resulted in less resuspension of sediment early in the growing season, allowing SAV cover to expand compared to years when carp had free access to the marsh. The exclusion of carp also likely reduced physical disturbance of SAV. Furthermore, benthivorous fish like carp are also known to reduce the erosion resistance of sediment which enhances sediment resuspension by wave action (Scheffer et al., 2003), lowering the capacity for SAV establishment. The changes in water clarity and SAV we observed suggest that the exclusion of carp coupled with the wind sheltered hydrogeomorphic nature of the west unit allowed for a rapid recovery in both water clarity and SAV there.
Historically, sago pondweed was a dominant species in Delta Marsh, and was very important as a food source to waterfowl (Anderson and Low 1976; Gordon 1985). Thus, there is significant interest in its response to carp exclusion. Anderson (1978) found that the distribution of S. pectinata at the marsh was highly correlated to site exposure and our observations regarding site exposure support this. The large exposed bays of the east 1 unit have seen greater shoreline and island erosion compared to the other marsh units (Wrubleski et al., 2016), and since the early 1970s. S. pectinata has remained consistently low in that unit. While the increase in SAV percent cover, primarily consisting of S. pectinata, was significantly greater in the west unit post-carp exclusion we observed a decrease in SAV areal extent in 2018 there that we speculate was due to early senescence caused by extremely low water levels, wave action, and grazing by waterfowl.
The exclusion of large carp from Delta Marsh resulted in increased water clarity and SAV cover and species richness despite carp exclusion coinciding with some of the highest water levels recorded on this system since the 1960s as a result of major flooding events in 2011 (Blais et al., 2016) and 2014 (Ahmari et al., 2016). Because increased water levels often decrease SAV abundance (Robel 1961; Scheffer et al., 1992) we expect that the exclusion of carp from Delta Marsh will result in greater water clarity and SAV cover under more normal water levels. Furthermore, these improvements have occurred during a period when Lake Manitoba, and Delta Marsh itself have experienced increased nutrient loading as a result of frequent operation of the Portage Diversion, which is the single largest input of nutrients to Lake Manitoba when it is in operation (Page 2011). Warm water temperatures and high nutrient availability in the water column promote blue-green algae growth which restricts water transparency (Kosten et al., 2012; Beaulieu et al., 2013). Nutrient loading can also mimic the detrimental impact of carp presence by promoting phytoplankton growth, possibly overriding the effects of carp on water quality in nutrient rich water (Badiou and Goldsborough 2015). Because benthivorous fish, like carp are known to interact synergistically with nutrient loading to increase phytoplankton biomass (Drenner et al., 1998), it is possible that our carp exclusion project is also helping to mitigate the impacts of high nutrient loading into Delta Marsh.
While the exclusion of large carp resulted in increased water clarity and SAV cover (this study) and increased use by diving ducks (Bortolotti et al., 2022—submitted), maintaining these conditions in light of other stressors being experienced at Delta Marsh such as increasing nutrient loading, stabilized water levels, and invasive hybrid cattail will necessitate ongoing monitoring and assessment of marsh conditions. Of these stressors, focusing on managing nutrient inputs from the agriculturally dominated portions of the Delta Marsh watershed should be investigated as a supplementary mechanism for sustaining and potentially improving conditions that favor water clarity and increased SAV cover over time.
Data availability statement
The raw data supporting the conclusions of this article will be made available by the authors, without undue reservation.
Author contributions
PK, PB, RE conceived and designed the analysis. PK, RE, LG collected the data. PK, RE, BP, DW, and LG contributed data or analysis tools. PK, RE, and BP organized the database. LA performed statistical analyses. PK wrote the first draft of the manuscript. PB wrote sections of the manuscript. All authors contributed to manuscript revision, read, and approved the submitted version.
Funding
This project was funded by Ducks Unlimited Canada, the United States Fish and Wildlife Service through the North American Wetlands Conservation Act, Manitoba Department of Natural Resources and Northern Development, Wildlife Habitat Canada, as well as grants and funds from numerous individuals and foundations. Research was conducted under Manitoba Fisheries Branch Scientific Collection permits, and Manitoba Wildlife Management Area Use permits. Delta Marsh is located within the traditional territory of the Anishinaabe and Dakota Peoples, and on the homeland of the Métis Nation. In the spirit of reconciliation, we respectfully acknowledge these Nations as the original caretakers of this land.
Acknowledgments
S. Witherly provided GIS support for submersed aquatic vegetation mapping and analyses. We thank the numerous research technicians and volunteers involved in the monitoring program for their hard work. Much of the data used in this paper was generated by the “Restoring the Tradition at Delta Marsh” project.
Conflict of interest
The authors declare that the research was conducted in the absence of any commercial or financial relationships that could be construed as a potential conflict of interest.
Publisher’s note
All claims expressed in this article are solely those of the authors and do not necessarily represent those of their affiliated organizations, or those of the publisher, the editors and the reviewers. Any product that may be evaluated in this article, or claim that may be made by its manufacturer, is not guaranteed or endorsed by the publisher.
References
Ahmari, H., Blais, E.-L., and Greshuk, J. (2016). The 2014 flood event in the Assiniboine River Basin: Causes, assessment and damage. Can. Water Resour. J./Revue Can. des ressources hydriques 41, 85–93. doi:10.1080/07011784.2015.1070695
Aminian, P. (2015). Manitoba: University of Manitoba. [master’s thesis]. [Winnipeg, (MB)] http://hdl.handle.net/1993/31013.Hydrodynamic modelling of Delta Marsh and simplified methods of discharge estimation for discontinuous inland coastal wetlands.
Anderson, M. G. (1978). Distribution and production of sago pondweed (Potamogeton Pectinatus L.) on a northern prairie marsh. Ecology 59, 154–160. doi:10.2307/1936642
Anderson, M. G., and Jones, R. E. (1976). Submerged aquatic vascular plants of east Delta Marsh. Winnipeg, MB, Canada: Manitoba Department of Renewable Resources and Transportation Services.
Anderson, M. G., and Low, J. B. (1976). Use of sago pondweed by waterfowl on the Delta marsh, Manitoba. J. Wildl. Manage. 40, 233–242. doi:10.2307/3800420
Angeler, D. G., Chow‐Fraser, P., Hanson, M. A., Sánchez‐Carrillo, S., and Zimmer, K. D. (2003). Biomanipulation: A useful tool for freshwater wetland mitigation? Freshw. Biol. 48 (12), 2203–2213. doi:10.1046/j.1365-2427.2003.01156.x
Arnold, T. W. (2010). Uninformative parameters and model selection using Akaike’s Information Criterion. J. Wildl. Manage 74, 1175–1178. doi:10.1111/j.1937-2817.2010.tb01236.x
Atton, F. M. (1959). The invasion of Manitoba and Saskatchewan by carp. Trans. Am. Fish. Soc. 88, 203–205. doi:10.1577/1548-8659(1959)88
Bachmann, R. W., Hoyer, M. V., and Canfield, D. E. (2000). The potential for wave disturbance in shallow Florida lakes. Lake Reserv. Manag. 16, 281–291. doi:10.1080/07438140009354236
Badiou, P. H. J., and Goldsborough, L. G. (2010). Ecological impacts of an exotic benthivorous fish in large experimental wetlands, Delta Marsh, Canada. Wetlands 30, 657–667. doi:10.1007/s13157-010-0071-5
Badiou, P. H. J., and Goldsborough, L. G. (2015). Ecological impacts of an exotic benthivorous fish, the common carp (Cyprinus carpio L.), on water quality, sedimentation, and submerged macrophyte biomass in wetland mesocosms. Hydrobiologia 755, 107–121. doi:10.1007/s10750-015-2220-6
Bajer, P. G., Chizinski, C. J., and Sorensen, P. W. (2011). Using the Judas technique to locate and remove wintertime aggregations of invasive common carp. Fish. Manag. Ecol. 18, 497–505. doi:10.1111/j.1365-2400.2011.00805.x
Bajer, P. G., Sullivan, G., and Sorensen, P. W. (2009). Effects of a rapidly increasing population of common carp on vegetative cover and waterfowl in a recently restored Midwestern shallow lake. Hydrobiologia 632, 235–245. doi:10.1007/s10750-009-9844-3
Bajkiewicz-Grabowska, E., Markowski, M., and Lemańczyk, K. (2016). Application of geoinformation techniques to determine zones of sediment resuspension induced by wind waves in lakes (using two lakes from Northern Poland as examples). Limnol. Rev. 16, 3–14. doi:10.2478/limre-2016-0001
Bartonek, J. C., and Hickey, J. J. (1969). Food habits of canvasbacks, redheads, and lesser scaup in Manitoba. Condor 71, 280–290. doi:10.2307/1366304
Beaulieu, M., Pick, F., and Gregory-Eaves, I. (2013). Nutrients and water temperature are significant predictors of cyanobacterial biomass in a 1147 lakes data set. Limnol. Oceanogr. 58, 1736–1746. doi:10.4319/lo.2013.58.5.1736
Blais, E.-L., Greshuk, J., and Stadnyk, T. (2016). The 2011 flood event in the Assiniboine River basin: Causes, assessment and damages. Can. Water Resour. J./Revue Can. des ressources hydriques 41, 74–84. doi:10.1080/07011784.2015.1046139
Bonneau, J. L., and Scarnecchia, D. L. (2015). Response of benthic macroinvertebrates to carp (Cyprinus carpio) biomanipulation in three tributaries of a eutrophic, great plains reservoir, USA. Trans. Kans. Acad. Sci. 118, 13–26. doi:10.1660/062.118.0103
Booth, J. G., Miller, R. L., McKee, B. A., and Leathers, R. A. (2000). Wind-induced bottom sediment resuspension in a microtidal coastal environment. Cont. Shelf Res. 20, 785–806. doi:10.1016/s0278-4343(00)00002-9
Bortoluzzi, T. (2013). Manitoba: University of Manitoba. [dissertation]. [Winnipeg (MB)] http://hdl.handle.net/1993/19686.Spatial and temporal patterns in the hydrology, water chemistry and algal nutrient status of Delta Marsh, as influenced by the hydrology of adjoining Lake Manitoba.
Brown, P., and Gilligan, D. (2014). Optimizing an integrated pest-management strategy for a spatially structured population of common carp (Cyprinus carpio) using meta-population modelling. Mar. Freshw. Res. 65, 538–550. doi:10.1071/MF13117
Carper, G. L., and Bachmann, R. W. (1984). Wind resuspension of sediments in a prairie lake. Can. J. Fish. Aquat. Sci. 41, 1763–1767. doi:10.1139/f84-217
Celewicz-Goldyn, S., and Kuczynska-Kippen, N. (2017). Ecological value of macrophyte cover in creating habitat for microalgae (diatoms) and zooplankton (rotifers and crustaceans) in small field and forest water bodies. PLoS ONE 12 (5), e0177317. doi:10.1371/journal.pone.0177317
Cho, H. J. (2007). Effects of prevailing winds on turbidity of a shallow estuary. Int. J. Environ. Res. Public Health 4, 185–192. doi:10.3390/ijerph2007040014
Chow-Fraser, P. (2006) Development of the wetland water quality index for assessing the quality of Great Lakes coastal wetlands. in T. Simon, and T. P. Stewart, editors. Coastal wetlands of the laurentian great lakes: Health, habitat and indicators. Authorhouse Press, Bloomington, IN, USA. http://greatlakeswetlands.ca/publications/development-of-the-water-quality-index-wqi-to-assess-effects-of-basin-wide-land-use-alteration-on-coastal-marshes-of-the-laurentian-great-lakes/(Accessed 2 January 2020).
Chow-Fraser, P. (1999). Seasonal, interannual, and spatial variability in the concentrations of total suspended solids in a degraded coastal wetland of Lake Ontario. J. Gt. Lakes. Res. 25, 799–813. doi:10.1016/S0380-1330(99)70778-1
Collias, N. E., and Colias, E. C. (1963). Selective feeding by wild ducklings of different species. Wilson Bull. 75, 6–14. doi:10.1016/0003-3472%2858%2990066-6
Crivelli, A. J. (1983). The destruction of aquatic vegetation by carp. Hydrobiologia 106, 37–41. doi:10.1007/bf00016414
Cvetkovic, M., Wei, A., and Chow-Fraser, P. (2010). Relative importance of macrophyte community versus water quality variables for predicting fish assemblages in coastal wetlands of the Laurentian Great Lakes. J. Gt. Lakes. Res. 36, 64–73. doi:10.1016/j.jglr.2009.10.003
Drenner, R. W., Gallo, K. L., Baca, R. M., and Smith, J. D. (1998). Synergistic effects of nutrient loading and omnivorous fish on phytoplankton biomass. Can. J. Fish. Aquat. Sci. 55, 2087–2096. doi:10.1139/f98-095
DuBowy, P. J. (1985). Feeding ecology and behavior of postbreeding male blue-winged teal and northern shovelers. Can. J. Zool. 63, 1292–1297. doi:10.1139/z85-194
Evelsizer, V. D., and Turner, A. M. (2006). Species-specific responses of aquatic macrophytes to fish exclusion in a prairie marsh: A manipulative experiment. Wetlands 26, 430–437. doi:10.1672/0277-5212(2006)26[430
Goldsborough, L. G., and Wrubleski, D. A. (2001) The decline of Delta Marsh, an internationally significant wetland in south-central Manitoba. In Sixth Prairie Conservation and Endangered Species Conference.
Gordon, D. H. (1985) Postbreeding ecology of male mallards on the Delta marsh, Manitoba. [PhD dissertation]. [East Lansing, (MI)]: Michigan State University, Michigan.
Hamilton, D. P., and Mitchell, S. F. (1996). An empirical model for sediment resuspension in shallow lakes. Hydrobiologia 317, 209–220. doi:10.1007/BF00036471
Hann, B. J. (1995). Invertebrate associations with submersed aquatic plants in a prairie wetland. Univ. Manit. Field Stn. 30, 78–84.
Hanson, M. A., and Butler, M. G. (1994). Responses of plankton, turbidity, and macrophytes to biomanipulation in a shallow prairie lake. Can. J. Fish. Aquat. Sci. 51, 1180–1188. doi:10.1139/f94-117
Hertam, S. C. (2010). The effects of common carp (Cyprinus carpio L.) on water quality, algae and submerged vegetation in Delta Marsh. Manitoba: University of Manitoba. Manitoba. [master’s thesis]. [Winnipeg (MB)] http://hdl.handle.net/1993/4207.
Hilt, S., and Gross, E. M. (2008). Can allelopathically active submerged macrophytes stabilize clear-water states in shallow lakes? Basic Appl. Ecol. 9, 422–432. doi:10.1016/j.baae.2007.04.003
Hinks, D., and Fryer, R. (1936). Aquatic plant survey 1936. Manitoba: Manitoba Department of Mines and Natural Resources Government Report.
Hinks, D. (1943). The fishes of Manitoba. Winnipeg, MB, Canada: Department of Mines and Natural Resources.
Hnatiuk, S. D. (2006). Manitoba: University of Manitoba. [master’s thesis]. [Winnipeg (MB)] http://hdl.handle.net/1993/7963. Experimental manipulation of ponds to determine the impact of common carp (Cyprinus carpio L.) in Delta Marsh, Manitoba: Effects on water quality, algae, and submersed vegetation.
Horppila, J., and Nurminen, L. (2005). Effects of different macrophyte growth forms on sediment and P resuspension in a shallow lake. Hydrobiologia 545, 167–175. doi:10.1007/s10750-005-2677-9
Huser, B. J., Bajer, P. G., Kittelson, S., Christenson, S., and Menken, K. (2022). Changes to water quality and sediment phosphorus forms in a shallow, eutrophic lake after removal of common carp (Cyprinus carpio). Inland Waters 12, 33–46. doi:10.1080/20442041.2020.1850096
Ivey, G. L., Cornely, J. E., and Ehlers, B. D. (1998). Carp impacts on waterfowl at malheur national Wildlife refuge. https://pdfs.semanticscholar.org/ed90/29bf2c4472074571d7af729dc4483c10ed9e.pdf (Accessed May 24, 2019).Oregon. Transactions of the 63rd North American Wildlife and Natural Resources Conference.
Jůza, T., Duras, J., Blabolil, P., Sajdlová, Z., Hess, J., Chocholoušková, Z., et al. (2019). Recovery of the Velky Bolevecky pond (Plzen, Czech Republic) via biomanipulation–key study for management. Ecol. Eng. 136, 167–176. doi:10.1016/j.ecoleng.2019.06.025
Kaemingk, M. A., Jolley, J. C., Paukert, C. P., Willis, D. W., Henderson, K., Holland, R. S., et al. (2016). Common carp disrupt ecosystem structure and function through middle-out effects. Mar. Freshw. Res. 68, 718–731. doi:10.1071/MF15068
Keast, A. (1984). The introduced aquatic macrophyte, Myriophyllum spicatum, as habitat for fish and their invertebrate prey. Can. J. Zool. 62, 1289–1303. doi:10.1139/z84-186
Keddy, P. A. (1982). Quantifying within-lake gradients of wave energy: Interrelationships of wave energy, substrate particle size and shoreline plants in Axe Lake, Ontario. Aquat. Bot. 14, 41–58. doi:10.1016/0304-3770(82)90085-7
Körner, S., and Nicklisch, A. (2002). Allelopathic growth inhibition of selected phytoplankton species by submerged macrophytes. J. Phycol. 38, 862–871. doi:10.1046/j.1529-8817.2002.t01-1-02001.x
Kosten, S., Huszar, V. L., Bécares, E., Costa, L. S., van Donk, E., Hansson, L. A., et al. (2012). Warmer climates boost cyanobacterial dominance in shallow lakes. Glob. Chang. Biol. 18, 118–126. doi:10.1111/j.1365-2486.2011.02488.x
Lapointe, G. D. (1986). St. Paul, (MN): University of Minnesota. [master’s thesis].Fish movement and predation on macroinvertebrates in a lakeshore marsh.
Lougheed, V. L., Crosbie, B., and Chow-Fraser, P. (1998). Predictions on the effect of common carp (Cyprinus carpio) exclusion on water quality, zooplankton, and submergent macrophytes in a Great Lakes wetland. Can. J. Fish. Aquat. Sci. 55, 1189–1197. doi:10.1139/f97-315
Lougheed, V. L., Theÿsmeÿer, T., and Chow-Fraser, P. (2004). Carp exclusion, food-web interactions, and the restoration of Cootes Paradise Marsh. J. Gt. Lakes. Res. 30, 44–57. doi:10.1016/S0380-1330(04)70328-7
Marker, A. F. H., Nusch, E. A., Rai, H., and Riemann, B. (1980). The measurement of photosynthetic pigments in freshwaters and standardization of methods: Conclusions and recommendations. Arch. Für Hydrobiol. 14, 91–106.
McColl, K. A., Sunarto, A., Williams, L. M., and Crane, M. St.J. (2007). Koi herpes virus: Dreaded pathogen or white knight? Aquac. Health Int. 9, 4–6. http://hdl.handle.net/102.100.100/127764?index=1.
Meijer, M. L., de Haan, M. W., Breukelaar, A. W., and Buiteveld, H. (1990). Is reduction of the benthivorous fish an important cause of high transparency following biomanipulation in shallow lakes? Hydrobiologia 200/201, 303–315. doi:10.1007/BF02530348
Miller, J. W., Kocovsky, P. M., Wiegmann, D., and Miner, J. G. (2018). Fish community responses to submerged aquatic vegetation in Maumee Bay, western Lake Erie. North Am. J. Fish. manage. 38, 623–629. doi:10.1002/nafm.10061
Page, E. C. M. (2011). Manitoba: University of Manitoba. [master’s thesis]. [Winnipeg (MB)] http://hdl.handle.net/1993/4831.A water quality assessment of Lake Manitoba, a large shallow lake in central Canada.
Randall, R. G., Minns, C. K., Cairns, V. W., and Moore, J. E. (1996). The relationship between an index of fish production and submerged macrophytes and other habitat features at three littoral areas in the Great Lakes. Can. J. Fish. Aquat. Sci. 53, 35–44. doi:10.1139/f95-271
Robel, R. J. (1961). Water depth and turbidity in relation to growth of sago pondweed. J. Wildl. Manage. 25, 436–438. doi:10.2307/3798837
Rohweder, J., Rogala, J. T., Johnson, B. L., Anderson, D., Clark, S., Chamberlin, F., et al. (2012). Application of wind fetch and wave models for habitat rehabilitation and enhancement projects – 2012 update. Contract Rep. Prep. U.S. Army Corps Engineers’ Up. Miss. River Restor. – Environ. Manag. Program. 1–52.
Scheffer, M., de Redelijkheid, M. R., and Noppert, F. (1992). Distribution and dynamics of submerged vegetation in a chain of shallow eutrophic lakes. Aquat. Bot. 42, 199–216. doi:10.1016/0304-3770(92)90022-B
Scheffer, M., Portielje, R., and Zambrano, L. (2003). Fish facilitate wave resuspension of sediment. Limnol. Oceanogr. 48, 1920–1926. doi:10.4319/lo.2003.48.5.1920
Scheffer, M. (1999). The effect of aquatic vegetation on turbidity; how important are the filter feeders? Hydrobiologia 408/409, 307–316. doi:10.1023/a:1017011320148
Scheffer, M., van den Berg, M., Breukelaar, A., Breukers, C., Coops, H., Doef, R., et al. (1994). Vegetated areas with clear water in turbid shallow lakes. Aquat. Bot. 49, 193–196. doi:10.1016/0304-3770(94)90038-8
Schrage, L. J., and Downing, J. A. (2004). Pathways of increased water clarity after fish removal from Ventura Marsh; a shallow, eutrophic wetland. Hydrobiologia 511, 215–231. doi:10.1023/B:HYDR.0000014065.82229.c2
Sorensen, P. W., and Bajer, P. G. (2020). Case studies demonstrate that common carp can be sustainably reduced by exploiting source-sink dynamics in Midwestern lakes. Fishes 5, 36. doi:10.3390/fishes5040036
Stuart, I. G., Williams, A., McKenzie, J., and Holt, T. (2011). Managing a migratory pest species: A selective trap for common carp. N. Am. J. Fish. Manag. 26, 888–893. doi:10.1577/M05-205.1
Swain, D. P. (1979). Biology of the carp (Cyprinus carpio) in North America and its distribution in Manitoba, North Dakota and neighbouring U.S. waters. Manitoba Winnipeg, MB, Canada: Manitoba Department of Natural Resources. Manuscript Report 79–73.
Tempero, G. W., Hicks, B. J., Ling, N., Morgan, D., Daniel, A. J., Özkundakci, D., et al. (2019). Fish community responses to invasive fish removal and installation of an exclusion barrier at Lake Ohinewai, Waikato. N. Z. J. Mar. Freshw. Res. 53, 397–415. doi:10.1080/00288330.2019.1579101
Tryon, C. A. (1954). The effect of carp exclosures on growth of submerged aquatic vegetation in Pymatuning Lake, Pennsylvania. J. Wildl. Manage. 18, 251–254. doi:10.2307/3797722
van Donk, E., Grimm, M. P., Gulati, R. D., and Klein Breteler, J. P. G. (1990). Whole-lake food-web manipulation as a means to study community interactions in a small ecosystem. Hydrobiologia 200/201, 275–289. doi:10.1007/BF02530346
Van Zuidam, B. G., and Peeters, E. T. H. M. (2015). Wave forces limit the establishment of submerged macrophytes in large shallow lakes. Limnol. Oceanogr. 60, 1536–1549. doi:10.1002/lno.10115
Verrill, D. D., and Berry, C. R. (1995). Effectiveness of an electrical barrier and lake drawdown for reducing common carp and bigmouth buffalo abundances. N. Am. J. Fish. Manage. 15, 137–141. doi:10.1577/1548-8675(1995)015%3C0137
Watchorn, K. E., Goldsborough, G. L., Wrubleski, D., and Mooney, B. G. (2012). A hydrogeomorphic inventory of coastal wetlands of the Manitoba great lakes: Lakes Winnipeg, Manitoba, and Winnipegosis. J. Gt. Lakes. Res. 38 (3), 115–122. doi:10.1016/j.jglr.2011.05.008
Weaver, M. J., Magnuson, J. J., and Murray, C. K. (1997). Distribution of littoral fishes in structurally complex macrophytes. Can. J. Fish. Aquat. Sci. 54, 2277–2289. doi:10.1139/f97-130
Wood, A. M., White, I. R., and Royston, P. (2008). How should variable selection Be performed with multiply imputed data? Stat. Med. 27, 3227–3246. doi:10.1002/sim.3177
Wrubleski, D., Badiou, P., and Goldsborough, G. (2016). “Coastal wetlands of Manitoba’s great lakes (Canada),” in The wetland book. Editors C. Finlayson, G. Milton, R. Prentice, and N. Davidson (Dordrecht: Springer). doi:10.1007/978-94-007-6173-5_190-1
Keywords: coastal wetland, freshwater marsh, common carp, invasive species, biomanipulation, water clarity, submersed aquatic vegetation
Citation: Kowal PD, Badiou P, Emery RB, Goldsborough LG, Wrubleski DA, Armstrong LM and Page B (2022) Improvements in water clarity and submersed aquatic vegetation cover after exclusion of invasive common carp from a large freshwater coastal wetland, Delta Marsh, Manitoba. Front. Environ. Sci. 10:992690. doi: 10.3389/fenvs.2022.992690
Received: 12 July 2022; Accepted: 22 August 2022;
Published: 21 September 2022.
Edited by:
He Yixin,Chengdu Institute of Biology (CAS), ChinaReviewed by:
Bo Kang, Hefei University of Technology, ChinaZihao Wen, Institute of Hydrobiology (CAS), China
Copyright © 2022 Kowal, Badiou, Emery, Goldsborough, Wrubleski, Armstrong and Page. This is an open-access article distributed under the terms of the Creative Commons Attribution License (CC BY). The use, distribution or reproduction in other forums is permitted, provided the original author(s) and the copyright owner(s) are credited and that the original publication in this journal is cited, in accordance with accepted academic practice. No use, distribution or reproduction is permitted which does not comply with these terms.
*Correspondence: Paige D. Kowal, p_kowal@ducks.ca