- University of Natural Resources and Life Sciences, Vienna, Department of Water, Atmosphere and Environment, Institute of Hydrobiology and Aquatic Ecosystem Management, Vienna, Austria
Rivers worldwide have been transformed into fragmented, impounded, channelized, and flow-regulated ecosystems. These anthropogenic transformations can reduce fish distribution and population status, especially of those species belonging to medium- or long-distance migratory guilds and those dependent on free-flowing rivers and intact sediment and habitat conditions. Here, we aim to understand how different hydro-morphological pressure types affect the distribution and population status of key potamodromous fish species of the rheophilic and lithophilic fish guilds, the barbel (Barbus barbus) and the nase (Chondrostoma nasus). We also assess the status of chub (Squalius cephalus) to include a species less sensitive to habitat degradation. For the first time, we assembled an extensive Austrian-wide GIS-based fish sampling database with hundreds of biological surveys, allowing us to analyze quantitatively >4,000 river kilometers for presence/absence of target fishes and to assess population status. The data reveal that the distribution range of target species decreased by around 40–60% compared to their natural ranges according to the reference standard (Leitbild). Hydro-morphological pressures affect target species’ population biomass, and trends between impact types can be detected. Chub and barbel exhibit the highest median biomass in free-flowing rivers and residual flow reaches, followed by reservoir sections. Of all pressure types, population biomass is lowest in hydropeaked river stretches. Nase biomass has a grand median of 0.0 kg/ha across all sites, showing hardly any differences between hydro-morphological pressure types. Overall, our results show a drastic shrinkage of the distribution range of three cyprinid fish species previously prominent in Austria. By linking current population vitality to hydro-morphological stressors and ecological status assessments, this study sets a baseline for data-based conservation actions of (Red-listed) species as well as policy and management frameworks.
Introduction
Anthropogenic stressors in the world’s rivers are at an all-time high—and still rising. Consequently, the state of freshwater biodiversity and aquatic integrity is far worse than that of other ecosystems (Darwall et al., 2018; WWF, 2020). In this regard, hydro-morphological impacts constitute a prominent stressor group that can reduce fish species’ distribution range or diminish their population status. For example, dams and weirs are known to block fish migration routes, leading to fragmented populations with restricted access to vital spawning grounds, likely constituting a serious ecological bottleneck (Mattocks et al., 2017). Similarly, water abstraction and reservoirs can reduce passability in the river system. Moreover, these conditions alter habitat structure and availability, which can have dire consequences for fish dependent upon free-flowing rivers and intact sediment conditions (Hayes et al., 2018; Schmutz and Moog, 2018). Other common stressors are rapid flow fluctuations through peak-flow production; such hydropeaked rivers often feature diminished ecological integrity (Hayes et al., 2021, 2022).
In light of ever-increasing pressures on river ecosystems, it is urgent to describe the current status of target fish populations. Indeed, conservation and restoration efforts rely more heavily on comparing the status quo with the natural-historic reference situation (Schmutz et al., 2000; Palmer et al., 2005). Knowing how fishes respond to riverine stressors on the large scale of an entire region or country can help guide goals and strategies to mitigate adverse effects at the local scale or vice versa (Palmer et al., 2005).
In this regard, Peňáz (1996) calls for studies that assess the present status of target populations from multiple rivers and link these data to anthropogenic stressors. To this call, we compared the natural-historic and current status of three fish species previously widespread in Austrian rivers that are heavily impacted by diverse hydro-morphological stressors (Muhar et al., 2019). We chose three cyprinid fish as target species for this study: barbel (Barbus barbus), nase (Chondrostoma nasus), and chub (Squalius cephalus). All these species are prevalent in the epipotamal fish region (barbel zone)—of which the barbel is also the name giver—but also inhabit other river sections, particularly the hyporhithral region (grayling zone; Huet, 1959; Kottelat and Freyhof, 2007). The rheophilic and lithophilic barbel and nase both undergo longer spawning migrations and are excellent indicators for functioning rivers and restoration measures, particularly regarding habitat connectivity (Melcher and Schmutz, 2010). Chub, in contrast, is a species of higher environmental plasticity as it can handle a variety of habitat conditions and is therefore known to be more tolerant of habitat degradation (Arlinghaus and Wolter, 2003). Unsurprisingly, the first two are classified as ‘near threatened’ according to the Austrian Red List, whereas the latter is a species of ‘least concern’ (Wolfram and Mikschi, 2007).
In detail, the objectives of this study are to analyze (i) the distribution range and (ii) the population status of three cyprinid species–chub, barbel, nase–with regard to the natural-historic situation and hydro-morphological stressors, and (iii) to link population vitality with the ecological status assessment of the EU Water Framework Directive (2000/60/EC). Moreover, by comparing species-specific results, we investigate how response patterns of species that are more or less sensitive to habitat degradation differ.
Materials and methods
Study area and Leitbild classification
Austria is a landlocked country in central Europe, with a river network of 31,600 km (catchment size ≥10 km2), flowing within towering Alpine mountains and alluvial lowlands (BMLFUW, 2017). Austrian rivers are impacted by various anthropogenic stressors, particularly by hydro-morphological alterations. For example, Austria exhibits, on average, one migration barrier per river kilometer. Water diversions affect 13.7% of the nation’s river network (≥10 km2 catchment area). Over 4% of all rivers have been transformed into reservoirs. Almost 900 river km (2.8%) are impacted by hydropeaking (BMLFUW, 2017).
The study area for each target fish species is the natural, type-specific, or historic distribution of the species according to the national Leitbild catalog (BAW, 2007). The Leitbild catalog classifies a species’ reference status at the river section level according to a four-tier level structure, being either dominant (‘Leitart’), accompanying (subdominant), rarely occurring, or not occurring. This target-vision benchmark classification is based on environmental parameters such as bioregion, catchment size, or altitude. Also, historic sources are used where available (Muhar et al., 1995; Schmutz et al., 2000; BAW, 2007). Here, we relied on the GIS-based version that was updated for the target species based on more recent literature and expert knowledge (Schaufler, 2021).
Fish data
We assembled an extensive GIS-based fish sampling database by combining the survey datasets of the Austrian Federal Ministry of Agriculture, Regions and Tourism with those of the Institute of Hydrobiology and Aquatic Ecosystem Management. In both datasets, sampling was conducted via quantitative electrofishing according to the national sampling guidelines (Haunschmid et al., 2006) in accordance to the EU Water Framework Directive. In short, electrofishing sampling was performed during low flow conditions in fall by wading, boat, or a mix of both, depending on the river size. In smaller water bodies, two- or three-pass electrofishing removal approaches of representative river reaches were conducted (DeLury, 1947; Seber and Le Cren, 1967). In larger water bodies, fish sampling was done via boats equipped with an anode boom, proportionally sub-sampling available habitats (Schmutz et al., 2001). Fish population status was calculated as biomass per hectare [kg/ha] based on the sampled area. We transferred sampling locations to the Austrian river network through river kilometer marks and geographic coordinates and corrected erroneous locations through site descriptions (Figure 1).
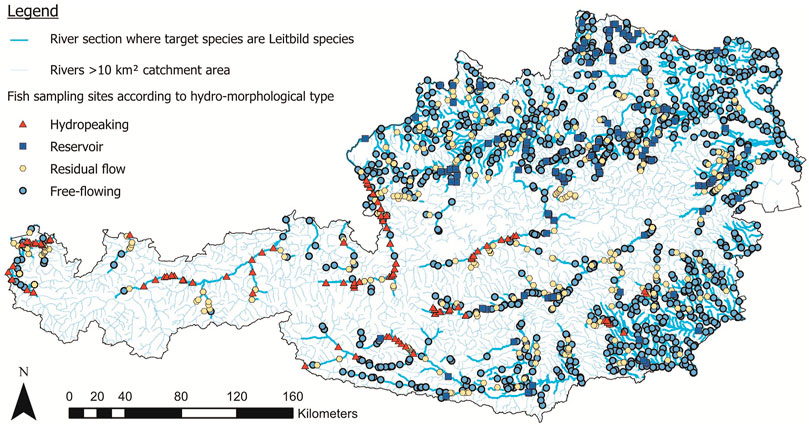
FIGURE 1. Fish sampling sites in Austria located in river sections where the target species chub, barbel and nase occur according to their historic-natural (‘Leitbild’) distribution range.
It was assumed that a fish sampling site reflects the occurrence or population status of a continuous river stretch with the same hydro-morphological characteristics. Hence, we divided the entire river network into subsections. We based the delineation of subsections on non-passable migration barriers (natural or artificial) and edges of hydro-morphological stressor categories (see below). Further, we split subsections >10 km in length into smaller routes to avoid single sampling sites representing a too-long river section. Therefore, to ensure a maximum subsection length of 10 km, we halved sections between 10 and 20 km; a section between 20 and 30 km was divided into thirds, a section between 30 and 40 km was divided into fourths, and so forth. This reach length was the basis for comparing the historic-natural with the current presence/absence status of the target species in terms of the distribution range in river kilometers (Figure 1).
If the same river reach was sampled for multiple years, we only considered the most recent one and excluded all others from the analysis. The selected most recent fish surveys were performed between 2000 and 2018 (median = 2010).
Hydro-morphological stressors
We linked possible hydro-morphological impacts to each sampling site by using the location of residual flow stretches, reservoirs, or hydropeaking sections as determined by the National River Basin Management Plan (BMLFUW, 2017).
Furthermore, we calculated the maximum corridor length of the mainstem river between two non-passable barriers at each sampling site to analyze the effect of river fragmentation on the target species. For this purpose, the rivers were divided at all non-passable barriers, at all non-passable residual flow stretches, and at all dams with a reservoir length >1 km. Migration studies indicate that long reservoirs influence fish movements (Havn et al., 2018; Unfer and Rauch, 2019). Therefore, following Hayes et al. (2021), we also classified dams with a reservoir length >1 km as migration barriers, even if a fish ladder is present.
Target variables and statistical analyses
First, using presence/absence data, we compared the current distribution range of the target species with the natural-historic range as determined by the Leitbild. Aside from assessing total shifts in distribution, we also analyzed how species distribution changed in distinct fish ecological river regions (Huet, 1959) using descriptive statistics. The river reach length detailed above was the basis for assessing the current distribution range in river kilometers (sum per species and fish region).
Secondly, we assessed species’ population status in different hydro-morphological river sections using fish biomass [kg/ha] as the dependent variable. Considering that even in good river conditions, a target species might exhibit low population biomass in areas where it is classified as ‘rarely occurring’ according to the Leitbild (BAW, 2007), we analyzed only sites where the respective species is classified as ‘dominant’ or ‘subdominant’ (Hayes et al., 2021). Of these, sites without the target species were assigned 0 kg/ha fish biomass; therefore, our target variables follow a non-parametric distribution. Hence, we used the median test to investigate if the biomass of the target species differs between free-flowing rivers, residual flow sections, reservoirs, or hydropeaked rivers. Following, we assessed the influence of river corridor length on population status in each of the four hydro-morphological categories using Spearman’s rank correlation. Correlation strength was interpreted according to Cohen (1988), where |ρ| = 0.1 indicates a weak correlation, |ρ| = 0.3 a medium one, and |ρ| = 0.5 a strong correlation. Considering that this dataset spans 18 years (2000–2018), we also used Spearman’s rank correlation to elucidate if temporal aspects were linked to fish biomass.
In the next step, we investigated fish population status [kg/ha] in those fish ecological river regions that constitute the target species’ main distribution range—the hyporhithral and the epipotamal region (Huet, 1959; Kottelat and Freyhof, 2007). We used the median test to assess differences between the hydro-morphological stressors per fish region and the same stressor category between the two fish ecological regions.
Finally, we linked the species’ population status (biomass) to the ecological assessment according to the EU Water Framework Directive (BMLFUW, 2017) to understand how well the Water Framework Directive classification reflects the population status of the three target species. Therefore, we used the median test to assess differences in species biomass between the categories ‘high’ to ‘bad’ (natural water bodies) and ‘good or better’ and ‘moderate or worse’ (heavily modified water bodies).
The level of significance was p < 0.05 for all statistical tests. Regarding the median tests, the significance values for pairwise post-hoc tests were Bonferroni-adjusted. Analyses were performed with IBM SPSS Statistics 27.
Results
Historic distribution range according to Leitbild status
The river reaches analysis revealed that chub has the largest natural distribution range of the three target species, totaling >14,100 river kilometers (rkm) according to the reference standard (Leitbild) (Figure 2A). In Austrian rivers, chub is classified as a dominant species in about 44% of its original range; in 23% of all cases, it is considered an accompanying species and a rare accompanying species in 33%.
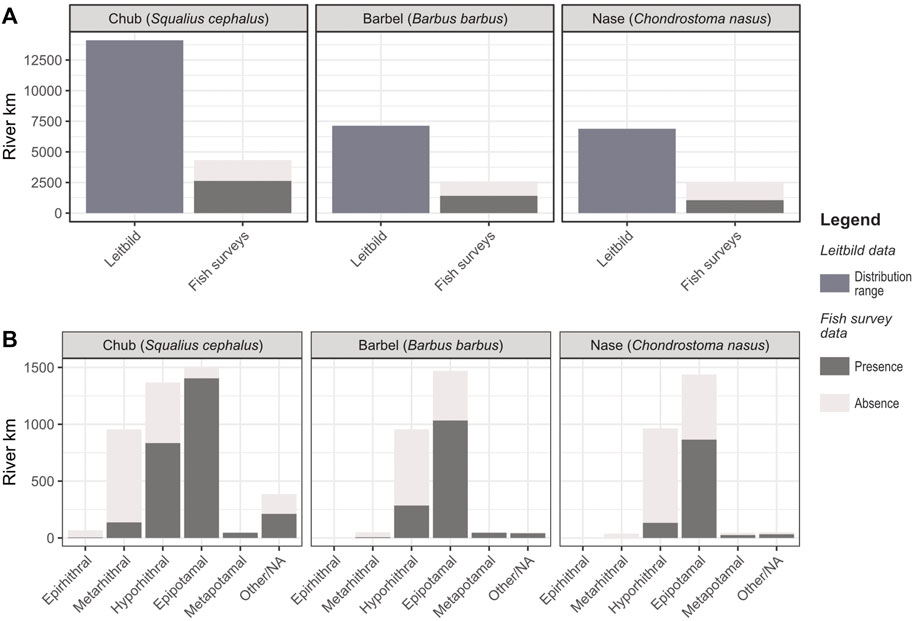
FIGURE 2. Presence/absence of target species in the fish survey dataset. (A) Comparison of the target species’ natural distribution range according to the Leitbild reference standard (left bar) and current presence/absence data (right bar), (B) species’ presence/absence in distinct fish ecological river regions based on fish survey data (chub: n = 1,430; barbel: n = 764; nase: n = 730).
The natural distribution range of the other two species is around half of the chub. Barbel inhabits around 7,130 rkm and nase 6,880 rkm according to the Leitbild classification (Figure 2A). The first is identified as a dominant species in 47%, an accompanying species in 22%, and a rare accompanying species in 31% of its Leitbild-based distribution range. For the nase, it is 50%, 20%, and 30%, respectively.
Present distribution range
Recent fish data are not available for all river reaches, but for 31% (4,327 rkm), 36% (2,581 rkm), and 37% (2,542 rkm) of all Leitbild river sections for chub, barbel, and nase, respectively. This constitutes 1,430, 764, and 730 sampling sites in each species’ natural range. In these subsections, presence/absence data reveals that nase suffers the greatest losses in the distribution range, decreasing by almost 60% of its original range. The distribution range of barbel decreased by 45% compared to its natural range. For chub, this loss is the lowest of all three species, with less than 40% (Figure 2A).
Presence/absence data of distinct fish ecological regions reveals that all target species entailed the greatest distribution losses in rhithral rivers. Chub is the only species with a Leitbild occurrence in the upper trout zone (epirhithral); in this region, however, it is absent in 92% of sampling sites. In the lower trout zone (metarhithral), chub and barbel reduced their distribution by 86% each. Further downstream, in the grayling zone (hyporhithral), nase suffered the largest losses (86%), followed by barbel (70%) and chub (39%). A similar pattern, albeit with lower losses, was found in the barbel zone (epipotamal); here, nase, barbel and chub are absent in 40%, 30%, and 7% of sampling sites, respectively (Figure 2B).
Population status and hydro-morphological stressors
Our dataset contains 730, 467, and 437 fish sampling sites where chub, barbel, and nase are classified as dominant or subdominant species. These survey stretches represent 2,450 rkm, 1,534 rkm, and 1,438 rkm, respectively. Most sites are located in free-flowing sections (51–66%, depending on species), followed by residual flow (16–20%) and reservoir stretches (14–22%). Hydropeaked sites are sampled least frequently, constituting 5–9% of all sites (Supplementary Table S1). In this sample subset, the biomass of chub ranges from 0.0 to 1,107.4 kg/ha. Biomass range of barbel and nase is 0.0–681.6 kg/ha and 0.0–1,075.6 kg/ha, respectively. Median biomass is highest for chub (14.3 kg/ha), followed by barbel (0.02 kg/ha) and nase (0.0 kg/ha).
We found evidence that the four hydro-morphological types affect the population status of chub (p = 0.001) and barbel (p = 0.002) but not of nase (p = 0.068) (Figure 3).
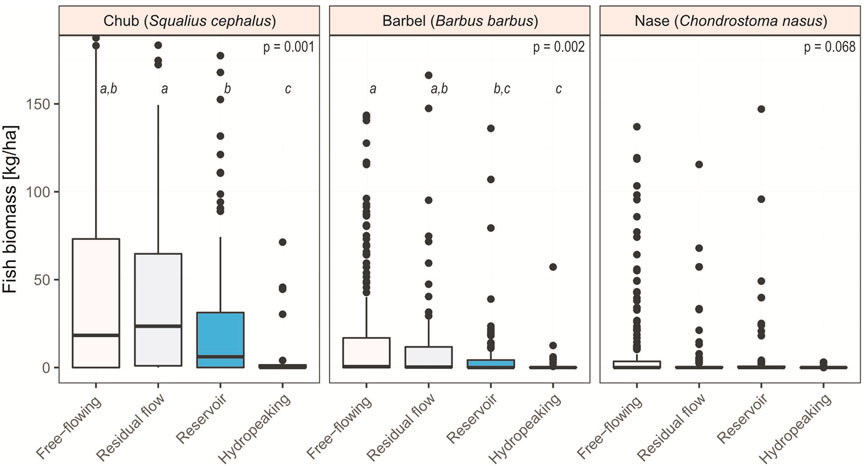
FIGURE 3. Biomass of the three target species in different hydro-morphological types. Grand median: Chub = 14.3 kg/ha, barbel = 0.02 kg/ha, nase = 0.0 kg/ha. Outliers >180 kg/ha have been graphically cut off for better readability (chub: n = 55, max = 1,107 kg/ha; barbel: n = 10, max = 682 kg/ha; nase: n = 9, max = 1,076 kg/ha). This dataset includes only river sections where the respective target species is classified as ‘dominant’ or ‘subdominant’ according to the Austrian Leitbild classification (BAW, 2007). The same letters above the boxplots indicate that the categories are considered equal, different letters indicate a significant difference.
In detail, regarding chub biomass, free-flowing sections (median = 18.3 kg/ha) do not differ from residual flow reaches (median = 23.5 kg/ha; p = 1.00) nor reservoir sections (median = 6.1 kg/ha; p = 0.129). Reservoir sections, however, exhibit significantly lower biomass than residual flow sections (p = 0.010). Hydropeaking sites have the lowest biomass values of all hydro-morphological types (median = 0.1 kg/ha), which was statistically significant for all three comparisons (p = 0.001, respectively).
Pairwise test of barbel biomass showed that free-flowing (median = 0.5 kg/ha) and residual flow sections (median = 0.3 kg/ha) have the same population status (p = 0.100). However, biomass in reservoirs (median = 0.0 kg/ha; p = 0.039) and hydropeaking rivers (median = 0.0 kg/ha; p = 0.008) is both lower compared to free-flowing sections. Except for hydropeaking and residual flow reaches (p = 0.016), all remaining pairwise comparisons do not reveal differences in barbel biomass.
Nase biomass does not differ between the hydro-morphological types as the grand median is 0.0 kg/ha (Figure 3).
In the next step, we linked species biomass to river corridor length to assess the effects of river fragmentation on population status. Overall, the target species’ biomass correlated weakly with river habitat length (chub: ρ = 0.038; barbel: ρ = 0.108; nase: ρ = 0.208; Supplementary Figure S1). Running the analysis with the respective data subset of the hydro-morphological categories (Figure 4) showed a low correlation for chub biomass in all four cases (min–max: ρ = -0.034–0.127). A medium correlation strength was detected for barbel in free-flowing rivers (ρ = 0.247), reservoir sections (ρ = 0.276), and hydropeaked rivers (ρ = -0.308). Similarly, the nase biomass exhibited a medium correlation strength with river corridor length in free-flowing stretches (ρ = 0.227), reservoirs (ρ = 0.229), and hydropeaked rivers (ρ = 0.352). In residual flow reaches, only a low correlation was observed (ρ = 0.186).
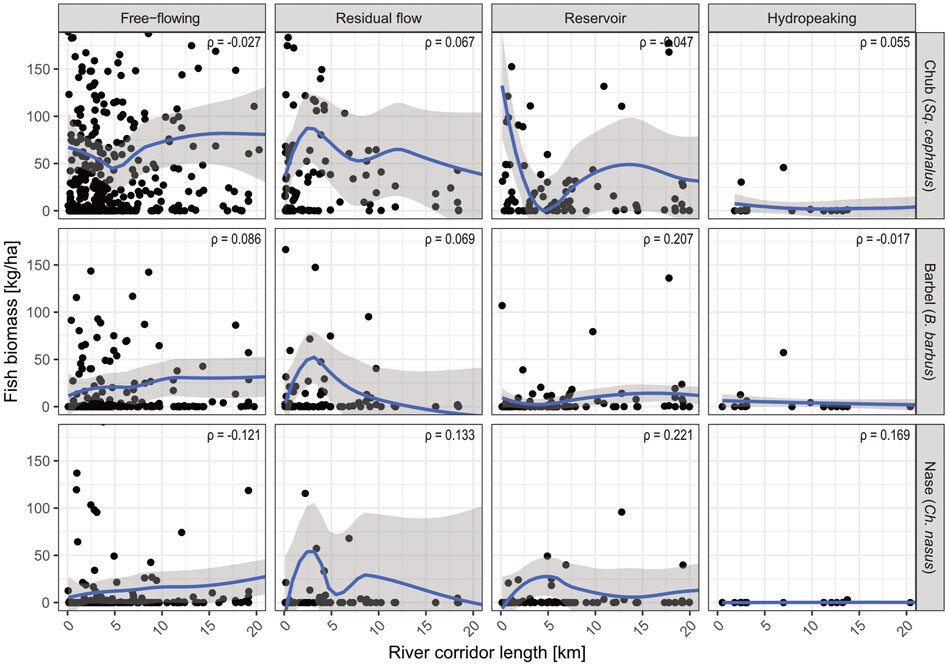
FIGURE 4. Scatterplot of fish biomass [kg/ha] and river corridor length [km] for each of the three target species. The shown curve is a LOESS interpolation. Sites with a corridor length >20 km and a biomass >180 kg/ha have been graphically cut for better readability (see Figure 3). This dataset includes only river sections where the respective target species is classified as ‘dominant’ or ‘subdominant’ according to the Austrian Leitbild classification (BAW, 2007).
Regarding population status in the target species’ main distribution range—the hyporhithral and epipotamal fish region (Figure 2B)—data showed strong evidence that the median total biomass of chub and barbel is higher in the epipotamal than in the hyporhithral fish region (Table 1). The test statistics also revealed a difference for the nase, despite a median of 0.0 kg/ha per fish region (Table 1). The correlation between fish biomass and river connectivity is weak for all three species and the two fish regions (|ρ| < 0.06). Only for the nase in the epipotamal a slightly higher correlation was detected (ρ = 0.215; Supplementary Figure S2).
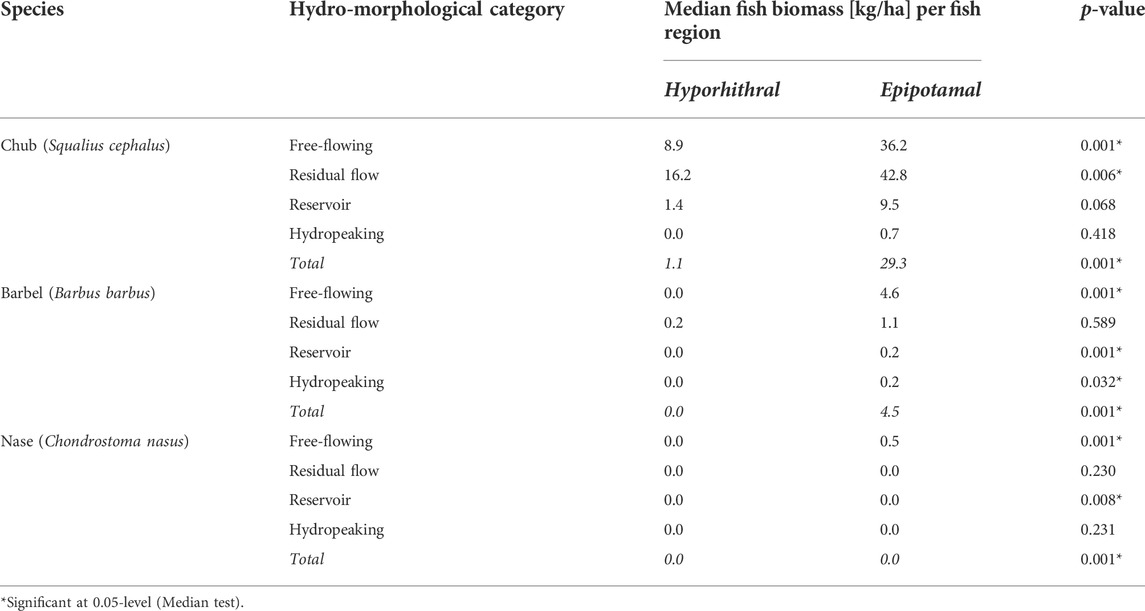
TABLE 1. Median biomass of target species in different hydro-morphological categories according to fish region (hyporhithral and epipotamal), as well as p-values of Median test assessing biomass differences between the fish regions.
Concerning the individual hydro-morphological groups (Figure 5), chub biomass in the two fish regions differs in free-flowing rivers and residual flow sections—showing higher biomass in the epipotamal than in the hyporhithral—but not in reservoirs or hydropeaked rivers (Table 1). Barbel biomass differs in free-flowing rivers, reservoirs, and hydropeaked sections, but is the same between the fish regions of residual flow sections. In all cases, however, median biomass is higher in the epipotamal than the hyporhithral. Nase only exhibits higher biomass in free-flowing sections of the epipotamal than the hyporhithral. In all other cases, median biomass is 0.0 kg/ha, irrespective of the fish region; still, reservoirs revealed a strong difference (p = 0.008; Table 1 and Figure 5).
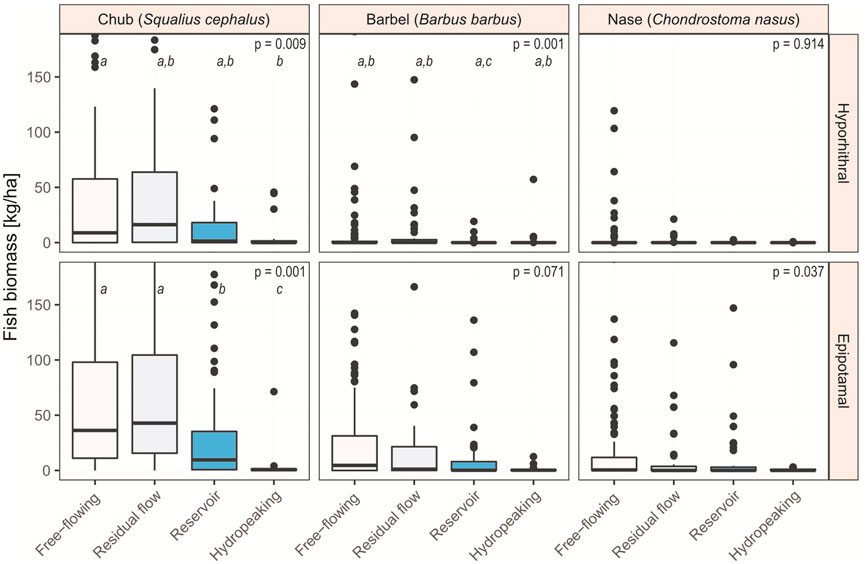
FIGURE 5. Biomass of the three target species in the hyporhithral and epipotamal fish region (grayling and barbel zone), separated by hydro-morphological impact type. Outliers and data points >180 kg/ha have been graphically cut off for better readability (chub: n = 55, max = 1,107 kg/ha; barbel: n = 10, max = 682 kg/ha; nase: n = 9, max = 1,076 kg/ha). This dataset includes only river sections where the respective target species is classified as ‘dominant’ or ‘subdominant’ according to the Austrian Leitbild classification (BAW, 2007). The same letters above the boxplots indicate that the categories are considered equal, different letters indicate a significant difference.
Regarding temporal effects, fish biomass and sampling year showed no more than a weak correlation (chub: ρ = -0.010; barbel: ρ = 0.103; nase: ρ = 0.008; Supplementary Figure S3). Also, no strong correlation was found regarding single hydro-morphological categories, the largest being ρ = 0.207 for barbel in reservoirs and ρ = 0.221 for nase in reservoirs. In most other instances, |ρ| was <0.1, indicating that the sampling year did not affect fish biomass (Supplementary Figure S4).
Fish population status and ecological status assessment
Linking target fish’ population status to the ecological status classification according to the EU Water Framework Directive (Figure 6; Supplementary Figure S5), median tests revealed that fish biomass of all species differs among the status classes (p < 0.001 each).
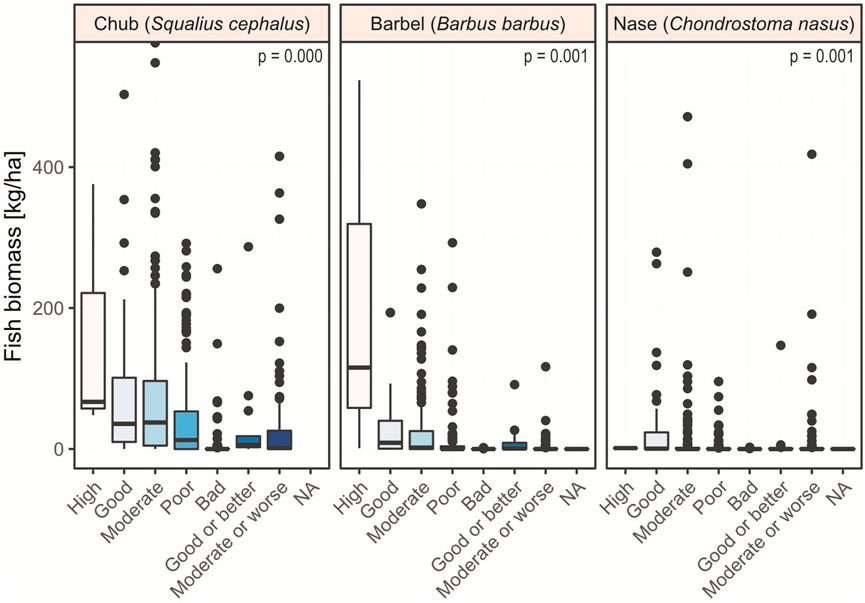
FIGURE 6. Biomass of the three target species with sites separated by the ecological status assessment according to the EU Water Framework Directive (2000/60/EC). Outliers and data points >550 kg/ha have been graphically cut off for better readability (chub: n = 7, max = 1,107 kg/ha; barbel: n = 1, max = 682 kg/ha; nase: n = 2, max = 1,076 kg/ha). This dataset includes only river sections where the respective target species is classified as ‘dominant’ or ‘subdominant’ according to the Austrian Leitbild classification (BAW, 2007).
In detail, chub biomass reveals a continuous decrease from the high to the bad status class (Figure 6). However, pairwise tests signaled no differences between the high status (median = 66.9 kg/ha) and other classes, probably because of the low case frequency in the high status class (n = 3). Chub biomass in the good status class (median = 35.8 kg/ha), however, is higher than that of the classifications ‘bad’ (median = 0.0 kg/ha), and ‘moderate or worse’ (median = 1.4 kg/ha; p < 0.001, respectively); the good status class does neither differ from ‘moderate’ (median = 37.6 kg/ha; p = 1.000), ‘poor’ (median = 12.7 kg/ha; p = 0.059), nor from ‘good or better’ (median = 6.1 kg/ha; p = 0.456). The moderate status distinguishes itself in chub biomass from ‘poor’, ‘bad’, and ‘moderate and worse’ (p < 0.001). The two grouped classes referring to the good ecological potential (i.e., ‘good or better’ and ‘moderate and worse’) do not differ (p = 0.468).
Like chub, barbel also features a biomass decline from the best categories to the others. In contrast, however, median biomass is close to zero already in the moderate class (Figure 6). In detail, the biomass of the high status class (median = 115.5 kg/ha) is larger than the class bad (median = 0.0 kg/ha; p = 0.020); however, the high class (n = 3) does not exhibit differences in the other five comparisons. The good class (median = 8.1 kg/ha) features higher barbel biomass than the classes ‘poor’ (median = 0.0 kg/ha), ‘bad’ (median = 0.0 kg/ha), and ‘moderate or worse’ (median = 0.0 kg/ha), and this is statistically significant (p < 0.001 each). Sites classified as ‘moderate’ (median = 2.0 kg/ha) exhibit higher barbel biomass than ‘poor’, ‘bad’, or ‘moderate or worse’ ones (p < 0.001 each). All remaining pairwise comparisons are non-significant.
Nase exhibits a median of 0.0 kg/ha in all categories except for ‘high’ (1.3 kg/ha), which, however, consists of only one site. The only comparisons that revealed significant differences were that the ‘good’ status differed from each of the following ones: ‘moderate’ (p = 0.003), ‘poor’ (p = 0.001), ‘bad’ (p = 0.001), and ‘moderate or worse’ (p = 0.002).
Discussion
Shrinking distribution range
In this study, we used an extensive fish sampling dataset to assess the current distribution and population status of three cyprinid fish species—chub, barbel, and nase—in Austrian rivers. Our data revealed that losses in the distribution range are around 40–60% compared to the species’ natural range. This loss is greater for barbel and nase than for chub. The higher reduction of barbel and nase presence at sampling sites may be due to their greater sensitivity to hydro-morphological pressures than chub. Both barbel and nase conduct spawning migrations which can reach up to 20 km (Ovidio et al., 2007; De Leeuw and Winter 2008) or 100 km (Steinmann et al., 1937; Povž, 1988) along the mainstem and into the tributaries. At the spawning grounds, they require fast-flowing flow velocities over clean gravel beds for reproduction (Melcher and Schmutz, 2010). Although the accessibility of feasible spawning grounds was not part of this study, it can be presumed that the high count of migration barriers in Austrian rivers (∼1/rkm) is a fundamental driver of this shrinking distribution range (Haidvogl et al., 2015; BMLFUW, 2017); however, it some instances, such as multi-basin studies, it has been difficult to establish the effect of connectivity losses on fish distribution (Branco et al., 2012).
However, not only do thousands of dams, weirs, and reservoirs constitute migration obstacles (Belletti et al., 2020), but also free-flowing sections for spawning are becoming scarcer in rivers where one reservoir follows another (Cheng et al., 2015). The clogging of gravel substrate and fine sediment infiltration is another common issue in many rivers and may lead to reduced reproduction success (Düregger et al., 2018). These environmental stressors may reduce the distribution range of riverine fish by affecting sensitive life cycle stages, such as spawning or egg-to-fry development.
In contrast to barbel and nase, the eurytopic chub is considered a species more robust to habitat degradation. Still, an absence in around 40% of all sampling sites is surprising, particularly considering that chub can occupy many fish ecological river regions. In contrast, barbel and nase have a narrower range (Figure 2). This marked decrease in chub appears to be mainly due to distribution losses in the metarhithral and hyporhitral fish regions.
It shall be noted that at the river basin level, distribution ranges would have shrunken more drastically than at national level. In the Salzach River, Austria, e.g., the presence of nase declined by around 80% of river segments surveyed in a historic study (Haidvogl et al., 2015). Also, chub and barbel exhibited marked declines of around 60% each from their historic distribution range in the Salzach River (Haidvogl et al., 2015).
Diminished population status
Analyses of population status revealed that free-flowing and residual flow sections do not differ in terms of chub and barbel biomass. This might be due to the following reasons. Firstly, free-flowing sections do not automatically constitute ‘reference sites’ as they can also be impacted by other stressors not assessed in this study, such as those related to flood protection, floodplain degradation, or land use (Schinegger et al., 2016; Müller et al., 2020). This notion is supported by low median biomass values in free-flowing river sections, particularly regarding barbel (0.5 kg/ha). Also, more than two-thirds of the assessed free-flowing sections only reach a moderate or worse ecological status/potential according to the EU Water Framework Directive, underlining the likeliness that other confounding factors impact fish population status in these river reaches.
Secondly, more and more residual flow stretches in Austria are receiving environmental flow allocations based on abiotic parameters determined by (the size of) the dominant fish species present (QZVÖ, 2010) and seasonally dynamic flows as recommended by the literature (e.g., Hayes et al., 2018). However, the overall low biomass values also indicate that the ecological effects of these measures are not yet sufficient on a large scale. Moreover, river corridor length exhibited only a weak correlation strength with chub biomass and a medium one with barbel biomass in free-flowing sections. This weak correlation underlines that more work is needed to understand stressor hierarchies on varying spatial levels to develop custom-tailored management solutions (Birk et al., 2020).
Results also show that chub and barbel biomass in reservoirs is consistently lower than in free-flowing and residual flow sections. This pattern may indicate that the conditions of these artificial environments are less suitable for these cyprinid species than free-flowing and residual flow sections (Schmutz and Moog, 2018), presuming that sampling efficiency in reservoirs is not the main cause of this result. One can assume that this pattern would be similar for the nase, but due to the low nase stocks throughout Austria, this cannot be concluded from the data at hand. However, research at the Austrian Danube revealed that adult barbel also select reservoir habitats during large parts of the year despite reachable free-flowing tributaries, which are predominantly accessed for spawning (Panchan et al., accepted).
The fourth impact type—hydropeaking—yielded the lowest chub and barbel biomass of all hydro-morphological types. This outcome was expected in light of the manifold impacts of artificial flow fluctuations (Schmutz et al., 2015; Hayes et al., 2019, 2022); however, regarding cyprinid fish, studies on the effects of hydropeaking on fundamental hydro-ecological processes such as fish drift and stranding are largely still outstanding (Boavida et al., 2020; but see Führer et al., 2022).
Nase populations exhibited median biomass of 0.0 kg/ha in sites throughout Austria. This value was the same for all hydro-morphological types, except for free-flowing sections of the epipotamal fish region, which showed median biomass of 0.5 kg/ha. Nase exhibited the best links between fish biomass and river corridor length of all target species, particularly in free-flowing sections, reservoirs, and hydropeaked rivers; in these cases, there was medium evidence that increased corridor length positively affected nase biomass. Nevertheless, adequate habitats for all life cycle stages are key to maintaining healthy fish populations (Jurajda, 1995; Schiemer et al., 2002). The Austrian Enns River, e.g., features a highly-impacted reservoir cascade. Surprisingly, one of these short sub-sections between two dams boasts a noticeable nase population with biomass values of around 17 kg/ha in the reservoir. This population status is maintained by tributaries entering this fragmented reach of the Enns River. These tributaries are accessible to the nase reservoir population (>7,000 adult spawners were counted in 2016) that benefits from the high-quality spawning and rearing habitats (Führer et al., 2017).
A call for conservation
In our dataset, the median biomass of nase populations was disturbingly low (0.0 kg/ha). Even sampling locations with biomass >3.6 kg/ha already constitute outliers in the dataset (n = 98). Indeed, this critical population status is not restricted to Austria but has been observed throughout the species distribution range (Jurajda, 1995; Peňáz, 1996). The data-based status assessment presented in this work necessitates enhanced conservation efforts. The first step towards such efforts would be to rework the nase’s national vulnerability classification, especially since the last update occurred almost two decades ago (Wolfram and Mikschi, 2007). Critical reviews of international vulnerability classifications are also required.
The same vulnerability re-classification should also be done for the barbel in Austria, as the grand median biomass was only slightly higher (0.02 kg/ha) than that of the nase, albeit having more sampling sites with higher biomass values. One reason for this species-specific difference in population decline may lie in the species’ spawning habitat requirements. In general, nase requires higher flow velocities than barbel (Melcher and Schmutz, 2010) and possibly looser substrate conditions for egg-to-fry development (Düregger et al., 2018; Pelz, 2022). Hence, this pattern of species difference can be explained by the fact that habitats with higher flow velocities and regularly rearranged sediments are increasingly difficult to find in many of today’s hydro-morphologically modified river systems.
Our results showed that species biomass was generally well aligned with the ecological status classification according to the EU Water Framework Directive. This underlines the notion that particularly barbel and nase are key indicators for assessing the environmental conditions of European rivers (Schiemer et al., 2002; Britton and Pegg, 2011).
Study limitations and future research needs
This study served as the first national assessment of the distribution and population status of chub, barbel, and nase. Here, we used four prevalent hydro-morphological impact types to compare biomass across categories and link this status with river corridor length. However, as indicated above, the target species might also be impacted by other and interacting stressors (Côté et al., 2016), including land use or changing water temperatures. Hence, future works should aim to understand and detangle the effects of multiple stressors on target species populations (Schinegger et al., 2016; Hayes et al., 2021).
Data availability statement
The data analyzed in this study is subject to the following licenses/restrictions: Basic data are subject to third party restrictions. Fish data are from the Austrian Federal Ministry of Agriculture, Regions and Tourism according to “Gewässerzustandsüberwachungsverordnung in Österreich gemäß Wasserrechtsgesetz 1959 idgF §§ 59 c-i bzw. Gewässerzustandsüberwachungsverordnung (GZÜV, BGBl II 2006/479 idgF); BMNT, Abteilung I/3”) and the Institute of Hydrobiology and Aquatic Ecosystem Management, University of Natural Resources and Life Sciences, Vienna. Requests to access these datasets should be directed to the Austrian Federal Ministry of Agriculture, Regions and Tourism, Abteilung I/3.
Author contributions
DH: Conceptualization, Methodology, Validation, Formal analysis, Writing–Original Draft, Writing–Review and Editing, Visualization, Funding acquisition. GS: Formal analysis, Writing–Review and Editing, Investigation, Data curation. SS: Methodology, Validation, Writing–Review and Editing, Supervision, Funding acquisition. GU: Conceptualization, Validation, Writing–Review and Editing. SF: Validation, Writing–Review and Editing, Funding acquisition. SA: Validation, Writing–Review and Editing, Funding acquisition. CS: Methodology, Validation, Resources, Writing–Review and Editing.
Funding
This research was funded in whole, or in part, by the Austrian Science Fund (FWF) (P 34061-B).
Acknowledgments
We thank the colleagues for their expert input for updating the GIS-based Leitbild version and the Austrian Federal Ministry of Agriculture, Regions and Tourism for providing fish sampling data.
Conflict of interest
The authors declare that the research was conducted in the absence of any commercial or financial relationships that could be construed as a potential conflict of interest.
Publisher’s note
All claims expressed in this article are solely those of the authors and do not necessarily represent those of their affiliated organizations, or those of the publisher, the editors and the reviewers. Any product that may be evaluated in this article, or claim that may be made by its manufacturer, is not guaranteed or endorsed by the publisher.
Supplementary material
The Supplementary Material for this article can be found online at: https://www.frontiersin.org/articles/10.3389/fenvs.2022.991722/full#supplementary-material
References
Arlinghaus, R., and Wolter, C. (2003). Amplitude of ecological potential: Chub Leuciscus cephalus (L.) spawning in an artificial lowland canal. J. Appl. Ichthyol. 19, 52–54. doi:10.1046/J.1439-0426.2003.00343.X
BAW (2007). Fisch index Austria (FIA) leitbildkatalog. Available at: https://www.baw.at/wasser-fische-IGF/abteilungen/gewaesseroekologie-igf/eu-wasserrahmenrichtlinie-eu-wrrl-/fisch-index-austria--fia-.html.
Belletti, B., Garcia de Leaniz, C., Jones, J., Bizzi, S., Börger, L., Segura, G., et al. (2020). More than one million barriers fragment Europe’s rivers. Nature 588, 436–441. doi:10.1038/s41586-020-3005-2
Birk, S., Chapman, D., Carvalho, L., Spears, B. M., Andersen, H. E., Argillier, C., et al. (2020). Impacts of multiple stressors on freshwater biota across spatial scales and ecosystems. Nat. Ecol. Evol. 4, 1060–1068. doi:10.1038/s41559-020-1216-4
BMLFUW (2017). Nationaler Gewässerbewirtschaftungsplan 2015 [National River Basin Management Plan 2015]. Vienna.
Boavida, I., Díaz-Redondo, M., Fuentes-Pérez, J. F., Hayes, D. S., Jesus, J., Moreira, M., et al. (2020). Ecohydraulics of river flow alterations and impacts on freshwater fish. Limnetica 39, 213–232. doi:10.23818/limn.39.14
Branco, P., Segurado, P., Santos, J. M., Pinheiro, P., and Ferreira, M. T. (2012). Does longitudinal connectivity loss affect the distribution of freshwater fish? Ecol. Eng. 48, 70–78. doi:10.1016/J.ECOLENG.2011.05.008
Britton, J. R., and Pegg, J. (2011). Ecology of European barbel Barbus barbus: Implications for river, fishery, and conservation management. Rev. Fish. Sci. 19, 321–330. doi:10.1080/10641262.2011.599886
Cheng, F., Li, W., Castello, L., Murphy, B. R., and Xie, S. (2015). Potential effects of dam cascade on fish: Lessons from the Yangtze river. Rev. Fish. Biol. Fish. 25, 569–585. doi:10.1007/S11160-015-9395-9
Cohen, J. (1988). Statistical power analysis for the behavioral sciences. Hillsdale: L. Erlbaum Associates.
Côté, I. M., Darling, E. S., and Brown, C. J. (2016). Interactions among ecosystem stressors and their importance in conservation. Proc. R. Soc. B 283, 20152592. doi:10.1098/rspb.2015.2592
Darwall, W., Bremerich, V., Wever, A. D., Dell, A. I., Freyhof, J., Gessner, M. O., et al. (2018). The Alliance for Freshwater Life: A global call to unite efforts for freshwater biodiversity science and conservation. Aquat. Conserv. 28, 1015–1022. doi:10.1002/AQC.2958
De Leeuw, J. J., and Winter, H. V. (2008). Migration of rheophilic fish in the large lowland rivers Meuse and Rhine, The Netherlands. Fish. Manag. Ecol. 15, 409–415. doi:10.1111/j.1365-2400.2008.00626.x
DeLury, D. B. (1947). On the estimation of biological populations. Biometrics 3, 145–164. doi:10.2307/3001390
Düregger, A., Pander, J., Palt, M., Müller, M., Nagel, C., and Geist, J. (2018). The importance of stream interstitial conditions for the early-life-stage development of the European nase (Chondrostoma nasus L.). Ecol. Freshw. Fish. 27, 920–932. doi:10.1111/eff.12403
Führer, S., Haslauer, M., Greimel, F., Zeiringer, B., and Unfer, G. (2017). Machbarkeitsstudie mittlere Enns. Ennsabschnitt Gesäuseausgang bis Steyrmündung. Vienna: University of Natural Ressources and Life Sciences.
Führer, S., Hayes, D. S., Hasler, T., Graf, D., Fauchery, E., Mameri, D., et al. (2022). Stranding of larval nase (Chondrostoma nasus L.) depending on bank slope, down-ramping rate and daytime. Front. Environ. Sci. 10. doi:10.3389/fenvs.2022.966418
Haidvogl, G., Pont, D., Dolak, H., and Hohensinner, S. (2015). Long-term evolution of fish communities in European mountainous rivers: Past log driving effects, river management and species introduction (Salzach River, Danube). Aquat. Sci. 77, 395–410. doi:10.1007/S00027-015-0398-3
Haunschmid, R., Honsig-Erlenburg, W., Petz-Glechner, R., Schmutz, S., Schotzko, N., Spindler, T., et al. (2006). Methodik – Handbuch, Fischbestandsaufnahmen in Fließgewässern. Scharfling: Institut für Gewässerökologie.
Havn, T. B., Thorstad, E. B., Teichert, M. A. K., Sæther, S. A., Heermann, L., Hedger, R. D., et al. (2018). Hydropower-related mortality and behaviour of Atlantic salmon smolts in the River Sieg, a German tributary to the Rhine. Hydrobiologia 805, 273–290. doi:10.1007/s10750-017-3311-3
Hayes, D. S., Brändle, J. M., Seliger, C., Zeiringer, B., Ferreira, T., and Schmutz, S. (2018). Advancing towards functional environmental flows for temperate floodplain rivers. Sci. Total Environ. 633, 1089–1104. doi:10.1016/j.scitotenv.2018.03.221
Hayes, D. S., Lautsch, E., Unfer, G., Greimel, F., Zeiringer, B., Höller, N., et al. (2021). Response of European grayling, Thymallus thymallus, to multiple stressors in hydropeaking rivers. J. Environ. Manage. 292, 112737. doi:10.1016/j.jenvman.2021.112737
Hayes, D. S., Moreira, M., Boavida, I., Haslauer, M., Unfer, G., Zeiringer, B., et al. (2019). Life stage-specific hydropeaking flow rules. Sustainability 11, 1547. doi:10.3390/su11061547
Hayes, D. S., Schülting, L., Carolli, M., Greimel, F., Batalla, R. J., and Casas-Mulet, R. (2022). “Hydropeaking: Processes, effects, and mitigation,” in Encyclopedia of inland waters. Editors T. Mehner, and K. Tockner (Amsterdam, Netherlands: Elsevier), 134–149. doi:10.1016/B978-0-12-819166-8.00171-7
Huet, M. (1959). Profiles and biology of western European streams as related to fish management. Trans. Am. Fish. Soc. 88, 155–163. doi:10.1577/1548-8659(1959)88[155:pabowe]2.0.co;2
Jurajda, P. (1995). Effect of channelization and regulation on fish recruitment in a flood plain river. Regul. Rivers Res. Mgmt. 10, 207–215. doi:10.1002/rrr.3450100215
Kottelat, M., and Freyhof, J. (2007). Handbook of European freshwater fishes. Delémont, Switzerland: Cornol Publications Kottelat.
Mattocks, S., Hall, C. J., and Jordaan, A. (2017). Damming, lost connectivity, and the historical role of anadromous fish in freshwater ecosystem dynamics. Bioscience 67, 713–728. doi:10.1093/BIOSCI/BIX069
Melcher, A. H., and Schmutz, S. (2010). The importance of structural features for spawning habitat of nase Chondrostoma nasus (L.) and barbel Barbus barbus (L.) in a pre-Alpine river. River Syst. 19, 33–42. doi:10.1127/1868-5749/2010/019-0033
Muhar, S., Schmutz, S., and Jungwirth, M. (1995). River restoration concepts - goals and perspectives. Hydrobiologia 303, 183–194. doi:10.1007/bf00034055
Muhar, S., Seliger, C., Schinegger, R., Scheikl, S., Brändle, J., Hayes, D. S., et al. (2019). “Status and protection of rivers. A pan-Alpine overview,” in Rivers of the alps. Diversity in nature and culture. Editors S. Muhar, A. Muhar, G. Egger, and D. Siegrist (Berne: Haupt), 302–319.
Müller, M., Bierschenk, A. M., Bierschenk, B. M., Pander, J., and Geist, J. (2020). Effects of multiple stressors on the distribution of fish communities in 203 headwater streams of Rhine, Elbe and Danube. Sci. Total Environ. 703, 134523. doi:10.1016/j.scitotenv.2019.134523
Ovidio, M., Parkinson, D., Philippart, J. C., and Baras, E. (2007). Multiyear homing and fidelity to residence areas by individual barbel (Barbus barbus). Belg. J. Zool. 137, 183–190.
Palmer, M. A., Bernhardt, E. S., Allan, J. D., Lake, P. S., Alexander, G., Brooks, S., et al. (2005). Standards for ecologically successful river restoration. J. Appl. Ecol. 42, 208–217. doi:10.1111/j.1365-2664.2005.01004.x
Panchan, R., Pinter, K., Schmutz, S., and Unfer, G. (accepted). Seasonal migration and habitat use of adult barbel (Barbus barbus) and nase (Chondrostoma nasus) along a river section of the Austrian Danube River. Environ. Biol. Fishes.
Pelz, D. (2022). Characterization of spawning habitat and spawning ecology of three rheophilic fish species Chondrostoma nasus Barbus barbus and Vimba vimba at the river Traisen, Austria.
Peňáz, M. (1996). “Chondrostoma nasus - its reproduction strategy and possible reasons for a widely observed population decline - a review,” in Conservation of endangered freshwater fish in Europe. Editors A. Kirchhofer, and D. Hefti (Basel: Birkhäuser Basel), 279–285. doi:10.1007/978-3-0348-9014-4_27
Povž, M. (1988). Migrations of the nase carps (Chondrostoma nasus l. 1758) in the river Sava. J. Aquat. Prod. 2, 149–163.
Schiemer, F., Keckeis, H., and Kamler, E. (2002). The early life history stages of riverine fish: Ecophysiological and environmental bottlenecks. Comp. Biochem. Physiology Part A Mol. Integr. Physiology 133, 439–449. doi:10.1016/S1095-6433(02)00246-5
Schinegger, R., Palt, M., Segurado, P., and Schmutz, S. (2016). Untangling the effects of multiple human stressors and their impacts on fish assemblages in European running waters. Sci. Total Environ. 573, 1079–1088. doi:10.1016/j.scitotenv.2016.08.143
Schmutz, S., Bakken, T. H., Friedrich, T., Greimel, F., Harby, A., Jungwirth, M., et al. (2015). Response of fish communities to hydrological and morphological alterations in hydropeaking rivers of Austria. River Res. Appl. 31, 919–930. doi:10.1002/rra.2795
Schmutz, S., Kaufmann, M., Vogel, B., Jungwirth, M., and Muhar, S. (2000). A multi-level concept for fish-based, river-type-specific assessment of ecological integrity. Hydrobiologia 422/423, 279–289. doi:10.1023/a:1017038820390
Schmutz, S., and Moog, O. (2018). “Dams: Ecological impacts and management,” in Riverine ecosystem management. Editors S. Schmutz, and J. Sendzimir (Cham, Switzerland: Springer Open), 111–127. doi:10.1007/978-3-319-73250-3_6
Schmutz, S., Zauner, G., Eberstaller, J., and Jungwirth, M. (2001). Die “Streifenbefischungsmethode”: Eine Methode zur Quantifizierung von Fischbeständen mittelgroßer Fließgewässer. Österreichs Fisch. 54, 14–27.
Seber, G. A. F., and Le Cren, E. D. (1967). Estimating population parameters from catches large relative to the population. J. Anim. Ecol. 36, 631–643. doi:10.2307/2818
Steinmann, P., Koch, W., and Scheuring, L. (1937). Die Wanderungen unserer Süßwasserfische dargestellt auf Grund von Markierungsversuchen. Z. F. Fisch. U. D. Hilfswiss. 35, 369–467.
Unfer, G., and Rauch, P. (2019). Fischschutz und Fischabstieg in Österreich - Teilbericht 2, Fallstudie Thaya. Vienna, Austria.
Wolfram, G., and Mikschi, E. (2007). “Rote Liste der Fische (pisces) Österreichs,” in Rote Listen gefährdeter Tiere Ö̈sterreichs Teil 2. Editor K. P. Zulka (Vienna, Austria: Bundesministerium für Land- und Forstwirtschaft), 61–198.
Keywords: hydromorphology, Cyprinidae, hydropower, dam, fragmentation, impoundment
Citation: Hayes DS, Schaufler G, Schmutz S, Unfer G, Führer S, Auer S and Seliger C (2022) Hydro-morphological stressors reduce distribution range and affect population status of cyprinid fishes in Austria. Front. Environ. Sci. 10:991722. doi: 10.3389/fenvs.2022.991722
Received: 11 July 2022; Accepted: 24 August 2022;
Published: 03 October 2022.
Edited by:
Rosana Mazzoni, Rio de Janeiro State University, BrazilReviewed by:
Vineet Dubey, Wildlife Institute of India, IndiaGao Jian, Hubei University of Technology, China
Copyright © 2022 Hayes, Schaufler, Schmutz, Unfer, Führer, Auer and Seliger. This is an open-access article distributed under the terms of the Creative Commons Attribution License (CC BY). The use, distribution or reproduction in other forums is permitted, provided the original author(s) and the copyright owner(s) are credited and that the original publication in this journal is cited, in accordance with accepted academic practice. No use, distribution or reproduction is permitted which does not comply with these terms.
*Correspondence: Daniel S. Hayes, daniel.hayes@boku.ac.at
†These authors have contributed equally to this work